- 1Department of Neurology, Yonsei University College of Medicine, Seoul, Republic of Korea
- 2Nash Family Center for Advanced Circuit Therapeutics, Icahn School of Medicine at Mount Sinai, New York, NY, United States
- 3Department of Neurology, Chung-Ang University College of Medicine and Graduate School of Medicine, Gwangmyeong Hospital, Gwangmyeong, Republic of Korea
- 4Severance Biomedical Science Institute, Yonsei University College of Medicine, Seoul, Republic of Korea
Introduction: Concomitant amyloid pathology is not uncommon and contributes to the clinical characteristics of Lewy body disease (LBD). We investigated the effect of amyloid on striatal 18F-FP-CIT uptake patterns in LBD, including Parkinson's disease (PD) and dementia with Lewy bodies.
Methods: We enrolled 125 patients with LBD who underwent 18F-florbetaben positron emission tomography (PET) and 18F-FP-CIT PET. Patients were divided into amyloid-positive and amyloid-negative groups. We investigated the effect of amyloid on striatal dopamine transporter (DAT) availability, depending on the type of LBD, using general linear models with interaction analysis after controlling for age, sex, education, deep white matter hyperintensity (WMH), periventricular WMH, and cognitive status.
Results: There was a significant interaction effect between the disease group and the presence of amyloid on DAT availability in the anterior putamen, posterior putamen, caudate, and ventral striatum. In the presence of amyloid, only the PD group exhibited decreased DAT availability in the anterior and posterior putamen. In both groups, the presence of amyloid was not associated with DAT availability in the caudate and ventral striatum. The presence of amyloid was not directly related to the worse parkinsonian motor symptoms in both groups. However, there was a significant indirect effect of amyloid on parkinsonian motor symptoms, which was mediated by anterior and posterior putaminal DAT availability in the PD group alone.
Discussion: This study demonstrates different amyloid-dependent or amyloid-independent 18F-FP-CIT PET patterns in patients with LBD, suggesting distinctive interactions between α-synuclein and amyloid pathology based on the type of LBD.
1. Introduction
Lewy body disease (LBD) encompasses Parkinson's disease (PD), PD dementia (PDD), and dementia with Lewy bodies (DLBs). The LBDs share pathological hallmarks, Lewy bodies, and similar clinical characteristics, including rapid eye movement sleep behavior disorder (RBD), parkinsonism, fluctuating cognition, and visual hallucinations. The only distinction between PDD and DLB is the time relationship between the onset of dementia and motor symptoms (McKeith et al., 2017); however, cognitive impairment or prognosis and caregiver burden are more severe in DLB than in PDD (Walker et al., 2007; Yoon et al., 2014; Jellinger, 2018).
Along with Lewy bodies, concomitant Alzheimer's disease (AD) pathology is not uncommon and contributes to the clinical characteristics of LBD. Amyloid deposition was related to worse attention-executive and language functions in LBD patients (Biundo et al., 2021). In patients with DLB, a higher amyloid burden is associated with the diagnosis of dementia at a younger age, greater cognitive decline (Sarro et al., 2016), lower cognitive performance (Ferreira et al., 2020), decreased dopamine transporter (DAT) activity (Yoo et al., 2020), and more extensive gray matter atrophy (Sarro et al., 2016; Lee et al., 2018b). Similarly, compared to a lower amyloid burden, a higher amyloid burden is related to reduced cognitive performance (McMillan and Wolk, 2016), faster cognitive decline (Gomperts et al., 2013; Yarnall et al., 2014), shorter time-to-dementia onset (Compta et al., 2011), and increased postural instability and gait difficulty (Muller et al., 2013) in patients with PD.
Pathological and imaging studies have provided ample evidence that patients with DLB have a higher amyloid burden than those with PD (Petrou et al., 2012; Hepp et al., 2016). Additionally, the type and distribution of amyloid in DLB differ from those in PD. For example, the neuritic plaque score is higher in DLB than in PDD, and amyloid is more frequently observed in the entorhinal cortex, amygdala, and putamen in DLB than in PDD (Hepp et al., 2016). Another study showed that patients with DLB had frequent amyloid deposition in the midbrain, while patients with PD had less or scent amyloid deposition in the midbrain (Fujishiro et al., 2010; Sierra et al., 2016; Jellinger, 2023). Moreover, recent studies have reported that the different clinical and pathological features of LBD may be ascribed to the underlying differences in α-synuclein strains (Candelise et al., 2019; Van der Perren et al., 2020), which may also lead to different interactions with other proteins (Sengupta et al., 2020). One autopsy study showed different distribution patterns of α-synuclein pathology in the LBD spectrum depending on the concomitant AD (Toledo et al., 2016). Although the biological effects of mixed pathologies or interactions between α-synuclein and other toxic proteins on neuronal degeneration have been suggested (Sengupta et al., 2020), little is known about how different α-synuclein strains would interact with other proteins.
How does concomitant amyloid accumulation affect the nigrostriatal dopaminergic system in the LBD spectrum and their relationship with parkinsonian motor symptoms is elusive? In this study, we hypothesized that amyloid pathology may interact in a different manner with the nigrostriatal dopaminergic system in DLB and PD; therefore, the patterns of nigrostriatal DAT availability would differ. To test this hypothesis, we performed an interaction analysis in patients with DLB and PD who had undergone 18F-florbetaben positron emission tomography (18F-FBB PET) to examine specific 18F-N-(3-fluoropropyl)-2β-carbon ethoxy-3β-(4-iodophenyl) nortropane (18F-FP-CIT) PET uptake, which interacts with amyloid depending on the type of LBD.
2. Materials and methods
2.1. Participants
We reviewed the medical records of 125 patients with PD or DLB who visited the movement and dementia clinics of Yonsei University Severance Hospital between July 2015 and December 2020. PD was diagnosed according to the United Kingdom Parkinson's Disease Brain Bank diagnostic criteria. PD with mild cognitive impairment (MCI) and PDD were diagnosed according to the level II PD-MCI criteria (Litvan et al., 2011) and the clinical criteria of probable PDD, respectively (Dubois et al., 2007). DLB was diagnosed using the 2017 revised criteria (McKeith et al., 2017); all patients satisfied the probable DLB criteria. All patients with MCI due to DLB met the probable DLB criteria, except for the presence of dementia. All patients in both groups underwent 18F-FP-CIT PET and were confirmed to have dopaminergic depletion. We excluded patients with severe white matter hyperintensities (WMHs), multiple lacunes in the basal ganglia, or hydrocephalus on magnetic resonance imaging, as well as patients with other neurologic, psychiatric, or metabolic illnesses.
