- 1Department of Neurology, University of Virginia, Charlottesville, VA, United States
- 2Department of Psychology, University of Virginia, Charlottesville, VA, United States
- 3Department of Psychology, University of Southern California, Los Angeles, CA, United States
Introduction: Social isolation is one of the strongest predictors of increased risk of mortality in older adulthood. The ability to form and maintain the social relationships that mitigate this risk is partially regulated by the oxytocinergic system and one’s ability to attend to and process social information. We have previously shown that an epigenetic change to the DNA of the oxytocin receptor gene (OXTR methylation) affects the salience of social information in young adults. Little is known about how the oxytocinergic system ages and what effect this aging system has on social cognitive abilities throughout the lifespan.
Methods: Here we explored age-related differences in the association between neural response during selective social attention and OXTR DNA methylation in young (age 18–31) and older (age 58-81) adults. Participants underwent fMRI during a selective social attention task and provided a DNA sample for the assessment of OXTR methylation.
Results and Discussion: We found that older adults activated diffuse areas of visual cortex and dorsolateral prefrontal cortex during selective social attention, consistent with the dedifferentiation and compensatory neural activation commonly reported in aging. We found a significant age-by-OXTR methylation interaction on neural response when attending to social stimuli in a complex display; young adults displayed a positive association between OXTR methylation and neural activation, replicating our prior finding that young adults with presumed diminished endogenous access to oxytocin recruit regions of the attentional cortex to a greater extent. This association did not hold for older adults. Instead, perceived social support interacted with OXTR methylation to influence neural response during selective social attention. These data suggest that environmental factors like social support moderate biological processes in aging and highlight the importance of a lifespan perspective for understanding associations between individual differences in the oxytocinergic system, neural function, and social behavior.
Introduction
Understanding the neurobiological factors that enable successful social functioning across the lifespan has important psychological and public health implications (Fabiani, 2012). The failure to form adequate social relationships is one of the greatest risk factors for mortality, akin to smoking 15 cigarettes a day (Holt-Lunstad et al., 2010). Maintaining strong social ties is particularly important for older adults (Matt and Dean, 1993; Seeman, 2000). Older individuals without a strong social support network suffer from an increased risk of dementia, heart disease, stroke, and suicide (Kuiper et al., 2015; Valtorta et al., 2016; Calati et al., 2019; National Academies and of Sciences, Engineering, and Medicine, 2020). Aging is accompanied by cognitive decline (Salthouse, 2010), including within the domains of social cognition (Ruffman et al., 2008; Henry et al., 2013) and attentional control (Madden et al., 2005; Zanto and Gazzaley, 2014). These forms of cognitive decline threaten older adults’ ability to recognize and attend to important social cues including human faces (Boutet et al., 2015; Meinhardt-Injac et al., 2017), a skill which is critical for the formation of social relationships that impact both physical and mental health throughout the lifespan (Holt-Lunstad et al., 2010).
Multiple streams of incoming information simultaneously compete for our attention. Because our perceptual and cognitive systems have a limited processing capacity, we must selectively attend to stimuli that are relevant to the task at hand while simultaneously ignoring distracting information (Desimone and Duncan, 1995). Salient information automatically captures attention without the need to exert volitional or top-down attentional resources. As highly social beings, social stimuli such as faces are often particularly relevant and considered an intrinsically salient class of stimuli (Theeuwes and Van der Stigchel, 2006; Bindemann et al., 2007; Langton et al., 2008; Wang and Adolphs, 2017). However, the extent to which social information automatically captures attention varies across the lifespan and between individuals (Klin et al., 2002; Meinhardt-Injac et al., 2017).
Additionally, our socio-cognitive abilities and motivations change across the lifespan. While affective empathy and social competencies generally improve with age, abilities to detect cues in human emotion and accurately attribute mental states to others decrease in older adulthood (Grainger et al., 2018). These changes may be impacted by both age-related motivational changes and changes in attentional control systems. For example, eye-tracking research indicates that older adults attend less to faces during conversations compared to adolescents and young adults (Grainger et al., 2018; De Lillo et al., 2021). According to socioemotional selectivity theory, older adults are more motivated to focus attention on familiar social partners, as compared to younger adults who are more motivated to engage with unfamiliar persons (Carstensen, 1992). However, little research exists examining the neural correlates of changes to social attention across the lifespan and how these changes might impact social support networks.
Individual differences in social attentional abilities across the lifespan may be in part driven by variability within the endogenous oxytocinergic system. Oxytocin is a naturally occurring mammalian hormone and neuromodulator critical for regulating a host of social behaviors across species (Ross and Young, 2009; Carter, 2014). It is hypothesized that oxytocin exerts its effects on social behavior by boosting the salience of social information (Shamay-Tsoory and Abu-Akel, 2016). For example, administration of exogenous oxytocin enhances signal detection for facial expressions (Schulze et al., 2011), biological motion (Kéri and Benedek, 2009), and social vocalizations (Marlin et al., 2015). The actions of oxytocin are dependent upon its receptor, which is expressed at varying levels across individuals. DNA methylation of the oxytocin receptor gene (OXTR) is partially responsible for this variability in expression. Our group has identified a cytosine-phosphate-guanine (CpG) site within the promoter of OXTR, site −934 (Gregory et al., 2009) (hg38_chr3:8,769,121-8,769,122), that displays wide variability in methylation levels across individuals (Puglia et al., 2015, 2018). Methylation at this site is significantly and negatively associated with OXTR expression in the human cortex (Gregory et al., 2009) and is therefore thought to play a regulatory role in gene transcription and the endogenous availability of oxytocin.
We have previously shown in a sample of healthy young adults that differences in neural response during selective social attention is associated with OXTR methylation at site −934 (Puglia et al., 2018). Specifically, we found that young adults with higher levels of OXTR methylation – i.e., presumed decreased ability to use endogenous oxytocin – recruited regions of the attentional control network to a greater extent when selectively attending to social stimuli within a complex display. The attentional control network is involved in the endogenous, volitional direction of attention and includes dorsolateral, prefrontal, and parietal cortices as key nodes (Hopfinger et al., 2000; Seeley et al., 2007). These results suggest that individuals with presumed diminished sensitivity to endogenous oxytocin (higher levels of OXTR methylation) fail to find social information intrinsically salient and therefore engage additional, compensatory neural mechanisms to attend to social information.
While a few studies have begun to investigate how the endogenous oxytocinergic system ages (Ebner et al., 2013, 2014; Huffmeijer et al., 2013; Sannino et al., 2017), no study to date has examined how aging impacts associations between the oxytocinergic system and neural function during social processes. Here we aimed to investigate how the aging brain maintains attention to social information presented within a complex display, and whether OXTR methylation is associated with social signal detection across adulthood. Additionally, we explored the moderating effect of social support in older adulthood on the relationship between OXTR methylation and neural response during selective social attention.
Methods
Participants
All participants provided written informed consent to participate in these studies which were reviewed and approved by the University of Virginia Institutional Review Board for Health Sciences Research. One hundred-three (48 males) younger adults aged 18–31 years (M = 21.14) and 88 (28 males) older adults aged 58–81 years (M = 68.27) participated in the present study. To control for potential population stratification artifacts related to epigenetic testing, only individuals who self-identified as white and of European descent were included in analyses. Older adults were recruited as a subset from the Virginia Cognitive Aging Project (VCAP), a longitudinal aging study ongoing since 2001. VCAP participants were determined to be cognitively normal (i.e., scoring above the mild cognitive impairment cutoff of 24; M = 29.24, SD = 0.93) on the Mini-Mental Status Exam (Folstein et al., 1975), and self-reported to be in very good health (Siedlecki et al., 2008; Likert scale from (1) excellent to (5) poor, M = 1.88, SD = 0.83). Additional cognitive related data including the Trail Making Test A assessing processing speed and working memory (Salthouse et al., 2000; Salthouse, 2012), the Trail Making Test B assessing executive functioning (Salthouse et al., 2000), estimated IQ (Salthouse, 2014), and years of completed education for these participants is provided in Table 1. Data from 54 young adults were collected previously and reported in Puglia et al. (2018). An additional 49 young adults were recruited as a replication sample and the combined young adult sample was used for age-based comparisons for the current study.
Epigenotyping
Participants provided 8 mL of blood in either mononuclear cell preparation tubes (BD Biosciences, Franklin Lanes, NJ) for assessment of peripheral blood mononuclear cell (PBMC) methylation (younger adults), or PAXGene Blood DNA Tubes (Qiagen, Valencia, CA) for assessment of whole blood methylation (older adults). An independent tissue comparison sample consisting of 156 adult participants (76 males) aged 16–64 (M = 21.78) years provided both sample types to determine whether methylation values differ across tissue collection methods. DNA was isolated and subjected to bisulfite treatment which converts non-methylated cytosines to uracil and leaves methylated cytosines unmodified. We then amplified a 116-base pair region of OXTR containing CpG site-934 (Gregory et al., 2009) (hg38, chr3: 8,769,121) using polymerase chain reaction, and assessed methylated cytosines via pyrosequencing. Reported epigenotypes are an average of three replicates.
