- 1College of Rehabilitation Sciences, Shanghai University of Medicine and Health Sciences, Shanghai, China
- 2School of Sports Sciences, Qufu Normal University, Qufu, China
- 3The Affiliated High School of Qufu Normal University, Qufu, China
Background: Mild cognitive impairment (MCI) is a precursor to dementia, and neuroinflammation in the brain is thought to be one of the main pathogenic mechanisms of MCI. However, the underlying neurobiological mechanisms have not been fully explored. The purpose of this study was to establish a visual model map of the articles in the field of neuroinflammation-induced MCI over the past 11 years to reveal the research hotspots and predict the future development trends in this field, which will help to promote the research and development for MCI.
Methods: The “neuroinflammation” and “mild cognitive impairment” were used as search terms, and literature about neuroinflammation-induced MCI published between 2011 and 2021 was collected from the Web of Science. CiteSpace and VOSviewer were used to create visual model maps, and assess collaboration among different authors, countries, and institutions. Finally, the current research hotspots and future research directions were analyzed by using high-frequency keywords analysis and co-cited reference burst analysis.
Results: A total of 226 articles were retrieved. The number of publications in neuroinflammation-induced MCI shows an upward trend. Since 2018, the number of papers published in this field has increased significantly, with an average of more than 100 published each year. The United States had the highest literature production and the number of cited journals in this research area, and the National Institute on Aging was the most productive research institution. Brooks D.J. and Heneka M.T. had the highest number of publications and had the highest frequency of co-citations. The co-cited references revealed the evolution of the research themes, and the current studies are mainly focused on the effects of various metabolites on the control of microglial activation. “Cerebrospinal fluid,” “mouse model,” “tau,” “microglial activation,” “astrocytes,” and “TREM2” were the current high-frequency and emerging keywords.
Conclusion: Research on neuroinflammation-induced MCI is burgeoning, and the close collaboration with different nations and institutions need to be further strengthened. Current research hotspots are focused on the effects of various metabolites on microglia activation. Future studies should focus on how to regulate the phenotypes of microglia and astrocyte to reduce neuroinflammation and treat MCI.
Introduction
Mild cognitive impairment (MCI) is a risk precursor to dementia, which has a high conversion rate to dementia, especially Alzheimer’s disease (AD) (Liu et al., 2021). There is no effective treatment for AD, and timely intervention during MCI could significantly reduce the prevalence of AD. Neuroinflammation is a brain’s defense mechanism, which initially protects the brain by removing or suppressing various pathogens. However, persistent inflammation is detrimental because it inhibits neurogenesis, and it is also a significant driver of MCI onset and progression to AD (Kwon and Koh, 2020; Zhou et al., 2021).
The primary cause of neuroinflammation is the activation of microglia and astrocytes. Microglia are resident immune cells of the central nervous system (CNS) and play an essential role in tissue homeostasis. Once activated, microglia can be categorized into M1 pro-inflammatory phenotype and M2 anti-inflammatory phenotype. Although this classification may be too simplistic, it still helps to illustrate the two primary functional features of microglia (Ransohoff, 2016). In endogenous or exogenous pathological injury, activated microglia transform into M1 or M2 phenotypes. Under normal physiological conditions, M1-type microglia secrete pro-inflammatory factors that enhance cytotoxicity to promote synaptic pruning or kill foreign pathogens, and M2-type microglia promote inflammation resolution and tissue healing by sensing injury signals and secreting anti-inflammatory neuroprotective factors. This dynamic balancing process is beneficial for maintaining synaptic plasticity (Bajetto et al., 2002; Owens et al., 2014; Solé-Domènech et al., 2016). As for astrocytes, their functions are analogous to housekeeping, including forming the outer layer of the blood-brain barrier (BBB), regulating blood flow, and providing energy metabolites for neurons. Moreover, astrocytes are also important modulators of neuroinflammation in the CNS, secreting pro-inflammatory (A1 phenotype) or anti-inflammatory (A2 phenotype) cytokines depending on the stimulus. When inflammation occurs, the pro-inflammatory A1 phenotype is activated via the NF-κB pathway, producing pro-inflammatory factors that may contribute to pathogen and toxin clearance. But once A1 astrocytes lose their homeostatic function, they will secrete soluble toxins to kill neurons and oligodendrocytes. What toxin is responsible for this killing effect is yet to be further investigated (Huang et al., 2017). By contrast, cerebral ischemia induces the A2 phenotype through the signal transducer and activator of the transcription 3 (STAT3) pathway, which exerts neuroprotective effects by releasing neurotrophic factors (Colonna and Brioschi, 2020; Giovannoni and Quintana, 2020). These processes typically stop after immune stimulation is eliminated.
However, it may be that the dynamic balance is disrupted due to the chronic inflammatory challenges or the presence of microglia dysfunction in the aging brain, which leads to the continued activation of microglia, mediating astrocyte toxicity and exacerbating inflammation response (Norden and Godbout, 2013; Spittau, 2017). Therefore, it is crucial to understand the role of neuroinflammation in the development of MCI, and as a target for therapeutic interventions.
Research on neuroinflammation-induced MCI has expanded rapidly in recent years, but the research hotspots and emerging research directions are challenging to identify. Therefore, we combed the articles in the field of neuroinflammation-induced MCI in recent 11 years, tried to establish a visual model for the study of neuroinflammatory MCI and analyze the current research hotspots, explain the evolution path of the subject in this field, and predict the future research direction. Findings from this study will not only provide a holistic picture and valuable insights on neuroinflammation-induced MCI for authors and researchers, but also a useful reference for future studies in managing neuroinflammation and treating MCI.
Data sources and analysis methods
Data sources
In the core database of Web of Science (WoS), 845 relevant articles published between 2011 and 2021 were initially identified using the keywords “mild cognitive impairment” and “neuroinflammation.” The visualization analysis revealed that the literature related to the search terms was mainly focused in the fields of Neuroscience, Clinical Neurology, Immunology, Cell Biology, Geriatrics Gerontology, and Biochemistry Molecular Biology. The literature types were limited to experimental and review papers. Irrelevant literature, including visualization analysis and meta-analyses, was excluded by browsing the literature title and abstract. Finally, 226 articles meeting the inclusion criteria were selected and extracted from the WoS database. Figure 1 shows the flow chart of the literature screening.
In addition, the function of Create Citation Report in the WoS database was used for citation analysis. The sum of the number of citations and the average number of citations per item were recorded. These records, including the titles, abstracts, and cited references, were exported to CiteSpace and VOSviewer for subsequent analysis. The impact factors (IFs) of the sources were obtained from Journal Citation Reports.
Analysis methods
CiteSpace 5. 7. R1 was developed by Dr. Chaomei Chen of Drexel University as information visualization software to identify major scientific institutions and authors, subject hotspots, and research frontiers in the scientific literature (Chen, 2004). The text data format was exported from WoS and pre-processed, then they were imported into CiteSpace for visualization and analysis. In Citespace’s visualizations map, the outermost purple ring represents the centrality of each node. The thicker the purple ring, the higher the centrality. The red ring represents a burst in a certain year. The color of the node represents a single time slice. The size of nodes and lines, respectively, represent the number of projects and the intensity of cooperation and co-occurrence.
VOSviewer 1.6.15 is free JAVA-based software developed by van Eck and Waltman of the Centre for Scientific and Technological Research at Leiden University, the Netherlands, in 2009 (van Eck and Waltman, 2010). The tab data format was exported from WoS and preprocessed, and then the data was imported into VOSviewer for visualization and analysis. In VOSviewer’s visualizations map, the size of nodes and lines represent the number of projects and the intensity of cooperation and co-occurrence, respectively. The color indicates individual time slices or the number of clusters. This study analyzed the collaborative relationships among authors, journals, the current development status, and the clustering analysis of keywords.
Results
Annual growth trends and basic information on publications
A total of 845 publications were identified in the WoS core database. Figure 2A shows the annual output of publications on neuroinflammation-induced MCI since 2011. The number of publications in this field has been increasing each year, and the number of publications in the past 3 years accounts for almost 50 percent of the total publications. In addition, the annual citations shows an uneven distribution. In 2015, although the number of publications was only 57, the number of citations was 6,015, the largest contributor was an article with 1,092 citations from the journal nature of Neuroscience, which belongs to medical region I and has an IF of approximately 33. Finally, the growth trend model (R2 = 0.9647) in Figure 2B predicts that approximately 156 articles on neuroinflammation-induced MCI will be published in 2022, indicating that this field continues to attract research interest from scholars and is one of the research hotspots in neurodegenerative diseases.