All patients underwent a neurologic examination. The severity of parkinsonism was assessed according to the Unified Parkinson's Disease Rating Scale (UPDRS) Part III score in the off-state. The Korean version of the Mini-Mental State Examination (K-MMSE) and the clinical dementia rating scale sum of boxes (CDR-SOB) were used to evaluate general cognition and staging dementia severity, respectively. Clinical features of LBD, including RBD, visual hallucinations, and cognitive fluctuation, were evaluated using semi-structured questionnaires, which were administered by caregivers. All patients underwent 18F-FBB PET. This study was approved by the Yonsei University Severance Hospital Institutional Review Board (No. 4-2016-0210), and the need for informed consent was waived because of the retrospective design of the study.
2.2. Acquisition and processing of MR data
In total, 71 participants were scanned using a Philips 3.0 T MR scanner (Philips Achieva; Philips Medical Systems, Best, The Netherlands) with a SENSE head coil (SENSE factor = 2). T1-weighted magnetic resonance imaging (MRI) data were obtained using a 3D T1-TFE sequence with the following parameters: 224 × 224 axial acquisition matrix; 256 × 256 reconstructed matrix with 170 slices; voxel size, 0.859 × 0.859 × 1 mm; field of view, 220 mm; echo time, 4.6 ms; repetition time, 9.8 ms; and flip angle, 8°. With the baseline image without weighting, diffusion-weighted MRI data were acquired from 32 diffusion sampling directions using a single-shot echo-planar acquisition with the following parameters: 128 × 128 acquisition matrix with 70 slices; voxel size, 1.75 × 1.75 × 2 mm; field of view, 220 mm; b-factor, 600 s/mm2; echo time, 70 ms; repetition time, 7.663 sec; and flip angle, 90°. Conventional two-dimensional fluid-attenuated inversion recovery images were obtained to evaluate white matter hyperintensities.
2.3. Measurement of regional white matter hyperintensities
A visual rating scale for WMH was modified from the Fazekas scale. Periventricular WMH (PWMH) areas were classified as P1 (cap and band < 5 mm), P2 (5 mm ≤ cap or band < 10 mm), and P3 (10 mm ≤ cap or band); deep WMH (DWMH) areas were classified as D1 (maximum diameter of deep white matter lesion < 10 mm), D2 (10 mm ≤ lesion < 25 mm), and D3 (≥ 25 mm).
2.4. Acquisition and interpretation of 18F-FBB PET scans
Detailed methods for 18F-FBB PET acquisition have been described in a previous study (Lee et al., 2018b). Quantitative PET image analysis was performed using a PET template (Kim and Jk, 2020). 18F-FBB PET images were then co-registered to the PET template using non-linear registration in advanced normalization tools to obtain the affine transformation matrix and deformation field. The inverse transformation was performed to warp the parcellation mask from the standard space of the PET template to the native space of each PET image. The participant-specific mask image comprised 68 cortical and 10 subcortical parcellated regions of the Desikan–Killiany Atlas (Klein and Tourville, 2012). Standardized uptake value ratios (SUVRs) were calculated according to the ADNI Florbetaben PET processing performed by UC Berkley (Sabri et al., 2015). Subregional SUVRs were calculated for the four predefined cortical regions of interest (ROIs) (frontal, lateral temporal, lateral parietal, and anterior/posterior cingulate) by overlaying the participant-specific composite masks. The whole cerebellum was used as the reference region. In addition, global SUVRs were established for each participant by volume-weighted averaging across four cortical regions and dividing this by the reference region. Patients who had composite Aβ retention above the threshold for Aβ positivity (global SUVRs > 1.20) were regarded as β-amyloid-positive (Martersteck, 2020) (see also https://adni.bitbucket.io/reference/docs/UCBERKELEYFBB/UCBerkeley_FBB_Methods_04.11.19.pdf).
2.5. Acquisition and quantitative analyses of 18F-FP-CIT PET images
The 18F-FP-CIT PET scans were acquired using a GE PET-CT DSTe scanner (GE Discovery STE; GE Healthcare; Milwaukee, WI, USA), which obtains images with a three-dimensional resolution of 2.3 mm full width at half maximum. All subjects were instructed to fast for ≥6 h before the PET scan, and 5mCi (185 MBq) of 18F-FP-CIT was intravenously administered to the subjects. After 90-min post-injection, images were acquired for 20 min in the three-dimensional mode.
Image processing was conducted using the SPM12 (Statistical Parametric Mapping 12, Wellcome Department of Imaging Neuroscience, Institute of Neurology, London, England) software package. For each patient, the reconstructed PET image was spatially normalized to the study-specific 18FP-CIT PET template created by a subset of two groups, including 44 patients with DLB and 27 patients with PD. To create the study-specific 18FP-CIT PET template, individual PET images were co-registered with the T1-weighted anatomical image and then normalized to the MNI152 template using the normalization parameters defined from the T1-weighted anatomical image. Then, the intensity was normalized by the uptake value of the occipital volume-of-interests (VOIs). The occipital VOI, including the calcarine fissure and surrounding cortex (V1), was selected from the AAL atlas. Finally, the processed 71 patients' PET images were averaged to generate a study-specific 18FP-CIT PET template. The striatum was divided into the caudate, anterior putamen, posterior putamen, and ventral striatum. The specific-to-non-specific binding ratio or DAT availability of each striatal subregion was defined as follows: (mean standardized uptake value of the striatal subregion VOIs—mean standardized uptake value of the occipital VOI)/(mean standardized uptake value of the occipital VOI). In addition, we further investigated two characteristic DAT patterns, which are the anterior–posterior gradient and right-left asymmetry. The inter-subregional ratio (ISR) was calculated by dividing the DAT availability of the anterior putamen by that of the posterior putamen. The ISR represents the anterior–posterior gradient of DAT uptake within the striatum. The asymmetricity ratio (AR) was calculated by dividing the DAT availability of the less affected posterior putamen by that of the more affected posterior putamen.
2.6. Statistical analysis
Statistical analyses were performed using the Statistical Package for the Social Sciences version 26.0 (IBM Corp., Armonk, NY). The independent t-test and χ2 test were performed to compare demographics and clinical features across disease groups.
