- 1Department of General Practice, The Sixth Medical Center of PLA General Hospital, Beijing, China
- 2Six Health Care Department, The Sixth Medical Center of PLA General Hospital, Beijing, China
To analyze the structural characteristics of intestinal microflora and changes of serum inflammatory factors of the Alzheimer’s disease, and to explore the relationship between them and dementia, we selected 30 patients in the AD group and 30 patients in the normal group, and collected stool samples to analyze the intestinal flora structure characteristics of the two groups of patients, and statistically analyzed the inflammatory cytokines TNF-α, IL-1β, IL-6, and IL-8 by ELISA from the venous blood of the two groups. The results show that the dominant Bacteroides in the two groups are Bacteroides, Firmicutes, Proteobacteria, and Actinobacteria. The abundance of Bacteroides, Firmicutes, and Proteobacteria in the AD group shows a statistical difference. At the genus level, the abundance of anti-inflammatory bacteria such as Lactobacillus, Bifidobacterium, and Ruminococcus drops in AD group, while the abundance of pro-inflammatory bacteria such as Escherichia and Enterococcus raises. Statistical analysis of inflammatory cytokines in the two groups suggests that TNF-α and IL-6 levels significantly increase in the AD group, with statistical differences. Therefore, it is speculated that the increased abundance of pro-inflammatory bacteria in intestinal flora may lead to or aggravate neuroinflammation through the release of inflammatory factors, thus further leading to the occurrence and development of AD.
Introduction
With the aging process of society, the incidence of dementia is increasing year by year and has become a common disease of the elderly. It is reported that by 2015, the number of global dementia patients was about 47 million and is expected to reach 131 million by 2050 (Livingston et al., 2017; Watermeyer and Calia, 2019), which is bound to place a heavy burden on families and society. Alzheimer’s disease dementia accounts for 60∼80%, which is the leading cause of disability and death in the elderly (Alzheimer’s Association, 2019; Alzheimer’s Disease International, 2019).
Alzheimer’s disease (AD) is a chronic neurodegenerative disease common to the elderly, characterized by a gradual decline in cognitive function that eventually leads to loss of memory, thinking, and reasoning capability. The pathological characteristics of AD are amyloid protein (Aβ) deposition and neurofibrillary tangles (NFT). However, the specific pathogenesis of AD is still unclear. It is reported that environmental factors and genetic factors play a role in developing AD. Many previous studies (Zhao and Gong, 2015; Swerdlow, 2018; Nguyen et al., 2020) suggest that mitochondrial dysfunction, insulin resistance and cerebral hypoperfusion may mediate, drive or even trigger pathological molecular cascades in AD, ultimately promoting Aβ accumulation, tau hyperphosphorylation, synaptic degeneration, and neuronal dysfunction. However, these studies cannot fully explain AD’s etiology and potential pathophysiological mechanism.
In recent years, studies have shown that intestinal flora is related to the incidence and progression of AD. The Human gut microbiome is considered as a “second brain” (Schneider et al., 2019; Sochocka et al., 2019). Some essential vitamins and substances involved in developing the central nervous system and immune regulation are produced by intestinal microbiota (Picca et al., 2018). In addition to destroying the stability of the intestinal environment, the imbalance of intestinal microorganisms will also affect behavior, learning, and memory, as well as the occurrence of nervous system diseases (Luczynski et al., 2016; Tremlett et al., 2017). Previous studies have suggested that the mechanism of intestinal microbiota leading to AD may start from the imbalance of intestinal microbiota, the development of local and systemic inflammation, and the disorder of the enteric-brain axis. The increased permeability of the intestinal epithelial barrier leads to the invasion of bacteria, viruses, and their neuroactive products and leads to neuroinflammatory responses in the brain, leading to AD (Sochocka et al., 2019). Combined with previous researches, we suggest that the occurrence of AD may be related to the low level of the inflammatory response caused by the structural disorder of intestinal flora. The change of intestinal flora structure and the increase of intestinal flora with pro-inflammatory effects, such as Bifidobacterium, will lead to the increase of inflammatory factor level, further lead to neuroinflammation and promote the occurrence and development of AD. This study aims to observe the structural characteristics of intestinal flora and the changes of serum inflammatory factors in patients with Alzheimer’s disease and explore the relationship between them and Alzheimer’s disease.
