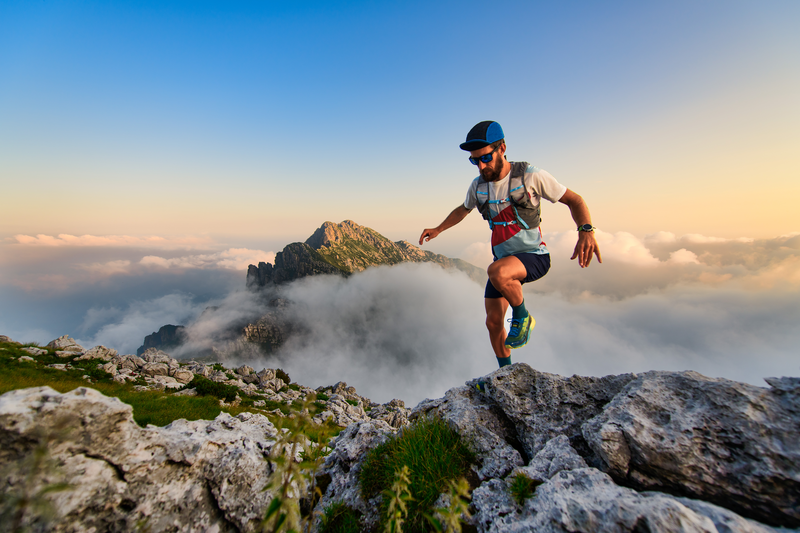
94% of researchers rate our articles as excellent or good
Learn more about the work of our research integrity team to safeguard the quality of each article we publish.
Find out more
BRIEF RESEARCH REPORT article
Front. Aging Neurosci. , 03 October 2023
Sec. Parkinson’s Disease and Aging-related Movement Disorders
Volume 15 - 2023 | https://doi.org/10.3389/fnagi.2023.1268751
Background: Parkinson’s disease (PD) is characterized by motor disorders and the composition of Lewy bodies (LBs) in the substantia nigra. Due to the lack of a definitive biomarker, the current treatments do not modify the progression of PD. Recently, researchers revealed lipid dysregulation and some potential volatile biomarkers of PD related to a unique odor from PD patients by metabolomics of sebum, which is supposed to cause a potential change for skin microflora. In this study, we identified the 4 Malassezia species in PD patients and compared them with healthy controls.
Methods: We collected 95 sebum samples (47 PDs and 48 Controls) by cotton swabs and extracted the DNA. The identification of Malassezia species was performed by Nested PCR. Specific primers for each species were used to amplify corresponding yeasts in each sample.
Results: M. restricta and M. globosa are the most common species for both groups. The prevalence of M. slooffiae and M. sympodialis were significantly higher in the PD group compared with controls (63.8% vs. 29.1 and 74.5% vs. 54.2% respectively), the binary logistic regression model further indicated that M. slooffiae (OR = 9.358, p < 0.001) was associated with PD. Moreover, the diversity of Malassezia species was significantly greater (3.5 vs. 2.9 species per individual, p = 0.002) in the PD group.
Conclusion: Based on our results, we preliminarily observed a change in Malassezia species incidence and diversity on the skin of PD patients, which could be associated with lipid dysregulation; meanwhile, it might also be a noninvasive biomarker for PD.
Parkinson’s disease (PD) is one of the most common progressive neurodegenerative disorders which is characterized by inducing motor disorders, rigidity, and tremor through the loss of dopaminergic neurons in the substantia nigra (Pisa et al., 2020; Niemann et al., 2021). PD affects more than 6 million people worldwide, of which the prevalence of patients over 65 years of age is about 1.8%, and as the aging demographics, the overall prevalence is expected to increase (Laurence et al., 2019; Pisa et al., 2020). Although it is currently known that the main pathological hallmark of PD is the formation of Lewy bodies, the etiology of PD still has not been fully understood.
