- 1Institute of Clinical Immunology, Faculty of Medicine, Leipzig University, Leipzig, Germany
- 2Institute of Medical Microbiology and Epidemiology of Infectious Diseases, Faculty of Medicine, Leipzig University, Leipzig, Germany
- 3Department of Medical Microbiology, College of Medicine and Health Sciences, University of Gondar, Gondar, Ethiopia
- 4Department of General and Molecular Pathophysiology, Bogomoletz Institute of Physiology, National Academy of Sciences of Ukraine, Kyiv, Ukraine
- 5IPAM Institute for Preventive and Anti-Aging Medicine, Berlin, Germany
Several studies have assessed the effects of intermittent hypoxia-normoxia training (IHNT), intermittent hypoxia-hyperoxia training (IHHT), and obstructive sleep apnea (OSA) on aging and age-related diseases in humans; however, the results remain contradictory. Therefore, this review aims to systematically summarize the available studies on the effects of IHNT, IHHT, and OSA on aging and age-related diseases. Relevant studies were searched from PubMed, Google Scholar, Cochrane Library databases, and through manual searching from reference lists of eligible studies. A total of 38 eligible studies were included in this systematic review. IHHT and IHNT provide positive effects on several age-related parameters including quality of life, cognitive and physical functions, plasma level of glucose and cholesterol/LDL, systolic blood pressure, red blood cells, and inflammation. Moreover, moderate intermittent hypoxia induces telomerase reverse transcriptase (TERT) activity and telomere stabilization, delays induction of senescence-associated markers expression and senescence-associated β-galactosidase, upregulates pluripotent marker (Oct4), activates a metabolic shift, and raises resistance to pro-apoptotic stimuli. On the contrary, intermittent hypoxia in OSA causes hypertension, metabolic syndrome, vascular function impairment, quality of life and cognitive scores reduction, advanced brain aging, increase in insulin resistance, plasma hydrogen peroxide, GSH, IL-6, hsCRP, leptin, and leukocyte telomere shortening. Thus, it can be speculated that the main factor that determines the direction of the intermittent hypoxia action is the intensity and duration of exposure. There is no direct study to prove that IHNT/IHHT actually increases life expectancy in humans. Therefore, further study is needed to investigate the actual effect of IHNT/IHHT on aging in humans.
Systematic Review Registration: www.crd.york.ac.uk/prospero, identifier CRD42022298499.
Introduction
Eukaryotic cells require sufficient concentration of oxygen (O2) to ensure survival and maintain a variety of biological activities. Due to the important roles of O2 in metabolism, respiration, and survival, eukaryotes have developed an effective and rapid O2 sensing system (Taylor, 2008). When the O2 demand surpasses its supply, O2 levels in the local tissues or whole body decrease (called hypoxia), leading to threatening physiological functions, metabolic crisis, and viability. These hypoxic responses are controlled by hypoxia-inducible factors (HIFs) (Semenza, 2000). The HIF-pathways cross talk with the AMP-activated protein kinase (AMPK), mammalian target of rapamycin complex 1 (mTORC1), nuclear factor κB (NFκB), sirtuins, and mechanistic UNC-51-like kinase 1 (ULK1) pathways in hypoxia and aging (Ruderman et al., 2010; Hong et al., 2014; Antikainen et al., 2017; Pan and Finkel, 2017).
Exposures to acute prolonged (4 h) severe hypoxia and long-term hypoxia increase oxidative stress (Joanny et al., 2001; Magalhães et al., 2004). Both duration and intensity of hypoxic exposure are known to play an important role in causing oxidative stress. It is known that a moderate amount of reactive oxygen species (ROS) is important to physiological processes leading to positive cellular adaptive responses, while large amounts of ROS can damage proteins, lipids, and DNA and result in pathophysiological responses (Hancock et al., 2001; Rains and Jain, 2011). Therefore, exposing healthy people or chronic patients to severe hypoxia could be harmful, generate high levels of ROS, and facilitate the progression of illness. On the contrary, a training program consisting of 15–24 sessions of intermittent hypoxia (10–12% O2)–normoxia (21% O2) (IHNT) and intermittent hypoxia (10–12% O2)–hyperoxia (30–40% O2) (IHHT) has been considered as an effective treatment in patients with a variety of age-related diseases (Burtscher et al., 2004; Bayer et al., 2017; Glazachev et al., 2017; Dudnik et al., 2018).