Selective social attention task
Participants underwent a 1-back selective social attention task previously described in Puglia et al. (2018) in which participants were presented with double-exposure images of a face overlaid with a house while undergoing fMRI. Each block consisted of 10 serially-presented images and 4 to 5 “same” 1-back hits. Images were presented for 1800 ms with an inter-stimulus interval ranging from 200 to 2,400 ms during which a white crosshair was displayed on a black background at center fixation. At the start of each 40-s block, participants were instructed to attend to either the face (6 blocks) or the house (6 blocks) in the stimulus while completing the 1-back task in alternating blocks. For example, if the block started with an “Attend Faces” prompt, participants were instructed to report whether the face depicted in the image was the same or different from the face depicted in the prior image. Participants responded “same” or “different” via button press while the image was still on the screen. Before entering the scanner, participants completed a practice double-exposure 1-back task to ensure they understood task instructions in which they viewed letters and numbers superimposed upon each other. As in the experimental task, each block began with a prompt to attend to either the letter or the number in the stimulus when making the same-different 1-back decision. Participants repeated the practice task as necessary until reaching an accuracy of 70% or higher.
The key manipulation in this task was one of selective attention. Although participants were always simultaneously viewing both faces and houses, attending to the faces enhances face-specific neural responses and activation within face-perception regions (O’Craven et al., 1999; Furey et al., 2006; Herrington et al., 2015; Puglia et al., 2018) to a greater extent as compared to the attend houses conditions. Individuals with possible deficits in the intrinsic salience of social information, including individuals with autism spectrum disorder (Herrington et al., 2015) and those with higher levels of OXTR methylation (Puglia et al., 2018) require the recruitment of additional attentional control regions to attend to faces relative to houses. We tested for associations between age and task accuracy (i.e., the proportion of items correct) with logistic regression models in R (Crawley, 2007; R Core Team, 2020).
Social support questionnaire
Participants in VCAP have been completing the Social Support Questionnaire (Shaw et al., 2007) at each study visit since 2009. This questionnaire assesses embeddedness within one’s social network, as well as the amount of social support enacted, provided, and perceived. Scores can range from 13 to 116, with higher scores indicating greater engagement within a more supportive social network. Two participants recruited for the older adult sample in the present study did not have Social Support Questionnaire data. All other older adult participants completed the questionnaire 1 to 5 times (M = 2.51) since 2009. Average social support scores ranged from 36 to 98.5.
Image acquisition and preprocessing
Imaging acquisition details for the original young adult sample are detailed in Puglia et al. (2018). Scanning for the young adult replication sample and the older adult sample was performed at the University of Virginia on a Siemens 3 Tesla MAGNETOM Prisma high-speed imaging device equipped with a 32-channel head-coil. High-resolution T1-weighted anatomical images were first acquired using Siemens’ magnetization-prepared rapid-acquired gradient echo (MPRAGE) pulse sequence with the following specifications: echo time (TE) = 2.98 ms; repetition time (TR) = 2,300 ms; flip angle (FA) = 9°; image matrix = 240 mm × 256 mm; slice thickness = 1 mm; 208 slices. Whole-brain functional images were then acquired using a T2* weighted echo planar imaging (EPI) sequence sensitive to blood oxygenation level dependent (BOLD) contrast with the following specifications: TE = 30 ms; TR = 800 ms; FA = 52°; image matrix = 90 mm x 90 mm; slice thickness = 2.4 mm; slice gap = 2.4 mm; 610 volumes of 60 slices coplanar with the anterior and posterior commissures. Stimuli were presented with the Psychophysics Toolbox (Brainard, 1997) for MATLAB (Mathworks, Natick, MA) using an LCD AVOTEC projector onto a screen located behind the subject’s head and viewed through an integrated head-coil mirror. MR-safe corrective lenses were provided as necessary.
Data preprocessing was carried out using the FMRI Expert Analysis Tool (FEAT) Version 6.00, part of the FMRIB Software Library (FSL) (Smith et al., 2004). The following pre-statistics processing was applied: motion correction using MCFLIRT (Jenkinson et al., 2002); slice timing correction using Fourier-space time-series phase-shifting; non-brain removal using BET (Smith, 2002); spatial smoothing using a Gaussian kernel of 5.0 mm full width at half maximum; grand-mean intensity normalization of the entire 4D dataset by a single multiplicative factor; high-pass temporal filtering (Gaussian-weighted least-squares straight line fitting, with sigma = 50.0 s). Additionally, each functional volume was registered to the participant’s high resolution anatomical image, and then to FSL’s standard Montreal Neurologic Institute (MNI 152, T1 2mm) template brain using FSL’s linear registration tool (FLIRT) (Jenkinson et al., 2002). Registration from high resolution structural to standard space was then further refined using FSL’s nonlinear registration, FNIRT (Andersson et al., 2007a,b).
fMRI analyses
Imaging analysis was conducted using FEAT. At the subject level, time-series statistical analysis was carried out using FSL’s improved linear model (FILM) with local autocorrelation correction (Woolrich et al., 2001). Regressors for each condition (Attend Faces, Attend Houses) were modeled by convolving the time course with a double-gamma hemodynamic response function (HRF), adding a temporal derivative, and applying temporal filtering. An Attend Faces > Attend Houses contrast was computed and the contrast of parameter estimates (COPE) from this analysis for each individual was carried forward to higher-level analysis.
Group-level analyses were conducted at the whole-brain level using FSL’s local analysis of mixed effects (FLAME) stage 1 (Beckmann et al., 2003; Woolrich et al., 2004; Woolrich, 2008) with FSL’s automatic outlier de-weighting algorithm applied, which identifies and de-weights outliers within the fMRI data (Woolrich, 2008). Z (Gaussianised T/F) statistic images were thresholded using clusters determined by Z > 2.3 (p < 0.01) and a corrected cluster significance threshold of p < 0.05 (Worsley, 2001). Clusters that survived correction were registered to subject space and mean Z-statistic values were extracted for each participant from these clusters. We then tested for outliers by ensuring that the absolute value of the median standardized residuals of the model was <3 for each data point. Finally, we tested for data points with undue influence on the model using Cook’s distance (D) > 1 (Cook and Weisberg, 1982) as criteria. The removal of outliers and/or influential points did not appreciably change results. All results are presented with outliers and/or influential points removed.
We first conducted a whole-brain analysis to determine the replicability of the association between OXTR methylation and task-specific Attend Faces > Attend Houses activation (Puglia et al., 2018) in our new sample of young adults (n = 49). Two regressors were included in the model – group mean and mean-centered OXTR methylation – and contrasts testing for positive and negative linear associations between OXTR methylation and Attend Faces > Attend Houses BOLD activation were computed. One outlier was removed from this analysis. All subsequent analyses considered the full young adult sample (n = 103).
We next tested for group-level differences in task-specific Attend Faces > Attend Houses activation using a two-sample unpaired t-test with task accuracy included as a nuisance regressor to account for differences in task performance across age groups. Three outliers (2 younger adults) were removed from this analysis.
We next tested for an interaction between age group and OXTR methylation to determine whether the slope of the linear association between OXTR methylation and Attend Faces > Attend Houses BOLD activation varied as a function of age group. Task accuracy was included as a nuisance regressor in this analysis. Eight outliers (3 younger adults) were removed from this analysis.
Finally, in an exploratory analysis we tested for associations between OXTR methylation, social support, and neural activation during selective social attention in the older adult group. We included the mean-centered measures (OXTR methylation, Social Support score) and the interaction terms (OXTR methylation x Social Support score) as regressors and Task Accuracy as a nuisance regressor in the model, and computed contrasts testing for linear associations between each regressor and Attend Faces > Attend Houses activation. Two outliers were removed from this analysis. Finally, we computed the percent of significant voxels that overlapped between the analysis considering total Support Score and analyses considering each of the individual subscales to explore whether any particular factor of social support most accounted for our results.
Results
OXTR methylation levels do not vary by tissue type or age
We first determined that methylation values do not differ across whole blood and PBMC tissue collection types. In the independent tissue comparison sample, PBMC methylation values averaged 46.72% (SD = 6.33). Whole blood methylation values averaged 46.45% (SD = 6.82). Methylation values were significantly correlated across tissue types (r = 0.95, 95% CI = [0.93, 0.96], p < 0.0001), indicating methylation values in our two imaging samples can be compared.
PBMC methylation for the younger adult sample averaged 47.30% (SD = 6.06). Whole blood methylation for the older adult sample averaged 47.09% (SD = 10.31). Mean methylation values did not significantly differ across age groups [t (146.82) = 0.09, 95% CI = (−2.37, 2.59), p = 0.930], although the variance in methylation values was significantly higher for older adults [F (95,102) = 3.20, p < 0.001].
Younger adults perform better than older adults on the selective social attention task
A logistic regression model predicting proportion correct from age group revealed a significant effect of age group on task performance (B = 0.43, p < 0.0001) such that younger adults performed significantly better on the task (M = 63.03%, SD = 20.72) than older adults (M = 52.63%, SD = 15.52). Performance on the Attend Faces and Attend Houses conditions was significantly correlated for both younger (r = 0.91, p < 0.001) and older (r = 0.85, p < 0.001) adults. Task performance as well as error type by task condition and age group are plotted in Figure 1.
