- Department of Psychiatry, University of California, San Diego, San Diego, CA, United States
People with HIV (PWH) continue to suffer from dysfunction of the central nervous system, as evidenced by HIV-associated neurocognitive disorder (HAND), despite antiretroviral therapy and suppressed viral loads. As PWH live longer they may also be at risk of age-related neurodegenerative diseases such Alzheimer’s disease (AD) and its precursor, amnestic mild cognitive impairment (aMCI). The complement system is associated with deposition of AD-related proteins such as beta amyloid (Aβ), neuroinflammation, and neurological dysfunction in PWH. Complement component 3 (C3) is a key protagonist in the complement cascade and complement factor H (CFH) is an antagonist of C3 activity. We investigated the relationship between C3 and CFH levels in the brain and Aβ plaques and neurological dysfunction in 22 PWH. We analyzed by immunoblot C3 and CFH protein levels in frontal cortex (FC) and cerebellum (CB) brain specimens from PWH previously characterized for Aβ plaque deposition. C3 and CFH protein levels were then correlated with specific cognitive domains. C3 protein levels in the FC were significantly increased in brains with Aβ plaques and in brains with HAND compared to controls. In the CB, C3 levels trended higher in brains with Aβ plaques. Overall C3 protein levels were significantly higher in the FC compared to the CB, but the opposite was true for CFH, having significantly higher levels of CFH protein in the CB compared to the FC. However, only CFH in the FC showed significant correlations with specific domains, executive function and motor performance. These findings corroborate previous results showing that complement system proteins are associated with HAND and AD neuropathogenesis.
Introduction
Central nervous system dysfunction remains a major problem for people with HIV (PWH) even in the presence of antiretroviral therapy (ART) (Cysique et al., 2021). HIV-associated neurocognitive disorders (HAND) persist in approximately 43% of PWH (Heaton et al., 2011; Wang et al., 2020). The developments in ART have led to increased longevity among PWH so that the population of PWH in the United States is now predominantly over the age of 50. However, this translates to increased susceptibility to age-related neuropathogenesis such as Alzheimer’s disease (AD) and its precursor, amnestic mild cognitive impairment (aMCI) (Althoff et al., 2022). Indeed, multiple studies from independent labs have implicated age-related proteinopathies in neuropathogenesis in PWH (Achim et al., 2009; Gisslen et al., 2009, 2019; Chen et al., 2014; Fields et al., 2018, 2020; Murray et al., 2022). Consequently, there is a need to better understand mechanisms of age-related neuropathogenesis in PWH.
Interest in the role of innate immune function in the brain has mounted as multiple studies have implicated a prolonged innate immune response as a potential etiology of neurodegenerative diseases (Heneka et al., 2014, 2015; Kim et al., 2021). The complement system is integral to innate immunity and it can be activated early on during immune responses (Merle et al., 2015a,b; Morgan, 2015). When controlled, the complement system plays a protective and even developmental role in the brain. However, there is ample evidence that, when dysregulated, the complement system may contribute to the pathogenesis of neurodegenerative diseases (Liddelow et al., 2017) such as HAND (Bryant et al., 2016; Nitkiewicz et al., 2017) and AD (Goetzl et al., 2018; Morgan, 2018).
Complement component 3 (C3) is a central player in the activation of both the classical and alternative activation pathways (Merle et al., 2015a,b). Complement factor H (CFH) acts as a negative regulator of C3, blocking the activation of the complement system on targeted cells that are meant to be protected from complement activity (Merle et al., 2015a,b). Recent studies have suggested that either the blocking of C3 or the augmentation of CFH may serve as a therapeutic strategy in neurodegenerative diseases (Li et al., 2012; Lukiw and Alexandrov, 2012; Lukiw et al., 2012; Hoh Kam et al., 2013). However, other studies show that C3 mediates clearance of Aβ plaques (Wyss-Coray et al., 2002; Maier et al., 2008). Clinically, low CSF C3 and CFH has been associated with worsening cognitive decline in MCI (Toledo et al., 2014; Hu et al., 2016). CFH genetic variants as well as expression of miRNA that target expression of CFH have been associated with increased risk for AD (Li et al., 2012; Lukiw and Alexandrov, 2012; Lukiw et al., 2012; Zhang et al., 2016). Thus the interplay between C3 and CFH may contribute to the neuropathogenesis of aging and age-related diseases in PWH.
Researchers from our group previously examined associations between plasma inflammatory biomarkers (e.g., C3, cystatin C, interleukin 6, soluble CD163, and soluble CD14) and metabolic syndrome (MetS) in 79 virally suppressed, older PWH compared to 47 human immunodeficiency virus (HIV)-seronegative controls (Bryant et al., 2016). Among PWH, higher C3 levels were significantly associated with MetS in addition to the individual MetS components of obesity, type II diabetes, dyslipidemia and hypertension. C3 levels were significantly higher in PWH with MetS vs. PWH without MetS, whereas no associations between C3 levels and MetS were observed among HIV-seronegative controls. Other studies show that HIV induces C3 expression in the brain (Nitkiewicz et al., 2017). Furthermore, C3 expression is associated with mitochondrial dysfunction in AD (Sekar et al., 2015), representing another link to the neuropathogenesis of AD. These findings suggest that C3 may be a marker of inflammatory processes that contribute to metabolic risk and, as examined in this proposal, brain function and AD risk. This may be particularly important because mitochondrial dysfunction is implicated in HAND and AD neuropathogenesis.
Aβ deposition is altered in the brains of PWH and may be associated with premature aging in this population. HIV infection of the brain is associated with increased levels of Aβ (Giometto et al., 1997; Esiri et al., 1998; Green et al., 2005), including intraneuronal Aβ (Achim et al., 2009) and other AD-related biomarkers including increased phospho-tau (ptau) (Brew et al., 2005; Clifford et al., 2009), and inflammatory cytokines, such as tumor necrosis factor (TNF)-α and interleukin (IL)-1β (Ortega and Ances, 2014; Gelman, 2015; Levine et al., 2016; Fields et al., 2018). In a study of frontal cortex (FC) samples from PWH, Aβ plaques were detected in 29% of 279 cases (Umlauf et al., 2019). To date, numerous studies have provided evidence of AD-related neuropathogenesis in HIV (Chen et al., 2014; Fields et al., 2018; Gisslen et al., 2019; Chemparthy et al., 2021; Murray et al., 2022), and thus, there is a need to better understand the common neuropathogenic mechanisms in AD and HAND.
In this study we utilized the post-mortem tissue repository, National NeuroAIDS Tissue Consortium (NNTC), to investigate C3 and CFH levels in the FC and cerebellum in older HIV + cases previously characterized for AD-associated neuropathology. We next examined how C3 and CFH levels relate to aMCI and HAND classification and performance on specific cognitive domains based on an antemortem cognitive evaluation within 18 months of death.
Materials and methods
Study population
For the present study, we evaluated brain tissues from a total of 22 older (at-least 50 years of age at death) HIV + donors from the NNTC [Institutional Review Board (IRB) #080323]. All studies adhered to the ethical guidelines of the National Institutes of Health and the University of California, San Diego. These cases had neuromedical and neuropsychological examinations within a median of 12 months before death. Subjects were excluded if they had a history of CNS opportunistic infections or non-HIV-related developmental, neurologic, psychiatric, or metabolic conditions that might affect CNS functioning (e.g., loss of consciousness exceeding 30 min, psychosis, etc.).
Neuromedical and neuropsychological testing
Participants underwent a comprehensive neuromedical evaluation that included assessment of medical history, structured medical and neurological examinations, and the collection of blood, cerebrospinal fluid (CSF), and urine samples, as previously described (Woods et al., 2004; Heaton et al., 2010). Clinical data [plasma viral load (VL), postmortem interval, CD4 count] were collected for the donor cohorts. A neuropsychological battery assessed seven cognitive domains commonly affected by HIV: verbal fluency, working memory, processing speed, episodic memory for verbal and visual stimuli, executive function, and complex motor function. Specific tests are described elsewhere (Cysique et al., 2011). Raw test scores were transformed into demographically adjusted T-scores, including adjustments for age, education, gender and race, based on normative samples of HIV-participants (Norman et al., 2011). As part of the neuropsychological battery, participants also completed self-report questionnaires of everyday functioning (i.e., Lawton and Brody Activities of Daily Living questionnaire (Lawton and Brody, 1969), and/or Patient’s Assessment of Own Functioning; PAOFI (Chelune and Baer, 1986; Chelune et al., 1986).