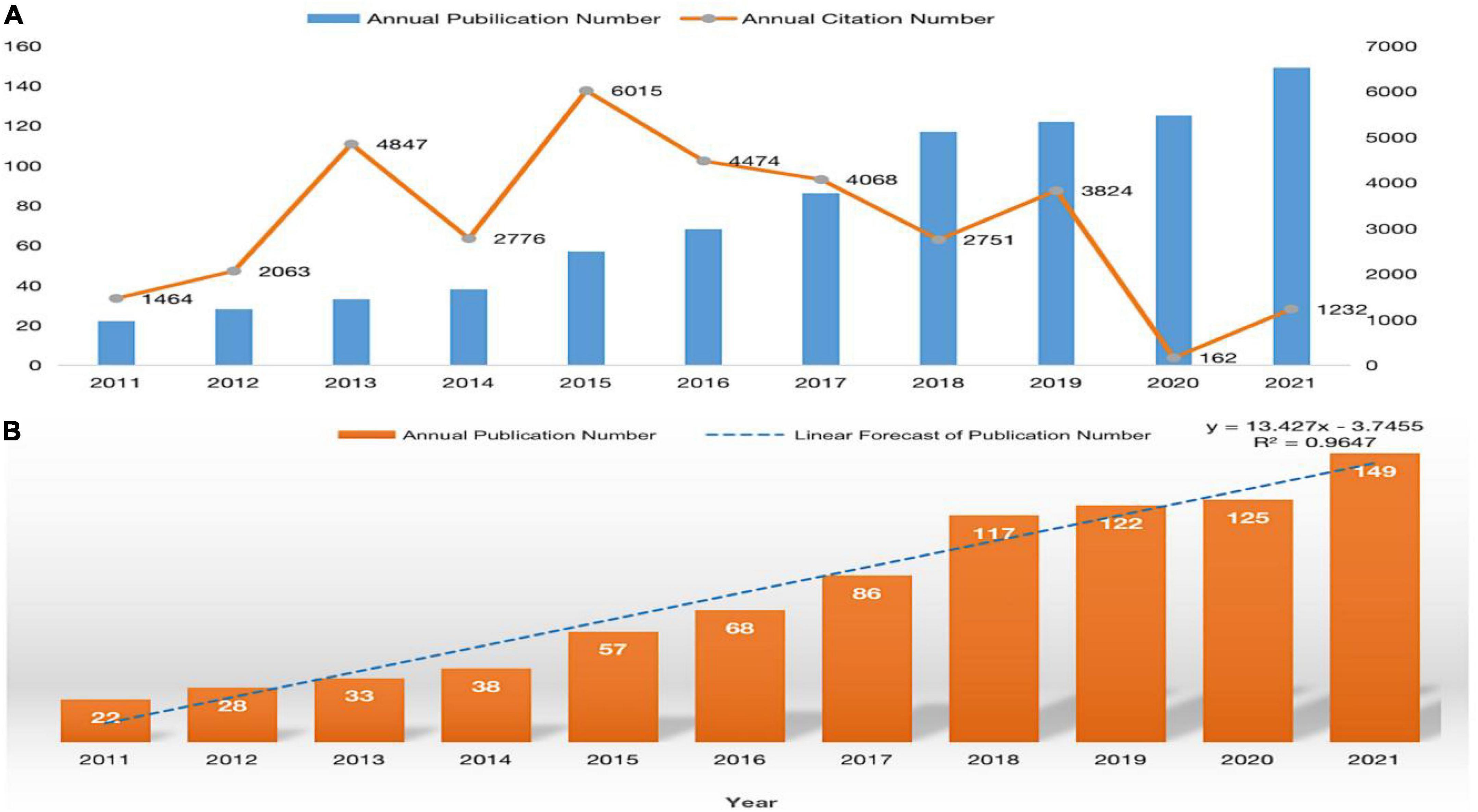
Figure 2. (A) The distribution of annual publications and citations on neuroinflammation-induced MCI, (B) publication forecast chart.
Analysis of country cooperation and volume of articles issued
Many countries have contributed to the field of neuroinflammation-induced MCI. We conducted a collaborative country network knowledge mapping study using CiteSpace for articles published from 2011 to 2021. As shown in Figure 3, only a few countries worked closely together, and most countries just stepped into this field of research. Figure 3 and Table 1 show that the United States was the leader in the number of publications, centrality, and citations. The US was followed by the United Kingdom, which ranked second in terms of the number of publications and average citations. China ranked third for the number of publications and second for centrality, and Australia was ranked last for the number of publications but had the highest average number of citations.
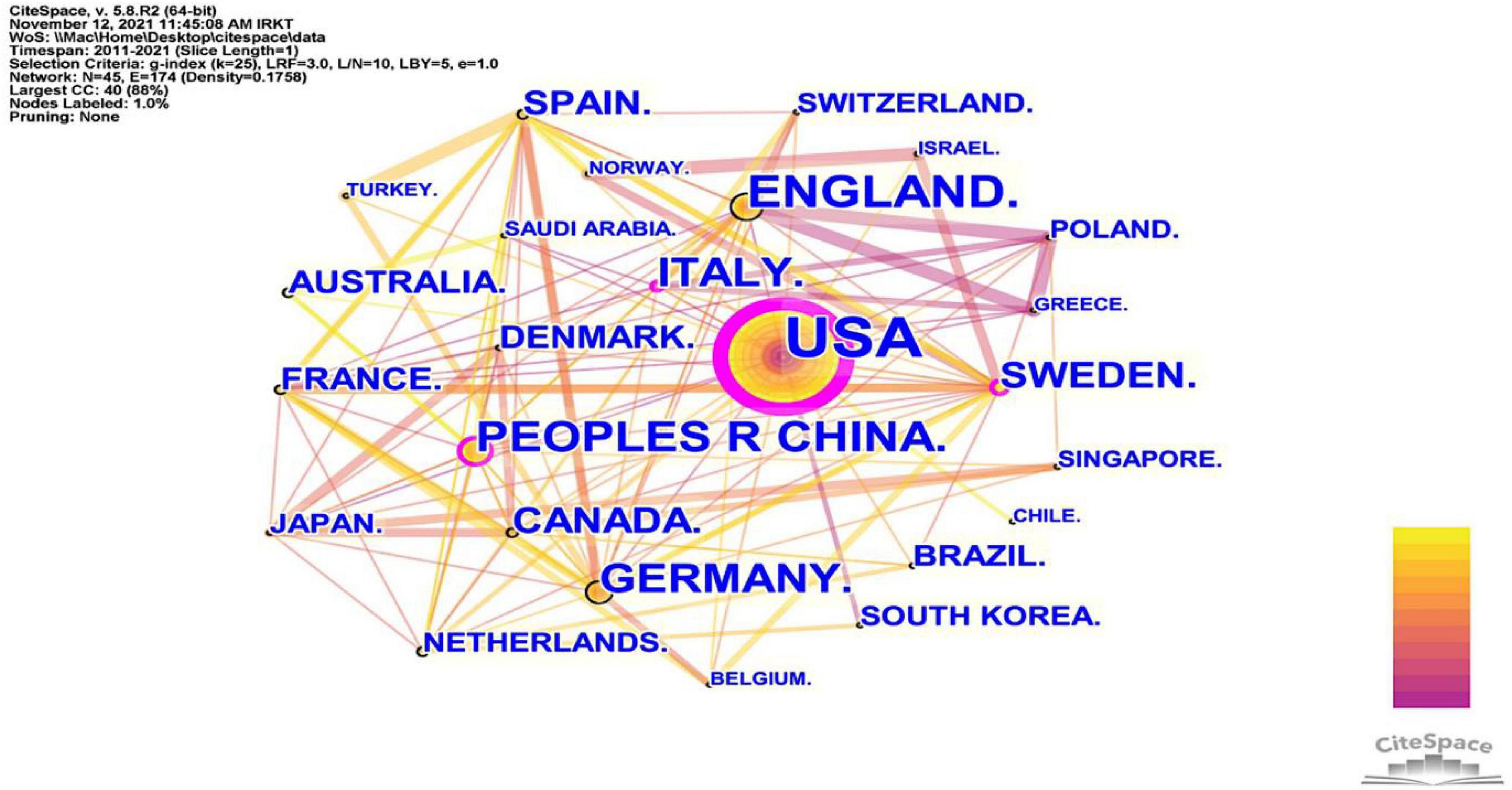
Figure 3. The cooperation network visualizations map of countries and regions created using CiteSpace. Node and line size represent the number of publications from a country and the cooperative relationship in the country, respectively. Color represents a single time slice. The diameter of the purple outer ring represents the size of the centrality.
Analysis of author and co-author collaboration and volume of publications
Since 2011, 1,483 authors have participated in research in the field of neuroinflammation-induced MCI. In Figure 4A, VOSviewer’s author collaboration mapping revealed seven collaborations between cited authors who were not closely related, and the publications were mainly concentrated between 2016 and 2019. Table 2 shows the chief five authors and co-authors. The top author was Brooks D.J. from Denmark (h index = 130) with 10 publications, the color around this name in Figure 4B is the darkest, indicating that this author had the highest number of publications. The second- and third-ranked authors were Edison P. and Blennow K., respectively, both with eight publications. The top co-cited authors were Heneka M.T., Petersen R.C., and Jack C.R. Heneka M.T., they contributed the most in this field, and the representative work “Neuroinflammation in Alzheimer’s disease” was the most frequently cited paper in the search results, this paper was published in 2015 in a top journal, Lancet Neurology.
