- 1Henan Joint International Research Laboratory of Neurorestoratology for Senile Dementia, Henan Key Laboratory of Neurorestoratology, Neurology, First Affiliated Hospital of Xinxiang Medical University, Xinxiang, China
- 2Imaging Department, First Affiliated Hospital of Xinxiang Medical University, Xinxiang, China
- 3Ann Romney Center for Neurologic Diseases, Brigham and Women’s Hospital and Harvard Medical School, Boston, MA, United States
- 4Sino-UK Joint Laboratory of Brain Function and Injury of Henan Province, Department of Physiology and Neurobiology, Xinxiang Medical University, Xinxiang, China
Objective: The study investigated the correlation and predictive value between the severity of cerebral microbleeds (CMBs) and the level of serum High Mobility Group Protein B1 (HMGB1) and the occurrence of cognitive impairment in patients with cerebral small vessel disease (CSVD).
Methods: A total of 139 patients with CSVD admitted to the Department of Neurology of the First Affiliated Hospital of Xinxiang Medical University from December 2020 to December 2022 were selected as study subjects. The Montreal Cognitive Assessment (MoCA) scale was used to assess the cognitive function and was divided into the cognitive impairment group and the cognitive normal group. Magnetic Resonance Imaging (MRI) and Susceptibility Weighted Imaging (SWI) were used to screen and assess the severity of CMBs. Serum HMGB1 levels of CSVD patients were measured by enzyme linked immunosorbent assay (ELISA). Multivariable logistic regression analysis was used to explore risk factors for cognitive impairment and CMBs. Pearson correlation analysis was used to investigate the correlation between HMGB1 and cognitive function. Receiver Operating Characteristics (ROC) curves were used to assess the predictive value of HMGB1 for the occurrence of cognitive impairment in patients with CMBs.
Results: High Mobility Group Protein B1, uric acid (UA), glycosylated hemoglobin (HbA1c), CMBs, lacunar cerebral infarction (LI), years of education, and history of hypertension were risk factors for cognitive impairment (P < 0.05); HMGB1 was significantly and negatively associated with total MoCA score, visuospatial/executive ability, and delayed recall ability (P < 0.05). HMGB1 was significantly and positively correlated with the number of CMBs (P < 0.05). The area under the ROC curve for HMGB1 predicting cognitive impairment in patients with CMBs was 0.807 (P < 0.001).
Conclusion: Serum HMGB1 levels are associated with the development of cognitive impairment in CSVD patients, and serum HMGB1 levels have a high predictive value for the development of cognitive impairment in CSVD patients with combined CMBs, which can be used for early clinical identification and intervention of vascular cognitive impairment.
1. Introduction
Cerebral small vessel disease refers to a series of clinical, imaging and pathological syndromes caused by various etiologies affecting small arteries and their distal branches, micro-arteries, capillaries, micro-venules and small veins in the brain. CMBs are a type of brain parenchymal damage caused by injury to the micro-vessels of the brain, whose main pathological changes are the deposition of iron-containing hemoglobin at the lesion as well as lipid hyalinosis of the micro-arteries and amyloid deposition in the vessel wall (Ikram et al., 2022). CMBs have been found to be widely present in patients with cognitive impairment due to cerebrovascular disease and neurodegenerative disorders, and there is a correlation between the number of CMBs and their location in the brain and cognitive function. CMBs play an important role in the total load of CSVD imaging markers, are a key factor in the development of cognitive impairment in CSVD patients, and may be involved in the onset and development of cognitive impairment (Wu et al., 2014).
High Mobility Group Protein B1 is an inflammatory factor and damage signaling molecule that affects the structural and functional changes of the blood-brain barrier (BBB) and has a role in regulating innate immunity and inducing the release of other inflammatory factors (Xue et al., 2021). In the presence of central nervous system damage caused by inflammation, neurodegenerative lesions, and vascular damage, HMGB1 is rapidly released and inhibits synaptic plasticity by modulating the inflammatory response, exacerbating the pathological damage to damaged tissues (Ikram et al., 2022), ultimately leading to learning memory decline and cognitive dysfunction.
The objectives of this study were to observe the risk factors for the development of cognitive impairment and CMBs, to investigate the level of HMGBl in patients with CMBs and its correlation with cognitive impairment, to assess the predictive value of HMGB1 for the development of cognitive impairment in patients with CMBs, and to provide valid evidence for the early identification of vascular cognitive impairment due to CSVD.
2. Object
A total of 139 patients with CSVD who were hospitalized in the Department of Neurology of the First Affiliated Hospital of Xinxiang Medical University from December 2020 to December 2022 were selected for the study, and the patients were divided into cognitive impairment group (n = 72) and cognitive normal group (n = 67) according to the cognitive function evaluation results. 61 patients with CMBs were screened according to the results of MRI and SWI tests.