HIV-associated neurocognitive disorder classification
Participant’s performance on the neuropsychological test battery and their responses to the everyday functioning questionnaires were utilized to assign HAND diagnoses following Frascati criteria (Antinori et al., 2007). A HAND diagnosis required impairment in at-least two cognitive domains, defined by performance of at-least 1.0 standard deviation (SD) below the demographically adjusted normative mean on neuropsychological tests. HAND status was further categorized as asymptomatic neurocognitive impairment (ANI; no interference in everyday function), mild neurocognitive disorder (MND; at-least mild interference in everyday function), and HIV-associated dementia (HAD; marked interference in everyday function).
Amnestic mild cognitive impairment classification
aMCI was classified using a version of the established, neuropsychological Jak/Bondi criteria (Bondi et al., 2014) that was previously adapted for the use of detecting aMCI symptoms amid a background of HAND in PWH (Sundermann et al., 2021). The Jak/Bondi criteria for aMCI requires two impaired tests (i.e., >1 SD below demographically corrected mean) within the memory domain. In order to capitalize on the retention deficit that is unique to aMCI/AD rather than the retrieval deficit that is common to both aMCI/AD and HAND, the Jak/Bondi MCI criteria was adapted to require at least one of the two impaired memory tests be a recognition test. The memory outcomes used in these criteria were the demographically adjusted T-scores of the Hopkins Verbal Learning Test-Revised (HVLT-R) and the Brief Visuospatial Memory Test-Revised (BVMT-R) (Norman et al., 2011) delayed recall and recognition subtests. Participants were classified as aMCI + if they showed impaired performance (T-score < 40) on at-least two of the four measures with at-least one of the impaired scores being a recognition measure. Of important note, the HAND classification criteria used in this study included BVMT-R and HVLT-R learning and delayed recall, but not recognition, scores to assess the learning and memory domain.
Immunoblot analysis of complement proteins
Further assessment of the expression levels of C3 and CFH in NNTC cases were performed by immunoblot analysis. Cerebellum and FC was homogenized in buffer (1.0 mmol/L HEPES (Gibco, cat. no. 15630–080), 5.0 mmol/L benzamidine, 2.0 mmol/L 2-mercaptoethanol (Gibco, cat. no. 21985), 3.0 mmol/L EDTA (Omni pur, cat. no. 4005), 0.5 mmol/L magnesium sulfate, 0.05% sodium azide; final pH 8.8) as described in a previous publication (Swinton et al., 2021). In brief, as previously described (Fields et al., 2013), tissues from brain samples (0.1 g) were homogenized in 0.7 ml of fractionation buffer containing phosphatase and protease inhibitor cocktails (Calbiochem, cat. nos. 524624 and 539131). Samples were precleared by centrifugation at 5000 × g for 5 min at room temperature. Supernatants were retained as the whole lysate and stored at –80 until use. As previously described (Swinton et al., 2021), after determination of the protein content of all samples by bicinchoninic acid assay (Thermo Fisher Scientific, cat. no. 23225) and denaturing in lamellae sample buffer (Bio Rad, cat. no. 1610747), whole lysates were loaded (15 μg total protein/lane) on 4–15% Criterion TGX stain free gels (Bio Rad, cat. no. 5678085) and electrophoresed in Tris/Glycine/SDS running buffer (Bio Rad, cat. no. 161–0772) and transferred onto LF PVDF membrane with Bio Rad transfer stacks and transfer buffer (Bio Rad, cat. no 1704275) using Bio Rad Trans Blot Turbo transfer system. After the transfer, total protein was imaged using Bio Rad ChemiDoc imager under the stain free blot setting for normalization purposes. Total protein transferred to the blot membrane, rather than the traditional use of housekeeping genes such as beta actin and glyceraldehyde phosphate dehydrogenase, was used to normalize protein expression because studies show that “housekeeping” genes are often altered in processes associated with neurodegenerative diseases (Kish et al., 1998; Mahmood et al., 2021). Therefore, our results are presented as the expression levels of the target gene compared total protein transferred to the blot membrane, a more accurate representation of protein expression in any given lysate. The membranes were then blocked in 1% casein in tris-buffered saline (TBS) (Bio Rad, cat. no. 1610782) for 1 h. Membranes were incubated overnight at 4°C with primary antibodies diluted in blocking buffer. All blots were then washed in PBST, and then incubated with species-specific IgG conjugated to HRP (American Qualex, cat. no. A102P5) diluted 1:5,000 in PBST and visualized with SuperSignal West Femto Maximum Sensitivity Substrate (ThermoFisher Scientific, cat. no. 34096). Images were obtained, and semi-quantitative analysis was performed with the ChemiDoc gel imaging system and Quantity One software (Bio-Rad).
Statistical analysis
We first examined and removed outliers in C3 and CFH protein levels as defined by levels greater than 3 SD from the sample mean. Statistical analysis was conducted using student t-test, one-way ANOVA, and effect size. Error bars represent standard error of the mean. Significance was set at a threshold of p < 0.05. We conducted a series of one-way ANOVAs to test mean differences in C3 and CFH protein expression levels in the FC and cerebellum by HAND classification and by aMCI classification. If there was a significant difference by HAND status, then we probed this difference by comparing protein expression levels among individual HAND types. We determined effect size of mean differences using Cohen’s d. We conducted a series of Spearman’s rank-order correlation, the non-parametric version of the Pearson product-moment correlation, to examine relationships between C3 and CFH expression levels in the FC and cerebellum and cognitive domain t-scores.
Results
One outlier for frontal and cerebellar CFH protein levels was identified and removed from analyses resulting in a final sample of N = 22 for C3 and N = 21 for CFH protein levels in the FC and N = 14 for C3 and N = 13 for CFH in the cerebellum. See Table 1 for sample characteristics of the largest sample (N = 22) by HAND and aMCI classification. Overall, age at death ranged from 50 to 66 (mean = 56.2, SD = 4.6) and the sample was 82% male and 73% Caucasian. Fifteen (68%) of the participants were classified as HAND with the following break-down: 7 (32%) ANI, 3 (14%) MND and 5 HAD (23%). Ten participants (45.5%) were classified as aMCI, with only one of these cases classified as non-HAND, suggesting that this case only exhibited memory impairment.
Complement component 3 protein is significantly upregulated in the frontal cortex of HIV brains with detectable beta amyloid plaques and in the frontal cortex from decedents with HIV-associated neurocognitive disorder
To determine the expression levels of C3 and CFH protein in frontal cortices of HIV + donors with and without AD-related pathology and HAND, we performed immunoblot of lysates stratified by presence of Aβ plaques (Umlauf et al., 2019; Figure 1A). Bands corresponding to C3 and CFH proteins were detected at approximately 75 and 150 kDa, respectively (Figure 1A). A second band at approximately 100 kDa is apparent in the CFH blot; however, this is not consistent with the predicted molecular weight of CFH and, to our knowledge this band has not been reported in the literature. Future studies may be necessary to determine the biological relevance of the 105 kDa band. When normalized to total protein transferred to the membrane, the average intensity of the band corresponding to C3 showed a significant (∼5-fold) increase in the Aβ + group compared to the Aβ- group (Figure 1B). Comparing C3 band intensity in CN vs. HAND, C3 was significantly higher in the HAND group as a whole compared to the CN group [F(1, 19) = 4.72, p = 0.04, Cohen’s d = 1.52; Figure 1C]. When stratified by HAND subgroups, the average intensity of the band corresponding to C3 was significantly higher (∼7-fold) in the ANI group compared to the CN group [F(1, 12) = 9.76, p = 0.009; Figure 1D]. There was no significant difference in C3 band intensity in aMCI- vs. aMCI + groups [F(1, 19) = 0.91, Cohen’s d = 0.43, p = 0.35; Figure 1E]. The average intensity of the band corresponding to CFH was not significantly different between the Aβ- and Aβ + groups (Figure 1F), the HAND and CN groups [F(1, 18) = 0.54, p = 0.47; Figures 1G,H], or the aMCI- and aMCI + groups [F(1, 18) = 0.69, Cohen’s d = 0.32, p = 0.42; Figure 1I]. We found no significant relationship between C3 or CFH levels in the FC and viral loads or CD4 + cell counts.
