- 1The Central Laboratory of the Second Affiliated Hospital, Kunming Medical University, Kunming, China
- 2Yunnan Provincial Key Laboratory of Molecular Biology for Sino Medicine, Yunnan University of Chinese Medicine, Kunming, China
- 3The Neurosurgery Department of the Second Affiliated Hospital, Kunming Medical University, Kunming, China
Neurodegenerative diseases (NDs) are characterized by progressive degeneration and necrosis of neurons, including Alzheimer’s disease (AD), Parkinson’s disease (PD), Huntington’s disease and others. There are no existing therapies that correct the progression of these diseases, and current therapies provide merely symptomatic relief. The use of polysaccharides has received significant attention due to extensive biological activities and application prospects. Previous studies suggest that the polysaccharides as a candidate participate in neuronal protection and protect against NDs. In this review, we demonstrate that various polysaccharides mediate NDs, and share several common mechanisms characterized by autophagy, apoptosis, neuroinflammation, oxidative stress, mitochondrial dysfunction in PD and AD. Furthermore, this review reveals potential role of polysaccharides in vitro and in vivo models of NDs, and highlights the contributions of polysaccharides and prospects of their mechanism studies for the treatment of NDs. Finally, we suggest some remaining questions for the field and areas for new development.
Introduction
Neurodegenerative diseases (NDs) are a series of the central nervous system (CNS) disorders that cause a slowly progressive loss of function of specific neuron populations and their connections including sporadic and hereditary forms (Reith, 2018). Most evidences suggest that approximately 25% of Alzheimer’s disease (AD) is familial and 75% is non-familial (Bird, 2018). Approximately 5–10% of Parkinson’s disease (PD) is inherited in an autosomal dominant, autosomal recessive, or even X-linked pattern of inheritance (Lesage and Brice, 2009). Moreover, the incidence of genetically confirmed Huntington’s disease (HD) in symptomatic individuals with no known family history of HD may be as high as 8% of all individuals with HD (Margolis and Ross, 2003). A number of NDs have an underlying genetic cause, with a 50% recurrence risk when inheritance is autosomal dominant (Roberts et al., 2020). The major pathological hallmarks of NDs are the accumulation and aggregation of proteins in specific neurons aggregation in the CNS. The prevalence of NDs is expected to rise with the increasing life expectancy. The complex and diverse pathological features, unclear molecular mechanisms, limited clinical examination options, difficulties in early diagnosis, and lack of specificity in treatment have introduced substantial social and economic burdens (Chen-Plotkin, 2014; Kwon et al., 2016; Marsh, 2019; Muddapu et al., 2020). There are various theories on the pathogenesis of AD, the dominant theories include the amyloid beta (Aβ) toxicity hypothesis, the microtubule-associated protein – tau protein functional abnormality hypothesis (Morris et al., 2014), the vascular gene hypothesis (De La Torre, 2010), and the gene mutation hypothesis. AD patients are characterized by accumulation of Aβ into senile plaques and hyperphosphorylated tau into neurofibrillary tangles (Spires-Jones and Hyman, 2014). AD impairs memory and cognitive judgment and is often accompanied by mood swings, disorientation and eventually delirium (Cass, 2017). Despite increases in medication dosage, the efficacy of pharmacological treatments still reduces with disease progresses. The current drugs for AD treatment include neurotransmitter agents such as cholinergic inhibitors (including tacrine, donepezil, rivastigmine, and galantamine; Sharma, 2019), drugs directed at β1 amyloid (E2069, MK-8931; Blume et al., 2018), antioxidant drugs (including monoamine oxidase inhibitors and melatonin; Cardinali et al., 2014; Ostadkarampour and Putnins, 2021), and calcium channel blockers (including nilvadipine, nimodipine, and flunarizine; Nimmrich and Eckert, 2013). Moreover, PD is a severe neurodegenerative disorder that affects around 2–3% of the population over 65 years old (Poewe et al., 2017). PD is characterized by the loss of dopaminergic (DA) neurons in the pars compacta of the substantia nigra and by accumulation of misfolded α-synuclein (α-syn; Balestrino and Schapira, 2020); its cardinal motor symptoms are bradykinesia, rigidity, postural instability, and tremor (Dickson, 2018). Despite significant progress made over the past several decades, PD is still an incurable disorder. The currently available therapeutic approaches focus on stimulation of DA signaling, such as levodopa (L-DOPA), DOPA decarboxylase inhibitors (Bandopadhyay et al., 2022), catechol-O-methyltransferase inhibitors (Salamon et al., 2022), dopamine agonists (Jenner, 2002), and inhibitors of the enzyme monoamine oxidase type B (Nagatsu and Sawada, 2006). Of these, L-DOPA remains the single most effective therapeutic agent for PD patients (Ngwuluka et al., 2010). HD is characterized by a general shrinkage of the brain and degeneration of the striatum (caudate nucleus and putamen) due to the mutation of Huntingtin (HTT) gene (Jimenez-Sanchez et al., 2017); The symptoms of HD encompasses psychiatric conditions, cognitive defects, motor impairment (e.g., chorea; Huang et al., 2016). Tetrabenazine and Deutetrabenazine, both approved by the Food and Drug Administration (FDA; Potkin and Potkin, 2018), are inhibitors of the vesicular monoamine transporter type 2, and functions through depletion of dopamine in the presynaptic terminals to improve chorea (Dean and Sung, 2018; Kumar et al., 2020), The different drug therapies available for the treatment of NDs are shown in (Table 1).
The currently available treatments for NDs are primarily focused on symptom management and no cure is yet available for this devastating disorder, the search for new and effective NDs therapies remains a priority. Herein, this review provides a summary on the pathogenesis and treatment of NDs, and summarizes the application and limitations of different forms of polysaccharides in the most common NDs.
Polysaccharides
Polysaccharides are naturally and synthetically active macromolecular substances formed by more than ten monosaccharides connected by glycosidic bonds. Natural polysaccharides are widely present in plants, animals, algae, and microorganisms. The polysaccharides are regarded as potential useful agent for the prevention of neuronal damage, and have received considerable attention for wide-ranging bioactivity (Dhahri et al., 2022). Polysaccharides have other beneficial properties including biodegradability, high stability and low toxicity, solubility in water, higher degrees of swelling capability, etc. (Mozammil Hasnain et al., 2019). Moreover, polysaccharide nanoparticles improve the bioavailability and bioactivity of functional ingredients, such as Ganoderma lucidum, Momordica charantia polysaccharides (Qin et al., 2018) and chitosan (Xue et al., 2020). The polysaccharides mainly include cellulose, chitin and other polysaccharides, and are classified into two major types: indigestible and digestible. The digestible polysaccharides are hydrolyzed to sugar subunits in the stomach, absorbed by the small intestine. Among indigestible polysaccharides, non-fermentable polysaccharides are unable to hydrolyze in the stomach and the small intestine, go through the large intestine, and are finally excreted out in the forms of waste or feces. Only the fermentable and indigestible polysaccharides are metabolized to produce various metabolites by the host intestinal microbiota, such as short chain fatty acids (SCFAs); then these metabolites modulate the host metabolism and pathophysiology process (Ahmadi et al., 2017). The polysaccharides have been widely concerned due to their immunomodulatory function. The polysaccharides bind to polysaccharides receptors, including Toll-like receptor family (TLR), C-type lectin receptor family, complement receptors (CR3), scavenger receptor (SR), mannose receptor family (MR) and so on; these receptors are bound to activate intracellular signaling pathways that generate immune response, such as effects on macrophages, T-lymphocytes, B-lymphocytes, dendritic cells, red blood cells and natural killer cells, affect complement system, and regulate cytokines secretion (Jiang et al., 2010; Zhao et al., 2020).
Recently, polysaccharides have become more prominent as energy sources and supporting tissue structures. The polysaccharides possess many several physiological functions and biological activities, including immune regulation (Lin et al., 2021), anti-tumor (Sha et al., 2022), anti-radiation protection (Li X. et al., 2021), anti-diabetic (Wang P.-C. et al., 2016), anti-virus (Zhang et al., 2022), anti-bacteria (Wang et al., 2021), anti-fatigue functions (Shen et al., 2021), anti-aging (Zhu et al., 2020), and regulation of ubiquitination (Yan et al., 2022), iron metabolism (Ren et al., 2021), intestinal flora (Cai et al., 2021), estrogen (Hwang et al., 2020), and autophagy (Wu et al., 2021b) and so on. Polysaccharides also reduce Aβ deposition (Li G. et al., 2021), inhibit neurotoxicity (Huang et al., 2021), reduce oxidative stress (Liu et al., 2020), exert anti-inflammatory actions (Wen et al., 2022), and inhibit neuronal apoptosis (Guo et al., 2016). These functions and mechanisms of polysaccharides suggest that it is possible for prevention and treatment targets in NDs. In addition, polysaccharides also have some negative effects, such as, lipopolysaccharide (LPS) and zymosan. Zymosan represents an acute inflammation model widely used for the quantification of neutrophils and inflammation-related soluble factors (Cash et al., 2009). LPS is an endotoxin in the outer membrane of most gram-negative bacteria, and is a direct glial-mediated inflammatory stimulus (Kim et al., 2019). LPS is used to model neuroinflammation associated with NDs via targeting TLR4 that is primarily expressed on microglial in CNS to produce proinflammatory cytokines (Page et al., 2022). LPS also stimulates glial cells, leads to neuroinflammation for modeling inflammation-mediated DA neurodegeneration in PD animals (Dutta et al., 2008). Nevertheless, low-dose LPS treatment can awake the peripheral immune system, finally delay the further progression of HD (Lee et al., 2018).
In dextran sodium sulfate-induced ulcerative colitis mice, Ficus carica polysaccharide changed the abundance of gut microbiota, suppressed the infiltration of inflammatory cell and cytokine formation to prevent the disease development (Zou et al., 2020). Another study demonstrated that Lycium barbarum polysaccharide reduced myocardial damage by regulating the intestinal microbiome and fecal metabolome (Zhang et al., 2020b). Bacteroides fragilis is an important member of the human gut microbiome, which regulated specific capsular polysaccharides to attract IgA binding and deploy specific capsules for immune attraction, potentially enabling stable mucosal colonization and clear pathogens (Donaldson et al., 2018). In nervous system, these receptors, including TLR2, TLR3, TLR4, MR, and SR, are also widely expressed and participate in CNS injury and NDs (Burudi and Régnier-Vigouroux, 2001; Husemann and Silverstein, 2001; Marsh and Stenzel-Poore, 2008; Perez-Pardo et al., 2019; Zhou et al., 2019). If the polysaccharides cross the blood-brain barrier (BBB), they as extrinsic ligands bind to the above receptors to mediate the intracellular signaling in NDs. Due to the poor BBB permeability of most polysaccharides, they may regulate intestinal flora and related metabolic products to exert neuroprotective effects.
In a mouse PD model, Astragalus polysaccharides decreased the bax/bcl2 ratio, reversed mitochondrial structural damage and attenuated motor dysfunction (Liu H. et al., 2018). G. lucidum polysaccharides regulated expression of apoptosis-associated proteins, inhibited oxidative stress-induced neuronal apoptosis, and had significant neuroprotective effects in cerebellar granule cells (Sun et al., 2017). Wang et al. reported that sulfated hetero-polysaccharides extracted from Saccharina japonica may had a “therapeutic anti-apoptosis” utility for PD by enhancing the phosphorylation of PI3K/Akt signaling pathway and impairing caspase-3 levels in H2O2-induced SH-SY5Y cells (Wang et al., 2017). Angelica polysaccharide significantly alleviated LPS-induced PC-12 cell viability inhibition, apoptosis and expression of inflammatory cytokines, inactivated NF-κB pathway by down-regulating miR-223 (Li R. et al., 2018). Astragalus polysaccharide activated the PI3K/AKT/mTOR to increase autophagy, finally elevated cell viability in PD cell model (Tan et al., 2020). Currently, it has reported that the common mechanisms of polysaccharides against PD and AD involve in neuroinflammation, oxidative stress, autophagy, apoptosis, mitochondrial dysfunction. And, the mechanisms of HD primarily involved in oxidative stress and neurotoxicity. Figure 1 shows the neuroprotective mechanisms of polysaccharides involved in NDs.
