- Department of Radiology, The Second Affiliated Hospital, Zhejiang University School of Medicine, Hangzhou, China
Background: Understanding genetic influences on Alzheimer’s disease (AD) may improve early identification. Polygenic hazard score (PHS) is associated with the age of AD onset and cognitive decline. It interacts with other risk factors, but the nature of such combined effects remains poorly understood.
Materials and methods: We examined the effect of genetic risk and hippocampal atrophy pattern on episodic memory in a sample of older adults ranging from cognitively normal to those diagnosed with AD using structural MRI. Participants included 51 memory unimpaired normal control (NC), 69 mild cognitive impairment (MCI), and 43 AD adults enrolled in the Alzheimer’s Disease Neuroimaging Initiative (ADNI). Hierarchical linear regression analyses examined the main and interaction effects of hippocampal subfield volumes and PHS, indicating genetic risk for AD, on a validated episodic memory composite score. Diagnosis-stratified models further assessed the role of PHS.
Results: Polygenic hazard score moderated the relationship between right fimbria/hippocampus volume ratio and episodic memory, such that patients with high PHS and lower volume ratio had lower episodic memory composite scores [ΔF = 6.730, p = 0.011, ΔR2 = 0.059]. This effect was also found among individuals with MCI [ΔF = 4.519, p = 0.038, ΔR2 = 0.050]. In contrast, no interaction effects were present for those NC or AD individuals. A follow-up mediation analysis also indicated that the right fimbria/hippocampus volume ratio might mediate the link between PHS and episodic memory performance in the MCI group, whereas no mediation effects were present for those NC or AD individuals.
Conclusion: These findings suggest that the interaction between AD genetic risk and hippocampal subfield volume ratio increases memory impairment among older adults. Also, the results highlighted a potential pathway in which genetic risk affects memory by degrading hippocampal subfield volume ratio in cognitive decline subjects.
Introduction
Alzheimer’s disease (AD) is the most frequent cause of dementia (Prince et al., 2013). Clinically, AD develops gradually and presents with progressive decline in multiple cognitive domains, particularly affecting episodic memory (Small et al., 1997; Elias et al., 2000). As the Chinese population is aging, more than seven million Chinese people live with AD currently, and the costs are predicted to reach US $507.49 billion in 2030 (Jia et al., 2018). It is, therefore, important to gain a better understanding of the factors associated with dementia, including brain and genetic markers. Understanding how genetic risk for AD affects the brain might shed light on mechanisms leading to AD cognitive decline later in life.
Studies have shown that genetic risk factors play a critical role in AD development (Gatz et al., 2006). Various susceptibility loci have been identified. For example, early-onset AD is considered to be caused by mutations in the amyloid precursor protein (APP), presenilin-1 (PRES-1), and presenilin-2 (PRES-2) genes (Goate et al., 1991; Levy-Lahad et al., 1995; Rogaev et al., 1995). The apolipoprotein E4 allele (APOE) is the most well-known genetic risk factor linked to late-onset AD (Strittmatter et al., 1993; Gatz et al., 2006; Bettens et al., 2013). But the majority of studies have focused on the risk associated with a single-candidate gene (Strittmatter et al., 1993; Wang et al., 2019). Based on a combination of APOE and 31 other genetic variants, a polygenic hazard score (PHS) has been developed and validated for quantifying AD dementia age of onset (Desikan et al., 2017). The PHS showed substantial improvement over APOE in predicting the age of AD onset and was associated with biomarkers of AD, including MRI-based hippocampal volume loss and cognitive impairment (Desikan et al., 2017; Kauppi et al., 2018).
The genetic effects on AD cognitive decline can be revealed in vivo in the human brain by structural and functional magnetic resonance imaging (MRI) methods. Structural MRI studies have provided evidence of hippocampal atrophy as a key factor in memory impairment in AD (Zarow et al., 2011; Weiner et al., 2013; Harper et al., 2014) and hippocampal volume is considered an index of the degree of cognitive decline (Kilpatrick et al., 1997; Ystad et al., 2009). However, the hippocampal formation is not homogeneous but is composed of several interconnected subregions, namely hippocampal subfields that are believed to have different functions (Duvernoy, 2008; Mueller et al., 2011). Their specialization makes the hippocampal subfields differentially susceptible to AD pathogenic disruptions (Apostolova et al., 2010; Pluta et al., 2012; Zeng et al., 2021). But how the anatomical vulnerability in hippocampal subfields observed in AD is linked to memory impairment remains unknown. This might be partially explained by regional differences in the vulnerability to tau accumulation and neurofibrillary tangles (NFTs), which are core pathological markers of AD (Braak and Braak, 1990; Ball, 1997; Schonheit et al., 2004). Therefore, the volume of hippocampal subfields might be more sensitive imaging biomarkers for understanding memory in AD.
Previous studies suggest that memory impairment in AD may be mediated through APOE-induced changes in the hippocampus (Han and Bondi, 2008; Caselli et al., 2009; Tuminello and Han, 2011; Wang et al., 2019). First, episodic memory is critically dependent on the hippocampus and is impaired in AD. Second, both the decrease of hippocampal volume and memory dysfunction follow a similar genetic trajectory, e.g., both decrease in healthy older people with the possession of the ε4 allele. Finally, in AD, APOE, which asserted different effects on episodic memory, was associated with different compensatory recruitment processes in the hippocampus. A recent study revealed that PHS moderated the relationship between the medial temporal lobe (MTL) volume and episodic memory in older AD adults (Prieto et al., 2020). However, it is unknown whether PHS has similar effects on the association of hippocampal subfield volumes with episodic memory across the AD continuum. Our main goal was to examine whether the PHS modulates the relationship between hippocampal subfield volumes and episodic memory in subjects with normal control (NC), mild cognitive impairment (MCI), and AD from the Alzheimer’s Disease Neuroimaging Initiative (ADNI) dataset. We hypothesized that individuals with high polygenic risk for AD and smaller hippocampal subfield volume ratios would show reduced episodic memory performance. And we intended to investigate if hippocampal subfield volume ratios mediated the effect of PHS on memory scores.
Materials and methods
Participants
Data used in the preparation of this article were obtained from the ADNI database.1 The ADNI was launched in 2003 as a public–private partnership, led by Principal Investigator Michael W. Weiner, MD. The primary goal of ADNI has been to test whether serial magnetic resonance imaging (MRI), positron emission tomography (PET), other biological markers, and clinical and neuropsychological assessment can be combined to measure the progression of MCI and early AD.
We selected subjects from the ADNI-2 population based on study forms downloaded from the website. Inclusive and exclusive criteria can be found in detail at http://www.adni-info.org. The ADNI criteria for normal controls (NC) were: (1) a Mini-Mental State Examination (MMSE) score of at least 24; (2) a Clinical Dementia Rating (CDR) score of 0; and (3) no report of any cognition complaint. The ADNI criteria for MCI were: (1) subjective memory complaints; (2) objective memory loss defined by the Wechsler Memory Scale (WMS-R) logical memory test (Wechsler, 1987); (3) a global CDR score of 0.5; (4) an MMSE score of equal to, or higher than, 24 out of 30; and (5) general cognitive and functional performance sufficiently preserved such that a diagnosis of dementia could not be made by the site physician at the time of screening. Diagnostic criteria for AD included MMSE scores between 20 and 26 and a global CDR of 0.5 or 1.0 at baseline (Petersen et al., 2010).
Based on the above criteria, we identified 163 subjects, including 51 subjects with NC, 69 patients with MCI, and 43 patients with AD. Individuals with a non-accelerated T1 MRI screening scan, PHS, and baseline visit episodic memory were included for analyses. Written informed consent was obtained from all participants or their authorized representatives. All the participants from ADNI-2 with PHS and a non-accelerated T1 MRI screening scan using SPGR were included.
Structural magnetic resonance imaging
Structural MRI brain scans were obtained with a standardized protocol, which is described in detail at www.loni.ucla.edu/ADNI. Sagittal 3D T1-weighted MRI sequence (TE/TI/TR = 2.98/900/2,300 ms, matrix size 256 × 256 × 176, slice thickness = 1.20 mm) was performed for each participant.
Hippocampal subfields volume estimation
Volumetric measures of hippocampal subfields were performed using FreeSurfer (version 6.0).2 Automated segmentation of the hippocampal subfields was performed based on a computational atlas of the hippocampal formation using a combination of ex vivo and in vivo MRI data (Iglesias et al., 2015). The atlas includes the hippocampal tail, subiculum, CA1, CA3, CA4, the hippocampal fissure, presubiculum, parasubiculum, the molecular layer (ML), the molecular and granule cell layers of the dentate gyrus (GC-ML-DG), fimbria, and the hippocampal amygdala transition area (HATA). The images from a normal subject are shown in Figure 1 as an example. Also, it is important to consider the relationship of the hippocampal subfields and the entire ipsilateral hippocampus (eiHP), which varies between individuals (Burwell and Agster, 2008). As described previously, the entire hippocampal volume by FreeSurfer is an atlas-based estimation approach (Iglesias et al., 2015). In this study, we used hippocampal subfield-to-eiHP volume ratio (VR) for further statistical analyses.
