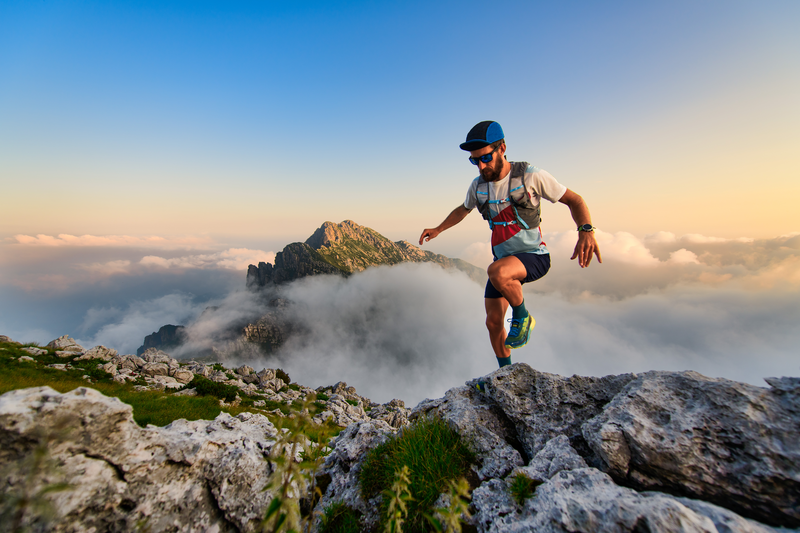
94% of researchers rate our articles as excellent or good
Learn more about the work of our research integrity team to safeguard the quality of each article we publish.
Find out more
REVIEW article
Front. Aging Neurosci. , 27 July 2023
Sec. Alzheimer's Disease and Related Dementias
Volume 15 - 2023 | https://doi.org/10.3389/fnagi.2023.1221653
This article is part of the Research Topic Reviews In Alzheimer's Disease and Related Dementias View all 12 articles
Sarcopenia is an age-related, involuntary loss of skeletal muscle mass and strength. Alzheimer's disease (AD) is the most common cause of dementia in elderly adults. To date, no effective cures for sarcopenia and AD are available. Physical and cognitive impairments are two major causes of disability in the elderly population, which severely decrease their quality of life and increase their economic burden. Clinically, sarcopenia is strongly associated with AD. However, the underlying factors for this association remain unknown. Mechanistic studies on muscle–brain crosstalk during cognitive impairment might shed light on new insights and novel therapeutic approaches for combating cognitive decline and AD. In this review, we summarize the latest studies emphasizing the association between sarcopenia and cognitive impairment. The underlying mechanisms involved in muscle–brain crosstalk and the potential implications of such crosstalk are discussed. Finally, future directions for drug development to improve age-related cognitive impairment and AD-related cognitive dysfunction are also explored.
Aging is often considered an inevitable progressive process along with deteriorating functional decline. With increased longevity and decreased mortality, population aging has been sweeping the world rapidly in recent years (Beard et al., 2016). In the United States, there were more than 56 million individuals aged over 65 years in 2020, which accounts for 16.9% of the national population, and is estimated to be roughly 22% by 2050.1
Aging is often associated with decreased function in multiple key organs, including the brain, skeletal muscle, and the heart (North and Sinclair, 2012; Hambright et al., 2019; Hou et al., 2019). The strategy connecting this triple functional system could be a key to Alzheimer's disease (AD) prevention and rehabilitation. Physical and cognitive impairments are two major causes of disability in the elderly. Sarcopenia, an age-related involuntary loss of skeletal muscle mass and strength, is strongly associated with AD, a neurodegenerative disease with a prevalence of over 47 million globally (DeTure and Dickson, 2019; Beeri et al., 2021). Evidence from both clinical (Salinas-Rodriguez et al., 2021; Hu et al., 2022; Ramoo et al., 2022) and animal studies (Nagase and Tohda, 2021; Lee and Lim, 2022) demonstrates that skeletal muscle dysfunction may be a key factor that can contribute to cognitive impairment. So far, there is no effective cure for sarcopenia or AD. Drugs approved for Alzheimer's disease are classified into acetylcholinesterase inhibitors and N-methyl-D-aspartate receptor antagonists (Lin et al., 2021). However, AD patients cannot benefit much from these treatments due to the biological restriction of the blood-brain barrier (BBB), the low bioavailability, poor pharmacokinetics and pharmacodynamics of these drugs (Nunes et al., 2022). Current therapies or drugs available for AD focus on managing symptoms and mostly target the acetylcholinesterase system, which so far has turned out to generate mild effects with low clinical outcomes (Marucci et al., 2021). In addition, currently, there are no drugs approved by the Food and Drug Administration for the treatment of sarcopenia (Cho et al., 2022).
Drug treatments provided for both Alzheimer's Disease and sarcopenia remain ineffective; however, exercise has been proven to be highly effective in maintaining muscle mass and cognitive health (Beckwee et al., 2019; Huang et al., 2022). A recent study showed that exercise enhanced muscle-derived circulating factors release, which increased synaptic plasticity and hippocampal neurogenesis and thus improved cognitive function via muscle–brain crosstalk (Rendeiro and Rhodes, 2018). Therefore, early diagnosis and intervention for sarcopenia may benefit AD.
Based on earlier studies, in the present review, we summarize the latest findings on the association between sarcopenia and cognitive impairment. We also discuss the underlying mechanisms responsible for muscle–brain crosstalk. Finally, we will explore the potential strategies for targeting such crosstalk and future directions for drug development to improve cognitive function during aging and AD.
Skeletal muscle is the largest organ in the human body by weight and is responsible for maintaining body posture and performing voluntary movements (Tieland et al., 2018). Normal physiological functioning of skeletal muscle allows both physical activity and metabolic regulation. Thus, changes in skeletal muscle function and mass may significantly affect metabolism due to its sensitivity to insulin (Stump et al., 2006). Interestingly, the brain is also an insulin-sensitive metabolic organ that consumes 25% of the glucose in the body (Rossi et al., 2001). Therefore, there is potential endocrine crosstalk between skeletal muscle and the brain. Both animal studies and clinical trials have shown a strong association between sarcopenia and cognitive impairment. It has been demonstrated that skeletal muscle atrophy may have detrimental effects on cognitive function in multiple animal models. For instance, in 5XFAD transgenic mice (an Alzheimer's disease mouse model), muscle atrophy accelerated the onset of cognitive impairment, and the underlying mechanism may be mediated by hemopexin secreted from the atrophy muscle (Nagase and Tohda, 2021). Myokines released by atrophying muscles caused aberrant energy metabolism and thus impaired cognition in a type 2 diabetes mellitus mouse model (Lee and Lim, 2022). Consistent with these animal studies, clinical studies and meta-analyses revealed that sarcopenia is implicated in increased risk for cognitive impairment (Wu et al., 2021; Hu et al., 2022; Li et al., 2022). A meta-analysis including 18,788 participants based on 26 cohort, cross-sectional, and case-control studies found that participants with sarcopenia showed a higher risk of developing cognitive impairment [OR = 1.75; 95% CI = 1.57, 1.95; P < 0.00001]. Additionally, the MMSE score was lower in the sarcopenia group than that in the non-sarcopenia group [OR = −2.23; 95% CI = −2.48, −1.99; P < 0.00001] (Chen et al., 2022). Similarly, other meta-analyses demonstrated that sarcopenia is an independent risk factor for cognitive impairment (Chang et al., 2016; Cipolli et al., 2019; Peng et al., 2020). Two recent clinical studies further demonstrated the higher prevalence of cognitive impairment in older adults with sarcopenia. Longitudinal associations between sarcopenia and mild cognitive impairment (OR = 1.74; 95% CI 1.02, 2.96; P = 0.04), decreased cognitive function (β = −0.57; 95% CI−0.93, −0.21; P < 0.01), immediate verbal recall (β = −0.14; 95% CI−0.28, −0.01; P = 0.04), delayed verbal recall (β = −0.12; 95% CI−0.23, −0.01; P = 0.03), and semantic verbal fluency (β = −0.17; 95% CI−0.28, −0.05; P = 0.01) have been found in a study including 496 older Mexican adults. Sarcopenic elderly adults showed a 0.7% higher annual rate of mild cognitive impairment (Salinas-Rodriguez et al., 2021). Another cohort study including 1,946 respondents in rural Malaysia showed similar results. Sarcopenic elderly adults have an 80% higher risk of cognitive impairment compared with those without sarcopenia (RR 1.80; 95% CI 1.18–2.75) (Ramoo et al., 2022). Taken together, these studies support a strong link between muscle atrophy and cognitive impairment. Early diagnosis and intervention for sarcopenia may impede the progression of cognitive impairment.