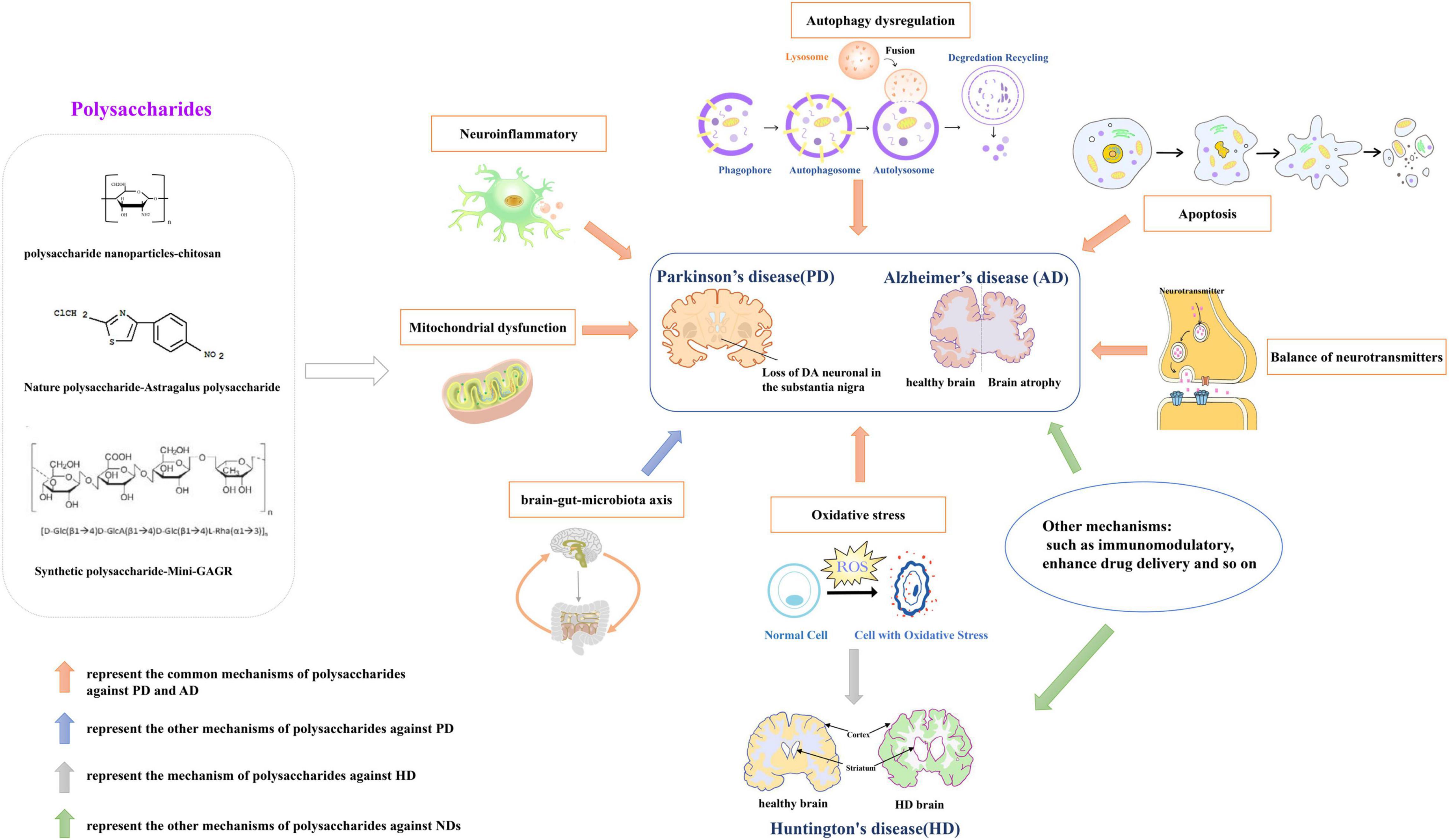
Figure 1. The neuroprotective mechanisms of polysaccharides involved in neurodegenerative diseases (NDs). Drawn by Chen Shen, The Central laboratory of the Second Affiliated Hospital, Kunming Medical University.
The Role of Polysaccharides in Neurodegenerative Diseases
Parkinson’s Disease
Parkinson’s disease is the second most frequent neurodegenerative disorder of aging, affecting 7–10 million people worldwide, is diagnosed clinically based on typical motor symptoms, including bradykinesia, rigidity, abnormal posture, and resting tremor. Exposure to pesticides or herbicides, carbon monoxide, organic solvents, carbon disulfide, well water, rural environment, plant-derived toxins, and infection are all considered as causes of PD. The pathological feature of PD is the loss of DA neurons, and abnormal accumulation of α-syn. α-syn is a small (140 aa) protein generally enriched in the presynaptic compartment that regulate vesicle dynamics and trafficking, and neurotransmitter release (Emamzadeh, 2016).
Although the etiology of PD remains unclear, the death of DA neurons during PD progress is revealed to be associated with the abnormal aggregation of α-syn (Luk et al., 2012), mitochondrial dysfunction (Mandemakers et al., 2007), overactivated oxidative stress (Ren and Butterfield, 2021), apoptosis (Liu J. et al., 2018), or autophagy (Hou X. et al., 2020), microglia response caused by neuroinflammation (Tiwari and Pal, 2017), microbiota-gut-brain axis (Menozzi et al., 2021), autosomal dominant and recessive sexual heredity-related genetic changes (Filatova et al., 2014), and other factors are closely related. PD is a significant disease that affects human health and life, and brings substantial social and economic burdens to families and society. Since no viable treatment is available for PD to cease or reverse the disease progression, there is an urgent need for novel therapeutic approaches.
Oxidative stress is caused by increasing reactive oxygen species (ROS) product and weakening antioxidant capacity, damaging lipids, proteins, and DNA. ROS in the physiological state are important redox messengers that regulate various signaling pathways and play a crucial role in regulating cellular metabolism, post-transcriptional modification of proteins, and antioxidant defense mechanisms. However, excessive ROS damage lipids, proteins and DNA, and inhibit normal cell function. Oxidative stress is a state of stress damage caused by the imbalance of oxidation and anti-oxidation in the cell (Dröge, 2002). For example, in 1-methyl-4-phenyl-1,2,3,6-tetrahydropyridine (MPTP)-induced mouse PD models, M. charantia polysaccharides inhibited oxidative stress products in the brain, thereby increased DA levels, alleviated the impairment of coordination and motor ability by regulating the TLR4/MyD88/NF-κB pathway (Guo et al., 2021). A blue-green alga spirulina platensis polysaccharide attenuated the reduction in tyrosine hydroxylase and the dopamine transporter expression (DA-specific markers) via increasing in the activities of superoxide dismutase (SOD), and glutathione peroxidase (GSH-Px) in same mouse PD models (Zhang et al., 2015), SOD and GSH-px are important endogenous antioxidants. In 6-hydroxydopamine (6-OHDA) – induced mouse PD model, Antrodia camphorata polysaccharide inhibited the activation of ROS via increasing the activity and expression of antioxidant enzymes, ultimately reduced DA neuronal damage in the substantia nigra, improves motor performance (Han et al., 2020). Many accumulated evidences show that a prominent feature in many NDs is excessive ROS, it would attack lipid membranes, proteins and DNA (Wu et al., 2006), and eventually contribute to the injury and death of neuronal cells. In H2O2 – induced PC12 cells, wild desert plant – drived Cynomorium songaricum Rupr polysaccharide maintained the antioxidant system, protected the cells against oxidative damage (Wang F. et al., 2016). A selenium polysaccharide from Platycodon grandiflorum attenuated intracellular ROS formation to inhibit the decrease of cell viability against PC12 cells injury (Sheng et al., 2017). Radix Ophiopogonis is the one of most widely used Chinese herb in Traditional Chinese Medicine (Wang et al., 2015), this polysaccharide protected PC12 cells through suppressing the increase of the intracellular oxidative stress and endoplasmic reticulum stress (Liu and Li, 2018).
In neuronal cells, mitochondria can be found to enhance at areas of increased energy demand, to alter their motility in axons and at synapses in order to maintain energy homeostasis that is essential for synaptic functions (Sheng, 2017). Mitochondria-derived ATP production provides most of the axonal energy, and damaged mitochondria fail to produce ATP. Biological energy deficits and chronic oxidative stress trigger axonal pathology and synaptic dysfunction, thus contributing to pathogenesis of NDs (Theocharopoulou, 2020). Research showed that sulfated chitosan suppressed rotenone – induced – mitochondrial dysfunction, nuclear condensation, and DNA fragmentation in human neuroblastoma SH-SY5Y cell line (Manigandan et al., 2019). Likewise, in rotenone – induced PD animal model, fucoidan extracted from Laminaria japonica enhanced mitochondrial respiratory function through the PGC-1α/NRF2 pathway, alleviated DA degeneration and motor impairments (Zhang L. et al., 2018).
Neuroinflammatory responses are also major pathogenic factors in neurodegeneration, and microglia is key participant in neuroinflammatory. Microglia activation and increased inflammatory cytokines have been implicated in the cognitive decline associated with NDs. Microglia activation produces a variety of pro-inflammatory mediators and pro-inflammatory cytokines, plays a crucial role in various NDs (Luo et al., 2013). These cytokines enter the brain, stimulate microglia to trigger neuroinflammatory responses, induce neuronal degeneration and death. Traditional Chinese medicine – Schisandra chinensis protected against DA neurodegeneration by suppressing neuroinflammation via the BDNF/Nrf2/NF-κB pathway in 6-OHDA-induced PD mice (Yan et al., 2021). And G. lucidum prevented the production of microglia-derived proinflammatory and cytotoxic factors in MPP+-treated MES 23.5 cell membranes (Zhang et al., 2011).
Apoptosis occurs normally during development and aging and as a homeostatic mechanism to maintain cell populations, including normal cell turnover, proper development and functioning of the immune system, hormone-dependent atrophy, embryonic development and chemical-induced cell death (Elmore, 2007), and apoptosis and caspase-mediated cell death are important mediators of neuronal death in NDs. Traditional herbal medicine has been used for centuries in China to treat PD and remains in use, suggesting that medicinal herbs may be a good source of drug candidates for the treatment of PD (Chen et al., 2007). Gynostemma pentaphyllum Makino is a well-known edible and medicinal plant in Asia (Su et al., 2021), its extract polysaccharides decreased Bax/Bcl2 ratio, attenuated the activation of caspase-3/9, and improved PC12 cell viability of MPP+ – induced PD cell model (Deng and Yang, 2014). Lycium barbarum polysaccharides reduced apoptosis to reverse the decreased viability of PC12 cells in a vitro PD model (Gao et al., 2014).
Autophagy is a cellular basic metabolic process that degrades the aggregated or misfolded proteins and abnormal organelles in cells. The abnormal regulation of neuronal autophagy is accompanied by the accumulation and deposition of irregular proteins, leading to changes in neuron homeostasis and neurodegeneration. Autophagy is a unique mechanism for cells to protect themselves. When autophagy is weakened, abnormal protein aggregation will accelerate the onset of PD; and when autophagy is too strong, it will lead to the autophagy death of nerve cells. Studies show that PTEN-induced putative kinase 1 (PINK1) and E3 ubiquitin protein ligase (Parkin) participate in mitochondrial autophagy, mutations in PINK1 and Parkin genes result in defective mitochondria and trigger early onset PD (Meka et al., 2015). Ren et al. has reported that G. lucidum extract attenuated autophagy, and declined in the expression of PINK1 and Parkin, improved behavioral performance in PD model (Ren et al., 2019).
Polymannuronic acid (PM) is a water-soluble homopolysaccharide and can be easily separated via pH fractionation from alginate hydrolyzate (Li et al., 2013), it increased gut microbial diversity and fecal SCFAs production, improved the integrity of the intestinal barrier and BBB, prevented DA neuronal loss and improved motor functions in MPTP-induced PD mice (Dong et al., 2020).
