- 1Department of Anatomy and Cell Biology, University of Illinois at Chicago, Chicago, IL, United States
- 2University of Illinois College of Medicine, Chicago, IL, United States
- 3University of Illinois College of Medicine, Peoria, IL, United States
- 4School of Medicine, St. George’s University, St. George’s, Grenada
Increasing evidence supports that age, APOE and sex interact to modulate Alzheimer’s disease (AD) risk, however the underlying pathways are unclear. One way that AD risk factors may modulate cognition is by impacting amyloid beta (Aβ) accumulation as plaques, and/or neuroinflammation Therefore, the goal of the present study was to evaluate the extent to which age, APOE and sex modulate Aβ pathology, neuroinflammation and behavior in vivo. To achieve this goal, we utilized the EFAD mice, which express human APOE3 or APOE4 and have five familial AD mutations (FAD) that result in Aβ42 overproduction. We assessed Aβ levels, reactive glia and Morris water maze performance in 6-, 10-, 14-, and 18-month-old EFAD mice. Female APOE4 mice had the highest Aβ deposition, fibrillar amyloid deposits and neuroinflammation as well as earlier behavior deficits. Interestingly, we found that female APOE3 mice and male APOE4 mice had similar levels of pathology. Collectively our data support that the combination of APOE4 and female sex is the most detrimental combination for AD, and that at older ages, female sex may be equivalent to APOE4 genotype.
1. Introduction
Alzheimer’s disease (AD) is a progressive neurodegenerative disease associated with deficits in the cognitive system (Warren et al., 2023). Important risk factors for sporadic AD include age, and sex (Riedel et al., 2016). Age is the greatest overall AD risk factor, with 1 in 9 people over the age of 65 diagnosed with AD (Rajan et al., 2021). APOE, the gene encoding apolipoprotein E, is the greatest genetic risk factor with APOE4 increasing risk up to 15-fold compared to APOE3 (Corder et al., 1993; Strittmatter et al., 1993), and APOE4 is also associated with earlier age of AD onset (Corder et al., 1993; Locke et al., 1995; Bilgel et al., 2016; Polsinelli et al., 2023a). Females have increased AD risk compared to males (Brinton, 1999; Riedel et al., 2016). Importantly, there is evidence that the AD risk factors interact. For example, there is higher AD risk in female APOE4 carriers, compared to male APOE4 carriers (Farrer et al., 1997; Altmann et al., 2014), which may be pronounced at older ages (65–75 years of age) (Neu et al., 2017). Furthermore, in APOE4 mice, behavioral impairments and AD pathology are worse in females compared to males (Raber et al., 1998, 2002; van Meer et al., 2007; Christensen et al., 2020). Therefore, it is important to understand how these risk factors interact to impact AD risk.
One way that AD risk factors may modulate cognition is by impacting amyloid beta (Aβ) accumulation as plaques, and/or neuroinflammation. In fact, APOE4 is associated with greater levels of Aβ (Kok et al., 2009) and higher neuroinflammation (Reale et al., 2012; Ringman et al., 2012; Tzioras et al., 2019; Friedberg et al., 2020) compared to non-carriers. In addition, older women generally have higher levels of amyloid pathology compared to men (Oveisgharan et al., 2018) and dysregulated neuroinflammatory responses (Klein and Flanagan, 2016). These human data are recapitulated in vivo in young mice, as APOE4 is associated with increased Aβ deposition and neuroinflammation, compared to APOE3 mice (Youmans et al., 2012b; Rodriguez et al., 2014; Stephen et al., 2019, 2022). Therefore, evaluating the extent that APOE and sex impact pathology at older ages is important for advancing our understanding of how each risk factor contributes to AD pathogenesis across the lifespan.
The goal of our study was to understand the extent that APOE and sex interact with age to impact Aβ levels, neuroinflammation, neuronal density and behavior in vivo. To this end, we used EFAD mice as they express human APOE3 (E3FAD) or APOE4 (E4FAD) and overproduce Aβ42. Pathology and behavior were assessed in male and female EFAD mice at 6-, 10-, 14-, and 18-months-of-age using biochemistry, immunohistochemistry, and the Morris water maze test.
2. Materials and methods
2.1. Animals
All experiments follow the University of Illinois at Chicago Animal Care Committee protocols. EFAD mice express five familial AD (FAD) mutations and human APOE. Four groups of EFAD (5xFAD+/−/human APOE+/+) mice were used: male E3FAD, female E3FAD, male E4FAD, and female E4FAD mice as described previously (Youmans et al., 2012b). Mice from each of the four groups (male and female, E3FAD and E4FAD mice) were enrolled by age (i.e., 6, 10, 14, or 18 months euthanized together) as the breeding schedule permitted. Mice were ear-tagged during genotyping and investigators conducting experiments and data analysis were blinded for APOE genotype, sex, and age. Two cohorts of mice were used in this study (n = 13–25), one was used for biochemical and immunohistochemical analysis and the second for behavior analysis using Morris water maze.
2.2. Brain tissue harvest and processing
Mice were anesthetized via intraperitoneal injection with ketamine (100 mg/kg) and xylazine (5 mg/kg) and perfused transcardially with ~40 mL phosphate buffered saline with protease inhibitor cocktail. Then, the brains were removed and dissected at the midline to produce two hemi-brains, one each for immunohistochemical and biochemical analysis (Youmans et al., 2012b; Valencia-Olvera et al., 2023). The hemi for immunohistochemical analysis was drop-fixed in 4% paraformaldehyde for 24 h and then transferred to phosphate buffered saline containing 0.01% sodium azide until ready to section on a sliding microtome. Cortex was dissected from the hemi-brain for biochemical analysis, flash-frozen in liquid nitrogen and then stored at −80°C.
2.3. Immunohistochemical analysis
Serial sagittal brain sections (35 μm thick, 280 μm apart, ~0.24 mm – 3.44 mm lateral) from EFAD mice were immunostained for Aβ deposition, astrogliosis or microgliosis (Youmans et al., 2012b; Rodriguez et al., 2014; Valencia-Olvera et al., 2023) and stained for fibrillar amyloid deposition via Thio-S (see full list of antibodies and reagents used in this study in Supplementary Table S1). The stained sections were imaged at 10X magnification with a Zeiss Fluorescence microscope and analyzed for cortical area covered by MOAB-2, Thio-S, GFAP and Iba-1 in the cortex using ImageJ by investigators blinded to age, APOE genotype, and sex. Serial sagittal sections (three sections between ~0.72-and 1.80-mm lateral) from EFAD mice were stained for NeuN and neuron numbers quantification in layer 5 of the somatosensory cortex, was performed by a design-based stereology system (Stereo Investigator 9, MBF Bioscience, Williston, VT, USA) using an optical fractionator applying the Nv × Vref method (West, 2002; Schmitz and Hof, 2007).
2.4. Biochemical analysis
Cortices were homogenized in 70% formic acid at 1 mL/150 mg brain tissue and mixed by end-over-end rotation at room temperature for 2 h with vortexing. Samples were then centrifuged (100,000 × g, 1 h at 4°C), and the formic acid-soluble fraction was neutralized (with 20 volumes of 1 M Tris base), aliquoted, and frozen at −80°C (Youmans et al., 2011). Total protein in the formic acid soluble extracts was quantified using the Bradford assay and formic acid-soluble Aβ42 was measured by ELISA following the manufacturer’s instructions (Youmans et al., 2011, 2012a,b). Cortical drebrin levels were measured using Western blotting as described previously (Valencia-Olvera et al., 2023).
2.5. Behavioral test: Morris water maze
All behavioral data were recorded and analyzed with ANY-maze software (Stoelting Co, Wood Dale, IL, USA). In the week prior to sacrifice, mouse behavior was tested using a modified Morris water maze protocol with acquisition trials consisting of 4 × 1 min trials/day for 5 consecutive days with latency to the platform recorded for each trial. A single probe trial was run on day 6 with the platform removed, and the readouts included latency to platform area, platform area crosses and latency to target quadrant (Liu et al., 2015; Thomas et al., 2017; Valencia-Olvera et al., 2023).
2.6. Data and statistical analysis
Supplementary File S1 is a word file containing Supplementary Table S1 and Supplementary Figures S1–S6. Supplementary File S2 is an excel file containing all raw data and statistical analysis tables including number of mice used for each experiment. Morris water maze acquisition phase data was analyzed by using repeated measure univariate general linear model for within subjects’ effects with the independent variables day, age, APOE and sex in SPSS (IBM SPSS Statistics for Macintosh, Version 29.0.1.1). All other statistical analyses were conducted using univariate general linear models for between subjects’ effects with the independent variables (age, APOE genotype, sex) and their interactions, followed by Bonferroni’s post hoc tests in SPSS. For all statistical tests, p < 0.05 was considered significant. All data are presented as scatter plots with the mean and standard error of mean (SEM). The n size range is indicated in Figure legends. Outliers were excluded using the ROUT method (Q = 5%). All data, detailed n sizes and statistical analysis are provided in Supplementary File S2.
