- 1Neurovascular Research Unit, Department of Neurology, Copenhagen University Hospital – Herlev and Gentofte, Copenhagen, Denmark
- 2Department of Clinical Medicine, University of Copenhagen, Copenhagen, Denmark
- 3Steno Diabetes Center Copenhagen, Copenhagen, Denmark
- 4Department of Endocrinology, Copenhagen University Hospital – Bispebjerg and Frederiksberg, Copenhagen, Denmark
- 5Center for Neuropsychiatric Schizophrenia Research, Copenhagen University Hospital – Mental Health Center Glostrup, Copenhagen, Denmark
- 6Department of Neurobiology Research, Institute of Molecular Medicine, University of Southern Denmark, Odense, Denmark
- 7Department of Neurology, Odense University Hospital, Odense, Denmark
- 8BRIDGE – Brain Research-Inter-Disciplinary Guided Excellence, Department of Clinical Research, University of Southern Denmark, Odense, Denmark
Background and aims: Glucagon-like peptide 1 (GLP-1) receptor agonists (GLP-1RA) are widely used for the treatment of type 2 diabetes, and recent studies indicate that they may be cardio- and neuroprotective. The safety and effect of a single dose of exenatide, a short-acting GLP-1RA, on cerebral and peripheral arterial function remain unknown.
Methods: In this randomized, double-blind pilot trial, we assigned elderly healthy volunteers without diabetes and no previous history of stroke to receive a single dose of subcutaneous exenatide (5 μg) or placebo. Primary outcome was immediate changes over time in blood flow velocity of the middle cerebral arteries (VMCA) assessed by repeated transcranial Doppler measurements. Secondary outcomes were changes in peripheral arterial function with finger plethysmography, ankle-brachial index (ABI), and inflammatory- and endothelial-specific biomarkers.
Results: Healthy volunteers (13 women and 17 men) were included: (mean ± standard deviation) age: 62 ± 8 years; body weight: 79.6 ± 12.7 kg; VMCA: 65.3 ± 10.7 cm/s; fasting plasma glucose: 5.5 ± 0.5 mmol/L; HbA1c: 33.9 ± 4.1 mmol/mol (5.3 ± 0.38%). No differences between exenatide and placebo group were seen regarding VMCA (p = 0.058), systolic ABI (p = 0.71), plethysmography (p = 0.45), tumor necrosis factor (p = 0.33), interleukin-6 (p = 0.11), interleukin-1β (p = 0.34), vascular cell adhesion molecule 1 (p = 0.73), intercellular adhesion molecule 1 (p = 0.74), or E-selectin (p = 0.31). No severe adverse events were observed.
Conclusion: A single dose of exenatide did not change cerebral blood flow velocity or peripheral vessel function in elderly healthy volunteers. The medication was safe to use in persons without diabetes allowing us to investigate this drug further in search of the neuroprotective mechanisms.
Clinical Trial Registration: https://clinicaltrials.gov, Identifier NCT02838589.
Introduction
In ischemic stroke, removal of the blood clot by thrombolysis or by thrombectomy are currently used as treatment in the hyper acute phase of stroke (Phipps and Cronin, 2020). Due to a narrow therapeutic time window of 4.5–24 h and various contraindications to treatment, not all patients are eligible for this treatment (Phipps and Cronin, 2020). As a result, new therapeutic options for ischemic stroke are investigated with focus on both acute, preventive, and neuroprotective effects. Evidence increasingly suggests that glucagon-like peptide 1 (GLP-1) receptor agonists (GLP-1RAs), widely used for the treatment of type 2 diabetes, may also be used as cardio- or neuroprotectants (Salcedo et al., 2012; Gault and Hölscher, 2018).
GLP-1 is an incretin hormone that increases glucose-dependent pancreatic secretion of insulin (Nauck and Meier, 2016). Multiple drugs have been developed targeting the GLP-1 receptor, and are proven effective in treating diabetes and obesity (Vilsbøll et al., 2012). Recent large clinical studies show that GLP-1RAs reduced both cardio- and cerebrovascular events in patients with diabetes, but similar effects in individuals without diabetes, are yet to be identified in studies (Sheahan et al., 2020). The pharmacological effects of GLP-1RAs are diverse and not restricted to glycemic control (Maskery et al., 2021). Other effects include reduced gastric emptying, reduced food intake via direct neurochemical actions in the brain, and a modulating effect on systemic inflammation (Drucker, 2018). In animal models of ischemic stroke, GLP-1RAs reduce inflammation, endothelial leakage, oxidative stress, and apoptosis, all factors involved in stroke pathophysiology (Marlet et al., 2018). GLP-1RAs may also affect blood vessels directly, although presented results vary (Marlet et al., 2018). In a systematic review, Marlet et al. (2018) showed that one of four studies on animal stroke models found cerebral blood flow (CBF) to increase after GLP-RA treatment, while the three other studies showed no CBF effects. GLP-1RA was in one study found to induce vasodilation in rat mesenteric arteries (Bangshaab et al., 2019) and to induce coronary artery dilation in humans (Clarke et al., 2018). Furthermore, it improves recruitment of microvasculature in human muscles (Sjøberg et al., 2014), apparently nitric oxide-dependent (Chai et al., 2012).