To date, a number of literatures have been reported for the possible mechanism of traditional Chinese medicine in treating PD (Rai et al., 2021). As shown in Table 2, current research in PD show that polysaccharides play neuroprotective roles via inhibiting cell apoptosis, neuroinflammatory responses and oxidative stress, reversing mitochondrial dysfunction, regulating microbiome and its metabolic product, improving autophagy and other mechanisms.
The Role of Polysaccharides in Alzheimer’s Disease
Alzheimer’s disease is a common degenerative disease of the nervous system with insidious onset and progressive development worldwide. The AD progression develops from initial short-term memory loss to behavioral problems, gradual loss of physical function, and finally death (Tarawneh and Holtzman, 2012). It is believed that the pathogenesis of AD includes the excessive aggregation of Aβ and neurofibrillary tangles formed by hyperphosphorylation of Tau protein (Zhang et al., 2021), release of pro-inflammatory cytokines (Bellucci et al., 2004), mitochondrial dysfunction (Kukreja et al., 2014), oxidative stress (Guix et al., 2012; Wahlster et al., 2013), apoptosis (Zhu et al., 2006), autophagy (Plaza-Zabala et al., 2017), and so on.
The cause of AD remains unclear, and all current medications only relieve the symptoms or delay its development. Aβ is produced and released from the axon terminals of neurons already afflicted with tau pathology (Braak and Del Tredici, 2013). FDA approved AD drugs such as acetylcholinesterase (AChE) inhibitors and N-methyl-D-aspartate receptor antagonists only alleviate symptoms in about half of the patients for approximately 6–12 months (Winslow et al., 2011).
In amyloid precursor protein/presenin 1 (APP/PS1), a double transgenic mouse model of AD, Codonopsis pilosula polysaccharide decreased the expression of Aβ42 and Aβ40 in the hippocampus and alleviated cognitive impairment through the restoration of synaptic plasticity (Wan et al., 2020). Maitake polysaccharide, an edible/medicinal mushroom, activated microglia and astrocytes, promoted the recruitment of microglia to Aβ plaques, enhanced Aβ phagocytosis to reduce Aβ load, increased the number of surviving neurons and maintained the histomorphology of the hippocampus, finally improved learning and memory impairment in APP/PS1 mice (Bai et al., 2019). Furthermore, polysaccharides also have been found to have the same effect in cellular models. Lycium barbarum polysaccharide reduced the expression of Aβ42/Aβ40 against β-amyloid peptide neurotoxicity in N2a/APP695 cells (mouse neuroblastoma N2a cells stably expressing human APP695; Wu et al., 2021a). A novel pectin polysaccharide from Polygala tenuifolia enhanced the expression of insulin-degradation enzyme and Neprilysin (NEP) to attenuate Aβ42 production and inhibit Aβ42 aggregation (Zeng et al., 2020). Moreover, polysaccharide obtained from Coptis chinensis Franch could inhibit the deposition of Aβ via the reduction of Aβ-induced toxicity and delaying of the aging in a transgenic Caenorhabditis elegans (C. elegans) model of AD (Li Y. et al., 2018).
The presence of tau pathology and its correlation with cognitive deficits encouraged the statement of the “tau hypothesis” of AD (Kametani and Hasegawa, 2018). Tau is a microtubule-related protein that aggregates tubulin into microtubules, maintains complex neuronal cell microstructure promoting neuron maturation and regulating synaptic function (Dawson et al., 2001; Castellani and Perry, 2019), changes in synaptic distribution and interruption of interaction with synaptic proteins, damage neuron function and even lead to AD. Previous studies showed that polysaccharides from various plants alleviate cognitive impairment and pathological alterations in AD animal models (Ho et al., 2010; Zhang J. et al., 2016). In an adeno-associated virus serotype 2 (AAV2)-induced expression of human full-length Tau (hTau) in C57/BL6 mice to mimic AD tau pathology, C. pilosula polysaccharide significantly increased protein phosphatase-2A (PP2A) activity to attenuate tau phosphorylation in the hippocampus of mice (Zhang Q. et al., 2018). Polysaccharides, extracted from an edible fungus Pleurotus ostreatus, decreased Aβ accumulation and tau phosphorylation by increasing the expression of PP2A and glycogen synthase kinase 3beta (GSK3), alleviated cognitive impairment in AD rats (Zhang Y. et al., 2016). An intranasally applied polysaccharide Mini-GAGR can cross BBB to increase antioxidant enzymes in the hippocampus and cortex, decrease phosphorylated-tau (p-tau) and improve memory in AD mice (Murphy et al., 2018). Similarly, another 4.7 KD polysaccharide Midi-GAGR also penetrated the BBB to reduce free reactive radicals and microglia cells, increased neurite outgrowth and phosphorylated cAMP responsive element binding protein, decreased hyperphosphorylated tau for neuroprotection in the transgenic AD mouse model (Makani et al., 2016).
Acetylcholine (Ach) is a signal transmitter of cholinergic neurons, its metabolic processes influence learning and memory in AD brain (Ferreira-Vieira et al., 2016). A commonly used traditional Chinese herb Angelica sinensis is widely used for nourishing the blood. Angelica polysaccharide regulated the balance of neurotransmitters by decreasing AChE level and increasing Ach and acetyltransferase (ChAT), eventually meliorated spatial learning and memory deficiency in AD rats (Du et al., 2020). Porphyran, from red algae Pyropia haitanensis, increased ChAT activity and decreased AChE activity in the cortical and hippocampal, significantly ameliorated the learning and memory impairment induced by Aβ1-40 of AD mice (Zhang et al., 2020c). The changes of chAT and AchE activity are considered to be important indicators to indirectly reflect the cholinergic biochemical changes in AD (Mantzavinos and Alexiou, 2017).
In Aβ-induced AD rats, marine brown seaweed fucoidan, a complex sulfated polysaccharide, increased of Bcl2/Bax ratio and decreased of caspase-3, inhibited cell apoptosis followed by improving the learning and memory abilities (Gao et al., 2012). Non-saponin fraction with rich polysaccharides from ginseng ameliorated mitochondrial deficit in Aβ-treated HT22 cells and enhanced and restored the cognitive function of healthy and AD mice (Shin et al., 2021). Furthermore, study has shown that many natural polysaccharides are also called immune polysaccharides due to their immunological activity. Polysaccharides from Schisandra Chinensis Fructus significantly reduced the deposition of Aβ, improved the cognition and histopathological changes via downregulating the expression of pro-inflammatory cytokines such as IL-1β, IL-6, and TNF-α, and the activation of glial cells in the hippocampus of AD mice (Xu et al., 2019). Moreover, G. lucidum polysaccharides via oral administration promoted neural progenitor cell proliferation to enhance neurogenesis and alleviated cognitive deficits in transgenic AD mice, and also improved locomotor functions and prolonged the life span of Drosophila Aβ42-AD model (Huang et al., 2017).
As reported, the promotion of autophagy initiation and autolysosome formation can reduce the aggregate-prone proteins aggregation and its neurotoxicity in AD model (Nixon, 2013). Two trehalose analogs including lactulose and melibiose increase autophagy by upregulating the LC3II/LC3I and downregulating the p62, decrease neuroinflammation, eventually attenuated the short-term memory and the learning retrieval in AD mice (Lee et al., 2021). Moreover, In AD-like symptom C57BL/6J mice, polysaccharides from Taxus chinensis var. mairei Cheng et L.K.Fu (Taxaceae) inhibited oxidative stress, restored the impaired learning and cognitive function via regulating the expression of NF-E2-related factor 2 (Nrf2) which plays an important role in cell defense against oxidative stress in CNS (Zhang et al., 2020a). A fungal species Inonotus obliquus polysaccharide also decreased oxidative stress via Nrf2 signaling, improved the pathological behaviors related memory, and cognition in APP/PS1 mice (Han et al., 2019).
In conclusion, AD seriously threatens aged people’s health. As shown in Table 3, we speculate that polysaccharides can reduce oxidative stress, apoptosis and neuroinflammation, regulate the balance of neurotransmitters, increase autophagy, ultimately decrease Aβ peptide formation and tau phosphorylation, alleviate cognitive impairment in AD models. Consequently, it is plausible to recommend polysaccharide as one of the promising tools in the development of drug therapy for AD.
Huntington’s Disease
Huntington’s disease is a single-gene autosomal dominant neurodegenerative disorder with the disease-causing gene IT-15 (the HTT gene). The HD gene has been mapped in 4p16.3, which is abnormally amplified in CAG trinucleotide repeats in HD. When the number of CAG copies is abnormally increased due to gene mutation, the polyglutamine (polyQ) chain at the amino-terminal of the mutant Htt (mHtt) protein is prolonged and abnormally folded. The β lamellae structure is formed to cause the loss of normal function and toxic effect of mHtt protein (Southwell and Patterson, 2011). HD usually presents in midlife with chorea, dementia, and weight loss, with death typically occurring 15–20 years after symptom onset (Walker, 2007), the dance-like movements that are a primary clinical symptom of HD. Nonetheless, the therapies currently available to HD patients only moderate symptom relief and do not affect disease progression.
A prominent pathological feature of HD is the accumulation of mHtt in neurons, and mHtt plays a cytotoxic role by affecting transcription, mitochondrial function, synaptic transmission, and axon transport (Ramakrishnan and Gupta, 2021). Compared with ordinary Htt, mHtt has a longer polyQ (>36) extension at the N-terminal (Ross, 2002). mHtt undergoes proteolysis, misfolding, accumulation, self-aggregation, and eventually forms inclusion bodies (Cortes and La Spada, 2014). mHtt engages in various aberrant interactions that lead to the pathological gain of toxic functions and loss of normal functions. The study found that reduced AKT phosphorylation and inhibited AKT activity are involved in mHtt-induced cell death. Lycium barbarum polysaccharide alleviated the cytotoxicity of mHtt by activating AKT and reduceing mHtt levels in HD-transgenic mice (Fang et al., 2016). Astragalus membranaceus polysaccharide reduced polyQ aggregation and alleviated the associated neurotoxicity in C. elegans (Zhang et al., 2012). Peganum harmala L. polysaccharide inhibited polyQ aggregation through proteasome-mediated protein degradation, alleviated the neurotoxicity of C. elegans (Guo et al., 2020).
Soluble and aggregative mHtt has cytotoxicity, manifests primarily as excessive intracellular oxidative stress response leading to cell apoptosis in the mitochondrial pathway (Reijonen et al., 2008; Ayala-Peña, 2013), increases expression of apoptosis-related genes (Bae et al., 2005), and continuous degeneration of neurons caused by cell cycle re-entry (Pelegrí et al., 2008). Polysaccharide is capable of inhibiting behavioral dysfunction mediated by reducing polyQ aggregation. The study found that a kind of traditional Chinese medicine – epimedium is a genus of plants in the family Berberidaceae, and its polysaccharide enhanced antioxidant enzyme activities, decreased lipid peroxidation product and oxidative stress, increased the survival rates, attenuated behavioral dysfunction in polyQ transgenic C. elegans HD models (Xiang et al., 2017). In the similar HD models, the polysaccharide from a medicinal mushroom Dictyophora indusiata was capable of reducing ROS levels and alleviating chemosensory behavior deficits (Zhang J. et al., 2016). 3-nitropropionic acid (3-NP) induces a selective striatal pathology in HD, and has been widely used as an animal model of HD. Ginseng saponins decreased intracellular Ca2+ and cytotoxicity of striatal neurons, significantly improved behavioral impairment, and extended the survival of 3-NP-induced HD rats (Kim et al., 2005). Furthermore, polysaccharides and their effects on HD are shown in Table 4.