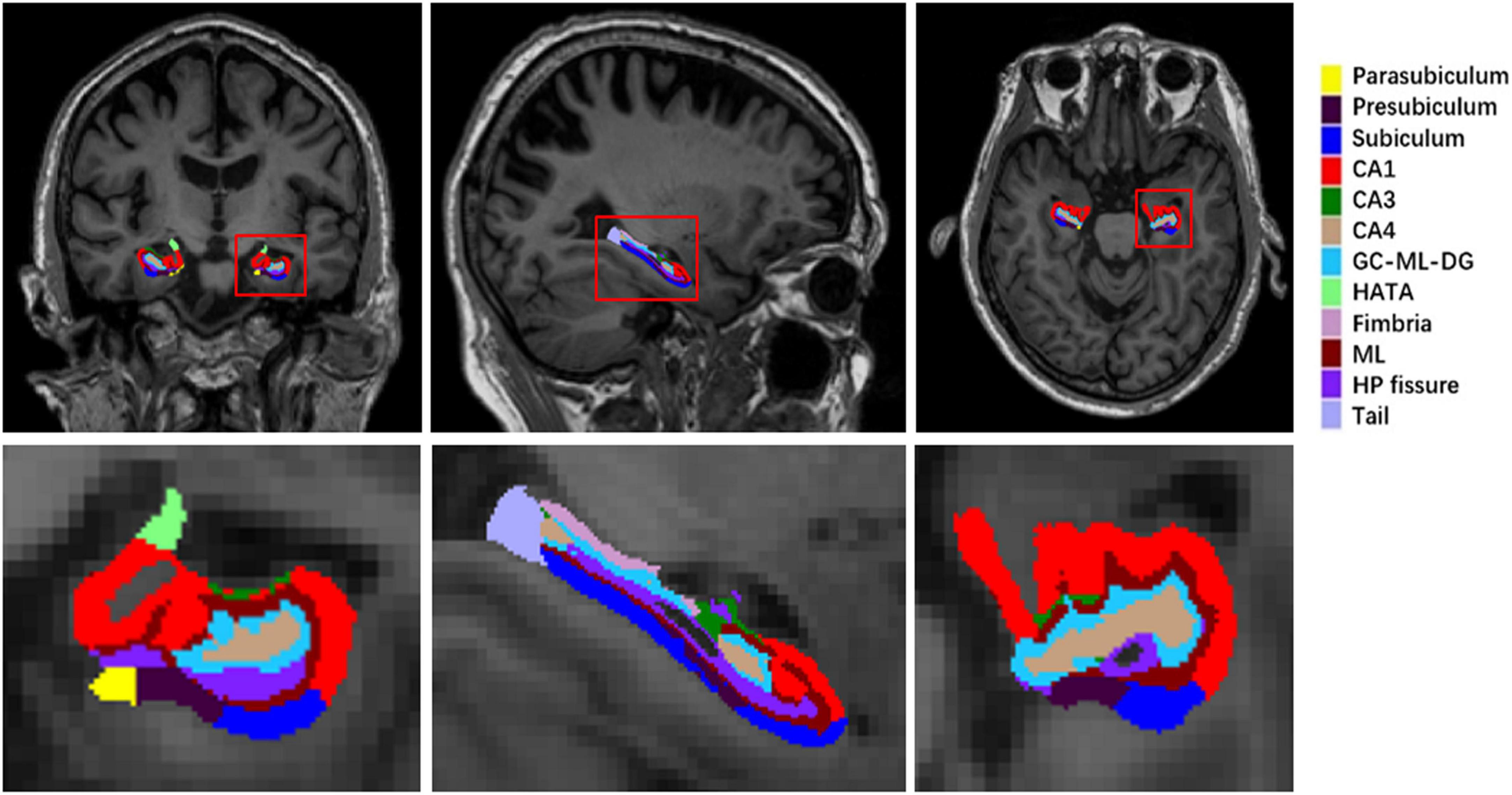
Figure 1. A sample of left hippocampal subfield automated segmentation. HP, hippocampus; ML, the molecular layer; CA, cornus ammonis; GC-ML-DG, the molecular and granule cell layers of the dentate gyrus; HATA, hippocampal amygdala transition area.
Polygenic hazard score
For all participants in this study, their individual PHS was computed, as described previously (Kauppi et al., 2018). Briefly, AD-associated single-nucleotide polymorphisms (SNPs) (at p < 10–5) were first delineated using genotype data from 17,008 AD cases and 37,154 controls from Stage 1 of the International Genomics of Alzheimer’s Project. Next, using genotype data from 6,409 patients with AD and 9,386 older controls from Phase 1 of the Alzheimer’s Disease Genetics Consortium (ADGC Phase 1), and corrected for the baseline allele frequencies using European genotypes from the 1,000 Genomes Project, a total of 31 AD-associated SNPs were identified from a stepwise Cox proportional hazards model to derive a PHS for each participant. Finally, by combining US population-based incidence rates and the genotype-derived PHS for each individual, estimates of instantaneous risk (i.e., cumulative incidence rate) for developing AD were derived based on genotype and age (Desikan et al., 2017; Tan et al., 2019). Considering APOE is strongly associated with both cognition and hippocampal volumes even in very early cases (Haller et al., 2019; Herrmann et al., 2019), we have completed all the analyses including APOE genotype as a covariate.
Memory composite score
The composite episodic memory score was derived from the neuropsychological battery administered in ADNI (Crane et al., 2012). The memory composite score was created from the following: longitudinal Rey Auditory Verbal Learning Test (RAVLT, two versions), AD assessment schedule - cognition (ADAS-Cog, three versions), memory components of MMSE, and logical memory task. Cognitive data from 803 ADNI participants were used. Composite scores have a mean of 0 and a standard deviation (SD) of 1 (Crane et al., 2012).
Statistical analysis
Demographics and cognitive outcomes were compared between diagnostic groups using analysis of variance (ANOVA) for continuous variables and Chi-squared for categorical variables in all subjects and within diagnostic groups.
General linear mixed models were used to evaluate the associations between diagnosis and PHS status on HP subfield volume ratios in all subjects. In this study, the model included diagnosis (normal control, MCI, and AD), PHS status (high PHS and low PHS), and the diagnosis × PHS status interaction term. Covariates were age, gender, and education. If significant subfields were identified, we calculated the difference of HP subfield VR between each diagnosis group which was found to be statistically significant at the alpha = 0.05 level in the least significant difference (LSD) test.
If significant interaction effects were observed, partial correlation analyses were conducted to determine the relationship between HP subfield VR and memory score in all subjects and within diagnostic groups regressing out the same covariates. HP subfield VR and memory scores were compared between high PHS and low PHS groups using Student’s t-test in all subjects and within diagnostic groups.
To verify the presence of an interaction between PHS and HP subfield VR on composite episodic memory scores, we used hierarchical linear regression models with an added interaction term. Covariates included in the first step of the linear regression model were: sex, age, and education. The second step of the model assessed for the main effects of PHS and HP subfield VR. The third step of the model added the interaction between PHS and HP subfield VR. Diagnosis-stratified analyses were conducted to establish if the effects were more prominent in a particular diagnostic group (NC, MCI, AD). The resultant p-values for the associations of the PHS × HP subfield VR interaction with memory performance were corrected for multiple comparisons with false discovery rate (FDR) (Benjamini and Hochberg, 1995). IBM SPSS version 19.0 was used to perform all statistical analyses. A two-tailed p-value of less than 0.05 was considered to be statistically significant (corrected for multiple comparisons with Bonferroni).
As one of our main objectives was to test whether HP subfield VR drove PHS’s influence on memory, we further conducted a mediation analysis to test whether HP subfield VR was a potential mediator between PHS (independent variable) and memory score (outcome variable). A statistic toolbox (PROCESS Procedure for SPSS Release 2.16.3),3 a validated, freely available computational tool, was used. First, we tested whether the primary independent variable, in this case PHS, predicted the outcome measure, memory score. Next, we tested the direct effects of the primary predictor on the mediator and the direct relationship between the mediator and the outcome. Finally, we tested the indirect mediating effect or the extent to which the relationship between PHS and memory score operates statistically through HP subfield VR. We set the Bootstrap samples = 5,000, 95% confidence level for confidence intervals and we control age, gender, and education on the mediator and outcome. Figure 2 illustrates the direct and indirect (mediation) statistical models graphically. The outcome of the indirect effect was considered statistically significant (p < 0.05, two-tailed) when zero is not included in the 95% confidence interval (Preacher and Hayes, 2004; Hayes, 2013).
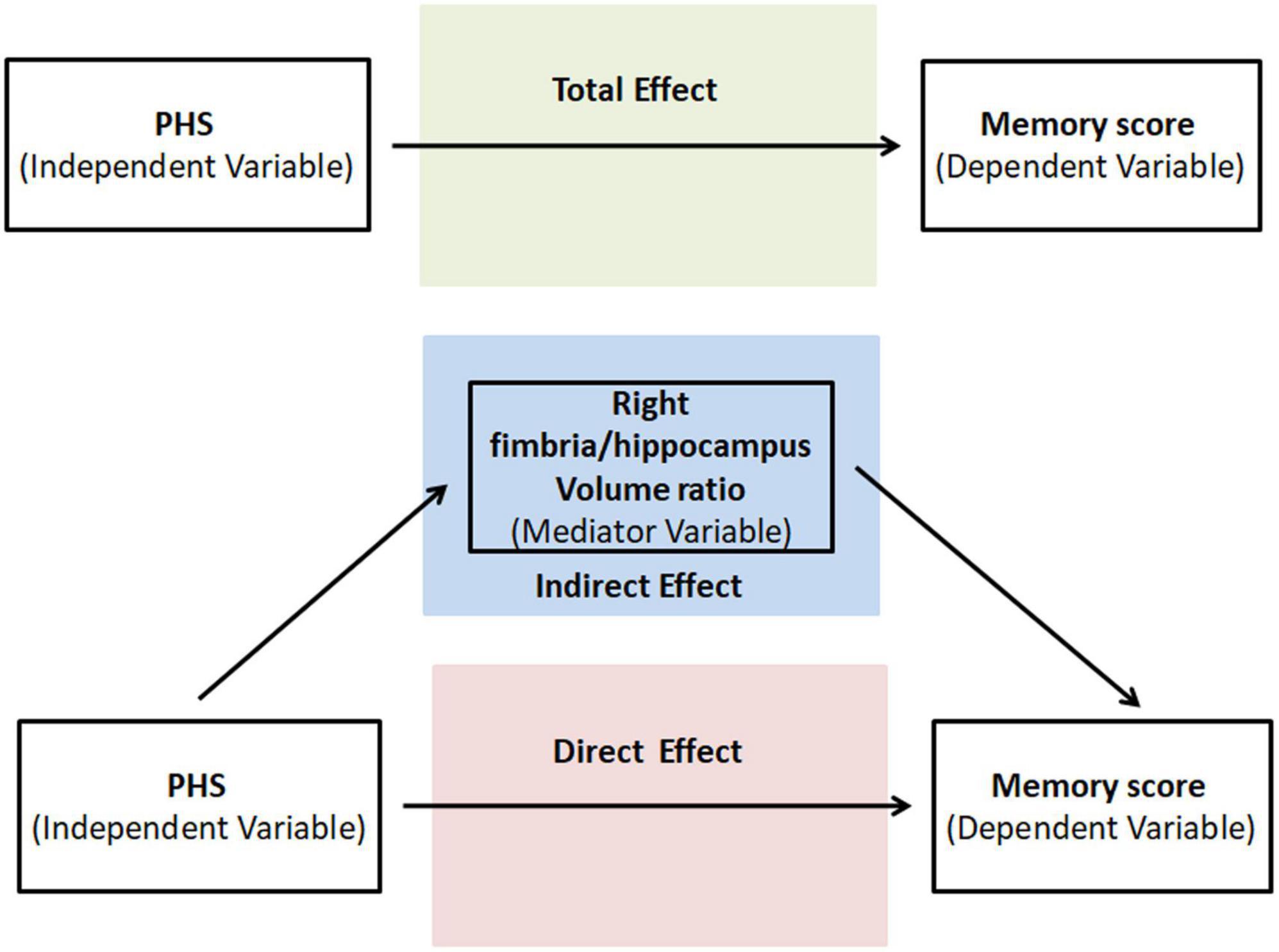
Figure 2. Graphical illustration of the total, direct, and indirect (mediation) statistical models run in the study.
