- 1Transversal Translational Medicine, Luxembourg Institute of Health, Strassen, Luxembourg
- 2Faculty of Science, Technology and Medicine, University of Luxembourg, Esch-sur-Alzette, Luxembourg
- 3Luxembourg Centre for Systems Biomedicine, University of Luxembourg, Esch-sur-Alzette, Luxembourg
- 4Parkinson Research Clinic, Centre Hospitalier de Luxembourg, Strassen, Luxembourg
- 5Department of Social Sciences, Institute for Research on Socio-Economic Inequality, University of Luxembourg, Esch-sur-Alzette, Luxembourg
Background: Freezing of gait (FOG), is associated with impairment of different cognitive functions. Previous studies hypothesized that FOG may be due to a loss of automaticity.
Research question: To explore whether FOG is associated with impairment in cognitive functions, focusing on retrograde procedural memory, the memory responsible for the automatic, implicit stored procedures that have been acquired in earlier life stages.
Methods: In this cross-sectional, case–control study, 288 people with typical Parkinson’s disease (PD) from the Luxembourg Parkinson’s Study were assigned to Freezers (FOG+) and non-Freezers (FOG−) based on the MDS-UPDRS 2.13 (self-reported FOG episodes) and 3.11 (FOG evaluated by clinicians during gait assessment). Both groups were matched on age, sex and disease duration. Global cognition (MoCA), retrograde procedural memory and visuo-constructive abilities (CUPRO), psychomotor speed and mental flexibility (TMT) were assessed. Furthermore, we repeated our analyses by additionally controlling for depression (BDI-I).
Results: Besides lower global cognition (MoCA; p = 0.007) and mental flexibility (TMT-B and Delta-TMT; p < 0.001), FOG+ showed a lower performance in retrograde procedural memory (CUPRO-IS1; p < 0.001) compared to FOG−. After controlling additionally for depression, our main outcome variable CUPRO-IS1 remained significantly lower in FOG+ (p = 0.010).
Conclusion: Our findings demonstrated that besides lower global cognition and mental flexibility scores, FOG+ showed lower performance in retrograde procedural memory compared to matched FOG-control patients, even when accounting for factors such as age, sex, disease duration or depression.
Significance: In the context of limited treatment options, especially for non-invasive therapeutic approaches, these insights on procedural memory and FOG may lead to new hypotheses on FOG etiology and consequently the development of new treatment options.
1 Introduction
Freezing of gait (FOG) is an abnormal gait pattern, defined by brief, temporary episodes of difficulty or even inability to move the feet despite the intention to walk (Nutt et al., 2011). FOG is common in people with Parkinson’s disease (PD), affecting approximately 38% in early disease stages and up to 65% in advanced disease stages (Zhang et al., 2021). By reducing quality of life and independence, FOG poses a substantial burden for patients and caregivers (Perez-Lloret et al., 2014). Besides a proven link with disease progression (Macht et al., 2007), additional symptoms like impaired cognition are observed in People with PD (PwPD) with FOG (FOG+) (Peterson et al., 2016).
Early findings indicated that gait is controlled by the central pattern generators in the spinal cord and brain stem. Even though these brain areas are highly implicated in locomotion, recent evidence from behavioral and imaging studies demonstrated the implication of higher-level cortical structures in gait, highlighting the importance of cognitive function in the process (Peterson et al., 2016). Nevertheless, studies related to this topic are still controversial. Some studies suggested impaired cognition, most notably in executive and visuospatial functions in FOG+ (Amboni et al., 2007; Cohen et al., 2014; Jha et al., 2015; Peterson et al., 2016; Gan et al., 2023). Others provided no evidence for differences in cognition (Morris et al., 2020; Taximaimaiti and Wang, 2021). These divergences could be due to the heterogeneity in their study populations (e.g., age, education), covariates (e.g., disease severity and medication), varying definitions of FOG, different applied neuropsychological assessments or to the fact that cognitive functions more directly associated with FOG have not been tested yet.
Despite that FOG in PD has been characterized as a de-automatization deficit (Hallett, 2008; Nutt et al., 2011; Heremans et al., 2013; Vandenbossche et al., 2013b), little is known about the relation between FOG and procedural memory. In PD, movements become less automatic, mainly due to the loss of dopaminergic input to the striatum, a brain area that plays an essential role in procedural skills, such as walking (Lehéricy et al., 2005; Doyon et al., 2009). Therefore, these skills require more attentional control in PD, relying on a shift in neural activation from sub-cortical, implicit and automatic behaviour to cortical brain areas, explicit and goal-directed behavior, as a compensation strategy (Vandenbossche et al., 2013b; Wu et al., 2015).
As procedural memory is often imprecisely defined, and we have judged Crystal’s and colleagues’ definition, which divides procedural memory into anterograde and retrograde components, as the most accurate, we are applying this terminology in our study (Crystal et al., 1989). Anterograde procedural memory involves the acquisition of new skills, while the ability to execute skills acquired in earlier life stages is part of retrograde procedural memory. An affected anterograde procedural memory demonstrates difficulties with the ability to learn new skills, by repetition. An affected retrograde procedural memory demonstrates difficulties in recalling and executing learned procedural skills that had once reached automatization. Despite numerous studies on procedural memory in PD, the conclusions are inconsistent. Studies on procedural memory in PD investigated mainly the anterograde procedural memory. While most of these studies have described an impairment of the learning of new procedural skills (Frith et al., 1986; Saint-Cyr et al., 1988; Heindel et al., 1989; Ferraro et al., 1993; Jackson et al., 1995; Roncacci et al., 1996; Westwater et al., 1998; Krebs et al., 2001; Sarazin et al., 2002; Muslimović et al., 2007; Vandenbossche et al., 2013a; Vakil et al., 2021) others did not observe any significant differences (Agostino, 1996; Seidler et al., 2007; Beauchamp et al., 2008; Pendt et al., 2011; Panouillères et al., 2016) or only mild to moderate or partial impairments (Harrington et al., 1990; Pascual-Leone et al., 1993; Allain et al., 1995; Thomas-Antérion et al., 1996; Sommer et al., 1999). Even though, the unique characteristic of procedural memory is its robustness in time, and its longevity, only a limited number of studies investigated retrograde procedural memory (Mochizuki-Kawai et al., 2004; Cohen and Pourcher, 2007; Heremans et al., 2016). Assessing procedural memory still encounters difficulties on both levels, theoretical and clinical (Van der Linden and Seron, 2014). This might be explained by the not fully understood theoretical background and the lack of easy-to-apply assessments. Specific impairments in procedural skills, like handwriting (Heremans et al., 2016), and in the acquiring new procedural skills (Vandenbossche et al., 2013a) in FOG+ compared to a control group without FOG (FOG−), have been documented. However, to our knowledge, apart from the previously mentioned handwriting studies, retrograde procedural memory has not been systematically assessed in FOG+. We recently provided the extended evaluation system of the Cube Copying Task, the CUPRO (short for CUbe drawing PROcedure) to assess this memory concept and demonstrated impairments in PwPD compared to matched control subjects (Pauly et al., 2022). We hypothesized that the Cube Copying Task meets the conditions of assessing retrograde procedural memory: by copying the Cube, a (i) previously acquireded procedureis (ii) unconciously applied. Through assessing discriminant validity with several tests representing related constructs, no evidence was found indicating that general motor symptoms prevalent in PD as well as deficits in visuo-cognition, planning or executive functions might interfere with the Cube coping performance (Pauly et al., 2022). Aiming to investigate the relationship between cognition and FOG, with a focus on retrograde procedural memory, we hypothesized that this memory deficit, already observed in PD (Pauly et al., 2022), may be more prominent among FOG+ compared to matched FOG−. In addition to procedural memory, we assessed global cognition, visuo-constructive function, psychomotor speed and mental flexibility.