Discussion
Previous studies suggest that polysaccharides are employed to protect against NDs. Herein, this review presents multiform polysaccharides protect against NDs via mediating various mechanisms. Despite many signaling pathways involved in, the common mechanisms of polysaccharides mainly include neuroinflammation, oxidative stress, autophagy, apoptosis and mitochondrial dysfunction in PD and AD for neuroprotective effects. And, HD related studies are still rare. In the above literatures, a majority of polysaccharide in NDs have been studied in vitro. Most polysaccharides have a poor BBB permeability besides chitosan nanoparticles and LPS. Therefore, it is rarely reported that the polysaccharides directly activate polysaccharides receptors in the CNS of NDs. How do the polysaccharides work in the CNS? Up to now, only polysaccharide receptor TLR-4 has been reported to be bind with LPS to increase accumulation of Aβ in AD. Based on above studies and the metabolism of polysaccharides, the polysaccharides may directly penetrate BBB to act on the polysaccharide receptors or mediate intestinal microorganism and metabolites to activate downstream signaling for neuroprotective effect in NDs. Whereas, the related studies are still limited.
Most NDs patients develop gastrointestinal motility abnormalities and constipation, hence intestinal flora might be closely related to the development of NDs. The imbalance also affects the intestinal mucosal barrier, triggers intestinal and peripheral neuroinflammatory responses. These phenomena may lead to neuroinflammation or neurodegeneration in CNS (Pellegrini et al., 2018). Polysaccharide molecules are closely related to the regulation of intestinal flora, the role of the microbiota provides new therapeutic targets for NDs (Sun et al., 2020). Nerves, immunity, and the endocrine system could alter the microbiota-gut-brain axis. The intestine, the brain and immune system dysfunctions promotes the development and progression of NDs. The intestinal microbiota and its primary metabolites SCFAs affect brain activity and behavior via endocrine, vagus nerve, immune pathways and other humoral pathways (Shaik et al., 2020). SCFAs promote the development and growth of brain microglia, play a central role in brain development and the maintenance of CNS homeostasis (Erny et al., 2015). Natural polysaccharides have received increasing attention and have become popular dietary nutrients because of their various biological functions. Some natural polysaccharides are favorable for the proliferation of SCFA-producing bacteria, the presence of which improves the intestinal microenvironment. Moreover, natural polysaccharides also suppress excessive inflammatory responses by improving the intestinal microbiota composition, promoting SCFAs production, strengthening intestinal barrier function and reducing pro-inflammatory mediators (Tang et al., 2019). A plant polysaccharide from Cistanche deserticola improves cognitive function in mice in a D-galactose induced aging model by restoring homeostasis of the gut-microbiota-brain axis (Gao et al., 2021). Therefore, polysaccharides may mediate intestinal flora and related metabolites against NDs.
The natural polysaccharides contain polysaccharides and their derivatives (such as nanoparticles). Using polysaccharides nanoparticles for drug delivery can enhance the aqueous solubility of the drug (Kang et al., 2015). Additionally, specific polysaccharides can provide targeting mechanisms due to receptor recognition and binding (Yadav et al., 2008), mucosal adhesion and transport (Feng et al., 2015), site specific enzymatic degradation (Castelli et al., 2008). Polysaccharide-based drug delivery, specially, polysaccharide nanoparticle has been improved the uptake and specificity of drugs and the bioavailability of poorly soluble drugs. Such as, polysaccharide chitosan as the only essentially natural cationic polysaccharide is useful for chemical modification and electrostatic interactions in drug delivery systems in NDs (Sarvaiya and Agrawal, 2015; Liu et al., 2017; Raj et al., 2018). Starch is a glucose-based polysaccharide produced by many green plants to store energy, often as an excipient in pharmaceutical products, have been used to modify the properties of drug delivery systems to improve sustained release and directly to enhance drug delivery in PD (Odeniyi et al., 2018; Becker et al., 2021). An energy storage polysaccharide Inulin is found in plants of the Compositae family, and also indigestible to humans and digested by the enzymes of bacteria in the gut, allowing to be exploited for colon targeted delivery (Castelli et al., 2008). Polysaccharides represent a platform for therapeutic delivery applications, and these multiple functions are likely to be increasingly applied in the future.
There are growing evidences on the fact that distinct immune responses, involving the adaptive as well as the innate immune system, are crucially implicated in NDs (Chitnis and Weiner, 2017), and immunotherapy is one of the most studied therapeutic strategies in NDs (Ciccocioppo et al., 2020). Moreover, microglia and astrocytes protect the brain from infectious agents, while their prolonged activation causes neuroinflammation that promote neurodegeneration (Barbalace et al., 2019). Neuroinflammation significantly initiates and enhances neurodegenerative pathological change, natural anti-inflammatory compounds may be good candidates for the development of successful therapeutic strategies. Polysaccharides have significant pharmaceutical importance due to their strong anti-inflammatory and immunomodulatory properties (Hou C. et al., 2020). The polysaccharides in AD, PD, and HD share a common mechanism – oxidative stress. Growing reports have demonstrated that oxidative stress is implicated in the development and progression of many chronic diseases, and oxidative stress is a condition of imbalance between ROS formation and cellular antioxidant capacity due to enhance ROS generation or dysfunction of the antioxidant system. Many polysaccharides are reported to have potent reducing power and free radical scavenging ability in vitro, and reduce the levels of ROS and associated peroxidation products in cellular and animal models under oxidative stress. Furthermore, oxidative stress can interact with many other stresses to induce neurodegeneration, so antioxidant polysaccharides play significant role as a pharmacological potential in treatment NDs.
In addition, the polysaccharides also develop for clinical applications. Acemannan, the main bioactive polysaccharide of Aloe vera, is a neuroprotective immunomodulator and antioxidant, and improved the cognitive performances of middle-aged patients suffering from mental fatigue (Liu et al., 2019). Furthermore, chitosan as a biomaterial for the development of a flexible, thin film, laser-activated surgical adhesive termed surgical adhesive, are FDA approved and successfully used in a variety of biomedical applications and products. Therefore, chitosan is an alternative to microsurgery for peripheral nerve reconstruction (Foster and Karsten, 2012). Chitosan nerve tube can improve peripheral sensory nerve regeneration of the patients in traumatic sensory nerve lesions of the hand (Neubrech et al., 2018). So polysaccharides and their mechanisms deserve further study as potential candidates for preventing and treating NDs.
Author Contributions
YW wrote the manuscript. NZ and JY contributed to writing and review by the manuscript. RC, QW, and XC were implied in collecting and sorting literatures and references. ZY wrote a small part and offered some ideas for the first manuscript, and improved language, revised, refined the manuscript in the process of revision. All authors read and approved the final manuscript.
Funding
This work is supported by the National Natural Science Foundation Program of China (grant no. 31860274), Department of Science and Technology of Yunnan Province (grant nos. 202101AT070251, 202201AS070084, 202005AC160058, and 2019FI016).
Conflict of Interest
The authors declare that the research was conducted in the absence of any commercial or financial relationships that could be construed as a potential conflict of interest.
Publisher’s Note
All claims expressed in this article are solely those of the authors and do not necessarily represent those of their affiliated organizations, or those of the publisher, the editors and the reviewers. Any product that may be evaluated in this article, or claim that may be made by its manufacturer, is not guaranteed or endorsed by the publisher.
Acknowledgments
We sincerely thank Chen Shen (The Central laboratory of the Second Affiliated Hospital, Kunming Medical University) for drawing the graphical summary.
References
Ahmadi, S., Mainali, R., Nagpal, R., Sheikh-Zeinoddin, M., Soleimanian-Zad, S., Wang, S., et al. (2017). Dietary polysaccharides in the amelioration of gut microbiome dysbiosis and metabolic diseases. Obes. Control Ther. 4:10.15226/2374-8354/4/2/00140. doi: 10.15226/2374-8354/4/2/00140
Ayala-Peña, S. (2013). Role of oxidative DNA damage in mitochondrial dysfunction and Huntington’s disease pathogenesis. Free Radic. Biol. Med. 62, 102–110. doi: 10.1016/j.freeradbiomed.2013.04.017
Bae, B. I., Xu, H., Igarashi, S., Fujimuro, M., Agrawal, N., Taya, Y., et al. (2005). p53 mediates cellular dysfunction and behavioral abnormalities in Huntington’s disease. Neuron 47, 29–41. doi: 10.1016/j.neuron.2005.06.005
Bai, Y., Chen, L., Chen, Y., Chen, X., Dong, Y., Zheng, S., et al. (2019). A Maitake (Grifola frondosa) polysaccharide ameliorates Alzheimer’s disease-like pathology and cognitive impairments by enhancing microglial amyloid-β clearance. RSC Adv. 9, 37127–37135. doi: 10.1039/c9ra08245j
Balestrino, R., and Schapira, A. (2020). Parkinson disease. Eur. J. Neurol. 27, 27–42. doi: 10.1111/ene.14108
Bandopadhyay, R., Mishra, N., Rana, R., Kaur, G., Ghoneim, M. M., Alshehri, S., et al. (2022). Molecular mechanisms and therapeutic strategies for levodopa-induced dyskinesia in Parkinson’s disease: a perspective through preclinical and clinical evidence. Front. Pharmacol. 13:805388. doi: 10.3389/fphar.2022.805388
Barbalace, M. C., Malaguti, M., Giusti, L., Lucacchini, A., Hrelia, S., and Angeloni, C. (2019). Anti-Inflammatory activities of marine algae in neurodegenerative diseases. Int. J. Mol. Sci. 20:3061. doi: 10.3390/ijms20123061
Becker, A., Pierre Schmartz, G., Groger, L., Grammes, N., Galata, V., Philippeit, H., et al. (2021). Effects of resistant starch on symptoms, fecal markers and gut microbiota in Parkinson’s disease - the RESISTA-PD trial. Genomics Proteomics Bioinform. S1672-0229(21)00245-X. [Online ahead of print], doi: 10.1016/j.gpb.2021.08.009
Bellucci, A., Westwood, A. J., Ingram, E., Casamenti, F., Goedert, M., and Spillantini, M. G. (2004). Induction of inflammatory mediators and microglial activation in mice transgenic for mutant human P301S tau protein. Am. J. Pathol. 165, 1643–1652. doi: 10.1016/S0002-9440(10)63421-9
Bird, T. D. (2018). Alzheimer Disease Overview. GeneReviews® [Internet]. Seattle, DC: University of Washington.
Blume, T., Filser, S., Jaworska, A., Blain, J.-F., Koenig, G., Moschke, K., et al. (2018). BACE1 inhibitor MK-8931 alters formation but not stability of dendritic spines. Front. Aging Neurosci. 10:229. doi: 10.3389/fnagi.2018.00229
Braak, H., and Del Tredici, K. (2013). Amyloid-β may be released from non-junctional varicosities of axons generated from abnormal tau-containing brainstem nuclei in sporadic Alzheimer’s disease: a hypothesis. Acta Neuropathol. 126, 303–306. doi: 10.1007/s00401-013-1153-2
Burudi, E. M., and Régnier-Vigouroux, A. (2001). Regional and cellular expression of the mannose receptor in the post-natal developing mouse brain. Cell Tissue Res. 303, 307–317. doi: 10.1007/s004410000311
Cai, G., Wusiman, A., Gu, P., Mao, N., Xu, S., Zhu, T., et al. (2021). Supplementation of Alhagi honey polysaccharides contributes to the improvement of the intestinal immunity regulating the structure of intestinal flora in mice. Food Funct. 12, 9693–9707. doi: 10.1039/d1fo01860d
Cardinali, D. P., Vigo, D. E., Olivar, N., Vidal, M. F., and Brusco, L. I. (2014). Melatonin therapy in patients with Alzheimer’s disease. Antioxidants (Basel, Switzerland) 3, 245–277. doi: 10.3390/antiox3020245
Cash, J. L., White, G. E., and Greaves, D. R. (2009). Chapter 17. Zymosan-induced peritonitis as a simple experimental system for the study of inflammation. Methods Enzymol. 461, 379–396. doi: 10.1016/S0076-6879(09)05417-2
Cass, S. P. (2017). Alzheimer’s disease and exercise: a literature review. Curr. Sports Med. Rep. 16, 19–22.