Data and methods
General information
We routinely performed preliminary screening of MMSE + MOCA on patients in the neurology outpatient department and ward of the cadres of our hospital. Patients with cognitive dysfunction were judged according to the diagnostic criteria of AD stipulated by IWG-2 (2014). Those meeting the diagnostic criteria of AD were enrolled in the AD group, and those without both cognitive dysfunction and AD dementia were classified as the normal group. In addition, all the patients in the above two groups had no infectious diseases, gastrointestinal diseases, or oral probiotics within the last 3°months after screening. The cut off for the subdivision of the sample between AD group and normal group is as follows:
Normal Group: MMSE ≥ 27 MOCA ≥ 26
Alzheimer’s disease Group: secondary school education and above: MMSE ≤ 24
primary school education: MMSE ≤ 20
illiterate: MMSE ≤ 17
MOCA:11 ∼ 21
Inspection methods
Three°ml of fasting venous blood was taken from the two groups early morning and centrifuged at 2,000r/min for 10°min to separate the upper serum. Inflammatory cytokines TNF-α, IL-1β, IL-6, and IL-8 were detected by ELISA.
Collect fresh, middle, rear, and internal fecal samples from the two groups. Each sample needs 2G. After collection, it shall be frozen and stored in the −80° refrigerator immediately. Remember to freeze and repeatedly thaw during storage.
Stool DNA Kit (Omega, USA) extracted total DNA from fecal samples. The specific operation was carried out according to the instructions. The extracted whole-genome DNA was inspected, and the qualified bacteria were amplified in the V4 variable region of 16S rDNA. The amplicons were recovered, purified, and quantified, and double-ended sequencing was performed according to the standard process of the Illumina miseq 250 sequencing platform. The double-ended sequences obtained by sequencing were spliced into a target region sequence, and the target sequence was filtered by quality control. The filtered sequence is compared with the reference database, and the chimeric sequence is removed to obtain the final optimized sequence. Out cluster analysis and species taxonomic annotation were carried out based on the optimized sequence.
Statistical analysis
SPSS statistical software was used to analyze the data. The measurement was expressed as (x ± s). One way, an OVA was used when the data were in line with normal distribution and the variance was uniform; otherwise, the rank-sum test was used; the Chi-square test was used to compare the rates. P < 0.05 was considered statistically significant.
Results
Basic characteristics
Thirty subjects were included in the AD group and the normal group respectively. Statistical analysis results showed that there were no significant statistical differences in age gender, smoking history, drinking history and general diet between the two groups (Table 1). (In this study, the subjects were randomly selected from the inpatients in the cadre ward, so the proportion of males was higher than females).
Coverage of sample flora
The Coverage index refers to the sequencing coverage of each sample strain. Each ‘group’s values are above 0.9, indicating a low probability of not being measured in the sample sequence (Table 2).
Alpha diversity analysis
Alpha diversity analysis reflects the richness of microorganisms in samples by diversity index. We selected the Shannon index and Simpson index for statistical analysis. The larger Shannon index and the smaller Simpson index, the higher species diversity in the sample is (Table 3).
The structure and abundance of bacteria at the phylum level in each group
The bacterial community structure of the two groups of samples at the phylum level is shown in Figure 1. The dominant phyla are Bacteroidetes, Firmicutes, Proteobacteria, and Actinomycetes, respectively. Compared with the normal, the abundance of Bacteroidetes (P = 0.014) and Proteobacteria (P = 0.004) in the AD group increased, and the abundance of Firmicutes decreased (P = 0.000) (Table 4).
The inflammatory factors and abundance of anti-inflammatory bacteria and pro-inflammatory bacteria in each group
The levels of inflammatory factors TNF-α (P = 0.004) and IL-6 (P = 0.001) are significantly different between the normal group and AD group, but there are no significant differences in the levels of IL-1β (P = 1.000) and IL-8 (P = 0.072) between the two groups.
We selected some of the dominant flora in the gut, such as Bifidobacterium, Lactobacillus, Ruminococcus with anti-inflammatory effects and Escherichia, Klebsiella, and Enterococcus with pro-inflammatory effects, and statistically analyzed the abundance values of the above bacteria in the two groups of samples. Compared with the normal, the abundance of Ruminococcus (P = 0.000), Lactobacillus (P = 0.000), and Bifidobacterium (P = 0.000) in the AD group decreased, and the abundance of Escherichia coli (P = 0.000) and Enterococcus increased (P = 0.025) (Table 5).