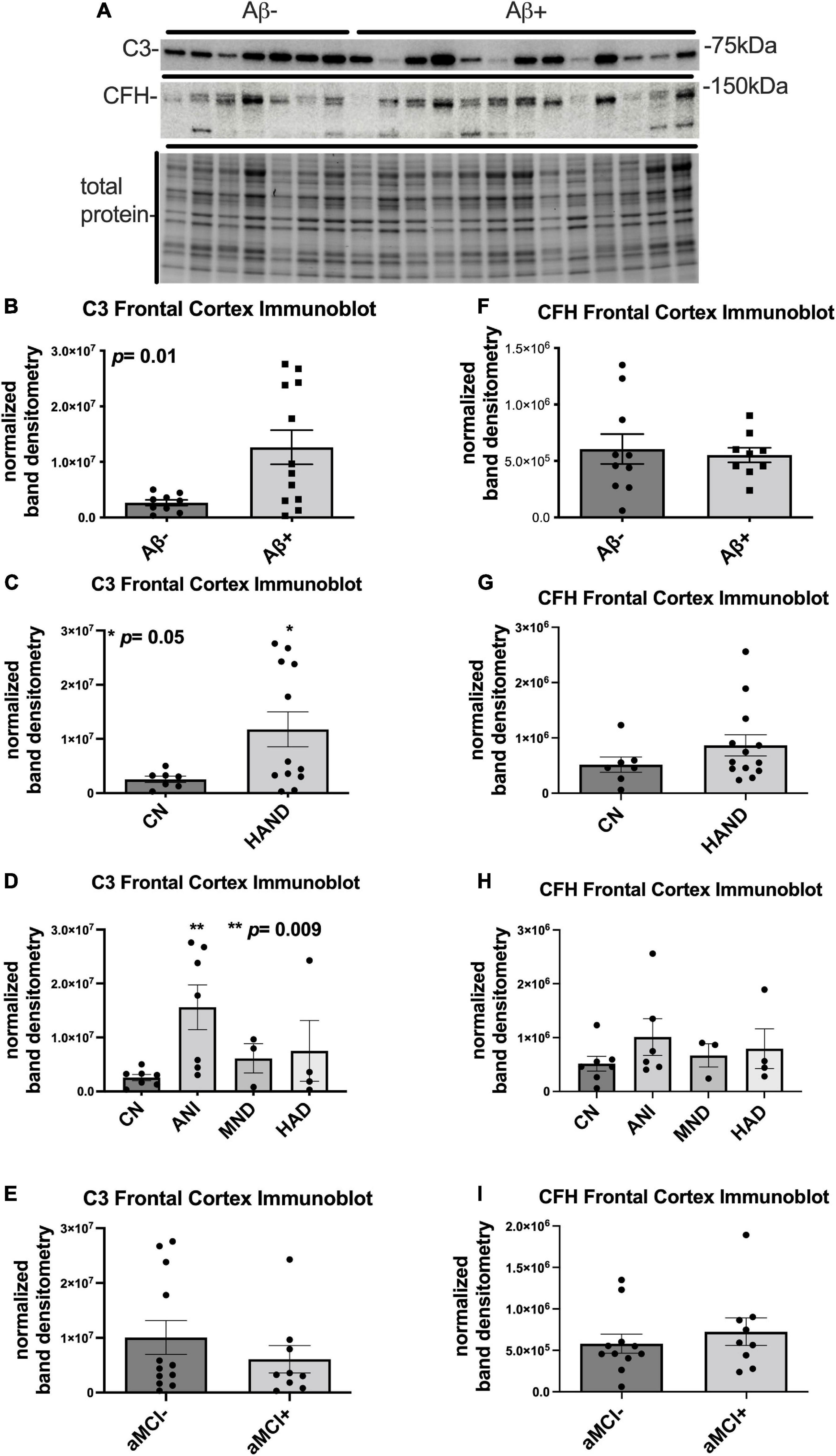
Figure 1. C3 protein is significantly upregulated in the frontal cortex of HIV brains with detectable Aβ plaques and in the frontal cortex from decedents with HAND. (A) Immunoblot for C3 and CFH and total protein transferred to the membrane using brain lysates from the frontal cortex from HIV brains that were determined to be negative or positive for Aβ plaques. (B) Quantification of C3 band intensity normalized to total protein transferred to the membrane stratified as negative or positive for Aβ plaques. (C) Quantification of C3 band intensity normalized to total protein and stratified by cognitive normal vs. HAND. (D) Quantification of C3 band intensity normalized to total protein and stratified by HAND sub-categories. (E) Quantification of C3 band intensity normalized to total protein and stratified by aMCI– vs. aMCI +. (F) Quantification of CFH band intensity normalized to total protein transferred to the membrane stratified as negative or positive for Aβ plaques. (G) Quantification of CFH band intensity normalized to total protein and stratified by cognitive normal vs. HAND. (H) Quantification of CFH band intensity normalized to total protein and stratified by HAND sub-categories. (I) Quantification of CFH band intensity normalized to total protein and stratified by aMCI– vs. aMCI +. Statistical significance was determined by an unpaired t-test (*p < 0.05, **p < 0.01).
Complement component 3 protein trends upward in the cerebellum of human immunodeficiency virus brains with detectable beta amyloid plaques
To determine the expression levels of C3 and CFH protein in the cerebellum of HIV + donors with and without AD-related pathology and HAND, we performed immunoblot of lysates stratified by presence of Aβ plaques (Umlauf et al., 2019; Figure 2A). Bands corresponding to C3 and CFH proteins were detected at approximately 75 and 150 kDa, respectively (Figure 2A). When normalized to total protein transferred to the membrane, the average intensity of the band corresponding to C3 was ∼5-fold higher in Aβ + group compared to the Aβ- group (Figure 2B), though this difference did not reach significance. The average intensity of the band corresponding to C3 did not significantly differ in HAND compared to the CN group (Figure 2C) nor when stratified by HAND subgroups (Figure 2D). There was no significant difference in C3 band intensity in aMCI- vs. aMCI + groups (Figure 2E). The average intensity of the band corresponding to CFH was not significantly different between the Aβ- and Aβ + groups (Figure 2F), the HAND and CN groups (Figures 2G,H), or the aMCI- and aMCI + groups (Figure 2I). We found no significant relationship between C3 or CFH levels in the CB and viral loads or CD4 + cell counts.