3. Methodology
3.1. Research subjects
Inclusion criteria:
(1) Age 40∼85 years;
(2) Patients with confirmed diagnosis of CSVD by cranial MRI findings (Shaaban et al., 2019) and must meet the diagnostic criteria for CMBs;
(3) Those who have good cranial MRI and SWI data and laboratory data;
(4) Agree to participate in this study and sign the informed consent form.
Exclusion Criteria:
(1) Atherosclerotic ischemic stroke or cardiogenic embolism in large arteries;
(2) Those with severe organic lesions such as malignant tumors or severe heart, lung, liver and kidney diseases and genetic metabolic disorders.
(3) Those who have mental illness and are on long-term antipsychotic medication.
(4) Patients who refused to cooperate with the study or were unable to sign the informed consent form.
The study was approved by the Ethics Committee of the First Affiliated Hospital of Xinxiang Medical University (approval number: EC-022-078) and registered with the Chinese Clinical Trials Registry (ChiCTR2200060083; 18 May 2022). All study procedures were performed in accordance with the latest version of the Declaration of Helsinki, and all participants signed a written informed consent form.
3.2. Clinical data collection
Clinical data were collected from both groups, including gender, age, years of education, medical history (cerebral infarction, cerebral hemorrhage, hypertension, diabetes, heart disease), personal history (smoking, drinking), and peripheral blood biochemical parameters, namely, total cholesterol (TC), triglyceride (TG), high density lipoprotein cholesterol (HDL-C), low density lipoprotein cholesterol (LDL-C), fasting blood glucose (FBG), glycated hemoglobin (HbAlc), homocysteine (Hcy), urea (UREA), creatinine (Cr), uric acid (UA), acrylic aminotransferase (ALT), and aspartate transaminases (AST) levels.
3.3. Neuropsychological assessment
The MoCA scale was applied to the patient’s cognitive function by a physician who was unaware of the imaging findings. MoCA scale (Carlew et al., 2021) contains 7 cognitive domains: visuospatial and executive, naming, attention, language, abstraction, delayed recall and orientation, and is usually completed within 10 min. A MoCA score of < 26 was considered to be cognitive impairment.
3.4. Imaging
In this study, a 3.0T magnetic resonance scanner manufactured by GE, USA, was used for imaging the study subjects. All the patients in this study underwent conventional MRI (T1WI, T2WI, FLAIR, and DWI) and SWI of the skull, and all the imaging results were hidden from the patients’ basic information (The purpose of this is to avoid errors due to subjectivity and to ensure that the imaging results of each subject are evaluated without discrimination) and interpreted by two imaging physicians with more than 5 years of experience to determine the presence of CSVD imaging markers: CMBs, white matter hyperintensity (WMH), LI, enlarged perivascular space (EPVS). T1WI: repetition time (TR) = 450 ms, echo time (TE) = 14 ms; ② fast spin echo T2WI: TR 4 800 ms, TE 102 ms; ③ FLAIR: TR 8 600 ms, TE 102 ms; ④ DWI: TR 3,000 ms, TE 65 ms; ⑤ SWI: TR 3,000 ms, TE 65 ms; ⑤ SWI: TR 55 ms, TE 45 ms. The CMBs were graded according to the number of CMBs on the SWI of the head, and CMBs were grouped into 3 grades: 1∼2 for Grade 1, 3∼10 for Grade 2, >10 for Grade 3 (Lee et al., 2002). The locations of CMBs were classified into lobes, basal ganglia, thalamus, hippocampus, brainstem, cerebellum and mixed sites according to the microbleed anatomical rating scale (MARS) (Gregoire et al., 2009).
3.5. Detection of serum HMGB1 level
A total of 4 ml of early morning elbow venous blood was drawn from the enrolled patients in a non-anticoagulated blood collection tube, left at room temperature for 10 min, then centrifuged for 10 min using a centrifuge (3,000 r/min) to separate the upper layer of serum, and the serum HMGB1 level was measured by ELISA according to the kit instructions. In our study, we diluted the serum 10-fold, applied the HMGB1 kit for detection, and then expanded the data 10-fold to obtain the final results (Wuhan Fine Biotech Co., Ltd.).
3.6. Statistical treatment
Data were analyzed using SPSS 26.0 and GraphPad Prism 8.0. The measurement data that conformed to normal distribution were expressed as (x ± s), and two independent samples t-test was used for comparison between groups; the measurement data that did not conform to normal distribution were expressed as M (P25, P75), and Mann–Whitney U test was used for comparison between groups; the count data were expressed as relative numbers, and χ2 test was used for comparison between groups. Multivariable logistic regression analysis was performed to investigate the factors affecting cognitive impairment in patients with CMBs; Pearson correlation test was used to analyze the correlation between HMGB1 and different cognitive domains and the number of CMBs. ROC curves were used to evaluate the predictive value of HMGB1 on the occurrence of cognitive impairment in patients with CMBs. The differences were considered statistically significant at P < 0.05.