In addition to the motor symptoms, non-motor symptoms and alterations of the autonomic nervous system are common in PD and may precede the diagnosis of PD. As early as 1927, Krestin reported that seborrheic facies as a cutaneous manifestation in PD patients (Ravn et al., 2017). Changes in the skin are common symptoms of PD patients; the skin of many patients is shiny, greasy, or flaky, especially on the face and scalp. Interestingly, Morgan reported that Joy Milne, who is a human super smeller, smelled a unique, musky odor on the skin of her husband with PD (Morgan, 2016). In the following pilot study, she successfully identified 11 out of 12 PD patients or control by smelling, except one misidentified control subject who also had the special odor; surprisingly, this control subject was later diagnosed with PD (Morgan, 2016). Barran et al. have indicated that the source of the odor was associated with altered biochemical composition of the sebum, and localized to the back of the neck (Trivedi et al., 2019). There are many sebaceous glands in this region of skin that produce sebum. We hypothesized that changes in the skin microflora of PD patients might be involved as well, such as Malaasezia.
Malassezia, lipophilic fungi, is the most prevalent member of cutaneous microbiota in normal humans and other animals (Laurence et al., 2019; Malinovská et al., 2019). The Malassezia yeasts currently include 17 species, the most frequently found species in humans include M. restricta, M. globosa, M. slooffiae, and M. sympodialis (Arendrup et al., 2014; Malinovská et al., 2019). Others, i.e., M. dermatis and M. japonica, occur in higher frequencies in East Asia only, while some, such as M. furfur are predominant in neonates (Boekhout et al., 2010; Velegraki et al., 2015). In humans, Malassezia inhabits the skin in sebum-rich areas, such as the face and scalp; and the distribution of Malassezia species varies by body part and age but not by gender (Prohic, 2014). In addition, under certain conditions, Malassezia is also an opportunistic organism and cause various skin disease, such as M. furfur (Malinovská et al., 2019). For example, Malassezia plays an important role in the development of seborrheic dermatitis (SD) which is a common chronic inflammatory skin disease (Ravn et al., 2017).
In this study, the main purpose is to detect the potential change in the distribution of Malassezia species in PD patients and controls. Considering the relationship between Malassezia and skin diseases, we collected the sebum samples from the individuals without skin disease and identified the distribution of Malassezia species using nested PCR.
A total of 95 sebum samples were collected by swabbing from 47 patients with PD and, 48 controls. The retroauricular skin was rubbed with a sterile cotton swab (HARTMANN, Germany), which was stored in a sterile 15 mL centrifuge tube at −80°C until DNA extraction. The clinical information of each participant is shown in Table 1. All PD patients were clinically diagnosed and treated in the Department of Neurology, University Hospital Bonn. Only PD patients without any overt, skin diseases, especially seborrheic dermatitis (SD), based on the clinical exam will be included. Meanwhile, the spouse control or age-matched healthy control individuals were also collected. The study received informed consent from all participants (ethics approval 145/17).
Table 1. Description of PD patients and controls, distribution of Malassezia species in PD patients and controls.
The DNA was extracted by a QIAamp DNA Mini Kit (QIAGEN N.V., Venlo, Netherlands) according to the manufacturer’s instructions; each DNA sample was eluted in 100ul AE buffer. Due to the high background DNA from human tissue, we chose a nested PCR approach to increase the specificity for Malassezia. The 1st PCR primers and species-specific primers for nested PCR were taken from the internal transcribed spacer region of the rRNA gene, according to Zhang, et al. (Hao et al., 2014).
The first amplification was performed in a 25ul PCR reaction system, the mixture consisted of 2 μL extracted DNA from each sample, 12.5ul 2× HotStarTaq Plus Master Mix (QIAGEN, Germany), 0.5 μL 10 μM F primer and R primer (F: 5’-ACCTGCAGAAGGAT CATTAGTGA-3′ and R: 5’-TCCTCCGCTTATTGATATGC-3′), add water to 25ul. PCR was conducted in a Thermal Cycler (Biometra TAdvanced, Analytik Jena AG, Germany) with a program consisting of an initial denaturation for 5 min at 95°C, followed by 25 cycles of 95°C for 30s, 52°C for 45 s, 72°C for 1 min, and a 7 min final extension at 72°C.