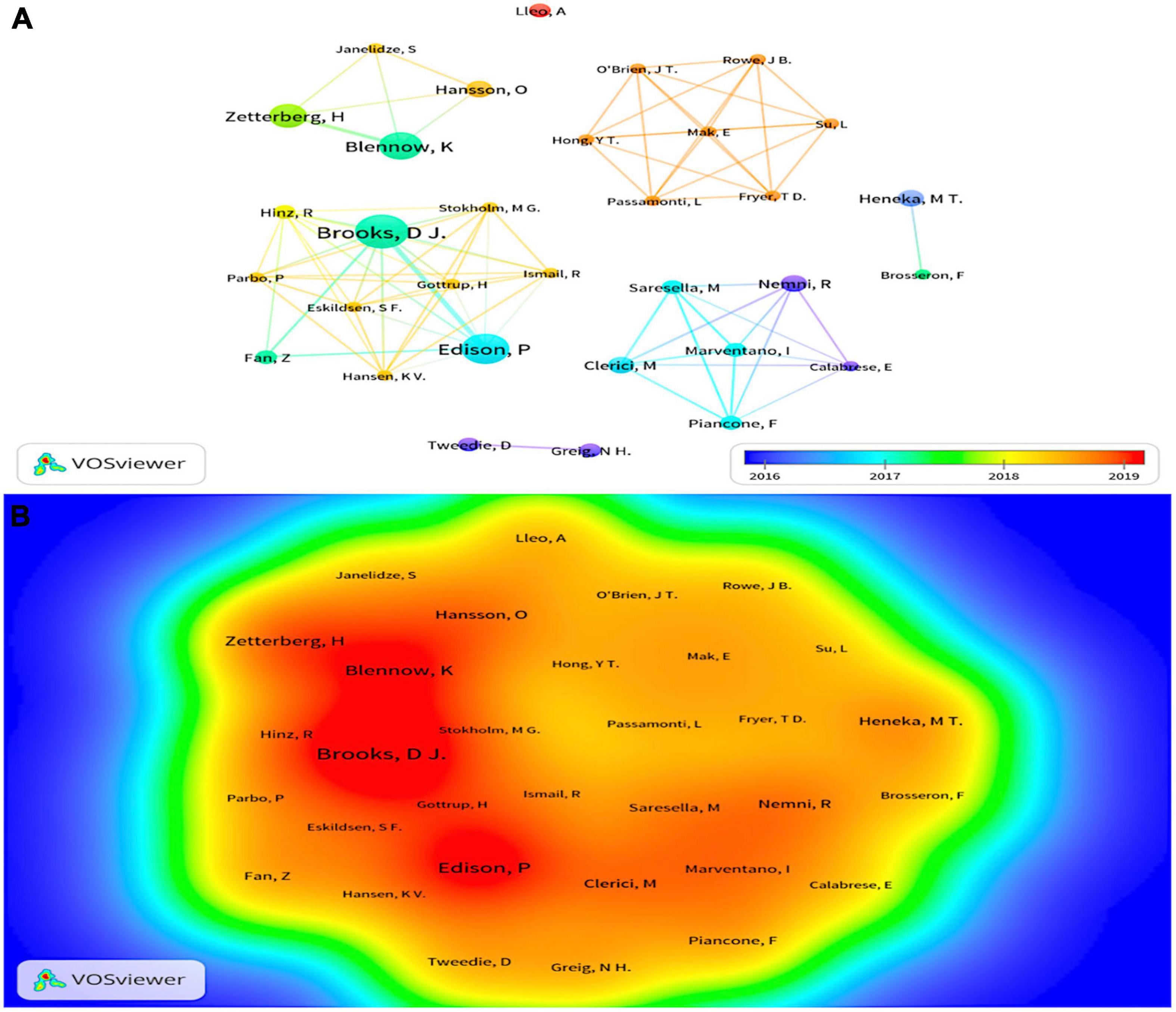
Figure 4. (A) The cluster visualization map of cited authors created using VOSviewer from 2016 to 2019, (B) the density visualization map of cited authors created using VOSviewer. Node size and color represents total number of citations and a single time slice, respectively. The darker the color, the more publications.
Analysis of journal collaboration and volume of publications
A total of 57 journals related to the field of neuroinflammation-induced MCI were included in this data analysis. Figure 5 shows the journal collaboration mapping created using VOSviewer, which shows that the Journal of Neuroinflammation and Journal of Alzheimer’s Disease had the highest volume of relevant published literature. The top five journals in terms of the number of publications are listed in Table 3. The top journal was the British Journal of Neuroinflammation (IF = 8.322), with 31 publications related to neuroinflammation and MCI focusing on components related to innate immune responses in the central nervous system, including microglia, astrocytes, and various cytokines and chemokines. These immune responses, which are central to the pathogenesis of various neurological disorders, are an area of notable research advances. The Journal of Neuroinflammation was followed by the British journal Brain (IF = 13.501) and the Dutch Journal of Alzheimer’s Disease (IF = 4.472).
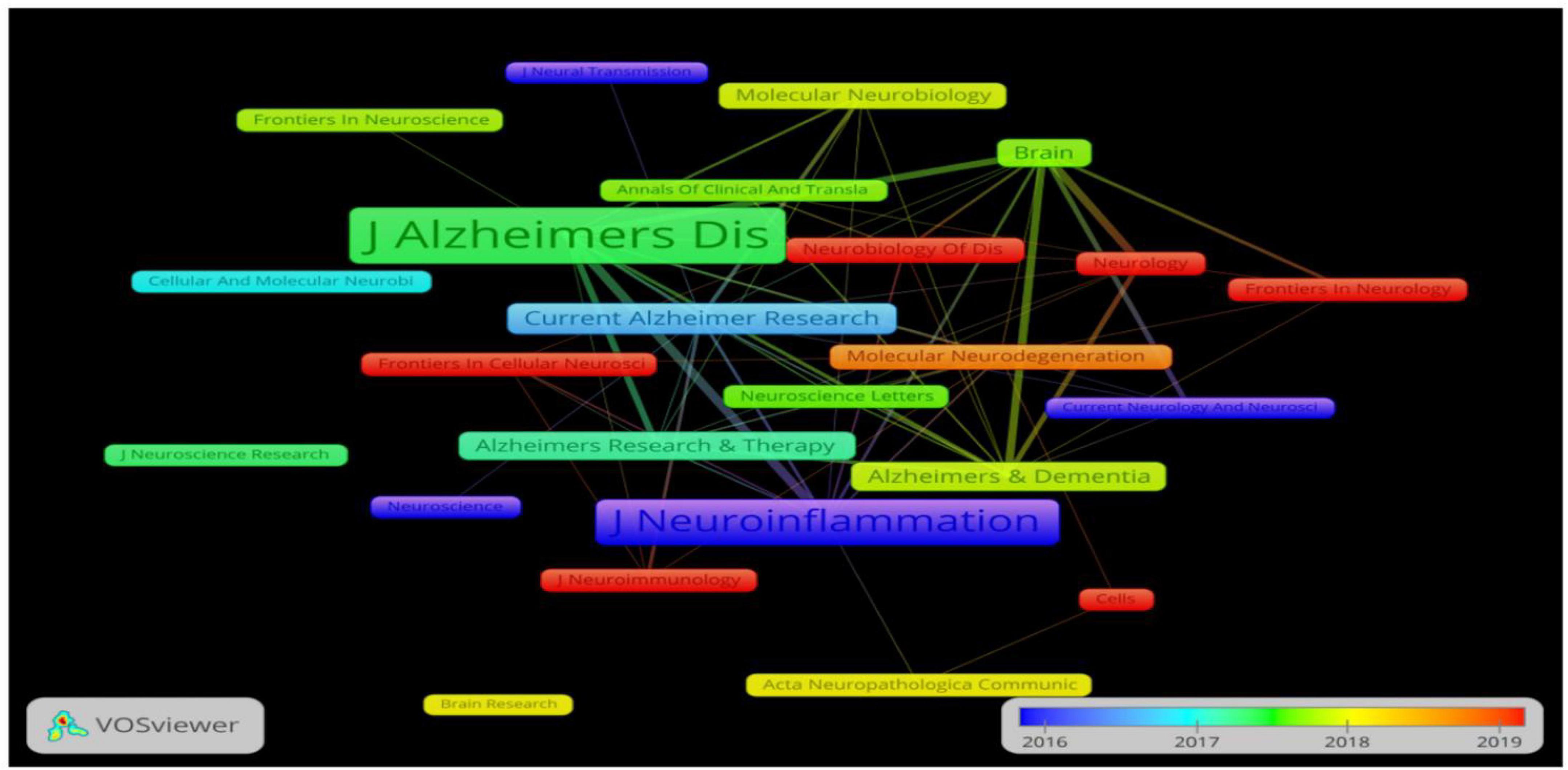
Figure 5. The cooperation network visualization map of journals created using VOSviewer for articles published from 2016 to 2019. Node size and color represents total number of citations and a single time slice, respectively.
Analysis of institutions’ collaboration and volume of publications
This study included publications from 527 institutions, and the inter-institutional linkages were visually analyzed using CiteSpace software. The information on communication and collaboration between different institutions is presented in Figure 6. The results show that Albany Medical College, AD Drug Discovery Foundation, and Acumen Pharmaceuticals have the most clearly defined purple outer circles. The affiliated institutions are distributed around them, indicating that these three institutions had higher centrality than other institutions. To understand the details of the published articles of these institutions, these institutions were ranked in terms of the number of published articles. And the top five institutions were selected and listed in Table 4. This table shows that the NIA was ranked first, with ten published articles and a centrality of 0.09. Three of the top five institutions are in the United States, suggesting that many institutions in the United States are engaged in research on neuroinflammation and MCI.
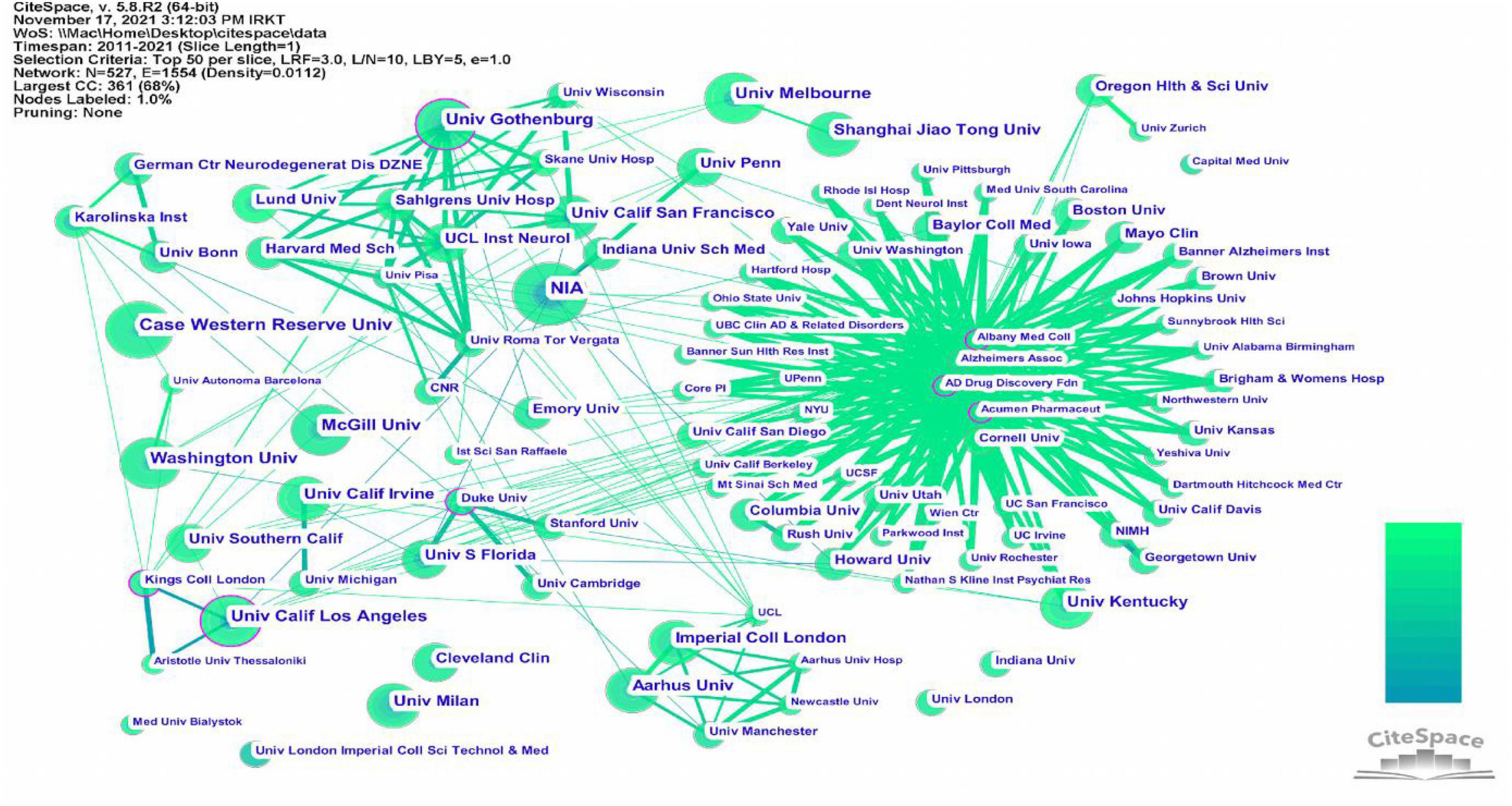
Figure 6. The cooperation network visualizations map of institutions created using CiteSpace. Node and line size represent the number of publications from an institution and the cooperative relationship in the institution, respectively. Color represents a single time slice. The diameter of the purple outer ring represents the size of the centrality.