4. Results
4.1. Comparison of clinical data, biochemical data, and imaging markers between two groups of patients
Patients with cognitive impairment had significantly higher proportions of female, history of hypertension, occurrence of CMBs, number of CMBs >10, Lobes CMBs, and LI than those in the group without cognitive impairment (P < 0.05). Patients with cognitive impairment had significantly higher levels of HMGB1, UA, and HbAlc than the group without cognitive impairment (P < 0.05). The years of education of patients in the cognitive impairment group were significantly lower than those in the no cognitive impairment group (P < 0.05) (Tables 1–3).
4.2. Multivariable logistic regression analysis to investigate risk factors affecting cognitive function
In addition to the statistically significant indicators in Tables 1–3, including gender, years of education, history of hypertension, and levels of HMGB1, UA, and HbAlc, as well as the presence of CMBs and LI, the effects of age, history of cerebral infarction, history of cerebral hemorrhage, and other imaging markers of CSVD: WMH and EVPS, on cognitive function should also be considered. Therefore, in this study, Logistic regression analysis was performed with the presence or absence of cognitive impairment as the dependent variable and the above-mentioned indicators that may have an impact on cognitive function as independent variables. The results showed that HMGB1, UA, HbAlc, years of education and history of hypertension were strongly associated with the occurrence of cognitive impairment in patients with CSVD (P < 0.05) (Table 4).
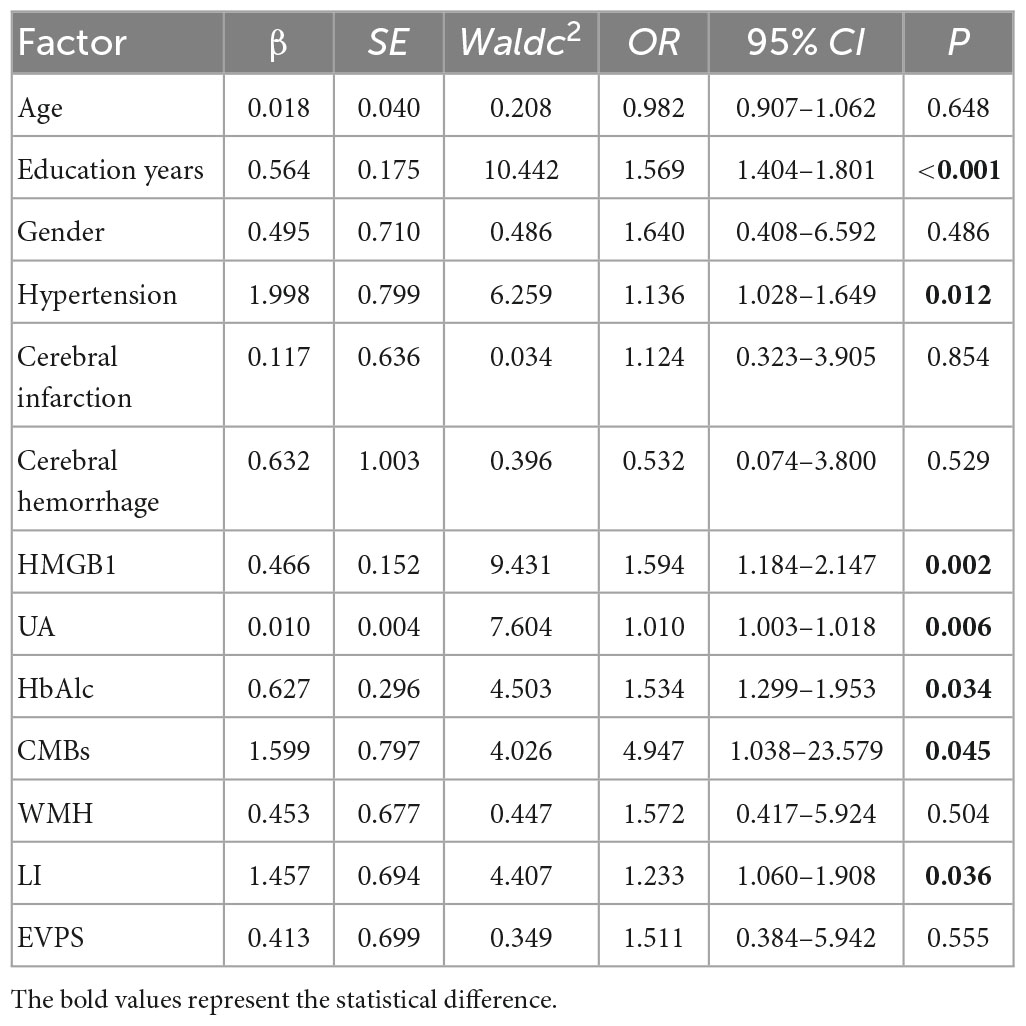
Table 4. Multivariable logistic regression analysis to explore the risk factors for cognitive function.