Clinical studies support a potential association between skeletal muscle and cognitive function, but the underlying mechanisms remain unknown. In this review, we have focused on the association between sarcopenia and cognitive function during aging and AD. It is very likely that the aging brain could have an impact on skeletal muscle function, and it is a challenge to identify all the connecting dots. People with cognitive dysfunction or AD may have less physical activity, causing a decline in muscle function. Skeletal muscle could release different cytokines and other muscle fiber-derived peptides or myokines under distinct conditions (So et al., 2014). However, here, we emphasize the myokines signal within the muscle, which potentially leads to crosstalk between skeletal muscle and the brain, involving age as a common denominator between cognitive dysfunction and AD-related cognitive impairment. Thus, skeletal muscle could act as an active endocrine organ and regulate the function of distant organs or tissues. These cytokines and myokines serve as messengers for communication between skeletal muscle and the brain (Kim et al., 2019). In the present review, we summarize potential beneficial factors and detrimental factors in muscle–brain crosstalk during aging.
Insulin-like growth factor-1 (IGF-1) is a 70-amino acid polypeptide and is synthesized by hepatocytes and other organs, including skeletal muscle (Yakar et al., 2018; Li et al., 2019). IGF-1 is essential to skeletal myogenesis, which plays a critical role in maintaining muscle mass and function (Vitale et al., 2019). Decreased IGF-1 was observed in muscle atrophy (Grounds, 2002) and was also noted to be essential for brain function. Previous studies demonstrated that IGF-1 deficiency induced cognitive impairment during aging both in human and rodent models (Deak and Sonntag, 2012; Toth et al., 2022). The level of IGF-1 is significantly decreased in sarcopenia patients, which might be due to physical inactivity (Widajanti et al., 2022). Skeletal muscle release of IGF-1 was also decreased in aged mice. However, no specific effects on muscle recovery were observed when IGF-1 alone was replenished; the combination of IGF-1 and exercise was demonstrated to reduce skeletal muscle wasting to some extent (McMahon et al., 2014). This study indicates the specific role of skeletal muscle-secreted IGF-1 in improving muscle loss and cognitive function. So far, no direct evidence shows that exogenous IGF-1 could improve cognitive function during aging. But exogenous supplementation of IGF-1 showed improvement in cognitive function. The beneficial effect is likely due to inhibition of inflammation and oxidative stress (Wang et al., 2020).
Brain-derived neurotrophic factor (BDNF), which is classified into the neurotrophin family, was initially found to be essential for brain development and the nervous system (Chao et al., 2006). BDNF is extensively expressed in the nervous system, but recent studies show that skeletal muscle can also release BDNF, which supports the myokine role of BDNF (Lebrun et al., 2006; Raschke et al., 2013). BDNF also promotes myoblast differentiation, maintains the survival of motor neurons (Sakuma et al., 2015), and functions as a contractile-inducible protein (Moreira-Pais et al., 2022). Therefore, deterioration of skeletal muscle mass in sarcopenia, along with limited physical activity or a sedentary lifestyle in the elderly, may contribute to decreased levels of BDNF. Low BDNF levels were closely related to cognitive impairment, which may increase the incidence of AD (Bathina and Das, 2015; Siuda et al., 2017). Taken together, these findings support the idea that dysregulated BDNF could be the potential link between sarcopenia and AD.
In recent years, Irisin has emerged as a key factor secreted mostly from the skeletal muscle that provides beneficial effects to both the skeletal muscle and the brain. At the molecular level, Irisin is a pro-myogenic factor that is a cleaved form of fibronectin type III domain-containing protein 5 (FNDC5) (Moreno-Navarrete et al., 2013). Irisin has been proven to enhance insulin sensitivity and boost glucose and lipid metabolism in skeletal muscle (Shen et al., 2022). Irisin has been demonstrated to ameliorate muscle wasting by accelerating myoblast fusion and protein synthesis (Huh et al., 2014). A recent study found that the level of irisin decreased during aging, and chronic administration of irisin can improve metabolic dysfunction and ameliorate skeletal muscle atrophy in aged mice (Guo et al., 2023). Regarding brain function, the genetic deletion of irisin impaired cognitive function (Islam et al., 2021). As a mediator of muscle-brain crosstalk, irisin improved cognitive function with an increase in BDNF expression (Peng and Wu, 2022). Loss of irisin is involved in cognitive impairment during aging, and exogenous administration of irisin improved cognitive function in the AD preclinical model (Madhu et al., 2022). Hence, irisin may be a promising cure for aging-related sarcopenia and cognitive impairment (Gao et al., 2013).
Secreted protein acidic and rich in cysteine (SPARC) is a novel secretory matricellular glycoprotein, defined as a myokine, which is released by skeletal muscle contraction during exercise (Aoi et al., 2013). SPARC is involved in skeletal muscle biology, which is upregulated during muscle regeneration (Petersson et al., 2013). It could counteract the abnormal deposition and accumulation of adipose tissue in aged skeletal muscle (Ko et al., 2016). In addition, during aging, the level of SPARC is decreased and SPARC knockout mice showed the sarcopenia phenotype (Ghanemi et al., 2022). Overall, there are potential beneficial roles for SPARC in skeletal muscle, and studies demonstrate that SPARC can be a potential new therapeutic target via muscle–brain crosstalk.
Interleukin-15 (IL-15) is a pleiotropic myokine released by skeletal muscle during exercise. IL-15 is widely involved in skeletal muscle metabolism (Quinn et al., 1995) and protects proteins from degradation while improving insulin sensitivity and promoting myogenesis (O'Leary et al., 2017; Nadeau and Aguer, 2019). During aging, the level of IL-15 in skeletal muscle decreases (Quinn et al., 2010) as shown in a cross-sectional study with 160 outpatient elderly people which demonstrated the inverse correlation between plasma IL-15 levels and sarcopenia (Yalcin et al., 2018). The human data correlate well with the animal data, in which it was noted that IL-15 was decreased in gastrocnemius muscle in aged rats (Marzetti et al., 2009). Another study identified that IL-15 could also serve as a detrimental pro-inflammatory factor in the brain and showed increased serum IL-15 levels, which can be utilized as a biomarker for Alzheimer's disease since IL-15 has been extensively studied in AD pathophysiology (Rentzos et al., 2006; Bishnoi et al., 2015). The contradictory roles of IL-15 in the skeletal muscle and in the brain may be due to an aging-related inflammation environment and different sources of IL-15. Low-grade inflammation during aging increases the release of the pro-inflammatory IL-15 from reticular stromal cells and other myeloid cell types, while it decreases the release of IL-15 from skeletal muscle (Naismith and Pangrazzi, 2019). The level of IL-15 could be a potential contributor to exercise benefits in sarcopenia and cognitive function improvement (Tsai et al., 2019; Pahlavani, 2022).
Leukemia inhibitory factor (LIF) is an important member of the IL-6 type cytokine family with 180 amino acid residues (Nicola and Babon, 2015). Skeletal muscle produces and releases LIF (Broholm and Pedersen, 2010), which is associated with the skeletal muscle homeostasis. Previous reports showed that LIF increased muscle glucose uptake via the PI3K-Akt signaling pathway (Brandt et al., 2015). Additionally, LIF stimulates the proliferation of satellite cells and muscle regeneration, which is a promising therapy for muscle atrophy (Kurek et al., 1998; Broholm et al., 2011). The level of LIF has been found to decline in sarcopenic obesity (Pahlavani, 2022). Importantly, LIF could cross the blood-spinal cord barrier and behave as a neuropoietic cytokine in the central nervous system. LIF is essential for the recovery of the nervous system from injury. Furthermore, LIF mediates inflammatory reactions in AD (Lemke et al., 1996). In AD patients, a higher expression of LIF was observed in degenerating human brains compared with normal brains (Soilu-Hanninen et al., 2010). However, the specific mechanism by which LIF could influence the AD brain remains unknown.
Interleukin-6 (IL-6), a member of the cytokine family, has both inflammatory and anti-inflammatory effects. The proinflammatory function of IL-6 is involved in aging-related diseases. Studies in the elderly have found that an increased level of IL-6 is associated with the occurrence of sarcopenia (Bian et al., 2017; Rong et al., 2018). However, enhanced release of IL-6 during muscle contraction also induced myogenic differentiation (Steyn et al., 2019). A combination of recombinant IL-6 and treadmill training in old mice could enhance their endurance training adaptation together with functional capacity improvement (Leuchtmann et al., 2022). However, due to the sedentary lifestyle of sarcopenia patients, the beneficial role of IL-6 may decrease, and the inflammatory effects may overwhelm the anti-inflammatory roles. As an inflammatory factor, IL-6 can cross the BBB and impair brain function (Banks et al., 1994). Inflammatory IL-6 is involved in cognitive impairment during AD. An increase in IL-6 levels in AD brains was observed, and its neutralization or inhibition of the IL-6 signaling pathway alleviated cognition decline (Silva et al., 2021). Based on the above evidence, it is likely that during aging, the release of muscle-derived anti-inflammatory IL-6 decreases, accelerating the progression of AD.