3. Results
The goal of our study was to determine the extent to which age, APOE and sex modulates Aβ pathology, neuroinflammation and behavior in vivo. To this end, we used EFAD mice that overproduce Aβ42 (via 5xFAD mutations) and express human APOE3 (E3FAD) or APOE4 (E4FAD). EFAD mice were developed to identify how APOE modulates progression of AD-relevant pathology and behavior (Youmans et al., 2012b). Previous studies in EFAD mice have demonstrated that at younger ages (≤ 6 months), Aβ levels, reactive microglia and astrocytes are higher with APOE4 compared to APOE3 (Youmans et al., 2012b; Stephen et al., 2019, 2022), and that there are behavioral deficits in female APOE4 mice (Liu et al., 2015). Therefore, we determined the extent that APOE, age and sex impact Aβ pathology, gliosis, and behavior in young (6 and 10 months), middle-aged (14 months) and old (18 months) EFAD mice.
3.1. APOE and sex impact Aβ pathology: E4FAD females > E4FAD males = E3FAD females > E3FAD
Extracellular Aβ in fibrillar amyloid deposits are used to diagnose AD and are a current therapeutic target (Zhang Y. et al., 2023). Although APOE4 is associated with higher amyloid deposits in human and in vivo, the extent that APOE and sex modulate extracellular Aβ with age is unclear. Therefore, we initially evaluated cortical extracellular Aβ levels (MOAB-2 immunostaining for Aβ: Figure 1A) and fibrillar amyloid deposits (Thio-S staining: Supplementary Figure S1A) in male and female, E3FAD and E4FAD mice. We found that age interacts with APOE to modulate Aβ and fibrillar amyloid deposition (Figures 1B,C). This interaction was due to greater levels of Aβ at 10 and 18 months and amyloid deposits at 10, 14, and 18 months of age, but not at 6 months, in E4FAD mice compared to E3FAD mice. In general, amyloid deposition increased at every age (18 > 14 > 10 > 6 months) in E4FAD mice, whereas levels plateaued at ~10–14 months of age in E3FAD mice (Figures 1B,C). There was also an interaction between age and sex in modulating Aβ deposition, with greater levels in females compared to males at older ages (10, 14, and 18 months) but not at younger ages (6 months). For fibrillar amyloid deposition there was an APOE by sex interaction as levels were in the order E4FAD females > E4FAD males = E3FAD females > E3FAD males (Figure 1C), however there was no age x sex interaction (Supplementary Figure S1B). We also evaluated the levels of formic acid soluble Aβ42 in the cortex (Supplementary Figure S2). In general, the pattern of insoluble Aβ42 levels appears like extracellular Aβ deposition, however the levels were more varied. Thus, at the statistical level formic acid Aβ42 levels were higher with APOE4 compared to APOE3 and in females vs. males at older ages (10–18 months).
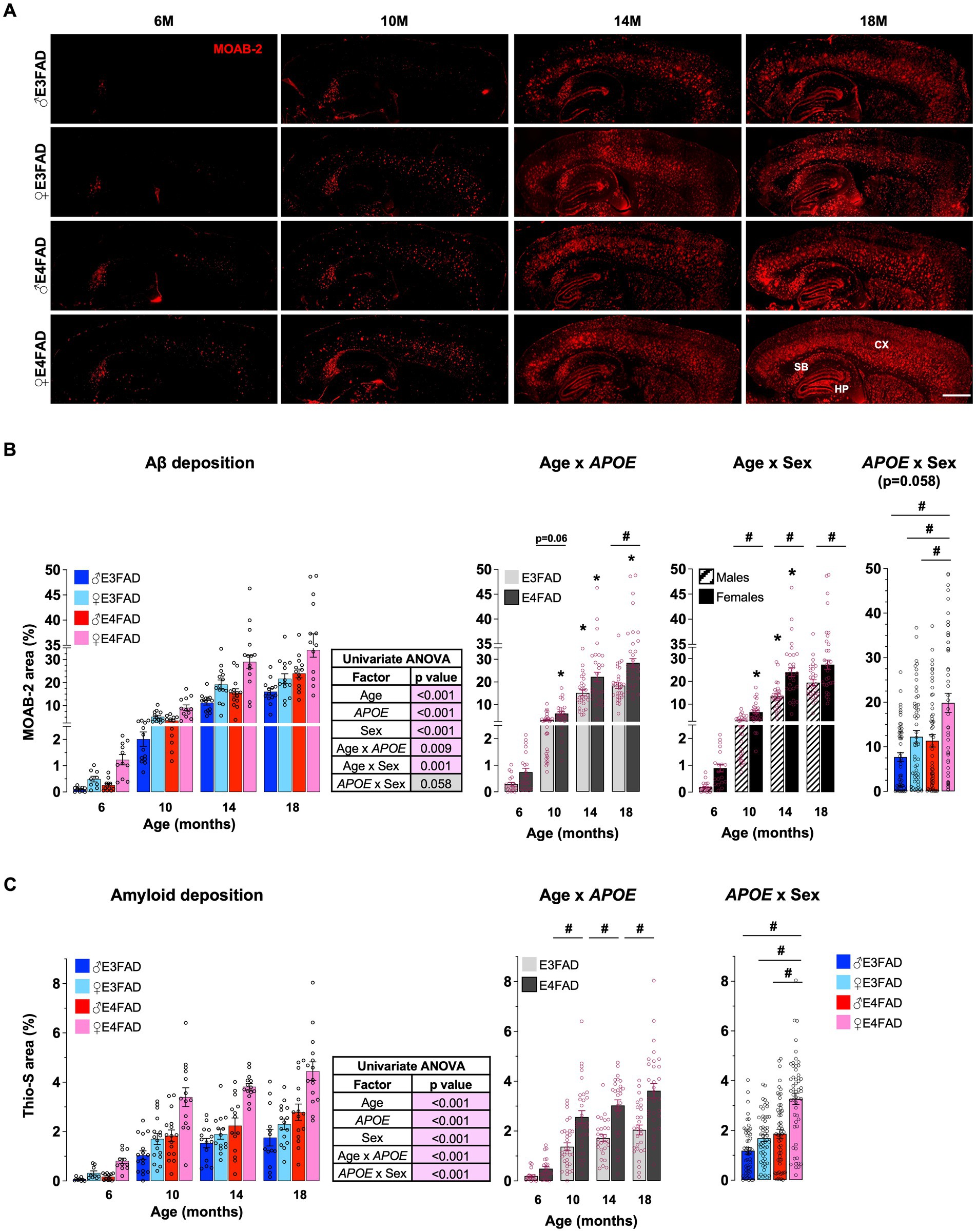
Figure 1. APOE and sex impact Aβ pathology: E4FAD females > E4FAD males = E3FAD females > E3FAD. Cortical extracellular Aβ deposits were assessed using histochemical analysis in male and female, E3FAD and E4FAD mice. (A) Brain sections were immunostained for Aβ using MOAB-2 (Red, scale bars: 1000 μm) and the percentage area quantified (B). (C) Brain sections stained with Thio-S for fibrillar amyloid deposits (see Supplementary Figure S1 for representative images) were quantified for cortical amyloid burden. Data are expressed as mean ± S.E.M and analyzed by univariate general linear modeling followed by Bonferroni’s post-hoc analysis (n = 9–15, # APOE/sex difference within an age group, * vs. previous age within APOE/sex combination p < 0.05). See Supplementary File S2 for detailed n sizes and statistical analysis.
Collectively these data support that female sex and APOE have a strong effect on extracellular Aβ deposition. That interaction results in highest fibrillar amyloid deposits in female APOE4 carriers, equivalent levels in female APOE3 and male APOE4 carriers, and lowest levels in male APOE3 carriers at older ages.
3.2. APOE and sex affect neuroinflammation: E4FAD females > E3FAD females ≥ E4FAD males > E3FAD males
APOE4 is associated with higher inflammatory responses in younger EFAD mice (Vitek et al., 2012; Rodriguez et al., 2014; Stephen et al., 2022) and in humans (Reale et al., 2012; Ringman et al., 2012; Tzioras et al., 2019; Friedberg et al., 2020). Therefore, we next evaluated the extent that APOE, age and sex impacted levels of reactive astrocytes (GFAP: Figure 2A) and microglia (Iba-1: Figure 2C) via immunostaining, in male and female, E3FAD and E4FAD mice. We found that GFAP coverage was higher with APOE4 compared to APOE3, and for Iba-1 there was interaction between APOE and age with higher coverage in E4FAD mice compared to E3FAD mice at 18 months of age with no effect at other ages (there were also no interactions between age and APOE or APOE and sex, Supplementary Figure S3A). As for extracellular Aβ, we found that age interacts with sex to modulate both astrogliosis and microgliosis. This interaction was due to significantly greater GFAP and Iba-1 coverage in the cortex of female mice between 10 and 18 months (Figures 2B,D). Furthermore, both percentage area covered by GFAP and Iba-1 increased at every age (18 > 14 > 10 > 6 months) in female mice, whereas levels plateaued at ~10–14 months of age in male mice (Figures 2B,D). Overall, these results support that neuroinflammation measured as gliosis was significantly impacted by female sex and APOE.