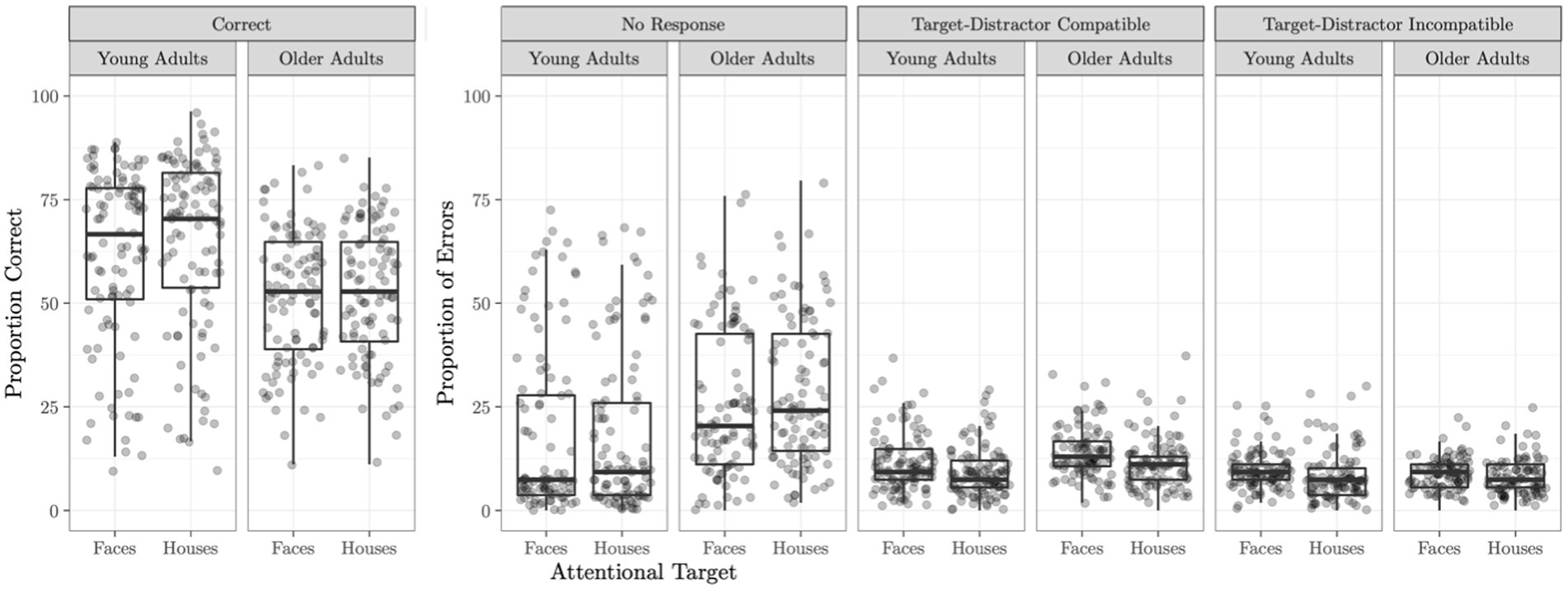
Figure 1. Selective social attention task accuracy. Task accuracy and proportion of errors on the Attend Faces and Attend Houses conditions of the selective social attention task is plotted for the young and older adult samples. Compatible trials are those which the attentional target (e.g., the face in the Attend Faces condition) and the distractor stimulus (e.g., the house in the Attend Faces condition) both warrant the same response (e.g., both the face and the house repeated). Errors on these trials can be considered blatantly wrong. Incompatible trials are those in which the attentional target and the distractor stimulus require a different response (e.g., the attended face was different but the underlying house was the same). Errors on these trials can potentially arise if the participant was distracted by the non-attended target.
The association between OXTR methylation and neural response during selective social attention replicates in a young adult sample
We obtained a novel young adult sample (n = 48) to test for the replicability of the association between OXTR methylation and neural response during selective social attention we previously identified in young adults (Puglia et al., 2018). We found a significant positive association between OXTR methylation and Attend Faces > Attend Houses BOLD response in several regions including left dorsolateral prefrontal cortex and right superior parietal lobule (Figure 2; Table 2), replicating our previous results.
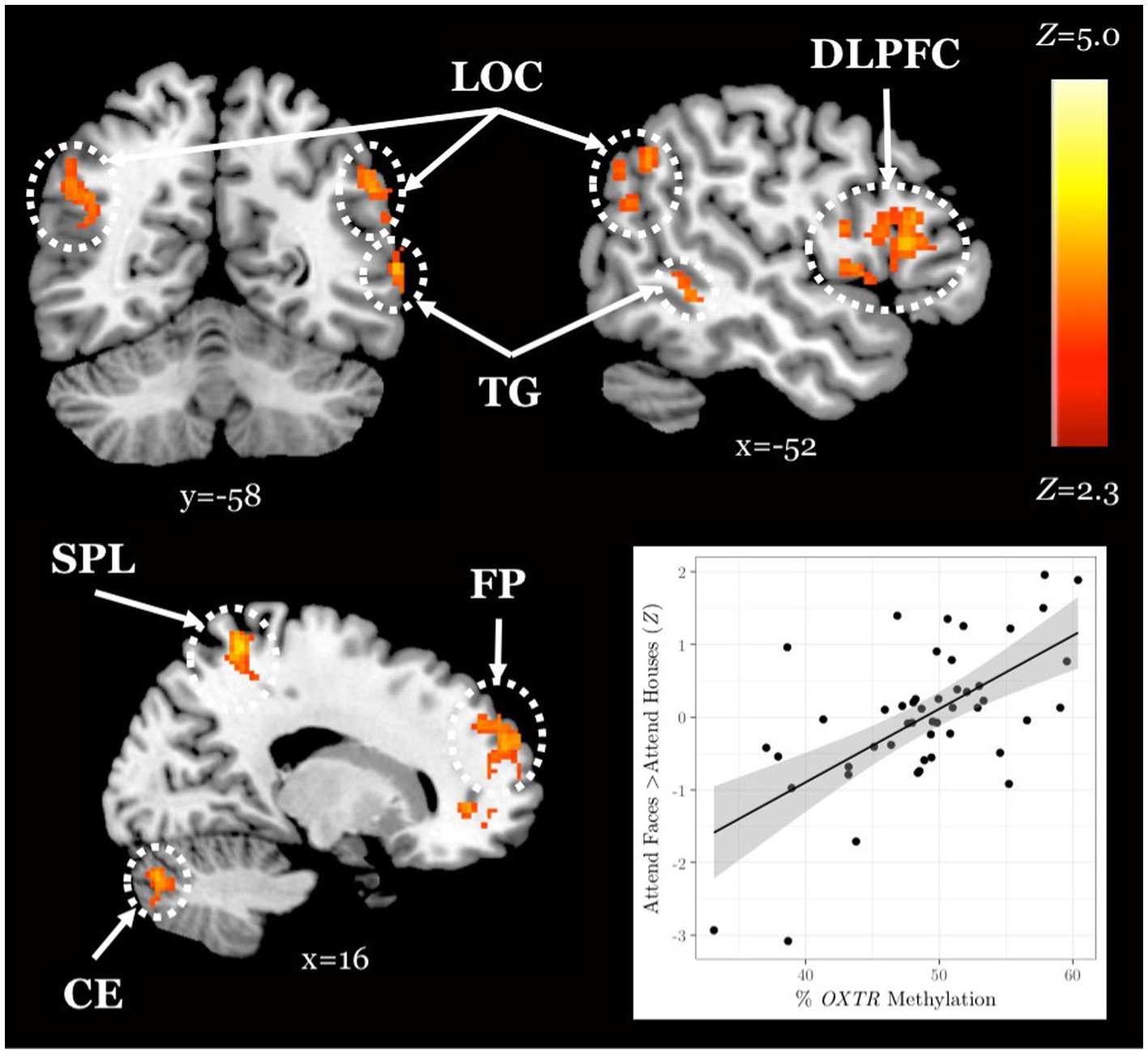
Figure 2. The association between OXTR methylation and neural response during selective social attention replicates in a novel young adult sample. Z statistic map of regions showing a significant positive association between OXTR methylation and Attend Faces > Attend Houses BOLD activity within our young adult replication sample (n = 49). Images are whole-brain FWE cluster-corrected at Z > 2.3 and depicted in MNI space and radiological orientation. (Inset) Mean Z statistic values are plotted against percent OXTR methylation for each participant. Solid line depicts the best-fit line; grey shading depicts 95% confidence interval. LOC, lateral occipital cortex; DLPFC, dorsolateral prefrontal cortex; TG, temporal gyrus; SPL, superior parietal lobule; FP, frontal pole; CE, cerebellum.
Older adults activate a wider area of the brain during selective social attention
When comparing neural response to the selective social perception task across age groups, we found younger adults (n = 101) activated specific regions of the face perception network including left orbitofrontal cortex and posterior cingulate cortex to a greater extent than older adults (n = 87, Figure 3A; Table 3). Conversely, older adults showed greater activation than younger adults within large regions of visual cortex including bilateral fusiform and lateral occipital cortex (Figure 3C; Table 3). These age-sensitive brain regions were binarized and used to extract mean percent signal change from the Attend Faces and the Attend Houses conditions (Figure 3) to visualize activation for each condition and age group. Regardless of age, we found participants that performed better on the selective social attention task showed greater activation within right dorsolateral prefrontal cortex and right superior parietal lobule (Figure 4; Table 3).