Lactate is a metabolic substrate, secreted from skeletal muscle during mechanical muscle contractile stimulation. A previous study showed that lactate promoted myoblast differentiation in vitro via myogenic determination protein-dependent signaling pathway, and moreover, lactate could cross the BBB, facilitating the expression of BDNF in the brain (El Hayek et al., 2019). The role of lactate in cognitive function is not clear, and additional studies are necessary for understanding its role in the brain. A cross-sectional study including 2,523 participants showed that a higher plasma lactate level was associated with systemic inflammation and an increased probability of mild cognitive impairment (Pan et al., 2019). However, another study evaluating the cerebrospinal fluid (CSF) lactate in 267 outpatients reported the opposite results, and the level of lactate in CSF was decreased in patients with AD (Bonomi et al., 2021). Therefore, additional studies are needed to explain these contradictory observations.
Cathepsin B is a typical member of the cysteine lysosomal protease family. It is recognized as a myokine released from skeletal muscle following exercise (Kim et al., 2019). An in vitro study showed that cathepsin B participated in myotube formation (Jane et al., 2002). During aging, cathepsin B levels were upregulated in microglia, which contributed to the generation of mitochondrial-derived reactive oxygen species (ROS), causing increased inflammation and thereby impaired memory (Ni et al., 2019). Similarly, enhanced translocation of cathepsin B reduced sirtuins and promoted proinflammatory reactions in senescent microglia, resulting in cognitive impairment (Meng et al., 2020). On the other hand, cathepsin B could cross the BBB and promote BDNF expression in the hippocampal area and improve memory function (Moon et al., 2016). It is obvious from the above reports that additional studies are needed to determine the function of cathepsin B in muscle–brain crosstalk in elderly adults.
Myostatin, which is known as growth and differentiation factor 8, is secreted by skeletal muscle. It is a negative mediator in skeletal muscle growth (Gao et al., 2013), which decreases muscle size and mass. Myostatin deficiency is beneficial to skeletal muscle metabolism (Cleasby et al., 2016). Myostatin inhibits the expression of myogenic differentiation-related genes, such as Myod and Myf5, in a smad3-dependent manner (Langley et al., 2002). Myostatin could accelerate proteolysis in the soleus and impede protein turnover in vivo and in C2C12 cells. The potential mechanism may be mediated by the phosphorylation of Smad3 (Manfredi et al., 2017). Interestingly, the level of myostatin in 12-month-old double transgenic amyloid precursor protein and presenilin 1 (APP/PS1) mice was elevated, which may trigger skeletal muscle atrophy and cognitive deficits. Knockdown of myostatin with shRNA in these mice attenuated skeletal muscle degradation and memory loss (Lin et al., 2019). Importantly, increased release of myostatin by skeletal muscle in sarcopenia patients promoted cognition decline in the elderly population, thus increasing the risk of AD (Siriett et al., 2006; Bergen et al., 2015).
Growth differentiation factor-15 (GDF-15) belongs to the transforming growth factor β (TGF-β) superfamily. Stress enhances its release. GDF-15 has been reported as a biomarker for sarcopenia. An elevated level of GDF-15 during aging was found, which was related to the decline of skeletal muscle mass and function (Kim et al., 2020). Cross-sectional and 2 year prospective analyses involving 788 participants supported the finding that an increased level of GDF-15 was associated with the prevalent sarcopenia (Kim et al., 2022). GDF-15 has also been recognized as a biomarker for aging-related cognitive decline (Jiang et al., 2016). A cohort study with 1,603 participants demonstrated an association between elevated plasma GDF-15 and an increased risk of dementia (McGrath et al., 2020). Another longitudinal Sydney Memory and Aging Study, consisting of 1,037 participants, also reached similar conclusions (Fuchs et al., 2013). Hence, GDF-15 is a detrimental mediator for muscle–brain crosstalk and a potential target for the treatment of sarcopenia and cognitive dysfunction.
Interleukin 8 (IL-8) is a CXC member of the chemokine family, which is a myokine released by skeletal muscle during exercise (Akerstrom et al., 2005). IL-8 acts as a pro-inflammatory factor in sarcopenia (da Costa Teixeira et al., 2023). A UK cohort study including 336 community-dwelling elderly men and women demonstrated that an elevated IL-8 level was associated with an increased risk of sarcopenia (Oflazoglu et al., 2020). Consistent with this, several clinical studies have shown that sarcopenia in the elderly had higher levels of IL-8 compared with the non-sarcopenia group (Fan et al., 2022; Teixeira et al., 2022). Interestingly, IL-8 is also identified as a biomarker during AD progression (Swardfager et al., 2010; Alsadany et al., 2013). In addition, a higher level of IL-8 in the elderly was associated with poorer cognitive performance (Baune et al., 2008). The potential mechanism may be related to its role in microglia migration toward Aβ deposits associated with senile plaques and activation of microglial cells (Li et al., 2009). However, besides skeletal muscle cells, other cell types, including macrophages and endothelial cells, also release IL-8 (Nielsen and Pedersen, 2007; Luo et al., 2022). Therefore, the cellular sources of IL-8 and its biological role in sarcopenia and AD need further study.
Different studies support the fact that exercises benefit the skeletal muscle system and improve memory function. Here, we summarize aerobic exercise and resistance exercise, which are two major types of exercise, along with their effects on skeletal muscle and cognitive function during aging.
Multiple animal studies demonstrate the beneficial effects of AE on sarcopenia. These studies show that AE improved skeletal muscle atrophy in sarcopenia mice and reversed chronic inflammation and dysfunctional mitochondria via sestrin2 in an AMPK alpha-2-dependent manner (Liu et al., 2021). Lifelong aerobic exercise activates autophagy and inhibits protein degradation via AMPK/PGC-1α signaling, thereby improving aging-related muscle atrophy (Liang et al., 2021). Similar findings showed the therapeutic effects of habitual aerobic exercise on sarcopenia in a senescence-accelerated mice prone8 model via enhanced mitochondrial maintenance and muscle protein synthesis (Aoki et al., 2020). Despite promising results in animal experiments, so far limited clinical studies regarding AE and its beneficial effects in humans are inconclusive. A cross-sectional study including older women treated with aerobic training, secondary lifestyle, and resistance training showed that AE could not decrease the prevalence of sarcopenia, but resistance training was effective (Supriya et al., 2022). AE showed beneficial effects on cognitive impairment as well. A meta-analysis involving 1,364 mild cognitive impairment participants demonstrated that AE could improve the cognitive function of older adults with mild cognitive impairment (Yong et al., 2021). The underlying mechanism of such beneficial effects might be due to activation of the NF-κB/miR-503/BDNF pathway (Niu et al., 2018), increased myokines (BDNF and IGF-1), and reduction of inflammatory cytokines (Tsai et al., 2018, 2019).
Resistance exercise (RE) is a form of exercise intended to increase muscular strength and endurance. Consistent evidence from clinical trials supports the benefits of RE in sarcopenia. RE improves muscle strength, muscle quality, and muscle performance in elderly adults with sarcopenia (Chen et al., 2021; Mende et al., 2022; Zhao et al., 2022). Potential mechanisms include the rejuvenation of satellite cells (Hsu et al., 2022) and the improvement of mitochondrial and autophagic function in skeletal muscle (White et al., 2016). Furthermore, a number of multilevel meta-analyses were used to demonstrate that RE enhances cognitive function regardless of cognitive status and age (Northey et al., 2018; Wilke et al., 2019; Landrigan et al., 2020; Zhang et al., 2020). It is believed that myokines are the key factors in RE that contribute to cognitive function improvement. However, it was demonstrated that RE could either increase or demonstrate no effect on the IGF-1 level (Titus et al., 2021). Furthermore, compared with traditional resistance exercise, the combination of RE and cognitive tasks improved brain function and BDNF level (Castano et al., 2022). The effect of RE on myokine release was also sex-dependent, and previous studies showed that mixed low-resistance training only increased plasma levels of BDNF in male participants, but no changes in female participants were noted. RE could be beneficial to counteract sarcopenia and memory loss in elderly adults. However, RE might be suitable only for early intervention. Patients suffering from dementia, AD, or sarcopenia are significantly associated with a low level of physical activity, higher disability, and poor quality of life. Thus, physical exercise is not a good option for these patients. In either case, a better understanding of the molecular and cellular mechanisms that mediate the benefits of physical activity will help in the development of potential therapeutic approaches.