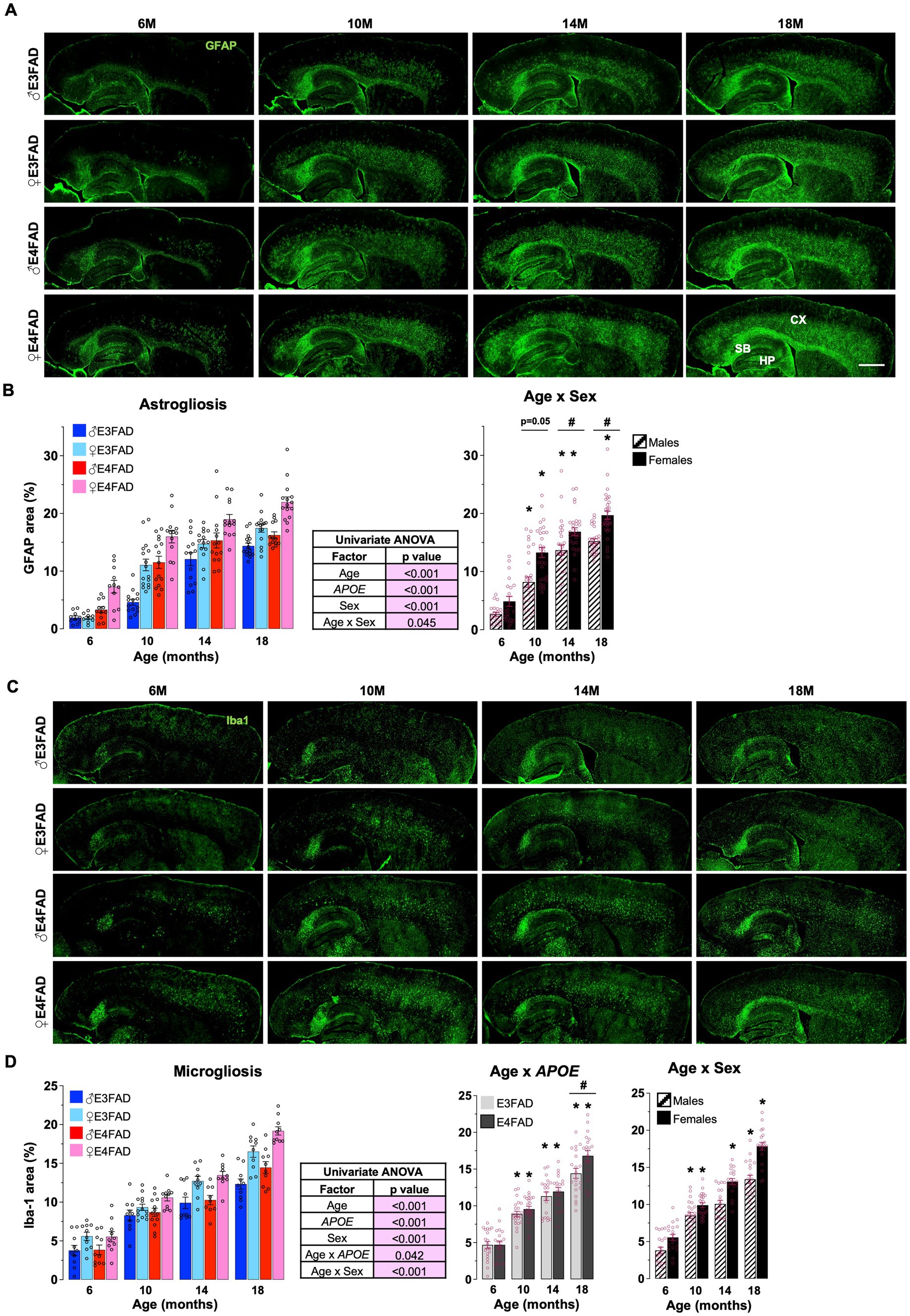
Figure 2. Sex impact neuroinflammation: E4FAD females > E3FAD females > E4FAD males > E3FAD males. Reactive astrocytes and microglia were assessed using immunohistochemical analysis in male and female, E3FAD and E4FAD mice. (A) Brain sections were immunostained for astrogliosis using GFAP (green, scale bars: 1000 μm) and the percentage area of cortex covered was quantified (B). (C) Reactive microglia in brain sections were immunostained for using Iba-1 (green, scale bars: 1000 μm) and the percentage area of cortex was quantified (D). Data are expressed as mean ± S.E.M and analyzed by univariate general linear modeling followed by Bonferroni’s post-hoc analysis (n = 9–15, # APOE/sex difference within an age group, * vs. previous age within APOE/sex combination p < 0.05). See Supplementary File S2 for detailed n sizes and statistical analysis.
3.3. Female E4FAD mice have early behavioral deficits in acquisition phase of Morris water maze
AD is associated with changes in cognitive trajectory in humans (Jack et al., 2015; Rasmussen et al., 2018) and behavioral deficits in FAD mice (Webster et al., 2014; Jankowsky and Zheng, 2017) including EFAD mice (Liu et al., 2015; Marottoli et al., 2017; Thomas et al., 2017). Therefore, we evaluated the effect of age, APOE and sex in EFAD mice using a modified Morris water maze protocol. It is important to note that our protocol did not include a visible platform. Therefore, we cannot discern whether the swim speeds differ among the four cohorts of mice with age, which in turn may affect their performance in the water maze test. In the acquisition phase, we found that there was an interaction among age, APOE, sex and training day. This interaction was due to a few factors, one of which was differences among groups at each age. When comparing training day 1 to 5, all the four groups of mice learned the location of the platform 6 months of age (Figure 3A: 6 M) (i.e., no effect of APOE or sex). However, at 10-and 14-months of age, female E4FAD mice did not improve in performance from day 1 to day 5 (Figure 3A: 10 M and 14 M). Furthermore, at 18 months of age, only male E3FAD mice showed a significant latency reduction from day 1 to day 5 (Figure 3A: 18 M). These data supported that female E4FAD mice had impairments in acquisition at an earlier age than other groups. Infact, when the day 5 latencies at 6 months of age were compared to other ages, we found deficits at 10 months in E4FAD female mice, at 18 months in E3FAD females and E4FAD males, with no changes in E3FAD males (Supplementary Figure S4A). We also evaluated memory 24 h after the last training day using a single probe trial. We found that most probe measures (latency to platform, latency to target quadrant and platform crosses) were impacted by APOE (Figure 3B). However, age, APOE and sex did not affect time spent in target quadrant during the probe trials (Supplementary Figure S4B). Also, there were no interactions among age, APOE and sex for the probe trial measures (Supplementary Figures S4C–F). Importantly, the latency to platform was independently modulated by APOE due to a greater latency to find the platform area and target quadrant in E4FAD compared to E3FAD mice.
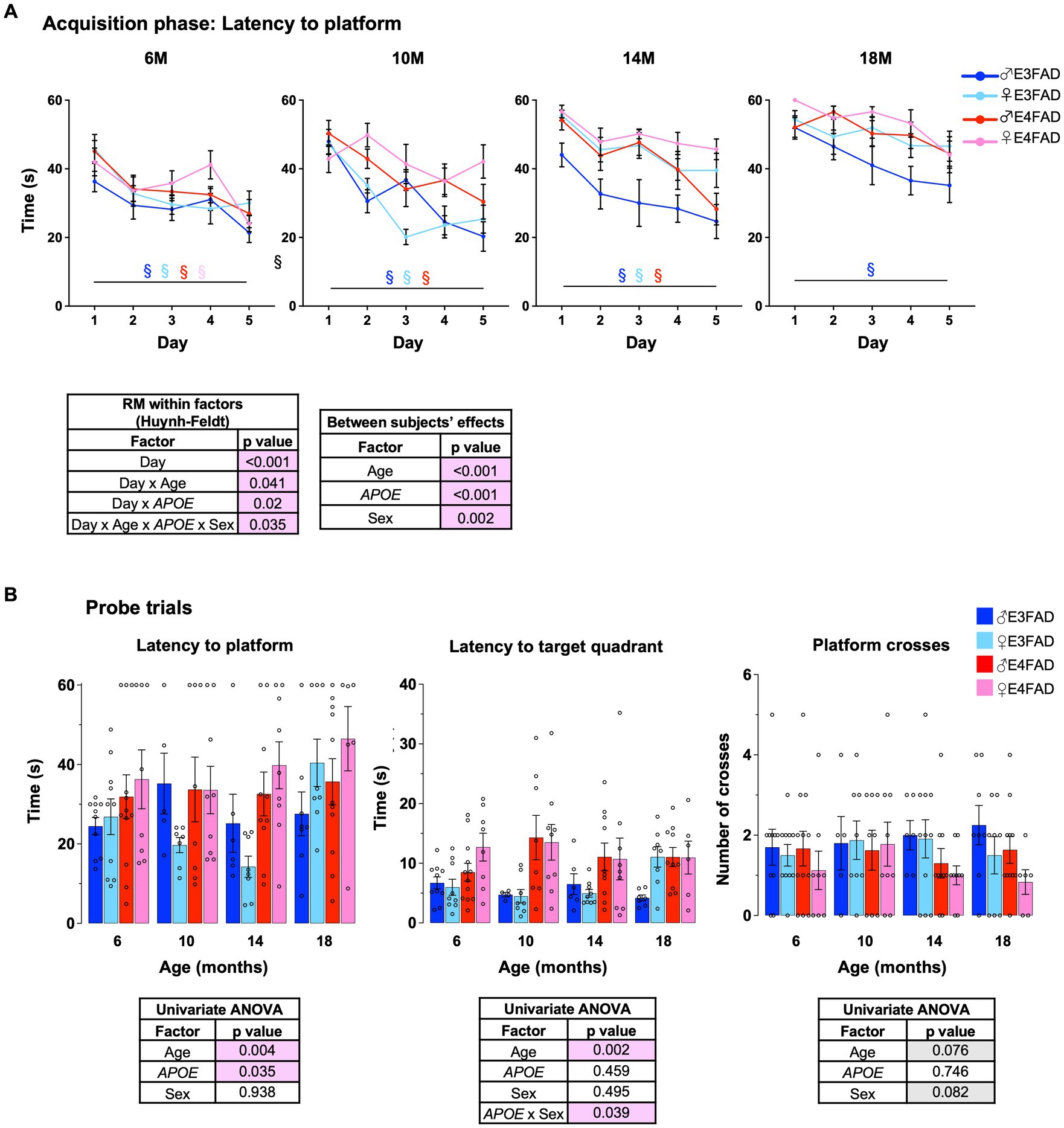
Figure 3. Female E4FAD mice have early behavioral deficits. Learning and memory were assessed via Morris water maze. (A) EFAD mice were trained to locate the location of a platform over 5 days (acquisition phase) and (B) the ability to remember the location of the platform 24 h after the last training day (probe trial). Data are expressed as mean ± S.E.M. Latency to platform during acquisition phase was analyzed by repeated measure general linear modeling followed by Bonferroni’s post-hoc analysis (n = 9–15, § day 1 vs. day 5 matched by mouse group color, p < 0.05). Probe trial measures were analyzed by univariate general linear modeling followed by Bonferroni’s post-hoc analysis (n = 9–15, # APOE/sex difference within an age group, * vs. previous age within APOE/sex combination p < 0.05). See Supplementary File S2 for detailed n sizes and statistical analysis.