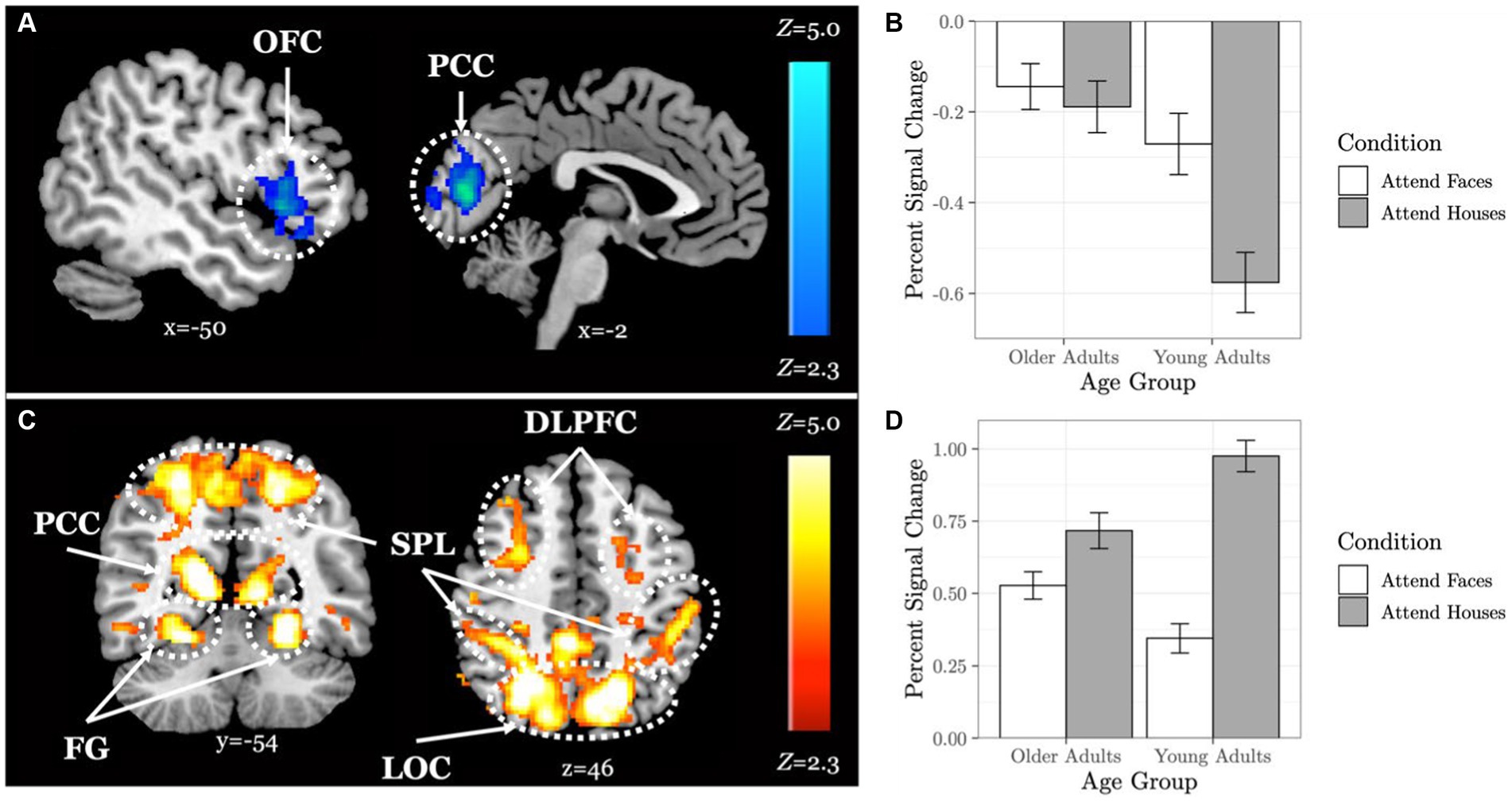
Figure 3. Older adults display neural dedifferentiation during selective social attention. Z statistic maps of regions showing significant differences across age groups on Attend Faces > Attend Houses BOLD activity, controlling for task performance. Images are whole-brain FWE cluster-corrected at Z > 2.3 and depicted in MNI space and radiological orientation. (A) Regions that older adults (n = 101) activate Attend Faces>Attend Houses to a lesser extent than younger adults (n = 87). (B) Mean percent signal change for each condition in regions that older adults activate Attend Faces>Attend Houses to a lesser extent than younger adults. (C) Regions that older adults activate Attend Faces>Attend Houses to a greater extent than younger adults. (D) Mean percent signal change for each condition in regions that older adults activate Attend Faces>Attend Houses to a greater extent than younger adults. OFC, orbitofrontal cortex; PCC, posterior cingulate cortex; DLPFC, dorsolateral prefrontal cortex; SPL, superior parietal lobule; FG, fusiform gyrus; LOC, lateral occipital cortex.
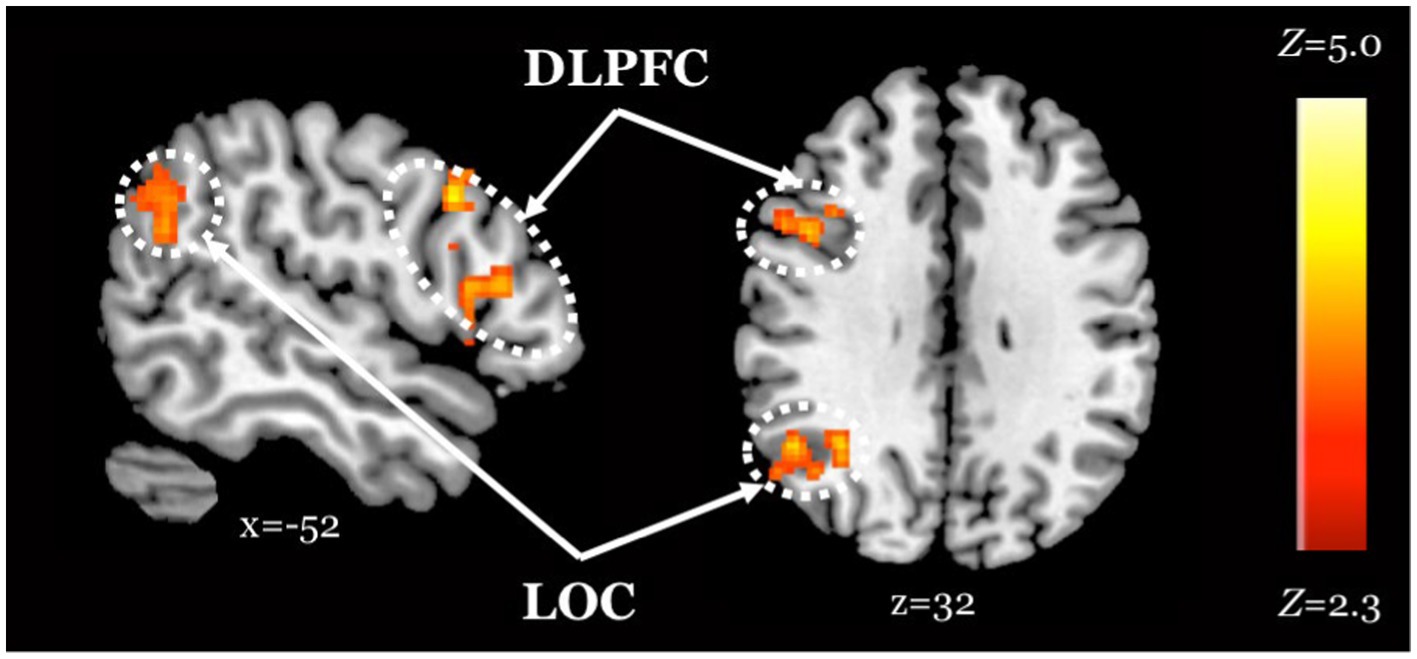
Figure 4. Improved task performance is associated with increased activity within regions of the attentional control network, regardless of age. Z statistic maps of regions showing a significant positive association between task performance and Attend Faces > Attend Houses BOLD activity, controlling for age. Images are whole-brain FWE cluster-corrected at Z > 2.3 and depicted in MNI space and radiological orientation. DLPFC, dorsolateral prefrontal cortex; LOC, lateral occipital cortex.
Younger but not older adults show a positive association between OXTR methylation and neural response during selective social attention
We found a significant interaction between age group and OXTR methylation associated with neural response during selective social attention. The slope of the association between OXTR methylation and neural response during selective social attention was significantly greater for younger (n = 98) than older (n = 85) adults within regions of the attentional control network including anterior cingulate cortex, left dorsolateral prefrontal cortex, and left superior parietal lobule (Figure 5; Table 4). There were no regions in which older adults showed a greater slope in the association between OXTR methylation and neural response during selective social attention than younger adults.