For the second amplification, one forward primer (Malassezia 2nd F: 5′- GTGAATTGCAGAATTCCGTGAAT −3′) was combined with one of the following species-specific reverse primers:
M. restricta R: 5’-GCGAGCCTGTGCTAGGTA-3′.
M. globosa R: 5’-GAGCTTTTTCTAGAGAAGAAAAG-3′.
M. slooffiae R: 5’-CTTTTCGAGCGAGCCTACCAA-3′.
M. sympodialis R: 5’-TACAATCCCCAGGCAGCAA-3’.
Specifically, 1 μL unpurified 1st PCR product was amplified in a 25ul reaction mixture, the mixture also included 12.5ul 2× HotStarTaq Plus Master Mix, 0.5 μL 10 μM F primer and R primer, 2.5ul Coraload, add water to 25ul. Thermocycling conditions consisted of an initial denaturation for 5 min at 95°C, followed by 25 cycles of 95°C for 30s, 58°C for 45 s, and 72°C for 45 s, and a 7 min final extension at 72°C. Positive and negative controls were included in each amplification. Sizes of the expected products were confirmed by agarose gel electrophoresis. The specificity of the different primer pairs was verified by sequencing (not shown).
We applied PCR product cloning to synthesize the positive controls for each Malassezia species. The individual Malassezia species PCR fragments were purified from agarose gel by Gel DNA Recovery Kit (ZYMO RESEARCH, United States) and cloned in pJET1.2 using the CloneJET PCR Cloning Kit (Thermo Scientific, United States) according to the manufacturer’s instructions. Clones were sequenced (Eurofins Genomics) and inserted fragments verified by BLAST search.1 In addition, the specificity of each positive control was also confirmed by PCR.
SPSS 21.0 statistical analysis software was used to analyze the experimental data. Age and Malassezia species diversity are presented as the mean ± standard deviation, categorical variables are presented as the percentage. The Wilcoxon rank sum test was applied to analyze the age and Malassezia species diversity. The chi-square test was applied to analyze the differences in the categorical variables including different Malassezia species and sex. The binary logistic regression model was performed to explore the potential Malassezia species related to PD. The diagnosis of PD served as a dependent variable; sex, M. slooffiae, and M. sympodialis as independent variables. Values were considered significant for p < 0.05.
The specificity of different primer pairs was confirmed by sequencing, the four species-specific primer pairs are specific to the corresponding Malassezia species. Meanwhile, the four positive controls can only be amplified by the matched species-specific primer pairs (Figure 1).
Figure 1. The DNA fragments of positive controls and 4 Malassezia species in PD patients and controls. (A–D) The positive controls of M. Restricta (183 bp, A), M. globosa (187 bp, B), M. Sloofiae (192 bp, C), and M. Sympodialis (171 bp, D) can only be amplified by the matched species-specific primer pairs (Lane M: 100 bp ladder; Lanes 1–5: M. restricta, M. globosa, M. slooffiae, M. sympodialis, negative control.
We collected 95 sebum samples from 47 PD patients and 48 controls. There is no difference in age between the PD and control group, but there are more male participants in the PD group compared with controls (p < 0.05). The diversity of Malassezia species was significantly greater (3.5 vs. 2.9 species per individual, p < 0.05) in the PD group (Table 1).
After the second amplification, there are specific bands for each Malassezia species. Representative results of 10 PD patients and controls are displayed in Figures 2A,B.
Figure 2. The positive rates and DNA fragments of four Malassezia species in PD patients and controls. (A) The DNA fragments of four Malassezia species in 10 controls (Lanes1–12: Controls1–10, positive control and negative control, M: DNA ladder, upper band 200 bp, lower band 100 bp). (B) The DNA fragments of four Malassezia species in 10 PD patients (Lanes1–12: PD patirents1–10, positive control and negative control, M: DNA ladder, upper band 200 bp, lower band 100 bp). (C) The positive rates of different Malassezia species (***p < 0.001; *p < 0.05).