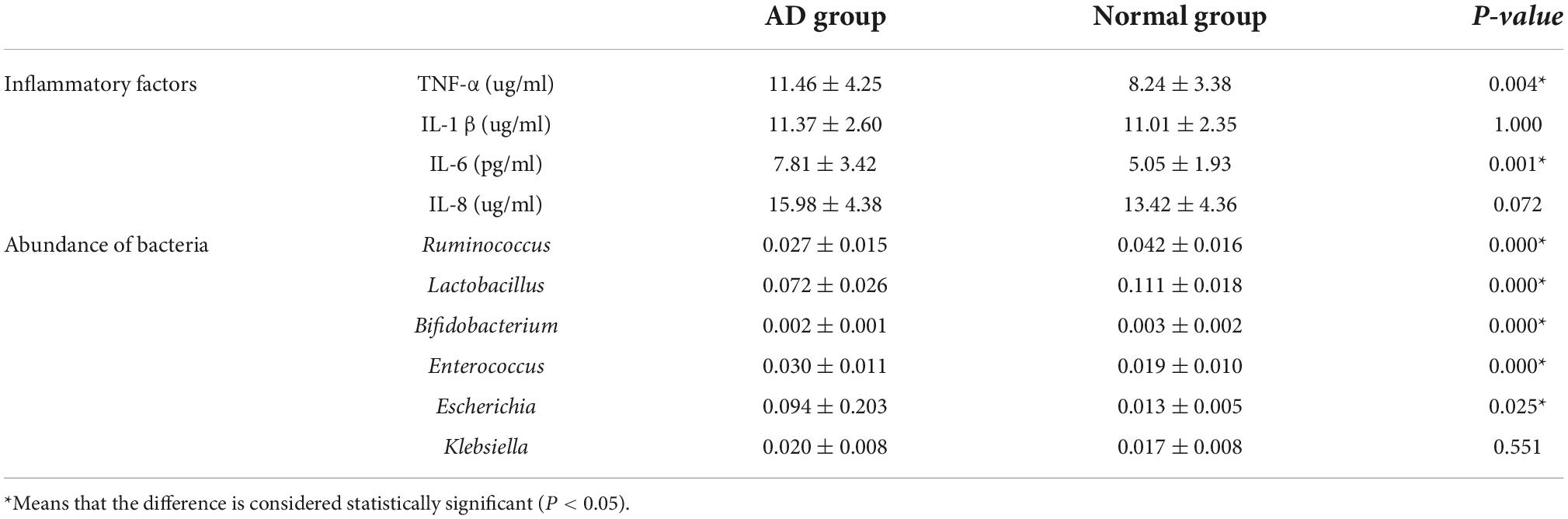
Table 5. Inflammatory factors and abundance of anti-inflammatory bacteria and pro-inflammatory bacteria in each group.
Discussion
Alzheimer’s disease is an age-related neurodegenerative disease, but its etiology and mechanism are not well-understood, and there is no effective treatment in the clinic. At present, the relevant drug treatment just can delay the progress of the disease to a certain extent. For a long time, the research on the pathogenesis of AD has never stopped. The relationship between intestinal flora, neuroinflammation, and AD occurrence and development is a hot topic.
Intestinal flora
A large number of microorganisms settle in the human body. It is estimated that there are 500∼1,000 kinds of bacteria in the human body (Gilbert et al., 2018), most of which settle in the intestine (Ursell et al., 2012). Intestinal microorganisms play a vital role in the host’s health, such as defeating invading pathogens, producing neurotransmitters, regulating the immune system and so on. Recent studies have shown that the occurrence and development of neurodegenerative diseases such as AD are related to changes in intestinal flora (Al Bander et al., 2020). Intestinal flora regulates the development and homeostasis of the central nervous system through immune, circulatory and neural pathways. In turn, the central nervous system shapes the intestinal microbial community through stress and endocrine response. The two-way effect of this gut-brain axis may be related to complex central nervous system diseases (Tremlett et al., 2017). The mechanism of intestinal flora leading to AD may begin with the imbalance of intestinal microbiota, the development of local and systemic inflammation, and the imbalance of the intestinal brain axis. The increase of intestinal epithelial barrier permeability leads to the invasion of bacteria, viruses, and their neuroactive products and causes the neuroinflammatory response of the brain, leading to AD (Sochocka et al., 2019). Our study brings valuable information about the correlation between intestinal flora and AD by exploring intestinal flora’s diversity and structural changes in patients with AD and normal cognition.