It is yet to be demonstrated by which mechanisms GLP-1RAs protect against cardio- and cerebrovascular events in diabetics or if GLP-1RAs affect the human cerebral vasculature directly. In this study, we aim to examine if a single dose GLP-1RA affects the human cerebral vasculature and changes CBF velocity in healthy elderly individuals without diabetes or cerebrovascular disease, in search of the mechanism behind the neuroprotective effect of GLP-1RAs. Further, we wanted to examine the effect of GLP-1RAs on peripheral arterial function using finger plethysmography and ankle-brachial index (ABI), and by measuring vascular and inflammatory biomarkers. We hypothesize that a single dose of GLP-1RA will change CBF velocity in the middle cerebral arteries (MCA) due to age-related endothelial dysfunction. Further, we hypothesize it will improve peripheral vascular reactivity in the fingers, improve ABI, and reduce levels of inflammatory biomarkers.
This study may improve our understanding of how GLP-1RAs affect the human cerebral and peripheral vasculature in older subjects without diabetes prior to designing larger multi center studies in stroke patients of similar age. Before the intervention and outcomes are tested in stroke patients, it is important to test it in healthy persons as a safety measure.
Materials and methods
The trial followed guidelines for good clinical practice (GCP) and was monitored by the GCP-unit in the Capital Region, Denmark. The Ethics Committee in the Capital Region of Denmark (H-16022532), the Danish Medicines Agency (EudraCT-number: 2016-001221-14), and the Danish Data Protection Agency (j.nr.: HGH-2016-058) approved this study. The trial was registered at ClinicalTrials.gov (NCT02838589). We obtained informed consent according to the Declaration of Helsinki of 1964, as revised in 2008.
Subjects
We included healthy volunteers above the age of 50 years with no prior history of cerebrovascular disease, diabetes, chronic heart failure (NYHA ≥ II), chronic kidney disease, or pancreatitis. Fulfillments of in- and exclusion criteria (Supplementary Material) were established by blood samples, electrocardiogram (ECG), and by a questionnaire. Volunteers were recruited between 2016 and 2017 through advertisement at the hospital and public webpages.1
Design and experimental protocol
This study was conducted as a randomized, double-blind, placebo controlled, parallel-arm pilot trial. Trial eligibility was determined using in- and exclusion criteria on a screening day, where we also performed a neurological examination, obtained blood pressure and heart rate measurements (Microlife BP A100, Taipei, Taiwan), electrocardiogram (GE Healthcare MAC 5500 HD, Chicago, IL, United States), as well as a basic blood screening with same-day standard analysis. We performed a Montreal Cognitive Assessment (MoCA), National Institute of Health Stroke Scale (NIHSS), and modified Rankin Scale (mRS) screening.
On the trial day (Figure 1), patients were asked to refrain from food and drinks, except for essential medication, for 8 h prior to start of trial day (usually from midnight). Patients received a single subcutaneous dose of either 5 μg (0.05 mL) exenatide (Byetta® Pen Injector, AstraZeneca) or placebo (0.9% saline water, 0.05 mL) by a trained study nurse not otherwise involved in the study. For blinding, the investigator left the examination room during administration and the participants were blindfolded during the procedure. In order to detect an immediate vascular effect, we aimed to find a compound with a quick time to maximal concentration (Tmax) and a short half-life (T1/2). Exenatide was chosen because of the relatively short T1/2 of 2.4 h. Exenatide reaches maximal plasma concentration (Cmax) after 2 h, hence we collected the final results after 3 h. Medication/placebo were randomized, packed in similar boxes, and delivered from the Capital Region Hospital Pharmacy, Denmark. The primary endpoint was changes in mean CBF velocity over time in the middle cerebral arteries (VMCA), measured repeatedly by transcranial Doppler (TCD). Secondary endpoints were changes in peripheral endothelial response measured by EndoPAT2000, ABI, blood pressure, serum insulin and plasma glucose, and endothelial and inflammatory biomarkers in peripheral blood samples collected from a peripheral cubital venous catheter. Biomarkers measured were E-selectin, tumor necrosis factor (TNF), interleukin (IL)-6, IL-1β, vascular cell adhesion molecule 1 (VCAM1), and intercellular adhesion molecule 1 (ICAM1). Side effects were recorded on the trial days in a dedicated questionnaire up to 24 h after drug administration corresponding to ten times T1/2. Trial days were performed by author IM and assisted by JÖ. The experiment was conducted with the participant in supine position on a comfortable bed in a quiet and dimly lit room at 21–24°C. A trial day lasted approximately 5 h.
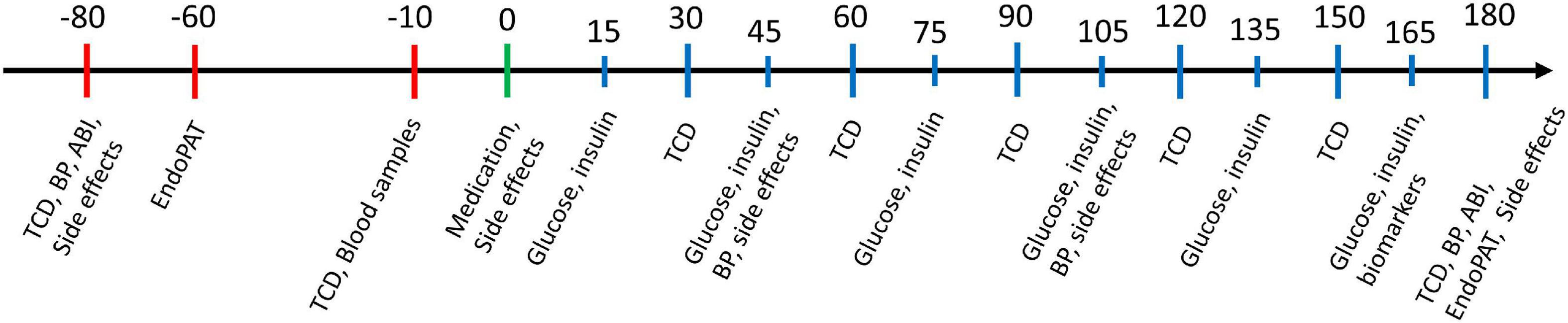
Figure 1. Trial day flowchart. Time shown in minutes with medication time point set to 0 min. Red bars indicate pre-medication methods, green bar indicates medication time point and blue bars indicate post-medication methods. ABI, ankle brachial index; BP, blood pressure; TCD, transcranial doppler.