M. restricta and M. globosa are the most common two Malassezia species with a prevalence of 100% in PDs and controls (Figure 2C). Interestingly, the positive rates of M. slooffiae and M. sympodialis were 63.8 and 74.5% in PD patients respectively, which are significantly higher than in controls (p < 0.05) (Figure 2C). Meanwhile, the logistic regression model indicated that the high incidence of M. slooffiae (OR = 9.358, p < 0.05) was associated with PD, but not M. sympodialis. In addition, due to the different sex distribution between the two groups, we further analyzed the positive rate of M. slooffiae in males and females between PD and control groups, respectively. There is a similarly rising trend for its incidence in both sexes in PD compared with the control group (Table 2).
To date, the diagnosis of PD mainly depends on the clinical motor symptoms; no cheap, reliable, and non-invasive biomarker is available to support its clinical diagnosis. Regrettably, almost half of the dopamine neurons are lost before motor symptoms emerge, thus a tool for early, pre-motor symptom diagnosis is urgently needed. In this study, we observed a change in Malassezia species diversity and incidence in PD patients based on a non-culture method, which could evolve into a potential non-invasive biomarker of PD.
The concept of skin as a mirror of PD pathology can be traced back to the early 20th century (Arsic Arsenijevic et al., 2014). Regarding the study in the last years, peripheral autonomic dysfunction may be an important source of cutaneous problems, such as impaired sweating and sebum production dysregulation (Dabby et al., 2006). The reduction of epidermal nerve fiber density and the loss of dermal nerve fiber in PD has been demonstrated before (Dabby et al., 2006; Navarro-Otano et al., 2015). Alpha-synuclein is the main protein component of Lewy bodies, its deposits in the central nervous system (CNS) have already been implicated in the pathogenesis of PD. Meanwhile, several studies repeatedly reported that misfolded alpha-synuclein deposit was also found in the peripheral autonomic nervous system, including skin biopsy (Donadio et al., 2014,2016; Doppler et al., 2014; Fayyad et al., 2019; Ma et al., 2019). Perhaps, alpha-synuclein deposits could contribute to the cutaneous autonomic dysfunction (Zange et al., 2015), which still needs to be further determined. Furthermore, some skin diseases such as Seborrheic dermatitis (SD) Bullous pemphigoid, and Melanoma are overrepresented in PD, which may be a long-term effect of autonomic dysfunction (Niemann et al., 2021). These emerging pieces of evidence indicated that the skin could also be a helpful tool in the diagnosis of PD.
Malassezia, as a lipid-dependent fungus, depends on host lipids for survival by secreting extracellular lipases (Laurence et al., 2019). Previously, most of the focus has been on Malassezia and related skin diseases such as SD. Interestingly, Tanner et al. found that the risk of PD is increased for individuals with a diagnosis of SD and SD might be a potential premotor feature of PD (Tanner et al., 2012); and Arsenijevic et al. reported that there is a positive correlation between SD, PD, and M. globosa presence and density (Arsic Arsenijevic et al., 2014). Seborrhea, defined as an elevated sebum secretion rate (SER), was also reported in the last century in PD patients (Laurence et al., 2019). Nevertheless, it’s notable that the relationship among SD, SER, and PD remains to be elucidated. Increasingly evidence suggests that SD and SER are completely different clinical entities; and SER in PD patients is also controversial, which is only found in some studies (Burton and Pye, 1983; Cowley et al., 1990; Laurence et al., 2019). Furthermore, several lines of evidence supported that Malassezia may directly contribute to PD: (1) many PD risk alleles affect the metabolism of lipids, (2) the invasiveness of Malassezia is stimulated by L-DOPA, and (3) low CD4 + T cell counts observed in PD might lead to the over-proliferation of Malassezia (Laurence et al., 2019). Sinclair et al. also revealed lipid dysregulation in PD patients’ skin by performing an LC–MS analysis of sebum (Sinclair et al., 2021). It could lead to a preference for particular Malassezia species (in PD patients), which may also account for the increased prevalence of SD in PD patients. Therefore, it is worth detecting whether PD will directly affect the presence and diversity of Malassezia. To our knowledge, this is the first study to detect the diversity of Malassezia species based on non-culture methods in PD patients without skin disease.