Initially, we evaluated the differences in subregional DAT availability, ISR, and AR between the PD and DLB groups using general linear models (GLMs) after controlling for age, sex, DWMH, PWMH, and cognitive status. Cognitive status was regarded as a binary variable; 0 for patients without dementia and 1 for patients with dementia. We used cognitive status as a covariate since there was a significant difference in the proportion of patients with dementia between the two disease groups. Next, we evaluated the interaction effects of the disease group and the presence of amyloid on subregional DAT availability, ISR, AR, and the UPDRS Part III score using GLM after controlling for age, sex, DWMH, PWMH, and cognitive status. If there was a significant interaction effect between the disease group and the presence of amyloid, we investigated the effect of the presence of amyloid on subregional DAT availability or UPDRS Part III score in the DLB and PD groups separately. If the interaction term was not significant, GLM analyses were performed for all participants, regardless of group. In addition, the disease group and the presence of amyloid were entered as predictors. We applied the false discovery rate (FDR) method to correct multiple comparisons and an interaction term with Q (FDR-corrected P-value) < 0.2 was considered to be significant (Selvin, 2004).
We further investigated whether amyloid affects parkinsonian motor symptoms through the nigra dopaminergic pathway. Mediation analyses were used to evaluate whether DAT availability of the anterior or posterior putamen mediates associations between amyloid and UPDRS Part III scores after controlling for age, sex, DWMH, and PWMH in each disease group. Since dopamine depletion in the putamen is a core pathological feature and best reflects disease severity in patients with PD (Fearnley and Lees, 1991), we chose the anterior putamen and posterior putamen for further analysis. We used the PROCESS macro in SPSS with 5,000 bootstrapped samples for mediation analysis. A confidence interval (CI) that does not contain zero indicates a significant mediation effect.
3. Results
3.1. Demographics and clinical characteristics
A total of 125 patients with LBD (82 patients with DLB and 43 patients with PD) were included in the study. Patient demographics are presented in Table 1. There were no significant differences in age, sex, education level, UPDRS Part III score, DWMH, and PWMH between the groups. The MMSE score was lower in the DLB group than in the PD group (P < 0.001). In addition, the CDR-SOB score and proportion of patients with dementia were higher in the DLB group than in the PD group (P < 0.001). Patients with DLB experienced fluctuations and hallucinations more frequently than those with PD (P < 0.001). The proportion of patients with RBD was similar between the groups. Amyloid positivity was higher in the DLB group than in the PD group (53.7 vs. 20.9%; P < 0.001).
3.2. DAT availability in patients with PD and DLB
The subregional DAT availability, ISR, and AR in the patients with PD and DLB are shown in Table 2. There was no significant difference between DAT availability in the anterior putamen, caudate, and ventral striatum. DAT availability in the posterior putamen was higher in patients with DLB than those with PD (3.51 ± 1.12 vs. 3.10 ± 1.13; P = 0.037), but this relationship was not significant after correction for the multiple comparison (Q = 0.111). In addition, patients with both PD and DLB had similar patterns of symmetry in the bilateral posterior putamen. The ISR in the putamen was higher in the PD group than in the DLB group (1.14 ± 0.22 vs. 1.03 ± 0.16; P = 0.021), but this relationship was not significant after correction for the multiple comparison (Q = 0.111).
3.3. Effect of amyloid on the subregional DAT availability
The subregional DAT availability according to amyloid positivity is shown for each group in Figure 1. The results of the interaction analysis on DAT availability are shown in Table 3. The interaction effect between the disease group and the presence of amyloid was significant for DAT availability in the anterior putamen, posterior putamen, caudate, and ventral striatum (Q = 0.093) (Table 3). Thus, further analyses were performed within each group.
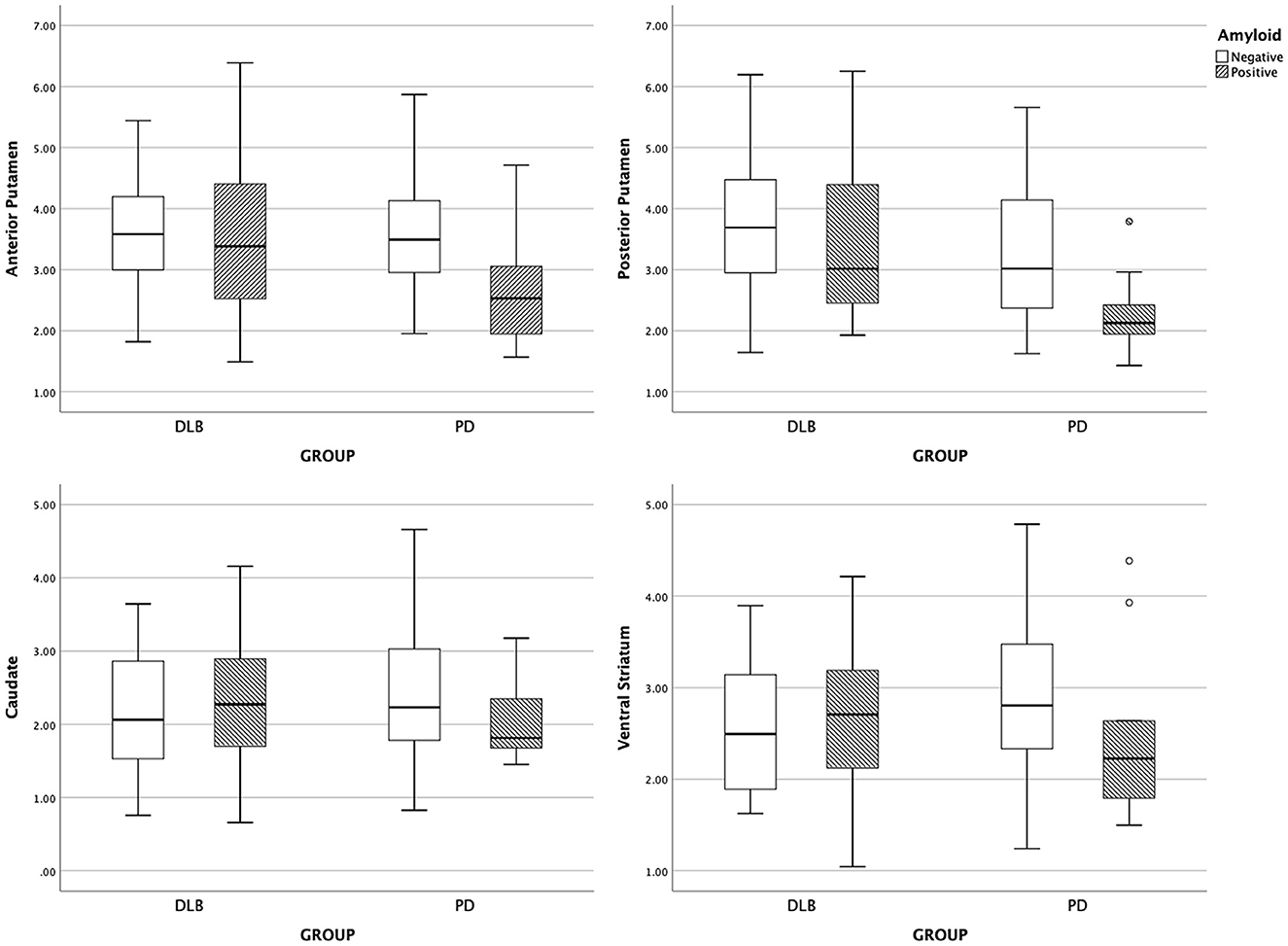
Figure 1. Boxplot of subregional DAT uptake. DLB, dementia with Lewy bodies; PD, Parkinson's disease.
