Corrigendum: Research trends and hotspots of glial fibrillary acidic protein within the area of Alzheimer's disease: a bibliometric analysis
- 1Department of Laboratory Medicine, Peking Union Medical College Hospital, Peking Union Medical College and Chinese Academy of Medical Science, Beijing, China
- 2Medical Science Research Center (MRC), Peking Union Medical College Hospital, Peking Union Medical College and Chinese Academy of Medical Sciences, Beijing, China
- 3Department of Neurology, Peking Union Medical College Hospital, Peking Union Medical College and Chinese Academy of Medical Sciences, Beijing, China
- 4State Key Laboratory of Complex Severe and Rare Diseases, Peking Union Medical College Hospital, Peking Union Medical College and Chinese Academy of Medical Sciences, Beijing, China
Objective: Our aim was to analyze the trends and hotspots on glial fibrillary acidic protein (GFAP) within the area of Alzheimer’s disease (AD) by using a bibliometric method, which is currently missing.
Methods: All articles and reviews on GFAP within the area of AD from inception to December 31, 2022, were searched from the Web of Science Core Collection. Full records were derived, imported into Microsoft Excel, and analyzed by BIBLIOMETRC, VOSviewer, and CiteSpace.
Results: In total, 2,269 publications, including 2,166 articles, were ultimately included. The number of publications from 81 countries/regions and 527 academic journals increased annually. The top three prolific countries and institutions were the USA, China, and England, the University of Gothenburg (Sweden), Federal University of Rio Grande do Sul (Brazil), and UCL Queen Square Institute of Neurology (England). Henrik Zetterberg from the University of Gothenburg, Kaj Blennow from the University of Gothenburg, and Alexei Verkhratsky from the University of Manchester were the top three prolific and cited authors; Journal of Alzheimer’s Disease, Brain Research, and Neuroscience contributed the most publications. The top key areas of research included “molecular, biology, and genetics” and “molecular, biology, and immunology,” and the top published and linked meaningful keywords included oxidative stress, inflammation/neuroinflammation, microglia, hippocampus, amyloid, cognitive impairment, tau, and dysfunction.
Conclusion: Based on the bibliometric analysis, the number of publications on GFAP within the area of AD has been rapidly increasing, especially in the past several years. Oxidative stress and inflammation are research hotspots, and GFAP in body fluids, especially blood, could be used for large-scale screening for AD.
1. Introduction
As the most common form of dementia, Alzheimer’s disease (AD) comprises 60–80% of all dementia cases. Worldwide, the numbers of patients with AD are expected to reach 65.7 million in 2030 and 115.4 million in 2050, with a rate of one new case every 3 s (Prince et al., 2013). As a fatal illness, AD’s average survival time ranges from 4 to 8 years for patients aged 65 years and older (Larson et al., 2004). Since AD is still not curable, early diagnosis and intervention are critical to slow the development of AD and improve the quality of life of patients. In addition to the classical biomarkers recommended by guidelines (Zou et al., 2023), many other biomarkers in cerebrospinal fluid (CSF) and/or serum/plasma, such as neurofilament light (NfL), glial fibrillary acidic protein (GFAP), and alpha-synuclein, were recently recommended for the early and differential diagnosis of AD (Teunissen et al., 2022; Johnson et al., 2023).
As an intermediate filament structural protein involved in cytoskeleton assembly and integrity, GFAP is highly expressed in activated glial cells (Ganne et al., 2022). Thus, GFAP serves as a biomarker of neurodegenerative diseases, including AD, dementia with Lewy bodies, and frontotemporal dementia. In recent years, an increasing number of studies have aimed to explore the effect of GFAP on the development, diagnosis, and prognosis of AD. GFAP in body fluids, including CSF and serum/plasma, is considered a good biomarker for the early diagnosis and prognosis of AD, especially blood GFAP, which is considered an excellent biomarker for large-scale screening (Heimfarth et al., 2022; Teunissen et al., 2022; Guo et al., 2023). Moreover, it was reported that GFAP plays important roles in AD development, such as neuropathic aggregate accrual, and could become a novel promising therapeutic target to alleviate, delay, and even prevent AD (Ganne et al., 2022). However, GFAP is not specific for AD but is related to many neurological diseases (Heimfarth et al., 2022). Thus, it is still urgent to further explore the relationship between GFAP and AD and clarify the effect of GFAP on the occurrence, development, diagnosis, and prognosis of AD.
Thus, we conducted the first bibliometric analysis to identify research trends and hotspots on GFAP within the area of AD to provide a comprehensive understanding of this topic and further guide future research directions.
2. Methods
2.1. Search strategy
All data were collected from the Science Citation Index Expanded (SCI-E) of the Web of Science Core Collection database (WoSCC). The search strategy was as follows: ((TS = (GFAP)) OR TS = (glial fibrillary acidic protein)) AND ((((((TS = (alzheimer)) OR TS = (alzheimer’s)) OR TS = (alzheimer-disease)) OR TS = (alzheimers)) OR TS = (alzheimers-disease)) OR TS = (AD)). Only articles and reviews from inception to December 31, 2022, were included in this study. No language and region were restricted.
2.2. Analytical tool
Full records and cited references of all publications were derived from the WoSCC database. Important bibliometric parameters such as title, publication year, author, institution, keywords, journal, and citation were extracted, imported into Microsoft Excel 16.0 (Redmond, WA, US), and analyzed by the BIBLIOMETRC Online analysis platform1, VOSviewer (version 1.6.18), and CiteSpace (version 6.1 R3) software. The data on publication trends, countries/regions, and annual hot keywords were analyzed by the BIBLIOMETRC Online analysis platform, interdisciplinary relationships, and the top 15 keywords were analyzed by CiteSpace software, and other analyses were conducted by VOSviewer software.
3. Results
3.1. General publication trends
As of December 31, 2022, a total of 2,269 publications about GFAP within the area of AD were recognized, of which 2,166 publications were recognized as articles, and others were reviews. As shown in Figure 1, an overall upward trend for the number of publications on GFAP within the area of AD was found despite some minor fluctuations. Since 2014, the upward trend has been successive and remarkable, which means that increasing attention has been given to GFAP within the area of AD in recent years. Moreover, studies focused on this area have also become a hotspot in China, with remarkably more publications in recent years.
3.2. Analysis of countries/regions
Based on the VOSviewer software, the international collaboration among different countries/regions in this field is depicted in Figure 2A. With the minimum number of documents of a country set at three, 57 of 81 countries/regions were selected for visualization (Figure 2B). The USA, China, England, Japan, Spain, Germany, and Sweden are the largest nodes and are more broadly connected, indicating their close collaboration and significant academic influence in the field. Moreover, the top 10 contributing countries are shown in Figure 2C. The USA contributed the most publications in the field, with 776 publications and 47,971 total citations. China ranked second, with 326 publications and 7,181 total citations, and England ranked third, with 192 publications and 10,612 total citations.