Despite that FOG is one of the main causes of falls and reduced quality of life, knowledge of treatment options, especially for non-invasive therapeutic approaches, is limited (Perez-Lloret et al., 2014; Walton et al., 2015). Previous studies demonstrated improved FOG symptoms in PwPD after cognitive rehabilitative training that may lead to neuroplastic changes by reinforcing cognitive strategies (Walton et al., 2018). Therefore, insights on specific cognitive impairment patterns, such as on procedural memory in PwPD and FOG may lead to a better understanding of the etiology of FOG. Consequently, a better characterization of the cognitive impairments observed in PD may support the targeted development of cognitive rehabilitation training and reeducation therapies, aiming to maintain, or even reinforce cognitive function and indirectly improve the quality of life of PwPD.
2 Materials and methods
2.1 Participants
Participants were recruited from the Luxembourg Parkinson’s Study of the National Centre of Excellence in Research on PD (NCER-PD) (Hipp et al., 2018) and provided informed consent according to the Declaration of Helsinki. Inclusion criteria were the age of 18 years or older and the ability to sign the consent. We excluded participants with Deep Brain Stimulation, with a Montreal Cognitive Assessment (MoCA) score below 21 or having been diagnosed with PD with dementia (Dubois et al., 2007), atypical forms of parkinsonism, other neurological or severe psychiatric disorders. In the present study, 288 participants with typical PD were selected and two groups were defined differing in FOG status (FOG+ = 144; FOG− = 144) propensity score matched on age, sex and disease duration. The diagnosis was based on the UK PD Society Brain Bank Clinical Diagnostic Criteria (Hughes et al., 1992). Each subject underwent a neurological examination and provided information on early symptoms, disease history and current treatment. Patients were tested while being on their regular medication. Levodopa Equivalent Daily Dose (LEDD) was calculated for each participant (Tomlinson et al., 2010). The Movement Disorder Society - Unified Parkinson’s Disease Rating Scale (MDS-UPDRS) (Goetz et al., 2007) and the modified Hoehn and Yahr scale (Goetz et al., 2004) were used to assess motor symptoms and disease stages.
2.2 FOG evaluation
Current and past FOG symptoms were explored using information (i) on self-reported FOG episodes (MDS-UPDRS part II sub-item 2.13), and (ii) on FOG symptoms reported by the specialized neurologist during gait assessment (MDS-UPDRS part III sub-item 3.11). Participants were categorized into two groups; the FOG+ group with participants reporting or presenting FOG episodes (MDS-UPDRS 2.13 or MDS-UPDRS 3.11 score range 1–4) in at least one of their visits at the research clinic and the FOG-group without FOG symptoms (MDS-UPDRS 2.13 = 0 and MDS-UPDRS 3.11 = 0).
A detailed flowchart can be found in the Supplementary Figure S1.
2.3 Neuropsychological assessment
With the CUPRO evaluation system, we assessed our main outcome variable, the Cube copying procedure (Intermediate Score 1 – IS1), representing retrograde procedural memory and the final result of the Cube (Intermediate Score 2 – IS2), representing visuo-constructive functions (Pauly et al., 2022).
The CUPRO is an extended evaluation score for the Cube Copying Task, that was initially evaluated, with the classical scoring system established by Nasreddine et al. (2005). Following the classical scoring system, one point was administered for a correct final result, assessing visuo-constructive functions: the drawing must be three-dimensional, the orientation of the drawing must be correct, the final result must be correct and the point was not given if any of these criteria were not met. We extended upon this scoring system to separately assess its three-dimensionality (1 point), the accuracy of its orientation (1 point), and the correctness of the final result (1 point) [Intermediate Score 2 (IS2)]. Subsequently, the Cube Copying Task was further extended to additionally evaluate the copying procedure itself. Based on the four, previously defined typical procedures, the extended scoring system evaluates the starting approach; 1 point is given if the subject started with one of the squares, surfaces, or the three axes. Further, the procedure itself is evaluated on 1 point (A.-D.). The final point is administered if the subject completes the copying procedure, by connecting the lines [Intermediate Score1 (IS1)]. To summarize, the total score of six points of the CUPRO evaluation system is composed of two intermediate scores. The first intermediate score of three points (IS1) evaluates the copying procedure. The second intermediate score (IS2) of three points allows us to infer aspects related to visuo-constructive functions. A detailed description of the development of the CUPRO can be found elsewhere (Pauly et al., 2022).
In addition to retrograde procedural memory, global cognition was evaluated with the MoCA (Nasreddine et al., 2005). Psychomotor speed and mental flexibility were measured with the Trail-Making-Test (TMT) part A and part B, respectively (Godefroy, 2008). Delta TMT is defined as (TMT-B) – (TMT-A).
2.4 Questionnaires
The Beck Depression Inventory (BDI-I) (Beck et al., 1961), Starkstein Apathy Scale (SAS) (Starkstein et al., 1992), PD Questionnaire (PDQ-39) (Peto et al., 1995), and the MDS-UPDRS (I&II) (Goetz et al., 2007), were used to assess the presence of depression symptomatology, apathy, quality of life, and non-motor and motor aspects of experiences of daily living, respectively. Functional Activity Questionnaire (FAQ) (Pfeffer et al., 1982) and the Short Informant Questionnaire on Cognitive Decline in the Elderly (IQCODE) (Jorm, 1994) were used to measure functional activity and cognitive decline reported by relatives.
For all the questionnaires, we investigate the summed item scores, except for the PDQ-39 we investigate the summary index, derived by the sum of all 39 items’ responses as a percentage score (Jenkinson et al., 1997).