With the presence of amyloid, the anterior putaminal DAT availability further decreased from 3.61 to 2.69 (B = −1.12, Q = 0.016), and the posterior putaminal DAT availability decreased from 3.32 to 2.28 (B = −1.20, Q = 0.016) in the PD group. However, the presence of amyloid had no effect on DAT availability in the ventral striatum (Q = 0.167) and caudate (Q = 0.358) in the PD group. In patients with DLB, the presence of amyloid exerted no effect on the DAT availability in striatal subregions, including the anterior putamen (Q = 0.705), posterior putamen (Q = 0.520), caudate (Q = 0.520), or ventral striatum (Q = 0.588) (Table 4).
In addition, since the interaction effect between the disease group and the presence of amyloid was not significant on the AR and ISR (Q = 0.899), further analyses were performed within both groups (Table 3). With the presence of amyloid, the AR increased from 1.08 ± 0.09 to 1.15 ± 0.17 in the DLB group and 1.13 ± 0.19 to 1.23 ± 0.23 in the PD group, showing no between-group difference. The presence of amyloid exerted no effect on the ISR in both the PD and DLB groups. However, the ISR was higher in the PD group than in the DLB group (Q = 0.016) (Table 5).
3.4. Effect of amyloid on the UPDRS Part III score
We evaluated the relationship between the presence of amyloid and parkinsonian motor symptoms in patients with LBD. There was no significant interaction effect between the disease group and the presence of amyloid on parkinsonian motor symptoms (P = 0.291). Within both disease groups, there was no significant effect of group (P = 0.310), or amyloid (P = 0.081). We further evaluated the indirect effect of amyloid on the UPDRS Part III score using putaminal DAT availability as a mediator in each disease group. The mediation analysis revealed that there was no significant mediation effect of DAT availability in the anterior putamen or posterior putamen on the UPDRS Part III score in the DLB group (Figures 2A, B). However, the indirect effect of amyloid on the UPDRS Part III score through the mediation of DAT availability in the anterior putamen (indirect effect, B = 4.713; 95% CI, 1.331–7.886; Figure 2C) and posterior putamen (indirect effect, B = 2.744; 95% CI, 0.299–5.936; Figure 2D) was significant in the PD group.
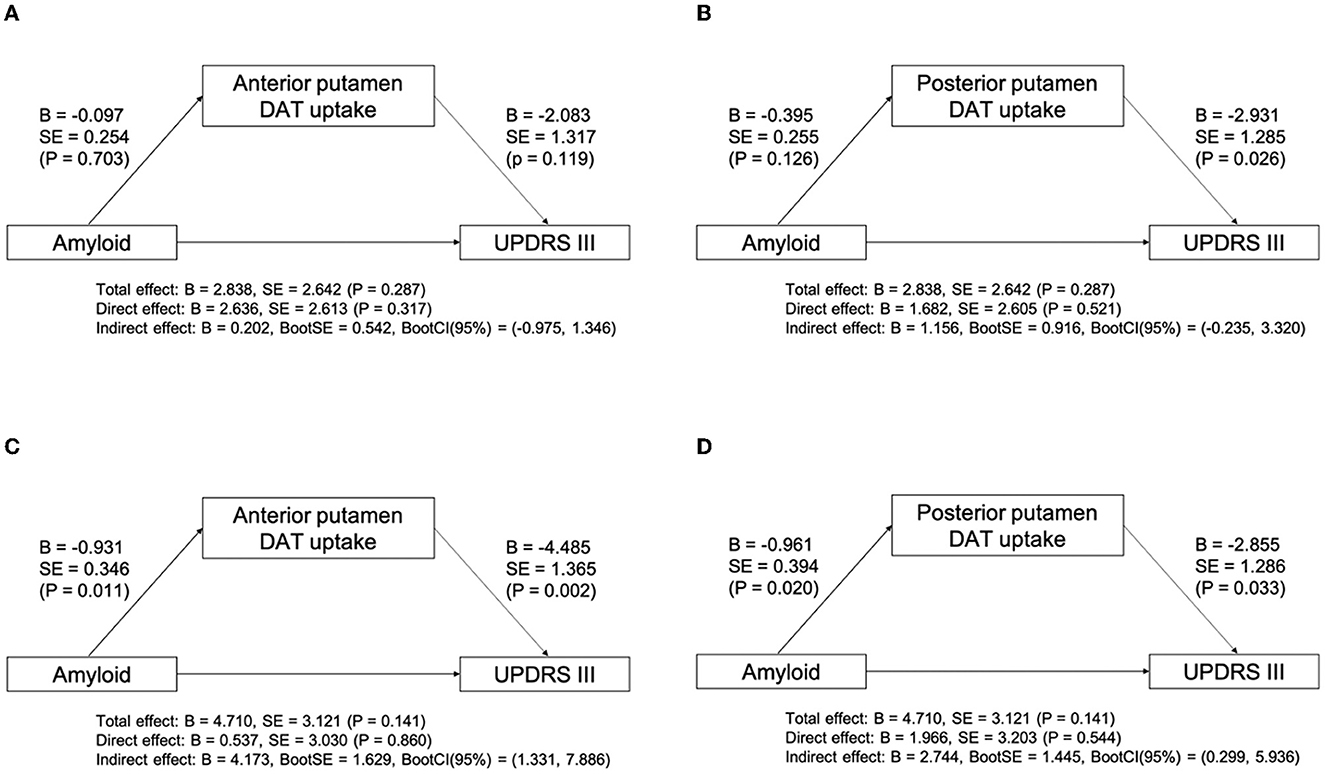
Figure 2. Mediation analysis between amyloid, subregional DAT uptake, and UPDRS Part III score after controlling for age, sex, DWMH, and PWMH in DLB (A, B) and PD (C, D) groups. B, unstandardized coefficient; BootCI, bootstrap confidence interval; BootSE, bootstrap SE; DWMH, deep WMH; DLB, dementia with Lewy bodies; P, P-value; PD, Parkinson's disease; PWMH, periventricular WMH; SE, standard error; UPDRS, unified Parkinson's disease rating scale; WMH, white matter hyperintensity.