In our study, we observed that the predominant Malassezia species are M. restricta and M. globosa in PD patients and controls; the positive rate of M. sloofiae and M. sympodialis is significantly higher in the PD group compared to controls. According to the binary logistic regression model, we found a high incidence of M. sloofiae associated with PD. Moreover, the diversity of Malassezia species found in the PD group was significantly greater (3.5 species per individual) than in the group of controls (2.9 species per individual). As we mentioned above, dysregulated lipid metabolism and increased sebum secretion in PD patients’ skin likely underlie this phenomenon. Recently, Barran et al. discovered some potential volatile biomarkers of PD from sebum, and the smell of which was also described as a similar odor of PD patients by Joy; especially, eicosane and octadecanal, which are up-regulated in PD subjects (Trivedi et al., 2019). The elevated production of these highly lipophilic molecules may in part contribute to the prevalence of different Malassezia species since the specific exogenous lipids are required for its growth (Trivedi et al., 2019), which still needs to be further investigated. Therefore, our results in part proved Barran’s hypothesis that the change of these volatile substances and odor in PD patients suggests a change of skin microflora and skin physiology that is highly specific to PD.
Interestingly, although Malassezia is thought to be limited to the skin before, more and more studies reported finding Malassezia in internal organs, including in the central nervous system (CNS) of patients with certain neurodegenerative disorders. A recently published comparative study reported that the incidence of Malassezia in the CNS of multiple sclerosis is higher compared to the controls (Laurence et al., 2019). Another study found the presence of mixed microbes in the CNS of PD patients, including Malassezia (Pisa et al., 2020). Although these studies provide preliminary evidence that Malassezia may also be involved in the pathogenesis of PD and other neurodegenerative disorders, caution is warranted with regard to the presumed presence of microbes in brains.
Furthermore, there are still some limitations in our study, and further studies in a large asymptomatic cohort of the elderly are needed to determine their causal association and whether similar changes might precede the onset of motor symptoms. In addition, we are going to establish an approach to detect the prevalence of Malassezia and other cutaneous microbiota in PD by next-generation sequencing (NGS).
The raw data supporting the conclusions of this article will be made available by the authors, without undue reservation.
The studies involving humans were approved by the Clinical Ethics Committee, University Hospital Bonn. The studies were conducted in accordance with the local legislation and institutional requirements. Written informed consent for participation was not required from the participants or the participants’ legal guardians/next of kin because we just collect the sebum samples from the skin by swabs, which is non-invasive; at the same time, we obtained the verbal consent of the patients.
XH: Conceptualization, Data curation, Investigation, Writing – original draft, Writing – review & editing, Methodology. JB: Conceptualization, Writing – review & editing. SP-S: Methodology, Writing – review & editing. IS: Writing – review & editing, Data curation. UW: Conceptualization, Project administration, Writing – review & editing.
The author(s) declare that no financial support was received for the research, authorship, and/or publication of this article.
Thanks for each participant that joined this research. Thanks for the methodology support offered by UW and SP-S. Thanks for the department of neurology, University hospital of Bonn.
The authors declare that the research was conducted in the absence of any commercial or financial relationships that could be construed as a potential conflict of interest.
All claims expressed in this article are solely those of the authors and do not necessarily represent those of their affiliated organizations, or those of the publisher, the editors and the reviewers. Any product that may be evaluated in this article, or claim that may be made by its manufacturer, is not guaranteed or endorsed by the publisher.