One of the key activating mechanisms for adaptive responses to intermittent hypoxia training (IHT) is the induction of ROS, which is involved in cell signaling during cell growth or tissue repair. Moreover, exposure to ROS induces the expression of certain genes, the products of which promote defense against cellular stress (Sen and Packer, 1996; Debevec et al., 2017). This effect is associated with an increase in antioxidant capacity, both enzymatic and non-enzymatic (Zepeda et al., 2013; Heß et al., 2019). The main pathways in adaptive responses are considered to be reductive stress within the mitochondria, decreased mitochondria redox potential, xanthine oxidase pathway activation, and augmented catecholamine production (Lukyanova et al., 2009; Lukyanova and Kirova, 2011). Mild hypoxic episodes exposures for short time can provide protection to specific organs, tissues, or cells against more severe hypoxia and ischemia (Debevec et al., 2015) and provide a cost-effective strategy for improving metabolic function (Mackenzie and Watt, 2016). According to some reports, IHHT enhances the ROS-induced signal without increasing hypoxia. This leads to the significant synthesis of protective intracellular protein molecules, mainly with antioxidant function (antioxidant enzymes, iron-binding proteins, and heat shock proteins) (Arkhipenko et al., 2005; Sazontova et al., 2017). The IHT also influences several underlying mechanisms of aging, such as the expression of p53 and p66 proteins that modulate inflammation and apoptosis, and tissue repair and DNA maintenance (Bianchi et al., 2006).
Human telomeres comprise a series of TTAGGG tandem repeats at the end of chromosomes, which maintains chromosomal integrity and stability and allows effective replication of DNA. Telomerase is responsible for maintaining telomere length (TL) by synthesizing TTAGGG repeats at the end (Blackburn, 2001). Some base pairs are normally lost from the terminal region of chromosomes during each cell division (Prasad et al., 2017). Therefore, TL has been used as a common biomarker of aging. In animal models, shortened or lengthened telomeres mean decreased or increased lifespan of mice, respectively (Armanios et al., 2009). Shortened telomeres may lead to the cessation of cell division, thus promoting cellular senescence and apoptosis (Prasad et al., 2017). Meanwhile, shortened telomeres are more sensitive to systematic inflammation and oxidative stress, which can further promote aging (Masi et al., 2011).
On the contrary, the mechanisms of maladaptive responses to intermittent hypoxia are mostly associated with OSA (Dempsey and Morgan, 2015; Beaudin et al., 2017). OSA is a common chronic sleep-related breathing disorder characterized by recurrent pauses in breathing, which are caused by collapsing upper airways (Lévy et al., 2015). The prevalence of OSA in the general population reaches up to 23% in women and 50% in men, and the risk of the disorder increases with higher body mass index, advancing age, and male sex (Mokros et al., 2018). OSA is commonly associated with age-related disorders such as hypertension, cardiovascular disorders, metabolic abnormalities like obesity or type 2 diabetes mellitus (Bradley and Floras, 2009; Gabryelska et al., 2021), and cancer (Hunyor and Cook, 2018). OSA causes chronic intermittent hypoxia, which is the main pathophysiological mechanism causing chronic inflammation and oxidative stress (Lévy et al., 2015), thereby leading to cellular damage and accelerated aging (López-Otín et al., 2013). Some studies showed that OSA is significantly related to shortened TL (Fyhrquist and Saijonmaa, 2012); however, Kim et al. (2010) and Polonis et al. (2017) demonstrated that TL elongated in moderate-to-severe OSA.