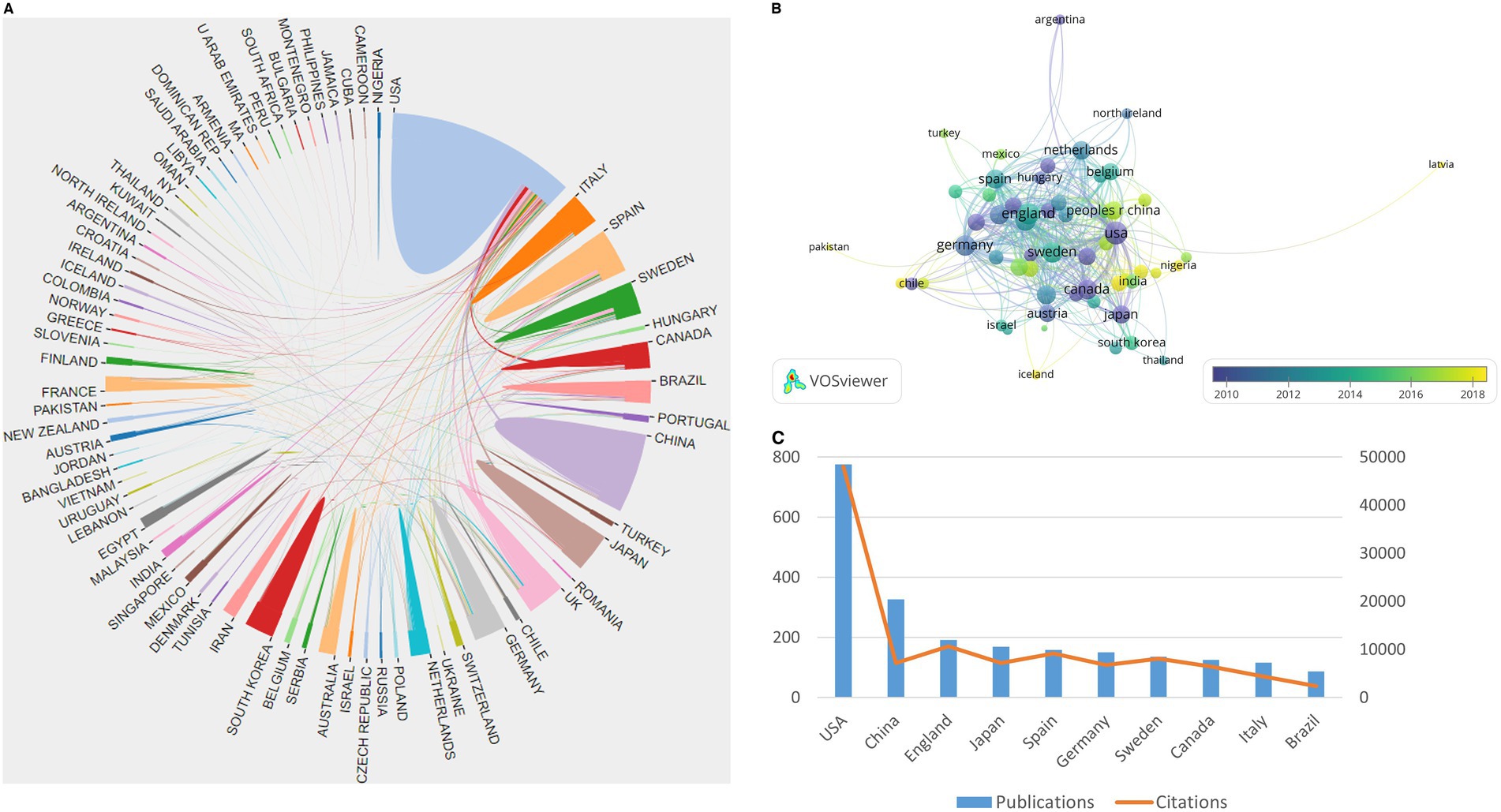
Figure 2. Summary of dominant countries/regions. (A) Collaboration among all countries; (B) Collaboration among 57 countries with a minimum number of documents of a country equal to three; the node size is based on the number of articles, and the total link strength is based on the power of cooperation between two nodes; (C) The publications and citations of the top 10 contributing countries.
3.3. Analysis of institutions
As shown in Table 1, the top 10 most prolific and highly cited institutions focused on GFAP within the area of AD are listed. The University of Gothenburg (Sweden) ranked first, with 91 publications and 475 total citations; the Federal University of Rio Grande do Sul (Brazil) ranked second, with 65 publications and 200 total citations; and the UCL Queen Square Institute of Neurology ranked third (England), with 63 articles. Of the top 10 institutions, three institutions are located in Sweden, and another three institutions are located in the USA. Figure 3 displays the network of collaborations among 41 dominant institutions with the minimum number of documents of an institution equal to 15. As one of the most central nodes, the University of Gothenburg (Sweden) contributed most publications, focused on research frontiers, and closely collaborated with many other institutions, including McGill University (Canada), the University of Barcelona (Spain), and the University of California, San Francisco (USA).