4.3. Comparison of cognitive abilities and correlation analysis between HMGB1 and various cognitive domains in the two groups
Patients with cognitive impairment had significantly lower MoCA total scores, visuospatial and executive abilities, and delayed recall abilities than those in the non-cognitively impaired group (P < 0.05). Pearson correlation analysis was used to explore the correlation between HMGB1 and each cognitive domain, and the results showed that HMGB1 levels were significantly negatively correlated with MoCA total score, visuospatial and executive abilities, and delayed recall abilities (P < 0.05) (Tables 5, 6).
4.4. Univariate and multivariate Logistic regression analysis to explore risk factors for CMBs
The presence or absence of CMBs was used as the dependent variable, and age, gender, years of education, CSVD imaging markers, patients’ past history, and biochemical data were used as independent variables in univariate Logistic regression analysis, which showed that age, history of cerebral hemorrhage, history of heart disease, history of hypertension, history of smoking, history of drinking, HMGB1, UA, ALT, and AST were risk factors for CMBs (P < 0.05). Multivariate Logistic regression analysis was performed again with the above statistically significant indicators as independent variables, and the results showed that HMGB1, ALT, history of hypertension, and history of heart disease were strongly associated with the presence of CMBs (P < 0.05) (Table 7).
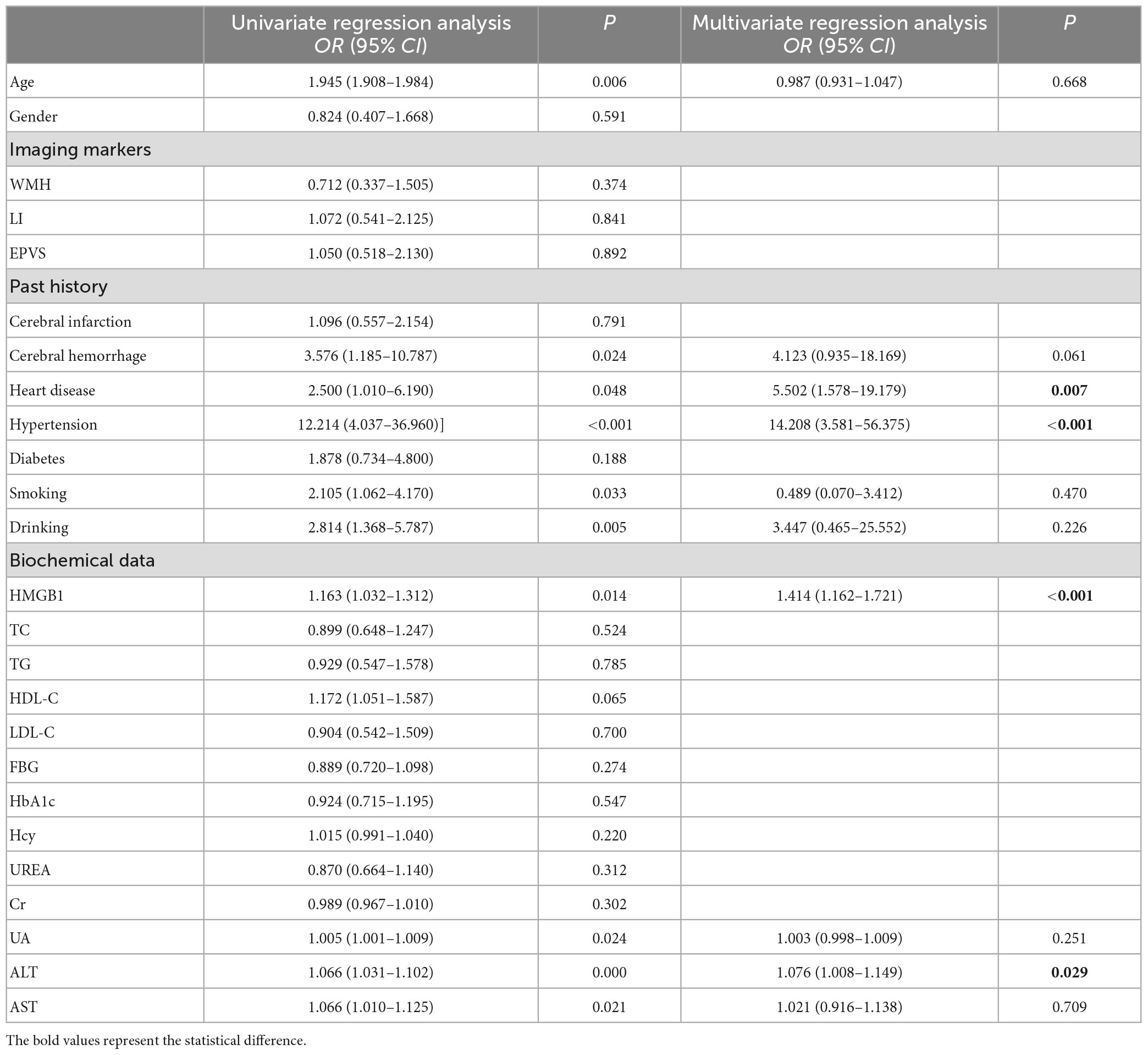
Table 7. Univariate and multivariate logistic regression analysis to explore the risk factors for CMBs.