We next assessed neuronal density (NeuN) in layer 5 of somatosensory cortex in male and female, E3FAD and E4FAD mice (Supplementary Figure S5A). Similar to the probe trial measures for memory, we found that neuronal density (Supplementary Figure S5B) and Drebrin levels (Supplementary Figure S5C) were impacted by age (lower with age) and APOE (APOE4 > APOE3). Collectively, our data support that E4FAD females demonstrated earlier behavioral deficits and loss of neurons at 6–10 months of age, compared to the other groups.
4. Discussion
4.1. Aβ deposition
Aβ deposition as plaques is one of the main AD pathologies (Rodrigue et al., 2009; Ruan and Sun, 2023; Suemoto and Leite, 2023). As a well-established AD risk (Kawas et al., 2000; Farfel et al., 2019), age has been proposed to modulate Aβ deposition. However, amyloid deposits occur in about 20% of cognitively normal elderly subjects and therefore may also be modulated by other AD risk factors. APOE has a pronounced impact on Aβ deposition. Aβ levels are higher with APOE4 in human (Villemagne et al., 2011; Chiotis et al., 2015; Jack et al., 2015; Resnick et al., 2015; Mishra et al., 2018; Ba et al., 2019; Baek et al., 2020; Therriault et al., 2020, 2021; Cacciaglia et al., 2022) and mouse studies (Youmans et al., 2012b; Moser and Pike, 2017; Montagne et al., 2021). Sex is also thought to impact Aβ deposition. The independent effect of sex in modulating Aβ deposition is conflicting in humans (Jack et al., 2015; Ba et al., 2019; Therriault et al., 2020, 2021; Cacciaglia et al., 2022), potentially due to variable hormone levels, disease stage, co-morbidities and other lifestyle factors. However, in FAD mice, females have greater Aβ pathology compared to males (Wang et al., 2003; Li et al., 2016; Dennison et al., 2021; Forner et al., 2021; Marazuela et al., 2022; Sil et al., 2022). Our data agree with in vivo and human studies that the combination of APOE4 and female sex results in highest levels of Aβ deposition compared to other groups (Figure 1; Supplementary Figure S2). Interestingly, our data also demonstrate that Aβ pathology is equivalent in female E3FAD mice and male E4FAD mice. These novel findings raise the possibility that female sex and APOE4 induce a similar impact on Aβ pathology. These findings support that female sex may be an important factor for enrolling in preclinical/clinical trials targeting Aβ (Shi et al., 2022).
4.2. Neuroinflammation
Neuroinflammation is also an important AD pathology, often defined as levels of reactive astrocytes and microglia (glia) in the brain (Streit et al., 2004). In general, in AD patients higher levels of reactive glia are thought to occur early in disease progression (Hoozemans et al., 2011), in females compared to males (Duarte-Guterman et al., 2020; Lynch, 2022) and with APOE4 (Reale et al., 2012; Ringman et al., 2012; Tzioras et al., 2019; Friedberg et al., 2020). However, the interaction among age, APOE and sex on reactive glia has yet to be characterized in AD patients, and therefore FAD mouse models are currently being used to address this issue. In vivo studies demonstrate that there is greater neuroinflammation in females compared to males in FAD mice (Hou et al., 2015; Schmid et al., 2019; Guillot-Sestier et al., 2021; Mifflin et al., 2021; Sil et al., 2022). This sex effect is pronounced with APOE4 in young mice (Stephen et al., 2019, 2022). We have extended these findings to older mice and demonstrate that the combination of APOE4 and female sex results in the highest levels of reactive glia with age. In addition, our data revealed that in general, female E3FAD mice have greater neuroinflammation compared to male E4FAD mice. Collectively, our data demonstrates that targeting neuroinflammation may be an attractive therapeutic approach for females AD patients.
4.3. Behavior
AD is typically characterized by memory loss, and a decline in overall cognitive function (Rao et al., 2023). Several studies demonstrate that with age, cognitive decline is accelerated in AD patients (Rosselli et al., 2000; Conde-Sala et al., 2013; Zhao et al., 2014; Pan et al., 2021) and FAD mice (Middei et al., 2006; Ferguson et al., 2013; Webster et al., 2013; Olesen et al., 2016; Forner et al., 2021; Locci et al., 2021). Among AD patients, cognitive decline is pronounced in APOE4, compared to APOE3 carriers (Emrani et al., 2020; Gharbi-Meliani et al., 2021; Qian et al., 2021, 2023; Polsinelli et al., 2023b). This effect is mirrored in FAD mice in the presence of APOE4, compared to APOE3 in young (Thomas et al., 2017) and old (Montagne et al., 2021) mice. In addition, sex impacts cognitive decline in AD patients and memory deficits in mice. In general, women display poorer cognitive profiles compared to men at the same stage of AD (Subramaniapillai et al., 2021) and is mirrored in female mice compared to male FAD mice (Poon et al., 2023), also reviewed in Li and Singh (2014) and Laws et al. (2016). In this study, we evaluated the combined effect of age, APOE and sex in Morris water maze performance and found that female E4FAD mice had earlier behavioral deficits during acquisition phase of Morris water maze compared to other groups (Figure 3A). Consistent with other readouts, APOE3 female and APOE4 male had similar behavioral deficits with age.
4.4. Limitations
There are some limitations due to the nature of our design. One issue is that we are limited in the extent that we can conclude APOE, sex and age impacted all aspects of AD pathology and behavior. Future studies could incorporate more detailed evaluation of Aβ production, clearance, and degradation pathways, the full neuroinflammatory phenotype, other AD-prevalent pathologies such as vascular function and metabolism, neuronal function, and a full battery of behavioral tests in the cognitive domain. Furthermore, it is important to validate our findings in other models that incorporate human APOE and human APP. For example, human APP knock in mice have been crossed with APOE targeted replacement mice, and data supports greater behavioral deficits and Aβ pathology with APOE4 (Holden et al., 2022). In addition, understanding the effect APOE2 genotype which is protective for AD risk is important, with the caveat that these mice have Type III hyperlipoproteinemia (Sullivan et al., 1998). Our study raises an important discussion of the possible underlying mechanisms of how APOE4 and female sex impact AD pathology and cognition. For example, structural differences between apoE isoforms may modulate Aβ levels, glial reactivity, and neuron function [reviewed in Hunsberger et al. (2019), Martinez-Martinez et al. (2020), Raulin et al. (2022), Yang et al. (2023), and Zhang L. et al. (2023)]. As for female sex, the increase in AD risk/pathology has been attributed to loss of sex hormones with age particularly at menopause in humans (Rocca et al., 2011; Levine et al., 2016; Jett et al., 2022; Mishra et al., 2022), which is recapitulated in ovariectomized mice (Ge et al., 2020; Sanchez et al., 2023). Although mice do not have the typical menopausal symptoms as humans, there are age-dependent changes in hormonal levels, which could impact AD-pathology and cognition in mice. Indeed, we observed that the number of mice in proestrus/estrus decrease with age in female EFAD mice (Supplementary Figure S6). In addition, there may be X chromosome-mediated modulation of AD risk (Guo et al., 2022) may partly explain the sex-biased differences in AD. Furthermore, our study has also raised questions for future research including: What aspect of AD pathology is proximal to behavior changes in EFAD mice? What is the effect of APOE3/4 genotype on AD pathology and the interaction with sex? Does the impact of sex and APOE on pathology modify responses to therapeutic treatments?