2.5 Genetical testing
Targeted Glucocerebrosidase (GBA) screening was performed by real-time single-molecule sequencing developed by Pacific BioScience (PacBio). More details about the GBA screening have been reported elsewhere (Pachchek et al., 2023). In the present study, we define the carriers of a known pathogenic mutation in the GBA gene as GBA+ and the non-carriers as GBA−.
2.6 Statistics
The two groups were matched by age, sex and disease duration by propensity score matching (matching tolerance = 0.05). Differences in demographic and clinical characteristics as well as cognitive performance were analyzed using the non-parametric Mann–Whitney U test and Pearson’s chi-squared test (two-tailed). Correlations were tested with the bivariate Spearman correlation test. The significance threshold was set at p ≤ 0.05. Where appropriate, we used the Bonferroni correction for multiple testing to prevent alpha error inflation. The same statistical analyses were repeated for the two groups matched additionally for depression (Supplementary material). All statistical analyses were performed using R version 4.2.0 GUI 1.78 and RStudio version 2023.03.1 + 446.
3 Results
Testing for sociodemographic differences between the groups confirmed successful matching, as the groups did not differ significantly in sex [χ2(1, N = 288) = 0.06, p = 0.802], age (u = 10,379, p = 0.988), or disease duration (u = 11,740, p = 0.677). After multiple testing corrections, FOG+ presented significantly higher scores for MDS-UPDRS-I (u = 6116.5, p < 0.001), II (u = 4955.5, p < 0.001) and III (u = 7853.5, p < 0.001), BDI-I (u = 6,418, p < 0.001), SAS (u = 6,627, p < 0.001), FAQ (u = 5,483, p < 0.001) and PDQ-39 (u = 4,820, p < 0.001). FOG+ presented nominally significantly higher scores for the short IQCODE (u = 6564.5, p = 0.039). FOG+ present shorter education duration and higher LEDD than FOG−, but these differences are insignificant (u = 11,740, p = 0.051; u = 7,304, p = 0.059 respectively). Similarly, the number of languages is not significantly different. Given that heterozygous GBA gene mutation carriers (severe, mild and low-risk pathogenic mutations) represent increased susceptibility for PD, gait impairment and cognitive dysfunction (Wang et al., 2014), we tested group differences. No significant difference was observed regarding the number of GBA carriers between the groups [χ2(1, N = 259) = 0.89, p = 0.583]. Descriptive statistics on the demographic and clinical data can be found in Table 1.
Our outcome variable of interest, CUPRO-IS1, was significantly lower in the FOG+ compared to FOG− (u = 12,651, p < 0.001). FOG+ presented significantly lower MoCA total scores (u = 12264.5, p = 0.007), as well as significantly higher TMT-A and TMT-B time scores (u = 8047.5, p = 0.021; u = 7,089, p < 0.001 respectively) and Delta TMT (u = 7,135, p < 0.001) compared to FOG−. No significant differences were observed for the CUPRO-IS2. Differences in neuropsychological measures between the two groups can be found in Table 2. No significant correlation was observed between the CUPRO scores and the FOG severity (MDS-UPDRS 2.13 /3.11). Results on the Spearman Correlations for the CUPRO scores in the FOG+ group can be found in Table 3.
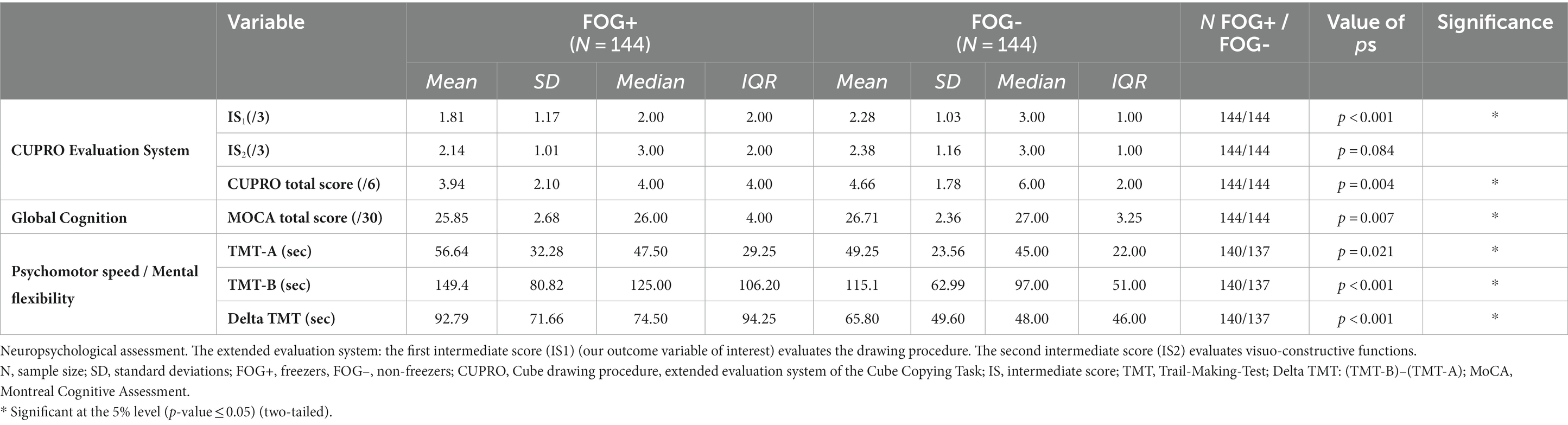
Table 2. Differences in neuropsychological measures between FOG+ (N = 144) and FOG- (N = 144) matched on age, sex and disease duration.
Given that depression can have an important impact on cognition, we repeated our analyses by additionally controlling for depression. After controlling additionally for this variable, our main outcome variable CUPRO-IS1 (u = 8,211, p = 0.010), as well as the variables for the TMT (TMTa: u = 5123.5, p = 0.006; TMTb: u = 5099.5, p = 0.005; Delta TMT: u = 5,418, p = 0.030) remained significantly lower in FOG+. Findings on the additional analyses can be found in Supplementary Tables S1, S2.
4 Discussion
The present study aimed to investigate if Freezing of gait (FOG) in Parkinson’s disease (PD) is associated with impairment in retrograde procedural memory. For this purpose, we used the CUPRO assessment tool (Pauly et al., 2022) and compared performance in FOG+ and FOG−, matched on age, sex and disease duration. The present study demonstrates significantly lower scores representing the performance in retrograde procedural memory in FOG+, even when matched for age, sex and disease duration to the control group. Similar observations on procedural learning have been described in FOG+ (Vandenbossche et al., 2013a).