Compared with the brain, muscles have more accessibility for intervention, especially using invasive strategies. Preclinical studies have shown that several muscle-targeted treatments enhance cognitive function. Muscle-specific overexpression of neprilysin and scFv59 or knockdown of myostatin using genetic approaches showed favorable effects on the brain. Overexpression of neprilysin in the muscle reduces Aβ amyloid deposits in the brain (Li et al., 2020), while increased scFv59 expression in the muscle reduces Aβ amyloid levels in the cerebrospinal fluid (Yang et al., 2013). Cell-based therapy is another promising strategy. For example, intramuscular injection of stem cells releasing regenerative factors enhanced neurogenesis and astrogliogenesis in the aged mouse hippocampus (Huntsman et al., 2018). These muscle-targeted strategies have translational potential in cognitive impairment therapy.
Sarcopenia and dementia are common geriatric diseases. AD is the most common cause of dementia and the fifth leading cause of death in elderly adults. Importantly, the estimated total healthcare costs for the treatment of AD in 2020 were estimated at US $305 billion, which is expected to increase to more than US $1 trillion as the population ages (Wong, 2020). To date, no effective cures for AD have been reported. Therefore, drug development for cognitive dysfunction and AD is important. Clinical observations and pre-clinical studies revealed muscle–brain crosstalk on cognitive function (Figure 1). In pre-clinical studies, it seems that myokines are the key mediators in muscle–brain crosstalk during cognitive dysfunction, but currently, no clinical trials on the effects of these myokines have been conducted. Exercise seems to be a promising intervention for sarcopenia and cognitive impairment. Due to physical inactivity in patients with sarcopenia or AD, exercise might not be the first-line intervention for patients in the late stages of the disease. However, exercise could be used as a platform to discover potential beneficial factors contributing to a favorable outcome. In addition, muscle-specific conditional knockout animals and AD preclinical models are useful for studying the underlying mechanisms. A few muscle-targeted approaches via the regulation of muscle gene expression, extrinsic supplementation, and stem cell transplantation showed promising results to improve cognitive function or promote neurogenesis. However, for future clinical applications, dose-dependent efficacy, pharmacokinetics, and delivery routes need to be taken into consideration.
Figure 1. Muscle–brain crosstalk in cognitive dysfunction. Myokines or cytokines released from skeletal muscle affect neurons and inflammation in the brain: (1) detrimental factors: cathepsin B, myostatin, and GDF-15; (2) dual roles: IL-15, IL-6, LIF, and lactate; (3) beneficial factors: FNDC5, BDNF, IGF-1, and SPARC. Exercise-enhancing release of beneficial factors and specific overexpression of neprilysin and scFv59 in the skeletal muscle could be promising strategies against cognitive dysfunction. GDF15, Growth differentiation factor-15; IL-8, interleukin 8; IL-15, interleukin-15; IL-6, interleukin-6; LIF, leukemia inhibitory factor; FNDC5, fibronectin type III domain containing protein 5; BDNF, brain-derived neurotrophic factor; IGF-1, insulin-like growth factor-1; SPARC, secreted protein acidic and rich in cysteine.
Evidence from pre-clinical studies and clinical observations supports the idea that muscle–brain crosstalk plays a critical role in cognitive function. Muscle-targeted intervention is promising for improving aging or AD-related cognitive decline. In the present review, we outlined three principal areas for skeletal muscle and brain crosstalk, namely, (a) beneficial strategies, (b) mediators that play dual roles (i.e., protective and damaging roles), and (c) strategies that may cause increased risk and advance the disease condition during aging.
XH drafted the manuscript. ST, MA, and WX reviewed and edited the manuscript. All authors have read and approved the final manuscript.
This study was supported by NIH AG070145 and USF startup funds (to WX), NIH DK119066 and William Saunders Endowment (to ST), and NIH AR070029 (to MA).
The authors declare that the research was conducted in the absence of any commercial or financial relationships that could be construed as a potential conflict of interest.
All claims expressed in this article are solely those of the authors and do not necessarily represent those of their affiliated organizations, or those of the publisher, the editors and the reviewers. Any product that may be evaluated in this article, or claim that may be made by its manufacturer, is not guaranteed or endorsed by the publisher.
Akerstrom, T., Steensberg, A., Keller, P., Keller, C., Penkowa, M., and Pedersen, B. K. (2005). Exercise induces interleukin-8 expression in human skeletal muscle. J. Physiol. 563, 507–516. doi: 10.1113/jphysiol.2004.077610
Alsadany, M. A., Shehata, H. H., Mohamad, M. I., and Mahfouz, R. G. (2013). Histone deacetylases enzyme, copper, and IL-8 levels in patients with Alzheimer's disease. Am. J. Alzheimers Dis. Other Demen. 28, 54–61. doi: 10.1177/1533317512467680
Aoi, W., Naito, Y., Takagi, T., Tanimura, Y., Takanami, Y., Kawai, Y., et al. (2013). A novel myokine, secreted protein acidic and rich in cysteine (SPARC), suppresses colon tumorigenesis via regular exercise. Gut. 62, 882–889. doi: 10.1136/gutjnl-2011-300776
Aoki, K., Konno, M., Honda, K., Abe, T., Nagata, T., Takehara, M., et al. (2020). Habitual aerobic exercise diminishes the effects of sarcopenia in senescence-accelerated mice prone8 model. Geriatrics. 5:48. doi: 10.3390/geriatrics5030048
Banks, W. A., Kastin, A. J., and Gutierrez, E. G. (1994). Penetration of interleukin-6 across the murine blood-brain-barrIER. Neurosci. Lett. 179, 53–56. doi: 10.1016/0304-3940(94)90933-4
Bathina, S., and Das, U. N. (2015). Brain-derived neurotrophic factor and its clinical implications. Arch. Med. Sci. 11, 1164–1178. doi: 10.5114/aoms.2015.56342
Baune, B. T., Ponath, G., Golledge, J., Varga, G., Arolt, V., Rothermundt, M., et al. (2008). Association between IL-8 cytokine and cognitive performance in an elderly general population - the MEMO-study. Neurobiol. Aging. 29, 937–944. doi: 10.1016/j.neurobiolaging.2006.12.003
Beard, J. R., Officer, A., de Carvalho, I. A., Sadana, R., Pot, A. M., Michel, J. P., et al. (2016). The world report on ageing and health: a policy framework for healthy ageing. Lancet. 387, 2145–2154. doi: 10.1016/S0140-6736(15)00516-4
Beckwee, D., Delaere, A., Aelbrecht, S., Baert, V., Beaudart, C., Bruyere, O., et al. (2019). Exercise Interventions for the prevention and treatment of sarcopenia. a systematic umbrella review. J. Nutr. Health Aging. 23, 494–502. doi: 10.1007/s12603-019-1196-8
Beeri, M. S., Leugrans, S. E., Delbono, O., Bennett, D. A., and Buchman, A. S. (2021). Sarcopenia is associated with incident Alzheimer's dementia, mild cognitive impairment, and cognitive decline. J. Am. Geriatr. Soc. 69, 1826–1835. doi: 10.1111/jgs.17206
Bergen, H. R., Farr, J. N., Vanderboom, P. M., Atkinson, E. J., White, T. A., Singh, R. J., et al. (2015). LeBrasseur NK. Myostatin as a mediator of sarcopenia versus homeostatic regulator of muscle mass: insights using a new mass spectrometry-based assay. Skelet. Muscle. 5, 21. doi: 10.1186/s13395-015-0047-5
Bian, A. L., Hu, H. Y., Rong, Y. D., Wang, J., Wang, J. X., and Zhou, X. Z. (2017). A study on relationship between elderly sarcopenia and inflammatory factors IL-6 and TNF-alpha. Eur. J. Med. Res. 22, 25. doi: 10.1186/s40001-017-0266-9
Bishnoi, R. J., Palmer, R. F., and Royall, D. R. (2015). Serum Interleukin (IL)-15 as a biomarker of Alzheimer's disease. PLoS ONE. 10:e117282. doi: 10.1371/journal.pone.0117282
Bonomi, C. G., De Lucia, V., Mascolo, A. P., Assogna, M., Motta, C., Scaricamazza, E., et al. (2021). Brain energy metabolism and neurodegeneration: hints from CSF lactate levels in dementias. Neurobiol. Aging. 105, 333–339. doi: 10.1016/j.neurobiolaging.2021.05.011
Brandt, N., O'Neill, H. M., Kleinert, M., Schjerling, P., Vernet, E., Steinberg, G. R., et al. (2015). Leukemia inhibitory factor increases glucose uptake in mouse skeletal muscle. Am. J. Physiol. Endocrinol. Metab. 309, E142–E153. doi: 10.1152/ajpendo.00313.2014
Broholm, C., Laye, M. J., Brandt, C., Vadalasetty, R., Pilegaard, H., Pedersen, B. K., et al. (2011). LIF is a contraction-induced myokine stimulating human myocyte proliferation. J. Appl. Physiol. 111, 251–259. doi: 10.1152/japplphysiol.01399.2010
Broholm, C., and Pedersen, B. K. (2010). Leukaemia inhibitory factor - an exercise-induced myokine. Exerc. Immunol. Rev. 16, 77–85.