Analysis of changes in research themes
Reference co-citation analysis is essential for analyzing trends and identifying research opportunities in a given field. For this purpose, we used CiteSpace to analyze co-cited references. In Table 5, the top five references selected include three reviews and two human experiments, and all of these articles are related to Alzheimer’s disease and neuroinflammation.
To better understand the current research themes and predict future directions in the field of neuroinflammation-induced MCI, we created a timeline diagram based on CiteSpace for the period 2011–2021 (Figure 7). This diagram is a knowledge network graph with 445 nodes and 1,547 links. A total of 10 reference clusters are listed in the figure, and their ranking is determined by the amount of literature contained. The clusters are described in Table 6, each group was assigned a silhouette value ranging from –1 to 1, and a value greater than 0.7 indicated that the cluster had a good range of identification.
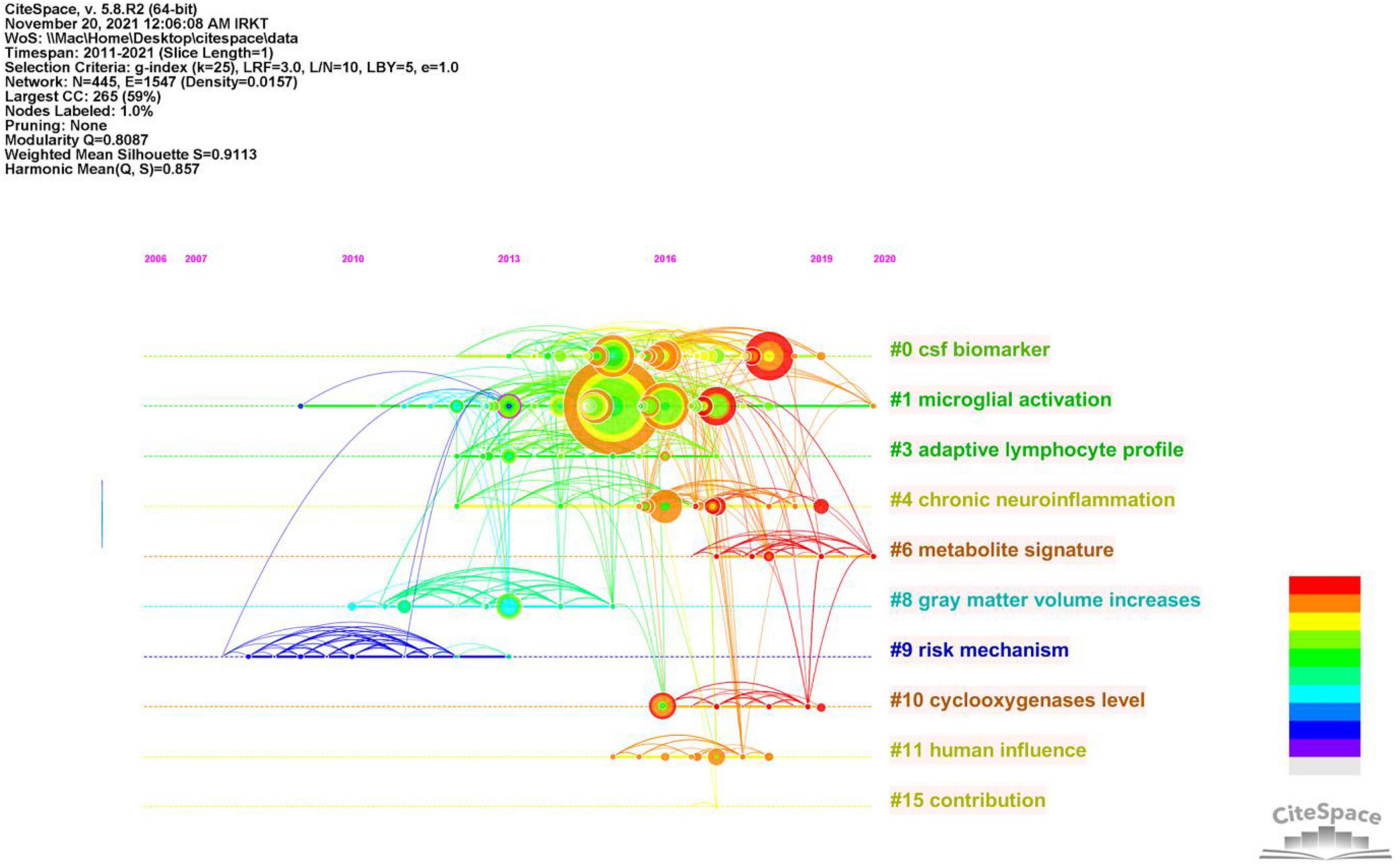
Figure 7. Timeline of co-citated document clusters from 2011 to 2021. Node size and color represents total number of citations and a single time slice, respectively. Lines of different colors show that the two articles were co-cited in an article.
Figure 8 and Table 7 show that the main research topics of MCI and neuroinflammation were related to studying various cell-intrinsic molecular mechanisms and metabolites. As shown in Figure 8, the publications in Cluster 0 were associated with cerebrospinal fluid biomarkers, and 55 references were included in this cluster. Biologically relevant cerebrospinal fluid markers have been highly researched in combination with time. Studies have found that elevated levels of inflammation-related features are detected in the cerebrospinal fluid and serum in patients with MCI and AD patients [e.g., YKL-40, interleukin (IL)-8, TREM2, and monocyte chemotactic protein-1 (MCP-1)] (Bowman et al., 2018; Nordengen et al., 2019).
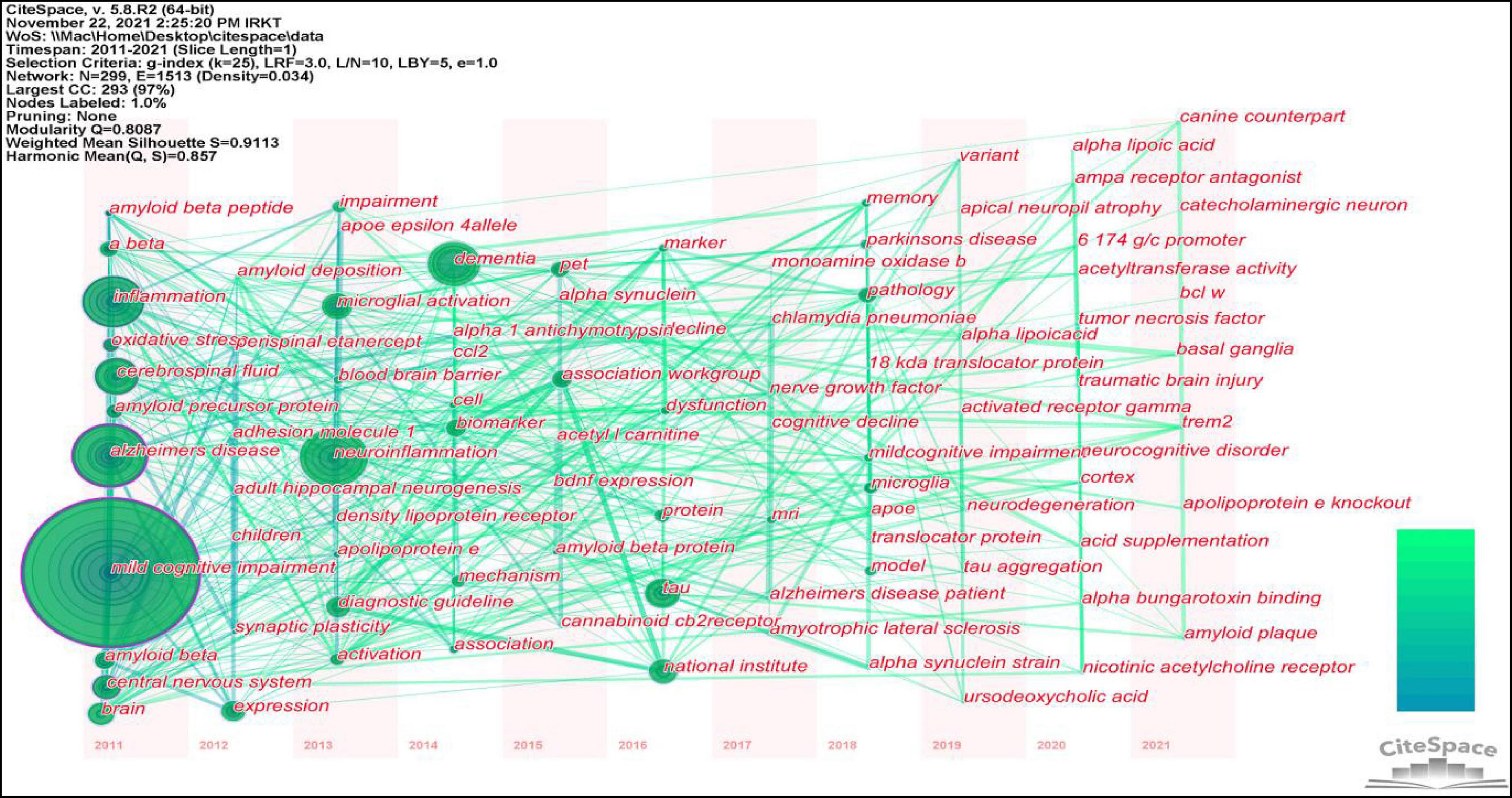
Figure 8. The co-citation network visualizations map of keywords created using VOSviewer. Node size and color represents the number of keywords and cluster.