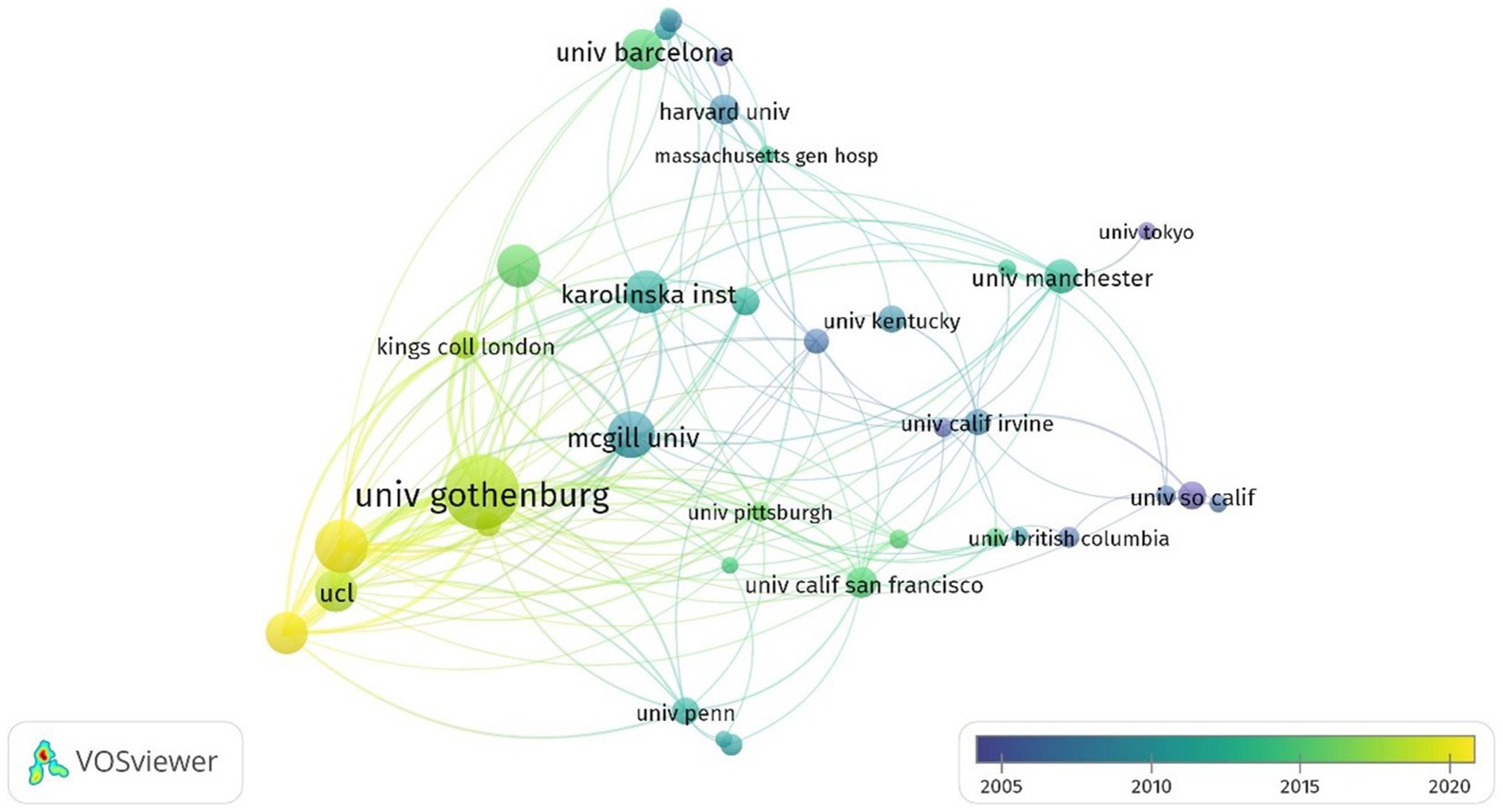
Figure 3. Summary of dominant institutions. The node size is based on the number of articles, and the total link strength is based on the power of cooperation between two nodes.
3.4. Analysis of authors
In total, 12,559 authors contributed to the publications on GFAP within the area of AD. The top 127 prolific and highly cited authors with at least five citations and their correlations are shown in Figure 4. The top three prolific and most cited authors were Henrik Zetterberg from the University of Gothenburg (publications, n = 52; total citations, n = 2,273); Kaj Blennow from the University of Gothenburg (publications, n = 45; total citations, n = 2,217); and Alexei Verkhratsky from the University of Manchester (publications, n = 17; total citations, n = 2,269). Of all the authors, Kaj Blennow and Henrik Zetterberg made more remarkable contributions in this area, with significantly higher total link strengths.
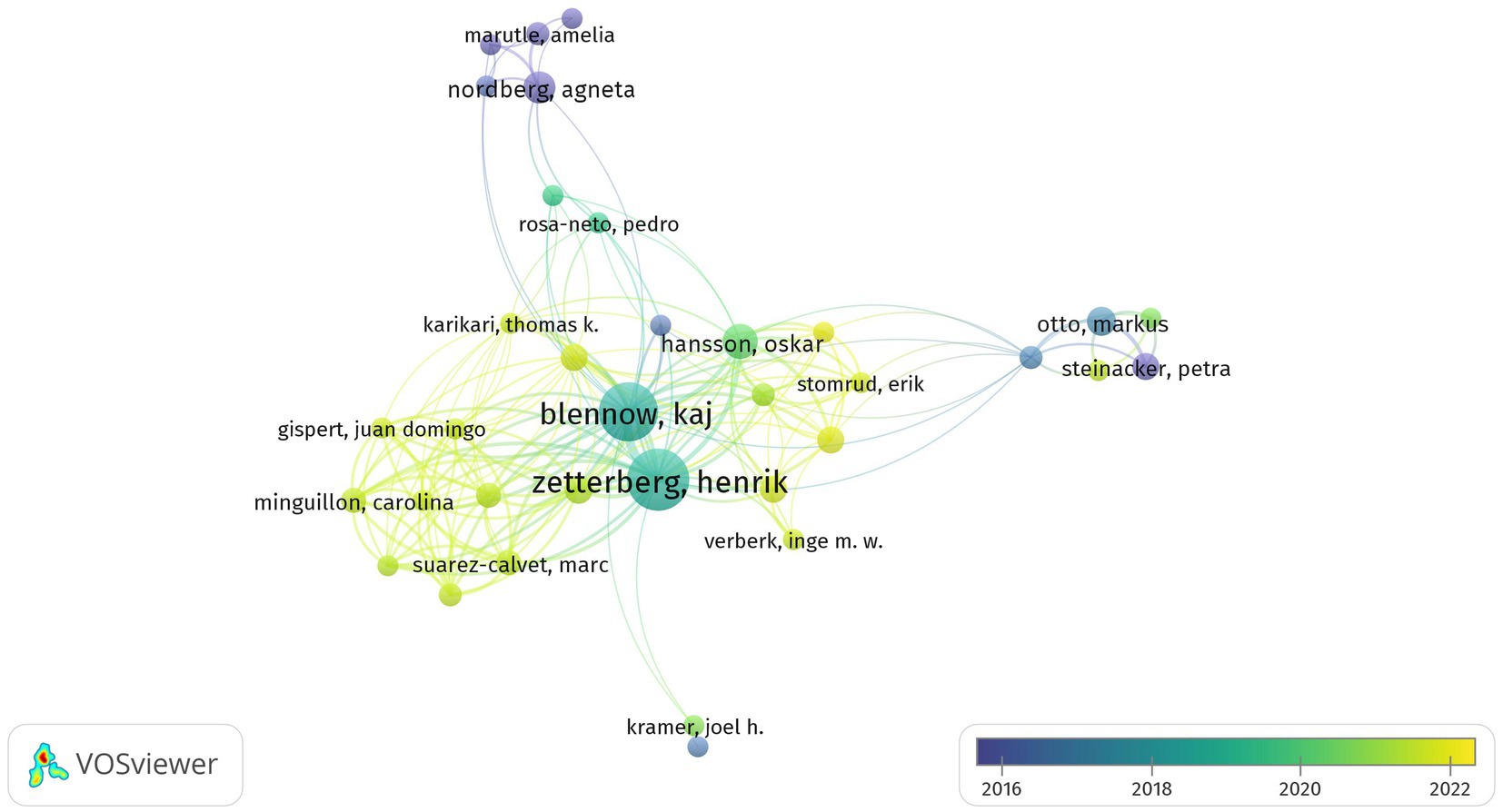
Figure 4. Summary of the dominant authors. The node size is based on the number of articles, and the total link strength is based on the power of cooperation between two nodes.