Results
Characteristics of the study population
Table 1 shows the demographic and clinical characteristics of our cohort. Three groups did not differ in terms of age (p = 0.474) and gender (p = 0.460). However, the NC group had a slightly higher educational level than the AD group (p = 0.015). There were significant differences in MMSE, CDR, PHS, and harmonized memory composite scores among the three groups.
Effect of disease status and PHS status on hippocampus subfield to entire ipsilateral hippocampus volume ratio
Among the total participants’ pool, the PHS status was partitioned into two groups, with either high (∼84‰) or low PHS (∼16‰). This point was defined by Tan et al. (2018) using ADNI data. The interaction effect of disease status (NC, MCI, and AD) and PHS status (high and low PHS) on the right fimbria-to-hippocampus volume ratio (R. fimbria/hippocampus VR) was statistically significant (see Table 2). The main effect of PHS was not statistically significant on R. fimbria/hippocampus VR, and the main effect of diagnosis was statistically significant on it as shown in Table 2. There was no significant interaction effect by PHS status × diagnosis found on other hippocampus subfields to eiHP volume ratio (p > 0.05). In the post hoc analysis, AD had significantly lower R. fimbria/hippocampus VR than NC (p < 0.001) and MCI (p = 0.013). But there was no significant difference between NC and MCI (p = 0.130). Since the only significant interaction effect was observed in the right fimbria, the following analysis was only carried out in the right fimbria.
Association of right fimbria/hippocampus volume ratio with memory
To examine the relationships between right fimbria/hippocampus volume ratio and memory score, partial correlation tests were performed in all subjects and within three diagnostic groups adjusted for age, gender, education, and APOE. As expected, a positive correlation between right fimbria/hippocampus VR and memory score was found in all subjects (r = 0.293, p < 0.001). Correlation between them was observed in NC (r = 0.144, p = 0.335), MCI (r = 0.222, p = 0.075), and AD (r = 0.385, p = 0.016).
Effect of PHS status on right fimbria/hippocampus volume ratio with memory
In the overall sample, high PHS status had lower memory scores compared to low PHS status (p = 0.021) (Table 3). In diagnosis-stratified analyses, high PHS status, compared to low PHS status, had lower memory scores (p = 0.020) and larger right fimbria/hippocampus volume ratio (p = 0.002) in the MCI, but not in either the NC or AD. Figure 3 shows that the high genetic risk for ADs outperform low-risk group on memory score among subjects with a large right fimbria/hippocampus volume ratio (right side of the x-axis) but this advantage gradually disappears and reverses to confer memory deficits among subjects with moderate to small VR (left side of the x-axis). Among full sample and MCIs with high PHS status, a lower volume ratio was associated with lower memory scores (full sample high PHS group: p < 0.001, r = 0.398; MCI high PHS group: p = 0.009, r = 0.353; controlling age, gender and education, Figure 3).
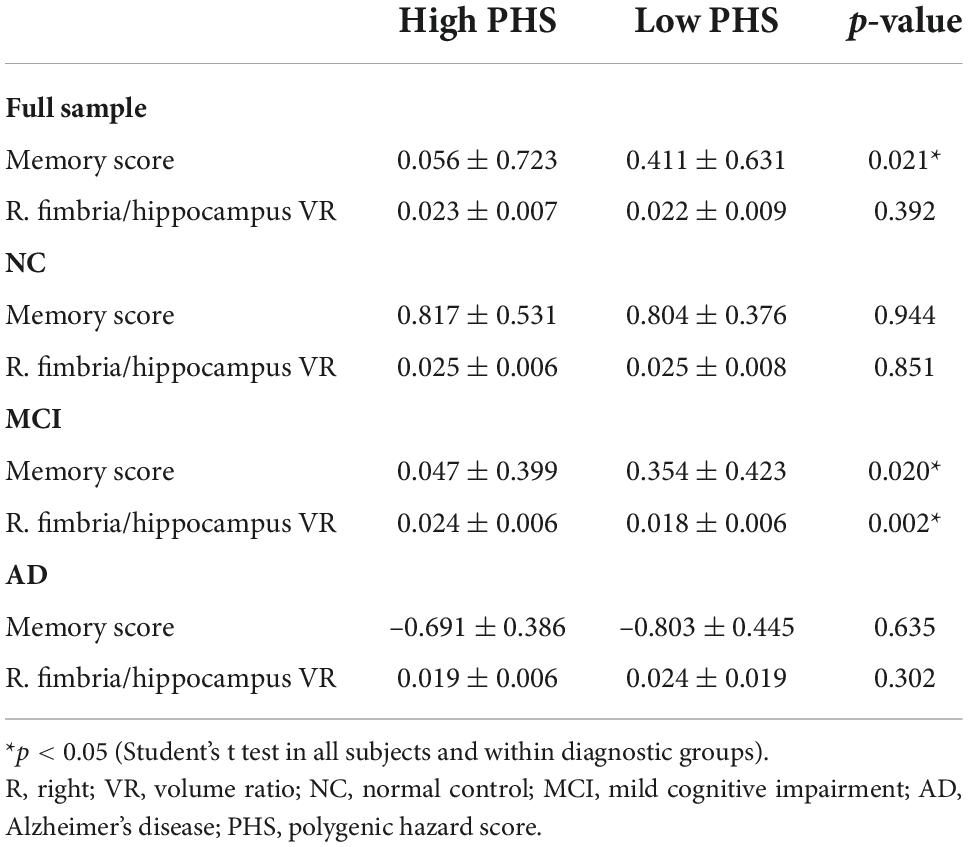
Table 3. Memory score and right fimbria/hippocampus volume ratio by diagnostic groups and PHS status.
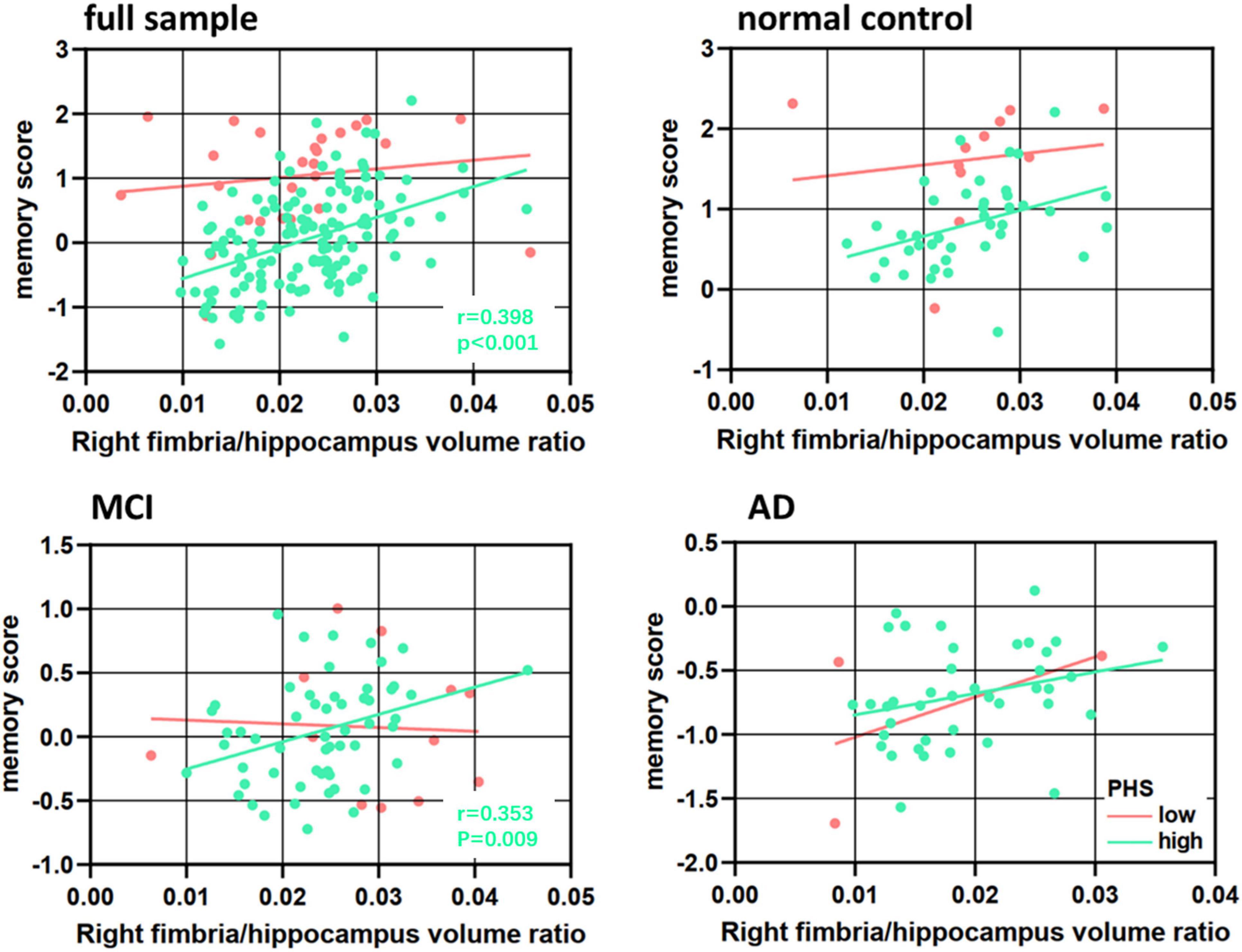
Figure 3. Association between right fimbria/hippocampus volume ratio and memory score in polygenic hazard score (PHS) low and high groups in the full sample, normal controls, mild cognitive impairment (MCI), and Alzheimer’s disease (AD).
PHS moderates the relationship between hippocampal subfield-to-eiHP volume ratio and memory
Polygenic hazard score moderated the relationship between the right fimbria volume ratio and memory, such that patients with high PHS and lower volume ratio had lower episodic memory composite scores (ΔF = 6.730, p = 0.011, ΔR2 = 0.059) (Table 4, Model 3). To parse the interaction effect, partial correlations were used to examine the relationship between right fimbria volume ratio and memory for low and high PHS. Adjusting for all covariates, results revealed that among patients with high PHS, a lower volume ratio was associated with lower memory score (high: r = 0.398, p < 0.001, Figure 3). No significant correlation was found in the low PHS group (low: r = −0.076, p = 0.731, Figure 3). Results revealed a significant difference (Fisher’s z statistic: z = −3.2698, p = 0.0011), indicating a stronger association between right fimbria/hippocampus volume ratio and memory among patients with high PHS compared to those with low PHS.