The focus of publications in Cluster 1 was microglia activation (the primary driver of neuroinflammation-induced MCI), which was the most heavily studied topic from 2013 to 2018 and became a hot research topic again in 2020.
The research in Cluster 3 focused on neuroinflammation and adaptive immune cells. Although there have been few studies on adaptive immune cells in recent years, their role cannot be ignored. One study identified CD4 T cells in the brains of healthy mice, and these cells promoted the development and maturation of microglia (Pasciuto et al., 2020). In mouse models, therapeutic depletion of B cells at disease onset delayed AD progression, suggesting that treatment targeting B cells may benefit patients with AD (Kim et al., 2021). The close functional link between the immune and the central nervous systems has increased recognition.
Publications in Cluster 4 focused on Neuroinflammation, which plays a vital role in neurodegenerative diseases. In the earliest stages, the initiation of glial cells, the release of pro-inflammatory factors, and the vicious cycle of neuronal damage cause irreversible damage; therefore, identifying the neural mechanisms of neuroinflammation-induced degenerative diseases is crucial for solving the problem (Needham et al., 2022).
Publications in Cluster 10 studied elevated levels of cyclic oxygenase (COX-1/2). COX-1/2 is an essential modulator of brain plasticity, but its overexpression in neurons can increase the progression of brain injury and mediate the expression of other inflammatory factors, accelerating neuronal death (Andreadou et al., 2021).
Analysis of keywords
Research hotspot analysis
Table 6 shows that the keywords with the highest frequency were mild cognitive impairment, Alzheimer’s disease, neuroinflammation, inflammation, cerebrospinal fluid, mouse model, tau, central nervous system, and microglial activation.
This study identified 1,466 keywords, and the keyword clustering was visualized using VOSviewer (Figure 9). It was noted that many of the keywords shown in Figure 8 appeared in highly co-cited references. The keywords were categorized into red, green, purple, and yellow clusters, representing four research directions. The main keywords in the red cluster are MCI, AD, A-beta, oxidative stress, and central nervous system. The main keywords in the purple cluster are tau, biomarkers, dementia, and cerebrospinal fluid, the main keywords in the green cluster are neuroinflammation, microglial activation, brain, memory, and PET, and the main keywords of the yellow cluster mainly are astrocytes and neurodegeneration.
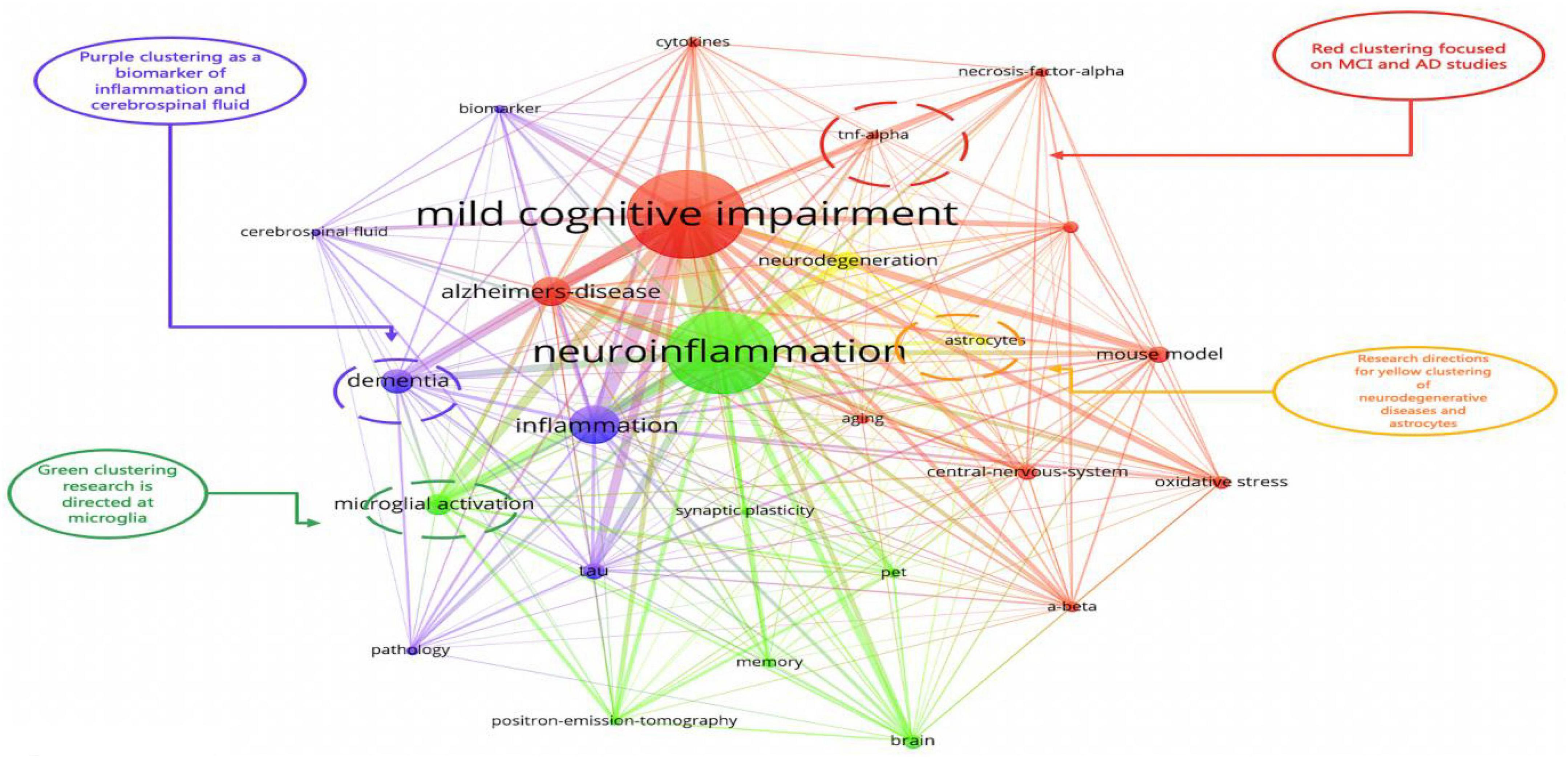
Figure 9. The co-citation timeline zone of keywords created using CiteSpace. The size of the node reflects the co-occurrence frequencies, and the link indicates the co-occurrence relationship. Nodes and lines change over time from 2012 to 2020.
One study showed that two-thirds of patients with AD were converted from MCI (Petersen, 2004). Aβ plaque deposition and neuronal dysfunction occur in the brain of patients with MCI, and Aβ plaque deposition is also a prominent pathological feature of AD. Microglia and astrocytes are the primary mediators of neuroinflammation. Under physiological conditions, microglia monitor the CNS microenvironment and its processes to sense injury signals; they are the first cells to sense inflammatory stimuli and, along with astrocytes, maintain CNS homeostasis (Leng and Edison, 2021). The function of both cell types is to clear Aβ plaques to restore neuronal health. In the aging brain, this function is probably compromised because of the saturation of microglia phagocytosis or the dysfunction of microglia, which limits Aβ clearance. Moreover, microglia are in a constant state of activation, and the release of large amounts of pro-inflammatory cytokines triggers neuroinflammation, leading to neuronal dysfunction and death. In addition, saturated cells recruit more microglia and peripheral macrophages to accumulate around Aβ plaques, disrupting the balance of pro-inflammatory and anti-inflammatory factors in the brain and triggering chronic inflammation (Kinney et al., 2018). In an in vitro study, microglia responded differently to different Aβ species, phagocytosing Aβ fibers rather than Aβ oligomers, which preferentially induced inflammatory cytokine production and inhibited the phagocytosis of Aβ fibers (Pan et al., 2011). The stimulation of small oligomers of Aβ is much greater than that of large oligomers of Aβ, inducing an activation response in microglia and producing more significant neurotoxicity (Del Bo et al., 1995; Akiyama et al., 2000). Tau is another pathological feature of AD. However, the correlation between tau deposition and microglial activation in patients with MCI is weak, and this correlation becomes more vital only in the development of the AD stage (Dani et al., 2018). In vitro studies have revealed that tau oligomers and fibers provide sufficient stimulation to induce microglia morphological changes and IL expression. Further in vivo studies using the tau mouse model confirmed the presence of tau-induced microglia activation, marked by the upregulation of DAM-related genes (disease-associated microglia) (Wes et al., 2014). In addition, most neuroimaging studies have been performed with PET and radioligands, which target transporter proteins (TSPO) to provide additional information on different glial cell phenotypes (Zimmer et al., 2014).