In this study, we sequenced the feces of AD patients and normal elderly patients. The sequencing depth of the two groups of samples was more than 99%. Alpha diversity analysis showed that the diversity of flora in AD patients was significantly lower than that in the normal group, suggesting that AD may be related to the decline of flora diversity to a certain extent. The flora structure analysis showed significant differences in the abundance of Bacteroidetes, Proteobacteria, and Firmicutes between the AD and normal groups. Vogt (Vogt et al., 2017) found that Firmicutes decreased and Bacteroidetes increased in the intestinal microbiota of AD patients, which was consistent with the results of this study. However, some studies have shown that Bacteroidetes in the intestinal microbiota of AD patients are reduced (Zhuang et al., 2018). The small sample size of these studies makes it difficult to draw consistent conclusions, so studies with larger samples are needed to provide more reliable evidence. Studies have shown that the ratio of Firmicutes to Bacteroidetes gradually decreases with age, accompanied by immune system disorders, and low-level intestinal inflammatory response gradually increases (Odamaki et al., 2016). Bacteroidetes belong to Gram-negative bacteria and can secrete a large amount of lipopolysaccharide. Lipopolysaccharide may act on Toll-like receptor four in leukocytes and microglia and promote nuclear factors κB mediated cytokine production, thereby, ncreasing Aβ Level, damaging oligodendrocytes and myelin sheath. At the same time, Aβ 1∼42 are also agonists of Toll-like receptor four, which can cause Aβ. The level increases, forming a vicious circle and aggravating AD’s progress (Zhan et al., 2018). Proteobacteria, which only account for a small part of the intestinal tract of healthy adults (Faith et al., 2013), once their abundance increases excessively, the ability of inherent internal bacteria to resist colonization by foreign pathogens will decrease, further promoting inflammatory response and pathogen invasion. Therefore, Proteobacteria enrichment is also considered to be a marker of intestinal flora disorder, with potential diagnostic value (Shin et al., 2015).
Alzheimer’s disease, inflammation, and intestinal flora
Many recent studies have found that immune and inflammatory control regulation is one of the related processes involved in the pathogenesis of neurodegenerative diseases. A large number of studies have shown that the participation of neuroinflammation plays a crucial role in the process of neuropathological changes observed in AD (Finneran and Nash, 2019). The key players currently known to be responsible for the induction of neuroinflammation are activated microglia and astrocytes (Ahmad et al., 2019). Long-term chronic inflammation and excessive microglia stimulation can increase the neuroinflammatory signal transduction of proinflammatory cytokines, reactive oxidation and nitrous stress sources, resulting in the death of neurons and glial cells and further promoting the occurrence and development of AD (Li et al., 2016). The inflammatory cytokines released by microglia in the elderly brain are mainly IL-1β, IL-6, and TNF-α (Ritzel et al., 2015).
We compared the levels of the TNF-α, IL-1β, IL-6, and IL-8 which are four proinflammatory factors in two groups of subjects. Results found that TNF-α and IL-6 levels are significantly higher than those in the normal group, which further confirm that inflammatory factors are involved in the occurrence of AD, especially TNF-α, IL-6.
TNF-α is a proinflammatory cytokine produced mainly by macrophages and monocytes and is involved in normal inflammatory and immune responses. The evidence found from previous studies (Di Bona et al., 2009; Swardfager et al., 2010) indicates that TNF-α is related to the pathogenesis of AD pathology and TNF-α is the primary signal molecule affecting memory and cognitive function (Kim et al., 2017). Previous studies have shown that overexpression of TNF-α can stimulate microglia to express inflammatory factors, thus promoting the formation of Aβ plaques (Dal Pra et al., 2008), causing neurotoxic cascade reactions (Wyss-Coray and Mucke, 2002), competing with neuro-nutrients, and leading to neuronal aging (Roux et al., 2001). In this study, we found that the level of TNF-α in AD group was significantly higher than that in the normal group. Combined with previous studies, it was further confirmed that TNF-α was involved in the occurrence and development of AD.
As an important member of the cytokine network, IL-6 can regulate the growth and differentiation of various cells, as well as the immune response, and participate in the pathological process of various clinical diseases. At the same time, IL-6 can promote the proliferation of T cells and B cells and participate in the body’s inflammatory response. Analysis in this study showed that IL-6 levels in AD patients were significantly higher than those in the normal group. This is consistent with previous meta-analyses (Swardfager et al., 2010). However, some studies have found that the increase of peripheral blood IL-6 level precedes the onset of Alzheimer’s disease rather than during the onset of Alzheimer’s disease (Uslu et al., 2012).