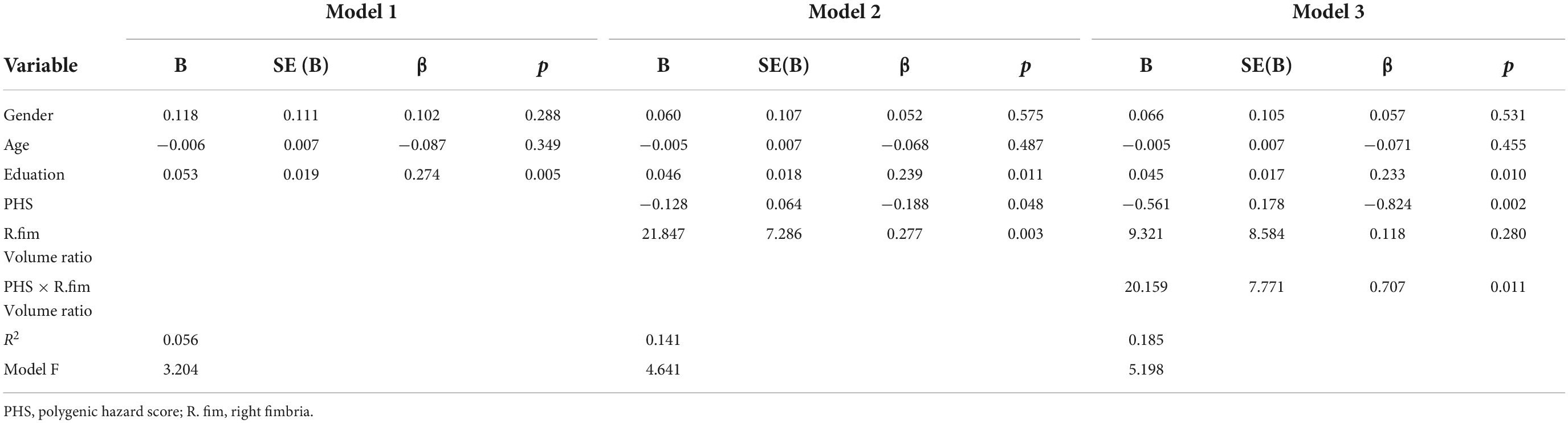
Table 4. Regression analysis for association with right fimbria/hippocampus volume ratio in MCI and AD groups.
Association between PHS and hippocampal subfield-to-eiHP volume ratio along the Alzheimer’s disease continuum
Diagnosis-stratified analyses were conducted for the right fimbria/hippocampus volume ratio which had a significant PHS × volume ratio interaction on memory. There was a significant interaction between right fimbria/hippocampus volume ratio and PHS in MCI (ΔF = 4.519, p = 0.038, ΔR2 = 0.050) (Table 5, Model 3). This relationship was only significant for MCIs with high PHS (r = 0.353, p = 0.009, Figure 3C). More specifically, the association between right fimbria/hippocampus volume ratio and memory was stronger in the high PHS group (r = 0.353, p = 0.009) compared to the low PHS group (r = −0.327, p = 0.391) in MCI. A significant main effect of PHS (r = −0.579, p = 0.003) in the MCI group was observed, such that higher genetic risk was associated with lower memory scores. No interactions were present in NC or AD.
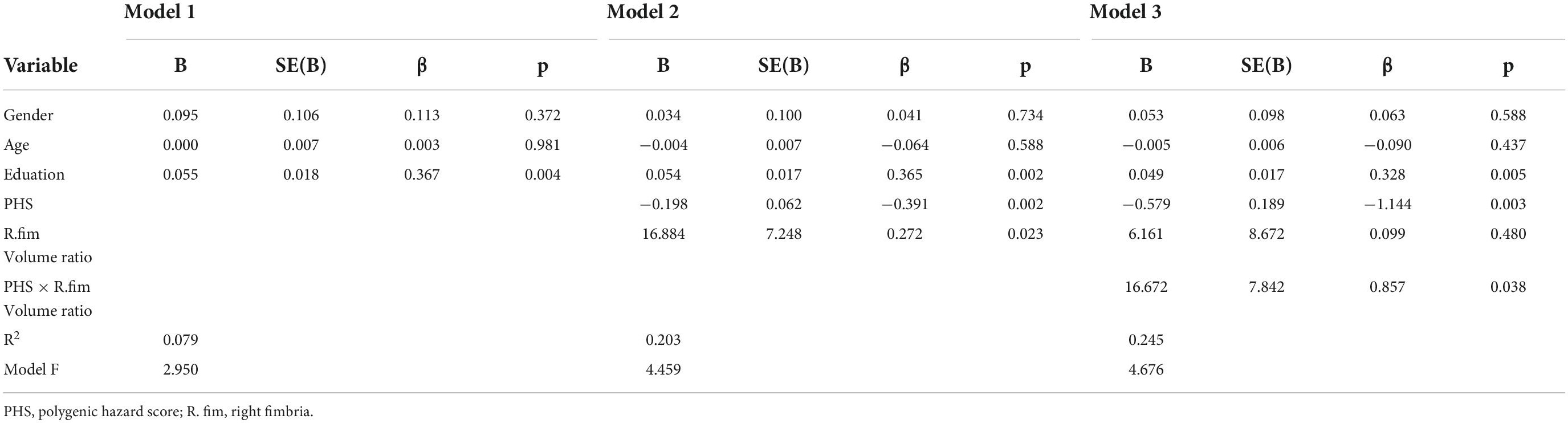
Table 5. Regression analysis for association with right fimbria/hippocampus volume ratio in MCI groups.
Mediation analysis of PHS on memory performance
Mediation models were performed to test the hypothesis that HP subfield VR contributed to PHS-related memory effects. So, we tested the right fimbria/hippocampus volume ratio in all subjects and within three diagnostic groups (Figure 4).
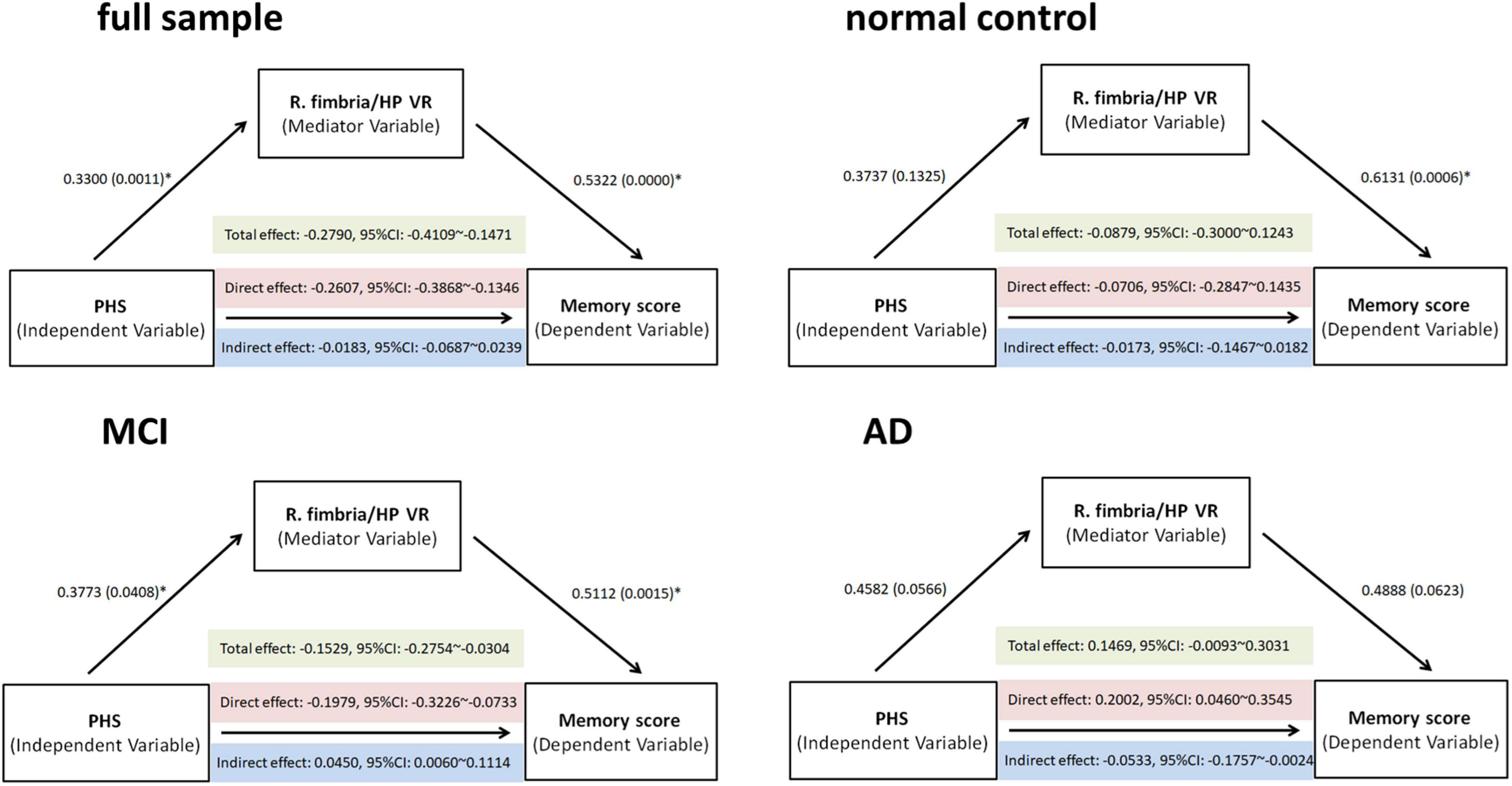
Figure 4. Total, direct, and indirect (mediated) effects of PHS on memory scores were estimated through regression modeling in the full sample, normal controls, mild cognitive impairment (MCI), and Alzheimer’s disease (AD). The effect through the mediating variable—R. fimbria/hippocampus VR—was significant (if bootstrapping 95% CI did not include zero). PHS: polygenic hazard score, R, right, VR, volume ratio; CI, confidence interval.