Transcranial doppler
A time-averaged mean of VMCA was recorded bilaterally by TCD (2 MHz, Multidop X Doppler, DWL; Lübech and Sipplingen, Sipplingen, Germany) using handheld probes according to previously published methods (Ölmestig et al., 2020). The average of four measurements was calculated over a 30 s time interval. A fixed point for measurements of VMCA was chosen along MCA as the point adjacent to the bifurcation between the MCA and the anterior cerebral artery. This fixed point was then used throughout the study in each subject, where every measurement was done after careful optimization of the signal from this point. We conducted two baseline measurements, one at 40 min pre-medication and one just before administration of medication. Measurements were then conducted every 30 min up to 3 h post-medication.
Finger plethysmography
Endothelial function and arterial stiffness were assessed non-invasively by finger plethysmography (EndoPAT2000; Itamar Medical Ltd., Caesarea, Israel) in accordance with our previously published methods (Hansen et al., 2017; Butt et al., 2018; Ölmestig et al., 2020). Measurements were done after 30 min rest, and included a 6-min baseline, 5-min occlusion, and 4-min reactive hyperemia phase. Endothelial function was calculated by measures of pulse wave amplitude on the index finger via pneumatic finger probes during the reactive hyperemia phase of the forearm’s vascular bed (Kuvin et al., 2003). A reactive hyperemia index (RHI) was automatically calculated by the EndoPAT software using an automated algorithm. The values were normalized to the simultaneous signal from the control arm to reduce potential systemic effects. Arterial stiffness, calculated as an augmentation index (AI), was calculated from the arterial waveform and pulse wave amplitude during the baseline period. The augmentation index was defined as the difference between the second and first systolic peaks in the arterial waveform, expressed as a percentage of the central pulse pressure. Due to the influence of heart rate on augmentation index, the values were automatically normalized to a heart rate of 75 beats per minute (QUlANzU=) by the EndoPAT2000 software. High AI values are an indication of stiff arteries. We performed EndoPAT recordings at baseline and 3 h post-medication.
Blood sample analysis
We placed a peripheral cubital venous catheter (BD Venflon Pro, NJ, United States) in the dominant arm and left the arm to rest for 30 min. Baseline blood samples, including insulin and plasma glucose, were drawn just prior to administering medication/placebo. Insulin and plasma glucose samples were then drawn every 30 min post-medication. Blood for biomarker analysis was sampled a second time at the end of the trial day. Blood samples were immediately centrifuged and frozen (-40°C). Biomarkers and insulin were analyzed when all samples for all participants were collected. Plasma glucose was measured using a glucometer. We used multiplex electrochemiluminescence assay kits (Mesoscale, MD, United States) for analyzing the following biomarkers: E-selectin, TNF, IL-6, IL-1β, VCAM1, and ICAM1 (Butt et al., 2018; Ölmestig et al., 2020). Samples were diluted twofold in Diluent 41 prior to measurements and analysis. Samples were analyzed using the MSD Discovery Workbench software. The concentration for the lower limit of detection was calculated based on a signal 2.5 SD above the blank (zero) calibration value. We accepted a coefficient of variation (CV) value cut-off of 25% (Butt et al., 2018; Ölmestig et al., 2020).
Blood pressure, heart rate, and ankle-brachial index
Blood pressure and heart rate (Microlife BP A100, Taipei, Taiwan) were measured twice at baseline and every hour after medication administration during the trial day until 3 h post-medication. ABI was chosen as measure of peripheral arterial function. Ankle blood pressure was measured with a D900 Doppler device with an 8 MHz probe (Huntleigh, Cardiff, Wales, United Kingdom) and brachial blood pressure was measured using the automatic blood pressure monitor. A value less than 0.9 were considered clinically significant.
Statistical Analyses
All values in text are presented as mean ± standard deviation (SD) unless otherwise stated. Sample size was based on previous studies where TCD was used as primary endpoint in patients with migraine and healthy individuals (Kruuse et al., 2002, 2003, 2005; Birk et al., 2004). For the primary endpoint, a mean was calculated for each measurement at the different time points. This mean was used for further statistical analyses. Correction of data was attained by subtraction of the mean of pre-medication baseline values. A repeated-measures ANOVA for TCD, blood pressure, serum insulin, and plasma glucose were performed. An unpaired-sample t-test was used to analyze plethysmography, ABI, and blood biomarkers. To compare the two treatment arms, delta values for the corresponding timepoints for active and placebo treatment were calculated. Raw baseline-subtracted data and delta values were analyzed using repeated-measures ANOVA. When a significant effect of time was seen, we performed a post hoc analysis using unpaired-sample t-tests. Statistics was performed using STATA v. 13.1. A two-sided P-value of < 0.05 was considered statistically significant.