IL-1β is produced by macrophages, endothelial cells, and astrocytes. Overexpression of IL-1β can impair microglia Aβ scavenging function (Wang et al., 2015) and induce blood-brain barrier disruption, thereby increasing Aβ deposition (Wang et al., 2014). On the contrary, studies have shown that IL-1β released by microglia has a protective effect on AD (Ng et al., 2018). IL-1β not only reduces A β production by enhancing α-lysis of APP, but also alleviates amyloid lesions by increasing clearance mechanisms. Overexpression of IL-1β can enhance microglial phagocytosis and lead to plaque clearance (Matousek et al., 2012). In summary, IL-1 β plays a complex role in AD, and its expression and mechanism in AD need to be further studied.
Normal brain tissue can produce a certain amount of IL-8. Brain-derived IL-8 is involved in normal brain tissue metabolism and function. Peripheral IL-8 is involved in immune inflammation. Neurological diseases such as AD can inhibit the production of brain-derived IL-8, while inflammatory cytokines such as IL-1β and TNF-α produced by inflammation caused by neurological diseases can further induce the production of IL-8 by endothelial cells and neutrophils, leading to inflammatory chemotaxis and thus aggravating the damage of this region. Thus, IL-8 has a dual role. In this study, there was no significant difference in IL-1β and IL-8 levels between the two groups, which may be due to the complex mechanism of IL-1β and IL-8 on the one hand, and the small sample size, which needs to be further expanded to further confirm the role of IL-1β and IL-8 in the occurrence and progression of AD.
At the bacterial level, we selected some of the dominant flora in the gut, such as Bifidobacterium, Lactobacillus, Ruminococcusi with anti-inflammatory effects and Escherichia, Klebsiella, and Enterococcus with pro-inflammatory effects. Studies have shown that Escherichia includes a considerable number of pathogenic bacteria, which can cause intestinal inflammatory reactions under certain conditions (Wall et al., 2014; Potgieter et al., 2015). In the past, Enterococcus was considered harmless to the human body. In recent years, studies have confirmed that some Enterococcus have virulence genes in long-term evolution, which can cause extensive infection. Ruminococcus can produce short-chain fatty acids by degrading cellulose, and short-chain fatty acids are one of the energy sources of intestinal cells. They can maintain the homeostasis of intestinal flora, and carry out immune regulation and anti-inflammatory effects. Bifidobacterium can interact closely with intestinal mucosal epithelial cells to form a protective biological barrier, repel pathogenic bacteria through itself and its metabolites, and maintain the balance of intestinal function. Lactobacillus can inhibit the reproduction of spoilage bacteria and pathogenic bacteria in the intestine, have immune regulation, promote the production of antibodies, activate macrophages, induce interferon production, and improve the body’s disease-resistance. The imbalance of intestinal flora may lead to low reactive inflammation and produce inflammatory factors to activate microglia and then produce neuroinflammation, resulting in reducing cognitive ability and the occurrence of AD (Caracciolo et al., 2014). Animal experiments show that oral probiotics (Bifidobacterium lactis, Lactobacillus casei, Bifidobacterium, and Lactobacillus acidophilus) can significantly improve memory impairment, brain neuron and synaptic damage, glial cell activation, improve the composition of the flora in intestine and brain, reduce the destruction of intestinal barrier and blood-brain barrier, and reduce the level of lipopolysaccharide in plasma and brain, reduce the level of inflammatory factors in the brain and intestine at the level of messenger RNA (Yang et al., 2020). The results showed that the abundance of Lactobacillus and Bifidobacterium decreased and the abundance of Escherichia coli increased in the AD group. Compared with the normal group, the Ruminococcusi abundance in the AD group decreased and the fecal cocci abundance increased. It is confirmed again that the bacteria that can cause inflammatory reaction in the intestine of AD patients is higher than those in the normal groups, while the bacteria that inhibit inflammatory reaction were lower.
Summary
This study found that the diversity of intestinal flora in AD patients is significantly reduced, and the structural changes of intestinal flora significantly increased the abundance of bacteria that may lead to inflammatory response while significantly decreasing the abundance of bacteria that may inhibit the inflammatory response and maintain intestinal function, and increased the level of pro-inflammatory factors. Therefore, we suggest that the occurrence of AD may be related to the low level of the inflammatory response caused by the structural disorder of intestinal flora.
Advantages and limitations
Many previous studies only studied the changes of intestinal microbiota structure in AD patients or the levels of inflammatory factors in AD patients. Compared with previous correlation studies, the advantage of this study is that we statistically analyzed the structure of gut microbiota between AD patients and controls at both phylum level and genus level. In addition, this study also analyzed the differences in intestinal microbiota structure and inflammatory factor levels in AD patients, and clarified that changes in intestinal microbiota structure could further lead to the occurrence and development of AD by causing low-level inflammatory reaction by combining the two. This study also has some limitations: Firstly, the sample size of this study is relatively small; Secondly, this study did not compare and analyze the differences in gut microbiota structure and inflammatory factor levels among AD patients with different degrees of dementia.