Castellani, R. J., and Perry, G. (2019). Tau biology, tauopathy, traumatic brain injury, and diagnostic challenges. J. Alzheimers Dis. 67, 447–467. doi: 10.3233/JAD-180721
Castelli, F., Sarpietro, M. G., Micieli, D., Ottimo, S., Pitarresi, G., Tripodo, G., et al. (2008). Differential scanning calorimetry study on drug release from an inulin-based hydrogel and its interaction with a biomembrane model: pH and loading effect. Eur. J. Pharm. Sci. 35, 76–85. doi: 10.1016/j.ejps.2008.06.005
Chen, L. W., Wang, Y. Q., Wei, L. C., Shi, M., and Chan, Y. S. (2007). Chinese herbs and herbal extracts for neuroprotection of dopaminergic neurons and potential therapeutic treatment of Parkinson’s disease. CNS Neurol. Disord. Drug Targets 6, 273–281. doi: 10.2174/187152707781387288
Chen-Plotkin, A. S. (2014). Unbiased approaches to biomarker discovery in neurodegenerative diseases. Neuron 84, 594–607. doi: 10.1016/j.neuron.2014.10.031
Chitnis, T., and Weiner, H. L. (2017). CNS inflammation and neurodegeneration. J. Clin. Invest. 127, 3577–3587. doi: 10.1172/JCI90609
Ciccocioppo, F., Bologna, G., Ercolino, E., Pierdomenico, L., Simeone, P., Lanuti, P., et al. (2020). Neurodegenerative diseases as proteinopathies-driven immune disorders. Neural Regen. Res. 15, 850–856. doi: 10.4103/1673-5374.268971
Cortes, C. J., and La Spada, A. R. (2014). The many faces of autophagy dysfunction in Huntington’s disease: from mechanism to therapy. Drug Discov. Today 19, 963–971. doi: 10.1016/j.drudis.2014.02.014
Dawson, H. N., Ferreira, A., Eyster, M. V., Ghoshal, N., Binder, L. I., and Vitek, M. P. (2001). Inhibition of neuronal maturation in primary hippocampal neurons from tau deficient mice. J. Cell Sci. 114(Pt 6), 1179–1187. doi: 10.1242/jcs.114.6.1179
De La Torre, J. C. (2010). The vascular hypothesis of Alzheimer’s disease: bench to bedside and beyond. Neurodegener. Dis. 7, 116–121. doi: 10.1159/000285520
Dean, M., and Sung, V. W. (2018). Review of deutetrabenazine: a novel treatment for chorea associated with Huntington’s disease. Drug Des. Devel. Ther. 12, 313–319. doi: 10.2147/DDDT.S138828
Deng, Q., and Yang, X. (2014). Protective effects of Gynostemma pentaphyllum polysaccharides on PC12 cells impaired by MPP(+). Int. J. Biol. Macromol. 69, 171–175. doi: 10.1016/j.ijbiomac.2014.05.049
Dhahri, M., Alghrably, M., Mohammed, H. A., Badshah, S. L., Noreen, N., Mouffouk, F., et al. (2022). Natural polysaccharides as preventive and therapeutic horizon for neurodegenerative diseases. Pharmaceutics 14:1. doi: 10.3390/pharmaceutics14010001
Dickson, D. W. (2018). Neuropathology of Parkinson disease. Parkinsonism Relat. Disord. 46(Suppl. 1) S30–S33. doi: 10.1016/j.parkreldis.2017.07.033
Donaldson, G. P., Ladinsky, M. S., Yu, K. B., Sanders, J. G., Yoo, B. B., Chou, W. C., et al. (2018). Gut microbiota utilize immunoglobulin A for mucosal colonization. Science 360, 795–800. doi: 10.1126/science.aaq0926
Dong, X. L., Wang, X., Liu, F., Liu, X., Du, Z. R., Li, R. W., et al. (2020). Polymannuronic acid prevents dopaminergic neuronal loss via brain-gut-microbiota axis in Parkinson’s disease model. Int. J. Biol. Macromol. 164, 994–1005. doi: 10.1016/j.ijbiomac.2020.07.180
Dröge, W. (2002). Free radicals in the physiological control of cell function. Physiol. Rev. 82, 47–95. doi: 10.1152/physrev.00018.2001
Du, Q., Zhu, X., and Si, J. (2020). Angelica polysaccharide ameliorates memory impairment in Alzheimer’s disease rat through activating BDNF/TrkB/CREB pathway. Exp. Biol. Med. (Maywood) 245, 1–10. doi: 10.1177/1535370219894558
Dutta, G., Zhang, P., and Liu, B. (2008). The lipopolysaccharide Parkinson’s disease animal model: mechanistic studies and drug discovery. Fundamental Clin. Pharmacol. 22, 453–464. doi: 10.1111/j.1472-8206.2008.00616.x
Elmore, S. (2007). Apoptosis: a review of programmed cell death. Toxicol. Pathol. 35, 495–516. doi: 10.1080/01926230701320337
Emamzadeh, F. N. (2016). Alpha-synuclein structure, functions, and interactions. J. Res. Med. Sci. Off. J. Isfahan Univ. Med. Sci. 21, 29–29. doi: 10.4103/1735-1995.181989
Erny, D., Hrabě De Angelis, A. L., Jaitin, D., Wieghofer, P., Staszewski, O., David, E., et al. (2015). Host microbiota constantly control maturation and function of microglia in the CNS. Nat. Neurosci. 18, 965–977. doi: 10.1038/nn.4030
Fang, F., Peng, T., Yang, S., Wang, W., Zhang, Y., and Li, H. (2016). Lycium barbarum polysaccharide attenuates the cytotoxicity of mutant huntingtin and increases the activity of AKT. Int. J. Dev. Neurosci. 52, 66–74. doi: 10.1016/j.ijdevneu.2016.05.004
Feng, C., Li, J., Kong, M., Liu, Y., Cheng, X. J., Li, Y., et al. (2015). Surface charge effect on mucoadhesion of chitosan based nanogels for local anti-colorectal cancer drug delivery. Colloids Surf. B Biointerfaces 128, 439–447. doi: 10.1016/j.colsurfb.2015.02.042
Ferreira-Vieira, T. H., Guimaraes, I. M., Silva, F. R., and Ribeiro, F. M. (2016). Alzheimer’s disease: targeting the cholinergic system. Curr. Neuropharmacol. 14, 101–115. doi: 10.2174/1570159x13666150716165726
Filatova, E. V., Alieva, A., Shadrina, M. I., Shulskaya, M. V., Fedotova, E. Y., Illarioshkin, S. N., et al. (2014). [Analysis of mutations in patients with suspected autosomal dominant form of the Parkinson disease]. Mol. Gen. Mikrobiol. Virusol. 29, 1–3.
Foster, L. J., and Karsten, E. (2012). A chitosan based, laser activated thin film surgical adhesive, ‘SurgiLux’: preparation and demonstration. J. Vis. Exp. 3527. doi: 10.3791/3527
Gao, K., Liu, M., Cao, J., Yao, M., Lu, Y., Li, J., et al. (2014). Protective effects of Lycium barbarum polysaccharide on 6-OHDA-induced apoptosis in PC12 cells through the ROS-NO pathway. Molecules 20, 293–308. doi: 10.3390/molecules20010293
Gao, Y., Li, B., Liu, H., Tian, Y., Gu, C., Du, X., et al. (2021). Cistanche deserticola polysaccharides alleviate cognitive decline in aging model mice by restoring the gut microbiota-brain axis. Aging (Albany NY) 13, 15320–15335. doi: 10.18632/aging.203090
Gao, Y., Li, C., Yin, J., Shen, J., Wang, H., Wu, Y., et al. (2012). Fucoidan, a sulfated polysaccharide from brown algae, improves cognitive impairment induced by infusion of Aβ peptide in rats. Environ. Toxicol. Pharmacol. 33, 304–311. doi: 10.1016/j.etap.2011.12.022
Guix, F. X., Wahle, T., Vennekens, K., Snellinx, A., Chávez-Gutiérrez, L., Ill-Raga, G., et al. (2012). Modification of γ-secretase by nitrosative stress links neuronal ageing to sporadic Alzheimer’s disease. EMBO Mol. Med. 4, 660–673. doi: 10.1002/emmm.201200243
Guo, D., Zhou, J., Zhang, M., Taximaimaiti, R., Wang, X., and Wang, H. (2021). Momordica Charantia polysaccharides attenuates MPP+-Induced injury in Parkinson’s disease mice and cell models by regulating TLR4/MyD88/NF-κB pathway. Int. J. Polym. Sci. 2021:5575636.
Guo, S. S., Cui, X. L., and Rausch, W. D. (2016). Ganoderma Lucidum polysaccharides protect against MPP(+) and rotenone-induced apoptosis in primary dopaminergic cell cultures through inhibiting oxidative stress. Am. J. Neurodegener. Dis. 5, 131–144.