Results
Thirty individuals were included and completed this trial. Subject characteristics are shown in Table 1 and results for all outcomes are presented in Tables 2, 3.
Transcranial doppler
Repeated measurements analyses of TCD showed no difference between treatment groups. Albeit non-significant, there was a trend toward a higher VMCA in the active group compared to placebo during the first hour after administration (Figure 2 and Table 3).
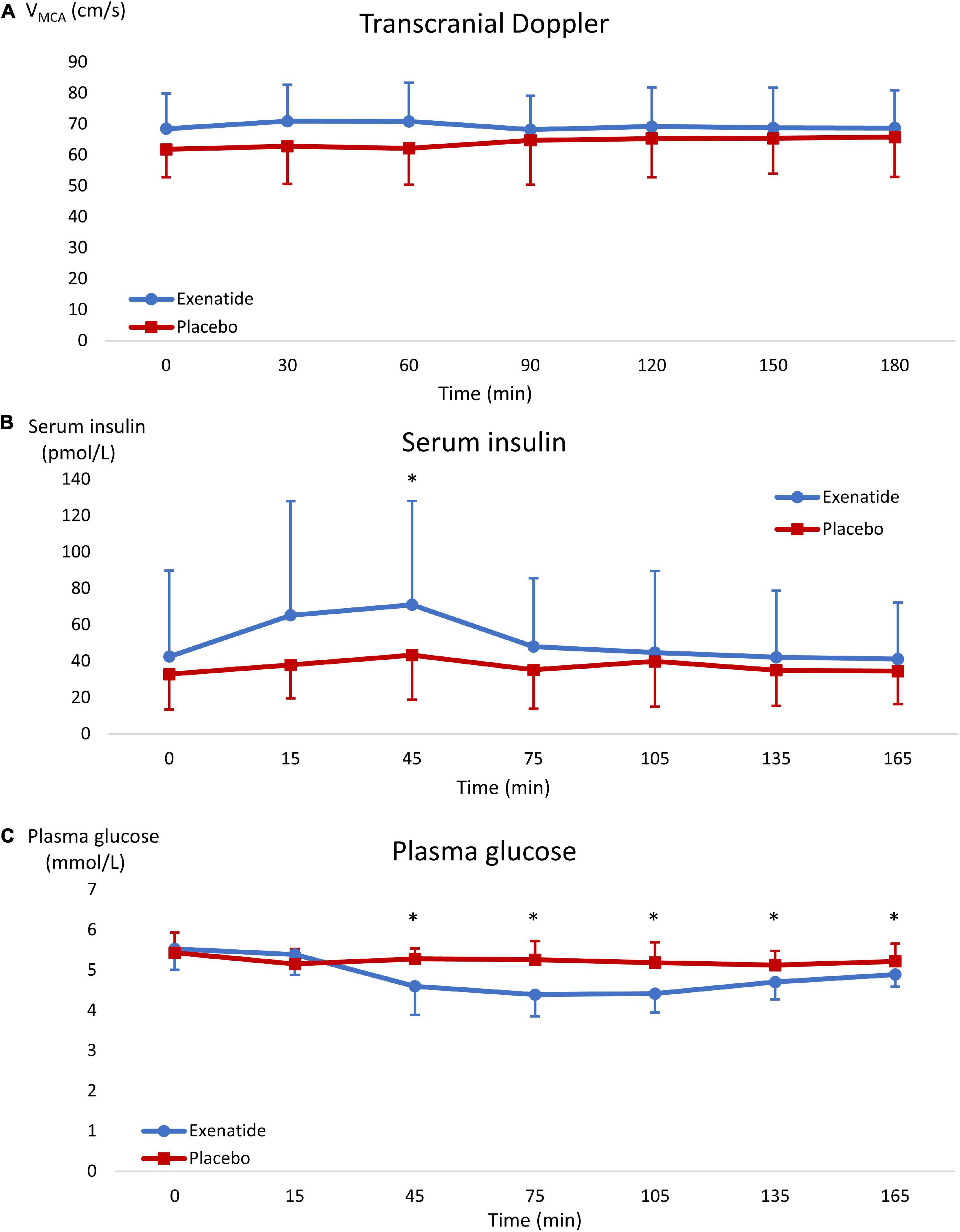
Figure 2. Results graphs. Results are shown as mean ± SD. Blue data series represent the exenatide group and red represents the placebo group. Graph (A) shows Transcranial Doppler, graph (B) shows serum insulin, and graph (C) shows plasma glucose. *, significant difference between groups.
Plethysmography
There was no difference in peripheral arterial function between treatment groups comparing change from baseline and 180 min post-medication assessment (Table 3).
Insulin, glucose, and biomarkers
There was a significant increase in measured insulin concentration at 45 min post-medication in the exenatide group compared to placebo with a mean difference of 28 ± 61 pmol/L (p = 0.0003) (Figure 2). Correspondingly, there was a significant decrease in plasma glucose in the exenatide group, starting from 45 min post-medication throughout the whole trial day (p = 0.0001) with a mean difference of 0.61 ± 0.41 mmol/L between groups (Figure 2). There was no difference in the measured biomarkers concentration from baseline to post-treatment between the groups (Table 3).
Ankle-brachial index and blood pressure
There was no difference in ABI and blood pressure measurements between groups when comparing changes from baseline to 180 min post-medication (Table 3).