4.5. Conclusion
Our data support that the combination of female sex and APOE4 result in the greatest levels of Aβ pathology, neuroinflammation and behavioral impairments. In addition, we propose that the effect of female sex is analogous to the presence of APOE4 for AD pathology. Through reviewing previous published in vivo and human data, we believe that this important concept may have been overlooked (Holland et al., 2013; Huynh et al., 2019; Stephen et al., 2019, 2022; Cho et al., 2021; Montagne et al., 2021; Palmer et al., 2022). The female sex and APOE4 equivalency could therefore impact the optimal treatment window for therapeutics aimed at preventing AD through targeting Aβ and/or neuroinflammation.
Data availability statement
The original contributions presented in the study are included in the article/Supplementary material, further inquiries can be directed to the corresponding author.
Ethics statement
The animal study was approved by University of Illinois Animal Care Committee. The study was conducted in accordance with the local legislation and institutional requirements.
Author contributions
DB: Writing – original draft, Writing – review & editing, Data curation, Formal analysis, Investigation, Project administration, Supervision. AV-O: Data curation, Investigation, Supervision, Writing – review & editing. ZI: Writing – review & editing, Methodology. CM: Writing – review & editing, Methodology. AH: Writing – review & editing, Methodology. TP: Writing – review & editing, Methodology. JY: Methodology, Writing – review & editing. ML: Conceptualization, Funding acquisition, Project administration, Supervision. LT: Writing – review & editing, Writing – original draft.
Funding
The author(s) declare financial support was received for the research, authorship, and/or publication of this article. Previous funding to ML included National Institutes of Health (NIH) grants (R01 AG058068, R01AG057008, R21AG044682), University of Illinois at Chicago Institutional funds, and philanthropic support from Louis and Christine Friedrich. LT was supported by R01AG061114 (NIH) and University of Illinois at Chicago Institutional funds.
In memoriam
This manuscript is in memory of Dr. Mary Jo LaDu, who recently tragically passed away. She will be missed by all.
Conflict of interest
The authors declare that the research was conducted in the absence of any commercial or financial relationships that could be construed as a potential conflict of interest.
The author(s) declared that they were an editorial board member of Frontiers, at the time of submission. This had no impact on the peer review process and the final decision.
Publisher’s note
All claims expressed in this article are solely those of the authors and do not necessarily represent those of their affiliated organizations, or those of the publisher, the editors and the reviewers. Any product that may be evaluated in this article, or claim that may be made by its manufacturer, is not guaranteed or endorsed by the publisher.
Supplementary material
The Supplementary material for this article can be found online at: https://www.frontiersin.org/articles/10.3389/fnagi.2023.1279343/full#supplementary-material
References
Altmann, A., Tian, L., Henderson, V. W., and Greicius, M. D. (2014). Sex modifies the APOE-related risk of developing Alzheimer disease. Ann. Neurol. 75, 563–573. doi: 10.1002/ana.24135
Ba, M., Ng, K. P., Gao, X., Kong, M., Guan, L., Yu, L., et al. (2019). The combination of apolipoprotein E4, age and Alzheimer’s disease assessment scale – cognitive subscale improves the prediction of amyloid positron emission tomography status in clinically diagnosed mild cognitive impairment. Eur. J. Neurol. 26, 733–e53. doi: 10.1111/ene.13881
Baek, M. S., Cho, H., Lee, H. S., Lee, J. H., Ryu, Y. H., and Lyoo, C. H. (2020). Effect of APOE epsilon 4 genotype on amyloid-beta and tau accumulation in Alzheimer’s disease. Alzheimers Res. Ther. 12:140. doi: 10.1186/s13195-020-00710-6
Bilgel, M., An, Y., Zhou, Y., Wong, D. F., Prince, J. L., Ferrucci, L., et al. (2016). Individual estimates of age at detectable amyloid onset for risk factor assessment. Alzheimers Dement. 12, 373–379. doi: 10.1016/j.jalz.2015.08.166
Brinton, R. D. (1999). A women’s health issue: Alzheimer’s disease and strategies for maintaining cognitive health. Int. J. Fertil. Womens Med. 44, 174–185.
Cacciaglia, R., Salvado, G., Molinuevo, J. L., Shekari, M., Falcon, C., Operto, G., et al. (2022). Age, sex and APOE-epsilon4 modify the balance between soluble and fibrillar beta-amyloid in non-demented individuals: topographical patterns across two independent cohorts. Mol. Psychiatry 27, 2010–2018. doi: 10.1038/s41380-022-01436-7
Chiotis, K., Carter, S. F., Farid, K., Savitcheva, I., and Nordberg, A., Diagnostic Molecular Imaging (DiMI) network and the Alzheimer’s Disease Neuroimaging Initiative (2015). Amyloid PET in European and north American cohorts; and exploring age as a limit to clinical use of amyloid imaging. Eur. J. Nucl. Med. Mol. Imaging 42, 1492–1506. doi: 10.1007/s00259-015-3115-5
Cho, S. H., Woo, S., Kim, C., Kim, H. J., Jang, H., Kim, B. C., et al. (2021). Disease progression modelling from preclinical Alzheimer’s disease (AD) to AD dementia. Sci. Rep. 11:4168. doi: 10.1038/s41598-021-83585-3
Christensen, A., Liu, J., and Pike, C. J. (2020). Aging reduces estradiol protection against neural but not metabolic effects of obesity in female 3xTg-AD mice. Front. Aging Neurosci. 12:113. doi: 10.3389/fnagi.2020.00113
Conde-Sala, J. L., Garre-Olmo, J., Vilalta-Franch, J., Llinas-Regla, J., Turro-Garriga, O., Lozano-Gallego, M., et al. (2013). Cognitive decline in Alzheimer’s disease. A follow three or more years of a sample of patients. Rev. Neurol. 56, 593–600. doi: 10.33588/rn.5612.2013118
Corder, E. H., Saunders, A. M., Strittmatter, W. J., Schmechel, D. E., Gaskell, P. C., Small, G. W., et al. (1993). Gene dose of apolipoprotein E type 4 allele and the risk of Alzheimer’s disease in late onset families. Science 261, 921–923. doi: 10.1126/science.8346443
Dennison, J. L., Ricciardi, N. R., Lohse, I., Volmar, C. H., and Wahlestedt, C. (2021). Sexual dimorphism in the 3xTg-AD mouse model and its impact on pre-clinical research. J. Alzheimers Dis. 80, 41–52. doi: 10.3233/JAD-201014
Duarte-Guterman, P., Albert, A. Y., Inkster, A. M., Barha, C. K., and Galea, L. A. M., Alzheimer’s Disease Neuroimaging Initiative (2020). Inflammation in Alzheimer’s disease: do sex and APOE matter? J. Alzheimers Dis. 78, 627–641. doi: 10.3233/JAD-200982
Emrani, S., Arain, H. A., DeMarshall, C., and Nuriel, T. (2020). APOE4 is associated with cognitive and pathological heterogeneity in patients with Alzheimer’s disease: a systematic review. Alzheimers Res. Ther. 12:141. doi: 10.1186/s13195-020-00712-4
Farfel, J. M., Yu, L., Boyle, P. A., Leurgans, S., Shah, R. C., Schneider, J. A., et al. (2019). Alzheimer’s disease frequency peaks in the tenth decade and is lower afterwards. Acta Neuropathol. Commun. 7:104. doi: 10.1186/s40478-019-0752-0
Farrer, L. A., Cupples, L. A., Haines, J. L., Hyman, B., Kukull, W. A., Mayeux, R., et al. (1997). Effects of age, sex, and ethnicity on the association between apolipoprotein E genotype and Alzheimer disease. A meta-analysis. APOE and Alzheimer disease Meta analysis consortium. JAMA 278, 1349–1356. doi: 10.1001/jama.1997.03550160069041
Ferguson, S. A., Sarkar, S., and Schmued, L. C. (2013). Longitudinal behavioral changes in the APP/PS1 transgenic Alzheimer’s disease model. Behav. Brain Res. 242, 125–134. doi: 10.1016/j.bbr.2012.12.055
Forner, S., Kawauchi, S., Balderrama-Gutierrez, G., Kramar, E. A., Matheos, D. P., Phan, J., et al. (2021). Systematic phenotyping and characterization of the 5xFAD mouse model of Alzheimer’s disease. Sci. Data 8:270. doi: 10.1038/s41597-021-01054-y