Castano, L. A. A., Castillo de Lima, V., Barbieri, J. F., de Lucena, E. G. P., Gaspari, A. F., Arai, H., et al. (2022). Resistance training combined with cognitive training increases brain derived neurotrophic factor and improves cognitive function in healthy older adults. Front. Psychol. 13:870561. doi: 10.3389/fpsyg.2022.870561
Chang, K. V., Hsu, T. H., Wu, W. T., Huang, K. C., and Han, D. S. (2016). Association between sarcopenia and cognitive impairment: a systematic review and meta-analysis. J. Am. Med. Dir. Assoc. 17, 1164.e7–1164.e15. doi: 10.1016/j.jamda.2016.09.013
Chao, M. V., Rajagopal, R., and Lee, F. S. (2006). Neurotrophin signalling in health and disease. Clin. Sci. 110, 167–173. doi: 10.1042/CS20050163
Chen, N., He, X. F., Feng, Y. W., Ainsworth, B. E., and Liu, Y. (2021). Effects of resistance training in healthy older people with sarcopenia: a systematic review and meta-analysis of randomized controlled trials. Eur. Rev. Aging Phys. Act. 18, 23. doi: 10.1186/s11556-021-00277-7
Chen, X., Cao, M. J., Liu, M., Liu, S. P., Zhao, Z. L., and Chen, H. Y. (2022). Association between sarcopenia and cognitive impairment in the older people: a meta-analysis. Eur. Geriatr. Med. 13, 771–787. doi: 10.1007/s41999-022-00661-1
Cho, M. R., Lee, S., and Song, S. K. A. (2022). Review of sarcopenia pathophysiology, diagnosis, treatment and future direction. J. Korean Med. Sci. 37:e146. doi: 10.3346/jkms.2022.37.e146
Cipolli, G. C., Yassuda, M. S., and Aprahamian, I. (2019). Sarcopenia is associated with cognitive impairment in older adults: a systematic review and meta-analysis. J. Nutr. Health Aging. 23, 525–531. doi: 10.1007/s12603-019-1188-8
Cleasby, M. E., Jamieson, P. M., and Atherton, P. J. (2016). Insulin resistance and sarcopenia: mechanistic links between common co-morbidities. J. Endocrinol. 229, R67–R81. doi: 10.1530/JOE-15-0533
da Costa Teixeira, L. A., Avelar, N. C. P., Peixoto, M. F. D., Parentoni, A. N., Santos, J. M. D., Pereira, F. S. M., et al. (2023). Inflammatory biomarkers at different stages of Sarcopenia in older women. Sci. Rep. 13, 10367. doi: 10.1038/s41598-023-37229-3
Deak, F., and Sonntag, W. E. (2012). Aging, synaptic dysfunction, and insulin-like growth factor (IGF)-1. J. Gerontol. A. Biol. Sci. Med. Sci. 67, 611–625. doi: 10.1093/gerona/gls118
DeTure, M. A., and Dickson, D. W. (2019). The neuropathological diagnosis of Alzheimer's disease. Mol. Neurodegener. 14, 32. doi: 10.1186/s13024-019-0333-5
El Hayek, L., Khalifeh, M., Zibara, V., Assaad, R. A., Emmanuel, N., Karnib, N., et al. (2019). Lactate mediates the effects of exercise on learning and memory through SIRT1-dependent activation of hippocampal Brain-Derived Neurotrophic Factor (BDNF). J. Neurosci. 39, 2369–2382. doi: 10.1523/JNEUROSCI.1661-18.2019
Fan, Z., Yang, J. Y., Guo, Y., Liu, Y. X., and Zhong, X. Y. (2022). Altered levels of circulating mitochondrial DNA in elderly people with sarcopenia: association with mitochondrial impairment. Exp. Gerontol. 163:111802. doi: 10.1016/j.exger.2022.111802
Fuchs, T., Trollor, J. N., Crawford, J., Brown, D. A., Baune, B. T., Samaras, K., et al. (2013). Macrophage inhibitory cytokine-1 is associated with cognitive impairment and predicts cognitive decline - the Sydney memory and aging study. Aging Cell. 12, 882–889. doi: 10.1111/acel.12116
Gao, F., Kishida, T., Ejima, A., Gojo, S., and Mazda, O. (2013). Myostatin acts as an autocrine/paracrine negative regulator in myoblast differentiation from human induced pluripotent stem cells. Biochem. Biophys. Res. Commun. 431, 309–314. doi: 10.1016/j.bbrc.2012.12.105
Ghanemi, A., Melouane, A., Yoshioka, M., and St-Amand, J. (2022). Secreted protein acidic and rich in cysteine (Sparc) KO leads to an accelerated ageing phenotype which is improved by exercise whereas SPARC overexpression mimics exercise effects in mice. Metabolites. 12:125. doi: 10.3390/metabo12020125
Grounds, M. D. (2002). Reasons for the degeneration of ageing skeletal muscle: a central role for IGF-1 signalling. Biogerontology. 3, 19–24. doi: 10.1023/A:1015234709314
Guo, M. W., Yao, J., Li, J., Zhang, J., Wang, D. M., Zuo, H., et al. (2023). Irisin ameliorates age-associated sarcopenia and metabolic dysfunction. J. Cachexia Sarcopenia Muscle 14, 391–405. doi: 10.1002/jcsm.13141
Hambright, W. S., Niedernhofer, L. J., Huard, J., and Robbins, P. D. (2019). Murine models of accelerated aging and musculoskeletal disease. Bone. 125, 122–127. doi: 10.1016/j.bone.2019.03.002
Hou, Y. J., Dan, X. L., Babbar, M., Wei, Y., Hasselbalch, S. G., Croteau, D. L., et al. (2019). Ageing as a risk factor for neurodegenerative disease. Nat. Rev. Neurol. 15, 565–581. doi: 10.1038/s41582-019-0244-7
Hsu, W. B., Lin, S. J., Hung, J. S., Chen, M. H., Lin, C. Y., Hsu, W. H., et al. (2022). Effect of resistance training on satellite cells in old mice - a transcriptome study. Bone Joint Res. 11, 121–133. doi: 10.1302/2046-3758.112.BJR-2021-0079.R2
Hu, Y. S., Peng, W. J., Ren, R. J., Wang, Y., and Wang, G. (2022). Sarcopenia and mild cognitive impairment among elderly adults: the first longitudinal evidence from CHARLS. J. Cachexia Sarcopenia Muscle 13, 2944–2952. doi: 10.1002/jcsm.13081
Huang, X. X., Zhao, X. Y., Li, B., Cai, Y., Zhang, S. F., Wan, Q. Q., et al. (2022). Comparative efficacy of various exercise interventions on cognitive function in patients with mild cognitive impairment or dementia: a systematic review and network meta-analysis. J. Sport Health Sci. 11, 212–223. doi: 10.1016/j.jshs.2021.05.003
Huh, J. Y., Dincer, F., Mesfum, E., and Mantzoros, C. S. (2014). Irisin stimulates muscle growth-related genes and regulates adipocyte differentiation and metabolism in humans. Int. J. Obes. 38, 1538–1544. doi: 10.1038/ijo.2014.42
Huntsman, H. D., Rendeiro, C., Merritt, J. R., Pincu, Y., Cobert, A., De Lisio, M., et al. (2018). The impact of mechanically stimulated muscle-derived stromal cells on aged skeletal muscle. Exp. Gerontol. 103, 35–46. doi: 10.1016/j.exger.2017.12.012
Islam, M. R., Valaris, S., Young, M. F., Haley, E. B., Luo, R., Bond, S. F., et al. (2021). Exercise hormone irisin is a critical regulator of cognitive function. Nat. Metab. 3, 1058–1070. doi: 10.1038/s42255-021-00438-z
Jane, D. T., DaSilva, L., Koblinski, J., Horwitz, M., Sloane, B. F., and Dufresne, M. J. (2002). Evidence for the involvement of cathepsin B in skeletal myoblast differentiation. J. Cell. Biochem. 84, 520–531. doi: 10.1002/jcb.10042
Jiang, J. Y., Wen, W., and Sachdev, P. S. (2016). Macrophage inhibitory cytokine-1/growth differentiation factor 15 as a marker of cognitive ageing and dementia. Curr. Opin. Psychiatry. 29, 181–186. doi: 10.1097/YCO.0000000000000225
Kim, H., Kim, K. M., Kang, M. J., and Lim, S. (2020). Growth differentiation factor-15 as a biomarker for sarcopenia in aging humans and mice. Exp. Gerontol. 142:111115. doi: 10.1016/j.exger.2020.111115
Kim, M., Walston, J. D., and Won, C. W. (2022). Associations between elevated growth differentiation factor-15 and sarcopenia among community-dwelling older adults. J. Gerontol. A. Biol. Sci. Med. Sci. 77, 770–780. doi: 10.1093/gerona/glab201
Kim, S., Choi, J. Y., Moon, S., Park, D. H., Kwak, H. B., and Kang, J. H. (2019). Roles of myokines in exercise-induced improvement of neuropsychiatric function. Pflugers Arch. 471, 491–505. doi: 10.1007/s00424-019-02253-8
Ko, B. J., Chang, Y., Jung, H. S., Yun, K. E., Kim, C. W., Park, H. S., et al. (2016). Relationship between low relative muscle mass and coronary artery calcification in healthy adults. Arterioscler. Thromb. Vasc. Biol. 36, 1016–1021. doi: 10.1161/ATVBAHA.116.307156
Kurek, J. B., Bower, J. J., White, J. D., Muldoon, C. M., and Austin, L. (1998). Leukaemia inhibitory factor and other cytokines as factors influencing regeneration of skeletal muscle. Basic Appl. Myol. 8, 347–360.