3.5. Analysis of journals and top 10 most cited publications
All the retrieved publications were from 527 different journals, and the top 10 most prolific journals are listed in Table 2. The top five journals in terms of number of publications were Journal of Alzheimer’s Disease (78 publications), Brain Research (69 publications), Neuroscience (63 publications), Neurobiology of Aging (58 publications), and Journal of Neuroinflammation (55 publications); the top five cited journals were Journal of Neuroscience (5,915 citations), Neurobiology of Aging (4,598 citations), Neuroscience (4,193 citations), Brain Research (3,680 citations), and Journal of Alzheimer’s Disease (3,059 citations). Of all 527 journals, the Journal of Alzheimer’s Disease contributed to the most total link strength for the publications on GFAP within the area of AD. Moreover, the top 10 most cited publications are shown in Table 3, seven of which are review publications. The most cited publication (Olsson et al., 2016) was a systematic review and meta-analysis, which summarized the diagnostic performance of common CSF and blood biomarkers for AD. However, published articles about the diagnostic performance of CSF and/or blood GFAP at that time were still limited; thus, new meta-analysis is still needed.
3.6. Analysis of research areas and keywords
As shown in Figure 5, the dual-map overlay of the discipline distribution of publications revealed the basis of discipline orientation and interaction between disciplines. Of 21 disciplines, “molecular, biology, genetics,” “molecular, biology, immunology”; and “psychology education social” were the key research areas. In addition, the published articles on “molecular, biology, immunology” were based on those on “molecular, biology, genetics,” suggesting that “genetics” had provided a foundation for this area, especially the “immunology” discipline.
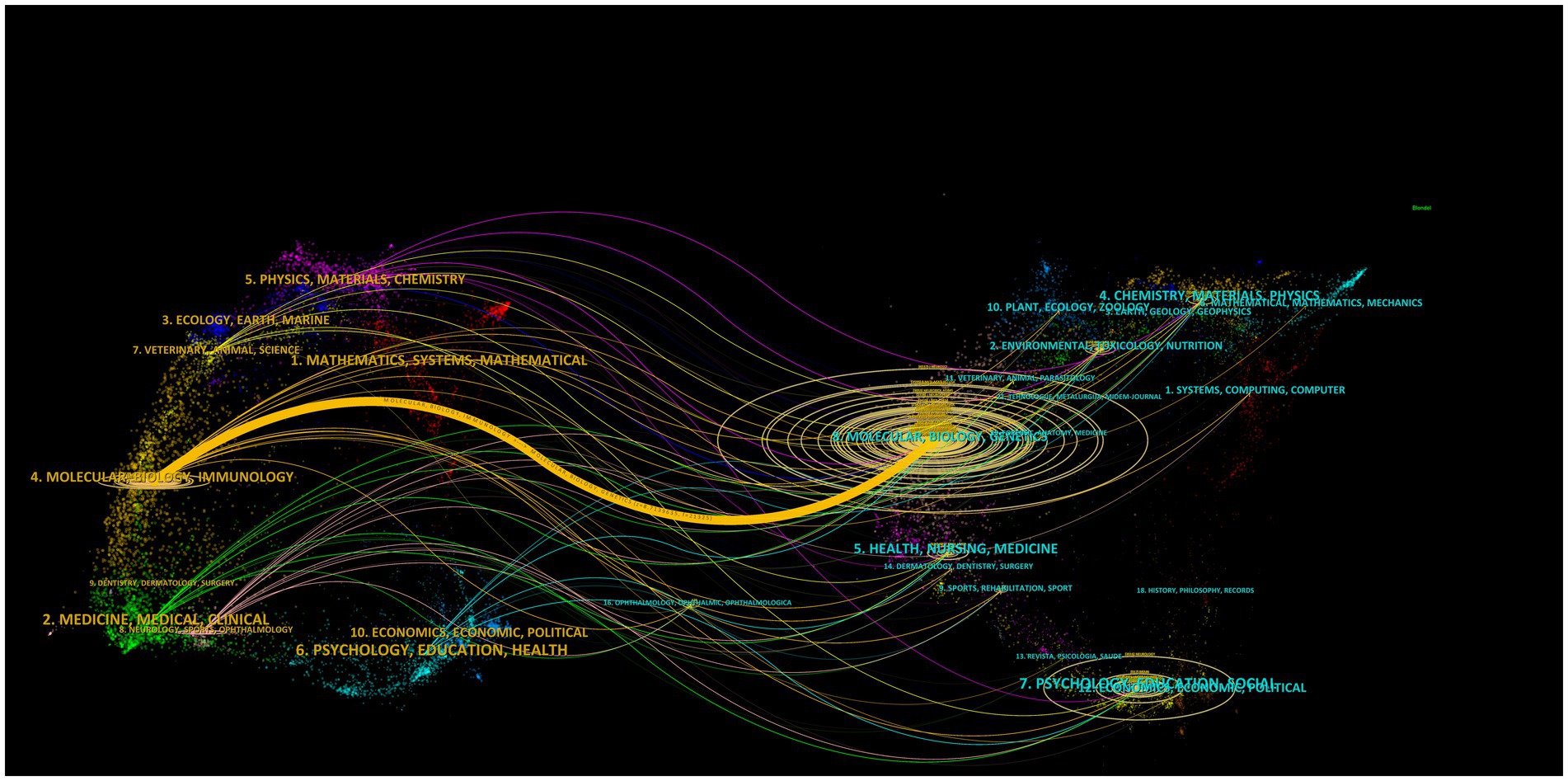
Figure 5. The dual-map overlay of the discipline distribution of publications. The left part represents citing journals, and the right part represents cited journals; the lines represent relationships between the citing and cited journals; the horizontal axis of the ellipse shows the number of authors, while the vertical axis shows the number of published articles.
In total, 8,891 keywords were recognized from previous publications, 285 of which occurred at least 15 times. The co-occurrence of these 285 keywords is shown in Figure 6A. Of all keywords, the most published and linked meaningful keywords included “astrocytes,” “brain,” “oxidative stress,” “neuroinflammation,” “microglia,” “inflammation,” “neurodegeneration,” “central nervous system,” “hippocampus,” and “mouse model.” As shown in Figure 6B, the number of publications focused on the association between GFAP and “aging,” “oxidative stress,” “inflammation,” “microglia,” “hippocampus,” and “amyloid” within the area of AD annually increased in general, and “immunohistochemistry” was applied more for this area. Moreover, “inflammation,” “cognitive impairment,” “neuroinflammation,” “tau,” and “dysfunction” were the hot associated keywords in the past 5 years (Figure 6C).