Friedberg, J. S., Aytan, N., Cherry, J. D., Xia, W., Standring, O. J., Alvarez, V. E., et al. (2020). Associations between brain inflammatory profiles and human neuropathology are altered based on apolipoprotein E epsilon4 genotype. Sci. Rep. 10:2924. doi: 10.1038/s41598-020-59869-5
Ge, F., Yang, H., Lu, W., Shi, H., Chen, Q., Luo, Y., et al. (2020). Ovariectomy induces microglial cell activation and inflammatory response in rat prefrontal cortices to accelerate the chronic unpredictable stress-mediated anxiety and depression. Biomed. Res. Int. 2020, 3609758–3609713. doi: 10.1155/2020/3609758
Gharbi-Meliani, A., Dugravot, A., Sabia, S., Regy, M., Fayosse, A., Schnitzler, A., et al. (2021). The association of APOE epsilon4 with cognitive function over the adult life course and incidence of dementia: 20 years follow-up of the Whitehall II study. Alzheimers Res. Ther. 13:5. doi: 10.1186/s13195-020-00740-0
Guillot-Sestier, M. V., Araiz, A. R., Mela, V., Gaban, A. S., O’Neill, E., Joshi, L., et al. (2021). Microglial metabolism is a pivotal factor in sexual dimorphism in Alzheimer’s disease. Commun. Biol. 4:711. doi: 10.1038/s42003-021-02259-y
Guo, L., Zhong, M. B., Zhang, L., Zhang, B., and Cai, D. (2022). Sex differences in Alzheimer’s disease: insights from the multiomics landscape. Biol. Psychiatry 91, 61–71. doi: 10.1016/j.biopsych.2021.02.968
Holden, S., Kundu, P., Torres, E. R. S., Sudhakar, R., Krenik, D., Grygoryev, D., et al. (2022). Apolipoprotein E isoform-dependent effects on human amyloid precursor protein/Abeta-induced behavioral alterations and cognitive impairments and insoluble cortical Abeta 42 levels. Front. Aging Neurosci. 14:767558. doi: 10.3389/fnagi.2022.767558
Holland, D., Desikan, R. S., Dale, A. M., and McEvoy, L. K., Alzheimer’s Disease Neuroimaging (2013). Higher rates of decline for women and apolipoprotein E epsilon4 carriers. AJNR Am. J. Neuroradiol. 34, 2287–2293. doi: 10.3174/ajnr.A3601
Hoozemans, J. J., Rozemuller, A. J., van Haastert, E. S., Eikelenboom, P., and van Gool, W. A. (2011). Neuroinflammation in Alzheimer’s disease wanes with age. J. Neuroinflammation 8:171. doi: 10.1186/1742-2094-8-171
Hou, X., Adeosun, S. O., Zhang, Q., Barlow, B., Brents, M., Zheng, B., et al. (2015). Differential contributions of Apo E4 and female sex to BACE1 activity and expression mediate a deposition and learning and memory in mouse models of Alzheimer’s disease. Front. Aging Neurosci. 7:207. doi: 10.3389/fnagi.2015.00207
Hunsberger, H. C., Pinky, P. D., Smith, W., Suppiramaniam, V., and Reed, M. N. (2019). The role of APOE4 in Alzheimer’s disease: strategies for future therapeutic interventions. Neuronal Signals 3:NS20180203. doi: 10.1042/NS20180203
Huynh, T. V., Wang, C., Tran, A. C., Tabor, G. T., Mahan, T. E., Francis, C. M., et al. (2019). Lack of hepatic apo E does not influence early Abeta deposition: observations from a new APOE knock-in model. Mol. Neurodegener. 14:37. doi: 10.1186/s13024-019-0337-1
Jack, C. R. Jr., Wiste, H. J., Weigand, S. D., Knopman, D. S., Vemuri, P., Mielke, M. M., et al. (2015). Age, sex, and APOE epsilon4 effects on memory, brain structure, and beta-amyloid across the adult life span. JAMA Neurol. 72, 511–519. doi: 10.1001/jamaneurol.2014.4821
Jankowsky, J. L., and Zheng, H. (2017). Practical considerations for choosing a mouse model of Alzheimer’s disease. Mol. Neurodegener. 12:89. doi: 10.1186/s13024-017-0231-7
Jett, S., Malviya, N., Schelbaum, E., Jang, G., Jahan, E., Clancy, K., et al. (2022). Endogenous and exogenous estrogen exposures: how Women’s reproductive health can drive brain aging and inform Alzheimer’s prevention. Front. Aging Neurosci. 14:831807. doi: 10.3389/fnagi.2022.831807
Kawas, C., Gray, S., Brookmeyer, R., Fozard, J., and Zonderman, A. (2000). Age-specific incidence rates of Alzheimer’s disease: the Baltimore longitudinal study of aging. Neurology 54, 2072–2077. doi: 10.1212/wnl.54.11.2072
Klein, S. L., and Flanagan, K. L. (2016). Sex differences in immune responses. Nat. Rev. Immunol. 16, 626–638. doi: 10.1038/nri.2016.90
Kok, E., Haikonen, S., Luoto, T., Huhtala, H., Goebeler, S., Haapasalo, H., et al. (2009). Apolipoprotein E-dependent accumulation of Alzheimer disease-related lesions begins in middle age. Ann. Neurol. 65, 650–657. doi: 10.1002/ana.21696
Laws, K. R., Irvine, K., and Gale, T. M. (2016). Sex differences in cognitive impairment in Alzheimer’s disease. World J. Psychiatry 6, 54–65. doi: 10.5498/wjp.v6.i1.54
Levine, M. E., Lu, A. T., Chen, B. H., Hernandez, D. G., Singleton, A. B., Ferrucci, L., et al. (2016). Menopause accelerates biological aging. Proc. Natl. Acad. Sci. U. S. A. 113, 9327–9332. doi: 10.1073/pnas.1604558113
Li, X., Feng, Y., Wu, W., Zhao, J., Fu, C., Li, Y., et al. (2016). Sex differences between APPswePS1dE9 mice in A-beta accumulation and pancreatic islet function during the development of Alzheimer’s disease. Lab. Anim. 50, 275–285. doi: 10.1177/0023677215615269
Li, R., and Singh, M. (2014). Sex differences in cognitive impairment and Alzheimer’s disease. Front. Neuroendocrinol. 35, 385–403. doi: 10.1016/j.yfrne.2014.01.002
Liu, D. S., Pan, X. D., Zhang, J., Shen, H., Collins, N. C., Cole, A. M., et al. (2015). APOE4 enhances age-dependent decline in cognitive function by down-regulating an NMDA receptor pathway in EFAD-Tg mice. Mol. Neurodegener. 10:7. doi: 10.1186/s13024-015-0002-2
Locci, A., Orellana, H., Rodriguez, G., Gottliebson, M., McClarty, B., Dominguez, S., et al. (2021). Comparison of memory, affective behavior, and neuropathology in APP (NLGF) knock-in mice to 5xFAD and APP/PS1 mice. Behav. Brain Res. 404:113192. doi: 10.1016/j.bbr.2021.113192
Locke, P. A., Conneally, P. M., Tanzi, R. E., Gusella, J. F., and Haines, J. L. (1995). Apolipoprotein E4 allele and Alzheimer disease: examination of allelic association and effect on age at onset in both early-and late-onset cases. Genet. Epidemiol. 12, 83–92. doi: 10.1002/gepi.1370120108
Lynch, M. A. (2022). Exploring sex-related differences in microglia may be a game-changer in precision medicine. Front. Aging Neurosci. 14:868448. doi: 10.3389/fnagi.2022.868448
Marazuela, P., Paez-Montserrat, B., Bonaterra-Pastra, A., Sole, M., and Hernandez-Guillamon, M. (2022). Impact of cerebral amyloid Angiopathy in two transgenic mouse models of cerebral beta-amyloidosis: a neuropathological study. Int. J. Mol. Sci. 23:4972. doi: 10.3390/ijms23094972
Marottoli, F. M., Katsumata, Y., Koster, K. P., Thomas, R., Fardo, D. W., and Tai, L. M. (2017). Peripheral inflammation, apolipoprotein E4, and amyloid-beta interact to induce cognitive and cerebrovascular dysfunction. ASN Neuro 9:1759091417719201. doi: 10.1177/1759091417719201
Martinez-Martinez, A. B., Torres-Perez, E., Devanney, N., Del Moral, R., Johnson, L. A., and Arbones-Mainar, J. M. (2020). Beyond the CNS: the many peripheral roles of APOE. Neurobiol. Dis. 138:104809. doi: 10.1016/j.nbd.2020.104809
Middei, S., Daniele, S., Caprioli, A., Ghirardi, O., and Ammassari-Teule, M. (2006). Progressive cognitive decline in a transgenic mouse model of Alzheimer’s disease overexpressing mutant hAPPswe. Genes Brain Behav. 5, 249–256. doi: 10.1111/j.1601-183X.2005.00160.x
Mifflin, M. A., Winslow, W., Surendra, L., Tallino, S., Vural, A., and Velazquez, R. (2021). Sex differences in the Intelli cage and the Morris water maze in the APP/PS1 mouse model of amyloidosis. Neurobiol. Aging 101, 130–140. doi: 10.1016/j.neurobiolaging.2021.01.018
Mishra, S., Blazey, T. M., Holtzman, D. M., Cruchaga, C., Su, Y., Morris, J. C., et al. (2018). Longitudinal brain imaging in preclinical Alzheimer disease: impact of APOE epsilon4 genotype. Brain 141, 1828–1839. doi: 10.1093/brain/awy103