Furthermore, we tested for differences in other cognitive domains. Previous studies have suggested cognitive deficits in FOG+ (Vandenbossche et al., 2013a; Cohen et al., 2014; Jha et al., 2015; Heremans et al., 2016), but only a few found no differences in cognition (Morris et al., 2020). These discrepancies can be explained by the varying definitions of FOG and non-controlled covariates. Our results support previous studies which demonstrated impaired psychomotor speed, procedural skills (Vandenbossche et al., 2013a; Heremans et al., 2016) and executive functions (Amboni et al., 2007; Peterson et al., 2016), such as mental flexibility in FOG+ compared to FOG−. We did not see any significant differences for visuo-constructive functions. Nevertheless, we need to keep in mind that a small test battery was used. To validate the findings, future research should apply a larger neuropsychological test battery.
Our findings of impaired procedural memory and mental flexibility, part of the executive functions, support the Vandenbossche model (Vandenbossche et al., 2013b). The model hypothesizes that those two functions, regulated by the frontostriatal circuitry, are crucial for understanding the pathogenesis of FOG. In case of disturbances of automaticity/procedural memory, one would expect a shift in neural activation from sub-cortical to cortical brain areas as a compensation strategy. In case of additional impairment of executive functions, this could lead to a FOG episode (Vandenbossche et al., 2013b). Recent brain imaging studies support this finding by describing increased involvement of attention as a compensatory strategy in PD compared to control subjects after motor learning (Wu et al., 2015). Functional neuroimaging studies suggested that FOG in PD is caused by abnormal interactions between frontoparietal cortical and subcortical structures, such as the striatum (Shine et al., 2013). This is in line with our observation of impaired retrograde procedural memory in FOG+, as the basal ganglia, especially the dorsolateral striatum, play an essential role in procedural memory (Mishkin and Appenzeller, 1987).
Measured by the absence of significant correlations, neither, global cognition, mental flexibility, nor retrograde procedural memory, were affected more severely by the worsening of the FOG symptoms. This previously mentioned shift might therefore not be gradual, defined by a temporal gradient but more by a spontaneous shift.
Furthermore, our findings that FOG+ show significantly more non-motor and motor symptoms, lower quality of life and higher disease stages compared to their matched control group, are in line with previous findings (Perez-Lloret et al., 2014). Given that depression can have an important impact on cognition, we repeated our analyses by additionally controlling for depression. The results for the main outcome variable, retrograde procedural memory, remained significantly lower in FOG+. This is in line with our observations made in a previous study comparing retrograde procedural memory in PwPD and control subjects, where we did not find significant associations between depression symptomatology and retrograde procedural memory (Pauly et al., 2022).
We took into consideration recently published recommendations for studies on cognition and FOG in PD (Monaghan et al., 2023); First, we ensured that the FOG cohorts were well characterized for clinical demographics including age, sex, education and, what is often neglected, for disease duration. Second, we reported medication status, by calculating the Levodopa Equivalent Daily Dose (LEDD) for each participant, so that the impact of dopamine medication can be interpreted. In the current study, we did not see any significant difference in LEDD between both groups. Third, apart from the novel CUPRO evaluation system, we used validated neuropsychological assessment tools and questionnaires to facilitate future comparisons across studies. The present study has the advantage that we included people with current and initial FOG symptoms. Given that dopaminergic medication can have a positive impact on gait abnormalities (Giladi, 2008), a medically well-adjusted patient may have his FOG masked.
Although the differences in dopaminergic medication were not statistically significant, they may still influence our outcome variables, as dopaminergic treatments can potentially shape the neural connectivity of cognitive networks in PD (Aracil-Bolaños et al., 2021). Since no significant correlation between retrograde procedural memory and LEDD have been observed in our previous study (Pauly et al., 2022), we do not anticipate a substantial impact on retrograde procedural memory. Furthermore, despite the fact that the years of education did not significantly differ between both groups, we cannot rule out the possibility that it might have an impact on our outcome variable, considering that previous findings have shown that the number of years of education completed is positively correlated with their cognitive functions (Lövdén et al., 2020).
Even though FOG is one of the main causes of falls and reduced quality of life, knowledge of treatment options, especially for non-invasive therapeutic approaches, is limited. Therefore, getting a deeper understanding of the relation between the pathophysiology of FOG and cognitive functions such as retrograde procedural memory is important, as these insights can lead to new hypotheses on the etiology of FOG. Previous findings demonstrated that cognitive rehabilitative training improves FOG symptoms in PwPD, leading to neuroplastic changes by reinforcing cognitive strategies (Walton et al., 2018). Research developing cognitive rehabilitation training reinforcing cognitive compensation strategies in people with FOG may have the potential to improve the quality of life of FOG patients.
The present study aimed to investigate if Freezing of Gait (FOG) in Parkinson’s disease (PD) is associated with impairment in retrograde procedural memory. By comparing retrograde procedural memory performance in FOG+ and FOG−, measured by the CUPRO assessment, we observed significantly lower CUPRO-IS1 scores, suggestive of impaired retrograde procedural memory, in FOG+, even when accounting for possible confounding factors such as age, sex, disease duration or depression.
Although FOG is a significant contributor to falls and a decline in the quality of life, our knowledge of treatment options, particularly non-invasive therapeutic methods, is still limited. Therefore, gaining insights into specific patterns of cognitive impairment, such as procedural memory in PwPD and FOG, and its suggested relationships with other cognitive domains in other studies, may improve our understanding of FOG’s causes. Consequently, a more thorough understanding of the cognitive deficits observed in PD may facilitate the targeted development of cognitive rehabilitation training and reeducation therapies. These efforts aim to preserve or even enhance cognitive function, ultimately leading to an improvement in the quality of life for individuals with PD.
Data availability statement
The original contributions presented in the study are included in the article/Supplementary material, further inquiries can be directed to the corresponding authors.
Ethics statement
All participants taking part in the Luxembourg Parkinson’s Study agreed and signed a written informed consent. The study was conducted in accordance with the local legislation and institutional requirements and has obtained a positive opinion from the National Research Ethics Committee (CNER Ref: 201407/13).
Author contributions
LP: Conceptualization, Data curation, Formal analysis, Investigation, Methodology, Project administration, Visualization, Writing – original draft, Writing – review & editing. CP: Conceptualization, Data curation, Investigation, Methodology, Project administration, Writing – review & editing. MH: Conceptualization, Data curation, Investigation, Methodology, Project administration, Writing – review & editing. VS: Conceptualization, Data curation, Writing – review & editing. AR: Conceptualization, Data curation, Formal analysis, Investigation, Writing – review & editing. AL: Conceptualization, Data curation, Formal analysis, Investigation, Methodology, Project administration, Supervision, Writing – review & editing. RK: Conceptualization, Data curation, Formal analysis, Funding acquisition, Investigation, Methodology, Project administration, Resources, Supervision, Writing – review & editing.
Funding
The author(s) declare financial support was received for the research, authorship, and/or publication of this article. The National Centre of Excellence in Research on Parkinson’s Disease (NCER-PD) is funded by the Luxembourg National Research Fund (FNR/NCER13/BM/11264123).