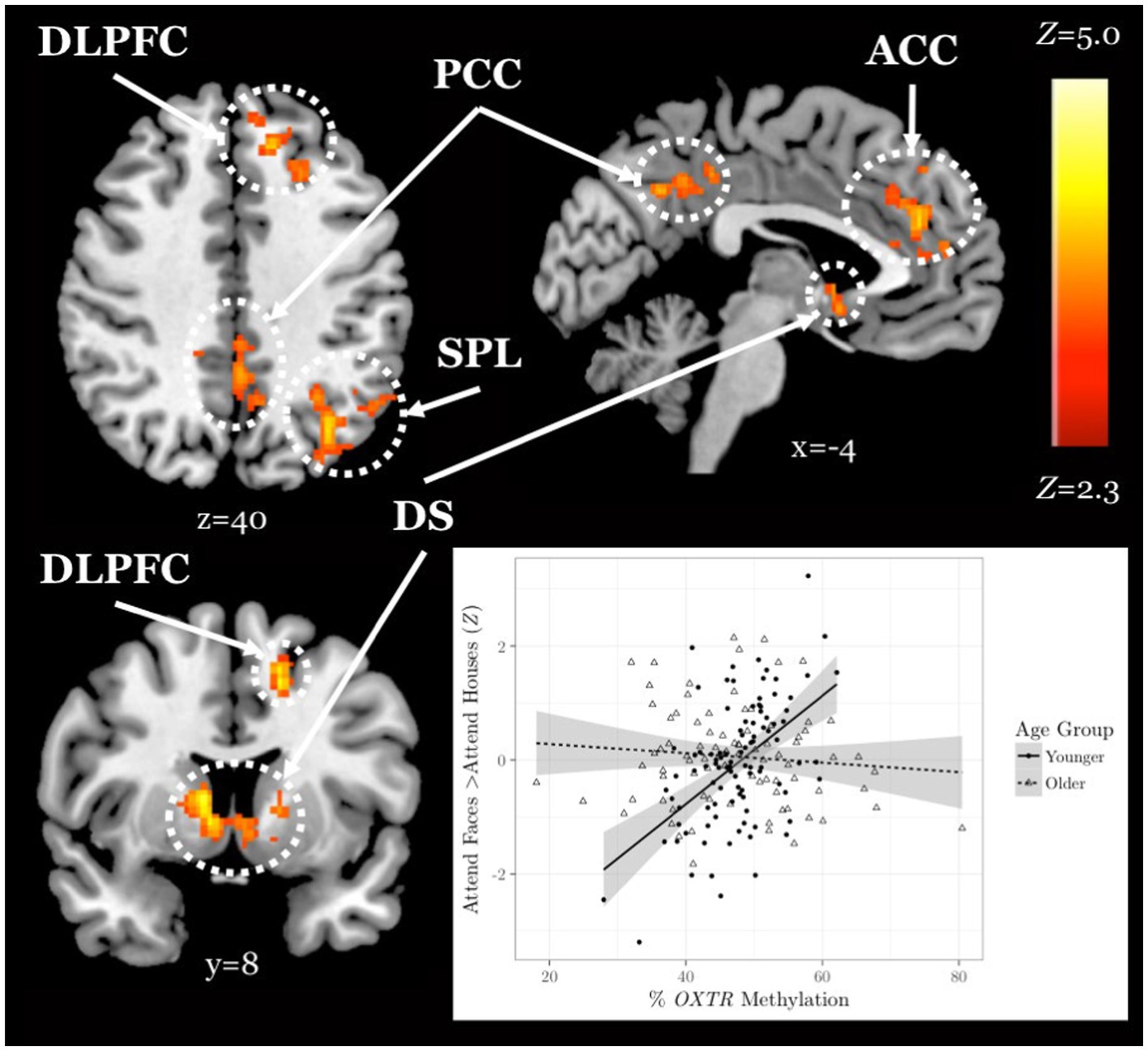
Figure 5. Younger but not older adults show a positive association between OXTR methylation and neural response during selective social attention. Z statistic maps of regions showing a significant interaction between OXTR methylation and age group on Attend Faces > Attend Houses BOLD activity, controlling for task performance. Images are whole-brain FWE cluster-corrected at Z > 2.3 and depicted in MNI space and radiological orientation. (Inset) Mean Z statistic values are plotted against percent OXTR methylation for each participant. Best-fit lines are plotted for younger (solid, n = 98) and older (dashed, n = 85) adults; grey shading depicts 95% confidence intervals. DLPFC, dorsolateral prefrontal cortex; PCC, posterior cingulate cortex; ACC, anterior cingulate cortex; SPL, superior parietal lobule; DS, dorsal striatum.
Social support and OXTR methylation interact to account for differences in neural response during selective social attention among older adults
Finally, in an exploratory analysis, we investigated the influence of OXTR methylation and social support on neural response during selective social attention within our older adult sample (n = 84). We found a significant interaction between OXTR methylation and reported social support on neural response during selective social attention. To illustrate the interaction, we plotted social support by median split and found that older adults who reported lower levels of social support displayed a positive association between OXTR methylation and neural activation within right dorsolateral prefrontal cortex, whereas this association was negative for those that reported higher levels of social support (Figure 6; MNI coordinates: x = 52, y = −18, z = 34; Z = 4.13; cluster extent k = 1,648). These results were primarily driven by the Enacted Support subscale (Table 5).
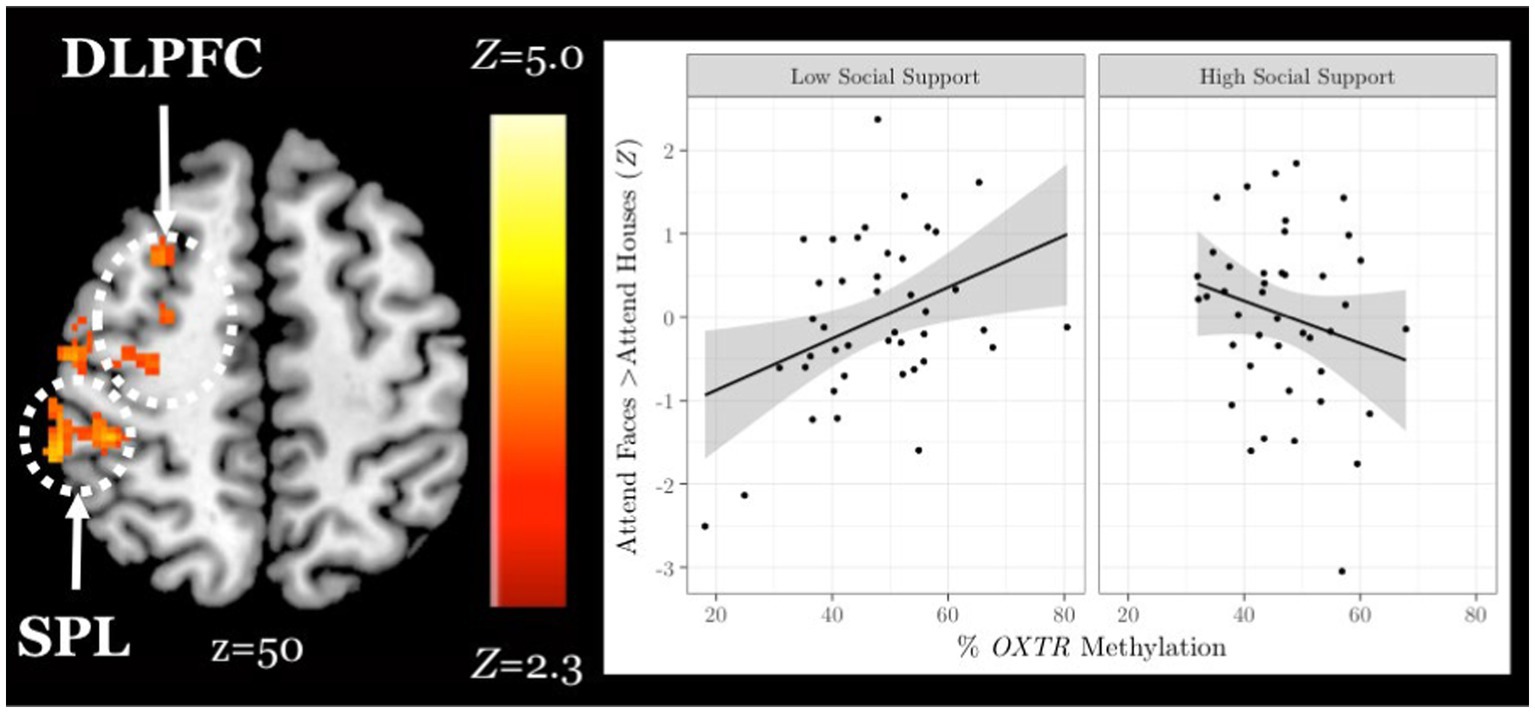
Figure 6. Social support and OXTR methylation interact to account for differences in neural response during selective social attention among older adults. Z statistic map of regions showing a significant interaction between OXTR methylation and self-reported social support on Attend Faces > Attend Houses BOLD activity in older adults (n = 84). Images are whole-brain FWE cluster-corrected at Z > 2.3 and depicted in MNI space and radiological orientation. (Inset) Mean Z statistic values are plotted against percent OXTR methylation for each participant. To illustrate the interaction, social support is plotted by median split. Solid lines depict the best-fit line; grey shading depicts 95% confidence intervals. DLPFC, dorsolateral prefrontal cortex; SPL, superior parietal lobule.
Discussion
In this study, we examined age-related differences in brain response mediated by individual differences in oxytocinergic system function during social attention. After controlling for differences in task performance, we found that older adults activated broad regions of visual and fusiform cortices and recruited dorsolateral prefrontal cortex to a greater extent than younger adults during selective social attention. Older adults also activated orbitofrontal and posterior cingulate cortices, focal regions specialized for social perception (Adolphs, 1999), to a lesser extent than younger adults when attending to faces. These results are consistent with two of the most commonly reported age-related differences in neural activation – the compensatory recruitment of prefrontal regions, and reduced neural specificity, or dedifferentiation, within regions that typically elicit highly specialized responses to specific stimuli in young adults (Park et al., 2004; Reuter-Lorenz and Cappell, 2008; Fabiani, 2012; Sugiura, 2016). Given that aging is associated with cognitive decline, specifically in the social realm (Ruffman et al., 2008; Henry et al., 2013), it is unsurprising that compensatory recruitment of brain regions and more diffuse activation was observed in this study. Dedifferentiation is hypothesized to be caused by brain tissue atrophy (Park et al., 2004), and each of the regions we found implicated in the dedifferentiation of activation have been found to atrophy and change with age (Mann et al., 2011; Evangelista et al., 2021; Shah et al., 2021). It is therefore possible that age-related atrophy of these cortices leads to diffusion of the neural response and the need to recruit prefrontal regions of the brain to a greater extent.