4.5. Correlation studies among HMGB1 levels, severity of CMBs, and cognitive impairment
Pearson correlation analysis was used to explore the correlation between HMGB1 and CMBs, and the results showed a significant positive correlation between HMGB1 levels and the number of CMBs (r = 0.530, P < 0.05). ROC curve analysis of the predictive value of HMGB1 showed that HMGBI had a high predictive value for the development of cognitive impairment in patients with CMBs, and the area under the ROC curve was 0.807 (95% CI:0.683- 0.931, P < 0.001). The optimal threshold value was 5.04 μg/L, with a sensitivity of 86.8% and specificity of 69.6% (Figures 1, 2).
5. Discussion and conclusion
Cerebral microbleeds are focal deposits of blood degradation products contained in intracranial macrophages around blood vessels, and together with WMH, LI, and EPVS, they are known as imaging markers of CSVD (Peng et al., 2019). There is a correlation between the occurrence of CMBs and various physiological and pathological states. The most common symptom currently considered to be affected by CMBs is altered cognitive function (Meng et al., 2019). The consequences of their effects may depend on a variety of factors, including the number, size, and location of CMBs and the co-occurrence of other disorders. Research evidence suggests that greater numbers of CMBs are associated with more severe cognitive impairment and that the number of CMBs is an independent predictor of multiple cognitive domains and dementia severity (Miwa et al., 2014). A cross-sectional study has shown that CMBs are associated with impaired whole-brain cognition, reduced attention and processing speed, and impaired executive function (Yates et al., 2014). There is also an anatomical relationship between the distribution of CMBs and the degree of cognitive impairment and the cognitive domains impaired. A Meta-analysis showed that CMBs in the lobes, basal ganglia, and thalamus were associated with significant cognitive decline (Wu et al., 2014), and it has also been found that temporal CMBs are associated with memory and attention impairment, while frontal CMBs are associated with memory impairment, concept transfer, psychomotor speed and attention (van Norden et al., 2011). To date, however, the mechanisms by which CMBs contribute to cognitive impairment remain elusive. Initial studies suggested that CMBs may directly damage cortical, subcortical, and deep brain tissue through local deposition of iron-containing heme, leading to impairment of their connecting fiber tracts and neurotransmission pathways. With the development of neuroimmune science, many scholars have proposed that CMBs may lead to dysfunction of surrounding tissues, causing a sustained neuroinflammatory response with inflammatory mediators mediating oxidative stress damaging neurovascular units and causing local blood-brain barrier disruption (Eisele et al., 2016), microglia and perivascular macrophage activation, leading to cognitive impairment (Brundel et al., 2012; Del Brutto et al., 2016). The present study showed that CMBs were a risk factor for cognitive impairment, and the number of CMBs >10 and the location of CMBs in the lobes of the brain were significantly higher in the cognitively impaired group than in the non-cognitively impaired group, which is generally consistent with the results of previous studies. The present study also found that LI, history of hypertension, and low education level were also risk factors for cognitive impairment. LI caused neurological deficits and cognitive impairment by affecting cerebral blood supply. The number and volume of infarcts are important factors affecting cognitive performance; long-term hypertension causes impaired circulation in the deep white matter of the brain and changes in the BBB, leading to demyelination and affecting cognitive function; in patients with low education level, they are less stimulated by cultural knowledge, causing early decline of sensitive neurons, while high education level can resist age-related neuropsychological changes and slow down cognitive decline (Ding et al., 2019).
High Mobility Group Protein B1 is a highly conserved chromosomal binding protein, mainly found in the nucleus of eukaryotic cells, involved in gene replication, transcription and repair processes, and can also be actively secreted or passively released extracellularly to participate in the inflammatory response as an injury-associated molecule (Hisaoka-Nakashima et al., 2022). When monocytes-macrophages and astrocytes are exposed to exogenous pathogen-associated molecular patterns such as bacteria and viruses or endogenous inflammatory transmitters such as interleukin (IL)-1 and tumor necrosis factor-α (TNF-α), HMGB1 is actively secreted extracellularly. HMGB1 can bind to specific receptors and then promote the production of inflammatory factors through autocrine or paracrine forms to induce inflammatory responses; at the same time, HMGB1 itself has pro-inflammatory activity, which can directly cause BBB disruption and enhance inflammatory response (Manivannan et al., 2021). In addition to its pro-inflammatory activity, extracellular HMGBl also acts as a chemokine and growth factor, providing nutrition to cells, promoting neuroprotrusion and cell migration, and regulating the balance between autophagy and apoptosis. It has been shown that HMGBl is highly correlated with the development of cognitive impairment caused by acute brain injury, Parkinson’s, depression, epilepsy, and type 2 diabetes (Chu et al., 2013; Rolstad et al., 2015; Sun et al., 2015; Vidyanti et al., 2020; Piatkowska-Chmiel et al., 2021). However, the effect of HMGB1 on cognitive impairment in CSVD patients has rarely been investigated. In the present study, HMGB1 was found to be a risk factor for cognitive impairment in CSVD patients, and HMGB1 was significantly and negatively correlated with total MoCA score, visuospatial/executive abilities, and delayed recall abilities, the reason may be that the release of HMGB1 mediates the activation of microglia, and the oxidative stress and nitrative stress it causes aggravates neurovascular unit damage and BBB disruption, interfering with the electrophysiological activity of neurons and eventually causing degenerative necrosis of neurons, leading to cognitive impairment.