Mishra, A., Wang, Y., Yin, F., Vitali, F., Rodgers, K. E., Soto, M., et al. (2022). A tale of two systems: lessons learned from female mid-life aging with implications for Alzheimer’s prevention & treatment. Ageing Res. Rev. 74:101542. doi: 10.1016/j.arr.2021.101542
Montagne, A., Nikolakopoulou, A. M., Huuskonen, M. T., Sagare, A. P., Lawson, E. J., Lazic, D., et al. (2021). APOE4 accelerates advanced-stage vascular and neurodegenerative disorder in old Alzheimer’s mice via cyclophilin a independently of amyloid-beta. Nat. Aging 1, 506–520. doi: 10.1038/s43587-021-00073-z
Moser, V. A., and Pike, C. J. (2017). Obesity accelerates Alzheimer-related pathology in APOE4 but not APOE3 mice. eNeuro 4, ENEURO.0077–ENEU17.2017. doi: 10.1523/ENEURO.0077-17.2017
Neu, S. C., Pa, J., Kukull, W., Beekly, D., Kuzma, A., Gangadharan, P., et al. (2017). Apolipoprotein E genotype and sex risk factors for Alzheimer disease: a Meta-analysis. JAMA Neurol. 74, 1178–1189. doi: 10.1001/jamaneurol.2017.2188
Olesen, L. O., Bouzinova, E. V., Severino, M., Sivasaravanaparan, M., Hasselstrom, J. B., Finsen, B., et al. (2016). Behavioural phenotyping of APPswe/PS1deltaE9 mice: age-related changes and effect of long-term paroxetine treatment. PLoS One 11:e0165144. doi: 10.1371/journal.pone.0165144
Oveisgharan, S., Arvanitakis, Z., Yu, L., Farfel, J., Schneider, J. A., and Bennett, D. A. (2018). Sex differences in Alzheimer’s disease and common neuropathologies of aging. Acta Neuropathol. 136, 887–900. doi: 10.1007/s00401-018-1920-1
Palmer, J. A., Kaufman, C. S., Vidoni, E. D., Honea, R. A., Burns, J. M., and Billinger, S. A. (2022). Sex differences in resilience and resistance to brain pathology and dysfunction moderated by cerebrovascular response to exercise and genetic risk for Alzheimer’s disease. J. Alzheimers Dis. 90, 535–542. doi: 10.3233/JAD-220359
Pan, C. C., Chu, C. S., Chen, C. L., Chuang, Y. C., and Chen, N. C. (2021). Factors affecting rapid cognitive decline in patients with Alzheimer’s disease: a longitudinal follow-up study. Int. J. Environ. Res. Public Health 18:8576. doi: 10.3390/ijerph18168576
Polsinelli, A. J., Lane, K. A., Manchella, M. K., Logan, P. E., Gao, S., and Apostolova, L. G. (2023a). APOE epsilon4 is associated with earlier symptom onset in LOAD but later symptom onset in EOAD. Alzheimers Dement. 19, 2212–2217. doi: 10.1002/alz.12955
Polsinelli, A. J., Logan, P. E., Lane, K. A., Manchella, M. K., Nemes, S., Sanjay, A. B., et al. (2023b). APOE epsilon4 carrier status and sex differentiate rates of cognitive decline in early- and late-onset Alzheimer’s disease. Alzheimers Dement. 19, 1983–1993. doi: 10.1002/alz.12831
Poon, C. H., Wong, S. T. N., Roy, J., Wang, Y., Chan, H. W. H., Steinbusch, H., et al. (2023). Sex differences between neuronal loss and the early onset of amyloid deposits and behavioral consequences in 5xFAD transgenic mouse as a model for Alzheimer’s disease. Cells 12:780. doi: 10.3390/cells12050780
Qian, J., Betensky, R. A., Hyman, B. T., and Serrano-Pozo, A. (2021). Association of APOE genotype with heterogeneity of cognitive decline rate in Alzheimer disease. Neurology 96, e2414–e2428. doi: 10.1212/WNL.0000000000011883
Qian, J., Zhang, Y., Betensky, R. A., Hyman, B. T., and Serrano-Pozo, A. (2023). Neuropathology-independent association between APOE genotype and cognitive decline rate in the Normal aging-early Alzheimer continuum. Neurol. Genet. 9:e200055. doi: 10.1212/NXG.0000000000200055
Raber, J., Bongers, G., LeFevour, A., Buttini, M., and Mucke, L. (2002). Androgens protect against apolipoprotein E4-induced cognitive deficits. J. Neurosci. 22, 5204–5209. doi: 10.1523/JNEUROSCI.22-12-05204.2002
Raber, J., Wong, D., Buttini, M., Orth, M., Bellosta, S., Pitas, R. E., et al. (1998). Isoform-specific effects of human apolipoprotein E on brain function revealed in Apo E knockout mice: increased susceptibility of females. Proc. Natl. Acad. Sci. U. S. A. 95, 10914–10919. doi: 10.1073/pnas.95.18.10914
Rajan, K. B., Weuve, J., Barnes, L. L., McAninch, E. A., Wilson, R. S., and Evans, D. A. (2021). Population estimate of people with clinical Alzheimer’s disease and mild cognitive impairment in the United States (2020-2060). Alzheimers Dement. 17, 1966–1975. doi: 10.1002/alz.12362
Rao, R. V., Subramaniam, K. G., Gregory, J., Bredesen, A. L., Coward, C., Okada, S., et al. (2023). Rationale for a multi-factorial approach for the reversal of cognitive decline in Alzheimer’s disease and MCI: a review. Int. J. Mol. Sci. 24:1659. doi: 10.3390/ijms24021659
Rasmussen, K. L., Tybjaerg-Hansen, A., Nordestgaard, B. G., and Frikke-Schmidt, R. (2018). Absolute 10-year risk of dementia by age, sex and APOE genotype: a population-based cohort study. CMAJ 190, E1033–E1041. doi: 10.1503/cmaj.180066
Raulin, A. C., Doss, S. V., Trottier, Z. A., Ikezu, T. C., Bu, G., and Liu, C. C. (2022). Apo E in Alzheimer’s disease: pathophysiology and therapeutic strategies. Mol. Neurodegener. 17:72. doi: 10.1186/s13024-022-00574-4
Reale, M., Kamal, M. A., Velluto, L., Gambi, D., Di Nicola, M., and Greig, N. H. (2012). Relationship between inflammatory mediators, Abeta levels and Apo E genotype in Alzheimer disease. Curr. Alzheimer Res. 9, 447–457. doi: 10.2174/156720512800492549
Resnick, S. M., Bilgel, M., Moghekar, A., An, Y., Cai, Q., Wang, M. C., et al. (2015). Changes in Abeta biomarkers and associations with APOE genotype in 2 longitudinal cohorts. Neurobiol. Aging 36, 2333–2339. doi: 10.1016/j.neurobiolaging.2015.04.001
Riedel, B. C., Thompson, P. M., and Brinton, R. D. (2016). Age, APOE and sex: triad of risk of Alzheimer’s disease. J. Steroid Biochem. Mol. Biol. 160, 134–147. doi: 10.1016/j.jsbmb.2016.03.012
Ringman, J. M., Elashoff, D., Geschwind, D. H., Welsh, B. T., Gylys, K. H., Lee, C., et al. (2012). Plasma signaling proteins in persons at genetic risk for Alzheimer disease: influence of APOE genotype. Arch. Neurol. 69, 757–764. doi: 10.1001/archneurol.2012.277
Rocca, W. A., Grossardt, B. R., and Shuster, L. T. (2011). Oophorectomy, menopause, estrogen treatment, and cognitive aging: clinical evidence for a window of opportunity. Brain Res. 1379, 188–198. doi: 10.1016/j.brainres.2010.10.031
Rodrigue, K. M., Kennedy, K. M., and Park, D. C. (2009). Beta-amyloid deposition and the aging brain. Neuropsychol. Rev. 19, 436–450. doi: 10.1007/s11065-009-9118-x
Rodriguez, G. A., Tai, L. M., LaDu, M. J., and Rebeck, G. W. (2014). Human APOE4 increases microglia reactivity at Abeta plaques in a mouse model of Abeta deposition. J. Neuroinflammation 11:111. doi: 10.1186/1742-2094-11-111
Rosselli, M. C., Ardila, A. C., Moreno, S. C., Standish, V. C., Arango-Lasprilla, J. C., Tirado, V. M., et al. (2000). Cognitive decline in patients with familial Alzheimer’s disease associated with E280a presenilin-1 mutation: a longitudinal study. J. Clin. Exp. Neuropsychol. 22, 483–495. doi: 10.1076/1380-3395(200008)22:4;1-0;FT483
Ruan, D., and Sun, L. (2023). Amyloid-beta PET in Alzheimer’s disease: a systematic review and Bayesian meta-analysis. Brain Behav. 13:e2850. doi: 10.1002/brb3.2850
Sanchez, K., Wu, S. L., Kakkar, R., Darling, J. S., Harper, C. S., and Fonken, L. K. (2023). Ovariectomy in mice primes hippocampal microglia to exacerbate behavioral sickness responses. Brain Behav. Immun Health 30:100638. doi: 10.1016/j.bbih.2023.100638
Schmid, S., Rammes, G., Blobner, M., Kellermann, K., Bratke, S., Fendl, D., et al. (2019). Cognitive decline in Tg2576 mice shows sex-specific differences and correlates with cerebral amyloid-beta. Behav. Brain Res. 359, 408–417. doi: 10.1016/j.bbr.2018.11.022
Schmitz, C., and Hof, P. R. (2007). “Design-Based Stereology in Brain Aging Research” in Brain Aging: Models, Methods, and Mechanisms. ed. D. R. Riddle (Boca Raton, FL: CRC Press)