Guo, X., Yuan, J., Song, X., Wang, X., Sun, Q., Tian, J., et al. (2020). Bacteria metabolites from Peganum harmala L. polysaccharides inhibits polyQ aggregation through proteasome-mediated protein degradation in C. elegans. Int. J. Biol. Macromol. 161, 681–691. doi: 10.1016/j.ijbiomac.2020.06.091
Han, C., Shen, H., Yang, Y., Sheng, Y., Wang, J., Li, W., et al. (2020). Antrodia camphorata polysaccharide resists 6-OHDA-induced dopaminergic neuronal damage by inhibiting ROS-NLRP3 activation. Brain Behav. 10:e01824. doi: 10.1002/brb3.1824
Han, Y., Nan, S., Fan, J., Chen, Q., and Zhang, Y. (2019). Inonotus obliquus polysaccharides protect against Alzheimer’s disease by regulating Nrf2 signaling and exerting antioxidative and antiapoptotic effects. Int. J. Biol. Macromol. 131, 769–778. doi: 10.1016/j.ijbiomac.2019.03.033
Ho, Y. S., Yu, M. S., Yang, X. F., So, K. F., Yuen, W. H., and Chang, R. C. (2010). Neuroprotective effects of polysaccharides from wolfberry, the fruits of Lycium barbarum, against homocysteine-induced toxicity in rat cortical neurons. J. Alzheimers Dis. 19, 813–827. doi: 10.3233/JAD-2010-1280
Hou, C., Chen, L., Yang, L., and Ji, X. (2020). An insight into anti-inflammatory effects of natural polysaccharides. Int. J. Biol. Macromol. 153, 248–255. doi: 10.1016/j.ijbiomac.2020.02.315
Hou, X., Watzlawik, J. O., Fiesel, F. C., and Springer, W. (2020). Autophagy in Parkinson’s disease. J. Mol. Biol. 432, 2651–2672. doi: 10.1016/j.jmb.2020.01.037
Huang, S., Mao, J., Ding, K., Zhou, Y., Zeng, X., Yang, W., et al. (2017). Polysaccharides from ganoderma lucidum promote cognitive function and neural progenitor proliferation in mouse model of Alzheimer’s disease. Stem Cell Rep. 8, 84–94. doi: 10.1016/j.stemcr.2016.12.007
Huang, S., Yuan, H., Li, W., Liu, X., Zhang, X., Xiang, D., et al. (2021). Polygonatum sibiricum polysaccharides protect against MPP-Induced neurotoxicity via the Akt/mTOR and Nrf2 pathways. Oxid. Med. Cell Longev. 2021:8843899. doi: 10.1155/2021/8843899
Huang, W. J., Chen, W. W., and Zhang, X. (2016). Huntington’s disease: molecular basis of pathology and status of current therapeutic approaches. Exp. Ther. Med. 12, 1951–1956. doi: 10.3892/etm.2016.3566
Husemann, J., and Silverstein, S. C. (2001). Expression of scavenger receptor class B, type I, by astrocytes and vascular smooth muscle cells in normal adult mouse and human brain and in Alzheimer’s disease brain. Am. J. Pathol. 158, 825–832. doi: 10.1016/S0002-9440(10)64030-8
Hwang, Y. H., Jang, S. A., Lee, A., Cho, C. W., Song, Y. R., Hong, H. D., et al. (2020). Polysaccharides isolated from lotus leaves (LLEP) exert anti-osteoporotic effects by inhibiting osteoclastogenesis. Int. J. Biol. Macromol. 161, 449–456. doi: 10.1016/j.ijbiomac.2020.06.059
Jenner, P. (2002). Pharmacology of dopamine agonists in the treatment of Parkinson’s disease. Neurology 58(Suppl. 1) S1–S8. doi: 10.1212/wnl.58.suppl_1.s1
Jiang, M. H., Zhu, L., and Jiang, J. G. (2010). Immunoregulatory actions of polysaccharides from Chinese herbal medicine. Expert Opin. Ther. Targets 14, 1367–1402. doi: 10.1517/14728222.2010.531010
Jimenez-Sanchez, M., Licitra, F., Underwood, B. R., and Rubinsztein, D. C. (2017). Huntington’s disease: mechanisms of pathogenesis and therapeutic strategies. Cold Spring Harb. Perspect. Med. 7:a024240. doi: 10.1101/cshperspect.a024240
Kametani, F., and Hasegawa, M. (2018). Reconsideration of amyloid hypothesis and tau hypothesis in Alzheimer’s disease. Front. Neurosci. 12:25. doi: 10.3389/fnins.2018.00025
Kang, B., Opatz, T., Landfester, K., and Wurm, F. R. (2015). Carbohydrate nanocarriers in biomedical applications: functionalization and construction. Chem. Soc. Rev. 44, 8301–8325. doi: 10.1039/c5cs00092k
Kim, J., Karthivashan, G., Kweon, M. H., Kim, D. H., and Choi, D. K. (2019). The ameliorative effects of the Ethyl acetate extract of Salicornia europaea L. and its bioactive candidate, Irilin B, on LPS-induced microglial inflammation and MPTP-intoxicated PD-like mouse model. Oxid. Med. Cell Longev. 2019:6764756. doi: 10.1155/2019/6764756
Kim, J. H., Kim, S., Yoon, I. S., Lee, J. H., Jang, B. J., Jeong, S. M., et al. (2005). Protective effects of ginseng saponins on 3-nitropropionic acid-induced striatal degeneration in rats. Neuropharmacology 48, 743–756. doi: 10.1016/j.neuropharm.2004.12.013
Kukreja, L., Kujoth, G. C., Prolla, T. A., Van Leuven, F., and Vassar, R. (2014). Increased mtDNA mutations with aging promotes amyloid accumulation and brain atrophy in the APP/Ld transgenic mouse model of Alzheimer’s disease. Mol. Neurodegener. 9:16. doi: 10.1186/1750-1326-9-16
Kumar, A., Kumar, V., Singh, K., Kumar, S., Kim, Y. S., Lee, Y. M., et al. (2020). Therapeutic advances for Huntington’s disease. Brain Sci. 10:43. doi: 10.3390/brainsci10010043
Kwon, M. J., Kim, S., Han, M. H., and Lee, S. B. (2016). Epigenetic changes in neurodegenerative diseases. Mol. Cells 39, 783–789. doi: 10.14348/molcells.2016.0233
Lee, S. W., Park, H. J., Im, W., Kim, M., and Hong, S. (2018). Repeated immune activation with low-dose lipopolysaccharide attenuates the severity of Huntington’s disease in R6/2 transgenic mice. Anim. Cells Syst. 22, 219–226. doi: 10.1080/19768354.2018.1473291
Lee, Y. S., Lai, D. M., Huang, H. J., Lee-Chen, G. J., Chang, C. H., Hsieh-Li, H. M., et al. (2021). Prebiotic lactulose ameliorates the cognitive deficit in Alzheimer’s disease mouse model through macroautophagy and chaperone-mediated autophagy pathways. J. Agric. Food Chem. 69, 2422–2437. doi: 10.1021/acs.jafc.0c07327
Lesage, S., and Brice, A. (2009). Parkinson’s disease: from monogenic forms to genetic susceptibility factors. Hum. Mol. Genet. 18, R48–R59. doi: 10.1093/hmg/ddp012
Li, G., Zhou, Y., Yang, W. Y., Zhang, C., Hong, L., and Jia, L. (2021). Inhibitory effects of sulfated polysaccharides from the sea cucumber cucumaria frondosa against Aβ40 aggregation and cytotoxicity. ACS Chem. Neurosci. 12, 1854–1859. doi: 10.1021/acschemneuro.1c00223
Li, Q., Li, C., Yang, C., Liu, C., Yu, G., and Guan, H. (2013). Preparation, characterization and antioxidant activities of polymannuronic acid phosphate, H-phosphonate and sulfate. Int. J. Biol. Macromol. 62, 281–286. doi: 10.1016/j.ijbiomac.2013.09.012
Li, R., Yin, F., Guo, Y., Ruan, Q., and Zhu, Q. (2018). Angelica polysaccharide protects PC-12 cells from lipopolysaccharide-induced injury via down-regulating microRNA-223. Biomed. Pharmacother. 108, 1320–1327. doi: 10.1016/j.biopha.2018.09.147
Li, X., Chen, C., Leng, A., and Qu, J. (2021). Advances in the extraction, purification, structural characteristics and biological activities of Eleutherococcus senticosus polysaccharides: a promising medicinal and edible resource with development value. Front. Pharmacol. 12:753007.
Li, Y., Guan, S., Liu, C., Chen, X., Zhu, Y., Xie, Y., et al. (2018). Neuroprotective effects of Coptis chinensis Franch polysaccharide on amyloid-beta (Aβ)-induced toxicity in a transgenic Caenorhabditis elegans model of Alzheimer’s disease (AD). Int. J. Biol. Macromol. 113, 991–995. doi: 10.1016/j.ijbiomac.2018.03.035
Lin, Y. R., Guan, Q. Y., Li, L. Y., Tang, Z. M., Zhang, Q., and Zhao, X. H. (2021). In vitro immuno-modulatory potentials of purslane (Portulaca oleracea L.) polysaccharides with a chemical selenylation. Foods 11:14. doi: 10.3390/foods11010014
Liu, C., Cui, Y., Pi, F., Cheng, Y., Guo, Y., and Qian, H. (2019). Extraction, purification, structural characteristics, biological activities and pharmacological applications of acemannan, a polysaccharide from Aloe vera: a review. Molecules 24:1554. doi: 10.3390/molecules24081554
Liu, H., Chen, S., Guo, C., Tang, W., Liu, W., and Liu, Y. (2018). Astragalus polysaccharide protects neurons and stabilizes mitochondrial in a mouse model of Parkinson disease. Med. Sci. Monit. 24, 5192–5199. doi: 10.12659/MSM.908021
Liu, J., Liu, W., Lu, Y., Tian, H., Duan, C., Lu, L., et al. (2018). Piperlongumine restores the balance of autophagy and apoptosis by increasing BCL2 phosphorylation in rotenone-induced Parkinson disease models. Autophagy 14, 845–861. doi: 10.1080/15548627.2017.1390636
Liu, J., Pu, H., Liu, S., Kan, J., and Jin, C. (2017). Synthesis, characterization, bioactivity and potential application of phenolic acid grafted chitosan: a review. Carbohydr. Polym. 174, 999–1017. doi: 10.1016/j.carbpol.2017.07.014
Liu, L., Sha, X. Y., Wu, Y. N., Chen, M. T., and Zhong, J. X. (2020). Lycium barbarum polysaccharides protects retinal ganglion cells against oxidative stress injury. Neural Regen. Res. 15, 1526–1531. doi: 10.4103/1673-5374.274349
Liu, R., and Li, X. (2018). Radix Ophiopogonis polysaccharide extracts alleviate MPP(+)-induced PC-12 cell injury through inhibition of Notch signaling pathway. Int. J. Clin. Exp. Pathol. 11, 99–109.
Luk, K. C., Kehm, V., Carroll, J., Zhang, B., O’brien, P., Trojanowski, J. Q., et al. (2012). Pathological α-synuclein transmission initiates Parkinson-like neurodegeneration in nontransgenic mice. Science 338, 949–953. doi: 10.1126/science.1227157
Luo, T., Wu, J., Kabadi, S. V., Sabirzhanov, B., Guanciale, K., Hanscom, M., et al. (2013). Propofol limits microglial activation after experimental brain trauma through inhibition of nicotinamide adenine dinucleotide phosphate oxidase. Anesthesiology 119, 1370–1388. doi: 10.1097/ALN.0000000000000020
Makani, V., Jang, Y. G., Christopher, K., Judy, W., Eckstein, J., Hensley, K., et al. (2016). BBB-permeable, neuroprotective, and neurotrophic polysaccharide, Midi-GAGR. PLoS One 11:e0149715. doi: 10.1371/journal.pone.0149715
Mandemakers, W., Morais, V. A., and De Strooper, B. (2007). A cell biological perspective on mitochondrial dysfunction in Parkinson disease and other neurodegenerative diseases. J. Cell. Sci. 120(Pt 10), 1707–1716. doi: 10.1242/jcs.03443
Manigandan, V., Nataraj, J., Karthik, R., Manivasagam, T., Saravanan, R., Thenmozhi, A. J., et al. (2019). Low molecular weight sulfated chitosan: neuroprotective effect on rotenone-induced in vitro Parkinson’s disease. Neurotox Res. 35, 505–515. doi: 10.1007/s12640-018-9978-z
Mantzavinos, V., and Alexiou, A. (2017). Biomarkers for Alzheimer’s disease diagnosis. Curr. Alzheimer Res. 14, 1149–1154.
Margolis, R. L., and Ross, C. A. (2003). Diagnosis of Huntington disease. Clin. Chem. 49, 1726–1732. doi: 10.1373/49.10.1726
Marsh, A. P. (2019). Molecular mechanisms of proteinopathies across neurodegenerative disease: a review. Neurol. Res. Pract. 1:35.