Several studies have reported the effects of IHNT, IHHT, and OSA on aging and age-related diseases in humans and cell culture; however, some of the findings are contradictory. Moreover, it is not well documented whether hypoxic conditioning via IHNT or IHHT may induce different physiological responses and whether one method may provide more advantages over the other. Furthermore, less is known whether acutely exposing people to severe IHNT and IHHT is associated with a potentially harmful change in their redox status. Therefore, this review aims to systematically summarize the available literature on the effects of IHNT, IHHT, and OSA on aging and age-related diseases.
Methods
Protocol Development and Review Approach
This systematic review was done based on the population, intervention, control, and outcomes (PICO) questions for interval hypoxia studies and condition-context-population (CoCoPop) review method for sleep apnea studies. PICO questions include the following: P = patients of all age groups with age-related diseases, I = IHNT and IHHT; C = patients with normoxia (21% O2) exposure; O = changes of age-related diseases condition and/or aging markers; study design = randomized controlled clinical trial, non-randomized controlled clinical trials, or non-controlled randomized clinical trial study. CoCoPop review questions include the following: Co = sleep apnea and age-related diseases, Co = sleep apnea occurs at night time in a supine position and in a state of sleep accompanied by hypercapnia, age-related diseases diagnosed by disease-specific tests, and aging measured based on aging biomarkers including telomere length and cellular senescence among other markers; Pop = patients of all age groups; study design = cohort, cross-sectional, or case-control study. Each section of the review was done and reported according to the Preferred Reporting Items for Systematic Reviews and Meta-Analyses (PRISMA) guideline using the published protocol (registration number = CRD42022298499) (Moher et al., 2009).
Data Sources and Search Strategy
Google Scholar, PubMed, and Cochrane Library electronic databases were searched for relevant studies published from August 1970 to November 2021; last search was performed on 24 November 2021. Additional studies were searched manually from the lists of references of included studies. The following search terms were used for comprehensive search of available relevant studies from the electronic databases: (A) Google Scholar search terms: allintitle: “hypoxia” OR “sleep apnea” OR “intermittent hypoxia” OR “hypoxia-normoxia” OR “interval hypoxia” OR “hypoxic-normoxic” OR “hypoxia-hyperoxia” OR “hypoxic-hyperoxic” AND “aging” OR “ageing” OR “anti-aging” OR “anti-ageing”. (B) PubMed search terms: ((((((((hypoxia[MeSH Terms]) OR sleep apnea[MeSH Terms]) OR interval hypoxia[MeSH Terms]) OR intermittent hypoxia[MeSH Terms]) OR hypoxia-normoxia[MeSH Terms]) OR hypoxic-normoxic[MeSH Terms]) OR hypoxia-hyperoxia[MeSH Terms]) OR hypoxic-hyperoxic[MeSH Terms]) AND ((((aging[MeSH Terms]) OR aging[MeSH Terms]) OR anti-aging[MeSH Terms]) OR anti-ageing[MeSH Terms]). (C) Cochrane Library: Trials matching Hypoxia in Record Title OR Sleep apnea in Record Title AND Aging in Record Title – in Trials (Word variations have been searched).
Study Selection and Eligibility Criteria
A two-step selection strategy was employed to select eligible articles after duplicate studies were excluded. In the first step, titles and abstracts of identified studies were screened for eligible original articles, which are published in English based on our inclusion and exclusion criteria. In the second step, full-text articles of the selected studies were downloaded and reviewed according to the following inclusion and exclusion criteria.
Inclusion Criteria
Studies were conducted to assess the effects of IHT (IHNT and IHHT), and for the cell culture model, continuous hypoxia training (CHT) on aging and age-related diseases was performed using the described protocol. Studies were conducted on sleep apnea (SA) such as obstructive sleep apnea (OSA), central sleep apnea (CSA), and mixed sleep apnea (MSA) as non-regulated, pathogenic hypoxia on aging and age-related diseases with the described protocol. Experimental studies were performed in human and cell line models. Studies conducted to assess the effect of IHT or SA on potential anti-aging biomarkers including telomere shortening, cellular senescence, genomic instability, epigenetic changes, oxidative stress, antioxidants, mitochondrial dysfunction, decreased autophagy, decreased proteostasis, stem cell exhaustion, altered intercellular communication, and deregulated nutrient-sensing were included in this systematic review.