Side effects
The main side effects reported after administration of exenatide were headache and gastro-intestinal discomfort, but no serious adverse advents were reported. All side effects were self-limited within one day. For further information on side effects, see Table 4.
Discussion
GLP-1RAs show increasing potential as neuroprotective and stroke prophylactic agents due to positive results on stroke incidence in GLP-1RA treated patients with diabetes (Bellastella et al., 2020). However, little is known on potential mechanisms of action. In this randomized, double-blind, placebo-controlled parallel-arm pilot study, we found no effect of exenatide on cerebral and peripheral vasculature or on inflammatory biomarkers in healthy elderly volunteers. A repeated measurement analysis showed a near-significant difference between groups with an increase in VMCA in the exenatide group. However, this most likely reflects a difference in baseline values between groups, where VMCA was higher in the exenatide-group at baseline.
Our study is the first to assess effect of GLP-1RA on human cerebral and peripheral vasculature as well as inflammatory response in non-diabetic subjects. Since this is a pilot trial, some limitations may apply. First, it is a small pilot study with 15 subjects in each treatment arm. Though previously shown to detect small changes, TCD may be sensitive to head or hand movement. We tried to reduce risk of variations by optimizing the signal to the same anatomical area for each measurement. Second, we did not assess end-tidal carbon dioxide concomitant to TCD measurements. Changes in end-tidal carbon dioxide is known to affect VMCA (Cencetti et al., 1997). However, all individuals in the trial were awake and relaxed in supine position during measurements, thus no change in breathing pattern and end-tidal carbon dioxide was expected.
When we applied TCD measurements, we assessed the CBF velocity in the large arteries in the brain. A change in velocity is an expression of a change in the vascular tone and can be used as a surrogate for blood flow, but only when assuming that the caliber of larger vessels is constant (Kontos, 1989). We found no effect of a single dose exenatide on the MCA indicating that GLP-1RA does not immediately change large artery diameter. However, a single dose of GLP-1RA may not reflect possible long-term vascular changes, which requires further evaluation. In this study, we did not assess possible effects on the cerebral microvasculature, which could be the target for GLP-1RAs in the cerebral vasculature (Chai et al., 2012). To identify general or regional brain microvascular changes in humans, other measurements could be applied, e.g., arterial spin labeling magnetic resonance imaging (MRI) or H2O positron emission tomography (PET). The lack of vascular effects of GLP-1RA in this trial, may relate to inclusion of healthy aging subjects. In both pre-clinical and clinical studies, GLP-1 seemed to improve endothelial dysfunction only with concomitant hyperglycemia, as reported in studies on rat mesenteric arteries (Salheen et al., 2015; Bangshaab et al., 2019) and subcutaneous human arterioles (Koska et al., 2015). We only included individuals without diabetes, and no individuals were observed to have hyperglycemia during the trial, as they were observed during fasting to reduce variance in outcome. This may result in a possible missed hyperglycemic-dependent vascular effect. Exenatide as single dose was found not to have immediate effect on blood pressure. While others also found no acute effect of GLP-1RA on blood pressure (Goud et al., 2016), long-term treatment GLP-1RA treatment had a minor blood pressure reducing effect (Wang et al., 2013; Sun et al., 2015). We found no effect of exenatide on peripheral arterial function measured with EndoPAT. We have previously shown a substantial consistency on day-to-day measurements with EndoPAT but less consistency on same-day measurements, which may be reflected in our findings of no change (Hansen et al., 2017; Ölmestig et al., 2020). ABI is used to estimate peripheral artery disease with analysis of the systolic blood pressure in the ankle divided by the systolic blood pressure in the arm. We found no effect of exenatide on ABI. Since GLP-1RAs had no acute effect on blood pressure, it is less likely to have acute effects on ABI measurements.
It has recently been suggested that GLP-1RA acts as an anti-inflammatory drug (Lee and Jun, 2016). We found that a single dose of exenatide did not change levels of endothelial and inflammatory biomarkers. The lack of effect on inflammatory parameters in this study is probably due to the inclusion of healthy individuals without vascular or chronic inflammatory disease. In contrast to this, previous studies using multiple doses of GLP-1RAs found reductions in circulating pro-inflammatory biomarkers in both animals and humans with diabetes (Lee and Jun, 2016; Zobel et al., 2021). Inflammation appears to play an important role in several acute and chronic diseases, including vascular diseases and diabetes and could be a potential treatment target (Lee and Jun, 2016). In animal models of acute stroke, GLP-1RA reduce TNF and IL-6 levels (Marlet et al., 2018), both pro-inflammatory cytokines involved in stroke pathology (Wilkins and Swerdlow, 2015; Lambertsen et al., 2019). Increased TNF levels have also been confirmed in one human stroke study (Bokhari et al., 2014).
The side-effects of exenatide are few, and it is safe to use in diabetic patients (Knop et al., 2017). We found exenatide to have few and non-severe side-effects in this population of healthy elderly subjects. Most reported side-effects were headache and gastro-intestinal discomfort; however, these were also seen to some degree in the placebo group and may represent an adverse event linked to the trial day and fasting and not the trial drug.