Marsh, B. J., and Stenzel-Poore, M. P. (2008). Toll-like receptors: novel pharmacological targets for the treatment of neurological diseases. Curr. Opin. Pharmacol. 8, 8–13. doi: 10.1016/j.coph.2007.09.009
Meka, D. P., Müller-Rischart, A. K., Nidadavolu, P., Mohammadi, B., Motori, E., Ponna, S. K., et al. (2015). Parkin cooperates with GDNF/RET signaling to prevent dopaminergic neuron degeneration. J. Clin. Invest. 125, 1873–1885. doi: 10.1172/JCI79300
Menozzi, E., Macnaughtan, J., and Schapira, A. H. V. (2021). The gut-brain axis and Parkinson disease: clinical and pathogenetic relevance. Ann. Med. 53, 611–625. doi: 10.1080/07853890.2021.1890330
Morris, G. P., Clark, I. A., and Vissel, B. (2014). Inconsistencies and controversies surrounding the amyloid hypothesis of Alzheimer’s disease. Acta Neuropathol. Commun. 2:135. doi: 10.1186/s40478-014-0135-5
Mozammil Hasnain, S. M., Hasnain, M. S., and Nayak, A. K. (2019). “Chapter 1 - Natural polysaccharides: sources and extraction methodologies,” in Natural Polysaccharides in Drug Delivery and Biomedical Applications, eds M. S. Hasnain and A. K. Nayak (Cambridge, MA: Academic Press), 1–14. doi: 10.1007/978-1-0716-1398-6_16
Muddapu, V. R., Dharshini, S. A. P., Chakravarthy, V. S., and Gromiha, M. M. (2020). Neurodegenerative diseases – is metabolic deficiency the root cause? Front. Neurosci. 14:213. doi: 10.3389/fnins.2020.00213
Murphy, K., Llewellyn, K., Wakser, S., Pontasch, J., Samanich, N., Flemer, M., et al. (2018). Mini-GAGR, an intranasally applied polysaccharide, activates the neuronal Nrf2-mediated antioxidant defense system. J. Biol. Chem. 293, 18242–18269. doi: 10.1074/jbc.RA117.001245
Nagatsu, T., and Sawada, M. (2006). Molecular mechanism of the relation of monoamine oxidase B and its inhibitors to Parkinson’s disease: possible implications of glial cells. J. Neural Transm. Suppl. 71, 53–65. doi: 10.1007/978-3-211-33328-0_7
Neubrech, F., Sauerbier, M., Moll, W., Seegmüller, J., Heider, S., Harhaus, L., et al. (2018). Enhancing the outcome of traumatic sensory nerve lesions of the hand by additional use of a chitosan nerve tube in primary nerve repair: a randomized controlled bicentric trial. Plastic Reconstruct. Surg. 142, 415–424. doi: 10.1097/PRS.0000000000004574
Ngwuluka, N., Pillay, V., Du Toit, L. C., Ndesendo, V., Choonara, Y., Modi, G., et al. (2010). Levodopa delivery systems: advancements in delivery of the gold standard. Expert Opin. Drug Deliv. 7, 203–224. doi: 10.1517/17425240903483166
Nimmrich, V., and Eckert, A. (2013). Calcium channel blockers and dementia. Br. J. Pharmacol. 169, 1203–1210. doi: 10.1111/bph.12240
Nixon, R. A. (2013). The role of autophagy in neurodegenerative disease. Nat. Med. 19, 983–997. doi: 10.1038/nm.3232
Odeniyi, M. A., Omoteso, O. A., Adepoju, A. O., and Jaiyeoba, K. T. (2018). Starch nanoparticles in drug delivery: a review. Polim. Med. 48, 41–45. doi: 10.17219/pim/99993
Ostadkarampour, M., and Putnins, E. E. (2021). Monoamine oxidase inhibitors: a review of their anti-inflammatory therapeutic potential and mechanisms of action. Front. Pharmacol. 12:676239. doi: 10.3389/fphar.2021.676239
Page, M. J., Kell, D. B., and Pretorius, E. (2022). The role of lipopolysaccharide-induced cell signalling in chronic inflammation. Chronic Stress (Thousand Oaks, Calif.) 6:24705470221076390. doi: 10.1177/24705470221076390
Pelegrí, C., Duran-Vilaregut, J., Del Valle, J., Crespo-Biel, N., Ferrer, I., Pallàs, M., et al. (2008). Cell cycle activation in striatal neurons from Huntington’s disease patients and rats treated with 3-nitropropionic acid. Int. J. Dev. Neurosci. 26, 665–671. doi: 10.1016/j.ijdevneu.2008.07.016
Pellegrini, C., Antonioli, L., Colucci, R., Blandizzi, C., and Fornai, M. (2018). Interplay among gut microbiota, intestinal mucosal barrier and enteric neuro-immune system: a common path to neurodegenerative diseases? Acta Neuropathol. 136, 345–361. doi: 10.1007/s00401-018-1856-5
Perez-Pardo, P., Dodiya, H. B., Engen, P. A., Forsyth, C. B., Huschens, A. M., Shaikh, M., et al. (2019). Role of TLR4 in the gut-brain axis in Parkinson’s disease: a translational study from men to mice. Gut 68, 829–843. doi: 10.1136/gutjnl-2018-316844
Plaza-Zabala, A., Sierra-Torre, V., and Sierra, A. (2017). Autophagy and microglia: novel partners in neurodegeneration and aging. Int. J. Mol. Sci. 18:598. doi: 10.3390/ijms18030598
Poewe, W., Seppi, K., Tanner, C. M., Halliday, G. M., Brundin, P., Volkmann, J., et al. (2017). Parkinson disease. Nat. Rev. Dis. Primers 3:17013. doi: 10.1038/nrdp.2017.13
Potkin, K. T., and Potkin, S. G. (2018). New directions in therapeutics for Huntington disease. Future Neurol. 13, 101–121. doi: 10.2217/fnl-2017-0035
Qin, Y., Xiong, L., Li, M., Liu, J., Wu, H., Qiu, H., et al. (2018). Preparation of bioactive polysaccharide nanoparticles with enhanced radical scavenging activity and antimicrobial activity. J. Agric. Food Chem. 66, 4373–4383. doi: 10.1021/acs.jafc.8b00388
Rai, S. N., Singh, P., Varshney, R., Chaturvedi, V. K., Vamanu, E., Singh, M. P., et al. (2021). Promising drug targets and associated therapeutic interventions in Parkinson’s disease. Neural Regen. Res. 16, 1730–1739. doi: 10.4103/1673-5374.306066
Raj, R., Wairkar, S., Sridhar, V., and Gaud, R. (2018). Pramipexole dihydrochloride loaded chitosan nanoparticles for nose to brain delivery: development, characterization and in vivo anti-Parkinson activity. Int. J. Biol. Macromol. 109, 27–35. doi: 10.1016/j.ijbiomac.2017.12.056
Ramakrishnan, S., and Gupta, V. (2021). Trinucleotide repeat disorders. StatPearls. Trinucleotide repeat disorders. Annu. Rev. Neurosci. 30, 575–621.
Reijonen, S., Putkonen, N., Nørremølle, A., Lindholm, D., and Korhonen, L. (2008). Inhibition of endoplasmic reticulum stress counteracts neuronal cell death and protein aggregation caused by N-terminal mutant huntingtin proteins. Exp. Cell. Res. 314, 950–960. doi: 10.1016/j.yexcr.2007.12.025
Reith, W. (2018). [Neurodegenerative diseases]. Radiologe 58, 241–258. doi: 10.1007/s00117-018-0363-y
Ren, F., Yang, Y., Wu, K., Zhao, T., Shi, Y., Song, M., et al. (2021). The effects of dandelion polysaccharides on iron metabolism by regulating hepcidin via JAK/STAT signaling pathway. Oxid. Med. Cell. Longev. 2021:7184760. doi: 10.1155/2021/7184760
Ren, X., and Butterfield, D. A. (2021). Fidelity of the PINK1 knockout rat to oxidative stress and other characteristics of Parkinson disease. Free Radic. Biol. Med. 163, 88–101. doi: 10.1016/j.freeradbiomed.2020.12.004
Ren, Z. L., Wang, C. D., Wang, T., Ding, H., Zhou, M., Yang, N., et al. (2019). Ganoderma lucidum extract ameliorates MPTP-induced parkinsonism and protects dopaminergic neurons from oxidative stress via regulating mitochondrial function, autophagy, and apoptosis. Acta Pharmacol. Sin. 40, 441–450. doi: 10.1038/s41401-018-0077-8
Roberts, J. S., Patterson, A. K., and Uhlmann, W. R. (2020). Genetic testing for neurodegenerative diseases: ethical and health communication challenges. Neurobiol. Dis. 141:104871. doi: 10.1016/j.nbd.2020.104871
Ross, C. A. (2002). Polyglutamine pathogenesis: emergence of unifying mechanisms for Huntington’s disease and related disorders. Neuron 35, 819–822. doi: 10.1016/s0896-6273(02)00872-3
Salamon, A., Zádori, D., Szpisjak, L., Klivényi, P., and Vécsei, L. (2022). What is the impact of catechol-O-methyltransferase (COMT) on Parkinson’s disease treatment? Expert Opin. Pharmacother. 1–6. [Online ahead of print], doi: 10.1080/14656566.2022.2060738
Sarvaiya, J., and Agrawal, Y. K. (2015). Chitosan as a suitable nanocarrier material for anti-Alzheimer drug delivery. Int. J. Biol. Macromol. 72, 454–465. doi: 10.1016/j.ijbiomac.2014.08.052
Sha, X., Xu, X., Liao, S., Chen, H., and Rui, W. (2022). Evidence of immunogenic cancer cell death induced by honey-processed Astragalus polysaccharides in vitro and in vivo. Exp. Cell Res. 410:112948. doi: 10.1016/j.yexcr.2021.112948
Shaik, L., Kashyap, R., Thotamgari, S. R., Singh, R., and Khanna, S. (2020). Gut-Brain axis and its neuro-psychiatric effects: a narrative review. Cureus 12:e11131. doi: 10.7759/cureus.11131
Sharma, K. (2019). Cholinesterase inhibitors as Alzheimer’s therapeutics (Review). Mol. Med. Rep. 20, 1479–1487. doi: 10.3892/mmr.2019.10374
Shen, W. D., Li, X. Y., Deng, Y. Y., Zha, X. Q., Pan, L. H., Li, Q. M., et al. (2021). Polygonatum cyrtonema Hua polysaccharide exhibits anti-fatigue activity via regulating osteocalcin signaling. Int. J. Biol. Macromol. 175, 235–241. doi: 10.1016/j.ijbiomac.2021.01.200
Sheng, Y., Liu, G., Wang, M., Lv, Z., and Du, P. (2017). A selenium polysaccharide from Platycodon grandiflorum rescues PC12 cell death caused by H(2)O(2) via inhibiting oxidative stress. Int. J. Biol. Macromol. 104(Pt A), 393–399. doi: 10.1016/j.ijbiomac.2017.06.052
Sheng, Z. H. (2017). The interplay of axonal energy homeostasis and mitochondrial trafficking and anchoring. Trends Cell Biol. 27, 403–416. doi: 10.1016/j.tcb.2017.01.005
Shin, S. J., Nam, Y., Park, Y. H., Kim, M. J., Lee, E., Jeon, S. G., et al. (2021). Therapeutic effects of non-saponin fraction with rich polysaccharide from Korean red ginseng on aging and Alzheimer’s disease. Free Radic. Biol. Med. 164, 233–248. doi: 10.1016/j.freeradbiomed.2020.12.454
Southwell, A. L., and Patterson, P. H. (2011). Gene therapy in mouse models of huntington disease. Neuroscientist 17, 153–162. doi: 10.1177/1073858410386236
Spires-Jones, T. L., and Hyman, B. T. (2014). The intersection of amyloid beta and tau at synapses in Alzheimer’s disease. Neuron 82, 756–771. doi: 10.1016/j.neuron.2014.05.004
Su, C., Li, N., Ren, R., Wang, Y., Su, X., Lu, F., et al. (2021). Progress in the medicinal value, bioactive compounds, and pharmacological activities of Gynostemma pentaphyllum. Molecules 26:6249. doi: 10.3390/molecules26206249
Sun, Q., Cheng, L., Zeng, X., Zhang, X., Wu, Z., and Weng, P. (2020). The modulatory effect of plant polysaccharides on gut flora and the implication for neurodegenerative diseases from the perspective of the microbiota-gut-brain axis. Int. J. Biol. Macromol. 164, 1484–1492. doi: 10.1016/j.ijbiomac.2020.07.208
Sun, X. Z., Liao, Y., Li, W., and Guo, L. M. (2017). Neuroprotective effects of ganoderma lucidum polysaccharides against oxidative stress-induced neuronal apoptosis. Neural Regen. Res. 12, 953–958. doi: 10.4103/1673-5374.208590
Tan, Y., Yin, L., Sun, Z., Shao, S., Chen, W., Man, X., et al. (2020). Astragalus polysaccharide exerts anti-Parkinson via activating the PI3K/AKT/mTOR pathway to increase cellular autophagy level in vitro. Int. J. Biol. Macromol. 153, 349–356. doi: 10.1016/j.ijbiomac.2020.02.282
Tang, C., Ding, R., Sun, J., Liu, J., Kan, J., and Jin, C. (2019). The impacts of natural polysaccharides on intestinal microbiota and immune responses - a review. Food Funct. 10, 2290–2312. doi: 10.1039/c8fo01946k
Tarawneh, R., and Holtzman, D. M. (2012). The clinical problem of symptomatic Alzheimer disease and mild cognitive impairment. Cold Spring Harb. Perspect. Med. 2:a006148. doi: 10.1101/cshperspect.a006148
Theocharopoulou, G. (2020). The ubiquitous role of mitochondria in Parkinson and other neurodegenerative diseases. AIMS Neurosci. 7, 43–65. doi: 10.3934/Neuroscience.2020004
Tiwari, P. C., and Pal, R. (2017). The potential role of neuroinflammation and transcription factors in Parkinson disease. Dialogues Clin. Neurosci. 19, 71–80. doi: 10.31887/DCNS.2017.19.1/rpal
Wahlster, L., Arimon, M., Nasser-Ghodsi, N., Post, K. L., Serrano-Pozo, A., Uemura, K., et al. (2013). Presenilin-1 adopts pathogenic conformation in normal aging and in sporadic Alzheimer’s disease. Acta Neuropathol. 125, 187–199. doi: 10.1007/s00401-012-1065-6
Wan, L., Zhang, Q., Luo, H., Xu, Z., Huang, S., Yang, F., et al. (2020). Codonopsis pilosula polysaccharide attenuates Aβ toxicity and cognitive defects in APP/PS1 mice. Aging (Albany NY) 12, 13422–13436. doi: 10.18632/aging.103445
Wang, F., Liu, Q., Wang, W., Li, X., and Zhang, J. (2016). A polysaccharide isolated from Cynomorium songaricum Rupr. protects PC12 cells against H2O2-induced injury. Int. J. Biol. Macromol. 87, 222–228. doi: 10.1016/j.ijbiomac.2016.02.011
Wang, J., Liu, H., Zhang, X., Li, X., Geng, L., Zhang, H., et al. (2017). Sulfated hetero-polysaccharides protect SH-SY5Y cells from H2O2-Induced apoptosis by affecting the PI3K/Akt signaling pathway. Mar. Drugs 15:110. doi: 10.3390/md15040110
Wang, L., Yao, C., Wu, F., Lin, X., Shen, L., and Feng, Y. (2015). Targeting delivery of Radix Ophiopogonis polysaccharide to ischemic/reperfused rat myocardium by long-circulating macromolecular and liposomal carriers. Int. J. Nanomed. 10, 5729–5737. doi: 10.2147/IJN.S89445
Wang, P.-C., Zhao, S., Yang, B.-Y., Wang, Q.-H., and Kuang, H.-X. (2016). Anti-diabetic polysaccharides from natural sources: a review. Carbohydrate Polym. 148, 86–97. doi: 10.1016/j.carbpol.2016.02.060
Wang, Z., Sun, Q., Zhang, H., Wang, J., Fu, Q., Qiao, H., et al. (2021). Insight into antibacterial mechanism of polysaccharides: a review. LWT 150:111929.