Data availability statement
The original contributions presented in the study are publicly available. This data can be found here: doi: 10.6084/m9.figshare.21353970.
Ethics statement
The studies involving human participants were reviewed and approved by the Medical Ethics Committee of the Sixth Medical Center of the PLA General Hospital. The patients/participants provided their written informed consent to participate in this study. Written informed consent was obtained from the individual(s) for the publication of any potentially identifiable images or data included in this article.
Author contributions
S-SW and X-HL performed the experiments and data analysis and wrote the manuscript. PL designed and supervised the study and edited the manuscript. JL revised statistical analysis. LL critically revised the manuscript. All authors contributed to the article and approved the submitted version.
Funding
This work was supported by the project “Study on the relationship between senile dementia and intestinal microflora structure and inflammatory factors” (Health Bureau of the Logistics Support Department of the Military Commission 18BJZ36).
Conflict of interest
The authors declare that the research was conducted in the absence of any commercial or financial relationships that could be construed as a potential conflict of interest.
Publisher’s note
All claims expressed in this article are solely those of the authors and do not necessarily represent those of their affiliated organizations, or those of the publisher, the editors and the reviewers. Any product that may be evaluated in this article, or claim that may be made by its manufacturer, is not guaranteed or endorsed by the publisher.
References
Ahmad, M. H., Fatima, M., and Mondal, A. C. (2019). Influence of microglia and astrocyte activation in the neuroinflammatory pathogenesis of Alzheimer’s disease: Rational insights for the therapeutic approaches. J. Clin. Neurosci. 59, 6–11. doi: 10.1016/j.jocn.2018.10.034
Al Bander, Z., Nitert, M. D., Mousa, A., and Naderpoor, N. (2020). The gut microbiota and inflammation: An overview. Int. J. Environ. Res. Public Health 17:7618. doi: 10.3390/ijerph17207618
Alzheimer’s Association (2019). Alzheimer’s disease facts & figures. Alzheimers Dement. 15, 321–387. doi: 10.1016/j.jalz.2019.01.010
Alzheimer’s Disease International (2019). World Alzheimer Report 2019[EB/OL]. London: Alzheimer’s Disease International.
Caracciolo, B., Xu, W., Collins, S., and Fratiglioni, L. (2014). Cognitive decline, dietary factors and gut- brain interactions. Mech. Aging Dev. 13, 59–69. doi: 10.1016/j.mad.2013.11.011
Dal Pra, I., Chiarini, A., Pacchiana, R., Chakravarthy, B., Whitfield, J. F., Armato, U., et al. (2008). Emerging concepts of how β- amyloid proteins and pro-inflammatory cytokines might collaborate to produce an ‘Alzheimer brain. Mol. Med. Rep. 1, 173–178.
Di Bona, D., Candore, G., Franceschi, C., Licastro, F., Colonna-Romano, G., Cammà, C., et al. (2009). Systematic review by meta-analyses on the possible role of TNF-alpha polymorphisms in association with Alzheimer’s disease. Brain Res. Rev. 61, 60–68. doi: 10.1016/j.brainresrev.2009.05.001
Faith, J. J., Guruge, J. L., and Charbonneau, M. (2013). The long -term stability of the human gut microbiota. Science 341:1237439. doi: 10.1126/science.1237439
Finneran, D. J., and Nash, K. R. (2019). Neuroinflammation and fractalkine signaling in Alzheimer’s disease. J. Neuroinflammation 16:30. doi: 10.1186/s12974-019-1412-9
Gilbert, J. A., Blaser, M. J., Caporaso, J. G., Jansson, J. K., Lynch, S. V., Knight, R., et al. (2018). Current understanding of the human microbiome. Nat. Med. 24, 392–400. doi: 10.1038/nm.4517