Landrigan, J. F., Bell, T., Crowe, M., Clay, O. J., and Mirman, D. (2020). Lifting cognition: a meta-analysis of effects of resistance exercise on cognition. Psychol. Res. 84, 1167–1183. doi: 10.1007/s00426-019-01145-x
Langley, B., Thomas, M., Bishop, A., Sharma, M., Gilmour, S., and Kambadur, R. (2002). Myostatin inhibits myoblast differentiation by down-regulating MyoD expression. J. Biol. Chem. 277, 49831–49840. doi: 10.1074/jbc.M204291200
Lebrun, B., Bariohay, B., Moyse, E., and Jean, A. (2006). Brain-derived neurotrophic factor (BDNF) and food intake regulation: a minireview. Auton. Neurosci. 126, 30–38. doi: 10.1016/j.autneu.2006.02.027
Lee, H., and Lim, Y. (2022). The potential role of myokines/hepatokines in the progression of neuronal damage in streptozotocin and high-fat diet-induced type 2 diabetes mellitus mice. Biomedicines. 10:1521. doi: 10.3390/biomedicines10071521
Lemke, R., Gadient, R. A., Schliebs, R., Bigl, V., and Patterson, P. H. (1996). Neuronal expression of leukemia inhibitory factor (LIF) in the rat brain. Neurosci. Lett. 215, 205–208. doi: 10.1016/0304-3940(96)12986-4
Leuchtmann, A. B., Furrer, R., Steurer, S. A., Schneider-Heieck, K., Karrer-Cardel, B., Sagot, Y., et al. (2022). Interleukin-6 potentiates endurance training adaptation and improves functional capacity in old mice. J. Cachexia Sarcopenia Muscle. 13, 1164–1176. doi: 10.1002/jcsm.12949
Li, F. K., Bian, D. S., Bai, T. T., Jin, H. Y., Sun, X., Lu, J. C., et al. (2022). Cognitive impairment is associated with sarcopenia mainly related to attention and calculation in hospitalized Chinese elderly men. Asia Pac. J. Clin. Nutr. 31, 534–542. doi: 10.6133/apjcn.202209_31(3)0.0021
Li, J., Choi, E., Yu, H. T., and Bai, X. C. (2019). Structural basis of the activation of type 1 insulin-like growth factor receptor. Nat. Commun. 10, 4567. doi: 10.1038/s41467-019-12564-0
Li, K. S., Liu, S. Y., Yao, S. P., Wang, B. Y., Dai, D. W., and Yao, L. F. (2009). Interaction between Interleukin-8 and methylenetetrahydrofolate reductase genes modulates Alzheimer's disease risk. Dement. Geriatr. Cogn. Disord. 27, 286–291. doi: 10.1159/000204766
Li, Y., Wang, Y., Wang, J., Chong, K. Y., Xu, J., Liu, Z., et al. (2020). Expression of neprilysin in skeletal muscle by ultrasound-mediated gene transfer (Sonoporation) reduces amyloid burden for AD. Mol. Ther. Methods Clin. Dev. 17, 300–308. doi: 10.1016/j.omtm.2019.12.012
Liang, J. L., Zhang, H., Zeng, Z. Z., Wu, L. W., Zhang, Y., Guo, Y. J., et al. (2021). Lifelong aerobic exercise alleviates sarcopenia by activating autophagy and inhibiting protein degradation via the AMPK/PGC-1 alpha signaling pathway. Metabolites. 11:323. doi: 10.3390/metabo11050323
Lin, P., Sun, J. Y., Cheng, Q., Yang, Y., Cordato, D., and Gao, J. Q. (2021). The development of pharmacological therapies for Alzheimer's disease. Neurol. Ther. 10, 609–626. doi: 10.1007/s40120-021-00282-z
Lin, Y. S., Lin, F. Y., and Hsiao, Y. H. (2019). Myostatin is associated with cognitive decline in an animal model of Alzheimer's disease. Mol. Neurobiol. 56, 1984–1991. doi: 10.1007/s12035-018-1201-y
Liu, S. J., Yu, C. X., Xie, L. J., Niu, Y. M., and Fu, L. (2021). Aerobic exercise improves mitochondrial function in sarcopenia mice through Sestrin2 in an AMPK alpha 2-dependent manner. J. Gerontol. A. Biol. Sci. Med. Sci. 76, 1161–1168. doi: 10.1093/gerona/glab029
Luo, J., Zhang, M., Wang, Z., Yan, L., and Liu, Y. (2022). Anti-beta(2)GPI/beta(2)GPI induces neutrophil pyroptosis and thereby enhances ICAM-1 and IL-8 expression in endothelial cells. Int. J. Mol. Med. 49, 64. doi: 10.3892/ijmm.2022.5120
Madhu, L. N., Somayaji, Y., and Shetty, A. K. (2022). Promise of irisin to attenuate cognitive dysfunction in aging and Alzheimer's disease. Ageing Res. Rev. 78:101637. doi: 10.1016/j.arr.2022.101637
Manfredi, L. H., Paula-Gomes, S., Zanon, N. M., and Kettelhut, I. C. (2017). Myostatin promotes distinct responses on protein metabolism of skeletal and cardiac muscle fibers of rodents. Braz. J. Med. Biol. Res. 50:e6733. doi: 10.1590/1414-431x20176733
Marucci, G., Buccioni, M., Dal Ben, D., Lambertucci, C., Volpini, R., and Amenta, F. (2021). Efficacy of acetylcholinesterase inhibitors in Alzheimer's disease. Neuropharmacology. 190:108352. doi: 10.1016/j.neuropharm.2020.108352
Marzetti, E., Carter, C. S., Wohlgemuth, S. E., Lees, H. A., Giovannini, S., Anderson, B., et al. (2009). Changes in IL-15 expression and death-receptor apoptotic signaling in rat gastrocnemius muscle with aging and life-long calorie restriction. Mech. Ageing Dev. 130, 272–280. doi: 10.1016/j.mad.2008.12.008
McGrath, E. R., Himali, J. J., Levy, D., Conner, S. C., DeCarli, C., Pase, M. P., et al. (2020). Growth differentiation factor 15 and NT-proBNP as blood-based markers of vascular brain injury and dementia. J. Am. Heart Assoc. 9:e014659. doi: 10.1161/JAHA.119.014659
McMahon, C. D., Chai, R., Radley-Crabb, H. G., Watson, T., Matthews, K. G., Sheard, P. W., et al. (2014). Lifelong exercise and locally produced insulin-like growth factor-1 (IGF-1) have a modest influence on reducing age-related muscle wasting in mice. Scand. J. Med. Sci. Sports. 24, E423–E435. doi: 10.1111/sms.12200
Mende, E., Moeinnia, N., Schaller, N., Weiss, M., Haller, B., Halle, M., et al. (2022). Progressive machine-based resistance training for prevention and treatment of sarcopenia in the oldest old: a systematic review and meta-analysis. Exp. Gerontol. 163:111767. doi: 10.1016/j.exger.2022.111767
Meng, J., Liu, Y. C., Xie, Z., Qing, H., Lei, P., and Ni, J. J. (2020). Nucleus distribution of cathepsin B in senescent microglia promotes brain aging through degradation of sirtuins. Neurobiol. Aging. 96, 255–266. doi: 10.1016/j.neurobiolaging.2020.09.001
Moon, H. Y., Becke, A., Berron, D., Becker, B., Sah, N., Benoni, G., et al. (2016). Running-induced systemic cathepsin B secretion is associated with memory function. Cell Metab. 24, 332–340. doi: 10.1016/j.cmet.2016.05.025
Moreira-Pais, A., Ferreira, R., Oliveira, P. A., and Duarte, J. A. A. (2022). neuromuscular perspective of sarcopenia pathogenesis: deciphering the signaling pathways involved. Geroscience. 44, 1199–1213. doi: 10.1007/s11357-021-00510-2
Moreno-Navarrete, J. M., Ortega, F., Serrano, M., Guerra, E., Pardo, G., Tinahones, F., et al. (2013). Irisin is expressed and produced by human muscle and adipose tissue in association with obesity and insulin resistance. J. Clin. Endocrinol. Metab. 98, E769–E778. doi: 10.1210/jc.2012-2749
Nadeau, L., and Aguer, C. (2019). Interleukin-15 as a myokine: mechanistic insight into its effect on skeletal muscle metabolism. Appl. Physiol. Nutr. Metab. 44, 229–238. doi: 10.1139/apnm-2018-0022
Nagase, T., and Tohda, C. (2021). Skeletal muscle atrophy-induced hemopexin accelerates onset of cognitive impairment in Alzheimer's disease. J. Cachexia Sarcopenia Muscle. 12, 2199–2210. doi: 10.1002/jcsm.12830
Naismith, E., and Pangrazzi, L. (2019). The impact of oxidative stress, inflammation, and senescence on the maintenance of immunological memory in the bone marrow in old age. Biosci. Rep. 39:BSR20190371. doi: 10.1042/BSR20190371
Ni, J. J., Wu, Z., Stoka, V., Meng, J., Hayashi, Y., Peters, C., et al. (2019). Increased expression and altered subcellular distribution of cathepsin B in microglia induce cognitive impairment through oxidative stress and inflammatory response in mice. Aging Cell. 18:e12856. doi: 10.1111/acel.12856
Nicola, N. A., and Babon, J. J. (2015). Leukemia inhibitory factor (LIF). Cytokine Growth Factor Rev. 26, 533–544. doi: 10.1016/j.cytogfr.2015.07.001
Nielsen, A. R., and Pedersen, B. K. (2007). The biological roles of exercise-induced cytokines: IL-6, IL-8, and IL-15. Appl. Physiol. Nutr. Metab. 32, 833–839. doi: 10.1139/H07-054
Niu, Y. L., Wan, C. X., Zhou, B., Wang, J. L., Wang, J., Chen, X. N., et al. (2018). Aerobic exercise relieved vascular cognitive impairment via NF-kappa B/miR-503/BDNF pathway. Am. J. Transl. Res. 10, 753–761.