We found that individuals who performed better on the task activated both lateral occipital cortex, a region of the face perception network (Nagy et al., 2012), and dorsolateral prefrontal cortex, a region of the attentional control network (Hopfinger et al., 2000; Seeley et al., 2007), to a greater extent when attending to faces, regardless of age. These results suggest that, across age groups, those who exerted greater attentional “effort” and processed faces to a greater extent performed better on the task.
A whole-brain, age-by-methylation interaction analysis revealed that younger adults with higher levels of OXTR methylation, and therefore presumed decreased sensitivity to endogenous oxytocin, recruited regions of the attentional control network to a greater extent when attending to faces. This positive association between OXTR methylation and activity within the attentional control network during selective social attention in young adults is robust; here, we replicated an effect previously reported in young adults (Puglia et al., 2018) despite changes in scanner and scanning protocol across our original and replication young adult samples. However, we found no association between OXTR methylation and neural response during selective social attention in the older adult sample. This finding is supported by the only other study to date to examine OXTR methylation across adulthood; Ebner and colleagues also found associations between site −934 methylation and social behavior in younger but not older adults (Ebner et al., 2019). Interestingly, while we found no mean differences in total methylation levels across younger and older adults, methylation levels at site −934 were significantly more variable in our older adult sample – a finding also reported by Ebner and colleagues (Ebner et al., 2019). Early life experiences have been shown to impact OXTR methylation at an analogous site in the prairie vole such that pups that received lower levels of parental care within the first few days of life displayed increased levels of OXTR methylation in both brain and blood (Perkeybile et al., 2019). It is therefore possible that methylation levels were more widely distributed in the older adult sample because this sample may have a more heterogeneous developmental history. Alternatively, the aging process itself may result in differences in methylation levels (Richardson, 2003; Koch and Wagner, 2011; Horvath et al., 2012). While we previously found OXTR methylation levels at site −934 are stable in the blood across young adulthood (Connelly and Morris, 2016), interindividual changes have not yet been studied longitudinally into older adulthood. It is important to note that changes in cellular composition associated with aging may confound age-related methylation effects (Jaffe and Irizarry, 2014), although data from our lab suggests that methylation values at site −934 do not vary by cell type (Puglia et al., 2018). Aging is a highly heterogeneous process (Lowsky et al., 2014), and this variability in methylation values in the older adult population could reflect aging differences that are unrelated to chronological age.
We therefore hypothesized that an epigene-by-environment interaction may account for individual differences in neural response during selective social attention in our older adult sample. In an exploratory analysis, we found an interaction between OXTR methylation and social support was significantly associated with neural response within dorsolateral prefrontal cortex and superior parietal lobule in older adults. Older adults who reported lower levels of social support resembled the younger adult population; individuals with lower levels of social support and higher levels of OXTR methylation exerted greater attentional effort to attend to social information. However, as social support levels increased, the slope between OXTR methylation and neural response within attentional control regions decreased. This association provides a potential neurochemical mechanism of behavior in support of the socioemotional selectivity theory. Specifically, older adults tend to be more selective in their relationships, avoiding the formation of new relationships and shedding peripheral social ties to instead invest more in intimate social relationships (Carstensen, 1992; Shaw et al., 2007). Perhaps, older adults who are both highly supported and presumably highly sensitive to endogenous oxytocin (low OXTR methylation) continue to exert effort and recruit regions of the attentional control network because they intrinsically find social information worthy of attention and investment of cognitive resources. Conversely, with increasing methylation (presumed less sensitivity to endogenous oxytocin), those who report a supportive social environment may not feel the need to exert extra effort to attend to the social information in this task.
Limitations and future directions
A limitation to the present study is that we did not administer the Social Support Questionnaire to our younger adult samples, so we are unable to determine whether the association we found in our older adult sample is specific to an aging population. Future research directly probing whether age-related shifts in social motivation or specific dimensions of social support (Barrera, 1986) are associated with oxytocinergic system variability and neural function during social perception is warranted. In addition, a more thorough understanding of the connections between the oxytocinergic system and social attentional networks could inform further research into neurodevelopmental disabilities that impair social functioning such as autism spectrum disorders (ASD). For example, the differences that were observed in aging in this study such as the diffusion of activation during social perception could be used to better understand social attentional differences present in ASD. Furthermore, given associations between OXTR DNA methylation and autism (Puglia et al., 2018; Moerkerke et al., 2021), future research is needed to understand how the aging oxytocinergic system and brain impact autistic traits later in life.
Our sample had fewer older males (n = 28) than older females (n = 60). Therefore, while we could not reliably assess sex differences in the older adult sample, we found a positive association between OXTR methylation and neural response within attentional control regions across sexes in our younger adult sample. The oxytocinergic system, social behavior, and their associated neural systems all show sex differences (Carter et al., 2008); therefore, investigating sex differences in aging will be an important avenue for future research, and should include additional sex-related factors such as reproductive history.
Although we controlled for task performance by including this variable as a nuisance regressor in all analyses, future research should set individual threshold levels for task performance so that the neural and epigenetic mechanisms supporting task performance are matched across cognitive demand. However, that task performance varied greatly within both age groups reflects an advantage for our study design as young adults typically score at ceiling on many social cognitive tasks. Additionally, to further probe the hypothesized mechanism of OXTR methylation and neural response during selective social attention for the theory of socioemotional selectivity, future research should present both familiar and unfamiliar stimuli to understand how age and methylation impact differential activation of attentional control regions during the perception of familiar vs. unfamiliar faces. Finally, age-related changes in functional connectivity within attention and salience networks should be explored in future work, particularly given that DLPFC activation and functional connectivity during selective social attention interacted to impact task performance in our prior young adult work (Puglia et al., 2018).
Conclusion
Our results highlight the importance of adopting a lifespan perspective for understanding associations between individual differences in oxytocinergic system function, neural systems supporting social cognition, and social behavior. Maintaining social relationships is critical for longevity, happiness (Holt-Lunstad et al., 2010), and reduced likelihood of developing late-life dementia (Wilson et al., 2007). Particularly as the world’s population continues to age, understanding the neurobiological factors that contribute to healthy social functioning into older adulthood will have critical implications from both a humanitarian and economic standpoint.
Data availability statement
The raw data supporting the conclusions of this article will be made available by the authors, without undue reservation.
Ethics statement
The studies involving humans were approved by the University of Virginia Institutional Review Board for Health Sciences Research. The studies were conducted in accordance with the local legislation and institutional requirements. The participants provided their written informed consent to participate in this study.
Author contributions
MP, JC, and JM designed and conceptualized the study. MP, ML, and MN wrote the paper. MP collected the younger adult data and performed the fMRI and epigenetic analyses. ML collected the older adult data and isolated whole blood DNA for the older adult samples. JC and JM provided edits and comments to the manuscript and funding for the work. All authors contributed to the article and approved the submitted version.
Funding
This research was supported by the National Science Foundation Grants 1228522 and 1657726 to JC and JM and the National Institute on Aging grant R01AG024270 to Timothy A. Salthouse.
Acknowledgments
We thank Timothy A. Salthouse for providing access to the Virginia Cognitive Aging Project and for his helpful edits and comments on the manuscript. We also thank Andrew Graves, Hazel Lindal, Amalia McDonald, Jessica Pham, Tyler Santander, and Brenda Straka for assistance with data collection.
Conflict of interest
The authors declare that the research was conducted in the absence of any commercial or financial relationships that could be construed as a potential conflict of interest.
Publisher’s note
All claims expressed in this article are solely those of the authors and do not necessarily represent those of their affiliated organizations, or those of the publisher, the editors and the reviewers. Any product that may be evaluated in this article, or claim that may be made by its manufacturer, is not guaranteed or endorsed by the publisher.
References
Adolphs, R. (1999). Social cognition and the human brain. Trends Cogn. Sci. 3, 469–479. doi: 10.1016/S1364-6613(99)01399-6
Andersson, J. L. R., Jenkinson, M., and Smith, S. (2007a). Non-linear optimisation FMRIB Technial report TR07JA1. Oxford, UK: FMRIB Centre.
Andersson, J. L. R., Jenkinson, M., and Smith, S. (2007b). Non-linear registration aka spatial normalisation FMRIB Technial report TR07JA2. Oxford, UK: FMRIB Centre.