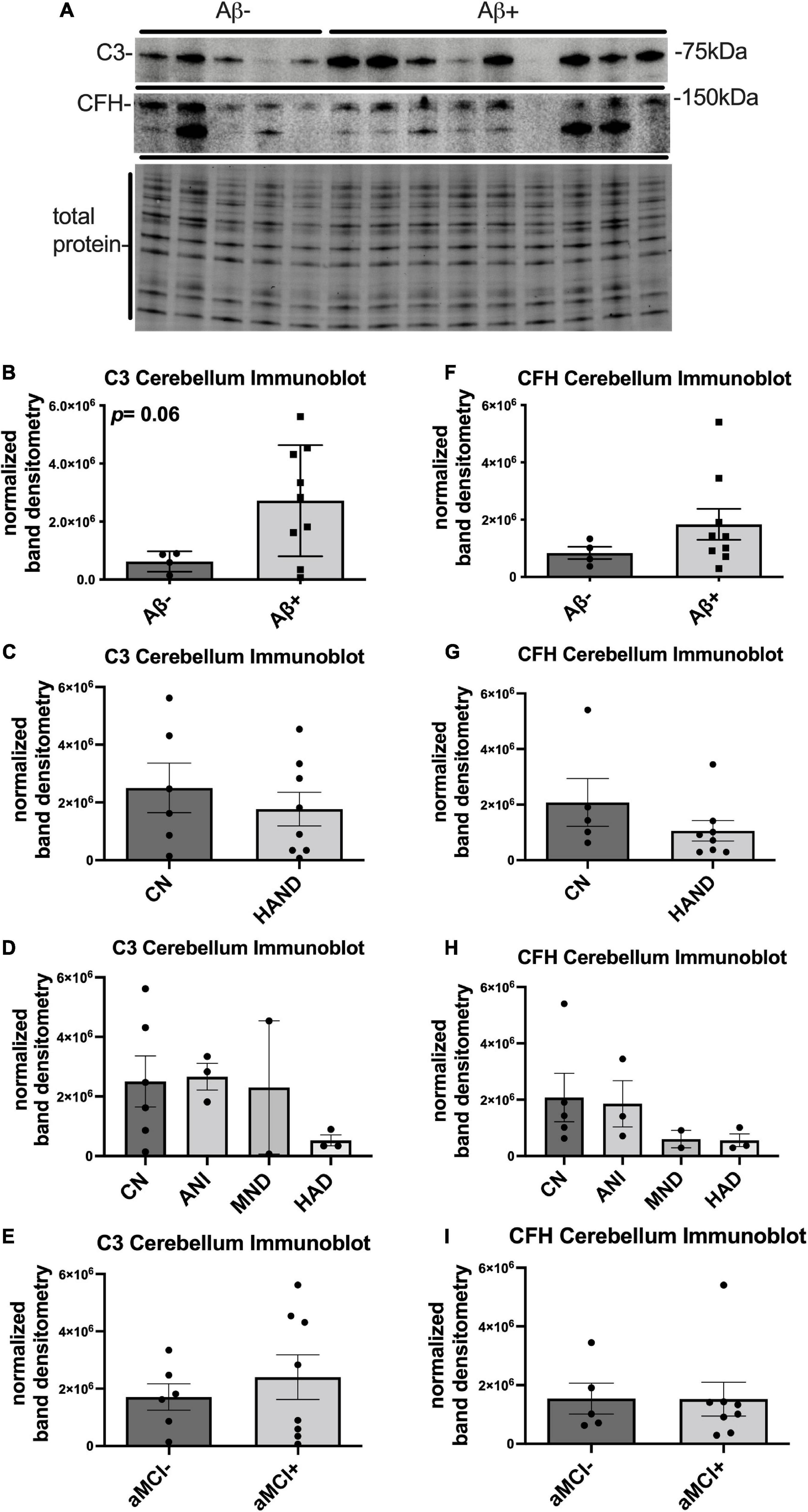
Figure 2. C3 protein trends upward in the cerebellum HIV brains with detectable Aβ plaques. (A) Immunoblot for C3 and CFH and total protein transferred to the membrane using brain lysates from the cerebellum from HIV brains that were determined to be negative or positive for Aβ plaques. (B) Quantification of C3 band intensity normalized to total protein transferred to the membrane stratified as negative or positive for Aβ plaques. (C) Quantification of C3 band intensity normalized to total protein and stratified by cognitive normal vs. HAND. (D) Quantification of C3 band intensity normalized to total protein and stratified by HAND sub-categories. (E) Quantification of C3 band intensity normalized to total protein and stratified by aMCI– vs. aMCI+. (F) Quantification of CFH band intensity normalized to total protein transferred to the membrane stratified as negative or positive for Aβ plaques. (G) Quantification of CFH band intensity normalized to total protein and stratified by cognitive normal vs. HAND. (H) Quantification of CFH band intensity normalized to total protein and stratified by HAND sub-categories. (I) Quantification of CFH band intensity normalized to total protein and stratified by aMCI– vs. aMCI+. Statistical significance was determined by an unpaired t-test.
Complement component 3 protein expression is higher in the frontal cortex than the cerebellum and complement factor H protein expression is higher in the cerebellum than in the frontal cortex
To determine differences in C3 and CFH expression in the FC and cerebellum, densitometry analyses quantities for each protein were compared between the two brain regions. The mean densitometry levels for C3 were significantly higher (∼4-fold) in the FC than in the cerebellum (Figure 3A). The mean densitometry levels for CFH were significantly higher (∼2.5-fold) in the cerebellum than in the FC (Figure 3B).
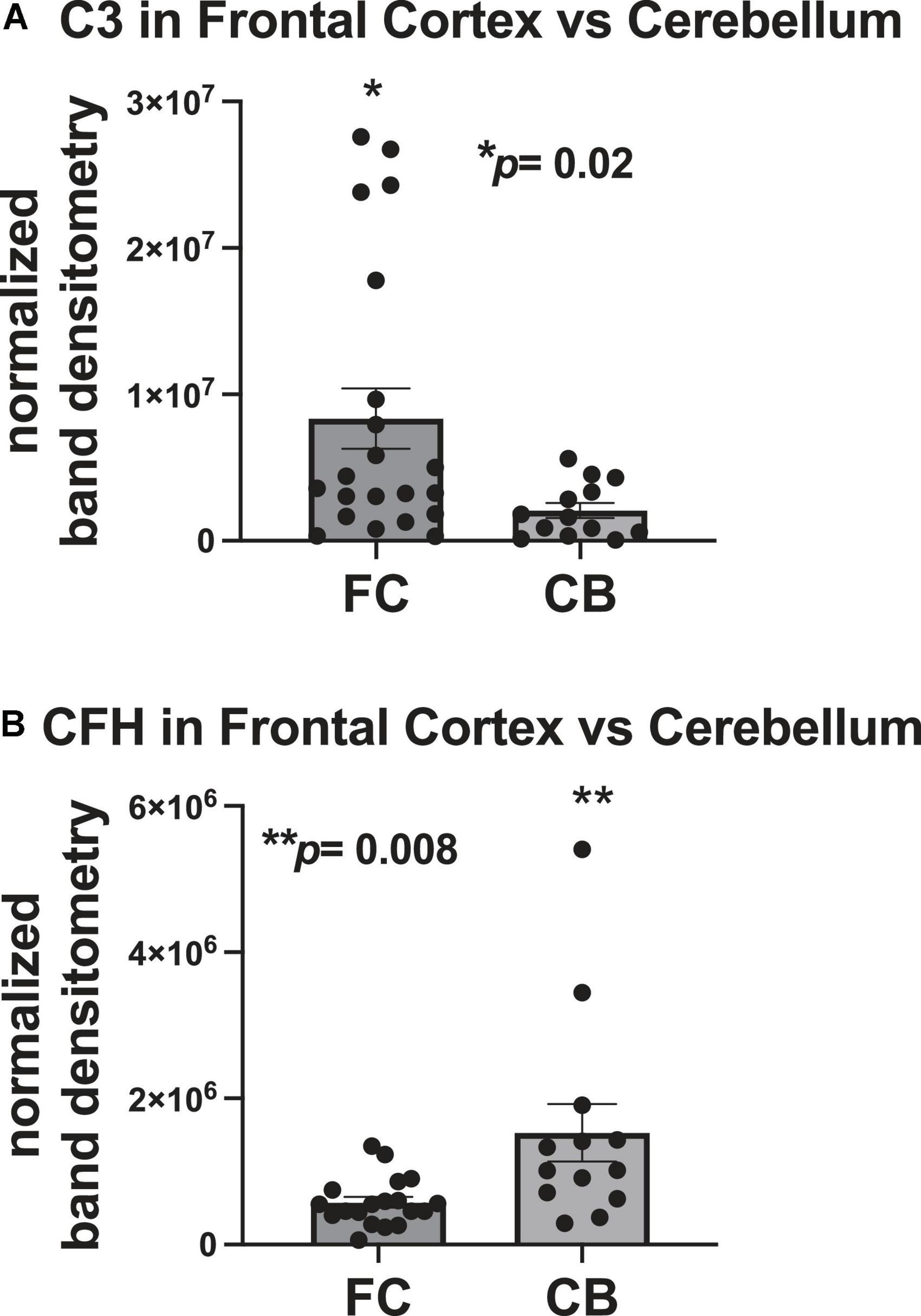
Figure 3. C3 protein expression is higher in the frontal cortex than the cerebellum and CFH protein expression is higher in the cerebellum than in the frontal cortex. (A) Quantification of C3 band intensity normalized to total protein and stratified by frontal cortex vs. cerebellum. (B) Quantification of CFH band intensity normalized to total protein transferred to the membrane stratified by frontal cortex vs. cerebellum. Statistical significance was determined by an unpaired t-test (*p < 0.05, **p < 0.01).
Complement factor H protein levels relate to motor (frontal and cerebellar complement factor H) and executive function (frontal complement factor H only) performance
In correlational analyses, frontal and cerebellar C3 levels did not relate to any domain-specific T-scores (p> 0.05). There were moderate-sized correlations between higher frontal CFH protein levels and poorer motor domain scores (ρ = 0.54, p = 0.02) and poorer executive domain performance (ρ = –0.40, p = 0.08; Figures 4A,B), with the former a significant relationship and the latter a statistical trend. Lower cerebellar CFH protein levels significantly related to poorer motor domain T-score (ρ = 0.66, p = 0.03; Figure 4C). Frontal or cerebellar CFH levels did not relate to any other domain-specific T-score (p > 0.05).