North, B. J., and Sinclair, D. A. (2012). The intersection between aging and cardiovascular disease. Circ. Res. 110, 1097–1108. doi: 10.1161/CIRCRESAHA.111.246876
Northey, J. M., Cherbuin, N., Pumpa, K. L., Smee, D. J., and Rattray, B. (2018). Exercise interventions for cognitive function in adults older than 50: a systematic review with meta-analysis. Br. J. Sport Med. 52, 154–160. doi: 10.1136/bjsports-2016-096587
Nunes, D., Loureiro, J. A., and Pereira, M. C. (2022). Drug delivery systems as a strategy to improve the efficacy of FDA-approved Alzheimer's drugs. Pharmaceutics. 14:2296. doi: 10.3390/pharmaceutics14112296
Oflazoglu, U., Alacacioglu, A., Varol, U., Kucukzeybek, Y., Salman, T., Onal, H. T., et al. (2020). The role of inflammation in adjuvant chemotherapy-induced sarcopenia (Izmir Oncology Group (IZOG) study). Support. Care Cancer. 28, 3965–3977. doi: 10.1007/s00520-020-05477-y
O'Leary, M. F., Wallace, G. R., Bennett, A. J., Tsintzas, K., and Jones, S. W. (2017). IL-15 promotes human myogenesis and mitigates the detrimental effects of TNF alpha on myotube development. Sci. Rep. 7, 12997. doi: 10.1038/s41598-017-13479-w
Pahlavani, H. A. (2022). Exercise therapy for people with sarcopenic obesity: myokines and adipokines as effective actors. Front. Endocrinol. 13:811751. doi: 10.3389/fendo.2022.811751
Pan, H., Huang, X. J., Li, F., Ren, M., Zhang, J., Xu, M. T., et al. (2019). Association among plasma lactate, systemic inflammation, and mild cognitive impairment: a community-based study. Neurol. Sci. 40, 1667–1673. doi: 10.1007/s10072-019-03900-9
Peng, J., and Wu, J. H. (2022). Effects of the FNDC5/Irisin on elderly dementia and cognitive impairment. Front. Aging Neurosci. 14:863901. doi: 10.3389/fnagi.2022.863901
Peng, T. C., Chen, W. L., Wu, L. W., Chang, Y. W., and Kao, T. W. (2020). Sarcopenia and cognitive impairment: a systematic review and meta-analysis. Clin. Nutr. 39, 2695–2701. doi: 10.1016/j.clnu.2019.12.014
Petersson, S. J., Jorgensen, L. H., Andersen, D. C., Norgaard, R. C., Jensen, C. H., and Schroder, H. D. (2013). SPARC is up-regulated during skeletal muscle regeneration and inhibits myoblast differentiation. Histol. Histopathol. 28, 1451–1460. doi: 10.14670/HH-28.1451
Quinn, L. S., Anderson, B. G., Strait-Bodey, L., and Wolden-Hanson, T. (2010). Serum and muscle interleukin-15 levels decrease in aging mice: correlation with declines in soluble interleukin-15 receptor alpha expression. Exp. Gerontol. 45, 106–112. doi: 10.1016/j.exger.2009.10.012
Quinn, L. S., Haugk, K. L., and Grabstein, K. H. (1995). Interleukin-15 - a novel anabolic cytokine for skeletal-muscle. Endocrinology. 136, 3669–3672. doi: 10.1210/endo.136.8.7628408
Ramoo, K., Hairi, N. N., Yahya, A., Choo, W. Y., Hairi, F. M., Peramalah, D., et al. (2022). Longitudinal association between sarcopenia and cognitive impairment among older adults in rural Malaysia. Int. J. Environ. Res. Public Health. 19:4723. doi: 10.3390/ijerph19084723
Raschke, S., Elsen, M., Gassenhuber, H., Sommerfeld, M., Schwahn, U., Brockmann, B., et al. (2013). Evidence against a Beneficial Effect of Irisin in Humans. PLoS ONE. 8:e73680. doi: 10.1371/journal.pone.0073680
Rendeiro, C., and Rhodes, J. S. (2018). A new perspective of the hippocampus in the origin of exercise-brain interactions. Brain Struct. Funct. 223, 2527–2545. doi: 10.1007/s00429-018-1665-6
Rentzos, M., Zoga, M., Paraskevas, G. P., Kapaki, E., Rombos, A., Nikolaou, C., et al. (2006). IL-15 is elevated in cerebrospinal fluid of patients with Alzheimer's disease and frontotemporal dementia. J. Geriatr. Psychiatry Neurol. 19, 114–117. doi: 10.1177/0891988706286226
Rong, Y. D., Bian, A. L., Hu, H. Y., Ma, Y., and Zhou, X. Z. (2018). Study on relationship between elderly sarcopenia and inflammatory cytokine IL-6, anti-inflammatory cytokine IL-10. BMC Geriatr. 18, 308. doi: 10.1186/s12877-018-1007-9
Rossi, S., Zanier, E. R., Mauri, I., Columbo, A., and Stocchetti, N. (2001). Brain temperature, body core temperature, and intracranial pressure in acute cerebral damage. J. Neurol. Neurosurg. Psychiatry. 71, 448–454. doi: 10.1136/jnnp.71.4.448
Sakuma, K., Aoi, W., and Yamaguchi, A. (2015). Current understanding of sarcopenia: possible candidates modulating muscle mass. Pflugers Archiv. 467, 213–229. doi: 10.1007/s00424-014-1527-x
Salinas-Rodriguez, A., Palazuelos-Gonzalez, R., Rivera-Almaraz, A., and Manrique-Espinoza, B. (2021). Longitudinal association of sarcopenia and mild cognitive impairment among older Mexican adults. J. Cachexia Sarcopenia Muscle. 12, 1848–1859. doi: 10.1002/jcsm.12787
Shen, S. N., Liao, Q. W., Chen, X. P., Peng, C., and Lin, L. G. (2022). The role of irisin in metabolic flexibility: beyond adipose tissue browning. Drug Discov. Today. 27, 2261–2267. doi: 10.1016/j.drudis.2022.03.019
Silva, N., Goncalves, R. A., Pascoal, T. A., Lima, R. A. S., Resende, E. D. F., Vieira, E. L. M., et al. (2021). Pro-inflammatory interleukin-6 signaling links cognitive impairments and peripheral metabolic alterations in Alzheimer's disease. Transl. Psychiatry. 11, 251. doi: 10.1038/s41398-021-01349-z
Siriett, V., Platt, L., Salerno, M. S., Ling, N., Kambadur, R., and Sharma, M. (2006). Prolonged absence of myostatin reduces sarcopenia. J. Cell. Physiol. 209, 866–873. doi: 10.1002/jcp.20778
Siuda, J., Patalong-Ogiewa, M., Zmuda, W., Targosz-Gajniak, M., Niewiadomska, E., Matuszek, I., et al. (2017). Cognitive impairment and BDNF serum levels. Neurol. Neurochir. Pol. 51, 24–32. doi: 10.1016/j.pjnns.2016.10.001
So, B., Kim, H. J., Kim, J., and Song, W. (2014). Exercise-induced myokines in health and metabolic diseases. Integr. Med. Res. 3, 172–179. doi: 10.1016/j.imr.2014.09.007
Soilu-Hanninen, M., Broberg, E., Roytta, M., Mattila, P., Rinne, J., and Hukkanen, V. (2010). Expression of LIF and LIF receptor beta in Alzheimer's and Parkinson's diseases. Acta. Neurol. Scand. 121, 44–50. doi: 10.1111/j.1600-0404.2009.01179.x
Steyn, P. J., Dzobo, K., Smith, R. I., and Myburgh, K. H. (2019). Interleukin-6 induces myogenic differentiation via JAK2-STAT3 Signaling in Mouse C2C12 myoblast cell line and primary human myoblasts. Int. J. Mol. Sci. 20:5273. doi: 10.3390/ijms20215273
Stump, C. S., Henriksen, E. J., Wei, Y. Z., and Sowers, J. R. (2006). The metabolic syndrome: role of skeletal muscle metabolism. Ann. Med. 38, 389–402. doi: 10.1080/07853890600888413