Barrera, M. (1986). Distinctions between social support concepts, measures, and models. Am. J. Community Psychol. 14, 413–445. doi: 10.1007/BF00922627
Beckmann, C. F., Jenkinson, M., and Smith, S. M. (2003). General multilevel linear modeling for group analysis in FMRI. NeuroImage 20, 1052–1063. doi: 10.1016/S1053-8119(03)00435-X
Bindemann, M., Burton, A. M., Langton, S. R. H., Schweinberger, S. R., and Doherty, M. J. (2007). The control of attention to faces. J. Vis. 7:15. doi: 10.1167/7.10.15
Boutet, I., Taler, V., and Collin, C. A. (2015). On the particular vulnerability of face recognition to aging: a review of three hypotheses. Front. Psychol. 6:1139. doi: 10.3389/fpsyg.2015.01139
Brainard, D. H. (1997). The psychophysics toolbox. Spat. Vis. 10, 433–436. doi: 10.1163/156856897X00357
Calati, R., Ferrari, C., Brittner, M., Oasi, O., Olié, E., Carvalho, A. F., et al. (2019). Suicidal thoughts and behaviors and social isolation: A narrative review of the literature. J. Affect. Disord. 245, 653–667. doi: 10.1016/J.JAD.2018.11.022
Carstensen, L. L. (1992). Social and emotional patterns in adulthood: support for socioemotional selectivity theory. Psychol. Aging 7, 331–338. doi: 10.1037/0882-7974.7.3.331
Carter, C. S. (2014). Oxytocin pathways and the evolution of human behavior. Annu. Rev. Psychol. 65, 17–39. doi: 10.1146/annurev-psych-010213-115110
Carter, C. S., Grippo, A. J., Pournajafi-Nazarloo, H., Ruscio, M. G., and Porges, S. W. (2008). Oxytocin, vasopressin and sociality. Prog. Brain Res. 170, 331–336. doi: 10.1016/S0079-6123(08)00427-5
Connelly, J. J., and Morris, J. P. (2016). “Epigenetics and social behavior” in The Oxford handbook of cultural neuroscience. eds. J. Y. Chiao, S.-C. Li, R. Seligman, and R. Turner (New York, NY: Oxford University Press), 299–313.
Cook, R. D., and Weisberg, S. (1982). Residuals and influence in regression. New York: Chapman and Hall.
Crawley, M. J. (2007). “Proportion data” in The R book (Chichester, UK: John Wiley & Sons, Ltd.), 569–591.
De Lillo, M., Foley, R., Fysh, M. C., Stimson, A., Bradford, E. E. F., Woodrow-Hill, C., et al. (2021). Tracking developmental differences in real-world social attention across adolescence, young adulthood and older adulthood. Nat. Hum. Behav. 5, 1381–1390. doi: 10.1038/S41562-021-01113-9
Desimone, R., and Duncan, J. (1995). Neural mechanisms of selective visual attention. Annu. Rev. Neurosci. 18, 193–222. doi: 10.1146/annurev.ne.18.030195.001205
Ebner, N. C., Kamin, H., Diaz, V., Cohen, R. A., and MacDonald, K. (2014). Hormones as “difference makers” in cognitive and socioemotional aging processes. Front. Psychol. 5:1595. doi: 10.3389/fpsyg.2014.01595
Ebner, N. C., Lin, T., Muradoglu, M., Weir, D. H., Plasencia, G. M., Lillard, T. S., et al. (2019). Associations between oxytocin receptor gene (OXTR) methylation, plasma oxytocin, and attachment across adulthood. Int. J. Psychophysiol. 136, 22–32. doi: 10.1016/J.IJPSYCHO.2018.01.008
Ebner, N. C., Maura, G. M., MacDonald, K., Westberg, L., and Fischer, H. (2013). Oxytocin and socioemotional aging: current knowledge and future trends. Front. Hum. Neurosci. 7:487. doi: 10.3389/fnhum.2013.00487
Evangelista, N. D., O’Shea, A., Kraft, J. N., Hausman, H. K., Boutzoukas, E. M., Nissim, N. R., et al. (2021). Independent Contributions of Dorsolateral Prefrontal Structure and Function to Working Memory in Healthy Older Adults. Cereb. Cortex. 31:1732. doi: 10.1093/CERCOR/BHAA322
Fabiani, M. (2012). It was the best of times, it was the worst of times: a psychophysiologist’s view of cognitive aging. Psychophysiology 49, 283–304. doi: 10.1111/j.1469-8986.2011.01331.x
Folstein, M. F., Folstein, S. E., and McHugh, P. R. (1975). “Mini-mental state”. A practical method for grading the cognitive state of patients for the clinician. J. Psychiatr. Res. 12, 189–198. doi: 10.1016/0022-3956(75)90026-6
Furey, M. L., Tanskanen, T., Beauchamp, M. S., Avikainen, S., Uutela, K., Hari, R., et al. (2006). Dissociation of face-selective cortical responses by attention. Proc. Natl. Acad. Sci. U. S. A. 103, 1065–1070. doi: 10.1073/pnas.0510124103
Grainger, S. A., Henry, J. D., Steinvik, H. R., Vanman, E. J., Rendell, P. G., and Labuschagne, I. (2018). Intranasal oxytocin does not reduce age-related difficulties in social cognition. Horm. Behav. 99, 25–34. doi: 10.1016/J.YHBEH.2018.01.009
Gregory, S. G., Connelly, J. J., Towers, A. J., Johnson, J., Biscocho, D., Markunas, C., et al. (2009). Genomic and epigenetic evidence for oxytocin receptor deficiency in autism. BMC Med. 7:62. doi: 10.1186/1741-7015-7-62
Henry, J. D., Phillips, L. H., Ruffman, T., and Bailey, P. E. (2013). A meta-analytic review of age differences in theory of mind. Psychol. Aging 28, 826–839. doi: 10.1037/a0030677
Herrington, J. D., Riley, M. E., Grupe, D. W., and Schultz, R. T. (2015). Successful face recognition is associated with increased prefrontal cortex activation in autism Spectrum disorder. J. Autism Dev. Disord. 45, 902–910. doi: 10.1007/s10803-014-2233-4
Holt-Lunstad, J., Smith, T. B., and Layton, J. B. (2010). Social relationships and mortality risk: a meta-analytic review. PLoS Med. 7:e1000316. doi: 10.1371/journal.pmed.1000316
Hopfinger, J. B., Buonocore, M. H., and Mangun, G. R. (2000). The neural mechanisms of top-down attentional control. Nat. Neurosci. 3, 284–291. doi: 10.1038/72999
Horvath, S., Zhang, Y., Langfelder, P., Kahn, R. S., Boks, M. P. M., van Eijk, K., et al. (2012). Aging effects on DNA methylation modules in human brain and blood tissue. Genome Biol. 13:R97. doi: 10.1186/gb-2012-13-10-r97
Huffmeijer, R., van IJzendoorn, M. H., and Bakermans-Kranenburg, M. J. (2013). Ageing and oxytocin: a call for extending human oxytocin research to ageing populations – a mini-review. Gerontology 59, 32–39. doi: 10.1159/000341333
Jaffe, A. E., and Irizarry, R. A. (2014). Accounting for cellular heterogeneity is critical in epigenome-wide association studies. Genome Biol. 15:R31. doi: 10.1186/gb-2014-15-2-r31
Jenkinson, M., Bannister, P., Brady, M., and Smith, S. (2002). Improved optimization for the robust and accurate linear registration and motion correction of brain images. NeuroImage 17, 825–841. doi: 10.1016/S1053-8119(02)91132-8
Kéri, S., and Benedek, G. (2009). Oxytocin enhances the perception of biological motion in humans. Cogn. Affect. Behav. Neurosci. 9, 237–241. doi: 10.3758/CABN.9.3.237
Klin, A., Jones, W., Schultz, R., Volkmar, F., and Cohen, D., (2002). Visual Fixation Patterns During Viewing of Naturalistic Social Situations as Predictors of Social Competence in Individuals With Autism. Arch. Gen. Psychiatry. 59:809. doi: 10.1001/archpsyc.59.9.809
Koch, C. M., and Wagner, W. (2011). Epigenetic-aging-signature to determine age in different tissues. Aging 3, 1018–1027. doi: 10.18632/aging.100395
Kuiper, J. S., Zuidersma, M., Oude Voshaar, R. C., Zuidema, S. U., van den Heuvel, E. R., Stolk, R. P., et al. (2015). Social relationships and risk of dementia: A systematic review and meta-analysis of longitudinal cohort studies. Ageing Res. Rev. 22, 39–57. doi: 10.1016/J.ARR.2015.04.006
Langton, S. R. H., Law, A. S., Burton, A. M., and Schweinberger, S. R. (2008). Attention capture by faces. Cognition 107, 330–342. doi: 10.1016/J.COGNITION.2007.07.012
Lowsky, D. J., Olshansky, S. J., Bhattacharya, J., and Goldman, D. P. (2014). Heterogeneity in healthy aging. J. Gerontol. A Biol. Sci. Med. Sci. 69, 640–649. doi: 10.1093/gerona/glt162
Madden, D. J., Wythe, L. W., and Huettel, S. A. (2005). “Age-related changes in neural activity during visual perception and attention” in Cognitive neuroscience of aging: linking cognitive and cerebral aging. eds. R. Cabeza, L. Nyberg, and D. Park (New York, NY: Oxford University Press), 156–185.
Mann, S. L., Hazlett, E. A., Byne, W., Hof, P. R., Buchsbaum, M. S., Cohen, B. H., et al. (2011). Anterior and Posterior Cingulate Cortex Volume in Healthy Adults: Effects of Aging and Gender Differences. Brain Res. 1401, 18–29. doi: 10.1016/J.BRAINRES.2011.05.050
Marlin, B. J., Mitre, M., D’amour, J. A., Chao, M. V., and Froemke, R. C. (2015). Oxytocin enables maternal behaviour by balancing cortical inhibition. Nature 520, 499–504. doi: 10.1038/nature14402
Matt, G. E., and Dean, A. (1993). Social support from friends and psychological distress among elderly persons: moderator effects of age. J. Health Soc. Behav. 34, 187–200. doi: 10.2307/2137201
Meinhardt-Injac, B., Boutet, I., Persike, M., Meinhardt, G., and Imhof, M. (2017). From development to aging: Holistic face perception in children, younger and older adults. Cognition, 158, 134–146. doi: 10.1016/J.COGNITION.2016.10.020
Moerkerke, M., Bonte, M. L., Daniels, N., Chubar, V., Alaerts, K., Steyaert, J., et al. (2021). Oxytocin receptor gene (OXTR) DNA methylation is associated with autism and related social traits – A systematic review. Res. Autism Spectr. Disord. 85:101785. doi: 10.1016/J.RASD.2021.101785
Nagy, K., Greenlee, M. W., and Kovács, G. (2012). The lateral occipital cortex in the face perception network: an effective connectivity study. Front. Psychol. 3:141. doi: 10.3389/fpsyg.2012.00141
National Academies and of Sciences, Engineering, and Medicine. (2020). Social isolation and loneliness in older adults: opportunities for the health care system. Washington, DC: The National Academies Press.