The interaction between microglia and astrocytes also significantly affects neuroinflammation. Microglia and astrocytes are part of the neurovascular unit (NVU) and are activated by various brain injuries (Liu et al., 2020). Microglia are more sensitive to damage, they are first activated into the M1 phenotype by pathogen-associated molecular pattern molecules (PAMPs) and damage-associated molecular pattern molecules (DAMPs) and promote the secretion of inflammatory factors (e.g., TNF-a and IL-1) to trigger reactive astrocytes (A1). With damage limitation and NVU remodeling, the local environment is altered, and microglia shift to the M2 phenotype (Liddelow et al., 2017). At the same time, local environmental factors may facilitate the conversion of astrocytes to A2, promoting neuronal survival and repair. It has been reported that a single astrocyte can supervise more than 100,000 synapses (Bushong et al., 2002; Wang et al., 2006; Halassa et al., 2007; Allen et al., 2012). Astrocytes extend terminal protrusions at approximately 99% to cover the surface of brain vessels to regulate cerebral blood flow (CBF) or the BBB (Filosa et al., 2016). Moreover, astrocytes can organize into syncytial structures of up to 100 units through gap junctions to facilitate long-distance signaling (Giaume, 2010; Charvériat et al., 2017). As mentioned above, when injury and neuronal death increase, M1 microglia trigger more reactive astrocytes (A1) via inflammatory signaling inflammation, thereby amplifying cascading neuroinflammation (Liu et al., 2020).
Research frontier identification
Figure 8 plots the keywords throughout 2011–2021, showing the changes in the core research areas in the past decade. The position of the keyword circles represents the year in which the keyword first appeared, and the lines between circles represent the connections between each keyword. The circle for the keyword “mild cognitive impairment” is the largest, indicating the most common keyword used. TREM2 and Bcl-w were among the emerging keywords in 2021, and recent studies have suggested that TREM2 is the most potent risk factor for AD. In one study, TREM2 was also shown to suppress inflammatory responses in mouse microglia by inhibiting PI3K/NF-κB signaling (Li et al., 2019). Under the relevant conditions of AD, TREM2 interacted with lipoproteins, anionic lipids, and Aβ, contributing to the microglial promotion of amyloid plaque deposition and phagocytosis of cellular tissue debris (Xue and Du, 2021). In another study, TREM2 deficiency in the presence of Aβ pathology promoted tau protein accumulation, proliferation, and brain atrophy, which facilitated microglia survival and conversion to DAM and exacerbated the disease process (Haass, 2021). These findings can be attributed to differences between animal models or experimental disease stages, and the conflicting results emphasize the importance of careful evaluation. The mechanism of action of TREM2–ligand interaction remains unclear and requires further studies.
Discussion
From a bibliometric perspective, the increasing number of studies on neuroinflammation-induced MCI indicates a surge of interest in this research area. In the graph of the predicted number of publications in the literature, it was found that the number of papers related to neuroinflammation-induced MCI increased over time. However, there was no significant increase in magazines between 2019 and 2020, this may have been associated with COVID-19, which affected global academic communication and slowed most scientific research considerably. The country analysis showed that the research efforts in this field are uneven among countries; developed countries, led by the United States, have produced the most publications and have the highest centrality, which may be related to the high level of economic development of the country and the importance it places on this field. Regarding journals and institutions, most of those in the top positions are from highly developed Western countries, such as the United States, the United Kingdom, and the Netherlands, which may be related to national policies and financial support.
Publications with co-cited authors such as Brooks D.J., Edison P., Blennow K., Zetterberg H., and Heneka M.T. have significantly influenced current research trends and the current understanding of neuroinflammation-induced MCI. Heneka et al. (2015) focused on neural inflammation in AD, they elucidated the role of microglia and astrocytes in neuroinflammation and provided an outlook on future directions in the field. Edison (2021) focused on the potential mechanisms of glial cell activation, local and systemic inflammation on disease progression, and target translocator protein (TOSP) to assess the relationship between Aβ deposition and neuroinflammation. Pascoal et al. evaluated the association between microglia and tau and found that microglial activation was associated with soluble TREM2 in the cerebrospinal fluid (Pascoal et al., 2021). Teunissen et al. provided information about the potential of AD progression and detection of treatment effects, based mainly on studies of neurodegeneration markers in the blood (Teunissen et al., 2022). Most of these researchers have made significant contributions in the field of neuroinflammation-induced MCI. In the future, researchers should enhance their collaboration efforts to maximize advances in the related field.
The publications covered in the analysis of research themes are the most influential in this field and they represent, to some extent, a change in research trends. As these themes have changed in recent years, the effects of metabolites on the brain and microglial activation have begun to draw attention. The use of metabolomics to measure the biochemical products produced by cellular processes can measure alterations in the biochemical pathways of disease and explore disease markers (Varma et al., 2018). The concentrations of the critical markers Aβ1-42, T-tau, P-tau181, neurofilament light chain (NfL), and glial fibrillary acidic protein (GFAP) in the cerebrospinal fluid have diagnostic value in diagnosing AD (Bjerke and Engelborghs, 2018). A recent study found that plasma concentrations of the phospholipid metabolite 2-aminoethyl dihydrogen phosphate normalized to taurine concentration could better identify patients with early AD (Banack et al., 2022).
Through a keyword analysis of neuroinflammation-induced MCI, it was possible to identify that neurotoxicity is mainly mediated by microglia and astrocytes. The interaction between them may be an effective and precise therapeutic target in the future (Liu et al., 2020). This represents a new research direction for MCI. In the early stages of MCI, activated microglia are initially protective, but as the disease progresses, the chronic activation of microglia results in a pro-inflammatory phenotype. This leads to damage to neuronal networks, which may be due to acquired or genetic factors, impairing microglia’s protective function. For example, the APOE-ε4 allele is the most potent genetic risk factor for sporadic AD. The upregulation of the APOE-ε4 allele has been found in microglia in diseased brains, and APOE-ε4 increases microglia activation responses and converts microglia to a pro-inflammatory phenotype (Grubman et al., 2019).
In addition, the phenotypic changes in microglia are associated with TREM2, whose activation induces an APOE-ε4-dependent pathway in microglia, exacerbating the loss of homeostatic function and the up-regulation of pro-inflammatory molecules in microglia (Krasemann et al., 2017). In recent studies on microglial phenotypes, CD33 has been identified as a sialic acid-binding immunoglobulin-type lectin receptor on microglia. The long CD33M subtype inhibits microglial phagocytosis, migration, and proliferation, whereas the short subtype increases microglia proliferation; furthermore, the CD33 receptor on microglia is dependent on the presence of TREM2 (Griciuc et al., 2019). Because TREM2 is an essential upstream mediator of microglia activation and phenotypic changes, it is also an attractive target for drug regulation (Keren-Shaul et al., 2017; Krasemann et al., 2017). Therefore, in the future study, exploring the inner link between phenotypic changes in microglia and neuroinflammation may provide a potential target for treating MCI and preventing AD.
Recent studies have shown that women over the age of 65 are more likely to develop AD than men in the same age group, and that hippocampal atrophy is more severe in women than in men. Genetic dysregulation of microglia was also found in animal models, particularly in the temporal cortex and parahippocampal gyrus. This dysregulation was more pronounced in females, and microglia activation, amyloid deposition, and behavioral changes occurred earlier in female mice. However, the clinical evidence is limited because the female population was under-sampled, leading to unbalanced samples and gender bias. Future studies should focus on gender differences to elucidate the specific disease mechanisms (Lynch, 2022). Evidence suggests that gut microbes can influence neuroinflammation in the brain via the microbiota-gut-brain axis. Under pathological conditions, the abundance of anti-inflammatory flora decreases but the pro-inflammatory flora increases, which cause dyshomeostasis of intestinal flora. This results in a more robust inflammatory response and the subsequent infiltration of inflammatory factors from the intestinal epithelium into the brain’s circulation in a vagal manner leading to neuroinflammation (Bonaz and Bernstein, 2013; Bairamian et al., 2022). In one study, analyzed of the gut microbial metabolomic analysis of patients with MCI and AD revealed alterations in the fecal microbiological profile of patients with AD, mainly focusing on indole-3-pyruvic acid (IPyA), which may be a mediator of intestinal ecological dysregulation and could be involved in the development of AD (Wu et al., 2021). Therefore, in future studies, exploring the inner link between IPyA and neuroinflammation may provide a potential target for treating MCI and preventing AD.
In addition, inflammatory factors can disrupt the BBB and thus allow leukocytes and other cells to infiltrate the brain, which will exacerbate neuroinflammation and act directly on microglia. The ability of microglia to monitor pathogens and cellular debris is thus disrupted, along with their ability to phagocytose Aβ (Bonaz and Bernstein, 2013; Bairamian et al., 2022). Therefore, targeting the microbiota rather than immune function appears feasible for preventing and controlling MCI.
In terms of current advances in treating MCI by targeting neuroinflammatory molecules, a clinical study demonstrated that subcutaneous injections of IFNβ1a could modulate neuroinflammation-induced MCI showed that the treatment tended to slow disease progression (Grimaldi et al., 2014). In addition, several other anti-inflammatory factors, including IL-2, IL-4, and IL-33, have the potential to attenuate microglial activation in the clinical setting. Tanshinone IIA reduced microglia and astrocyte activation and prevented neuronal synapse loss in APP/PS1 mice by inhibiting the RAGE/NF-κB signaling pathway in vivo and vitro (Ding et al., 2020). In APP/PS1 transgenic mice, the PPARγ agonists (pioglitazone and rosiglitazone) shifted microglia from the pro-inflammatory phenotype to the phagocytic phenotype and promoted amyloid clearance (Escribano et al., 2010; Yamanaka et al., 2012). In addition, exercise therapy and a healthy lifestyle were recently identified as non-pharmacological treatments that can mitigate chronic cognitive disorders, including MCI (Zotcheva et al., 2021).
Conclusion
Findings from this study show that research on neuroinflammation-induced MCI is burgeoning, and the close collaboration with different nations and institutions need to be further strengthened. Current research hotspots are focused on the effects of various metabolites on microglia activation. Future studies should focus on how to reduce smation and treat MCI via inhibiting the pro-inflammatory phenotype and enhancing the anti-inflammatory phenotype of microglia and astrocytes.