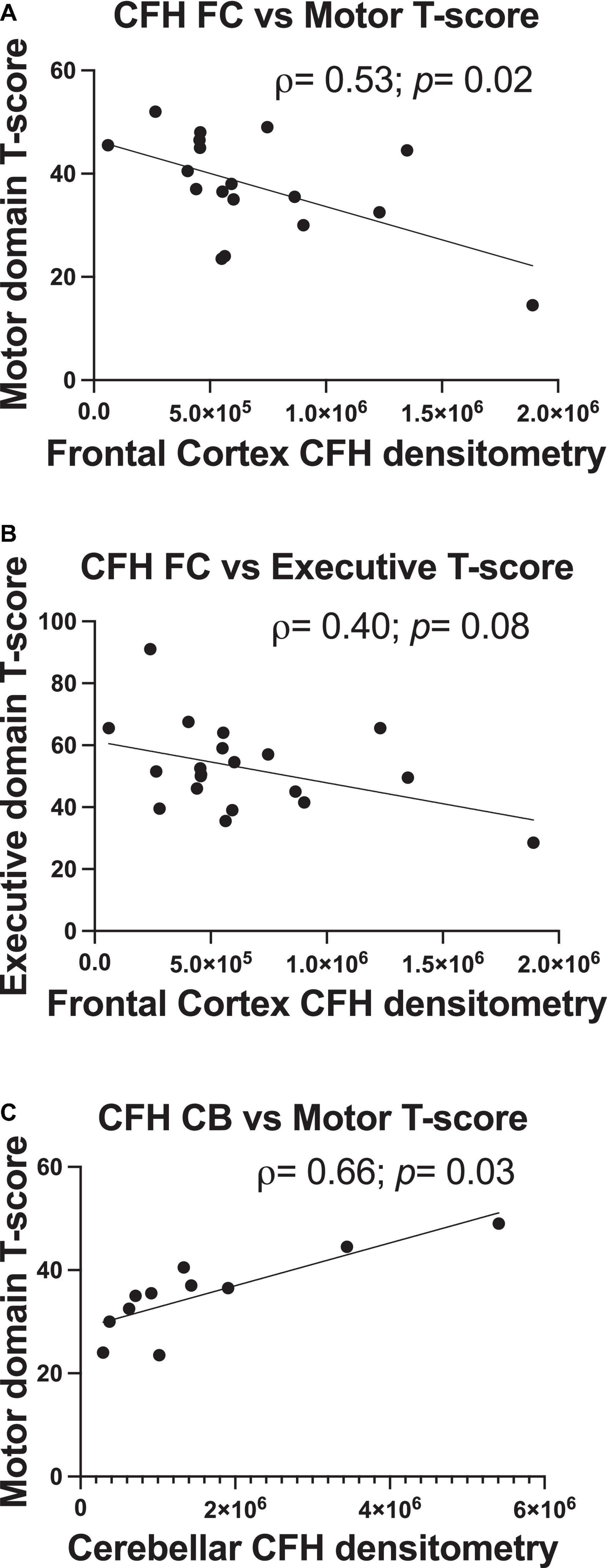
Figure 4. Frontal and cerebellar CFH protein levels relate to antemortem performance in specific cognitive domains. Quantification of frontal cortex CFH band intensity relates to motor domain (A) and executive function domain performance (B). Quantification of cerebellum CFH band intensity relates to motor domain performance (C). Statistical significance was determined by a Spearman’s rank-order correlation (ρ = Spearman’s rank correlation coefficient).
Discussion
The results of this study provide further evidence for the involvement of neuroinflammation and innate immune activation, with a specific emphasis on the complement system in the neuropathogenesis of HAND. This study also provides evidence for a role of the complement system at the nexus of HAND and AD neuropathogenesis. We identified increased C3 protein levels in FC tissues from decedents with HIV previously shown to have Aβ plaques in the cortex and in those diagnosed with HAND. Interestingly, and somewhat unexpectedly, C3 protein levels trended higher in the cerebellum of tissues with Aβ plaques in the cortex, despite having had fewer specimens available to analyze. Although there were no direct correlations with overall HAND status, altered levels of CFH protein in the FC were associated with deficiencies in executive and motor function performance. These findings are consistent with previous findings showing that alterations in the complement system may play a role in the neurodegenerative process in HAND and AD, highlighting the need to better understand the therapeutic potential of targeting this system.
C3 has long been suspected of being involved in the neurodegenerative process of HAND and AD. Our findings are consistent with a finding that C3 is positively associated with metabolic alterations in aged PWH (Bryant et al., 2016) and a significant role for complement in AD (Morgan, 2018). Elevated C3 levels in HAND and Aβ + brains are also consistent with studies showing that reactive astrocytes produce C3 (Liddelow et al., 2017; Nitkiewicz et al., 2017; Clarke et al., 2018; Vallee and Fields, 2022) and may contribute to metabolic deficiencies in neurons in HAND and AD (Jiang and Cadenas, 2014; Yin et al., 2016; Fields et al., 2019; Swinton et al., 2019). However, the relationship between C3 and AD neuropathogenesis is not clear. C3 deficiency promotes Aβ-associated neurotoxicity in animal models and enhanced levels of C3 in CSF are associated with MCI and AD (Wyss-Coray et al., 2002; Maier et al., 2008; Li et al., 2012; Lukiw and Alexandrov, 2012; Lukiw et al., 2012; Hoh Kam et al., 2013; Toledo et al., 2014; Hu et al., 2016; Zhang et al., 2016). C3 is also associated with the synaptic pruning process required for development and learning and memory formation (Schafer et al., 2012). This murky picture, when considering all available evidence, suggests that other factors are likely at play and interacting with the complement system to determine disease outcomes. Nevertheless, C3 consistently shows up in biomarker studies in association with HAND and AD neuropathogenesis, suggesting it may be useful as a therapeutic target or, when coupled with other biomarkers, a readout to determine therapeutic strategies and disease progression.
CFH plays a regulatory role in the complement pathway by dotting cells to be protected from C3 activity (Merle et al., 2015a,b). The elevated levels of CFH in the FC of PWH showing deficiencies in executive and motor function may represent a compensatory mechanism to reverse damage already done by C3 overactivation. On the other hand, CFH mutations and miRNA regulation have been shown to mute CFH function and be associated with disease (Li et al., 2012; Lukiw and Alexandrov, 2012; Lukiw et al., 2012; Zhang et al., 2016). In this case, higher levels may be negated by CFH dysfunction. However, further investigation into CFH sequences and miRNA expression in these brain tissues would be required to test this hypothesis. It is interesting that increases in CFH in the FC are not commensurate with the increases in C3 in the same brain tissues. This may indicate that more CFH in these tissues may protect cells from aberrant C3 overactivation. Moreover, the finding that C3 protein levels are higher in the FC than cerebellum while CFH protein levels are higher in the cerebellum than the FC adds a layer of complexity to interpreting the involvement of the complement system in HAND and AD. Triggering receptor on myeloid cells 2 (TREM2) is expressed on microglia and facilitates engulfment of synapses, dying cells, and Aβ (Kiialainen et al., 2005; Guerreiro et al., 2013). Increased levels of soluble TREM2 is associated with worse inflammation and neurodegenerative disease (Kiialainen et al., 2005; Benitez et al., 2013; Colonna and Wang, 2016; Henjum et al., 2016; Kobayashi et al., 2016). In light of recent reports of increased levels of soluble TREM2, by our group and others, may suggest pathogenic levels of synaptic pruning in PWH (Fields et al., 2018; Gisslen et al., 2019). It will be interesting to see if these differences are reflected in synaptic protein levels and synaptic health in the FC and cerebellum of these brain specimens. These findings suggest that along with C3, the role of CFH in neuropathogenesis of HIV in aging people deserves more attention.
The role of the cerebellum in HAND and AD has garnered little attention. However, recent studies suggest that the cerebellum is susceptible to Aβ deposition and toxicity (Hoxha et al., 2018). In brain specimens from humans and rodent models, Aβ deposition occurs in the region and is associated with synaptic damage (Sepulveda-Falla et al., 2011; Hoxha et al., 2012). While little is known about the cerebellum in HAND, our findings of C3 levels trending higher in the cerebellum in Aβ + brains are consistent with a role for this region in aging PWH. Similar to what we observed in the FC, the magnitude of change in CFH protein levels was not commensurate with C3 levels in the cerebellum. Moreover, the presence of motor dysfunction in many PWH may implicate a role for the cerebellum in neuropathogenesis of HIV. These findings in such a small cohort suggest more investigations are needed into the role of the cerebellum in PWH and neurological disorders.