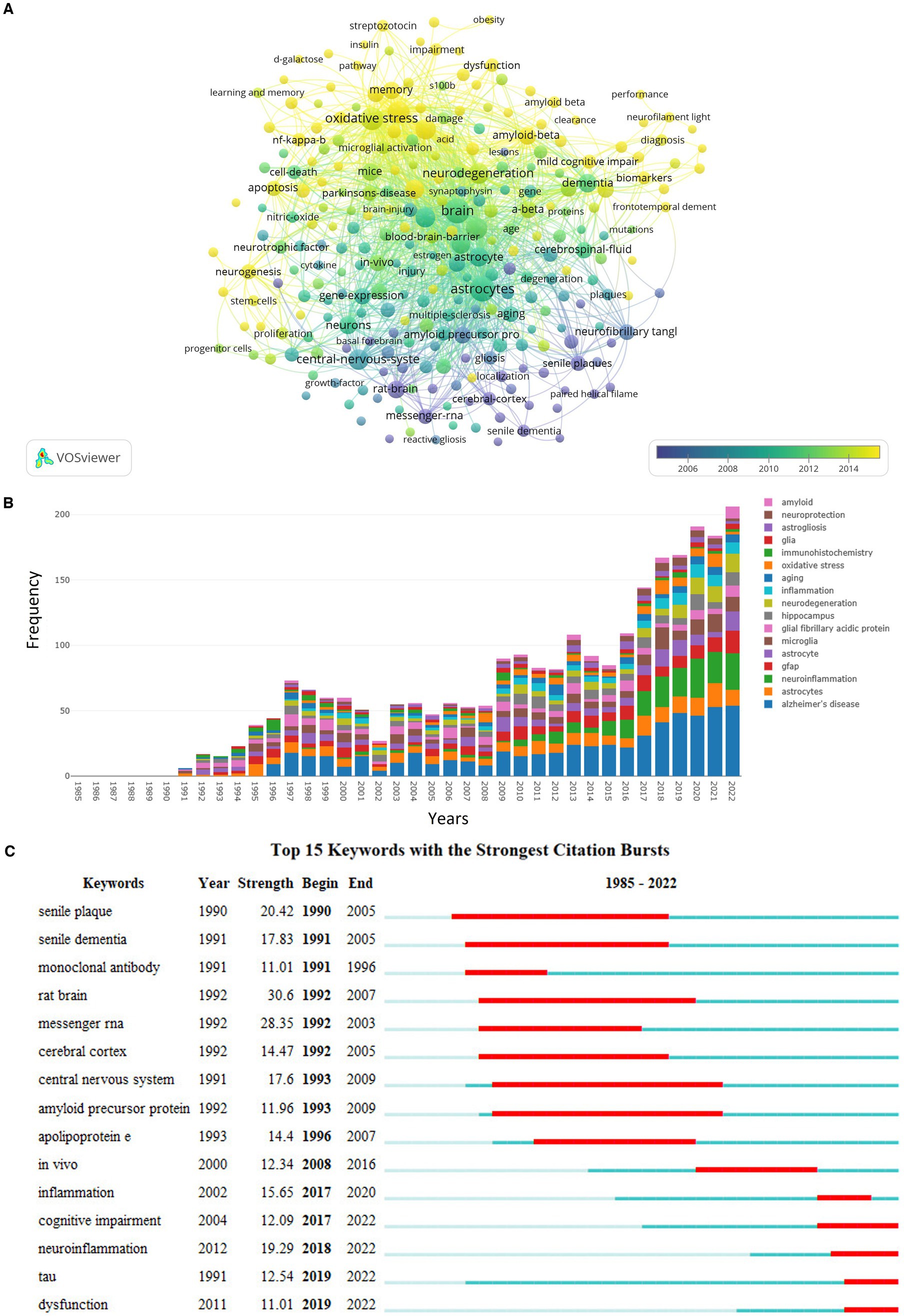
Figure 6. Summary of keywords. (A) Relationship among 285 keywords with a minimum number of occurrences equal to 15; the node size is based on the number of articles, and the total link strength is based on the power of cooperation between two nodes; (B) the annual frequency of top prolific keywords; (C) the top 15 keywords with the strongest citation bursts.
4. Discussion
To analyze the research trends and hotspots on GFAP within the area of AD, we conducted a bibliometric analysis and eventually included 2,269 articles and review publications. To our knowledge, this is the first bibliometric analysis to explore the research on GFAP and AD. Despite some minor fluctuations, the general trend for the number of publications was upward, especially from 2014, when the upward trend became successive and remarkable. Around this time, the diagnostic performance of GFAP in CSF and blood was explored based on biochip array technology (Rosén et al., 2011). Then, the most cited publication (Olsson et al., 2016) further evaluated the diagnostic performance of many CSF and blood biomarkers for AD by conducting a meta-analysis, including GFAP in CSF, which implied the importance of GFAP for the diagnosis of AD. However, related articles about GFAP in CSF for the diagnosis of AD were still limited at that time, and only two articles were included in the meta-analysis; thus, a null conclusion was reported. Moreover, the application of GFAP in serum/plasma in AD had not been comprehensively recognized at that time, thus this review did not include it. During the past few years, the diagnostic value of GFAP in body fluids, especially in blood, was further explored and it could be used for large-scale screening (Guo et al., 2023). Although astrocyte activation is not specific to AD pathophysiology, plasma GFAP was found to be more sensitive for the early diagnosis of AD than Aβ-PET, CSF and plasma p-tau181, and CSF total tau (Guo et al., 2023), and it linearly increased with increasing Aβ-PET burden (Asken et al., 2020). Blood GFAP levels can predict not only AD development in patients with mild cognitive impairment but also cognitive decline in cognitively unimpaired subjects (Verberk et al., 2020; Cicognola et al., 2021; Verberk et al., 2021). At the same time, more sensitive technology, such as ultrasensitive single-molecule array technology, was also developed (Rissin et al., 2010) and applied for the accurate measurement of blood GFAP (Bogoslovsky et al., 2017), which prompted its application. Under these circumstances, the diagnostic and prognostic performance of GFAP in CSF and serum/plasma for AD should be further evaluated and verified with better analytical assays in larger-scale cohorts, especially for different age groups and clinical contexts (Hansson et al., 2022). Moreover, new systematic reviews and meta-analyses based on more publications, which are currently lacking, need to be conducted.