O’Craven, K. M., Downing, P. E., and Kanwisher, N. (1999). fMRI evidence for objects as the units of attentional selection. Nature 401, 584–587. doi: 10.1038/44134
Park, D. C., Polk, T. A., Park, R., Minear, M., Savage, A., and Smith, M. R. (2004). Aging reduces neural specialization in ventral visual cortex. Proc. Natl. Acad. Sci. U. S. A. 101, 13091–13095. doi: 10.1073/pnas.0405148101
Perkeybile, A. M., Carter, C. S., Wroblewski, K. L., Puglia, M. H., Kenkel, W. M., Lillard, T. S., et al. (2019). Early nurture epigenetically tunes the oxytocin receptor. Psychoneuroendocrinology 99, 128–136. doi: 10.1016/j.psyneuen.2018.08.037
Puglia, M. H., Connelly, J. J., and Morris, J. P. (2018). Epigenetic regulation of the oxytocin receptor is associated with neural response during selective social attention. Transl. Psychiatry 8:116. doi: 10.1038/s41398-018-0159-x
Puglia, M. H., Lillard, T. S., Morris, J. P., and Connelly, J. J. (2015). Epigenetic modification of the oxytocin receptor gene influences the perception of anger and fear in the human brain. Proc. Natl. Acad. Sci. 112, 3308–3313. doi: 10.1073/pnas.1422096112
R Core Team. (2020). R: A language and environment for statistical computing. Vienna, Austria: R Foundation for Statistical Computing.
Reuter-Lorenz, P. A., and Cappell, K. A. (2008). Neurocognitive aging and the compensation hypothesis. Curr. Dir. Psychol. Sci. 17, 177–182. doi: 10.1111/j.1467-8721.2008.00570.x
Richardson, B. (2003). Impact of aging on DNA methylation. Ageing Res. Rev. 2, 245–261. doi: 10.1016/S1568-1637(03)00010-2
Ross, H. E., and Young, L. J. (2009). Oxytocin and the neural mechanisms regulating social cognition and affiliative behavior. Front. Neuroendocrinol. 30, 534–547. doi: 10.1016/j.yfrne.2009.05.004
Ruffman, T., Henry, J. D., Livingstone, V., and Phillips, L. H. (2008). A meta-analytic review of emotion recognition and aging: implications for neuropsychological models of aging. Neurosci. Biobehav. Rev. 32, 863–881. doi: 10.1016/J.NEUBIOREV.2008.01.001
Salthouse, T. A. (2010). Selective review of cognitive aging. J. Int. Neuropsychol. Soc. 16, 754–760. doi: 10.1017/S1355617710000706
Salthouse, T. (2012). What cognitive abilities are involved in trail-making performance? Intell. 39, 222–232.
Salthouse, T. A. (2014). Frequent Assessments May Obscure Cognitive Decline. Psychol. Assess. 26, 1063–1069.
Salthouse, T., Toth, J., Daniels, K., Parks, C., Pak, R., Wolbrette, M., et al. (2000). Effects of aging on the efficiency of task switching in a variant of the Trail Making Test. Neuropsychol. 14, 102–111.
Sannino, S., Chini, B., and Grinevich, V. (2017). Lifespan oxytocin signaling: maturation, flexibility, and stability in newborn, adolescent, and aged brain. Dev. Neurobiol. 77, 158–168. doi: 10.1002/dneu.22450
Schulze, L., Lischke, A., Greif, J., Herpertz, S. C., Heinrichs, M., and Domes, G. (2011). Oxytocin increases recognition of masked emotional faces. Psychoneuroendocrinology 36, 1378–1382. doi: 10.1016/J.PSYNEUEN.2011.03.011
Seeley, W. W., Menon, V., Schatzberg, A. F., Keller, J., Glover, G. H., Kenna, H., et al. (2007). Dissociable intrinsic connectivity networks for salience processing and executive control. J. Neurosci. 27, –2356. doi: 10.1523/JNEUROSCI.5587-06.2007
Seeman, T. E. (2000). Health promoting effects of friends and family on health outcomes in older adults. Am. J. Health Promot. 14, 362–370. doi: 10.4278/0890-1171-14.6.362
Shah, M., Kurth, F., and Luders, E. (2021). The Impact of Aging on the Subregions of the Fusiform Gyrus in Healthy Older Adults. J. Neurosci. Res. 99:263. doi: 10.1002/JNR.24586
Shamay-Tsoory, S. G., and Abu-Akel, A. (2016). The social salience hypothesis of oxytocin. Biol. Psychiatry 79, 194–202. doi: 10.1016/j.biopsych.2015.07.020
Shaw, B. A., Krause, N., Liang, J., and Bennett, J. (2007). Tracking changes in social relations throughout late life. J. Gerontol. Ser. B Psychol. Sci. Soc. Sci. 62, S90–S99. doi: 10.1093/geronb/62.2.S90
Siedlecki, K. L., Tucker-Drob, E. M., Oishi, S., and Salthouse, T. A. (2008). Life satisfaction across adulthood: different determinants at different ages? J. Posit. Psychol. 3, 153–164. doi: 10.1080/17439760701834602
Smith, S. M. (2002). Fast robust automated brain extraction. Hum. Brain Mapp. 17, 143–155. doi: 10.1002/hbm.10062
Smith, S. M., Jenkinson, M., Woolrich, M. W., Beckmann, C. F., Behrens, T. E. J., Johansen-Berg, H., et al. (2004). Advances in functional and structural MR image analysis and implementation as FSL. NeuroImage 23, S208–S219. doi: 10.1016/j.neuroimage.2004.07.051
Sugiura, M. (2016). Functional neuroimaging of normal aging: declining brain, adapting brain. Ageing Res. Rev. 30, 61–72. doi: 10.1016/J.ARR.2016.02.006
Theeuwes, J., and Van der Stigchel, S. (2006). Faces capture attention: evidence from inhibition of return. Vis. Cogn. 13, 657–665. doi: 10.1080/13506280500410949
Valtorta, N. K., Kanaan, M., Gilbody, S., Ronzi, S., and Hanratty, B. (2016). Loneliness and social isolation as risk factors for coronary heart disease and stroke: Systematic review and meta-analysis of longitudinal observational studies. Heart, 102, 1009–1016. doi: 10.1136/HEARTJNL-2015-308790
Wang, S., and Adolphs, R. (2017). “Social Saliency” in Computational and cognitive neuroscience of vision. ed. Q. Zhao (Singapore: Springer), 171–193.
Wilson, R. S., Krueger, K. R., Arnold, S. E., Schneider, J. A., Kelly, J. F., Barnes, L. L., et al. (2007). Loneliness and risk of Alzheimer disease. Arch. Gen. Psychiatry 64:234. doi: 10.1001/archpsyc.64.2.234
Woolrich, M. W. (2008). Robust group analysis using outlier inference. NeuroImage 41, 286–301. doi: 10.1016/j.neuroimage.2008.02.042
Woolrich, M. W., Behrens, T. E. J., Beckmann, C. F., Jenkinson, M., and Smith, S. M. (2004). Multilevel linear modelling for FMRI group analysis using Bayesian inference. NeuroImage 21, 1732–1747. doi: 10.1016/j.neuroimage.2003.12.023
Woolrich, M. W., Ripley, B. D., Brady, M., and Smith, S. M. (2001). Temporal autocorrelation in univariate linear modeling of FMRI data. NeuroImage 14, 1370–1386. doi: 10.1006/nimg.2001.0931
Worsley, K. J. (2001). “Statistical analysis of activation images” in Functional MRI: an introduction to methods. eds. P. J. Ezzard, P. M. Matthews, and S. M. Smith (New York, NY: Oxford University Press), 251–270.
Keywords: aging, social attention, OXTR methylation, fMRI, social cognition
Citation: Puglia MH, Lynch ME, Nance MG, Connelly JJ and Morris JP (2023) DNA methylation of the oxytocin receptor interacts with age to impact neural response to social stimuli. Front. Aging Neurosci. 15:1252478. doi: 10.3389/fnagi.2023.1252478
Edited by:
Cosimo Urgesi, University of Udine, ItalyReviewed by:
Anna MacKay-Brandt, Nathan Kline Institute for Psychiatric Research, United StatesRiccardo Manca, Brunel University London, United Kingdom
Michael Ntim, Kwame Nkrumah University of Science and Technology, Ghana
Bin Wang, Dalian Medical University, China
Copyright © 2023 Puglia, Lynch, Nance, Connelly and Morris. This is an open-access article distributed under the terms of the Creative Commons Attribution License (CC BY). The use, distribution or reproduction in other forums is permitted, provided the original author(s) and the copyright owner(s) are credited and that the original publication in this journal is cited, in accordance with accepted academic practice. No use, distribution or reproduction is permitted which does not comply with these terms.
*Correspondence: Meghan H. Puglia, meghan.puglia@virginia.edu