GLP-1RAs have shown to induce vasodilation and increase myocardial blood flow in patients with type 2 diabetes, however the mechanisms by which this occur are poorly understood (Gejl et al., 2012). Bangshaab et al. (2019) investigated a potential mechanism behind the vasodilatory properties of GLP-1RAs. They found that stimulation of the GLP-1 receptors by liraglutide was directly involved in relaxation of branched mesenteric arteries but not arteries without branches in rats (Bangshaab et al., 2019). They further found that GLP-1 improved endothelial function in arteries exposed to hyperglycemia but not normoglycemia (Bangshaab et al., 2019). These findings indicate that the target of GLP-1RAs may be branched arteries and that the vasodilatory effect is mainly seen during hyperglycemia, and possibly also why GLP-1RAs tend to reduce blood pressure in patients with diabetes (Wang et al., 2013; Sun et al., 2015; Goud et al., 2016). However, this effect is often seen after a longer treatment period (Wang et al., 2013; Sun et al., 2015; Goud et al., 2016), indicating that the pharmacological action of GLP-1RA on human vasculature might only present during continuous treatment. It would therefore be of interest to investigate if long-term treatment with a GLP-1RA can influence the cerebral vasculature. However, the effect of GLP-1RAs on vasculature is limited and probably does not fully explain the neuroprotective effect seen in animals and patients with diabetes. The neuroprotective mechanism of GLP-1RAs could be independent of vascular tone and involve other pharmacological actions of GLP-1, e.g., the anti-inflammatory properties (Lee and Jun, 2016) as previously mentioned.
Conclusion and perspectives
This trial investigated the effect and feasibility of a single dose of exenatide (5 μg) on cerebral and peripheral arterial function in healthy elderly volunteers. Exenatide was safe and feasible to use, but it had no acute effect on mean CBF velocity in the MCAs or on peripheral arteries. Previous studies have shown that GLP-1RAs directly affect vasculature, however, we believe we may overlook potential vascular effects by the methods used. Additional studies on the effect of GLP-1RAs on both global and regional CBF are needed, as well as investigation of the cerebral microvasculature, to shed light on the neuroprotective mechanisms behind GLP-1RAs. Finally, it is necessary to investigate this medication in patients with cerebrovascular disease, since GLP-1RAs might act differently in patients with vascular pathology and endothelial dysfunction.
Data availability statement
The raw data supporting the conclusions of this article will be made available by the authors, without undue reservation.
Ethics statement
The studies involving human participants were reviewed and approved by the Ethics Committee in the Capital Region of Denmark (H-16022532). The patients/participants provided their written informed consent to participate in this study.
Author contributions
IM, JÖ, and CK conceived the original data. IM, TV, JR, and CK designed the study. KL performed the analysis of biomarkers. ER performed the statistical analysis. JÖ wrote the manuscript. All authors contributed to the drafts and approved the final version.
Funding
Salary for IM and medical expenses were funded from scholarships from Aase og Ejnar Danielsens Fund (no grant number), Grosserer L. F. Foght’s Fund (no grant number), and AP Møller Foundation for the Advancement of Medical Sciences (no grant number). JÖ was funded from a scholarship from the University of Copenhagen. CK was funded by Borregaard Stipend, Novo Nordisk Foundation, grant number: NNF18OC0031840.
Acknowledgments
Ulla Damgaard Munk was acknowledged for her assistance with blood sample analysis.
Conflict of interest
The authors declare that the research was conducted in the absence of any commercial or financial relationships that could be construed as a potential conflict of interest.
Publisher’s note
All claims expressed in this article are solely those of the authors and do not necessarily represent those of their affiliated organizations, or those of the publisher, the editors and the reviewers. Any product that may be evaluated in this article, or claim that may be made by its manufacturer, is not guaranteed or endorsed by the publisher.
Supplementary material
The Supplementary Material for this article can be found online at: https://www.frontiersin.org/articles/10.3389/fnagi.2022.899389/full#supplementary-material
Abbreviations
ABI, ankle brachial index; AI@75, augmentation index standardized to a heart rate of 75; CBF, cerebral blood flow; CV, coefficient of variation; GCP, good clinical practice; GLP-1, glucagon-like peptide 1; GLP-1RA, glucagon-like peptide 1 receptor agonist; ICAM1, intracellular adhesion molecule 1; IL, interleukin; MoCA, Montreal cognitive assessment; MCA, middle cerebral artery; MRI, magnetic resonance imaging; mRS, modified Rankin scale; NIHSS, national institute of health stroke scale; PET, positron emission tomography; RHI, regional hyperemia index; TCD, transcranial doppler; TNF- α, tumor necrosis factor alpha; VCAM, vascular cell adhesion molecule; VMCA, mean blood flow velocity in the middle cerebral artery.
Footnotes
References
Bangshaab, M., Gutierrez, A., Huynh, K. D., Knudsen, J. S., Arcanjo, D. D. R., Petersen, A. G., et al. (2019). Different mechanisms involved in liraglutide and glucagon-like peptide-1 vasodilatation in rat mesenteric small arteries. Br. J. Pharmacol. 176, 386–399. doi: 10.1111/bph.14534
Bellastella, G., Maiorino, M. I., Longo, M., Scappaticcio, L., Chiodini, P., Esposito, K., et al. (2020). Glucagon-Like Peptide-1 Receptor Agonists and Prevention of Stroke Systematic Review of Cardiovascular Outcome Trials with Meta-Analysis. Stroke 51, 666–669. doi: 10.1161/STROKEAHA.119.027557
Birk, S., Kruuse, C., Petersen, K. A., Jonassen, O., Tfelt-Hansen, P., and Olesen, J. (2004). The phosphodiesterase 3 inhibitor cilostazol dilates large cerebral arteries in humans without affecting regional cerebral blood flow. J. Cereb. Blood Flow Metab. 24, 1352–1358. doi: 10.1097/01.Wcb.0000143536.22131.D7
Bokhari, F. A., Shakoori, T. A., Butt, A., and Ghafoor, F. (2014). TNF-alpha: a risk factor for ischemic stroke. J. Ayub Med. Coll. Abbottabad 26, 111–114.