Previous findings have shown that abnormal UA and HbA1c also contribute to cognitive impairment. It is believed that UA is the end product of purine metabolism and that high UA levels affect cognitive function by promoting endothelial inflammatory responses and oxidative stress, leading to endothelial dysfunction and white matter damage (Ryu et al., 2013; Murthy et al., 2020); and in the hyperglycemic state, oxygen radicals are overexpressed, neurons experience impaired energy metabolism, and sustained anaerobic metabolism produces large amounts of lactic acid, causing damage to blood vessels and neurons (Bellary et al., 2021), which ultimately leads to cognitive impairment. The present study reached a consistent conclusion.
This study also identified HMGB1 as a risk factor for CMBs, and HMGB1 levels were significantly and positively correlated with the number of CMBs. The release of large amounts of HMGB1 may disrupt the tightly linked structure of the BBB, and the interaction between activated brain macrophages and astrocytes may further amplify the inflammatory response by producing chemokines that lead to BBB destruction by affecting endothelial cells or promoting the migration of monocytes (Dohgu et al., 2019). Several studies have shown that BBB disruption is involved in the pathological process of CMBs (Wang et al., 2021), triggering the formation of CMBs, and that the inflammatory response induced by CMBs disrupts endothelial cell function, exacerbating BBB disruption and further increasing the permeability of the BBB. The increase in the number of CMBs, the increase in the expression level of HMGB1, and the increase in BBB disruption suggest that neuroinflammation has a cumulative effect on the disruption of CMBs and the BBB. In this study, ROC curve analysis of HMGB1 expression levels in patients with CMBs showed that HMGBI has a high predictive value for the development of cognitive impairment in patients with CMBs, implying that HMGB1 may produce neuroinflammatory effects through sustained release, destroying neurovascular units and the BBB, which results in the continuous generation of CMBs in various location of the brain and ultimately leads to cognitive impairment.
In summary, this study concluded that there is a close association between HMGB1, CMBs, BBB and cognitive impairment, and any change in any of these indicators may have a significant impact on the course of the disease. HMGB1 is involved in the pathogenesis of many inflammatory and autoimmune diseases as an important extracellular damage molecule. Therefore, HMGBl may serve as a new therapeutic target for patients with CMBs, providing a novel direction for the prevention and treatment of cognitive impairment, which has important implications for the early detection, treatment efficacy and prognostic assessment of patients with cognitive impairment.
6. Limitations and prospects
The present study is only a single-center, cross-sectional study and has a relatively small sample size. It also lacks theoretical results from animal experiments to provide more powerful support. In the future, we aim to conduct a multicenter, large sample size investigative study, as well as produce animal models of CMBs to explore the specific pathway mechanisms by which HMGB1 exerts its neuroinflammatory effects, in order to provide clinicians with help in early intervention and control of the occurrence and development of neuroinflammation.
Data availability statement
The raw data supporting the conclusions of this article will be made available by the authors, without undue reservation.
Ethics statement
The studies involving human participants were reviewed and approved by the Ethics Committee of the First Affiliated Hospital of Xinxiang Medical University. The patients/participants provided their written informed consent to participate in this study.
Author contributions
MW and JZ: conceptualization and formal analysis. MW, JZ, and SL: methodology. JL and FW: software. JZ: validation, resources, and writing—review and editing. MW and FW: investigation. MW: writing—original draft. QL and SJ: visualization. JZ and SJ: supervision. SL and CL: project administration. All authors have read and agreed to the published version of the manuscript.
Funding
This study was supported by the National Natural Science Foundation of China (81771517), Natural Science Foundation of Henan Province (182300410389); Joint Construction Project of Henan Medical Science and Technology Project (LHGJ20190437); Xinxiang Medical University Scientific Research Innovation Support Program Project (YJSCX202185Y); and Henan Province Science and Technology, Department of Science and Technology Tackling Program International Cooperation Project (232102521029).
Acknowledgments
We authors thank all the participants who devoted their free time to participate in this study.