Mediation analyses revealed a partial mediating effect in the MCI group: (i) a significant total effect of PHS value on the memory score (effect size = −0.1529, p = 0.0015); (ii) a significant direct effect of PHS value on the memory score (effect size = −0.1979, p = 0.0023); (iii) significant indirect effects of increased PHS value on the better memory score mediated by VR (effect size = 0.0450, bootstrapping: standard error = 0.0265, 95%CI: 0.0060–0.1114) (Figure 4). Therefore, PHS might affect memory performance through a change in the R. fimbria/hippocampus VR. No significant mediation effect in the full sample, NC, or AD group was observed.
All of the analyses include APOE genotype as a covariate
Characteristics of the study population
We have added the APOE state in Table 1.
Effect of disease status and PHS status on hippocampus subfield to entire ipsilateral hippocampus volume ratio
The interaction effect of disease status (NC, MCI, and AD) and PHS status (high and low PHS) on the right fimbria-to-hippocampus volume ratio (R. fimbria/hippocampus VR) was statistically significant (Supplementary Table 6: p = 0.020, general linear mixed model controlling age, gender, education, and APOE: ΔF = 4.742, p < 0.001, ΔR2 = 0.172). There was no significant interaction effect by PHS status × diagnosis found on other hippocampus subfields to eiHP volume ratio (p > 0.05).
Association of right fimbria/hippocampus volume ratio with memory
To examine the relationships between right fimbria/hippocampus volume ratio and memory score, partial correlation tests were performed in all subjects and within three diagnostic groups adjusted for age, gender, education, and APOE. As expected, a positive correlation between right fimbria/hippocampus VR and memory score was found in all subjects (r = 0.293, p < 0.001). Correlation between them was observed in NC (r = 0.144, p = 0.335), MCI (r = 0.222, p = 0.075), and AD (r = 0.385, p = 0.016).
Effect of PHS status on right fimbria/hippocampus volume ratio with memory
Among full sample and MCIs with high PHS status, a lower volume ratio was associated with lower memory scores (full sample high PHS group: p < 0.001, r = 0.364; MCI high PHS group: p = 0.008, r = 0.360; controlling age, gender, education, and APOE).
PHS moderates the relationship between hippocampal subfield-to-eiHP volume ratio and memory
Polygenic hazard score moderated the relationship between right fimbria volume ratio and memory, such that patients with high PHS and lower volume ratio had lower episodic memory composite scores (ΔF = 4.730, p = 0.010, ΔR2 = 0.190) (Supplementary Table 7, Model 3). To parse the interaction effect, partial correlations were used to examine the relationship between right fimbria volume ratio and memory for low and high PHS. Adjusting for all covariates including APOE, results revealed that among patients with high PHS, lower volume ratio was associated with lower memory score (high: r = 0.428, p < 0.001). No significant correlation was found in the low PHS group (low: r = −0.389, p = 0.212).
Association between PHS and hippocampal subfield-to-eiHP volume ratio along the Alzheimer’s disease continuum
Diagnosis-stratified analyses were conducted for the right fimbria/hippocampus volume ratio which had a significant PHS × volume ratio interaction on memory. There was a significant interaction between right fimbria/hippocampus volume ratio and PHS in MCI (ΔF = 3.945, p = 0.039, ΔR2 = 0.233) (Supplementary Table 8, Model 3). No interactions were present in NC or AD.
Mediation analysis of PHS on memory performance
Mediation analyses revealed a suppression effect in the MCI group: (i) a non-significant total effect of PHS value on the memory score (effect size = −0.1153, p = 0.3334); (ii) a non-significant direct effect of PHS value on the memory score (effect size = −0.2036, p = 0.0973); and (iii) significant indirect effects of increased PHS value on the better memory score mediated by VR (effect size = 0.0883, bootstrapping: standard error = 0.0495, 95%CI: 0.0127–0.2129) (Supplementary Figure 2). Therefore, PHS without APOE4 might affect memory performance through a change in the R. fimbria/hippocampus VR. But memory performance in MCI can be indicated by a multitude of imaging cues rather than one specific pattern through the right fimbria/hippocampus volume ratio change. No significant mediation effect in the full sample, NC or AD group was observed.
Executive function analysis for validation
Association of right fimbria/hippocampus volume ratio with executive function
To examine the relationships between right fimbria/hippocampus volume ratio and executive function score, partial correlation tests were performed in all subjects and within three diagnostic groups adjusted for age, gender, education, and APOE. As expected, a positive correlation between right fimbria/hippocampus VR and executive function score was found in all subjects (r = 0.181, p = 0.023). Correlation between them was observed in and NC (r = 0.361, p = 0.013), MCI (r = 0.046, p = 0.717), and AD (r = 0.011, p = 0.949).
Effect of PHS status on right fimbria/hippocampus volume ratio with executive function
There was no significant difference found in executive function scores between high and low PHS groups in full sample, NC, MCI, and AD (Supplementary Table 9). Among full sample and NCs with high PHS status, a lower volume ratio was associated with lower executive function scores (full sample high PHS group: p = 0.001, r = 0.278; NC high PHS group: p = 0.004, r = 0.464; controlling age, gender, and education).
PHS moderates the relationship between hippocampal subfield-to-eiHP volume ratio and executive function
Polygenic hazard score tended to moderate the relationship between the right fimbria volume ratio and executive function, such that patients with high PHS and lower volume ratio had lower executive function composite scores (ΔF = 3.053, p = 0.051, ΔR2 = 0.100).
Association between PHS and hippocampal subfield-to-eiHP volume ratio along the Alzheimer’s disease continuum
Diagnosis-stratified analyses were conducted for the right fimbria/hippocampus volume ratio which had a significant PHS × volume ratio interaction on executive function. No interactions were present in NC, MCI, or AD.
Mediation analysis of PHS on executive function
Mediation models were performed to test the hypothesis that HP subfield VR contributed to PHS-related executive function effects. So, we tested the right fimbria/hippocampus volume ratio in all subjects and within three diagnostic groups (Supplementary Figure 3).
Mediation analyses revealed a direct effect in full sample: (i) a significant total effect of the PHS value on executive function score (effect size = −0.1367, p = 0.0345); (ii) a significant direct effect of the PHS value on executive function score (effect size = −0.1259, p = 0.0483); and (iii) non-significant indirect effects mediated by VR (effect size = −0.0108, bootstrapping: standard error = 0.0156, 95%CI: −0.0516 to 0.0118) (Supplementary Figure 3). Therefore, PHS might affect executive function performance directly or through other pathways rather than the right fimbria/hippocampus volume ratio. No significant mediation effect in the NC, MCI, or AD group was observed (Supplementary Figure 3).
Discussion
The purpose of the current study was to examine neurobiological markers that influence cognitive performance related to AD. In this study, we investigated the effect of the PHS and hippocampal subfield volume ratios on a previously validated episodic memory composite score in the three disease status groups (NC, MCI, and AD). There were three main findings. First, a significant interaction between disease status and PHS status was primarily observed in the right fimbria. Second, patients with high PHS and lower right fimbria/hippocampus volume ratio had lower episodic memory composite scores. After conducting diagnosis-specific analyses, this interaction was only observed in the MCI group. Finally, the right fimbria/hippocampus volume ratio partially mediated the effects of PHS on memory performance in the MCI group. Together, these findings suggest that hippocampal subfield volume ratio and polygenic risk for AD represent important markers of episodic memory performance.
We observed no main effect of PHS status but prominent interaction between PHS and disease status (NC, MCI, and AD) in the right fimbria. This finding, although counter-intuitive, is supported by the previous work suggesting that brain injury in AD results from interactions between disease status and PHS (Prieto et al., 2020). We confirmed that the effect of genetic risks for AD on the hippocampus was driven by disease status (e.g., the clinical symptoms). Longitudinal studies exploring healthy aging and transition to MCI and AD will provide further clarity regarding these genetic effects. Further, our volume ratio analysis revealed a decreased VR in the right fimbria in the AD group, compared to the MCI and NC groups. This is reasonable. The fimbria is a white matter structure that extends from the alveus and eventually forms the fornix. It carries axons that emanate primarily from pyramidal neurons in the CA1 and subiculum (Joseph and Cardozo, 2004). In the previous study, a smaller volume of fimbria showed strong associations with poor cognitive/memory performance controlling for total hippocampal volume (Evans et al., 2018) and was commonly found in patients with MCI and AD. This may be, in part, due to its anatomical connections and location within functional pathways. For instance, the fimbria–fornix (FF) bundle, through which noradrenergic and cholinergic afferents reach the hippocampal formation, profoundly affects memory (Buzsáki et al., 1992; Cassel et al., 1997). Thus, the FF-lesion has been used as a model of age-dependent memory deficits (Mercerón-Martínez et al., 2020). So, its integrity is important in preserving the hippocampus’s key role in memory (Nilsson et al., 1987). A recent QSM study showed that the magnetic susceptibility of the fimbria was greater in patients with AD, implying microstructural changes in the tissue (Au et al., 2021). The authors believed that the susceptibility changes may also account for the downstream reduction in hippocampal/fimbrial volume. Diffusion tensor imaging study also has shown changes in the fimbria which were able to explain some variance in the memory tests (Cahn et al., 2021). Despite these promising findings, little is currently known about the biological underpinnings of a fimbria/hippocampus volume ratio, as previous models of neuropathology have primarily focused on the biological mechanisms involved in hippocampal atrophy.
Alterations in the output of hippocampus information due to alterations in the integrity of the fimbria could explain the patients’ reduced memory performance. This proposal seems to be further reinforced by the observed solid and direct correlations between the right fimbria/hippocampus VR and memory score (Figure 3). Our findings are also supported by previous studies, which have shown the reduced fimbria volume and associated altered memory in rats (Winters and Dunnett, 2004; Addy et al., 2005), asymptomatic adults (Tomaiuolo et al., 2004; Zheng et al., 2018), and patients with multiple sclerosis (González Torre et al., 2017). This observation highlights the importance of fine grain analyses at specific hippocampal subfields to detect correlates of memory deficits. In line with this, the destructive effect of the APOEε4 allele on white matter tracts in the right fornix was revealed in old participants, which was positively associated with memory impairment (Gold et al., 2010; Zhang et al., 2015). These findings propose the effects of fimbria volume and genetic risk for AD on memory preceding the development of dementia.
Results of this study showed the utility of the PHS to assess the current levels of hippocampus subfield atrophy and episodic memory function in old people. It is still not fully known through which mechanisms PHS impact AD risk. Still, hippocampus atrophy level and cognitive function are considered intermediate phenotypes that may mediate genetic effects on AD risk (Desikan et al., 2017; Kauppi et al., 2018). Previous studies using both survival analyses and linear mixed effect models showed an improved prediction of AD progression by combining MRI data with cognitive performance, and genetic risk for AD (McEvoy et al., 2009; Li et al., 2016; Kauppi et al., 2018). The predictive capabilities of PHS may have contributed to our finding of genetics moderating the relationship between the right fimbria/hippocampus VR and episodic memory. Similar findings were observed in a prior study in old adults (Prieto et al., 2020) that the PHS moderated episodic memory through the left hippocampus in the MCI group.