Exclusion Criteria
Publications other than original article such as conference proceedings, review articles, case series, editorial, commentary, and opinion; animal studies; drug efficacy trials; articles with no full text access, and studies published in other languages than English were excluded from the review.
Data Extraction
Data were extracted using a structured data sheet in Excel. The authors’ name, publication year, country of the study, study design, interval hypoxia protocol, criteria of sleep apnea, aging biomarkers, main findings, conclusions of the authors, safety issues, and methodological quality assessments or scores among other information associated with the review question were extracted. Authors (BT and BK) independently screened the identified studies and extracted the data. The data extracted by two authors from the selected studies were compared for consistency. The discrepancies were agreed and solved after rechecking the data and through discussion on the information. Studies were grouped as IHNT, IHHT, and OSA as follows: studies with the training program consisting of 15–24 sessions of intermittent hypoxia (10–12% O2)–normoxia (21% O2) were grouped as IHNT studies, while studies with the training program consisting of 15–24 sessions of intermittent hypoxia (10–12% O2)–hyperoxia (30–40% O2) were grouped as IHHT studies. Moreover, studies that investigated the effect of chronic sleep-related breathing disorder characterized by recurrent pauses in breathing on aging markers and age-related diseases were grouped as OSA studies. The systematic review process and main findings from the comprehensive literature search are summarized in figures and tables.
Risk of Bias Assessment
Two independent reviewers (BT and BK) did the assessment of the risk of bias for the included studies. The assessment of the methodological quality for interval hypoxia training studies was performed according to the Cochrane tool for risk of bias assessment checklist. Each section was graded as high, unclear, or low: low grade indicates the highest quality (Higgins et al., 2011). Similarly, the methodological quality of sleep apnea studies was assessed based on the Newcastle Ottawa Scale (NOS) (Stang, 2010): the overall quality score was assessed out of nine points for cohort and case–control studies, while the highest overall quality score for cross-sectional studies was seven. Nine and seven cores indicate the highest quality for cohort/case-control and cross-sectional studies, respectively.
Data Analysis
In this systematic review, we summarized the data of the primary studies on hypoxia intervention groups and sleep apnea cases by using a qualitative comparison with the control groups (normoxia and individuals without SA). However, pooling of the quantitative data from the individual studies in a meta-analysis was not possible due to the methodological variations among the primary studies.
Results
Search Results
The final databases search yielded 1,964 relevant studies (790 from PubMed, 923 from Google Scholar, and 251 from Cochrane Library). Manual searching from reference lists of eligible studies also identified 33 additional articles. The majority of the identified studies were excluded from this review for the reasons indicated in Figure 1. Finally, a total of 38 studies were eligible for data extraction and included in this systematic review.