Butt, J. H., Rostrup, E., Hansen, A. S., Lambertsen, K. L., and Kruuse, C. (2018). Induction of migraine-like headache, but not aura, by cilostazol in patients with migraine with aura. Brain 141, 2943–2951. doi: 10.1093/brain/awy228
Cencetti, S., Bandinelli, G., and Lagi, A. (1997). Effect of PCO2 changes induced by head-upright tilt on transcranial Doppler recordings. Stroke 28, 1195–1197. doi: 10.1161/01.str.28.6.1195
Chai, W., Dong, Z., Wang, N., Wang, W., Tao, L., Cao, W., et al. (2012). Glucagon-like peptide 1 recruits microvasculature and increases glucose use in muscle via a nitric oxide-dependent mechanism. Diabetes 61, 888–896. doi: 10.2337/db11-1073
Clarke, S. J., Giblett, J. P., Yang, L. L., Hubsch, A., Zhao, T., Aetesam-Ur-Rahman, M., et al. (2018). GLP-1 is a coronary artery vasodilator in humans. J. Am. Heart Assoc. 7:e010321. doi: 10.1161/JAHA.118.010321
Drucker, D. J. (2018). Cell Metabolism Review Mechanisms of Action and Therapeutic Application of Glucagon-like Peptide-1. Cell Metab. 27, 740–756. doi: 10.1016/j.cmet.2018.03.001
Gault, V. A., and Hölscher, C. (2018). GLP-1 receptor agonists show neuroprotective effects in animal models of diabetes. Peptides 100, 101–107. doi: 10.1016/J.PEPTIDES.2017.11.017
Gejl, M., Søndergaard, H. M., Stecher, C., Bibby, B. M., Møller, N., Bøtker, H. E., et al. (2012). Exenatide alters myocardial glucose transport and uptake depending on insulin resistance and increases myocardial blood flow in patients with type 2 diabetes. J. Clin. Endocrinol. Metab. 97, E1165–E1169. doi: 10.1210/JC.2011-3456
Goud, A., Zhong, J., Peters, M., Brook, R. D., and Rajagopalan, S. (2016). GLP-1 Agonists and Blood Pressure: a Review of the Evidence. Curr. Hypertens. Rep. 18:16. doi: 10.1007/s11906-015-0621-6
Hansen, A. S., Butt, J. H., Holm-Yildiz, S., Karlsson, W., and Kruuse, C. (2017). Validation of Repeated Endothelial Function Measurements Using EndoPAT in Stroke. Front. Neurol. 8:178. doi: 10.3389/fneur.2017.00178
Knop, F. K., Brønden, A., and Vilsbøll, T. (2017). Exenatide: pharmacokinetics, clinical use, and future directions. Exp. Opin. Pharmacother. 18, 555–571. doi: 10.1080/14656566.2017.1282463
Kontos, H. A. (1989). Validity of cerebral arterial blood flow calculations from velocity measurements. Stroke 20, 1–3. doi: 10.1161/01.STR.20.1.1
Koska, J., Sands, M., Burciu, C., D’Souza, K. M., Raravikar, K., Liu, J., et al. (2015). Exenatide protects against glucoseand lipid-induced endothelial dysfunction: evidence for direct vasodilation effect of glp-1 receptor agonists in humans. Diabetes 64, 2624–2635. doi: 10.2337/db14-0976
Kruuse, C., Khurana, T. S., Rybalkin, S. D., Birk, S., Engel, U., Edvinsson, L., et al. (2005). Phosphodiesterase 5 and effects of sildenafil on cerebral arteries of man and guinea pig. Eur. J. Pharmacol. 521, 105–114. doi: 10.1016/j.ejphar.2005.07.017
Kruuse, C., Thomsen, L. L., Birk, S., and Olesen, J. (2003). Migraine can be induced by sildenafil without changes in middle cerebral artery diameter. Brain 126, 241–247. doi: 10.1093/brain/awg009
Kruuse, C., Thomsen, L. L., Jacobsen, T. B., and Olesen, J. (2002). The phosphodiesterase 5 inhibitor sildenafil has no effect on cerebral blood flow or blood velocity, but nevertheless induces headache in healthy subjects. J. Cereb. Blood Flow Metab. 22, 1124–1131. doi: 10.1097/00004647-200209000-00010
Kuvin, J. T., Patel, A. R., Sliney, K. A., Pandian, N. G., Sheffy, J., Schnall, R. P., et al. (2003). Assessment of peripheral vascular endothelial function with finger arterial pulse wave amplitude. Am. Hear. J. 146, 168–174. doi: 10.1016/s0002-8703(03)00094-2
Lambertsen, K. L., Finsen, B., and Clausen, B. H. (2019). Post-stroke inflammation-target or tool for therapy? Acta Neuropathol. 137, 693–714. doi: 10.1007/S00401-018-1930-Z
Lee, Y. S., and Jun, H. S. (2016). Anti-Inflammatory Effects of GLP-1-Based Therapies beyond Glucose Control. Mediators Inflamm. 2016:3094642. doi: 10.1155/2016/3094642
Marlet, I. R., Ölmestig, J. N. E., Vilsbøll, T., Rungby, J., and Kruuse, C. (2018). Neuroprotective Mechanisms of Glucagon-like Peptide-1-based Therapies in Ischaemic Stroke: a Systematic Review based on Pre-Clinical Studies. Basic Clin. Pharmacol. Toxicol. 122, 559–569. doi: 10.1111/bcpt.12974