4. Discussion
In this study, we found distinct amyloid-dependent or amyloid-independent 18F-FP-CIT PET patterns in patients with LBD. First, the presence of amyloid had a detrimental effect on the DAT availability in the anterior and posterior putamen in the PD group only. Second, the presence of amyloid was not associated with DAT availability in any striatal subregion in the DLB group. Third, the presence of amyloid was related to the increased asymmetricity of posterior putaminal DAT availability in both PD and DLB groups. Finally, additional amyloid pathology was not directly related to the parkinsonian motor symptom, but indirectly related to the increased UPDRS Part III score, which was mediated by DAT availability in the anterior and posterior putamen in the PD group only. These data suggest that the interaction between amyloid pathology and the nigrostriatal dopaminergic system is different between DLB and PD groups.
Although the location and mechanism of the interactions between toxic protein pathologies are yet to be elucidated in neurodegenerative diseases, several clinicopathological studies have provided evidence of a close intergroup association between toxic proteins. In patients with LBD including PD or DLB, a pathological study reported that the deposition of amyloid detected by [11C] Pittsburgh compound B had a significant correlation with Lewy body score and the severity of the stage of neurofibrillary tangle (Colom-Cadena et al., 2013; Shirvan et al., 2019). Furthermore, the presence of amyloid was associated with greater α-synuclein accumulation in LBD (Miller et al., 2022). In addition, Lewy body counts were significantly correlated with senile plaque counts and, to a lesser extent, neurofibrillary tangles in patients with PDD (Apaydin et al., 2002). Functional imaging studies using multiple radiotracers reported similar results that the burden of amyloid and tau proteins was closely correlated with each other in patients with DLB (Lee et al., 2018a). Importantly, the pattern of tau accumulation in DLB patients was distinctive compared to patients with AD.
Interestingly, we found that the presence of amyloid pathology in LBD had a distinct effect on the patterns of DAT availability depending on the specific LBD disease. Considering that subregional DAT availability in the striatum corresponds to dopaminergic neuronal projections from the substantia nigra (SN) (Fearnley and Lees, 1991), striatal DAT patterns in this study may represent region-specific dopaminergic neuronal loss in the SN. This study showed that the detrimental effect of amyloid on the lateral tier of nigra dopaminergic neurons that project to the putamen was dependent on the specific disease type of LBD. This means that the presence of amyloid led to significantly decreased DAT availability in the putamen, respective of the disease groups. In contrast, amyloid-independent DAT availability in the caudate and striatum was not specific to the type of LBD, such that the presence of amyloid had no significant effects on dopaminergic neurons outside the lateral portion of the SN in both groups. In addition, the detrimental effect of the presence of amyloid on the AR between the bilateral posterior putamen was significant in the PD group. This suggests that concomitant amyloid pathology influences more on the more affected side of the substantia nigra and that the interaction between amyloid and α-synuclein could have a synergistic effect rather than an additive effect. However, the presence of amyloid was associated with increased AR, without having a significant effect on the putaminal DAT availability in the DLB group. This suggests that the interaction between amyloid and α-synuclein in DLB patients may be present, at least on the more affected side of the substantia nigra, but overall, the interaction is less prominent than that in the PD group. These results may provide indirect evidence for the region- and disease-dependent effects of amyloid on the nigra dopaminergic neurons.
Recently, accumulating evidence has suggested that various clinicopathological traits in α-synucleinopathies may be ascribed to different α-synuclein strains (Shahnawaz et al., 2020; Holec and Woerman, 2021; Ayers et al., 2022). In terms of α-synuclein characteristics in patients with DLB, toxicity to the dopaminergic neurons in the SN appears to be less potent compared to α-synuclein in PD (Van der Perren et al., 2020). Moreover, Toledo et al. showed that demented subjects with AD and Lewy body-related pathology (Dem-AD-LB, which could partially overlap DLB with an amyloid group in our study) had less reduced substantia nigra neurons than PD with AD or PD without AD, and DLB without AD. Dem-AD-LB patients were less likely to show involvement in Lewy body-related pathology in the brainstem (Toledo et al., 2016). Therefore, disease-specific characteristics of α-synuclein strains would explain the more detrimental effects of concomitant amyloid on the putaminal DAT availability observed in the PD group compared to the DLB group. This result implies that the α-synuclein strain interacts differently with β-amyloid, depending on the specific type of α-synucleinopathies, as well as on specific regions of nigra dopaminergic neurons. Further clinicopathological data are needed to uncover the relationship between α-synuclein strains and other toxic proteins.
This study also demonstrated that additional amyloid pathology was indirectly related to the increased UPDRS Part III score. In addition, the detrimental effect of amyloid on parkinsonian motor symptoms in patients with LBD was disease-specific. The indirect effect of amyloid was present in the PD group only. In terms of neuroanatomical correlates of parkinsonian motor symptoms, it has been postulated that parkinsonism in patients with PD is based on neuronal loss in the SN, whereas parkinsonism in DLB is attributed to widespread anatomical structures beyond the SN (Postuma et al., 2012). Given that the different neural correlates of parkinsonism exist in LBD, it is speculated that the amyloid pathology may exert effects differently in the distinct anatomical area responsible for parkinsonism, depending on the type of LBD. The mediation analysis revealed that the effect of amyloid on motor deficits was mediated by DAT availability in the anterior and posterior putamen in the PD group. Considering that the effect of amyloid on DAT availability in the anterior and posterior putamen was LBD type-specific, additional neurotoxic effects of amyloid on nigra dopaminergic neurons projecting into the putamen that exhibits a disease-specific pattern may result in additive motor deficits in the PD group. To date, the location and mechanisms of the interaction between amyloid and synuclein pathologies remain to be elucidated. In our study, the presence of amyloid was associated with putaminal dopaminergic depletion, further correlating with motor dysfunction. Therefore, among striatal subregions in patients with PD, the putamen might be the primary region where the interaction between amyloid and synuclein pathologies occurs. This result may provide a clue for clinical relevance regarding the interaction of mixed pathologies in neurodegenerative diseases.