Conflict of interest
The authors declare that the research was conducted in the absence of any commercial or financial relationships that could be construed as a potential conflict of interest.
Publisher’s note
All claims expressed in this article are solely those of the authors and do not necessarily represent those of their affiliated organizations, or those of the publisher, the editors and the reviewers. Any product that may be evaluated in this article, or claim that may be made by its manufacturer, is not guaranteed or endorsed by the publisher.
References
Bellary, S., Kyrou, I., Brown, J., and Bailey, C. (2021). Type 2 diabetes mellitus in older adults: Clinical considerations and management. Nat. Rev. Endocrinol. 17, 534–548.
Brundel, M., Heringa, S., de Bresser, J., Koek, H., Zwanenburg, J., Jaap Kappelle, L., et al. (2012). High prevalence of cerebral microbleeds at 7Tesla MRI in patients with early Alzheimer’s disease. J. Alzheimers Dis. 31, 259–263. doi: 10.3233/JAD-2012-120364
Carlew, A., Smith, E., Goette, W., Lippe, B., Lacritz, L., and Rossetti, H. (2021). Montreal cognitive assessment (MoCA) scores in medically compromised patients: A scoping review. Health Psychol. 40, 717–726. doi: 10.1037/hea0001138
Chu, Q., He, L., Zhang, W., Liu, C., Ai, Y., and Zhang, Q. (2013). Hydrogen sulfide attenuates surgical trauma-induced inflammatory response and cognitive deficits in mice. J. Surg. Res. 183, 330–336. doi: 10.1016/j.jss.2012.12.003
Del Brutto, O., Mera, R., Gillman, J., Ha, J., and Zambrano, M. (2016). Calcifications in the carotid siphon correlate with silent cerebral small vessel disease in community-dwelling older adults: A population-based study in rural Ecuador. Geriatr. Gerontol. Int. 16, 1063–1067. doi: 10.1111/ggi.12599
Ding, M., Xu, Y., Wang, Y., Li, P., Mao, Y., Yu, J., et al. (2019). Predictors of cognitive impairment after stroke: A prospective stroke cohort study. J. Alzheimers Dis. 71, 1139–1151.
Dohgu, S., Takata, F., Matsumoto, J., Kimura, I., Yamauchi, A., and Kataoka, Y. (2019). Monomeric α-synuclein induces blood-brain barrier dysfunction through activated brain pericytes releasing inflammatory mediators in vitro. Microvasc. Res. 124, 61–66. doi: 10.1016/j.mvr.2019.03.005
Eisele, P., Alonso, A., Griebe, M., Szabo, K., Hennerici, M., and Gass, A. (2016). Investigation of cerebral microbleeds in multiple sclerosis as a potential marker of blood-brain barrier dysfunction. Mult. Scler. Relat. Dis. 7, 61–64. doi: 10.1016/j.msard.2016.03.010
Gregoire, S., Chaudhary, U., Brown, M., Yousry, T., Kallis, C., Jäger, H., et al. (2009). The Microbleed Anatomical Rating Scale (MARS): Reliability of a tool to map brain microbleeds. Neurology 73, 1759–1766. doi: 10.1212/WNL.0b013e3181c34a7d
Hisaoka-Nakashima, K., Ohata, K., Yoshimoto, N., Tokuda, S., Yoshii, N., Nakamura, Y., et al. (2022). High-mobility group box 1-mediated hippocampal microglial activation induces cognitive impairment in mice with neuropathic pain. Exp. Neurol. 355:114146. doi: 10.1016/j.expneurol.2022.114146
Ikram, F., Arulsamy, A., Retinasamy, T., and Shaikh, M. (2022). The role of high mobility group box 1 (HMGB1) in neurodegeneration: A systematic review. Curr. Neuropharmacol. 20, 2221–2245. doi: 10.2174/1570159X20666220114153308
Lee, S., Bae, H., Yoon, B., Kim, H., Kim, D., and Roh, J. (2002). Low concentration of serum total cholesterol is associated with multifocal signal loss lesions on gradient-echo magnetic resonance imaging: Analysis of risk factors for multifocal signal loss lesions. Stroke 33, 2845–2849. doi: 10.1161/01.str.0000036092.23649.2e
Manivannan, S., Marei, O., Elalfy, O., and Zaben, M. (2021). Neurogenesis after traumatic brain injury - The complex role of HMGB1 and neuroinflammation. Neuropharmacology 183:108400. doi: 10.1016/j.neuropharm.2020.108400
Meng, L., Zhao, J., Liu, J., and Li, S. (2019). Cerebral small vessel disease and cognitive impairment. J. Neurorestoratol. 7, 184–195. doi: 10.26599/JNR.2019.9040023
Miwa, K., Tanaka, M., Okazaki, S., Yagita, Y., Sakaguchi, M., Mochizuki, H., et al. (2014). Multiple or mixed cerebral microbleeds and dementia in patients with vascular risk factors. Neurology 83, 646–653. doi: 10.1212/WNL.0000000000000692
Murthy, S., Diaz, I., Wu, X., Merkler, A., Iadecola, C., Safford, M., et al. (2020). Risk of arterial ischemic events after intracerebral hemorrhage. Stroke 51, 137–142. doi: 10.1161/STROKEAHA.119.026207
Peng, X., Zhao, J., Liu, J., and Li, S. (2019). Advances in biomarkers of cerebral small vessel disease. J. Neurorestoratol. 7, 171–183.