Acknowledgments
We would like to thank all participants of the Luxembourg Parkinson’s Study for their important support of our research.
We acknowledge the joint effort of the National Centre of Excellence in Research on Parkinson’s Disease (NCER-PD) Consortium members from the partner institutions Luxembourg Centre for Systems Biomedicine, Luxembourg Institute of Health, Centre Hospitalier de Luxembourg, and Laboratoire National de Santé generally contributing to the Luxembourg Parkinson’s Study as listed below: Geeta ACHARYA 2, Gloria AGUAYO 2, Myriam ALEXANDRE 2, Muhammad ALI 1, Wim AMMERLANN 2, Giuseppe ARENA 1, Rudi BALLING 1, Michele BASSIS 1, Roxane BATUTU 3, Katy BEAUMONT 2, Regina BECKER 1, Camille BELLORA 2, Guy BERCHEM 3, Daniela BERG 11, Alexandre BISDORFF 5, Ibrahim BOUSSAAD 1, David BOUVIER 4, Kathrin BROCKMANN 11, Jessica CALMES 2, Lorieza CASTILLO 2, Gessica CONTESOTTO 2, Nancy DE BREMAEKER 3, Nico DIEDERICH 3, Rene DONDELINGER 5, Nancy E. RAMIA 1, Daniela ESTEVES 2, Guy FAGHERAZZI 2, Jean-Yves FERRAND 2, Katrin FRAUENKNECHT 4, Manon GANTENBEIN 2, Thomas GASSER 11, Piotr GAWRON 1, Soumyabrata GHOSH 1, Marijus GIRAITIS 2,3, Enrico GLAAB 1, Martine GOERGEN 3, Elisa GÓMEZ DE LOPE 1, Jérôme GRAAS 2, Mariella GRAZIANO 17, Valentin GROUES 1, Anne GRÜNEWALD 1, Wei GU 1, Gaël HAMMOT 2, Anne-Marie HANFF 2, 20, 21, Linda HANSEN 1,3, Michael HENEKA 1, Estelle HENRY 2, Sylvia HERBRINK 6, Sascha HERZINGER 1, Michael HEYMANN 2, Michele HU 8, Alexander HUNDT 2, Nadine JACOBY 18, Jacek JAROSLAW LEBIODA 1, Yohan JAROSZ 1, Sonja JÓNSDÓTTIR 2, Quentin KLOPFENSTEIN 1, Jochen KLUCKEN 1,2,3, Rejko KRÜGER 1,2,3, Pauline LAMBERT 2, Zied LANDOULSI 1, Roseline LENTZ 7, Inga LIEPELT 11, Robert LISZKA 14, Laura LONGHINO 3, Victoria LORENTZ 2, Paula Cristina LUPU 2, Tainá M. MARQUES 1, Clare MACKAY 10, Walter MAETZLER 15, Katrin MARCUS 13, Guilherme MARQUES 2, Patricia MARTINS CONDE 1, Patrick MAY 1, Deborah MCINTYRE 2, Chouaib MEDIOUNI 2, Francoise MEISCH 1, Myriam MENSTER 2, Maura MINELLI 2, Michel MITTELBRONN 1,4, Brit MOLLENHAUER 12, Friedrich MÜHLSCHLEGEL 4, Romain NATI 3, Ulf NEHRBASS 2, Sarah NICKELS 1, Beatrice NICOLAI 3, Jean-Paul NICOLAY 19, Fozia NOOR 2, Marek OSTASZEWSKI 1, Clarissa P. C. GOMES 1, Sinthuja PACHCHEK 1, Claire PAULY 1,3, Laure PAULY 2, 20, Lukas PAVELKA 1,3, Magali PERQUIN 2, Rosalina RAMOS LIMA 2, Armin RAUSCHENBERGER 1, Rajesh RAWAL 1, Dheeraj REDDY BOBBILI 1, Kirsten ROOMP 1, Eduardo ROSALES 2, Isabel ROSETY 1, Estelle SANDT 2, Stefano SAPIENZA 1, Venkata SATAGOPAM 1, Margaux SCHMITT 2, Sabine SCHMITZ 1, Reinhard SCHNEIDER 1, Jens SCHWAMBORN 1, Raquel SEVERINO 2, Amir SHARIFY 2, Ekaterina SOBOLEVA 1, Kate SOKOLOWSKA 2, Hermann THIEN 2, Elodie THIRY 3, Rebecca TING JIIN LOO 1, Christophe TREFOIS 1, Johanna TROUET 2, Olena TSURKALENKO 2, Michel VAILLANT 2, Mesele VALENTI 2, Gilles VAN CUTSEM 1,3, Carlos VEGA 1, Liliana VILAS BOAS 3, Maharshi VYAS 1, Richard WADE-MARTINS 9, Paul WILMES 1, Evi WOLLSCHEID-LENGELING 1, Gelani ZELIMKHANOV 3. 1 Luxembourg Centre for Systems Biomedicine, University of Luxembourg, Esch-sur-Alzette, Luxembourg. 2 Luxembourg Institute of Health, Strassen, Luxembourg. 3 Centre Hospitalier de Luxembourg, Strassen, Luxembourg. 4 Laboratoire National de Santé, Dudelange, Luxembourg. 5 Centre Hospitalier Emile Mayrisch, Esch-sur-Alzette, Luxembourg. 6 Centre Hospitalier du Nord, Ettelbrück, Luxembourg. 7 Parkinson Luxembourg Association, Leudelange, Luxembourg. 8 Oxford Parkinson’s Disease Centre, Nuffield Department of Clinical Neurosciences, University of Oxford, Oxford, UK. 9 Oxford Parkinson’s Disease Centre, Department of Physiology, Anatomy and Genetics, University of Oxford, South Parks Road, Oxford, UK. 10 Oxford Centre for Human Brain Activity, Wellcome Centre for Integrative Neuroimaging, Department of Psychiatry, University of Oxford, Oxford, UK. 11 Center of Neurology and Hertie Institute for Clinical Brain Research, Department of Neurodegenerative Diseases, University Hospital Tübingen, Germany. 12 Paracelsus-Elena-Klinik, Kassel, Germany. 13 Ruhr-University of Bochum, Bochum, Germany. 14 Westpfalz-Klinikum GmbH, Kaiserslautern, Germany. 15 Department of Neurology, University Medical Center Schleswig-Holstein, Kiel, Germany. 16 Department of Neurology Philipps, University Marburg, Marburg, Germany. 17 Association of Physiotherapists in Parkinson’s Disease Europe, Esch-sur-Alzette, Luxembourg. 18 Private practice, Ettelbruck, Luxembourg. 19 Private practice, Luxembourg, Luxembourg. 20 Faculty of Science, Technology and Medicine, University of Luxembourg, Esch-sur-Alzette, Luxembourg. 21 Department of Epidemiology, CAPHRI School for Public Health and Primary Care, Maastricht University Medical Centre+, Maastricht, Netherlands.