Whereas our moderation analysis results are consistent with those previously found for PHS (Prieto et al., 2020), our meditational analyses reported novel findings. Mediation models in cognitive neurogenetic research provide a useful framework for directional hypotheses, which are generally appropriate for cognitive neurogenetics because effects generally transmit from genes to brain and cognition, rather than vice versa. The strong total, direct effects, and partial indirect effect of the PHS on episodic memory through the right fimbria/hippocampus VR may reflect specific hippocampus subfield atrophy patterns in differential diagnosis and prognosis of AD (Mizutani and Kasahara, 1997; de Flores et al., 2015; Zeng et al., 2021), which in turn alters episodic memory performance. The data indicate a gene–brain–cognition effect pathway (Green et al., 2013), whereby PHS influences episodic memory via downregulation in the right fimbria volume ratio in the MCI group. Investigators reported genetic variants influencing hippocampal subfield volume also modified the risk of developing AD (Hibar et al., 2017). The larger volume of the hippocampus subfields might have translated into behavioral advantages. Moreover, neuronal activity within specific subfields of the hippocampus might serve distinct aspects of memory (Squire, 2004; Mueller et al., 2011). Therefore, subfield-specific effects of PHS might further underline a proposed role for genotype in particular aspects of memory formation. For example, fimbria appears to support episodic detail generation, as well as retrieval of other types of episodic content (St-Laurent et al., 2014, 2016; Memel et al., 2020). Improved volume and better microstructure in fimbria have been associated with better memory performance in MCI subjects (Pereira et al., 2016; Kantarci et al., 2017; Berron et al., 2020). Thus, the observed decreased volume ratio in fimbria could have contributed to poorer memory results with high PHS status.
Interestingly, the moderate and partial mediated effect was most evident among patients with MCI, indicating that the complicated pathological process related to hippocampus atrophy and memory performance changes along the Alzheimer’s disease continuum. Genetic effects known to contribute to AD pathogenesis can modulate adult hippocampal neurogenesis (Berron et al., 2020). Notably, these alterations may occur at the very early stage of AD progression, prior to processes like neuronal loss and amyloid deposition which might lead to memory impairment (Jin et al., 2004; Mu and Gage, 2011). Further, episodic memory was also affected in the earliest stages of AD (Backman et al., 2001; Gold and Budson, 2008). This is consistent with functional imaging studies showing that in the first few years of AD different hippocampal subfields were affected in memory network organization and maintenance (Li et al., 2009; Yassa et al., 2010; Sun et al., 2017). In the AD group, mediation analyses revealed a suppression effect: we found no significant total effect of PHS on memory (effect size = 0.1469, 95%CI: −0.0093 to 0.3031), while the indirect effect of PHS on memory was negative (effect size = −0.0533, 95%CI: −0.1757 to −0.0024) and the direct effect was positive (effect size = 0.2002, 95%CI: 0.0460–0.3545). It is possible that by the time individuals progress to AD, even the combination of genetic risk and hippocampus subfield volumes can no longer explain the variance in episodic memory performance. Because these patients with AD probably suffer from great and multiple brain regions volume loss in structural plasticity and further along a cognitive decline trajectory. A promising avenue for future research on this topic could be the construct of multiple subfields of atrophy patterns as the mediate variable to test the effects of PHS on memory. Considering APOE is strongly associated with both cognition and hippocampal volumes even in very early cases (Haller et al., 2019; Herrmann et al., 2019), we have completed all the analyses including APOE genotype as a covariate. We found that a PHS score without APOE4 contribution keeps the same effect.
In addition, in our executive function analysis for validation, we found that the right fimbria/hippocampus VR had distinct associations with executive function. PHS tended to moderate the relationship between right fimbria volume ratio and executive function, such that patients with high PHS and lower volume ratio might have lower executive function composite scores. Significant total and direct effect but no significant mediation effect was found to test the hypothesis that HP subfield VR contributed to PHS-related executive function effects. The reason why this result was found in the executive function data may be that executive function performance can be indicated by a multitude of imaging cues rather than one specific pattern through the right fimbria/hippocampus volume ratio change. A promising avenue for future research on this topic could be the construct of multiple subfields of atrophy patterns as the mediate variable to test the effects of PHS on executive function. The results highlighted a specific pathway in which PHS affects memory rather than executive function by degrading the right fimbria/hippocampus VR.
Limitation
Our study has several limitations. First, we used T1-weighted images provided by a 3T MR scanner for hippocampal subfield segmentation. Sometimes it may be challenging to determine the boundaries, especially in several small volume subfields. Future studies using 7T MRI would be more precise to measure subfields. Second, since this was the first study to explain a potential pathway associating PHS-related episodic memory alteration with the hippocampus subfield volume ratio modification by structural MRI in old adults (all the MCIs were amnestic MCIs), studies in different cohorts, or recruiting a larger and more diverse population, may be needed to extend our findings. Third, because of the cross-sectional and retrospective design, we were unable to prove that alterations in hippocampus subfield volume ratios were actually a consequence of the high genetic risk for AD. Lastly, there must be other variables affecting the relationship between the hippocampus and memory performance that need to be further discussed.
Conclusion
The present study demonstrated the mediating effect of hippocampal subfield volume ratio and the moderating effect of polygenetic risk for AD between hippocampus subfield volume ratio and episodic memory performance. Genetic risk and hippocampal subfields should be considered as key variables in models tracking the progression of cognitive decline in healthy and pathological aging.
Data availability statement
The original contributions presented in the study are included in the article/Supplementary material, further inquiries can be directed to the corresponding author.
Ethics statement
The studies involving human participants were reviewed and approved by the Ethics committee of the Second Affiliated Hospital of Zhejiang University, School of Medicine. The patients/participants provided their written informed consent to participate in this study. Written informed consent was obtained from the individual(s) for the publication of any potentially identifiable images or data included in this article.
Author contributions
JX designed the study and wrote the first draft of the manuscript. XG analyzed the MRI data. JW, MZ, and XX assisted with the study design and interpretation of findings. All authors have contributed to and approved the final manuscript.
Funding
Data collection and sharing for this project were funded by the Alzheimer’s Disease Neuroimaging Initiative (ADNI) (National Institutes of Health Grant U01 AG024904) and DOD ADNI (Department of Defense award number W81XWH-12-2-0012). ADNI was funded by the National Institute on Aging, the National Institute of Biomedical Imaging and Bioengineering, and through generous contributions from the following: AbbVie, Alzheimer’s Association; Alzheimer’s Drug Discovery Foundation; Araclon Biotech; BioClinica, Inc.; Biogen; Bristol-Myers Squibb Company; CereSpir, Inc.; Cogstate; Eisai Inc.; Elan Pharmaceuticals, Inc.; Eli Lilly and Company; EuroImmun; F. Hoffmann-La Roche Ltd. and its affiliated company Genentech, Inc.; Fujirebio; GE Healthcare; IXICO Ltd.; Janssen Alzheimer’s Immunotherapy Research & Development, LLC.; Johnson & Johnson Pharmaceutical Research & Development LLC.; Lumosity; Lundbeck; Merck & Co., Inc.; Meso Scale Diagnostics, LLC.; NeuroRx Research; Neurotrack Technologies; Novartis Pharmaceuticals Corporation; Pfizer Inc.; Piramal Imaging; Servier; Takeda Pharmaceutical Company; and Transition Therapeutics. The Canadian Institutes of Health Research is providing funds to support ADNI clinical sites in Canada. Private sector contributions are facilitated by the Foundation for the National Institutes of Health (www.fnih.org). The grantee organization is the Northern California Institute for Research and Education, and the study is coordinated by the Alzheimer’s Therapeutic Research Institute at the University of Southern California. ADNI data are disseminated by the Laboratory for Neuro Imaging at the University of Southern California.
Conflict of interest
The authors declare that the research was conducted in the absence of any commercial or financial relationships that could be construed as a potential conflict of interest.
Publisher’s note
All claims expressed in this article are solely those of the authors and do not necessarily represent those of their affiliated organizations, or those of the publisher, the editors and the reviewers. Any product that may be evaluated in this article, or claim that may be made by its manufacturer, is not guaranteed or endorsed by the publisher.
Supplementary material
The Supplementary Material for this article can be found online at: https://www.frontiersin.org/articles/10.3389/fnagi.2022.943702/full#supplementary-material
Footnotes
References
Addy, N. A., Pocivavsek, A., and Levin, E. D. (2005). Reversal of clozapine effects on working memory in rats with fimbria-fornix lesions. Neuropsychopharmacology 30, 1121–1127. doi: 10.1038/sj.npp.1300669
Apostolova, L. G., Mosconi, L., Thompson, P. M., Green, A. E., Hwang, K. S., Ramirez, A., et al. (2010). Subregional hippocampal atrophy predicts Alzheimer’s dementia in the cognitively normal. Neurobiol. Aging 31, 1077–1088. doi: 10.1016/j.neurobiolaging.2008.08.008
Au, C. K. F., Abrigo, J., Liu, C., Liu, W., Lee, J., Au, L. W. C., et al. (2021). Quantitative susceptibility mapping of the hippocampal fimbria in Alzheimer’s disease. J. Magn. Reson. Imaging 53, 1823–1832. doi: 10.1002/jmri.27464
Backman, L., Small, B. J., and Fratiglioni, L. (2001). Stability of the preclinical episodic memory deficit in Alzheimer’s disease. Brain 124, 96–102. doi: 10.1093/brain/124.1.96
Ball, M. J. (1997). Neuronal loss, neurofibrillary tangles and granulovacuolar degeneration in the Hippocampus with ageing and dementia. A quantitative study. Acta Neuropathol. 37, 111–118. doi: 10.1007/BF00692056
Benjamini, Y., and Hochberg, Y. (1995). Controlling the false discovery rate: A practical and powerful approach to multiple testing. J. R. Stat. Soc. Series B Stat. Methodol. 57, 289–300.
Berron, D., van Westen, D., Ossenkoppele, R., Strandberg, O., and Hansson, O. (2020). Medial temporal lobe connectivity and its associations with cognition in early Alzheimer’s disease. Brain 143, 1233–1248. doi: 10.1093/brain/awaa068
Bettens, K., Sleegers, K., and Van Broeckhoven, C. (2013). Genetic insights in Alzheimer’s disease. Lancet Neurol. 12, 92–104. doi: 10.1016/S1474-4422(12)70259-4
Braak, H., and Braak, E. (1990). Alzheimer’s disease, striatal amyloid deposits and neurofibrillary changes. J. Neuropathol. Exp. Neurol. 49, 215–224.