In terms of spatial or asymmetric patterns, the AR and ISR did not differ between the DLB and PD groups. Thus, there was no difference between the two groups in the asymmetry or anterior–posterior gradient of substantia nigra degeneration evaluated by DAT imaging. However, when considering the presence of amyloid, the anterior–posterior gradient of DAT availability in the putamen was more prominent in the PD group relative to the DLB group. There has been controversy over differences in patterns of striatal DAT availability between DLB and PD patients. The patterns of striatal DAT availability were compatible between two groups (Colloby et al., 2004; Marquie et al., 2014; Gomperts et al., 2016; Pilotto et al., 2019), whereas others reported lower caudate DAT availability in DLB patients and greater asymmetricity or antero-posterior gradient of DAT availability in PD patients (Ransmayr et al., 2001; O'Brien et al., 2004; Walker et al., 2004). Our results imply that the presence of amyloid may influence the spatial or asymmetric distribution of DAT availability in the PD and DLD groups, and thus, the prevalence of amyloid positivity may contribute to the different results in previous studies.
Our study has some limitations. First, the diagnosis of LBD was not pathologically confirmed; thus, an alternative diagnosis of DLB may be possible. However, all DLB patients in this study met the probable DLB criteria from the 2017 revised criteria for DLB. In addition, we used additional supportive imaging biomarkers to diagnose patients more accurately with DLB using 18F-FP-CIT PET. Second, there was a significant difference in the proportion of patients with dementia between the two groups. Although we tried to overcome this difference by using cognitive status as covariates, the results should be interpreted with caution. Third, we used a dichotomized approach to amyloid rather than global SUVR as a continuous variable. Traditionally, quantitative cutoffs for amyloid PET positivity have been defined to discriminate AD patients from non-AD patients. However, there have been some studies dealing with the subthreshold amyloid accumulation which has clinical significance for a worse prognosis (Bischof and Jacobs, 2019). Therefore, we performed additional analysis with global SUVR as a continuous variable. However, there was no significant interaction effect or independent effect of amyloid when we treated the amyloid uptake as a continuous variable (Supplementary Table 1). This might suggest that a substantial accumulation of amyloid is needed to have significant interaction with PD. Finally, the patients in our study had a relatively older onset age. Older onset age could affect the prevalence of amyloid positivity or be associated with aggravated nigra neuronal cell death. However, there was no difference in the onset age between the two groups (74.07 ± 6.47 in the DLB group and 73.81 ± 6.56 in the PD group).
In conclusion, DLB and PD interact with amyloid pathology differently with regard to nigrostriatal dopaminergic dysfunction, which could provide indirect evidence of the presence of different α-synuclein strains in synucleinopathies and their distinct interaction with amyloid pathology.
Data availability statement
The data and code used in this work are available from the corresponding author upon reasonable request.
Ethics statement
The studies involving human participants were reviewed and approved by Yonsei University Severance Hospital Institutional Review Board. Written informed consent for participation was not required for this study in accordance with the national legislation and the institutional requirements.
Author contributions
KB, JC, and PL contributed to the conception and design of the manuscript. KB, SC, HY, and YS organized the database. KB, MP, YL, and JC performed the statistical analysis. KB wrote the first draft of the manuscript. JC, MP, and PL wrote sections of the manuscript. All authors contributed to the manuscript revision, read, and approved the submitted version.
Funding
This work was supported by a grant from the Korea Health Technology R&D Project through the Korean Healthy Industry Development Institute (KHIDI), funded by the Ministry of Health and Welfare, Republic of Korea (grant number: HU21C0053) awarded to PL.
Acknowledgments
The content of this manuscript has been presented as an abstract at the 2022 International Congress of Parkinson's Disease and Movement Disorders (Baik et al., 2022).
Conflict of interest
The authors declare that the research was conducted in the absence of any commercial or financial relationships that could be construed as a potential conflict of interest.
Publisher's note
All claims expressed in this article are solely those of the authors and do not necessarily represent those of their affiliated organizations, or those of the publisher, the editors and the reviewers. Any product that may be evaluated in this article, or claim that may be made by its manufacturer, is not guaranteed or endorsed by the publisher.
Supplementary material
The Supplementary Material for this article can be found online at: https://www.frontiersin.org/articles/10.3389/fnagi.2023.1196602/full#supplementary-material
References
Apaydin, H., Ahlskog, J. E., Parisi, J. E., Boeve, B. F., and Dickson, D. W. (2002). Parkinson disease neuropathology: later-developing dementia and loss of the levodopa response. Arch. Neurol. 59, 102–112. doi: 10.1001/archneur.59.1.102
Ayers, J. I., Lee, J., Monteiro, O., Woerman, A. L., Lazar, A. A., Condello, C., et al. (2022). Different α-synuclein prion strains cause dementia with Lewy bodies and multiple system atrophy. Proc. Nat. Acad. Sci. 119, e2113489119. doi: 10.1073/pnas.2113489119
Baik, K., Cha, J., Park, M., Lee, Y., Kang, S. W., and Yoon, S. H. Distinct amyloid-dependent patterns of nigral dopamine depletion in Lewy body diseases. Mov. Disord. (2022) 37(suppl. 1). Available online at: https://www.mdsabstracts.org/abstract/distinct-amyloid-dependent-patterns-of-nigral-dopamine-depletion-in-lewy-body-diseases/.