In conclusion, our findings represent one of the first studies to investigate the role of the complement system in the context of AD-related neuropathogenesis in PWH. We also investigate for the first time the complement system in the cerebellum of HIV + brains in the context of AD-related neuropathogenesis. The discovery of strong associations in a cohort of limited size suggests more studies are necessary to determine the therapeutic and biomarker potential of C3 and CFH in aging PWH.
Data availability statement
The raw data supporting the conclusions of this article will be made available by the authors, without undue reservation.
Ethics statement
The studies involving human participants were reviewed and approved by the UCSD Institutional Review Board. The patients/participants provided their written informed consent to participate in this study.
Author contributions
JF, ES, and DM designed the study, drafted, and edited the manuscript. MS, NS, and K-AV performed brain tissue analyses and edited the manuscript. All authors contributed to the article and approved the submitted version.
Funding
This research was funded by the National Institute of Mental Health (MH100928 and MH128108) and the National Institute on Aging (AG066215). This publication was made possible from NIH funding through the NIMH and NINDS Institutes by the following grants: Manhattan HIV Brain Bank (MHBB): U24MH100931; Texas NeuroAIDS Research Center (TNRC): U24MH100930 National Neurological AIDS Bank (NNAB): U24MH100929 California NeuroAIDS Tissue Network (CNTN): U24MH100928 Data Coordinating Center (DCC): U24MH100925. Its contents were solely the responsibility of the authors and did not necessarily represent the official view of the NNTC or NIH.
Conflict of interest
The authors declare that the research was conducted in the absence of any commercial or financial relationships that could be construed as a potential conflict of interest.
Publisher’s note
All claims expressed in this article are solely those of the authors and do not necessarily represent those of their affiliated organizations, or those of the publisher, the editors and the reviewers. Any product that may be evaluated in this article, or claim that may be made by its manufacturer, is not guaranteed or endorsed by the publisher.
References
Achim, C. L., Adame, A., Dumaop, W., Everall, I. P., and Masliah, E. (2009). Increased accumulation of intraneuronal amyloid beta in HIV-infected patients. J. Neuroimmune Pharmacol. 4, 190–199. doi: 10.1007/s11481-009-9152-8
Althoff, K. N., Stewart, C. N., Humes, E., Zhang, J., Gerace, L., Boyd, C. M., et al. (2022). shifting age distribution of people with HIV using antiretroviral therapy in the United States. AIDS 36, 459–471. doi: 10.1097/QAD.0000000000003128
Antinori, A., Arendt, G., Becker, J. T., Brew, B. J., Byrd, D. A., Cherner, M., et al. (2007). Updated research nosology for HIV-associated neurocognitive disorders. Neurology 69, 1789–1799. doi: 10.1212/01.WNL.0000287431.88658.8b
Benitez, B. A., Cruchaga, C., and United States-, G. (2013). Spain Parkinson’s Disease Research, TREM2 and neurodegenerative disease. N. Engl. J. Med. 369, 1567–1568. doi: 10.1056/NEJMc1306509
Bondi, M. W., Edmonds, E. C., Jak, A. J., Clark, L. R., Delano-Wood, L., McDonald, C. R., et al. (2014). Neuropsychological criteria for mild cognitive impairment improves diagnostic precision, biomarker associations, and progression rates. J. Alzheimers Dis. 42, 275–289. doi: 10.3233/JAD-140276
Brew, B. J., Pemberton, L., Blennow, K., Wallin, A., and Hagberg, L. (2005). CSF amyloid beta42 and tau levels correlate with AIDS dementia complex. Neurology 65, 1490–1492. doi: 10.1212/01.wnl.0000183293.95787.b7
Bryant, A. K., Fazeli, P. L., Letendre, S. L., Ellis, R. J., Potter, M., Burdo, T. H., et al. (2016). Complement Component 3 Is Associated with Metabolic Comorbidities in Older HIV-Positive Adults. AIDS Res. Hum. Retroviruses 32, 271–278. doi: 10.1089/aid.2015.0179
Chelune, G. J., and Baer, R. A. (1986). Developmental norms for the Wisconsin Card Sorting test. J. Clin. Exp. Neuropsychol. 8, 219–228. doi: 10.1080/01688638608401314
Chelune, G. J., Heaton, R. K., and Lehman, R. A. (1986). “Neuropsychological and personality correlates of patients’ complaints of disability,” in The Neuropsychological Handbook, eds D. Wedding, A. M. Horton, and J. S. Webster. (New York, NY: Springer), (489–525) doi: 10.1007/978-1-4613-2211-5_4
Chemparthy, D. T., Kannan, M., Gordon, L., Buch, S., and Sil, S. (2021). Alzheimer’s-Like Pathology at the Crossroads of HIV-Associated Neurological Disorders. Vaccines 9:930 doi: 10.3390/vaccines9080930
Chen, X., Hui, L., and Geiger, J. D. (2014). Amyloid beta accumulation in HIV-1 infected brain: The role of altered cholesterol homeostasis. Clin. Res. HIV AIDS 1:1011.
Clarke, L. E., Liddelow, S. A., Chakraborty, C., Munch, A. E., Heiman, M., and Barres, B. A. (2018). Normal aging induces A1-like astrocyte reactivity. Proc. Natl. Acad. Sci. U.S.A. 115, E1896–E1905. doi: 10.1073/pnas.1800165115
Clifford, D. B., Fagan, A. M., Holtzman, D. M., Morris, J. C., Teshome, M., Shah, A. R., et al. (2009). CSF biomarkers of Alzheimer disease in HIV-associated neurologic disease. Neurology 73, 1982–1987. doi: 10.1212/WNL.0b013e3181c5b445
Colonna, M., and Wang, Y. (2016). TREM2 variants: New keys to decipher Alzheimer disease pathogenesis. Nat. Rev. Neurosci. 17, 201–207. doi: 10.1038/nrn.2016.7
Cysique, L. A., Casaletto, K. B., and Heaton, R. K. (2021). Reliably Measuring Cognitive Change in the Era of Chronic HIV Infection and Chronic HIV-Associated Neurocognitive Disorders. Curr. Top. Behav. Neurosci. 50, 271–298. doi: 10.1007/7854_2019_116
Cysique, L. A., Franklin, D. Jr., Abramson, I., Ellis, R. J., Letendre, S., Collier, A., et al. (2011). Normative data and validation of a regression based summary score for assessing meaningful neuropsychological change. J. Clin. Exp Neuropsychol. 33, 505–522. doi: 10.1080/13803395.2010.535504
Esiri, M. M., Biddolph, S. C., and Morris, C. S. (1998). Prevalence of Alzheimer plaques in AIDS. J. Neurol. Neurosurg. Psychiatry 65, 29–33. doi: 10.1136/jnnp.65.1.29
Fields, J., Dumaop, W., Rockenstein, E., Mante, M., Spencer, B., Grant, I., et al. (2013). Age-dependent molecular alterations in the autophagy pathway in HIVE patients and in a gp120 tg mouse model: Reversal with beclin-1 gene transfer. J. Neurovirol. 19, 89–101. doi: 10.1007/s13365-012-0145-7
Fields, J. A., Spencer, B., Swinton, M., Qvale, E. M., Marquine, M. J., Alexeeva, A., et al. (2018). Alterations in brain TREM2 and Amyloid-beta levels are associated with neurocognitive impairment in HIV-infected persons on antiretroviral therapy. J. Neurochem. 147, 784–802 doi: 10.1111/jnc.14582