Maskery, M. P., Holscher, C., Jones, S. P., Price, C. I., Strain, W. D., Watkins, C. L., et al. (2021). Glucagon-like peptide-1 receptor agonists as neuroprotective agents for ischemic stroke: a systematic scoping review. J. Cereb. Blood Flow Metab. 41, 14–30. doi: 10.1177/0271678X20952011
Nauck, M. A., and Meier, J. J. (2016). The incretin effect in healthy individuals and those with type 2 diabetes: physiology, pathophysiology, and response to therapeutic interventions. Lancet Diabetes Endocrinol. 4, 525–536. doi: 10.1016/S2213-8587(15)00482-9
Ölmestig, J., Marlet, I. R., Hansen, R. H., Rehman, S., Krawcyk, R. S., Rostrup, E., et al. (2020). Tadalafil may improve cerebral perfusion in small vessel occlusion stroke – pilot study. Brain Commun. 2:fcaa020. doi: 10.1093/braincomms/fcaa020
Phipps, M. S., and Cronin, C. A. (2020). Management of acute ischemic stroke. BMJ 368:l6983. doi: 10.1136/bmj.l6983
Salcedo, I., Tweedie, D., Li, Y., and Greig, N. H. (2012). Neuroprotective and neurotrophic actions of glucagon-like peptide-1: an emerging opportunity to treat neurodegenerative and cerebrovascular disorders. Br. J. Pharmacol. 166:1586. doi: 10.1111/J.1476-5381.2012.01971.X
Salheen, S. M., Panchapakesan, U., Pollock, C. A., and Woodman, O. L. (2015). The DPP-4 inhibitor linagliptin and the GLP-1 receptor agonist exendin-4 improve endothelium-dependent relaxation of rat mesenteric arteries in the presence of high glucose. Pharmacol. Res. 94, 26–33. doi: 10.1016/j.phrs.2015.02.003
Sheahan, K. H., Wahlberg, E. A., and Gilbert, M. P. (2020). An overview of GLP-1 agonists and recent cardiovascular outcomes trials. Postgrad. Med. J. 96, 156–161. doi: 10.1136/postgradmedj-2019-137186
Sjøberg, K. A., Holst, J. J., Rattigan, S., Richter, E. A., and Kiens, B. (2014). GLP-1 increases microvascular recruitment but not glucose uptake in human and rat skeletal muscle. Am. J. Physiol. Endocrinol. Metab. 306, E355–E362. doi: 10.1152/ajpendo.00283.2013
Sun, F., Wu, S., Guo, S., Yu, K., Yang, Z., Li, L., et al. (2015). Impact of GLP-1 receptor agonists on blood pressure, heart rate and hypertension among patients with type 2 diabetes: a systematic review and network meta-analysis. Diabetes Res. Clin. Pract. 110, 26–37. doi: 10.1016/j.diabres.2015.07.015
Vilsbøll, T., Christensen, M., Junker, A. E., Knop, F. K., and Gluud, L. L. (2012). Effects of glucagon-like peptide-1 receptor agonists on weight loss: systematic review and meta-analyses of randomised controlled trials. BMJ 344:d7771. doi: 10.1136/BMJ.D7771
Wang, B., Zhong, J., Lin, H., Zhao, Z., Yan, Z., He, H., et al. (2013). Blood pressure-lowering effects of GLP-1 receptor agonists exenatide and liraglutide: a meta-analysis of clinical trials. Diabetes Obes. Metab. 15, 737–749. doi: 10.1111/dom.12085
Wilkins, H. M., and Swerdlow, R. H. (2015). TNFα in cerebral ischemia: another stroke against you? J. Neurochem. 132, 369–372. doi: 10.1111/jnc.13028
Keywords: cerebral blood flow, healthy volunteers, glucagon-like peptide 1, exenatide, clinical trial
Citation: Ölmestig J, Marlet IR, Vilsbøll T, Rungby J, Rostrup E, Lambertsen KL and Kruuse C (2022) A single dose of exenatide had no effect on blood flow velocity in the middle cerebral artery in elderly healthy volunteers: Randomized, placebo-controlled, double-blind clinical trial. Front. Aging Neurosci. 14:899389. doi: 10.3389/fnagi.2022.899389
Received: 18 March 2022; Accepted: 04 July 2022;
Published: 25 July 2022.
Edited by:
Changhee Jung, University of Ulsan, South KoreaReviewed by:
Ewa Maria Koźniewska, Mossakowski Medical Research Centre (PAN), PolandYun Kyung Cho, Asan Medical Center, South Korea
Copyright © 2022 Ölmestig, Marlet, Vilsbøll, Rungby, Rostrup, Lambertsen and Kruuse. This is an open-access article distributed under the terms of the Creative Commons Attribution License (CC BY). The use, distribution or reproduction in other forums is permitted, provided the original author(s) and the copyright owner(s) are credited and that the original publication in this journal is cited, in accordance with accepted academic practice. No use, distribution or reproduction is permitted which does not comply with these terms.
*Correspondence: Christina Kruuse, Y2tydXVzZUBkYWRsbmV0LmRr
†These authors have contributed equally to this work and share first authorship