Bischof, G. N., and Jacobs, H. I. (2019). Subthreshold amyloid and its biological and clinical meaning: long way ahead. Neurology 93, 72–79. doi: 10.1212/WNL.0000000000007747
Biundo, R., Weis, L., Fiorenzato, E., Pistonesi, F., Cagnin, A., Bertoldo, A., et al. (2021). The contribution of beta-amyloid to dementia in Lewy body diseases: a 1-year follow-up study. Brain Commun. 3, fcab180. doi: 10.1093/braincomms/fcab180
Candelise, N., Schmitz, M., Llorens, F., Villar-Piqu,é, A., Cramm, M., Thom, T., et al. (2019). Seeding variability of different alpha synuclein strains in synucleinopathies. Ann. Neurol. 85, 691–703. doi: 10.1002/ana.25446
Colloby, S. J., O'brien, J. T., Fenwick, J. D., Firbank, M. J., Burn, D. J., Mckeith, I. G., et al. (2004). The application of statistical parametric mapping to 123I-FP-CIT SPECT in dementia with Lewy bodies, Alzheimer's disease and Parkinson's disease. Neuroimage 23, 956–966. doi: 10.1016/j.neuroimage.2004.06.045
Colom-Cadena, M., Gelpi, E., Charif, S., Belbin, O., Blesa, R., Mart,í, M. J., et al. (2013). Confluence of α-synuclein, tau, and β-amyloid pathologies in dementia with Lewy bodies. J. Neuropathol. Exp. Neurol. 72, 1203–1212. doi: 10.1097/NEN.0000000000000018
Compta, Y., Parkkinen, L., O'sullivan, S. S., Vandrovcova, J., Holton, J. L., Collins, C., et al. (2011). Lewy- and Alzheimer-type pathologies in Parkinson's disease dementia: which is more important? Brain 134, 1493–1505. doi: 10.1093/brain/awr031
Dubois, B., Burn, D., Goetz, C., Aarsland, D., Brown, R. G., Broe, G. A., et al. (2007). Diagnostic procedures for Parkinson's disease dementia: recommendations from the movement disorder society task force. Mov. Dis. 22, 2314–2324. doi: 10.1002/mds.21844
Fearnley, J. M., and Lees, A. J. (1991). Ageing and parkinson's disease: substantia nigra regional selectivity. Brain 114, 2283–2301. doi: 10.1093/brain/114.5.2283
Ferreira, D., Przybelski, S. A., Lesnick, T. G., Lemstra, A. W., Londos, E., Blanc, F., et al. (2020). β-Amyloid and tau biomarkers and clinical phenotype in dementia with Lewy bodies. Neurology 95, e3257–e3268. doi: 10.1212/WNL.0000000000010943
Fujishiro, H., Iseki, E., Higashi, S., Kasanuki, K., Murayama, N., Togo, T., et al. (2010). Distribution of cerebral amyloid deposition and its relevance to clinical phenotype in Lewy body dementia. Neurosci. Lett. 486, 19–23. doi: 10.1016/j.neulet.2010.09.036
Gomperts, S. N., Locascio, J. J., Rentz, D., Santarlasci, A., Marquie, M., Johnson, K. A., et al. (2013). Amyloid is linked to cognitive decline in patients with Parkinson disease without dementia. Neurology 80, 85–91. doi: 10.1212/WNL.0b013e31827b1a07
Gomperts, S. N., Marquie, M., Locascio, J. J., Bayer, S., Johnson, K. A., and Growdon, J. H. (2016). PET radioligands reveal the basis of dementia in Parkinson's disease and dementia with Lewy bodies. Neurodegen. Dis. 16, 118–124. doi: 10.1159/000441421
Hepp, D. H., Vergoossen, D. L., Huisman, E., Lemstra, A. W., Bank, N. B., Berendse, H. W., et al. (2016). Distribution and load of amyloid-β pathology in Parkinson disease and dementia with Lewy bodies. J. Neuropathol. Exp. Neurol. 75, 936–945. doi: 10.1093/jnen/nlw070
Holec, S. A., and Woerman, A. L. (2021). Evidence of distinct α-synuclein strains underlying disease heterogeneity. Acta Neuropathol. 142, 73–86. doi: 10.1007/s00401-020-02163-5
Jellinger, K. A. (2018). Dementia with Lewy bodies and Parkinson's disease-dementia: current concepts and controversies. J. Neural. Transm. 125, 615–650. doi: 10.1007/s00702-017-1821-9
Jellinger, K. A. (2023). Morphological characteristics differentiate dementia with Lewy bodies from Parkinson disease with and without dementia. J. Neural Transm. 1–14. doi: 10.1007/s00702-023-02660-3
Klein, A., and Tourville, J. (2012). 101 labeled brain images and a consistent human cortical labeling protocol. Front. Neurosci. 6, 171. doi: 10.3389/fnins.2012.00171
Lee, S. H., Cho, H., Choi, J. Y., Lee, J. H., Ryu, Y. H., Lee, M. S., et al. (2018a). Distinct patterns of amyloid-dependent tau accumulation in Lewy body diseases. Mov. Disord. 33, 262–272. doi: 10.1002/mds.27252
Lee, Y.-G., Jeon, S., Yoo, H. S., Chung, S. J., Lee, S.-K., Lee, P. H., et al. (2018b). Amyloid-β-related and unrelated cortical thinning in dementia with Lewy bodies. Neurobiol. Aging 72, 32–39. doi: 10.1016/j.neurobiolaging.2018.08.007
Litvan, I., Aarsland, D., Adler, C. H., Goldman, J. G., Kulisevsky, J., Mollenhauer, B., et al. (2011). MDS Task Force on mild cognitive impairment in Parkinson's disease: critical review of PD-MCI. Mov. Disord. 26, 1814–1824. doi: 10.1002/mds.23823
Marquie, M., Locascio, J. J., Rentz, D. M., Becker, J. A., Hedden, T., Johnson, K. A., et al. (2014). Striatal and extrastriatal dopamine transporter levels relate to cognition in Lewy body diseases: an 11 C altropane positron emission tomography study. Alzheimer's Res. Therapy 6, 1–10. doi: 10.1186/s13195-014-0052-7
Martersteck, A. C. (2020). Network Failure, Atrophy, and Tau Pathology in Aphasic Alzheimer Disease. Evanston, IL: Northwestern University.