Conflict of interest
The authors declare that the research was conducted in the absence of any commercial or financial relationships that could be construed as a potential conflict of interest.
Publisher’s note
All claims expressed in this article are solely those of the authors and do not necessarily represent those of their affiliated organizations, or those of the publisher, the editors and the reviewers. Any product that may be evaluated in this article, or claim that may be made by its manufacturer, is not guaranteed or endorsed by the publisher.
Supplementary material
The Supplementary material for this article can be found online at: https://www.frontiersin.org/articles/10.3389/fnagi.2023.1296323/full#supplementary-material
References
Agostino, R. (1996). Motor skill learning in Parkinson’s disease. J. Neurol. Sci. 139, 218–226. doi: 10.1016/0022-510X(96)00060-3
Allain, H., Lieury, A., Thomas, V., Reymann, J. M., Gandon, J. M., and Belliard, S. (1995). Explicit and procedural memory in Parkinson’s disease. Biomed. Pharmacother. 49, 179–186. doi: 10.1016/0753-3322(96)82618-7
Amboni, M., Cozzolino, A., Longo, K., Picillo, M., and Barone, P. (2007) Freezing of gait and executive functions in patients with Parkinson’s disease.
Aracil-Bolaños, I., Sampedro, F., Pujol, J., Soriano-Mas, C., Gónzalez-de-Echávarri, J. M., Kulisevsky, J., et al. (2021). The impact of dopaminergic treatment over cognitive networks in Parkinson’s disease: stemming the tide? Hum. Brain Mapp. 42, 5736–5746. doi: 10.1002/hbm.25650
Beauchamp, M. H., Dagher, A., Panisset, M., and Doyon, J. (2008). Neural substrates of cognitive skill learning in Parkinson’s disease. Brain Cogn. 68, 134–143. doi: 10.1016/j.bandc.2008.03.008
Beck, A. T., Ward, C. H., Mendelson, M., Mock, J., and Erbaugh, J. (1961). An inventory for measuring depression. Arch. Gen. Psychiatry 4, 561–571. doi: 10.1001/archpsyc.1961.01710120031004
Cohen, R. G., Klein, K. A., Nomura, M., Fleming, M., Mancini, M., Giladi, N., et al. (2014). Inhibition, executive function, and freezing of gait. J. Parkinsons Dis. 4, 111–122. doi: 10.3233/JPD-130221
Cohen, H., and Pourcher, E. (2007). Intact encoding, impaired consolidation in procedural learning in Parkinson’s disease. Exp. Brain Res. 179, 703–708. doi: 10.1007/s00221-006-0827-6
Crystal, H. A., Grober, E., and Masur, D. (1989). Preservation of musical memory in Alzheimer’s disease. J. Neurol. Neurosurg. Psychiatry 52, 1415–1416. doi: 10.1136/jnnp.52.12.1415
Doyon, J., Bellec, P., Amsel, R., Penhune, V., Monchi, O., Carrier, J., et al. (2009). Contributions of the basal ganglia and functionally related brain structures to motor learning. Behav. Brain Res. 199, 61–75. doi: 10.1016/j.bbr.2008.11.012
Dubois, B., Burn, D., Goetz, C., Aarsland, D., Brown, R. G., Broe, G. A., et al. (2007). Diagnostic procedures for Parkinson’s disease dementia: recommendations from the Movement Disorder Society task force. Mov. Disord. 22, 2314–2324. doi: 10.1002/mds.21844
Ferraro, F. R., Balota, D. A., and Connor, L. T. (1993). Implicit memory and the formation of new associations in nondemented parkinson′s disease individuals and individuals with senile dementia of the alzheimer type: a serial reaction time (SRT) investigation. Brain Cogn. 21, 163–180. doi: 10.1006/brcg.1993.1013
Frith, C. D., Bloxham, C. A., and Carpenter, K. N. (1986). Impairments in the learning and performance of a new manual skill in patients with Parkinson’s disease. J. Neurol. Neurosurg. Psychiatry 49, 661–668. doi: 10.1136/jnnp.49.6.661
Gan, Y., Xie, H., Qin, G., Wu, D., Shan, M., Hu, T., et al. (2023). Association between cognitive impairment and freezing of gait in patients with Parkinson’s disease. J Clin Med 12. doi: 10.3390/jcm12082799
Giladi, N. (2008). Medical treatment of freezing of gait. Mov. Disord. 23, S482–S488. doi: 10.1002/mds.21914
Godefroy, O., (2008). Fonctions exécutives et pathologies neurologiques et psychiatriques. France: De Boeck Supérieur SA.
Goetz, C. G., Fahn, S., Martinez-Martin, P., Poewe, W., Sampaio, C., Stebbins, G. T., et al. (2007). Movement disorder society-sponsored revision of the unified Parkinson’s disease rating scale (MDS-UPDRS): process, format, and clinimetric testing plan. Mov. Disord. 22, 41–47. doi: 10.1002/mds.21198
Goetz, C. G., Poewe, W., Rascol, O., Sampaio, C., Stebbins, G. T., Counsell, C., et al. (2004). Movement Disorder Society task force report on the Hoehn and Yahr staging scale: status and recommendations. Mov. Disord. 19, 1020–1028. doi: 10.1002/mds.20213
Hallett, M. (2008). The intrinsic and extrinsic aspects of freezing of gait. Mov. Disord. 23, S439–S443. doi: 10.1002/mds.21836
Harrington, D. L., Haaland, K. Y., Yeo, R. A., and Marder, E. (1990). Procedural memory in Parkinson’s disease: impaired motor but not visuoperceptual learning. J. Clin. Exp. Neuropsychol. 12, 323–339. doi: 10.1080/01688639008400978
Heindel, W. C., Salmon, D. P., Shults, C. W., Walicke, P. A., and Butters, N. (1989). Neuropsychological evidence for multiple implicit memory systems: a comparison of Alzheimer’s, Huntington’s, and Parkinson’s disease patients. J. Neurosci. 9, 582–587. doi: 10.1523/JNEUROSCI.09-02-00582.1989
Heremans, E., Nackaerts, E., Vervoort, G., Broeder, S., Swinnen, S. P., and Nieuwboer, A. (2016). Impaired retention of motor learning of writing skills in patients with Parkinson’s disease with freezing of gait. PLoS One 11:e0148933. doi: 10.1371/journal.pone.0148933
Heremans, E., Nieuwboer, A., and Vercruysse, S. (2013). Freezing of gait in Parkinson’s disease: where are we now? vol. 13.