Burwell, R. D., and Agster, K. L. (2008). “Anatomy of the Hippocampus and the declarative memory system,” in Systems and neuroscience, ed. H. E. Eichenbaum (Oxford: Elsevier), 47–66.
Buzsáki, G., Hsu, M., Horváth, Z., and Gage, F. H. (1992). Physiological and behavioral consequences of delayed septal grafts in the subcortically denervated hippocampus. Restor. Neurol. Neurosci. 4, 369–380. doi: 10.3233/RNN-1992-4602
Cahn, A. J., Little, G., Beaulieu, C., and Tétreault, P. (2021). Diffusion properties of the fornix assessed by deterministic tractography shows age, sex, volume, cognitive, hemispheric, and twin relationships in young adults from the human connectome project. Brain Struct. Funct. 226, 381–395. doi: 10.1007/s00429-020-02181-9
Caselli, R. J., Dueck, A. C., Osborne, D., Sabbagh, M. N., Connor, D. J., Ahern, G. L., et al. (2009). Longitudinal modeling of age-related memory decline and the APOE epsilon4 effect. N. Engl. J. Med. 361, 255–263. doi: 10.1056/NEJMoa0809437
Cassel, J. C., Duconseille, E., Jeltsch, H., and Will, B. (1997). The fimbria-fornix/cingular bundle pathways: A review of neurochemical and behavioural approaches using lesions and transplantation techniques. Prog. Neurobiol. 51, 663–716. doi: 10.1016/s0301-0082(97)00009-9
Crane, P. K., Carle, A., Gibbons, L. E., Insel, P., Mackin, R. S., Gross, A., et al. (2012). Development and assessment of a composite score for memory in the Alzheimer’s disease neuroimaging initiative (ADNI). Brain Imaging Behav. 6, 502–516. doi: 10.1007/s11682-012-9186-z
de Flores, R., La Joie, R., and Chetelat, G. (2015). Structural imaging of hippocampal subfields in healthy aging and Alzheimer’s disease. Neuroscience 309, 29–50. doi: 10.1016/j.neuroscience.2015.08.033
Desikan, R. S., Fan, C. C., Wang, Y., Schork, A. J., Cabral, H. J., Cupples, L. A., et al. (2017). Genetic assessment of age-associated Alzheimer disease risk: Development and validation of a polygenic hazard score. PLoS Med. 14:e1002258. doi: 10.1371/journal.pmed.1002258
Duvernoy, H. M. (2008). The human Hippocampus, functional anatomy, vascularization and serial sections with MRI, 3rd Edn. Berlin: Springer-Verlag.
Elias, M. F., Beiser, A., Wolf, P. A., Au, R., White, R. F., and D’Agostino, R. B. (2000). The preclinical phase of Alzheimer disease: A 22-year prospective study of the Framingham Cohort. Arch. Neurol. 57, 808–813. doi: 10.1001/archneur.57.6.808
Evans, T. E., Adams, H. H. H., Licher, S., Wolters, F. J., van der Lugt, A., Ikram, M. K., et al. (2018). Subregional volumes of the Hippocampus in relation to cognitive function and risk of dementia. Neuroimage 178, 129–135. doi: 10.1016/j.neuroimage.2018.05.041
Gatz, M., Reynolds, C. A., Fratiglioni, L., Johansson, B., Mortimer, J. A., Berg, S., et al. (2006). Role of genes and environments for explaining Alzheimer disease. Arch. Gen. Psychiatry 63, 168–174. doi: 10.1001/archpsyc.63.2.168
Goate, A., Chartier-Harlin, M. C., Mullan, M., Brown, J., Crawford, F., Fidani, L., et al. (1991). Segregation of a missense mutation in the amyloid b-protein precursor gene with familial Alzheimer’s disease. Nature 349, 704–706. doi: 10.1038/349704a0
Gold, B. T., Powell, D. K., Andersen, A. H., and Smith, C. D. (2010). Alterations in multiple measures of white matter integrity in normal women at high risk for Alzheimer’s disease. Neuroimage 52, 1487–1494. doi: 10.1016/j.neuroimage.2010.05.036
Gold, C. A., and Budson, A. E. (2008). Memory loss in Alzheimer’s disease: Implications for development of therapeutics. Expert Rev. Neurother. 8, 1879–1891. doi: 10.1586/14737175.8.12.1879
González Torre, J. A., Cruz-Gómez, ÁJ., Belenguer, A., Sanchis-Segura, C., Ávila, C., and Forn, C. (2017). Hippocampal dysfunction is associated with memory impairment in multiple sclerosis: A volumetric and functional connectivity study. Mult. Scler. 23, 1854–1863. doi: 10.1177/1352458516688349
Green, A. E., Kraemer, D. J. M., Deyoung, C. G., Fossella, J. A., and Gray, J. R. (2013). A gene-brain-cognition pathway: Prefrontal activity mediates the effect of COMT on cognitive control and IQ. Cereb. Cortex 23, 552–559. doi: 10.1093/cercor/bhs035
Haller, S., Montandon, M. L., Rodriguez, C., Garibotto, V., Herrmann, F. R., and Giannakopoulos, P. (2019). Hippocampal volume loss, brain amyloid accumulation, and APOE Status in cognitively intact elderly subjects. Neurodegener. Dis. 19, 139–147. doi: 10.1159/000504302
Han, S. D., and Bondi, M. W. (2008). Revision of the apolipoprotein E compensatory mechanism recruitment hypothesis. Alzheimers Dement. 4, 251–254. doi: 10.1016/j.jalz.2008.02.006
Harper, L., Barkhof, F., Scheltens, P., Schott, J. M., and Fox, N. C. (2014). An algorithmic approach to structural imaging in dementia. J. Neurol. Neurosurg. Psychiatry 85, 692–698. doi: 10.1136/jnnp-2013-306285
Hayes, A. F. (2013). Introduction to mediation, moderation, and conditional process analysis: A regression-based approach. New York, NY: Guilford Press.
Herrmann, F. R., Rodriguez, C., Haller, S., Garibotto, V., Montandon, M. L., and Giannakopoulos, P. (2019). Gray matter densities in limbic areas and APOE4 Independently predict cognitive decline in normal brain aging. Front. Aging Neurosci. 11:157. doi: 10.3389/fnagi.2019.00157
Hibar, D. P., Adams, H. H. H., Jahanshad, N., Chauhan, G., Stein, J. L., Hofer, E., et al. (2017). Novel genetic loci associated with hippocampal volume. Nat. Commun. 8:13624. doi: 10.1038/ncomms13624
Iglesias, J. E., Augustinack, J. C., Nguyen, K., Player, C. M., Player, A., Wright, M., et al. (2015). A computational atlas of the hippocampal formation using ex vivo, ultra-high resolution MRI: Application to adaptive segmentation of in vivo MRI. Neuroimage 115, 117–137. doi: 10.1016/j.neuroimage.2015.04.042
Jia, J., Wei, C., Chen, S., Li, F., Tang, Y., Qin, W., et al. (2018). The cost of Alzheimer’s disease in China andre-estimation of costs worldwide. Alzheimers Dement. 14, 483–491. doi: 10.1016/j.jalz.2017.12.006
Jin, K., Galvan, V., Xie, L., Mao, X. O., Gorostiza, O. F., Bredesen, D. E., et al. (2004). Enhanced neurogenesis in Alzheimer’s disease transgenic (PDGF-APPSw, Ind) mice. Proc. Natl. Acad. Sci. U.S.A. 101, 13363–13367. doi: 10.1073/pnas.0403678101
Joseph, J. T., and Cardozo, D. L. (2004). Functional neuroanatomy: An interactive text and manual. Hoboken, NJ: Wiley-Liss.
Kantarci, K., Murray, M. E., Schwarz, C. G., Reid, R. I., Przybelski, S. A., Lesnick, T., et al. (2017). White-matter integrity on DTI and the pathologic staging of Alzheimer’s disease. Neurobiol. Aging 56, 172–179. doi: 10.1016/j.neurobiolaging.2017.04.024
Kauppi, K., Fan, C. C., McEvoy, L. K., Holland, D., Tan, C. H., Chen, C. H., et al. (2018). Combining polygenic hazard score with volumetric MRI and Cognitive measures improves prediction of progression from mild cognitive impairment to Alzheimer’s disease. Front. Neurosci. 30:260. doi: 10.3389/fnins.2018.00260
Kilpatrick, C., Murrie, V., Cook, M., Andrewes, D., Desmond, P., and Hopper, J. (1997). Degree of left hippocampal atrophy correlates with severity of neuropsychological deficits. Seizure 6, 213–218. doi: 10.1016/s1059-1311(97)80008-8
Levy-Lahad, E., Wasco, W., Poorkaj, P., Romano, D. M., Oshima, J., Pettingell, W. H., et al. (1995). Candidate gene for the chromosome 1 familial Alzheimer’s disease locus. Science 1995, 973–977. doi: 10.1126/science.7638622
Li, J. Q., Tan, L., Wang, H. F., Tan, M. S., Tan, L., Xu, W., et al. (2016). Risk factors for predicting progression from mild cognitive impairment to Alzheimer’s disease: A systematic review and meta-analysis of cohort studies. J. Neurol. Neurosurg. Psychiatry 87, 476–484. doi: 10.1136/jnnp-2014-310095
Li, Y., Mu, Y., and Gage, F. H. (2009). Development of neural circuits in the adult Hippocampus. Curr. Top. Dev. Biol. 87, 149–174.
McEvoy, L. K., Fennema-Notestine, C., Roddey, J. C., Hagler, D. J., Holland, D., Karow, D. S., et al. (2009). Alzheimer disease: Quantitative structural neuroimaging for detection and prediction of clinical and structural changes in mild cognitive impairment. Radiology 251, 195–205. doi: 10.1148/radiol.2511080924
Memel, M., Wank, A. A., Ryan, L., and Grilli, M. D. (2020). The relationship between episodic detail generation and anterotemporal, posteromedial, and hippocampal white matter tracts. Cortex 123, 124–140. doi: 10.1016/j.cortex.2019.10.010
Mercerón-Martínez, D., Almaguer-Melian, W., Alberti-Amador, E., Calderón-Peña, R., and Bergado, J. A. (2020). Amygdala stimulation ameliorates memory impairments and promotes c-Fos activity in fimbria-fornix-lesioned rats. Synapse 74:e22179. doi: 10.1002/syn.22179
Mizutani, T., and Kasahara, M. (1997). Hippocampal atrophy secondary to entorhinal cortical degeneration in Alzheimer- type dementia. Neurosci. Lett. 222, 119–122. doi: 10.1016/s0304-3940(97)13365-1
Mu, Y., and Gage, F. H. (2011). Adult hippocampal neurogenesis and its role in Alzheimer’s disease. Mol. Neurodegener. 6:85.