Interestingly, it is reported that the association of plasma GFAP with Aβ pathology appears stronger than that of CSF GFAP, which is different from other glial biomarkers (Pereira et al., 2021). A recent study found that plasma GFAP is more stable than CSF GFAP, without the effect of freeze–thaw cycles, making it a better biomarker for clinical application (Simrén et al., 2022). However, the reason why blood GFAP showed a stronger association with Aβ pathology is still unclear; thus, further work is still needed to investigate the mechanisms of GFAP release into the bloodstream under pathological conditions and clarify the key pathological substrates of the better separation of serum/plasma versus CSF in AD (Hansson et al., 2022). Moreover, even if the Alzheimer’s Association has recommended plasma GFAP as a promising and available biomarker since 2022 (Hansson et al., 2022; Teunissen et al., 2022), many problems still need to be solved, such as preanalytical confounders and biological factors. It was found that sample types with different anticoagulants could affect the concentration of blood GFAP, but centrifugation delay within 24 h, storage delay within 2 w at −20°C, centrifugation temperature and freeze–thaw cycle did not significantly affect plasma GFAP (Verberk et al., 2022). Additionally, significant differences were found for many AD-related biomarkers, including GFAP, between the postprandial group and fasting group, implying that the concentration of these biomarkers could be affected by food intake (Huber et al., 2023). Moreover, the concentration of blood GFAP is usually simultaneously measured with other biomarkers, such as amyloid and tau; thus, a standardized preanalytical process including sample drawing, treatment, and storage is still necessary (Verberk et al., 2022). Furthermore, it is important to evaluate the effects of clinical confounders and biological factors on the clinical diagnostic and prognostic performance of GFAP for AD (Hansson et al., 2022). Previous studies preliminarily found that impaired kidney function could affect the concentration of amyloid 1–42, p-tau181, and NfL but not plasma GFAP (Sedaghat et al., 2023; Zhang et al., 2023); however, more comprehensive studies with larger cohorts are still needed to explore the effect of more factors, such as peripheral neuropathies and body mass index, on blood GFAP for more accurate laboratory measurements and clinical interpretation (Hansson et al., 2022).
By this bibliometric analysis, it was also found that the USA, China, England, Japan, Spain, Germany, and Sweden contributed the most research on GFAP within the area of AD. The USA always took the lead in this area, but publications from China and England increased annually. As the top populous country with a rapidly increasing aging population, China has an increasing number of patients diagnosed with AD (Jia et al., 2020), and GFAP in CSF and serum/plasma has become a research hotspot, showing promising early diagnosis and prognosis value for AD. Of the top 10 prolific and highly cited institutions, three institutions are from the USA, and another three institutions are from Sweden, of which the University of Gothenburg contributed most publications in this area. Moreover, Henrik Zetterberg and Kaj Blennow, both professors at the University of Gothenburg, contributed the most prolific publications. As key leaders of the UK Dementia Research Institute and the Alzheimer’s Association, respectively, Henrik Zetterberg and Kaj Blennow focused on the fluid biomarkers of neurodegenerative diseases and made remarkable contributions in this area. Moreover, the Journal of Alzheimer’s Disease published most articles and reviews in this area, and the publications of Neurobiology of Aging had the highest average citations.
The discipline distribution analysis of publications revealed that “molecular, biology, genetics” and “molecular, biology, immunology” were the key research areas of 21 domain disciplines, and the published articles on “molecular, biology, immunology” were based on those on “molecular, biology, genetics,” which implied that “molecular, biology, genetics” had provided a foundation for this research area. Furthermore, the most published and hottest meaningful associated keywords included “oxidative stress,” “neuroinflammation” or “inflammation,” “microglia,” “neurodegeneration,” “hippocampus,” “mouse model,” “amyloid,” “immunohistochemistry,” of which “inflammation,” “cognitive impairment,” “neuroinflammation,” “tau,” and “dysfunction” were the hot associated keywords in the past 5 years. Because it is difficult to directly intervene in the human body and obtain human brain tissues, animal models especially mouse models are helpful and necessary for associated immunology and genetics studies (Qin et al., 2020), and immunohistochemistry remains the gold-standard technique for capturing the spatial expression patterns of astrocytes in postmortem tissue sections (Viejo et al., 2022). Since astrocytes and microglia could release proinflammatory cytokines and reactive oxygen, triggering neuroinflammation and oxidative stress (Novoa et al., 2022), it is not surprising that “inflammation,” “neuroinflammation,” and “oxidative stress” were hot keywords related to GFAP, which is an important biomarker for astrocyte activation. Moreover, the inflammatory response, oxidative stress, and glial activation (especially microglia and astrocytes) have been found as three main factors for AD progression based on both mouse models and human post-mortem brains (Companys-Alemany et al., 2022; Viejo et al., 2022). Furthermore, Aβ and tau are found able to activate astrocytes and microglia, and GFAP expression and protein concentrations were higher in areas surrounding dense Aβ plaques and increased with tau accumulation, implying the association of GFAP with amyloid and tau pathology (Simpson et al., 2010; Novoa et al., 2022). However, the results based on clinical individuals only showed a significant association between higher CSF and plasma GFAP levels and elevated Aβ-PET load but not for tau-PET load (Pereira et al., 2021; Ferrari-Souza et al., 2022). Moreover, it was found that glial biomarkers including GFAP increased with aging regardless of Aβ status and also in other neurological diseases besides AD (Milà-Alomà et al., 2020). Thus, the mechanisms of GFAP in the development of AD are complex due to the multiple functions of astrocytes, and more mechanism studies are still needed to clarify the specific causal relationship between GFAP and AD. Noticeably, all findings based on animal models need to be further verified based on human cohorts to ensure the clinical application.
In summary, the effects of GFAP on the occurrence, development, and prognosis of AD still need to be further researched, and our analysis could provide some guidance on further research trends. It should be emphasized that the GFAP change is not specific for AD but for many neurodegenerative diseases. Thus, GFAP in body fluid could only be used for the first screening but not a final diagnosis.
Author contributions
YZ: methodology, data curation, and writing – original draft preparation. LL, LG, and CCM: methodology and data curation. SY, XM, CHM, and JG: writing – reviewing and editing. LQ: conceptualization and methodology. All authors contributed to the article and approved the submitted version.
Funding
This work was funded by research grants from the National High-Level Hospital Clinical Research Funding (No. 2022-PUMCH-B-073), the National Key Research and Development Program of China (No.2020YFA0804500/2020YFA0804501), and the National Natural Science Foundation of China (82202651).
Acknowledgments
Thanks to Elsevier for the language editing service.
Conflict of interest
The authors declare that the research was conducted in the absence of any commercial or financial relationships that could be construed as a potential conflict of interest.
Publisher’s note
All claims expressed in this article are solely those of the authors and do not necessarily represent those of their affiliated organizations, or those of the publisher, the editors and the reviewers. Any product that may be evaluated in this article, or claim that may be made by its manufacturer, is not guaranteed or endorsed by the publisher.