Author contributions
MC and LZ conceived the study. YZ, SC, and ZW collected the data. YZ wrote the article. MC revised the article. All authors contributed to the article and approved the submitted version.
Funding
This work was sponsored by a grant from the Youth Fund Project of the Research Planning Foundation on Humanities and Social Sciences of the Ministry of Education (20YJCZH001), the Scientific Research Foundation of SUMHS (SSF-21-03-008), and a fund project of the Shandong Provincial Department of Education (SDYAL20103).
Acknowledgments
We would like to thank Native English speaker Katherine Dulgeroff for her contribution to the language revision of this article.
Conflict of interest
The authors declare that the research was conducted in the absence of any commercial or financial relationships that could be construed as a potential conflict of interest.
Publisher’s note
All claims expressed in this article are solely those of the authors and do not necessarily represent those of their affiliated organizations, or those of the publisher, the editors and the reviewers. Any product that may be evaluated in this article, or claim that may be made by its manufacturer, is not guaranteed or endorsed by the publisher.
References
Akiyama, H., Barger, S., Barnum, S., Bradt, B., Bauer, J., Cole, G. M., et al. (2000). Inflammation and Alzheimer’s disease. Neurobiol. Aging 21, 383–421. doi: 10.1016/s0197-4580(00)00124-x
Allen, N. J., Bennett, M. L., Foo, L. C., Wang, G. X., Chakraborty, C., Smith, S. J., et al. (2012). Astrocyte glypicans 4 and 6 promote formation of excitatory synapses via GluA1 AMPA receptors. Nature 486, 410–414. doi: 10.1038/nature11059
Andreadou, E. G., Katsipis, G., Tsolaki, M., and Pantazaki, A. A. (2021). Involvement and relationship of bacterial lipopolysaccharides and cyclooxygenases levels in Alzheimer’s Disease and Mild Cognitive Impairment patients. J. Neuroimmunol. 357:577561. doi: 10.1016/j.jneuroim.2021.577561
Bairamian, D., Sha, S., Rolhion, N., Sokol, H., Dorothée, G., Lemere, C. A., et al. (2022). Microbiota in neuroinflammation and synaptic dysfunction: a focus on Alzheimer’s disease. Mol. Neurodegener. 17:19. doi: 10.1186/s13024-022-00522-2
Bajetto, A., Bonavia, R., Barbero, S., and Schettini, G. (2002). Characterization of chemokines and their receptors in the central nervous system: physiopathological implications. J. Neurochem. 82, 1311–1329. doi: 10.1046/j.1471-4159.2002.01091.x
Banack, S. A., Stark, A. C., and Cox, P. A. (2022). A possible blood plasma biomarker for early-stage Alzheimer’s disease. PLoS One 17:e0267407. doi: 10.1371/journal.pone.0267407
Bjerke, M., and Engelborghs, S. (2018). Cerebrospinal Fluid Biomarkers for Early and Differential Alzheimer’s Disease Diagnosis. J. Alzheimers Dis. 62, 1199–1209. doi: 10.3233/JAD-170680
Bonaz, B. L., and Bernstein, C. N. (2013). Brain-gut interactions in inflammatory bowel disease. Gastroenterology 144, 36–49. doi: 10.1053/j.gastro.2012.10.003
Bowman, G. L., Dayon, L., Kirkland, R., Wojcik, J., Peyratout, G., Severin, I. C., et al. (2018). Blood-brain barrier breakdown, neuroinflammation, and cognitive decline in older adults. Alzheimers Dement. 14, 1640–1650. doi: 10.1016/j.jalz.2018.06.2857
Bushong, E. A., Martone, M. E., Jones, Y. Z., and Ellisman, M. H. (2002). Protoplasmic astrocytes in CA1 stratum radiatum occupy separate anatomical domains. J. Neurosci. 22, 183–192. doi: 10.1523/JNEUROSCI.22-01-00183.2002
Charvériat, M., Naus, C. C., Leybaert, L., Sáez, J. C., and Giaume, C. (2017). Connexin-Dependent Neuroglial Networking as a New Therapeutic Target. Front. Cell Neurosci. 11:174. doi: 10.3389/fncel.2017.00174
Chen, C. (2004). Searching for intellectual turning points: progressive knowledge domain visualization. Proc. Natl. Acad. Sci. U.S.A. 101, 5303–5310. doi: 10.1073/pnas.0307513100
Colonna, M., and Brioschi, S. (2020). Neuroinflammation and neurodegeneration in human brain at single-cell resolution. Nat. Rev. Immunol. 20, 81–82. doi: 10.1038/s41577-019-0262-0
Dani, M., Wood, M., Mizoguchi, R., Fan, Z., Walker, Z., Morgan, R., et al. (2018). Microglial activation correlates in vivo with both tau and amyloid in Alzheimer’s disease. Brain 141, 2740–2754. doi: 10.1093/brain/awy188
Del Bo, R., Angeretti, N., Lucca, E., De Simoni, M. G., and Forloni, G. (1995). Reciprocal control of inflammatory cytokines, IL-1 and IL-6, and beta-amyloid production in cultures. Neurosci. Lett. 188, 70–74. doi: 10.1016/0304-3940(95)11384-9
Ding, B., Lin, C., Liu, Q., He, Y., Ruganzu, J. B., Jin, H., et al. (2020). Tanshinone IIA attenuates neuroinflammation via inhibiting RAGE/NF-κB signaling pathway in vivo and in vitro. J. Neuroinflammation 17:302. doi: 10.1186/s12974-020-01981-4
Edison, P. (2021). Microglial activation and blood-brain barrier leakage: Chicken and egg? Brain 144, 1284–1285. doi: 10.1093/brain/awab149
Escribano, L., Simón, A. M., Gimeno, E., Cuadrado-Tejedor, M., López de Maturana, R., García-Osta, A., et al. (2010). Rosiglitazone rescues memory impairment in Alzheimer’s transgenic mice: mechanisms involving a reduced amyloid and tau pathology. Neuropsychopharmacology 35, 1593–1604. doi: 10.1038/npp.2010.32
Fan, Z., Aman, Y., Ahmed, I., Chetelat, G., Landeau, B., Ray Chaudhuri, K., et al. (2015). Influence of microglial activation on neuronal function in Alzheimer’s and Parkinson’s disease dementia. Alzheimers Dement. 11, 608–621e607. doi: 10.1016/j.jalz.2014.06.016
Filosa, J. A., Morrison, H. W., Iddings, J. A., Du, W., and Kim, K. J. (2016). Beyond neurovascular coupling, role of astrocytes in the regulation of vascular tone. Neuroscience 323, 96–109. doi: 10.1016/j.neuroscience.2015.03.064
Giaume, C. (2010). Astroglial Wiring is Adding Complexity to Neuroglial Networking. Front. Neuroenergetics 2:129. doi: 10.3389/fnene.2010.00129
Giovannoni, F., and Quintana, F. J. (2020). The Role of Astrocytes in CNS Inflammation. Trends Immunol. 41, 805–819. doi: 10.1016/j.it.2020.07.007
Griciuc, A., Patel, S., Federico, A. N., Choi, S. H., Innes, B. J., Oram, M. K., et al. (2019). TREM2 Acts Downstream of CD33 in Modulating Microglial Pathology in Alzheimer’s Disease. Neuron 103:820–835.e7. doi: 10.1016/j.neuron.2019.06.010
Grimaldi, L. M., Zappalà, G., Iemolo, F., Castellano, A. E., Ruggieri, S., Bruno, G., et al. (2014). A pilot study on the use of interferon beta-1a in early Alzheimer’s disease subjects. J. Neuroinflammation 11:30. doi: 10.1186/1742-2094-11-30
Grubman, A., Chew, G., Ouyang, J. F., Sun, G., Choo, X. Y., McLean, C., et al. (2019). A single-cell atlas of entorhinal cortex from individuals with Alzheimer’s disease reveals cell-type-specific gene expression regulation. Nat. Neurosci. 22, 2087–2097. doi: 10.1038/s41593-019-0539-4
Haass, C. (2021). Loss of TREM2 facilitates tau accumulation, spreading, and brain atrophy, but only in the presence of amyloid pathology. Neuron 109, 1243–1245. doi: 10.1016/j.neuron.2021.03.029
Halassa, M. M., Fellin, T., and Haydon, P. G. (2007). The tripartite synapse: roles for gliotransmission in health and disease. Trends Mol. Med. 13, 54–63. doi: 10.1016/j.molmed.2006.12.005
Hamelin, L., Lagarde, J., Dorothée, G., Leroy, C., Labit, M., Comley, R. A., et al. (2016). Early and protective microglial activation in Alzheimer’s disease: A prospective study using 18F-DPA-714 PET imaging. Brain 139(Pt 4), 1252–1264. doi: 10.1093/brain/aww017
Heneka, M. T., Carson, M. J., El Khoury, J., Landreth, G. E., Brosseron, F., Feinstein, D. L., et al. (2015). Neuroinflammation in Alzheimer’s disease. Lancet Neurol. 14, 388–405. doi: 10.1016/S1474-4422(15)70016-5
Heppner, F. L., Ransohoff, R. M., and Becher, B. (2015). Immune attack: The role of inflammation in Alzheimer disease. Nat. Rev. Neurosci. 16, 358–372. doi: 10.1038/nrn3880
Huang, W., Lan, X., Li, X., Wang, D., Sun, Y., and Wang, Q. (2017). Long non-coding RNA PVT1 promote LPS-induced septic acute kidney injury by regulating TNFα and JNK/NF-κB pathways in HK-2 cells. Int. Immunopharmacol. 47, 134–140. doi: 10.1016/j.intimp.2017.03.030
JACK, C. R. Jr., Bennett, D. A., Blennow, K., Carrillo, M. C., Dunn, B., Haeberlein, S. B., et al. (2018). NIA-AA Research framework: Toward a biological definition of Alzheimer’s disease. Alzheimers Dement. 14, 535–562. doi: 10.1016/j.jalz.2018.02.018