Mueller, S. G., Chao, L. L., Berman, B., and Weiner, M. W. (2011). Evidence for functional specialization of hippocampal subfields detected by MR subfield volumetry on high resolution images at 4T. Neuroimage 56, 851–857. doi: 10.1016/j.neuroimage.2011.03.028
Nilsson, O. G., Shapiro, M. L., Gage, F. H., Olton, D. S., and Björklund, A. (1987). Spatial learning and memory following fimbria-fornix transection and grafting of fetal septal neurons to the Hippocampus. Exp. Brain Res. 67, 195–215. doi: 10.1007/BF00269466
Pereira, J. B., Mijalkov, M., Kakaei, E., Mecocci, P., Vellas, B., Tsolaki, M., et al. (2016). Disrupted network topology in patients with stable and progressive mild cognitive impairment and Alzheimer’s disease. Cereb. Cortex 26, 3476–3493. doi: 10.1093/cercor/bhw128
Petersen, R. C., Aisen, P. S., Beckett, L. A., Donohue, M. C., Gamst, A. C., Harvey, D. J., et al. (2010). Alzheimer’s disease neuroimaging initiative (ADNI): Clinical characterization. Neurology 74, 201–209. doi: 10.1212/WNL.0b013e3181cb3e25
Pluta, J., Yushkevich, P., Das, S., and Wolk, D. (2012). In vivo analysis of hippocampal subfield atrophy in mild cognitive impairment via semi-automatic segmentation of T2-weighted MRI. J. Alzheimers Dis. 31, 85–99. doi: 10.3233/JAD-2012-111931
Preacher, K. J., and Hayes, A. F. (2004). SPSS and SAS procedures for estimating indirect effects in simple mediation models. Behav. Res. Methods Instrum. Comput. 36, 717–731. doi: 10.3758/bf03206553
Prieto, S., Valerio, K. E., Moody, J. N., Hayes, S. M., Hayes, J. P., and Alzheimer’s Disease Neuroimaging Initiative (2020). Genetic risk for Alzheimer’s disease moderates the association between medial temporal lobe volume and episodic memory performance among older adults. J. Alzheimers Dis. 76, 591–600. doi: 10.3233/JAD-191312
Prince, M., Bryce, R., Albanese, E., Wimo, A., Ribeiro, W., and Ferri, C. P. (2013). The global prevalence of dementia: A systematic review and meta-analysis. Alzheimers Dement. 9, 63–75. doi: 10.1016/j.jalz.2012.11.007
Rogaev, E., Sherrington, R., Rogaeva, E., Levesque, G., Ikeda, M., Liang, Y., et al. (1995). Familial Alzheimer’s disease in kindreds with missense mutations in a gene on chromosome 1 related to the Alzheimer’s disease type 3 gene. Nature 376, 775–778. doi: 10.1038/376775a0
Schonheit, B., Zarski, R., and Ohm, T. G. (2004). Spatial and temporal relationships between plaques and tangles in Alzheimer-pathology. Neurobiol. Aging 25, 697–711. doi: 10.1016/j.neurobiolaging.2003.09.009
Small, B. J., Herlitz, A., Fratiglioni, L., Almkvist, O., and Backman, L. (1997). Cognitive predictors of incident Alzheimer’s disease: A prospective longitudinal study. Neuropsychology 11, 413–420. doi: 10.1037//0894-4105.11.3.413
Squire, L. R. (2004). Memory systems of the brain: A brief history and current perspective. Neurobiol. Learn. Mem. 82, 171–177. doi: 10.1016/j.nlm.2004.06.005
St-Laurent, M., Moscovitch, M., and McAndrews, M. P. (2016). The retrieval of perceptual memory details depends on right hippocampal integrity and activation. Cortex 84, 15–33. doi: 10.1016/j.cortex.2016.08.010
St-Laurent, M., Moscovitch, M., Jadd, R., and McAndrews, M. P. (2014). The perceptual richness of complex memory episodes is compromised by medial temporal lobe damage. Hippocampus 24, 560–566. doi: 10.1002/hipo.22249
Strittmatter, W. J., Saunders, A. M., Schmechel, D., Pericak-Vance, M., Enghild, J., Salvesen, G. S., et al. (1993). Apolipoprotein E: High-avidity binding to beta-amyloid and increased frequency of type 4 allele in late-onset familial Alzheimer disease. Proc. Natl. Acad. Sci. U.S.A. 90, 1977–1981. doi: 10.1073/pnas.90.5.1977
Sun, Y., Wang, Y., Lu, J., Liu, R., Schwarz, C. G., Zhao, H., et al. (2017). Disrupted functional connectivity between perirhinal and parahippocampal cortices with hippocampal subfields in patients with mild cognitive impairment and Alzheimer’s disease. Oncotarget 8, 99112–99124. doi: 10.18632/oncotarget.17944
Tan, C. H., Bonham, L. W., Fan, C. C., Mormino, E. C., Sugrue, L. P., Broce, I. J., et al. (2019). Polygenic hazard score, amyloid deposition and Alzheimer’s neurodegeneration. Brain 142, 460–470. doi: 10.1093/brain/awy327
Tan, C. H., Fan, C. C., Mormino, E. C., Sugrue, L. P., Broce, I. J., Hess, C. P., et al. (2018). Polygenic hazard score: An enrichment marker for Alzheimer’s associated amyloid and tau deposition. Acta Neuropathol. 135, 85–93. doi: 10.1007/s00401-017-1789-4
Tomaiuolo, F., Carlesimo, G. A., Di, P. M., Petrides, M., Fera, F., Bonanni, R., et al. (2004). Gross morphology and morphometric sequelae in the Hippocampus, fornix, and corpus callosum of patients with severe non-missile traumatic brain injury without macroscopically detectable lesions: A T1 weighted MRI study. J. Neurol. Neurosurg. Psychiatry 75, 1314–1322. doi: 10.1136/jnnp.2003.017046
Tuminello, E. R., and Han, S. D. (2011). The apolipoprotein e antagonistic pleiotropy hypothesis: Review and recommendations. Int. J. Alzheimers Dis. 2011:726197. doi: 10.4061/2011/726197
Wang, X., Zhou, W., Ye, T., Lin, X., Zhang, J., and Alzheimer’s Disease Neuroimaging Initiative (2019). The relationship between hippocampal volumes and delayed recall is modified by APOE ε 4 in mild cognitive impairment. Front. Aging Neurosci. 11:36. doi: 10.3389/fnagi.2019.00036
Weiner, M. W., Veitch, D. P., Aisen, P. S., Beckett, L. A., Cairns, N. J., Green, R. C., et al. (2013). The Alzheimer’s disease neuroimaging initiative: A review of papers published since its inception. Alzheimers Dement. 9, e111–e194. doi: 10.1016/j.jalz.2013.05.1769
Winters, B. D., and Dunnett, S. B. (2004). Selective lesioning of the cholinergic septo-hippocampal pathway does not disrupt spatial short-term memory: A comparison with the effects of fimbria-fornix lesions. Behav. Neurosci. 118, 546–562. doi: 10.1037/0735-7044.118.3.546
Yassa, M. A., Stark, S. M., Bakker, A., Albert, M. S., Gallagher, M., and Stark, C. E. (2010). High-resolution structural and functional MRI of hippocampal CA3 and dentate gyrus in patients with amnestic mild cognitive impairment. Neuroimage 51, 1242–1252. doi: 10.1016/j.neuroimage.2010.03.040
Ystad, M. A., Lundervold, A. J., Wehling, E., Espeseth, T., Rootwelt, H., Westlye, L. T., et al. (2009). Hippocampal volumes are important predictors for memory function in elderly women. BMC Med. Imaging 9:17. doi: 10.1186/1471-2342-9-17
Zarow, C., Wang, L., Chui, H. C., Weiner, M. W., and Csernansky, J. G. (2011). MRI shows more severe hippocampal atrophy and shape deformation in hippocampal sclerosis than in Alzheimer’s disease. Int. J. Alzheimers Dis. 2011:483972. doi: 10.4061/2011/483972
Zeng, Q., Li, K., Luo, X., Wang, S., Xu, X., Li, Z., et al. (2021). Distinct atrophy pattern of hippocampal subfields in patients with progressive and stable mild cognitive impairment: A longitudinal MRI study. J. Alzheimers Dis. 79, 237–247.
Zhang, S., Chen, Y., Liu, Z., Zhang, J., Li, X., Cui, R., et al. (2015). Association of white matter integrity and cognitive functions in Chinese non-demented elderly with the APOE ε4 allele. J. Alzheimers Dis. 48, 781–791. doi: 10.3233/JAD-150357
Keywords: Alzheimer’s disease, hippocampus subfield, episodic memory, polygenic hazard score, volume ratio
Citation: Xu J, Guan X, Wen J, Zhang M and Xu X (2022) Polygenic hazard score modified the relationship between hippocampal subfield atrophy and episodic memory in older adults. Front. Aging Neurosci. 14:943702. doi: 10.3389/fnagi.2022.943702
Received: 14 May 2022; Accepted: 30 September 2022;
Published: 31 October 2022.
Edited by:
Nilton Custodio, Peruvian Institute of Neurosciences (IPN), PeruReviewed by:
Xiaopu Zhou, Hong Kong University of Science and Technology, Hong Kong SAR, ChinaPanteleimon Giannakopoulos, Université de Genève, Switzerland
Copyright © 2022 Xu, Guan, Wen, Zhang and Xu. This is an open-access article distributed under the terms of the Creative Commons Attribution License (CC BY). The use, distribution or reproduction in other forums is permitted, provided the original author(s) and the copyright owner(s) are credited and that the original publication in this journal is cited, in accordance with accepted academic practice. No use, distribution or reproduction is permitted which does not comply with these terms.
*Correspondence: Xiaojun Xu, xxjmailbox@zju.edu.cn
†Data used in the preparation of this article were obtained from the Alzheimer’s disease Neuroimaging Initiative (ADNI) database (https://adni.loni.usc.edu). As such, the investigators within the ADNI contributed to the design and implementation of ADNI and provided data but did not participate in the analysis or writing of this report. A complete listing of ADNI investigators can be found at http://adni.loni.usc.edu/wp-content/uploads/how_to_apply/ADNI_Acknowledgement_List.pdf.