Kim, Y. S., Lee, K. J., and Kim, H. (2017). Serum tumour necrosis factor-a and interleukin-6 levels in Alzheimer’s disease and mild cognitive impairment. Psychogeriatrics 17, 224–230. doi: 10.1111/psyg.12218
Li, C. Q., Zheng, Q., Wang, Q., and Zeng, Q. P. (2016). Biotic/abiotic stress-driven Alzheimer’s disease. Front. Cell. Neurosci. 10:269. doi: 10.3389/fncel.2016.00269
Livingston, G., Sommerlad, A., Orgeta, V., Costafreda, S. G., Huntley, J., Ames, D., et al. (2017). Dementia prevention, intervention, and care. Lancet 390, 2673–2734. doi: 10.1016/S0140-6736(17)31363-6
Luczynski, P., McVey Neufeld, K. A., Oriach, C. S., Clarke, G., Dinan, T. G., Cryan, J. F., et al. (2016). Growing up in a bubble: Using germ-free animals to assess the influence of the gut microbiota on brain and behavior. Int. J. Neuropsychopharmacol. 19:yw020. doi: 10.1093/ijnp/pyw020
Matousek, S. B., Ghosh, S., Shaftel, S. S., Kyrkanides, S., Olschowka, J. A., O’Banion, M. K., et al. (2012). Chronic IL-1 beta-mediated neuroinflammation mitigates amyloid pathology in a mouse model of Alzheimer’s disease without inducing overt neurodegeneration. J. Neuroimmune Pharmacol. 7, 156–164. doi: 10.1007/s11481-011-9331-2
Ng, A., Tam, W. W., Zhang, M. W., Ho, C. S., Husain, S. F., McIntyre, R. S., et al. (2018). IL-1 β, IL-6, TNF- α and CRP in elderly patients with depression or Alzheimer’s disease: Systematic review and meta-analysis. Sci. Rep. 8:12050. doi: 10.1038/s41598-018-30487-6
Nguyen, T. T., Ta, Q. T. H., Nguyen, T. T. D., Le, T. T., and Vo, V. G. (2020). Role of insulin resistance in the Alzheimer’s disease progression. Neurochem. Res. 45, 1481–1491. doi: 10.1007/s11064-020-03031-0
Odamaki, T., Kato, K., Sugahara, H., Nanami, H., Jin-Zhong, X., Sachiko, T., et al. (2016). Age-related changes in gut microbiota composition from newborn to centenarian: A cross-sectional study. BMC Microbiol. 16:90. doi: 10.1186/s12866-016-0708-5
Picca, A., Fanelli, F., Calvani, R., Mulè, G., Pesce, V., Sisto, A., et al. (2018). Gut dysbiosis and muscle aging: Searching for novel targets against sarcopenia. Mediators Inflamm. 2018:7026198. doi: 10.1155/2018/7026198
Potgieter, M., Bester, J., Kell, D. B., and Pretorius, E. (2015). The dormant blood micro-biome in chronic, inflammatory diseases. FEMS Microbiol. Rev. 39, 567–591. doi: 10.1093/femsre/fuv013
Ritzel, R. M., Patel, A. R., Pan, S., Crapser, J., Hammond, M., Jellison, E., et al. (2015). Age-and location-related changes in microglial function. Neurobiol. Aging 36, 2153–2163. 2015.02.016 doi: 10.1016/j.neurobiolaging
Roux, P. P., Bhakar, A. L., Kennedy, T. E., and Barker, P. A. (2001). The p75 neurotrophin receptor activates Akt (protein kinase B) through a phosphatidylinositol 3-kinase-dependent pathway. J. Biol. Chem. 276, 23097–23104. doi: 10.1074/jbc.M011520200
Schneider, S., Wright, C. M., and Heuckeroth, R. O. (2019). Unexpected roles for the second brain: Enteric nervous system as master regulator of bowel function. Annu. Rev. Physiol. 81, 235–259. doi: 10.1146/annurev-physiol-021317-121515
Shin, N. R., Whon, T. W., and Bae, J. W. (2015). Proteobacteria: Microbial signature of dysbiosis in gut microbiota. Trends Biotechnol. 33, 496–503. doi: 10.1016/j.tibtech.2015.06.011
Sochocka, M., Donskow-Lysoniewska, K., Diniz, B. S., Kurpas, D., Brzozowska, E., Leszek, J., et al. (2019). The Gut microbiome alterations and inflammation-driven pathogenesis of Alzheimer’s disease-a critical review. Mol. Neurobiol. 56, 1841–1851. doi: 10.1007/s12035-018-1188-4
Swardfager, W., Lanctot, K., Rothenburg, L., Wong, A., Cappell, J., Herrmann, N., et al. (2010). A metaanalysis of cytokines in Alzheimer’s disease. Biol. Psychiatry 68, 930–941. doi: 10.1016/j.biopsych.2010.06.012
Swerdlow, R. H. (2018). Mitochondria and Mitochondrial Cascades in Alzheimer’s Disease. J. Alzheimers Dis. 62, 1403–1416. doi: 10.3233/JAD-170585