Fields, J. A., Swinton, M. K., Carson, A., Soontornniyomkij, B., Lindsay, C., Han, M. M., et al. (2019). Tenofovir disoproxil fumarate induces peripheral neuropathy and alters inflammation and mitochondrial biogenesis in the brains of mice. Sci. Rep. 9:17158. doi: 10.1038/s41598-019-53466-x
Fields, J. A., Swinton, M. K., Soontornniyomkij, B., Carson, A., and Achim, C. L. (2020). Beta amyloid levels in CSF of HIV-infected people vary by exposure to antiretroviral therapy. AIDS 34, 1001–1007. doi: 10.1097/QAD.0000000000002506
Gelman, B. B. (2015). Neuropathology of HAND With Suppressive Antiretroviral Therapy: Encephalitis and Neurodegeneration Reconsidered. Curr. HIV/AIDS Rep. 12, 272–279. doi: 10.1007/s11904-015-0266-8
Giometto, B., An, S. F., Groves, M., Scaravilli, T., Geddes, J. F., Miller, R., et al. (1997). Accumulation of beta-amyloid precursor protein in HIV encephalitis: Relationship with neuropsychological abnormalities. Ann. Neurol. 42, 34–40. doi: 10.1002/ana.410420108
Gisslen, M., Heslegrave, A., Veleva, E., Yilmaz, A., Andersson, L. M., and Hagberg, L. (2019). CSF concentrations of soluble TREM2 as a marker of microglial activation in HIV-1 infection. Neurol. Neuroimmunol. Neuroinflamm. 6:e512. doi: 10.1212/NXI.0000000000000512
Gisslen, M., Krut, J., Andreasson, U., Blennow, K., Cinque, P., Brew, B. J., et al. (2009). Amyloid and tau cerebrospinal fluid biomarkers in HIV infection. BMC Neurol. 9:63. doi: 10.1186/1471-2377-9-63
Goetzl, E. J., Schwartz, J. B., Abner, E. L., Jicha, G. A., and Kapogiannis, D. (2018). High complement levels in astrocyte-derived exosomes of Alzheimer disease. Ann. Neurol. 83, 544–552. doi: 10.1002/ana.25172
Green, D. A., Masliah, E., Vinters, H. V., Beizai, P., Moore, D. J., and Achim, C. L. (2005). Brain deposition of beta-amyloid is a common pathologic feature in HIV positive patients. Aids 19, 407–411. doi: 10.1097/01.aids.0000161770.06158.5c
Guerreiro, R., Wojtas, A., Bras, J., Carrasquillo, M., Rogaeva, E., Majounie, E., et al. (2013). TREM2 variants in Alzheimer’s disease. N. Engl. J. Med. 368, 117–127. doi: 10.1056/NEJMoa1211851
Heaton, R. K., Clifford, D. B., Franklin, D. R. Jr., Woods, S. P., Ake, C., Vaida, F., et al. (2010). HIV-associated neurocognitive disorders persist in the era of potent antiretroviral therapy: CHARTER Study. Neurology 75, 2087–2096. doi: 10.1212/WNL.0b013e318200d727
Heaton, R. K., Franklin, D. R., Ellis, R. J., McCutchan, J. A., Letendre, S. L., Leblanc, S., et al. (2011). HIV-associated neurocognitive disorders before and during the era of combination antiretroviral therapy: Differences in rates, nature, and predictors. J. Neurovirol. 17, 3–16. doi: 10.1007/s13365-010-0006-1
Heneka, M. T., Carson, M. J., El Khoury, J., Landreth, G. E., Brosseron, F., Feinstein, D. L., et al. (2015). in Alzheimer’s disease. Lancet Neurol. 14, 388–405. doi: 10.1016/S1474-4422(15)70016-5
Heneka, M. T., Kummer, M. P., and Latz, E. (2014). Innate immune activation in neurodegenerative disease. Nat. Rev. Immunol. 14, 463–477. doi: 10.1038/nri3705
Henjum, K., Almdahl, I. S., Arskog, V., Minthon, L., Hansson, O., Fladby, T., et al. (2016). Cerebrospinal fluid soluble TREM2 in aging and Alzheimer’s disease. Alzheimers Res. Ther. 8:17. doi: 10.1186/s13195-016-0182-1
Hoh Kam, J., Lenassi, E., Malik, T. H., Pickering, M. C., and Jeffery, G. (2013). Complement component C3 plays a critical role in protecting the aging retina in a murine model of age-related macular degeneration. Am. J. Pathol. 183, 480–492. doi: 10.1016/j.ajpath.2013.04.008
Hoxha, E., Boda, E., Montarolo, F., Parolisi, R., and Tempia, F. (2012). Excitability and synaptic alterations in the cerebellum of APP/PS1 mice. PLoS One 7:e34726. doi: 10.1371/journal.pone.0034726
Hoxha, E., Lippiello, P., Zurlo, F., Balbo, I., Santamaria, R., Tempia, F., et al. (2018). The Emerging Role of Altered Cerebellar Synaptic Processing in Alzheimer’s Disease. Front. Aging Neurosci. 10:396. doi: 10.3389/fnagi.2018.00396
Hu, W. T., Watts, K. D., Tailor, P., Nguyen, T. P., Howell, J. C., Lee, R. C., et al. (2016). Neuro-Imaging, CSF complement 3 and factor H are staging biomarkers in Alzheimer’s disease. Acta Neuropathol. Commun. 4:14. doi: 10.1186/s40478-016-0277-8
Jiang, T., and Cadenas, E. (2014). Astrocytic metabolic and inflammatory changes as a function of age. Aging Cell 13, 1059–1067. doi: 10.1111/acel.12268
Kiialainen, A., Hovanes, K., Paloneva, J., Kopra, O., and Peltonen, L. (2005). Dap12 and Trem2, molecules involved in innate immunity and neurodegeneration, are co-expressed in the CNS. Neurobiol. Dis. 18, 314–322. doi: 10.1016/j.nbd.2004.09.007
Kim, C., Kwon, S., Iba, M., Spencer, B., Rockenstein, E., Mante, M., et al. (2021). Effects of innate immune receptor stimulation on extracellular alpha-synuclein uptake and degradation by brain resident cells. Exp. Mol. Med. 53, 281–290. doi: 10.1038/s12276-021-00562-6
Kish, S. J., Lopes-Cendes, I., Guttman, M., Furukawa, Y., Pandolfo, M., Rouleau, G. A., et al. (1998). Brain glyceraldehyde-3-phosphate dehydrogenase activity in human trinucleotide repeat disorders. Arch. Neurol. 55, 1299–1304. doi: 10.1001/archneur.55.10.1299
Kobayashi, M., Konishi, H., Sayo, A., Takai, T., and Kiyama, H. (2016). TREM2/DAP12 Signal Elicits Proinflammatory Response in Microglia and Exacerbates Neuropathic Pain. J. Neurosci. 36, 11138–11150. doi: 10.1523/JNEUROSCI.1238-16.2016
Lawton, M. P., and Brody, E. M. (1969). Assessment of older people: Self-maintaining and instrumental activities of daily living. Gerontologist 9, 179–186. doi: 10.1093/geront/9.3_Part_1.179
Levine, A. J., Soontornniyomkij, V., Achim, C. L., Masliah, E., Gelman, B. B., Sinsheimer, J. S., et al. (2016). Multilevel analysis of neuropathogenesis of neurocognitive impairment in HIV. J. Neurovirol. 22, 431–441. doi: 10.1007/s13365-015-0410-7
Li, Y. Y., Alexandrov, P. N., Pogue, A. I., Zhao, Y., Bhattacharjee, S., and Lukiw, W. J. (2012). miRNA-155 upregulation and complement factor H deficits in Down’s syndrome. Neuroreport 23, 168–173. doi: 10.1097/WNR.0b013e32834f4eb4
Liddelow, S. A., Guttenplan, K. A., Clarke, L. E., Bennett, F. C., Bohlen, C. J., Schirmer, L., et al. (2017). Neurotoxic reactive astrocytes are induced by activated microglia. Nature 541, 481–487. doi: 10.1038/nature21029
Lukiw, W. J., and Alexandrov, P. N. (2012). Regulation of complement factor H (CFH) by multiple miRNAs in Alzheimer’s disease (AD) brain. Mol. Neurobiol. 46, 11–19. doi: 10.1007/s12035-012-8234-4
Lukiw, W. J., Surjyadipta, B., Dua, P., and Alexandrov, P. N. (2012). Common micro RNAs (miRNAs) target complement factor H (CFH) regulation in Alzheimer’s disease (AD) and in age-related macular degeneration (AMD). Int. J. Biochem. Mol. Biol. 3, 105–116.