Piatkowska-Chmiel, I., Herbet, M., Gawronska-Grzywacz, M., Ostrowska-Lesko, M., and Dudka, J. (2021). The role of molecular and inflammatory indicators in the assessment of cognitive dysfunction in a mouse model of diabetes. Int. J. Mol. Sci. 22:3878. doi: 10.3390/ijms22083878
Rolstad, S., Jakobsson, J., Sellgren, C., Isgren, A., Ekman, C., Bjerke, M., et al. (2015). CSF neuroinflammatory biomarkers in bipolar disorder are associated with cognitive impairment. Eur. Neuropsychopharm. 25, 1091–1098.
Ryu, E., Kim, M., Shin, H., Jang, Y., Choi, H., Jo, I., et al. (2013). Uric acid-induced phenotypic transition of renal tubular cells as a novel mechanism of chronic kidney disease. Am. J. Physiol. Renal. 304, F471–F480. doi: 10.1152/ajprenal.00560.2012
Shaaban, C., Jorgensen, D., Gianaros, P., Mettenburg, J., and Rosano, C. (2019). Cerebrovascular disease: Neuroimaging of cerebral small vessel disease. Prog. Mol. Biol. Transl. 165, 225–255.
Sun, H., Wu, H., Yu, X., Zhang, G., Zhang, R., Zhan, S., et al. (2015). Angiotensin II and its receptor in activated microglia enhanced neuronal loss and cognitive impairment following pilocarpine-induced status epilepticus. Mol. Cell Neurosci. 65, 58–67. doi: 10.1016/j.mcn.2015.02.014
van Norden, A., van den Berg, H., de Laat, K., Gons, R., van Dijk, E., and de Leeuw, F. (2011). Frontal and temporal microbleeds are related to cognitive function: The Radboud University Nijmegen Diffusion Tensor and Magnetic Resonance Cohort (RUN DMC) study. Stroke 42, 3382–3386. doi: 10.1161/STROKEAHA.111.629634
Vidyanti, A., Hsieh, J., Lin, K., Fang, Y., Setyopranoto, I., and Hu, C. (2020). Role of HMGB1 in an animal model of vascular cognitive impairment induced by chronic cerebral hypoperfusion. Int. J. Mol. Sci. 21:2176. doi: 10.3390/ijms21062176
Wang, H., Zhang, C., Qiu, Y., Chen, A., Li, Y., and Hu, B. (2021). Dysfunction of the blood-brain barrier in cerebral microbleeds: From bedside to bench. Aging Dis. 12, 1898–1919. doi: 10.14336/AD.2021.0514
Wu, R., Feng, C., Zhao, Y., Jin, A., Fang, M., and Liu, X. (2014). A meta-analysis of association between cerebral microbleeds and cognitive impairment. Med. Sci. Monit. 20, 2189–2198.
Xue, J., Suarez, J., Minaai, M., Li, S., Gaudino, G., Pass, H., et al. (2021). HMGB1 as a therapeutic target in disease. J. Cell Physiol. 236, 3406–3419.
Keywords: cerebral small vessel disease, cerebral microbleeds, HMGB1, cognitive, blood-brain barrier
Citation: Wang M, Liu J, Wang F, Li Q, Zhang J, Ji S, Li S, Lu C and Zhao J (2023) The correlation between the severity of cerebral microbleeds and serum HMGB1 levels and cognitive impairment in patients with cerebral small vessel disease. Front. Aging Neurosci. 15:1221548. doi: 10.3389/fnagi.2023.1221548
Received: 12 May 2023; Accepted: 08 June 2023;
Published: 22 June 2023.
Edited by:
Yu-Min Kuo, National Cheng Kung University, TaiwanReviewed by:
Beimeng Yang, Roche Innovation Center China, ChinaQi Li, The First Affiliated Hospital of Chongqing Medical University, China
Copyright © 2023 Wang, Liu, Wang, Li, Zhang, Ji, Li, Lu and Zhao. This is an open-access article distributed under the terms of the Creative Commons Attribution License (CC BY). The use, distribution or reproduction in other forums is permitted, provided the original author(s) and the copyright owner(s) are credited and that the original publication in this journal is cited, in accordance with accepted academic practice. No use, distribution or reproduction is permitted which does not comply with these terms.
*Correspondence: Jianhua Zhao, MDUxMDkyQHh4bXUuZWR1LmNu