Wen, L., Sheng, Z., Wang, J., Jiang, Y., and Yang, B. (2022). Structure of water-soluble polysaccharides in spore of Ganoderma lucidum and their anti-inflammatory activity. Food Chem. 373(Pt A):131374. doi: 10.1016/j.foodchem.2021.131374
Winslow, B. T., Onysko, M. K., Stob, C. M., and Hazlewood, K. A. (2011). Treatment of Alzheimer disease. Am. Fam. Physician 83, 1403–1412.
Wu, J., Lin, C., Chen, X., Pan, N., and Liu, Z. (2021b). Polysaccharides isolated from Bangia fuscopurpurea induce apoptosis and autophagy in human ovarian cancer A2780 cells. Food Sci. Nutr. 9, 6707–6719. doi: 10.1002/fsn3.2621
Wu, J., Chen, T., Wan, F., Wang, J., Li, X., Li, W., et al. (2021a). Structural characterization of a polysaccharide from Lycium barbarum and its neuroprotective effect against β-amyloid peptide neurotoxicity. Int. J. Biol. Macromol. 176, 352–363. doi: 10.1016/j.ijbiomac.2021.02.016
Wu, J. H., Xu, C., Shan, C. Y., and Tan, R. X. (2006). Antioxidant properties and PC12 cell protective effects of APS-1, a polysaccharide from Aloe vera var. chinensis. Life Sci. 78, 622–630. doi: 10.1016/j.lfs.2005.05.097
Xiang, Y., Zhang, J., Li, H., Wang, Q., Xiao, L., Weng, H., et al. (2017). Epimedium polysaccharide alleviates polyglutamine-induced neurotoxicity in Caenorhabditis elegans by reducing oxidative stress. Rejuvenation Res. 20, 32–41. doi: 10.1089/rej.2016.1830
Xu, M., Yan, T., Fan, K., Wang, M., Qi, Y., Xiao, F., et al. (2019). Polysaccharide of Schisandra Chinensis Fructus ameliorates cognitive decline in a mouse model of Alzheimer’s disease. J. Ethnopharmacol. 237, 354–365. doi: 10.1016/j.jep.2019.02.046
Xue, Y., Wang, N., Zeng, Z., Huang, J., Xiang, Z., and Guan, Y.-Q. (2020). Neuroprotective effect of chitosan nanoparticle gene delivery system grafted with acteoside (ACT) in Parkinson’s disease models. J. Mater. Sci. Technol. 43, 197–207.
Yadav, A. K., Mishra, P., and Agrawal, G. P. (2008). An insight on hyaluronic acid in drug targeting and drug delivery. J. Drug Target 16, 91–107. doi: 10.1080/10611860802095494
Yan, H., Ma, X., Mi, Z., He, Z., and Rong, P. (2022). Extracellular polysaccharide from Rhizopus nigricans inhibits hepatocellular carcinoma via miR-494-3p/TRIM36 axis and cyclin E ubiquitination. J. Clin. Transl. Hepatol.
Yan, T., Mao, Q., Zhang, X., Wu, B., Bi, K., He, B., et al. (2021). Schisandra chinensis protects against dopaminergic neuronal oxidative stress, neuroinflammation and apoptosis via the BDNF/Nrf2/NF-κB pathway in 6-OHDA-induced Parkinson’s disease mice. Food Funct. 12, 4079–4091. doi: 10.1039/d0fo02836c
Zeng, H., Li, P., Zhou, L., and Ding, K. (2020). A novel pectin from Polygala tenuifolia blocks Aβ(42) aggregation and production by enhancing insulin-degradation enzyme and neprilysin. Int. J. Biol. Macromol. 161, 35–43. doi: 10.1016/j.ijbiomac.2020.05.212
Zhang, F., Lu, J., Zhang, J.-G., and Xie, J.-X. (2015). Protective effects of a polysaccharide from Spirulina platensis on dopaminergic neurons in an MPTP-induced Parkinson’s disease model in C57BL/6J mice. Neural Regener. Res. 10, 308–313. doi: 10.4103/1673-5374.152387
Zhang, H., Pan, N., Xiong, S., Zou, S., Li, H., Xiao, L., et al. (2012). Inhibition of polyglutamine-mediated proteotoxicity by Astragalus membranaceus polysaccharide through the DAF-16/FOXO transcription factor in Caenorhabditis elegans. Biochem. J. 441, 417–424. doi: 10.1042/BJ20110621
Zhang, H., Wei, W., Zhao, M., Ma, L., Jiang, X., Pei, H., et al. (2021). Interaction between Aβ and Tau in the pathogenesis of Alzheimer’s disease. Int. J. Biol. Sci. 17, 2181–2192. doi: 10.7150/ijbs.57078
Zhang, J., Shi, R., Li, H., Xiang, Y., Xiao, L., Hu, M., et al. (2016). Antioxidant and neuroprotective effects of Dictyophora indusiata polysaccharide in Caenorhabditis elegans. J. Ethnopharmacol. 192, 413–422. doi: 10.1016/j.jep.2016.09.031
Zhang, L., Hao, J., Zheng, Y., Su, R., Liao, Y., Gong, X., et al. (2018). Fucoidan protects dopaminergic neurons by enhancing the mitochondrial function in a rotenone-induced rat model of Parkinson’s disease. Aging Dis. 9, 590–604. doi: 10.14336/AD.2017.0831
Zhang, Q., Xia, Y., Luo, H., Huang, S., Wang, Y., Shentu, Y., et al. (2018). Codonopsis pilosula polysaccharide attenuates tau hyperphosphorylation and cognitive impairments in hTau infected mice. Front. Mol. Neurosci. 11:437. doi: 10.3389/fnmol.2018.00437
Zhang, R., Xu, S., Cai, Y., Zhou, M., Zuo, X., and Chan, P. (2011). Ganoderma lucidum protects dopaminergic neuron degeneration through inhibition of microglial activation. Evid. Based Complement. Alternat. Med. 2011:156810. doi: 10.1093/ecam/nep075
Zhang, S., Pei, R., Li, M., Su, H., Sun, H., Ding, Y., et al. (2022). Cocktail polysaccharides isolated from Ecklonia kurome against the SARS-CoV-2 infection. Carbohydr. Polym. 275:118779. doi: 10.1016/j.carbpol.2021.118779
Zhang, Y., Yang, X., Jin, G., Yang, X., and Zhang, Y. (2016). Polysaccharides from Pleurotus ostreatus alleviate cognitive impairment in a rat model of Alzheimer’s disease. Int. J. Biol. Macromol. 92, 935–941. doi: 10.1016/j.ijbiomac.2016.08.008
Zhang, Z., Liu, H., Yu, B., Tao, H., Li, J., Wu, Z., et al. (2020b). Lycium barbarum polysaccharide attenuates myocardial injury in high-fat diet-fed mice through manipulating the gut microbiome and fecal metabolome. Food Res. Int. 138(Pt B):109778. doi: 10.1016/j.foodres.2020.109778
Zhang, Z., Wang, X., Pan, Y., Wang, G., and Mao, G. (2020c). The degraded polysaccharide from Pyropia haitanensis represses amyloid beta peptide-induced neurotoxicity and memory in vivo. Int. J. Biol. Macromol. 146, 725–729. doi: 10.1016/j.ijbiomac.2019.09.243
Zhang, S., Li, L., Hu, J., Ma, P., and Zhu, H. (2020a). Polysaccharide of Taxus chinensis var. mairei Cheng et L.K.Fu attenuates neurotoxicity and cognitive dysfunction in mice with Alzheimer’s disease. Pharm. Biol. 58, 959–968. doi: 10.1080/13880209.2020.1817102
Zhao, Y., Yan, B., Wang, Z., Li, M., and Zhao, W. (2020). Natural polysaccharides with immunomodulatory activities. Mini Rev. Med. Chem. 20, 96–106. doi: 10.2174/1389557519666190913151632
Zhou, C., Sun, X., Hu, Y., Song, J., Dong, S., Kong, D., et al. (2019). Genomic deletion of TLR2 induces aggravated white matter damage and deteriorated neurobehavioral functions in mouse models of Alzheimer’s disease. Aging (Albany NY) 11, 7257–7273. doi: 10.18632/aging.102260
Zhu, X., Raina, A. K., Perry, G., and Smith, M. A. (2006). Apoptosis in Alzheimer disease: a mathematical improbability. Curr. Alzheimer Res. 3, 393–396. doi: 10.2174/156720506778249470
Zhu, Y., Yu, X., Ge, Q., Li, J., Wang, D., Wei, Y., et al. (2020). Antioxidant and anti-aging activities of polysaccharides from Cordyceps cicadae. Int. J. Biol. Macromol. 157, 394–400. doi: 10.1016/j.ijbiomac.2020.04.163
Keywords: mechanism, polysaccharides, Alzheimer’s disease, Parkinson’s disease, Huntington’s disease
Citation: Wang Y, Chen R, Yang Z, Wen Q, Cao X, Zhao N and Yan J (2022) Protective Effects of Polysaccharides in Neurodegenerative Diseases. Front. Aging Neurosci. 14:917629. doi: 10.3389/fnagi.2022.917629
Received: 11 April 2022; Accepted: 02 June 2022;
Published: 04 July 2022.
Edited by:
Jialiang Yang, Geneis (Beijing) Co. Ltd, ChinaCopyright © 2022 Wang, Chen, Yang, Wen, Cao, Zhao and Yan. This is an open-access article distributed under the terms of the Creative Commons Attribution License (CC BY). The use, distribution or reproduction in other forums is permitted, provided the original author(s) and the copyright owner(s) are credited and that the original publication in this journal is cited, in accordance with accepted academic practice. No use, distribution or reproduction is permitted which does not comply with these terms.
*Correspondence: Ninghui Zhao, zhaoninghui@hotmail.com; Jinyuan Yan, yanjinyuan1011@126.com