Shi, M., Chu, F., Zhu, F., and Zhu, J. (2022). Impact of anti-amyloid-beta monoclonal antibodies on the pathology and clinical profile of Alzheimer’s disease: a focus on Aducanumab and Lecanemab. Front. Aging Neurosci. 14:870517. doi: 10.3389/fnagi.2022.870517
Sil, A., Erfani, A., Lamb, N., Copland, R., Riedel, G., and Platt, B. (2022). Sex differences in behavior and molecular pathology in the 5XFAD model. J. Alzheimers Dis. 85, 755–778. doi: 10.3233/JAD-210523
Stephen, T. L., Breningstall, B., Suresh, S., McGill, C. J., and Pike, C. J. (2022). APOE genotype and biological sex regulate astroglial interactions with amyloid plaques in Alzheimer’s disease mice. J. Neuroinflammation 19:286. doi: 10.1186/s12974-022-02650-4
Stephen, T. L., Cacciottolo, M., Balu, D., Morgan, T. E., LaDu, M. J., Finch, C. E., et al. (2019). APOE genotype and sex affect microglial interactions with plaques in Alzheimer’s disease mice. Acta Neuropathol. Commun. 7:82. doi: 10.1186/s40478-019-0729-z
Streit, W. J., Mrak, R. E., and Griffin, W. S. (2004). Microglia and neuroinflammation: a pathological perspective. J. Neuroinflammation 1:14. doi: 10.1186/1742-2094-1-14
Strittmatter, W. J., Saunders, A. M., Schmechel, D., Pericak-Vance, M., Enghild, J., Salvesen, G. S., et al. (1993). Apolipoprotein E: high-avidity binding to beta-amyloid and increased frequency of type 4 allele in late-onset familial Alzheimer disease. Proc. Natl. Acad. Sci. U. S. A. 90, 1977–1981. doi: 10.1073/pnas.90.5.1977
Subramaniapillai, S., Almey, A., Natasha Rajah, M., and Einstein, G. (2021). Sex and gender differences in cognitive and brain reserve: implications for Alzheimer’s disease in women. Front. Neuroendocrinol. 60:100879. doi: 10.1016/j.yfrne.2020.100879
Suemoto, C. K., and Leite, R. E. P. (2023). Autopsy studies are key to identifying dementia cause. Lancet Healthy Longev 4, e94–e95. doi: 10.1016/S2666-7568(23)00022-3
Sullivan, P. M., Mezdour, H., Quarfordt, S. H., and Maeda, N. (1998). Type III hyperlipoproteinemia and spontaneous atherosclerosis in mice resulting from gene replacement of mouse Apoe with human Apoe*2. J. Clin. Invest. 102, 130–135. doi: 10.1172/JCI2673
Therriault, J., Benedet, A. L., Pascoal, T. A., Mathotaarachchi, S., Savard, M., Chamoun, M., et al. (2020). APOEepsilon4 potentiates the relationship between amyloid-beta and tau pathologies. Mol. Psychiatry 26, 5977–5988. doi: 10.1038/s41380-020-0688-6
Therriault, J., Pascoal, T. A., Benedet, A. L., Tissot, C., Savard, M., Chamoun, M., et al. (2021). Frequency of biologically defined Alzheimer disease in relation to age, sex, APOE epsilon4, and cognitive impairment. Neurology 96, e975–e985. doi: 10.1212/WNL.0000000000011416
Thomas, R., Morris, A. W. J., and Tai, L. M. (2017). Epidermal growth factor prevents APOE4-induced cognitive and cerebrovascular deficits in female mice. Heliyon 3:e00319. doi: 10.1016/j.heliyon.2017.e00319
Tzioras, M., Davies, C., Newman, A., Jackson, R., and Spires-Jones, T. (2019). Invited review: APOE at the interface of inflammation, neurodegeneration and pathological protein spread in Alzheimer’s disease. Neuropathol. Appl. Neurobiol. 45, 327–346. doi: 10.1111/nan.12529
Valencia-Olvera, A. C., Balu, D., Faulk, N., Amiridis, A., Wang, Y., Pham, C., et al. (2023). Inhibition of ACAT as a therapeutic target for Alzheimer’s disease is independent of Apo E4 Lipidation. Neurotherapeutics 20, 1120–1137. doi: 10.1007/s13311-023-01375-3
van Meer, P., Acevedo, S., and Raber, J. (2007). Impairments in spatial memory retention of GFAP-apo E4 female mice. Behav. Brain Res. 176, 372–375. doi: 10.1016/j.bbr.2006.10.024
Villemagne, V. L., Pike, K. E., Chetelat, G., Ellis, K. A., Mulligan, R. S., Bourgeat, P., et al. (2011). Longitudinal assessment of Abeta and cognition in aging and Alzheimer disease. Ann. Neurol. 69, 181–192. doi: 10.1002/ana.22248
Vitek, M. P., Christensen, D. J., Wilcock, D., Davis, J., Van Nostrand, W. E., Li, F. Q., et al. (2012). APOE-mimetic peptides reduce behavioral deficits, plaques and tangles in Alzheimer’s disease transgenics. Neurodegener Dis 10, 122–126. doi: 10.1159/000334914
Wang, J., Tanila, H., Puolivali, J., Kadish, I., and van Groen, T. (2003). Gender differences in the amount and deposition of amyloidbeta in APPswe and PS1 double transgenic mice. Neurobiol. Dis. 14, 318–327. doi: 10.1016/j.nbd.2003.08.009
Warren, S. L., Reid, E., Whitfield, P., Helal, A. M., Hamza, E. A. G., Tindle, R., et al. (2023). Cognitive and behavioral abnormalities in individuals with Alzheimer’s disease, mild cognitive impairment, and subjective memory complaints. Curr. Psychol. doi: 10.1007/s12144-023-04281-1
Webster, S. J., Bachstetter, A. D., Nelson, P. T., Schmitt, F. A., and Van Eldik, L. J. (2014). Using mice to model Alzheimer’s dementia: an overview of the clinical disease and the preclinical behavioral changes in 10 mouse models. Front. Genet. 5:88. doi: 10.3389/fgene.2014.00088
Webster, S. J., Bachstetter, A. D., and Van Eldik, L. J. (2013). Comprehensive behavioral characterization of an APP/PS-1 double knock-in mouse model of Alzheimer’s disease. Alzheimers Res. Ther. 5:28. doi: 10.1186/alzrt182
West, M. J. (2002). Design-based stereological methods for counting neurons. Prog. Brain Res. 135, 43–51. doi: 10.1016/S0079-6123(02)35006-4
Yang, L. G., March, Z. M., Stephenson, R. A., and Narayan, P. S. (2023). Apolipoprotein E in lipid metabolism and neurodegenerative disease. Trends Endocrinol. Metab. 34, 430–445. doi: 10.1016/j.tem.2023.05.002
Youmans, K. L., Leung, S., Zhang, J., Maus, E., Baysac, K., Bu, G., et al. (2011). Amyloid-beta 42 alters apolipoprotein E solubility in brains of mice with five familial AD mutations. J. Neurosci. Methods 196, 51–59. doi: 10.1016/j.jneumeth.2010.12.025
Youmans, K. L., Tai, L. M., Kanekiyo, T., Stine, W. B. Jr., Michon, S. C., Nwabuisi-Heath, E., et al. (2012a). Intraneuronal Abeta detection in 5xFAD mice by a new Abeta-specific antibody. Mol. Neurodegener. 7:8. doi: 10.1186/1750-1326-7-8
Youmans, K. L., Tai, L. M., Nwabuisi-Heath, E., Jungbauer, L., Kanekiyo, T., Gan, M., et al. (2012b). APOE4-specific changes in Abeta accumulation in a new transgenic mouse model of Alzheimer disease. J. Biol. Chem. 287, 41774–41786. doi: 10.1074/jbc.M112.407957
Zhang, Y., Chen, H., Li, R., Sterling, K., and Song, W. (2023). Amyloid beta-based therapy for Alzheimer’s disease: challenges, successes and future. Signal Transduct. Target. Ther. 8:248. doi: 10.1038/s41392-023-01484-7
Zhang, L., Xia, Y., and Gui, Y. (2023). Neuronal Apo E4 in Alzheimer’s disease and potential therapeutic targets. Front. Aging Neurosci. 15:1199434. doi: 10.3389/fnagi.2023.1199434
Keywords: Alzheimer’s disease, APOE4, female risk, transgenic mice, amyloid-beta
Citation: Balu D, Valencia-Olvera AC, Islam Z, Mielczarek C, Hansen A, Perez Ramos TM, York J, LaDu MJ and Tai LM (2023) APOE genotype and sex modulate Alzheimer’s disease pathology in aged EFAD transgenic mice. Front. Aging Neurosci. 15:1279343. doi: 10.3389/fnagi.2023.1279343
Edited by:
G. William Rebeck, Georgetown University, United StatesReviewed by:
Jacob Raber, Oregon Health and Science University, United StatesNahdia Jones, Imagen Technologies, Inc., United States
Copyright © 2023 Balu, Valencia-Olvera, Islam, Mielczarek, Hansen, Perez Ramos, York, LaDu and Tai. This is an open-access article distributed under the terms of the Creative Commons Attribution License (CC BY). The use, distribution or reproduction in other forums is permitted, provided the original author(s) and the copyright owner(s) are credited and that the original publication in this journal is cited, in accordance with accepted academic practice. No use, distribution or reproduction is permitted which does not comply with these terms.
*Correspondence: Leon M. Tai, bGVvbnRhaUB1aWMuZWR1