Keren-Shaul, H., Spinrad, A., Weiner, A., Matcovitch-Natan, O., Dvir-Szternfeld, R., Ulland, T. K., et al. (2017). A Unique Microglia Type Associated with Restricting Development of Alzheimer’s Disease. Cell 169:1276–1290.e17. doi: 10.1016/j.cell.2017.05.018
Kim, K., Wang, X., Ragonnaud, E., Bodogai, M., Illouz, T., DeLuca, M., et al. (2021). Therapeutic B-cell depletion reverses progression of Alzheimer’s disease. Nat. Commun. 12:2185. doi: 10.1038/s41467-021-22479-4
Kinney, J. W., Bemiller, S. M., Murtishaw, A. S., Leisgang, A. M., Salazar, A. M., and Lamb, B. T. (2018). Inflammation as a central mechanism in Alzheimer’s disease. Alzheimers Dement 4, 575–590. doi: 10.1016/j.trci.2018.06.014
Krasemann, S., Madore, C., Cialic, R., Baufeld, C., Calcagno, N., El Fatimy, R., et al. (2017). The TREM2-APOE Pathway Drives the Transcriptional Phenotype of Dysfunctional Microglia in Neurodegenerative Diseases. Immunity 47:566–581.e9. doi: 10.1016/j.immuni.2017.08.008
Kwon, H. S., and Koh, S. H. (2020). Neuroinflammation in neurodegenerative disorders: the roles of microglia and astrocytes. Transl Neurodegener. 9:42. doi: 10.1186/s40035-020-00221-2
Leng, F., and Edison, P. (2021). Neuroinflammation and microglial activation in Alzheimer disease: where do we go from here? Nat. Rev. Neurol. 17, 157–172. doi: 10.1038/s41582-020-00435-y
Li, C., Zhao, B., Lin, C., Gong, Z., and An, X. (2019). TREM2 inhibits inflammatory responses in mouse microglia by suppressing the PI3K/NF-κB signaling. Cell Biol. Int. 43, 360–372. doi: 10.1002/cbin.10975
Liddelow, S. A., Guttenplan, K. A., Clarke, L. E., Bennett, F. C., Bohlen, C. J., Schirmer, L., et al. (2017). Neurotoxic reactive astrocytes are induced by activated microglia. Nature 541, 481–487. doi: 10.1038/nature21029
Liu, L. R., Liu, J. C., Bao, J. S., Bai, Q. Q., and Wang, G. Q. (2020). Interaction of Microglia and Astrocytes in the Neurovascular Unit. Front. Immunol. 11:1024. doi: 10.3389/fimmu.2020.01024
Liu, W., Liu, L., Cheng, X., Ge, H., Hu, G., Xue, C., et al. (2021). Functional Integrity of Executive Control Network Contributed to Retained Executive Abilities in Mild Cognitive Impairment. Front. Aging Neurosci. 13:710172. doi: 10.3389/fnagi.2021.710172
Lynch, M. A. (2022). Exploring Sex-Related Differences in Microglia May Be a Game-Changer in Precision Medicine. Front. Aging Neurosci. 14:868448. doi: 10.3389/fnagi.2022.868448
Needham, B. D., Funabashi, M., Adame, M. D., Wang, Z., Boktor, J. C., Haney, J., et al. (2022). A gut-derived metabolite alters brain activity and anxiety behaviour in mice. Nature 602, 647–653. doi: 10.1038/s41586-022-04396-8
Norden, D. M., and Godbout, J. P. (2013). Review: microglia of the aged brain: primed to be activated and resistant to regulation. Neuropathol. Appl. Neurobiol. 39, 19–34. doi: 10.1111/j.1365-2990.2012.01306.x
Nordengen, K., Kirsebom, B. E., Henjum, K., Selnes, P., Gísladóttir, B., Wettergreen, M., et al. (2019). Glial activation and inflammation along the Alzheimer’s disease continuum. J. Neuroinflammation 16:46. doi: 10.1186/s12974-019-1399-2
Owens, T., Khorooshi, R., Wlodarczyk, A., and Asgari, N. (2014). Interferons in the central nervous system: a few instruments play many tunes. Glia 62, 339–355. doi: 10.1002/glia.22608
Pan, X. D., Zhu, Y. G., Lin, N., Zhang, J., Ye, Q. Y., Huang, H. P., et al. (2011). Microglial phagocytosis induced by fibrillar β-amyloid is attenuated by oligomeric β-amyloid: implications for Alzheimer’s disease. Mol. Neurodegener 6:45. doi: 10.1186/1750-1326-6-45
Pasciuto, E., Burton, O. T., Roca, C. P., Lagou, V., Rajan, W. D., Theys, T., et al. (2020). Microglia Require CD4 T Cells to Complete the Fetal-to-Adult Transition. Cell 182:625–640.e24. doi: 10.1016/j.cell.2020.06.026
Pascoal, T. A., Benedet, A. L., Ashton, N. J., Kang, M. S., Therriault, J., Chamoun, M., et al. (2021). Microglial activation and tau propagate jointly across Braak stages. Nat. Med. 27, 1592–1599. doi: 10.1038/s41591-021-01456-w
Petersen, R. C. (2004). Mild cognitive impairment as a diagnostic entity. J. Intern. Med. 256, 183–194. doi: 10.1111/j.1365-2796.2004.01388.x
Ransohoff, R. M. (2016). A polarizing question: do M1 and M2 microglia exist? Nat. Neurosci. 19, 987–991. doi: 10.1038/nn.4338
Solé-Domènech, S., Cruz, D. L., Capetillo-Zarate, E., and Maxfield, F. R. (2016). The endocytic pathway in microglia during health, aging and Alzheimer’s disease. Ageing Res. Rev. 32, 89–103. doi: 10.1016/j.arr.2016.07.002
Spittau, B. (2017). Aging Microglia-Phenotypes, Functions and Implications for Age-Related Neurodegenerative Diseases. Front. Aging Neurosci. 9:194. doi: 10.3389/fnagi.2017.00194
Teunissen, C. E., Verberk, I., Thijssen, E. H., Vermunt, L., Hansson, O., Zetterberg, H., et al. (2022). Blood-based biomarkers for Alzheimer’s disease: towards clinical implementation. Lancet Neurol. 21, 66–77. doi: 10.1016/S1474-4422(21)00361-6
van Eck, N. J., and Waltman, L. (2010). Software survey: VOSviewer, a computer program for bibliometric mapping. Scientometrics 84, 523–538. doi: 10.1007/s11192-009-0146-3
Varma, V. R., Oommen, A. M., Varma, S., Casanova, R., An, Y., Andrews, R. M., et al. (2018). Brain and blood metabolite signatures of pathology and progression in Alzheimer disease: A targeted metabolomics study. PLoS Med. 15:e1002482. doi: 10.1371/journal.pmed.1002482
Wang, A. T., Lee, S. S., Sigman, M., and Dapretto, M. (2006). Developmental changes in the neural basis of interpreting communicative intent. Soc. Cogn. Affect. Neurosci. 1, 107–121. doi: 10.1093/scan/nsl018
Wes, P. D., Easton, A., Corradi, J., Barten, D. M., Devidze, N., DeCarr, L. B., et al. (2014). Tau overexpression impacts a neuroinflammation gene expression network perturbed in Alzheimer’s disease. PLoS One 9:e106050. doi: 10.1371/journal.pone.0106050
Wu, L., Han, Y., Zheng, Z., Peng, G., Liu, P., Yue, S., et al. (2021). Altered gut microbial metabolites in amnestic mild cognitive impairment and Alzheimer’s disease: Signals in host-microbe interplay. Nutrients 13:228. doi: 10.3390/nu13010228
Xue, F., and Du, H. (2021). TREM2 Mediates Microglial Anti-Inflammatory Activations in Alzheimer’s Disease: Lessons Learned from Transcriptomics. Cells 10:321. doi: 10.3390/cells10020321
Yamanaka, M., Ishikawa, T., Griep, A., Axt, D., Kummer, M. P., and Heneka, M. T. (2012). PPARγ/RXRα-induced and CD36-mediated microglial amyloid-β phagocytosis results in cognitive improvement in amyloid precursor protein/presenilin 1 mice. J. Neurosci. 32, 17321–17331. doi: 10.1523/JNEUROSCI.1569-12.2012
Zhou, R., Ji, B., Kong, Y., Qin, L., Ren, W., Guan, Y., et al. (2021). PET Imaging of Neuroinflammation in Alzheimer’s Disease. Front. Immunol. 12:739130. doi: 10.3389/fimmu.2021.739130
Zimmer, E. R., Leuzy, A., Benedet, A. L., Breitner, J., Gauthier, S., and Rosa-Neto, P. (2014). Tracking neuroinflammation in Alzheimer’s disease: the role of positron emission tomography imaging. J. Neuroinflammation 11:120. doi: 10.1186/1742-2094-11-120
Keywords: mild cognitive impairment, neuroinflammation, CiteSpace, VOSviewer, microglial, astrocytes
Citation: Cai M, Zhang Y, Chen S, Wu Z and Zhu L (2022) The past, present, and future of research on neuroinflammation-induced mild cognitive impairment: A bibliometric analysis. Front. Aging Neurosci. 14:968444. doi: 10.3389/fnagi.2022.968444
Received: 14 June 2022; Accepted: 08 July 2022;
Published: 29 July 2022.
Edited by:
Ning Song, Qingdao University, ChinaReviewed by:
Andy Wai Kan Yeung, University of Hong Kong, ChinaXin-an Zhang, Shenyang Sport University, China
Copyright © 2022 Cai, Zhang, Chen, Wu and Zhu. This is an open-access article distributed under the terms of the Creative Commons Attribution License (CC BY). The use, distribution or reproduction in other forums is permitted, provided the original author(s) and the copyright owner(s) are credited and that the original publication in this journal is cited, in accordance with accepted academic practice. No use, distribution or reproduction is permitted which does not comply with these terms.
*Correspondence: Lei Zhu, emh1bGVpMzE2QDEyNi5jb20=
†These authors have contributed equally to this work and share first authorship