Footnotes
References
Asken, B. M., Elahi, F. M., La Joie, R., Strom, A., Staffaroni, A. M., Lindbergh, C. A., et al. (2020). Plasma glial fibrillary acidic protein levels differ along the spectra of amyloid burden and clinical disease stage. J. Alzheimers Dis. 78, 265–276. doi: 10.3233/JAD-200755
Bogoslovsky, T., Wilson, D., Chen, Y., Hanlon, D., Gill, J., Jeromin, A., et al. (2017). Increases of plasma levels of glial fibrillary acidic protein, tau, and amyloid β up to 90 days after traumatic brain injury. J. Neurotrauma 34, 66–73. doi: 10.1089/neu.2015.4333
Cicognola, C., Janelidze, S., Hertze, J., Zetterberg, H., Blennow, K., Mattsson-Carlgren, N., et al. (2021). Plasma glial fibrillary acidic protein detects Alzheimer pathology and predicts future conversion to Alzheimer dementia in patients with mild cognitive impairment. Alzheimers Res. Ther. 13:68. doi: 10.1186/s13195-021-00804-9
Companys-Alemany, J., Turcu, A. L., Vázquez, S., Pallàs, M., and Griñán-Ferré, C. (2022). Glial cell reactivity and oxidative stress prevention in Alzheimer’s disease mice model by an optimized NMDA receptor antagonist. Sci. Rep. 12:17908. doi: 10.1038/s41598-022-22963-x
Ferrari-Souza, J. P., Ferreira, P. C. L., Bellaver, B., Tissot, C., Wang, Y. T., Leffa, D. T., et al. (2022). Astrocyte biomarker signatures of amyloid-β and tau pathologies in Alzheimer’s disease. Mol. Psychiatry 27, 4781–4789. doi: 10.1038/s41380-022-01716-2
Frost, G. R., and Li, Y. M. (2017). The role of astrocytes in amyloid production and Alzheimer’s disease. Open. Biol. 7:170228. doi: 10.1098/rsob.170228
Ganne, A., Balasubramaniam, M., Griffin, W. S. T., Shmookler Reis, R. J., and Ayyadevara, S. (2022). Glial fibrillary acidic protein: a biomarker and drug target for Alzheimer’s disease. Pharmaceutics 14:1354. doi: 10.3390/pharmaceutics14071354
Guo, Y., Shen, X. N., Wang, H. F., Chen, S. D., Zhang, Y. R., Chen, S. F., et al. (2023). The dynamics of plasma biomarkers across the Alzheimer’s continuum. Alzheimers Res. Ther. 15:31. doi: 10.1186/s13195-023-01174-0
Hansson, O., Edelmayer, R. M., Boxer, A. L., Carrillo, M. C., Mielke, M. M., Rabinovici, G. D., et al. (2022). The Alzheimer’s Association appropriate use recommendations for blood biomarkers in Alzheimer’s disease. Alzheimers Dement. 18, 2669–2686. doi: 10.1002/alz.12756
Heimfarth, L., Passos, F. R. S., Monteiro, B. S., Araújo, A. A. S., Quintans Júnior, L. J., and Quintans, J. S. S. (2022). Serum glial fibrillary acidic protein is a body fluid biomarker: a valuable prognostic for neurological disease - a systematic review. Int. Immunopharmacol. 107:108624. doi: 10.1016/j.intimp.2022.108624
Hol, E. M., and Pekny, M. (2015). Glial fibrillary acidic protein (GFAP) and the astrocyte intermediate filament system in diseases of the central nervous system. Curr Opin Cell Biol. 32, 121–130. doi: 10.1016/j.ceb.2015.02.004
Huber, H., Ashton, N. J., Schieren, A., Montoliu-Gaya, L., Molfetta, G. D., Brum, W. S., et al. (2023). Levels of Alzheimer’s disease blood biomarkers are altered after food intake-a pilot intervention study in healthy adults. Alzheimers Dement. doi: 10.1002/alz.13163
Jia, L., Du, Y., Chu, L., Zhang, Z., Li, F., Lyu, D., et al. (2020). Prevalence, risk factors, and management of dementia and mild cognitive impairment in adults aged 60 years or older in China: a cross-sectional study. Lancet Public Health 5, e661–e671. doi: 10.1016/S2468-2667(20)30185-7
Johnson, S. C., Suárez-Calvet, M., Suridjan, I., Minguillón, C., Gispert, J. D., Jonaitis, E., et al. (2023). Identifying clinically useful biomarkers in neurodegenerative disease through a collaborative approach: the neuro ToolKit. Alzheimers Res. Ther. 15:25. doi: 10.1186/s13195-023-01168-y
Kamat, P. K., Kyles, P., Kalani, A., and Tyagi, N. (2016). Hydrogen Sulfide Ameliorates Homocysteine-Induced Alzheimer’s Disease-Like Pathology, Blood-Brain Barrier Disruption, and Synaptic Disorder. Mol. Neurobiol. 53, 2451–2467. doi: 10.1007/s12035-015-9212-4
Larson, E. B., Shadlen, M. F., Wang, L., McCormick, W. C., Bowen, J. D., Teri, L., et al. (2004). Survival after initial diagnosis of Alzheimer disease. Ann. Intern. Med. 140, 501–509. doi: 10.7326/0003-4819-140-7-200404060-00008
Milà-Alomà, M., Salvadó, G., Gispert, J. D., Vilor-Tejedor, N., Grau-Rivera, O., Sala-Vila, A., et al. (2020). Amyloid beta, tau, synaptic, neurodegeneration, and glial biomarkers in the preclinical stage of the Alzheimer’s continuum. Alzheimers Dement. 16, 1358–1371. doi: 10.1002/alz.12131
Muhammad, T., Ikram, M., Ullah, R., Rehman, S. U., and Kim, M. O. (2019). Hesperetin, a Citrus Flavonoid, Attenuates LPS-Induced Neuroinflammation, Apoptosis and Memory Impairments by Modulating TLR4/NF-κB Signaling. Nutrients. 11:648. doi: 10.3390/nu11030648
Novoa, C., Salazar, P., Cisternas, P., Gherardelli, C., Vera-Salazar, R., Zolezzi, J. M., et al. (2022). Inflammation context in Alzheimer’s disease, a relationship intricate to define. Biol. Res. 55:39. doi: 10.1186/s40659-022-00404-3
Olsson, B., Lautner, R., Andreasson, U., Öhrfelt, A., Portelius, E., Bjerke, M., et al. (2016). CSF and blood biomarkers for the diagnosis of Alzheimer’s disease: a systematic review and meta-analysis. Lancet Neurol. 15, 673–684. doi: 10.1016/S1474-4422(16)00070-3
Parpura, V., Heneka, M. T., Montana, V., Oliet, S. H., Schousboe, A., Haydon, P. G., et al., (2012). Glial cells in (patho)physiology. J. Neurochem. 121, 4–27. doi: 10.1111/j.1471-4159.2012.07664.x
Pekny, M., Wilhelmsson, U., and Pekna, M. (2014). The dual role of astrocyte activation and reactive gliosis. Neurosci. Lett. 565, 30–38. doi: 10.1016/j.neulet.2013.12.071
Pereira, J. B., Janelidze, S., Smith, R., Mattsson-Carlgren, N., Palmqvist, S., Teunissen, C. E., et al. (2021). Plasma GFAP is an early marker of amyloid-β but not tau pathology in Alzheimer’s disease. Brain 144, 3505–3516. doi: 10.1093/brain/awab223
Prince, M., Bryce, R., Albanese, E., Wimo, A., Ribeiro, W., and Ferri, C. P. (2013). The global prevalence of dementia: a systematic review and metaanalysis. Alzheimers Dement. 9, 63–75.e2. doi: 10.1016/j.jalz.2012.11.007
Qin, T., Prins, S., Groeneveld, G. J., Van Westen, G., de Vries, H. E., Wong, Y. C., et al. (2020). Utility of animal models to understand human Alzheimer’s disease, using the mastermind research approach to avoid unnecessary further sacrifices of animals. Int. J. Mol. Sci. 21:3158. doi: 10.3390/ijms21093158
Rissin, D. M., Kan, C. W., Campbell, T. G., Howes, S. C., Fournier, D. R., Song, L., et al. (2010). Single-molecule enzyme-linked immunosorbent assay detects serum proteins at subfemtomolar concentrations. Nat. Biotechnol. 28, 595–599. doi: 10.1038/nbt.1641
Rosén, C., Mattsson, N., Johansson, P. M., Andreasson, U., Wallin, A., Hansson, O., et al. (2011). Discriminatory analysis of biochip-derived protein patterns in CSF and plasma in neurodegenerative diseases. Front. Aging Neurosci. 3:1.