Tremlett, H., Bauer, K. C., Appel-Cresswell, S., Finlay, B. B., and Waubant, E. (2017). The gut microbiome in human neurological disease: A review. Ann. Neurol. 81, 369–382. doi: 10.1002/ana.24901
Ursell, L. K., Metcalf, J. L., Parfrey, L. W., and Knight, R. (2012). Defining the human microbiome. Nutr. Rev. 70:S38–S44. doi: 10.1111/j.1753-4887.2012.00493.x
Uslu, S., Akarkarasu, Z. E., Ozbabalik, D., Ozkan, S., Colak, O., Demirkan, E. S., et al. (2012). Levels of amyloid beta-42, interleukin-6 and tumor necrosis factor-alpha in Alzheimer’s disease and vascular dementia. Neurochem. Res. 37, 1554–1559. doi: 10.1007/s11064-012-0750-0
Vogt, N. M., Kerby, R. L., Dill-McFarland, K. A., Harding, S. J., Merluzzi, A. P., Johnson, S. C., et al. (2017). Gut microbiome alterations in Alzheimer’s disease. Sci. Rep. 7:13537. doi: 10.1038/s41598-017-13601-y
Wall, R., Cryan, J., and Ross, R. P. (2014). “Bacterial Neuroactive Com-pounds Produced by Psychobiotics,” in Microbial Endocrinology: The Microbiota- Gut- Brain Axisin Health and Disease, eds M. Lyte and J. F. Cryan (New York, NY: Springer), 221–239. doi: 10.1007/978-1-4939-0897-4_10
Wang, W. Y., Tan, M. S., Yu, J. T., and Tan, L. (2015). Role of pro-inflammatory cytokines released from microglia in Alzheimer’s disease. Ann. Transl. Med. 3:136.
Wang, Y., Jin, S., Sonobe, Y., Cheng, Y., Horiuchi, H., Parajuli, B., et al. (2014). Interleukin-1beta induces blood-brain barrier disruption by downregulating Sonic hedgehog in astrocytes. PLoS One 9:e110024. doi: 10.1371/journal.pone.0110024
Watermeyer, T., and Calia, C. (2019). Neuropsychological assessment in preclinical and prodromal Alzheimer disease: A global perspective. J. Glob. Health 9:010317. doi: 10.7189/jogh.09.010317
Wyss-Coray, T., and Mucke, L. (2002). Inflammation in neurodegenerative disease-a double-edged sword. Neuron 35, 419–432. doi: 10.1016/S0896-6273(02)00794-8
Yang, X., Yu, D., Xue, L., Li, H., and Du, J. (2020). Probiotics modulate the microbiota-gutbrain axis and improve memory deficits in aged SAMP8 mice. Acta Pharm. Sin. B 10, 475–487. doi: 10.1016/j.apsb.2019.07.001
Zhan, X., Stamova, B., and Sharp, F. R. (2018). Lipopolysaccharide associates with amyloid plaques, neurons and oligodendrocytes in Alzheimer’ s disease brain: A review. Front. Aging Neurosci. 10:42. doi: 10.3389/fnagi.2018.00042
Zhao, Y., and Gong, C. X. (2015). From chronic cerebral hypoperfusion to Alzheimer-like brain pathology and neurodegeneration. Cell. Mol. Neurobiol. 35, 101–110. doi: 10.1007/s10571-014-0127-9
Keywords: Alzheimer’s disease, intestinal microflora, inflammatory factors, TNF-α, IL-6
Citation: Wang S-s, Li X-h, Liu P, Li J and Liu L (2022) The relationship between Alzheimer’s disease and intestinal microflora structure and inflammatory factors. Front. Aging Neurosci. 14:972982. doi: 10.3389/fnagi.2022.972982
Received: 19 June 2022; Accepted: 15 September 2022;
Published: 09 November 2022.
Edited by:
Rodrigo Morales, University of Texas Health Science Center at Houston, United StatesReviewed by:
Bhanu Ganesh, University of Texas Health Science Center at Houston, United StatesTakuichi Sato, Niigata University, Japan
Cristian Bonvicini, San Giovanni di Dio Fatebenefratelli Center (IRCCS), Italy
Copyright © 2022 Wang, Li, Liu, Li and Liu. This is an open-access article distributed under the terms of the Creative Commons Attribution License (CC BY). The use, distribution or reproduction in other forums is permitted, provided the original author(s) and the copyright owner(s) are credited and that the original publication in this journal is cited, in accordance with accepted academic practice. No use, distribution or reproduction is permitted which does not comply with these terms.
*Correspondence: Ping Liu, ZHJfbGl1QDE2My5jb20=
†These authors share first authorship