Supriya, R., Singh, K. P., Gao, Y., Gu, Y. D., and Baker, J. S. (2022). Effect of exercise on secondary sarcopenia: a comprehensive literature review. Biology. 11:51. doi: 10.3390/biology11010051
Swardfager, W., Lanctot, K., Rothenburg, L., Wong, A., Cappell, J., and Herrmann, N. A. (2010). Meta-analysis of cytokines in Alzheimer's disease. Biol. Psychiatry. 68, 930–941. doi: 10.1016/j.biopsych.2010.06.012
Teixeira, L., dos Santos, J., Parentoni, A., Lima, L., Duarte, T., Brant, F., et al. (2022). Adiponectin is a contributing factor of low appendicular lean mass in older community-dwelling women: a cross-sectional study. J. Clin. Med. 11:7175. doi: 10.3390/jcm11237175
Tieland, M., Trouwborst, I., and Clark, B. C. (2018). Skeletal muscle performance and ageing. J. Cachexia Sarcopenia Muscle. 9, 3–19. doi: 10.1002/jcsm.12238
Titus, J., Bray, N. W., Kamkar, N., Camicioli, R., Nagamatsu, L. S., Speechley, M., et al. (2021). The role of physical exercise in modulating peripheral inflammatory and neurotrophic biomarkers in older adults: a systematic review and meta-analysis. Mech. Ageing Dev. 194:111431. doi: 10.1016/j.mad.2021.111431
Toth, L., Czigler, A., Hegedus, E., Komaromy, H., Amrein, K., Czeiter, E., et al. (2022). Age-related decline in circulating IGF-1 associates with impaired neurovascular coupling responses in older adults. Geroscience. 44, 2771–2783. doi: 10.1007/s11357-022-00623-2
Tsai, C. L., Pai, M. C., Ukropec, J., and Ukropcova, B. (2019). Distinctive effects of aerobic and resistance exercise modes on neuro-cognitive and biochemical changes in individuals with mild cognitive impairment. Curr. Alzheimer Res. 16, 316–332. doi: 10.2174/1567205016666190228125429
Tsai, C. L., Ukropec, J., Ukropcova, B., and Pai, M. C. (2018). An acute bout of aerobic or strength exercise specifically modifies circulating exerkine levels and neurocognitive functions in elderly individuals with mild cognitive impairment. Neuroimage Clin. 17, 272–284. doi: 10.1016/j.nicl.2017.10.028
Vitale, G., Pellegrino, G., Vollery, M., and Hofland, L. J. (2019). ROLE of IGF-1 system in the modulation of longevity: controversies and new insights from a centenarians' perspective. Front. Endocrinol. 10:27. doi: 10.3389/fendo.2019.00027
Wang, F., Wang, L., Wang, Y. F., Li, D., Hu, T. P., Sun, M. Y., et al. (2020). Exogenous IGF-1 improves cognitive function in rats with high-fat diet consumption. J. Mol. Endocrinol. 64, 115–123. doi: 10.1530/JME-19-0150
White, Z., Terrill, J., White, R. B., McMahon, C., Sheard, P., Grounds, M. D., et al. (2016). Voluntary resistance wheel exercise from mid-life prevents sarcopenia and increases markers of mitochondrial function and autophagy in muscles of old male and female C57BL/6J mice. Skelet. Muscle. 6, 45. doi: 10.1186/s13395-016-0117-3
Widajanti, N., Soelistijo, S., Hadi, U., Thaha, M., Aditiawardana, Widodo, et al. (2022). Association between sarcopenia and insulin-like growth factor-1, myostatin, and insulin resistance in elderly patients undergoing hemodialysis. J. Aging Res. 2022:1327332. doi: 10.1155/2022/1327332
Wilke, J., Giesche, F., Klier, K., Vogt, L., Herrmann, E., and Banzer, W. (2019). Acute effects of resistance exercise on cognitive function in healthy adults: a systematic review with multilevel meta-analysis. Sports Med. 49, 905–916. doi: 10.1007/s40279-019-01085-x
Wong, W. (2020). Economic burden of Alzheimer disease and managed care considerations. Am. J. Manag. Care. 26, S177–S183. doi: 10.37765/ajmc.2020.88482
Wu, B., Lyu, Y. B., Cao, Z. J., Wei, Y., Shi, W. Y., Gao, X., et al. (2021). Associations of sarcopenia, handgrip strength and calf circumference with cognitive impairment among Chinese older adults. Biomed. Environ. Sci. 34, 859–870. doi: 10.3967/bes2021.119
Yakar, S., Werner, H., and Rosen, C. J. (2018). Insulin-like growth factors: actions on the skeleton. J. Mol. Endocrinol. 61, T115–T137. doi: 10.1530/JME-17-0298
Yalcin, A., Silay, K., Balik, A. R., Avcioglu, G., and Aydin, A. S. (2018). The relationship between plasma interleukin-15 levels and sarcopenia in outpatient older people. Aging Clin. Exp. Res. 30, 783–790. doi: 10.1007/s40520-017-0848-y
Yang, J., Pattanayak, A., Song, M., Kou, J., Taguchi, H., Paul, S., et al. (2013). Muscle-directed anti-abeta single-chain antibody delivery via AAV1 reduces cerebral Abeta load in an Alzheimer's disease mouse model. J. Mol. Neurosci. 49, 277–288. doi: 10.1007/s12031-012-9877-3
Yong, L. M., Liu, L., Ding, T., Yang, G., Su, H. B., Wang, J. B., et al. (2021). Evidence of effect of aerobic exercise on cognitive intervention in older adults with mild cognitive impairment. Front. Psychiatry. 12:713671. doi: 10.3389/fpsyt.2021.713671
Zhang, L. L., Li, B., Yang, J. J., Wang, F. L., Tang, Q. Y., and Wang, S. H. (2020). Meta-analysis: resistance training improves cognition in mild cognitive impairment. Int. J. Sports Med. 41, 815–823. doi: 10.1055/a-1186-1272
Keywords: skeletal muscle, sarcopenia, cognitive impairment, Alzheimer's disease, aging
Citation: Han X, Ashraf M, Tipparaju SM and Xuan W (2023) Muscle–Brain crosstalk in cognitive impairment. Front. Aging Neurosci. 15:1221653. doi: 10.3389/fnagi.2023.1221653
Received: 12 May 2023; Accepted: 07 July 2023;
Published: 27 July 2023.
Edited by:
Marco Filardi, University of Bari Aldo Moro, ItalyReviewed by:
Csaba Jozsef Nyakas, Semmelweis University, HungaryCopyright © 2023 Han, Ashraf, Tipparaju and Xuan. This is an open-access article distributed under the terms of the Creative Commons Attribution License (CC BY). The use, distribution or reproduction in other forums is permitted, provided the original author(s) and the copyright owner(s) are credited and that the original publication in this journal is cited, in accordance with accepted academic practice. No use, distribution or reproduction is permitted which does not comply with these terms.
*Correspondence: Wanling Xuan, d3h1YW5AdXNmLmVkdQ==
Disclaimer: All claims expressed in this article are solely those of the authors and do not necessarily represent those of their affiliated organizations, or those of the publisher, the editors and the reviewers. Any product that may be evaluated in this article or claim that may be made by its manufacturer is not guaranteed or endorsed by the publisher.
Research integrity at Frontiers
Learn more about the work of our research integrity team to safeguard the quality of each article we publish.