Hipp, G., Vaillant, M., Diederich, N. J., Roomp, K., Satagopam, V. P., Banda, P., et al. (2018). The Luxembourg Parkinson’s study: a comprehensive approach for stratification and early diagnosis. Front. Aging Neurosci. 10:326. doi: 10.3389/fnagi.2018.00326
Hughes, A. J., Daniel, S. E., Kilford, L., and Lees, A. J. (1992). Accuracy of clinical diagnosis of idiopathic Parkinson’s disease: a clinico-pathological study of 100 cases. J. Neurol. Neurosurg. Psychiatry 55, 181–184. doi: 10.1136/jnnp.55.3.181
Jackson, G. M., Jackson, S. R., Harrison, J., Henderson, L., and Kennard, C. (1995). Serial reaction time learning and Parkinson’s disease: evidence for a procedural learning deficit. Neuropsychologia 33, 577–593. doi: 10.1016/0028-3932(95)00010-Z
Jenkinson, C., Fitzpatrick, R., Peto, V., Greenhall, R., and Hyman, N. (1997) The Parkinson’s disease questionnaire (PDQ-39): Development and validation of a Parkinson’s disease summary index score.
Jha, M., Jhunjhunwala, K., Sankara, B. B., Saini, J., Kumar, J. K., Yadav, R., et al. (2015). Neuropsychological and imaging profile of patients with Parkinson’s disease and freezing of gait. Parkinsonism Relat. Disord. 21, 1184–1190. doi: 10.1016/j.parkreldis.2015.08.009
Jorm, A. F. (1994). A short form of the informant questionnaire on cognitive decline in the elderly (IQCODE): development and cross-validation. Psychol. Med. 24, 145–153. doi: 10.1017/S003329170002691X
Krebs, H. I., Hogan, N., Hening, W., Adamovich, S. V., and Poizner, H. (2001). Procedural motor learning in Parkinson’s disease. Exp. Brain Res. 141, 425–437. doi: 10.1007/s002210100871
Lehéricy, S., Benali, H., Van De Moortele, P.-F., Pélégrini-Issac, M., Waechter, T., Ugurbil, K., et al. (2005) Distinct basal ganglia territories are engaged in early and advanced motor sequence learning.
Lövdén, M., Fratiglioni, L., Glymour, M. M., Lindenberger, U., and Tucker-Drob, E. M. (2020). Education and cognitive functioning across the life span. Psychol. Sci. Public Interest 21, 6–41. doi: 10.1177/1529100620920576
Macht, M., Kaussner, Y., Möller, J. C., Stiasny-Kolster, K., Eggert, K. M., Krüger, H. P., et al. (2007). Predictors of freezing in Parkinson’s disease: a survey of 6,620 patients. Mov. Disord. 22, 953–956. doi: 10.1002/mds.21458
Mishkin, M., and Appenzeller, T. (1987). The anatomy of memory. Sci. Am. 256, 80–89. doi: 10.1038/scientificamerican0687-80
Mochizuki-Kawai, H., Kawamura, M., Hasegawa, Y., Mochizuki, S., Oeda, R., Yamanaka, K., et al. (2004). Deficits in long-term retention of learned motor skills in patients with cortical or subcortical degeneration. Neuropsychologia 42, 1858–1863. doi: 10.1016/j.neuropsychologia.2004.03.012
Monaghan, A. S., Gordon, E., Graham, L., Hughes, E., Peterson, D. S., and Morris, R. (2023). Cognition and freezing of gait in Parkinson’s disease: a systematic review and meta-analysis. Neurosci. Biobehav. Rev. 147:105068. doi: 10.1016/j.neubiorev.2023.105068
Morris, R., Smulders, K., Peterson, D. S., Mancini, M., Carlson-Kuhta, P., Nutt, J. G., et al. (2020). Article cognitive function in people with and without freezing of gait in Parkinson’s disease.
Muslimović, D., Post, B., Speelman, J. D., and Schmand, B. (2007). Motor procedural learning in Parkinson’s disease. Brain 130, 2887–2897. doi: 10.1093/brain/awm211
Nasreddine, Z. S., Phillips, N. A., Bedirian, V., Charbonneau, S., Whitehead, V., Collin, I., et al. (2005). The Montreal cognitive assessment, MoCA: A Brief Screening. J. Am. Geriatr. Soc. 53, 695–699. doi: 10.1111/j.1532-5415.2005.53221.x
Nutt, J. G., Bloem, B. R., Giladi, N., Hallett, M., Horak, F. B., and Nieuwboer, A. (2011). Freezing of gait: moving forward on a mysterious clinical phenomenon. Lancet Neurol. 10, 734–744. doi: 10.1016/S1474-4422(11)70143-0
Pachchek, S., Landoulsi, Z., Pavelka, L., Schulte, C., Buena-Atienza, E., Gross, C., et al. (2023). Accurate long-read sequencing identified GBA1 as major risk factor in the Luxembourgish Parkinson’s study. npj Parkinsons Dis. 9:156. doi: 10.1038/s41531-023-00595-w
Panouillères, M. T. N., Tofaris, G. K., Brown, P., and Jenkinson, N. (2016). Intact acquisition and short-term retention of non-motor procedural learning in Parkinson’s disease. PLoS One 11:e0149224. doi: 10.1371/journal.pone.0149224
Pascual-Leone, A., Grafman, J., Clark, K., Stewart, M., Massaquoi, S., Lou, J.-S., et al. (1993). Procedural learning in Parkinson’s disease and cerebellar degeneration. Ann. Neurol. 34, 594–602. doi: 10.1002/ana.410340414
Pauly, L., Pauly, C., Hansen, M., Schröder, V. E., Rauschenberger, A., Leist, A. K., et al. (2022). Retrograde procedural memory in Parkinson’s disease: a cross-sectional, case-control study. J. Parkinsons Dis. 12, 1013–1022. doi: 10.3233/JPD-213081
Pendt, L. K., Reuter, I., and Müller, H. (2011). Motor skill learning, retention, and control deficits in Parkinson’s disease. PLoS One 6:e21669. doi: 10.1371/journal.pone.0021669
Perez-Lloret, S., Negre-Pages, L., Damier, P., Delval, A., Derkinderen, P., Destée, A., et al. (2014). Prevalence, determinants, and effect on quality of life of freezing of gait in Parkinson disease. JAMA Neurol. 71, 884–890. doi: 10.1001/jamaneurol.2014.753
Peterson, D. S., King, L. A., Cohen, R. G., and Horak, F. B. (2016) Cognitive contributions to freezing of gait in Parkinson disease: Implications for physical rehabilitation.
Peto, V., Jenkinson, C., Fitzpatrick, R., and Greenhail, R. (1995) The development and validation of a short measure of functioning and well being for individuals with Parkinson’s disease.