McKeith, I. G., Boeve, B. F., Dickson, D. W., Halliday, G., Taylor, J.-P., Weintraub, D., et al. (2017). Diagnosis and management of dementia with Lewy bodies: Fourth consensus report of the DLB Consortium. Neurology 89, 88–100. doi: 10.1212/WNL.0000000000004058
McMillan, C. T., and Wolk, D. A. (2016). Presence of cerebral amyloid modulates phenotype and pattern of neurodegeneration in early Parkinson's disease. J. Neurol. Neurosurg. Psychiatry 87, 1112–1122. doi: 10.1136/jnnp-2015-312690
Miller, R. L., Dhavale, D. D., O'shea, J. Y., Andruska, K. M., Liu, J., Franklin, E. E., et al. (2022). Quantifying regional α-synuclein, amyloid β, and tau accumulation in lewy body dementia. Annals Clin. Trans. Neurol. 9, 106–121. doi: 10.1002/acn3.51482
Muller, M. L., Frey, K. A., Petrou, M., Kotagal, V., Koeppe, R. A., Albin, R. L., et al. (2013). beta-Amyloid and postural instability and gait difficulty in Parkinson's disease at risk for dementia. Mov. Disord. 28, 296–301. doi: 10.1002/mds.25213
O'Brien, J. T., Colloby, S., Fenwick, J., Williams, E. D., Firbank, M., Burn, D., et al. (2004). Dopamine transporter loss visualized with FP-CIT SPECT in the differential diagnosis of dementia with Lewy bodies. Arch. Neurol. 61, 919–925. doi: 10.1001/archneur.61.6.919
Petrou, M., Bohnen, N. I., Müller, M. L., Koeppe, R. A., Albin, R. L., and Frey, K. A. (2012). Aβ-amyloid deposition in patients with Parkinson disease at risk for development of dementia. Neurology 79, 1161–1167. doi: 10.1212/WNL.0b013e3182698d4a
Pilotto, A., Di Cola, F. S., Premi, E., Grasso, R., Turrone, R., Gipponi, S., et al. (2019). Extrastriatal dopaminergic and serotonergic pathways in Parkinson's disease and in dementia with Lewy bodies: a 123 I-FP-CIT SPECT study. Eur. J. Nucl. Med. Mol. Imaging 46, 1642–1651. doi: 10.1007/s00259-019-04324-5
Postuma, R. B., Lang, A. E., Gagnon, J. F., Pelletier, A., and Montplaisir, J. Y. (2012). How does parkinsonism start? Prodromal parkinsonism motor changes in idiopathic REM sleep behaviour disorder. Brain 135, 1860–1870. doi: 10.1093/brain/aws093
Ransmayr, G., Seppi, K., Donnemiller, E., Luginger, E., Marksteiner, J., Riccabona, G., et al. (2001). Striatal dopamine transporter function in dementia with Lewy bodies and Parkinson's disease. Eur. J. Nucl. Med. 28, 1523–1528. doi: 10.1007/s002590100571
Sabri, O., Sabbagh, M. N., Seibyl, J., Barthel, H., Akatsu, H., Ouchi, Y., et al. (2015). Florbetaben PET imaging to detect amyloid beta plaques in Alzheimer's disease: phase 3 study. Alzheimer's & dementia 11, 964–974. doi: 10.1016/j.jalz.2015.02.004
Sarro, L., Senjem, M. L., Lundt, E. S., Przybelski, S. A., Lesnick, T. G., Graff-Radford, J., et al. (2016). Amyloid-β deposition and regional grey matter atrophy rates in dementia with Lewy bodies. Brain 139, 2740–2750. doi: 10.1093/brain/aww193
Sengupta, U., Puangmalai, N., Bhatt, N., Garcia, S., Zhao, Y., and Kayed, R. (2020). Polymorphic α-synuclein strains modified by dopamine and docosahexaenoic acid interact differentially with tau protein. Mol. Neurobiol. 57, 2741. doi: 10.1007/s12035-020-01913-6
Shahnawaz, M., Mukherjee, A., Pritzkow, S., Mendez, N., Rabadia, P., Liu, X., et al. (2020). Discriminating α-synuclein strains in Parkinson's disease and multiple system atrophy. Nature 578, 273–277. doi: 10.1038/s41586-020-1984-7
Shirvan, J., Clement, N., Ye, R., Katz, S., Schultz, A., Johnson, K. A., et al. (2019). Neuropathologic correlates of amyloid and dopamine transporter imaging in Lewy body disease. Neurology 93, e476–e484. doi: 10.1212/WNL.0000000000007855
Sierra, M., Gelpi, E., Mart,í, M., and Compta, Y. (2016). Lewy-and Alzheimer-type pathologies in midbrain and cerebellum across the Lewy body disorders spectrum. Neuropathol. Appl. Neurobiol. 42, 451–462. doi: 10.1111/nan.12308
Toledo, J. B., Gopal, P., Raible, K., Irwin, D. J., Brettschneider, J., Sedor, S., et al. (2016). Pathological α-synuclein distribution in subjects with coincident Alzheimer's and Lewy body pathology. Acta Neuropathol. 131, 393–409. doi: 10.1007/s00401-015-1526-9
Van der Perren, A., Gelders, G., Fenyi, A., Bousset, L., Brito, F., Peelaerts, W., et al. (2020). The structural differences between patient-derived α-synuclein strains dictate characteristics of Parkinson's disease, multiple system atrophy and dementia with Lewy bodies. Acta Neuropathol. 139, 977. doi: 10.1007/s00401-020-02157-3
Walker, Z., Costa, D., Walker, R., Lee, L., Livingston, G., Jaros, E., et al. (2004). Striatal dopamine transporter in dementia with Lewy bodies and Parkinson disease: a comparison. Neurology 62, 1568–1572. doi: 10.1212/01.WNL.0000123248.39847.1D
Walker, Z., Jaros, E., Walker, R. W., Lee, L., Costa, D. C., Livingston, G., et al. (2007). Dementia with Lewy bodies: a comparison of clinical diagnosis, FP-CIT single photon emission computed tomography imaging and autopsy. J. Neurol. Neurosurg. Psychiatry 78, 1176–1181. doi: 10.1136/jnnp.2006.110122
Yarnall, A. J., Breen, D. P., Duncan, G. W., Khoo, T. K., Coleman, S. Y., Firbank, M. J., et al. (2014). Characterizing mild cognitive impairment in incident Parkinson disease: the ICICLE-PD study. Neurology 82, 308–316. doi: 10.1212/WNL.0000000000000066
Yoo, H. S., Lee, S., Chung, S. J., Lee, Y. H., Ye, B. S., Sohn, Y. H., et al. (2020). Clinical and striatal dopamine transporter predictors of beta-amyloid in dementia with Lewy bodies. Neurology 94, e1344–e1352. doi: 10.1212/WNL.0000000000009168
Keywords: Parkinson's disease, dementia with Lewy bodies, Lewy body disease, florbetaben PET, dopamine transporter PET
Citation: Baik K, Cha J, Park M, Lee Y, Chung SJ, Yoo HS, Sohn YH and Lee PH (2023) Distinct amyloid-dependent patterns of nigra dopamine depletion in Lewy body diseases. Front. Aging Neurosci. 15:1196602. doi: 10.3389/fnagi.2023.1196602
Received: 30 March 2023; Accepted: 14 July 2023;
Published: 08 August 2023.
Edited by:
Kurt A. Jellinger, University of Vienna, AustriaReviewed by:
Rengasamy Balakrishnan, Konkuk University, Republic of KoreaHiroshige Fujishiro, Nagoya University, Japan
Copyright © 2023 Baik, Cha, Park, Lee, Chung, Yoo, Sohn and Lee. This is an open-access article distributed under the terms of the Creative Commons Attribution License (CC BY). The use, distribution or reproduction in other forums is permitted, provided the original author(s) and the copyright owner(s) are credited and that the original publication in this journal is cited, in accordance with accepted academic practice. No use, distribution or reproduction is permitted which does not comply with these terms.
*Correspondence: Phil Hyu Lee, cGhsZWUmI3gwMDA0MDt5dWhzLmFj
†These authors have contributed equally to this work and share first authorship