Sedaghat, S., Ji, Y., Hughes, T. M., Coresh, J., Grams, M. E., Folsom, A. R., et al. (2023). The Association of Kidney Function with plasma amyloid-β levels and brain amyloid deposition. J. Alzheimers Dis. 92, 229–239. doi: 10.3233/JAD-220765
Simpson, J. E., Ince, P. G., Lace, G., Forster, G., Shaw, P. J., Matthews, F., et al. (2010). Astrocyte phenotype in relation to Alzheimer-type pathology in the ageing brain. Neurobiol. Aging 31, 578–590. doi: 10.1016/j.neurobiolaging.2008.05.015
Simrén, J., Weninger, H., Brum, W. S., Khalil, S., Benedet, A. L., Blennow, K., et al. (2022). Differences between blood and cerebrospinal fluid glial fibrillary acidic protein levels: the effect of sample stability. Alzheimers Dement. 18, 1988–1992. doi: 10.1002/alz.12806
Teunissen, C. E., Verberk, I. M. W., Thijssen, E. H., Vermunt, L., Hansson, O., Zetterberg, H., et al. (2022). Blood-based biomarkers for Alzheimer’s disease: towards clinical implementation. Lancet Neurol. 21, 66–77. doi: 10.1016/S1474-4422(21)00361-6
Uddin, M. S., Stachowiak, A., Mamun, A. A., Tzvetkov, N. T., Takeda, S., Atanasov, A. G., et al. (2018). Autophagy and Alzheimer’s Disease: From Molecular Mechanisms to Therapeutic Implications. Front. Aging Neurosci. 10:4. doi: 10.3389/fnagi.2018.00004
Verberk, I. M. W., Laarhuis, M. B., van den Bosch, K. A., Ebenau, J. L., van Leeuwenstijn, M., Prins, N. D., et al. (2021). Serum markers glial fibrillary acidic protein and neurofilament light for prognosis and monitoring in cognitively normal older people: a prospective memory clinic-based cohort study. Lancet Healthy Longev 2, e87–e95. doi: 10.1016/S2666-7568(20)30061-1
Verberk, I. M. W., Misdorp, E. O., Koelewijn, J., Ball, A. J., Blennow, K., Dage, J. L., et al. (2022). Characterization of pre-analytical sample handling effects on a panel of Alzheimer’s disease-related blood-based biomarkers: results from the standardization of Alzheimer’s blood biomarkers (SABB) working group. Alzheimers Dement. 18, 1484–1497. doi: 10.1002/alz.12510
Verberk, I. M. W., Thijssen, E., Koelewijn, J., Mauroo, K., Vanbrabant, J., de Wilde, A., et al. (2020). Combination of plasma amyloid beta((1-42/1-40)) and glial fibrillary acidic protein strongly associates with cerebral amyloid pathology. Alzheimers Res. Ther. 12:118. doi: 10.1186/s13195-020-00682-7
Viejo, L., Noori, A., Merrill, E., Das, S., Hyman, B. T., and Serrano-Pozo, A. (2022). Systematic review of human post-mortem immunohistochemical studies and bioinformatics analyses unveil the complexity of astrocyte reaction in Alzheimer’s disease. Neuropathol. Appl. Neurobiol. 48:e12753. doi: 10.1111/nan.12753
Wang, K. K., Yang, Z., Zhu, T., Shi, Y., Rubenstein, R., Tyndall, J. A., et al. (2018). An update on diagnostic and prognostic biomarkers for traumatic brain injury. Expert Rev. Mol. Diagn. 18, 165–180. doi: 10.1080/14737159.2018.1428089
Zeppenfeld, D. M., Simon, M., Haswell, J. D., D’Abreo, D., Murchison, C., Quinn, J. F., et al. (2017). Association of Perivascular Localization of Aquaporin-4 With Cognition and Alzheimer Disease in Aging Brains. JAMA Neurol. 74, 91–99. doi: 10.1001/jamaneurol.2016.4370
Zhang, B., Zhang, C., Wang, Y., Chen, L., Qiao, Y., Wang, Y., et al. (2023). Effect of renal function on the diagnostic performance of plasma biomarkers for Alzheimer’s disease. Front. Aging Neurosci. 15:1150510. doi: 10.3389/fnagi.2023.1150510
Keywords: Alzheimer’s disease, glial fibrillary acidic protein, bibliometric approach, research trend analysis, body fluids
Citation: Zou Y, Li L, Guan L, Ma C, Yu S, Ma X, Mao C, Gao J and Qiu L (2023) Research trends and hotspots of glial fibrillary acidic protein within the area of Alzheimer’s disease: a bibliometric analysis. Front. Aging Neurosci. 15:1196272. doi: 10.3389/fnagi.2023.1196272
Edited by:
Allison B. Reiss, New York University, United StatesReviewed by:
Manisha Thaker, Scintillon Institute, United StatesParnetti Lucilla, University of Perugia, Italy
Copyright © 2023 Zou, Li, Guan, Ma, Yu, Ma, Mao, Gao and Qiu. This is an open-access article distributed under the terms of the Creative Commons Attribution License (CC BY). The use, distribution or reproduction in other forums is permitted, provided the original author(s) and the copyright owner(s) are credited and that the original publication in this journal is cited, in accordance with accepted academic practice. No use, distribution or reproduction is permitted which does not comply with these terms.
*Correspondence: Ling Qiu, lingqiubj@163.com