Mahmood, S. R., Xie, X., Hosny, N., El, S, Venit, T., Gunsalus, K. C., et al. (2021). beta-actin dependent chromatin remodeling mediates compartment level changes in 3D genome architecture. Nat. Commun. 12:5240. doi: 10.1038/s41467-021-25596-2
Maier, M., Peng, Y., Jiang, L., Seabrook, T. J., Carroll, M. C., and Lemere, C. A. (2008). Complement C3 deficiency leads to accelerated amyloid beta plaque deposition and neurodegeneration and modulation of the microglia/macrophage phenotype in amyloid precursor protein transgenic mice. J. Neurosci. 28, 6333–6341. doi: 10.1523/JNEUROSCI.0829-08.2008
Merle, N. S., Church, S. E., Fremeaux-Bacchi, V., and Roumenina, L. T. (2015a). Complement System Part I - Molecular Mechanisms of Activation and Regulation. Front. Immunol. 6:262. doi: 10.3389/fimmu.2015.00262
Merle, N. S., Noe, R., Halbwachs-Mecarelli, L., Fremeaux-Bacchi, V., and Roumenina, L. T. (2015b). Complement System Part II: Role in Immunity. Front. Immunol. 6:257. doi: 10.3389/fimmu.2015.00257
Morgan, B. P. (2015). The role of complement in neurological and neuropsychiatric diseases. Expert. Rev. Clin. Immunol. 11, 1109–1119. doi: 10.1586/1744666X.2015.1074039
Morgan, B. P. (2018). Complement in the pathogenesis of Alzheimer’s disease. Semin. Immunopathol. 40, 113–124. doi: 10.1007/s00281-017-0662-9
Murray, J., Meloni, G., Cortes, E. P., KimSilva, A., Jacobs, M., Ramkissoon, A., et al. (2022). Frontal lobe microglia, neurodegenerative protein accumulation, and cognitive function in people with HIV. Acta Neuropathol. Commun. 10:69. doi: 10.1186/s40478-022-01375-y
Nitkiewicz, J., Borjabad, A., Morgello, S., Murray, J., Chao, W., Emdad, L., et al. (2017). HIV induces expression of complement component C3 in astrocytes by NF-kappaB-dependent activation of interleukin-6 synthesis. J. Neuroinflammation 14:23. doi: 10.1186/s12974-017-0794-9
Norman, M. A., Moore, D. J., Taylor, M., Franklin, D. Jr., Cysique, L., Ake, C., et al. (2011). Demographically corrected norms for African Americans and Caucasians on the Hopkins Verbal Learning Test-Revised, Brief Visuospatial Memory Test-Revised, Stroop Color and Word Test, and Wisconsin Card Sorting Test 64-Card Version. J. Clin. Exp. Neuropsychol. 33, 793–804. doi: 10.1080/13803395.2011.559157
Ortega, M., and Ances, B. M. (2014). Role of HIV in amyloid metabolism. J. Neuroimmune Pharmacol. 9, 483–491. doi: 10.1007/s11481-014-9546-0
Schafer, D. P., Lehrman, E. K., Kautzman, A. G., Koyama, R., Mardinly, A. R., Yamasaki, R., et al. (2012). Microglia sculpt postnatal neural circuits in an activity and complement-dependent manner. Neuron 74, 691–705. doi: 10.1016/j.neuron.2012.03.026
Sekar, S., McDonald, J., Cuyugan, L., Aldrich, J., Kurdoglu, A., Adkins, J., et al. (2015). Alzheimer’s disease is associated with altered expression of genes involved in immune response and mitochondrial processes in astrocytes. Neurobiol. Aging 36, 583–591. doi: 10.1016/j.neurobiolaging.2014.09.027
Sepulveda-Falla, D., Matschke, J., Bernreuther, C., Hagel, C., Puig, B., Villegas, A., et al. (2011). Deposition of hyperphosphorylated tau in cerebellum of PS1 E280A Alzheimer’s disease. Brain Pathol. 21, 452–463. doi: 10.1111/j.1750-3639.2010.00469.x
Sundermann, E. E., Bondi, M. W., Campbell, L. M., Gouaux, B., Moore, R. C., Soontornniyomkij, V., et al. (2021). Distinguishing Amnestic Mild Cognitive Impairment From HIV-Associated Neurocognitive Disorders. J. Infect. Dis. 224, 435–442. doi: 10.1093/infdis/jiaa760
Swinton, M. K., Carson, A., Telese, F., Sanchez, A. B., Soontornniyomkij, B., Rad, L., et al. (2019). Mitochondrial biogenesis is altered in HIV+ brains exposed to ART: Implications for therapeutic targeting of astroglia. Neurobiol. Dis. 130:104502. doi: 10.1016/j.nbd.2019.104502
Swinton, M. K., Sundermann, E. E., Pedersen, L., Nguyen, J. D., Grelotti, D. J., Taffe, M. A., et al. (2021). Alterations in Brain Cannabinoid Receptor Levels Are Associated with HIV-Associated Neurocognitive Disorders in the ART Era: Implications for Therapeutic Strategies Targeting the Endocannabinoid System. Viruses 13:1742. doi: 10.3390/v13091742
Toledo, J. B., Korff, A., Shaw, L. M., Trojanowski, J. Q., and Zhang, J. (2014). Low levels of cerebrospinal fluid complement 3 and factor H predict faster cognitive decline in mild cognitive impairment. Alzheimers Res. Ther. 6:36. doi: 10.1186/alzrt266
Umlauf, A., Soontornniyomkij, B., Sundermann, E. E., Gouaux, B., Ellis, R. J., Levine, A. J., et al. (2019). Risk of developing cerebral beta-amyloid plaques with posttranslational modification among HIV-infected adults. AIDS 33, 2157–2166. doi: 10.1097/QAD.0000000000002336
Vallee, K. J., and Fields, J. A. (2022). Caloric Restriction Mimetic 2-Deoxyglucose Reduces Inflammatory Signaling in Human Astrocytes: Implications for Therapeutic Strategies Targeting Neurodegenerative Diseases. Brain Sci. 12:308 doi: 10.3390/brainsci12030308
Wang, Y., Liu, M., Lu, Q., Farrell, M., Lappin, J. M., Shi, J., et al. (2020). Global prevalence and burden of HIV-associated neurocognitive disorder: A meta-analysis. Neurology 95, e2610–e2621. doi: 10.1212/WNL.0000000000010752
Woods, S. P., Rippeth, J. D., Frol, A. B., Levy, J. K., Ryan, E., Soukup, V. M., et al. (2004). Interrater reliability of clinical ratings and neurocognitive diagnoses in HIV. J. Clin. Exp. Neuropsychol. 26, 759–778. doi: 10.1080/13803390490509565
Wyss-Coray, T., Yan, F., Lin, A. H., Lambris, J. D., Alexander, J. J., Quigg, R. J., et al. (2002). Prominent neurodegeneration and increased plaque formation in complement-inhibited Alzheimer’s mice. Proc. Natl. Acad. Sci. U.S.A. 99, 10837–10842. doi: 10.1073/pnas.162350199
Yin, F., Sancheti, H., Patil, I., and Cadenas, E. (2016). Energy metabolism and inflammation in brain aging and Alzheimer’s disease. Free Radic. Biol. Med. 100, 108–122. doi: 10.1016/j.freeradbiomed.2016.04.200
Keywords: complement factor H, complement—immunological term, Alzheimer’s disease, HIV, beta—amyloid peptide, complement component 3
Citation: Fields JA, Swinton M, Sundermann EE, Scrivens N, Vallee K-AJ and Moore DJ (2022) Complement component 3 and complement factor H protein levels are altered in brain tissues from people with human immunodeficiency virus: A pilot study. Front. Aging Neurosci. 14:981937. doi: 10.3389/fnagi.2022.981937
Received: 29 June 2022; Accepted: 01 August 2022;
Published: 31 August 2022.
Edited by:
Carmen Cristina Diaconu, Stefan S. Nicolau Institute of Virology, RomaniaReviewed by:
Dianne T. Langford, Temple University, United StatesJohnny He, Rosalind Franklin University of Medicine and Science, United States
Copyright © 2022 Fields, Swinton, Sundermann, Scrivens, Vallee and Moore. This is an open-access article distributed under the terms of the Creative Commons Attribution License (CC BY). The use, distribution or reproduction in other forums is permitted, provided the original author(s) and the copyright owner(s) are credited and that the original publication in this journal is cited, in accordance with accepted academic practice. No use, distribution or reproduction is permitted which does not comply with these terms.
*Correspondence: Jerel Adam Fields, amFmaWVsZHNAdWNzZC5lZHU=