Arendrup, M. C., Boekhout, T., Akova, M., Meis, J. F., Cornely, O. A., Lortholary, O., et al. (2014). ESCMID and ECMM joint clinical guidelines for the diagnosis and management of rare invasive yeast infections. Clin. Microbiol. Infect. 20, 76–98. doi: 10.1111/1469-0691.12360
Arsic Arsenijevic, V. S., Milobratovic, D., Barac, A. M., Vekic, B., Marinkovic, J., and Kostic, V. S. (2014). A laboratory-based study on patients with Parkinson’s disease and seborrheic dermatitis: the presence and density of Malassezia yeasts, their different species and enzymes production. BMC Dermatol. 14:5. doi: 10.1186/1471-5945-14-5
Boekhout, T., Guého-Kellermann, E., Mayser, P., and Velegraki, A. (2010). Malassezia and the skin: science and clinical practice. Berlin: Springer Verlag.
Burton, J., and Pye, R. (1983). Seborrhoea is not a feature of seborrhoeic dermatitis. Br. Med. J. (Clin. Res. Ed.) 286, 1169–1170. doi: 10.1136/bmj.286.6372.1169
Cowley, N., Farr, P., and Shuster, S. (1990). The permissive effect of sebum in seborrhoeic dermatitis: an explanation of the rash in neurological disorders. Br. J. Dermatol. 122, 71–76. doi: 10.1111/j.1365-2133.1990.tb08241.x
Dabby, R., Djaldetti, R., Shahmurov, M., Treves, T. A., Gabai, B., Melamed, E., et al. (2006). Skin biopsy for assessment of autonomic denervation in Parkinson’s disease. J. Neural Transm. 113, 1169–1176. doi: 10.1007/s00702-005-0431-0
Donadio, V., Incensi, A., Leta, V., Giannoccaro, M. P., Scaglione, C., Martinelli, P., et al. (2014). Skin nerve α-synuclein deposits: a biomarker for idiopathic Parkinson disease. Neurology 82, 1362–1369. doi: 10.1212/WNL.0000000000000316
Donadio, V., Incensi, A., Piccinini, C., Cortelli, P., Giannoccaro, M. P., Baruzzi, A., et al. (2016). Skin nerve misfolded α-synuclein in pure autonomic failure and P arkinson disease. Ann. Neurol. 79, 306–316. doi: 10.1002/ana.24567
Doppler, K., Ebert, S., Uceyler, N., Trenkwalder, C., Ebentheuer, J., Volkmann, J., et al. (2014). Cutaneous neuropathy in Parkinson’s disease: a window into brain pathology. Acta Neuropathol. 128, 99–109. doi: 10.1007/s00401-014-1284-0
Fayyad, M., Salim, S., Majbour, N., Erskine, D., Stoops, E., Mollenhauer, B., et al. (2019). Parkinson’s disease biomarkers based on alpha-synuclein. J. Neurochem. 150, 626–636. doi: 10.1111/jnc.14809
Hao, Z., Yu-Ping, R., Zhen, X., Ya-Ling, D., and Dermatovenerology, D. O. (2014). Identification of Malassezia microflora in human with culture-independent method. Chin. J. Dermat. 28, 242–247.
Laurence, M., Benito-Leon, J., and Calon, F. (2019). Malassezia and Parkinson’s disease. Front. Neurol. 10:758. doi: 10.3389/fneur.2019.00758
Ma, L. Y., Liu, G. L., Wang, D. X., Zhang, M. M., Kou, W. Y., and Feng, T. (2019). Alpha-synuclein in peripheral tissues in Parkinson’s disease. ACS Chem. Neurosci. 10, 812–823. doi: 10.1021/acschemneuro.8b00383
Malinovská, Z., Onková, E., Váczi, P., Harárová, M., and Bhmová, E. (2019). Occurrence of Malassezia Spp. on healthy human skin. Folia Veterinaria 63, 54–59. doi: 10.2478/fv-2019-0038
Morgan, J. (2016). Joy of super smeller: sebum clues for PD diagnostics. Lancet Neurol. 15, 138–139. doi: 10.1016/S1474-4422(15)00396-8
Navarro-Otano, J., Casanova-Molla, J., Morales, M., Valls-Sole, J., and Tolosa, E. (2015). Cutaneous autonomic denervation in Parkinson’s disease. J. Neural Transm. (Vienna) 122, 1149–1155. doi: 10.1007/s00702-014-1355-3
Niemann, N., Billnitzer, A., and Jankovic, J. (2021). Parkinson’s disease and skin. Parkinsonism Relat. Disord. 82, 61–76. doi: 10.1016/j.parkreldis.2020.11.017
Pisa, D., Alonso, R., and Carrasco, L. (2020). Parkinson’s disease: a comprehensive analysis of fungi and bacteria in brain tissue. Int. J. Biol. Sci. 16, 1135–1152. doi: 10.7150/ijbs.42257
Prohic, A. (2014). Distribution of Malassezia species on healthy human skin in Bosnia and Herzegovina: correlation with body part, age and gender. Iran. J. Microbiol. 6, 253–262.