Pfeffer, R. I., Kurosaki, T. T., Harrah, C. H., Chance, J. M., and Filos, S. (1982). Measurement of functional activities in older adults in the community. J. Gerontol. 37, 323–329. doi: 10.1093/geronj/37.3.323
Roncacci, S., Troisi, E., Carlesimo, G. A., Nocentini, U., and Caltagirone, C. (1996). Implicit memory in parkinsonian patients: evidence for deficient skill learning. Eur. Neurol. 36, 154–159. doi: 10.1159/000117234
Saint-Cyr, J. A., Taylor, A. E., and Lang, A. E. (1988) Procedural learning and neostriatal dysfunction in man.
Sarazin, M., Deweer, B., Merkl, A., Von Poser, N., Pillon, B., and Dubois, B. (2002). Procedural learning and striatofrontal dysfunction in Parkinson’s disease. Mov. Disord. 17, 265–273. doi: 10.1002/mds.10018
Seidler, R. D., Tuite, P., and Ashe, J. (2007). Selective impairments in implicit learning in Parkinson’s disease. Brain Res. 1137, 104–110. doi: 10.1016/j.brainres.2006.12.057
Shine, J. M., Matar, E., Ward, P. B., Frank, M. J., Moustafa, A. A., Pearson, M., et al. (2013). Freezing of gait in Parkinson’s disease is associated with functional decoupling between the cognitive control network and the basal ganglia. Brain 136, 3671–3681. doi: 10.1093/brain/awt272
Sommer, M., Grafman, J., Clark, K., and Hallett, M. (1999). Learning in Parkinson’s disease: Eyeblink conditioning, declarative learning, and procedural learning. Neurol. Neurosurg. Psychiatry 67, 27–34. doi: 10.1136/jnnp.67.1.27
Starkstein, S. E., Mayberg, H. S., Preziosi, T. J., Andrezejewski, P., Leiguarda, R., Robinson, R. G., et al. (1992). Reliability, validity, and clinical correlates of apathy in Parkinson’s disease. J. Neuropsychiatry Clin. Neurosci. 4, 134–139. doi: 10.1176/jnp.4.2.134
Taximaimaiti, R., and Wang, X. P. (2021). Comparing the clinical and neuropsychological characteristics of Parkinson’s disease with and without freezing of gait. Front. Neurosci. 15:660340. doi: 10.3389/fnins.2021.660340
Thomas-Antérion, C., Laurent, B., Foyatier-Michel, N., Michel, D., and Laporte, S. (1996). Procedural memory: computer learning in control subjects and in Parkinson’s disease patients. Behav. Neurol. 9, 127–134. doi: 10.1155/1996/659269
Tomlinson, C. L., Stowe, R., Patel, S., Rick, C., Gray, R., and Clarke, C. E. (2010). Systematic review of levodopa dose equivalency reporting in Parkinson’s disease. Mov. Disord. 25, 2649–2653. doi: 10.1002/mds.23429
Vakil, E., Schwizer Ashkenazi, S., Nevet-Perez, M., and Hassin-Baer, S. (2021). Implicit sequence learning in individuals with Parkinson’s disease: the added value of using an ocular version of the serial reaction time (O-SRT) task. Brain Cogn. 147:105654. doi: 10.1016/j.bandc.2020.105654
Van der Linden, M., and Seron, X. (2014). Traité de neuropsychologie clinique de l’adulte: Tome 1 - Évaluation. Chapter 13 “L’évaluation de la mémoire procédurale” Thierry Meulemans (p.193) (FR).
Vandenbossche, J., Deroost, N., Soetens, E., Coomans, D., Spildooren, J., Vercruysse, S., et al. (2013a). Impaired implicit sequence learning in parkinson’s disease patients with freezing of gait. Neuropsychology 27, 28–36. doi: 10.1037/a0031278
Vandenbossche, J., Deroost, N., Soetens, E., Coomans, D., Spildooren, J., Vercruysse, S., et al. (2013b). Freezing of gait in Parkinson’s disease: disturbances in automaticity and control. Front. Hum. Neurosci. 6, 1–19. doi: 10.3389/fnhum.2012.00356
Walton, C. C., Mowszowski, L., Gilat, M., Hall, J. M., O’Callaghan, C., Muller, A. J., et al. (2018). Cognitive training for freezing of gait in Parkinson’s disease: a randomized controlled trial. NPJ Parkinsons Dis 4:4. doi: 10.1038/s41531-018-0052-6
Walton, C. C., Shine, J. M., Hall, J. M., O’callaghan, C., Mowszowski, L., Gilat, M., et al. (2015) The major impact of freezing of gait on quality of life in Parkinson’s disease.
Wang, C., Cai, Y., Gu, Z., Ma, J., Zheng, Z., Tang, B. S., et al. (2014). Clinical profiles of Parkinson’s disease associated with common leucine-rich repeat kinase 2 and glucocerebrosidase genetic variants in Chinese individuals. Neurobiol. Aging 35, 725.e1–725.e6. doi: 10.1016/j.neurobiolaging.2013.08.012
Westwater, H., McDowall, J., Siegert, R., Mossman, S., and Abernethy, D. (1998). Implicit learning in Parkinson’s disease: evidence from a verbal version of the serial reaction time task. J. Clin. Exp. Neuropsychol. 20, 413–418. doi: 10.1076/jcen.20.3.413.826
Wu, T., Liu, J., Zhang, H., Hallett, M., Zheng, Z., and Chan, P. (2015). Attention to automatic movements in Parkinson’s disease: modified automatic mode in the striatum. Cereb. Cortex 25, 3330–3342. doi: 10.1093/cercor/bhu135
Keywords: freezing of gait, Parkinson’s disease, cognitive impairment, procedural memory, gait impairment
Citation: Pauly L, Pauly C, Hansen M, Schröder VE, Rauschenberger A, Leist AK and Krüger R (2024) Retrograde procedural memory is impaired in people with Parkinson’s disease with freezing of gait. Front. Aging Neurosci. 15:1296323. doi: 10.3389/fnagi.2023.1296323
Edited by:
Shigeki Arawaka, Osaka Medical and Pharmaceutical University, JapanReviewed by:
Kensuke Ikenaka, Osaka University, JapanKuan-yi Li, Chang Gung University, Taiwan
Jinsoo Koh, Wakayama Medical University, Japan
Copyright © 2024 Pauly, Pauly, Hansen, Schröder, Rauschenberger, Leist and Krüger. This is an open-access article distributed under the terms of the Creative Commons Attribution License (CC BY). The use, distribution or reproduction in other forums is permitted, provided the original author(s) and the copyright owner(s) are credited and that the original publication in this journal is cited, in accordance with accepted academic practice. No use, distribution or reproduction is permitted which does not comply with these terms.
*Correspondence: Laure Pauly, laure.pauly@lih.lu; Rejko Krüger, rejko.krueger@lih.lu