Ravn, A. H., Thyssen, J. P., and Egeberg, A. (2017). Skin disorders in Parkinson’s disease: potential biomarkers and risk factors. Clin. Cosmet. Investig. Dermatol. 10, 87–92. doi: 10.2147/CCID.S130319
Sinclair, E., Trivedi, D. K., Sarkar, D., Walton-Doyle, C., Milne, J., Kunath, T., et al. (2021). Metabolomics of sebum reveals lipid dysregulation in Parkinson’s disease. Nat. Commun. 12:1592. doi: 10.1038/s41467-021-21669-4
Tanner, C., Albers, K., Goldman, S., Fross, R., Leimpeter, A., Klingman, J., et al. (2012). Seborrheic dermatitis and risk of future Parkinson’s disease (PD) (S42. 001). Neurology. 78:S42.001. doi: 10.1212/WNL.78.1_MeetingAbstracts.S42.001
Trivedi, D. K., Sinclair, E., Xu, Y., Sarkar, D., Walton-Doyle, C., Liscio, C., et al. (2019). Discovery of volatile biomarkers of Parkinson’s disease from sebum. ACS Cent. Sci. 5, 599–606. doi: 10.1021/acscentsci.8b00879
Velegraki, A., Cafarchia, C., Gaitanis, G., Iatta, R., and Boekhout, T. (2015). Malassezia infections in humans and animals: pathophysiology, detection, and treatment. PLoS Path. 11:e1004523. doi: 10.1371/journal.ppat.1004523
Keywords: Parkinson’s disease, Malassezia, odor, sebum, biomarker
Citation: Han X, Bedarf J, Proske-Schmitz S, Schmitt I and Wüllner U (2023) Increased diversity of Malassezia species on the skin of Parkinson’s disease patients. Front. Aging Neurosci. 15:1268751. doi: 10.3389/fnagi.2023.1268751
Received: 02 August 2023; Accepted: 14 September 2023;
Published: 03 October 2023.
Edited by:
Wenquan Zou, The First Affiliated Hospital of Nanchang University, ChinaReviewed by:
Vincenzo Donadio, IRCCS Institute of Neurological Sciences of Bologna (ISNB), ItalyCopyright © 2023 Han, Bedarf, Proske-Schmitz, Schmitt and Wüllner. This is an open-access article distributed under the terms of the Creative Commons Attribution License (CC BY). The use, distribution or reproduction in other forums is permitted, provided the original author(s) and the copyright owner(s) are credited and that the original publication in this journal is cited, in accordance with accepted academic practice. No use, distribution or reproduction is permitted which does not comply with these terms.
*Correspondence: Xinyu Han, aHh5bWVkQHllYWgubmV0; Ullrich Wüllner, dWxscmljaC53dWVsbG5lckB1a2Jvbm4uZGU=
Disclaimer: All claims expressed in this article are solely those of the authors and do not necessarily represent those of their affiliated organizations, or those of the publisher, the editors and the reviewers. Any product that may be evaluated in this article or claim that may be made by its manufacturer is not guaranteed or endorsed by the publisher.
Research integrity at Frontiers
Learn more about the work of our research integrity team to safeguard the quality of each article we publish.