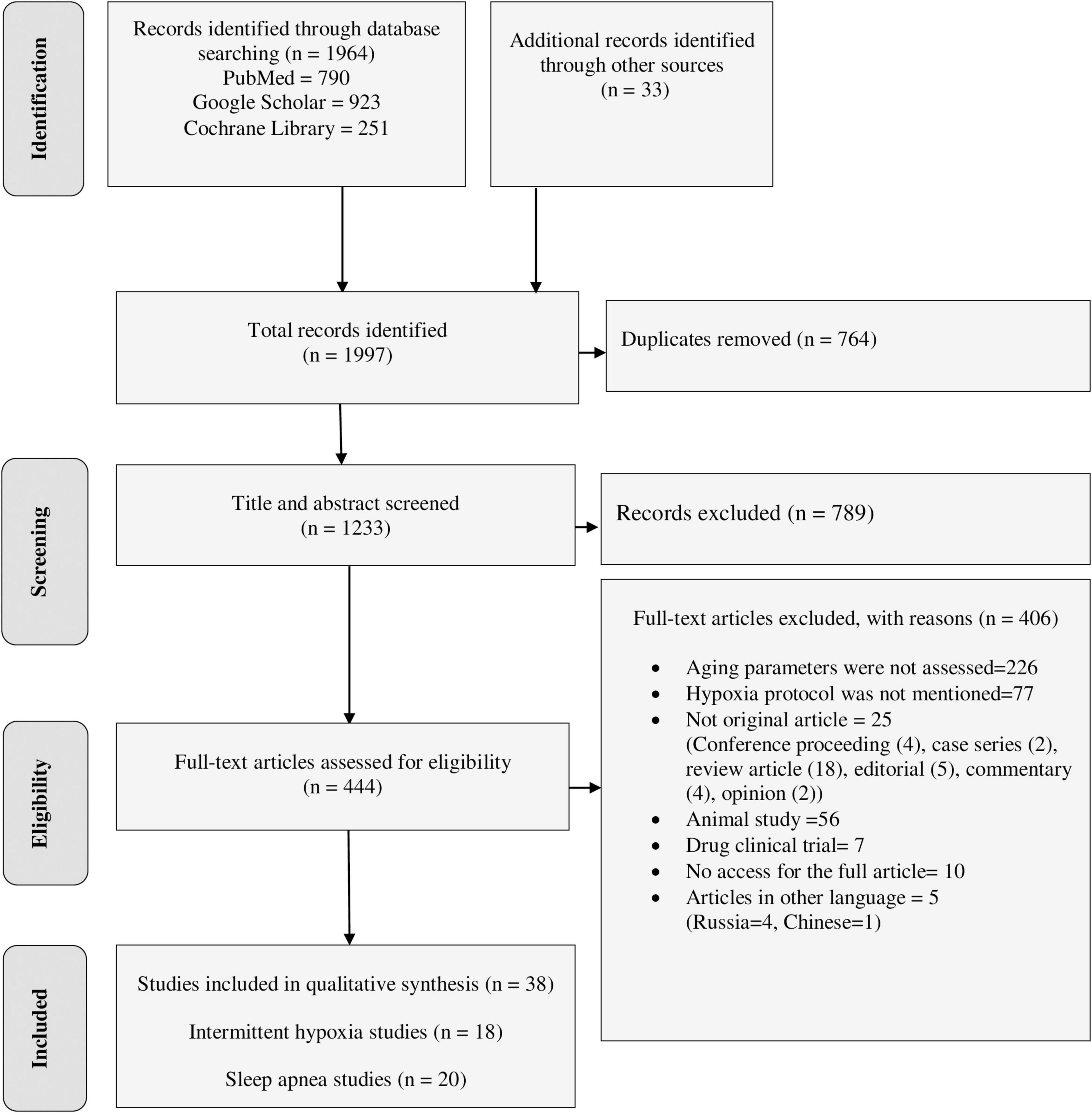
Figure 1. PRISMA flow diagram shows the searching strategy and screening of eligible studies at different levels of the review process (Moher et al., 2009).
Characteristics of Included Studies
Of the total 38 studies, 18 studies investigated the effect of IHT on aging and age-related diseases, while 20 studies reported the effect of SA on aging and age-related diseases. Of the 18 IHT studies, 13 studies were done in humans and 5 studies were conducted in cell culture model. Of the 13 studies done in humans, eight studies were conducted on IHNT, one study was conducted on both IHNT and CHT (Table 1 and Supplementary Table 1), two studies were done on IHHT, and two studies were done on both IHHT and IHNT (Table 2 and Supplementary Table 2). Of the 5 studies done in the cell culture model, three studies were done on CHT and two studies were done on IHNT (Table 3 and Supplementary Table 3). All the twenty SA studies were conducted on the effect of OSA on aging and age-related diseases (Tables 4, 5 and Supplementary Tables 4, 5).
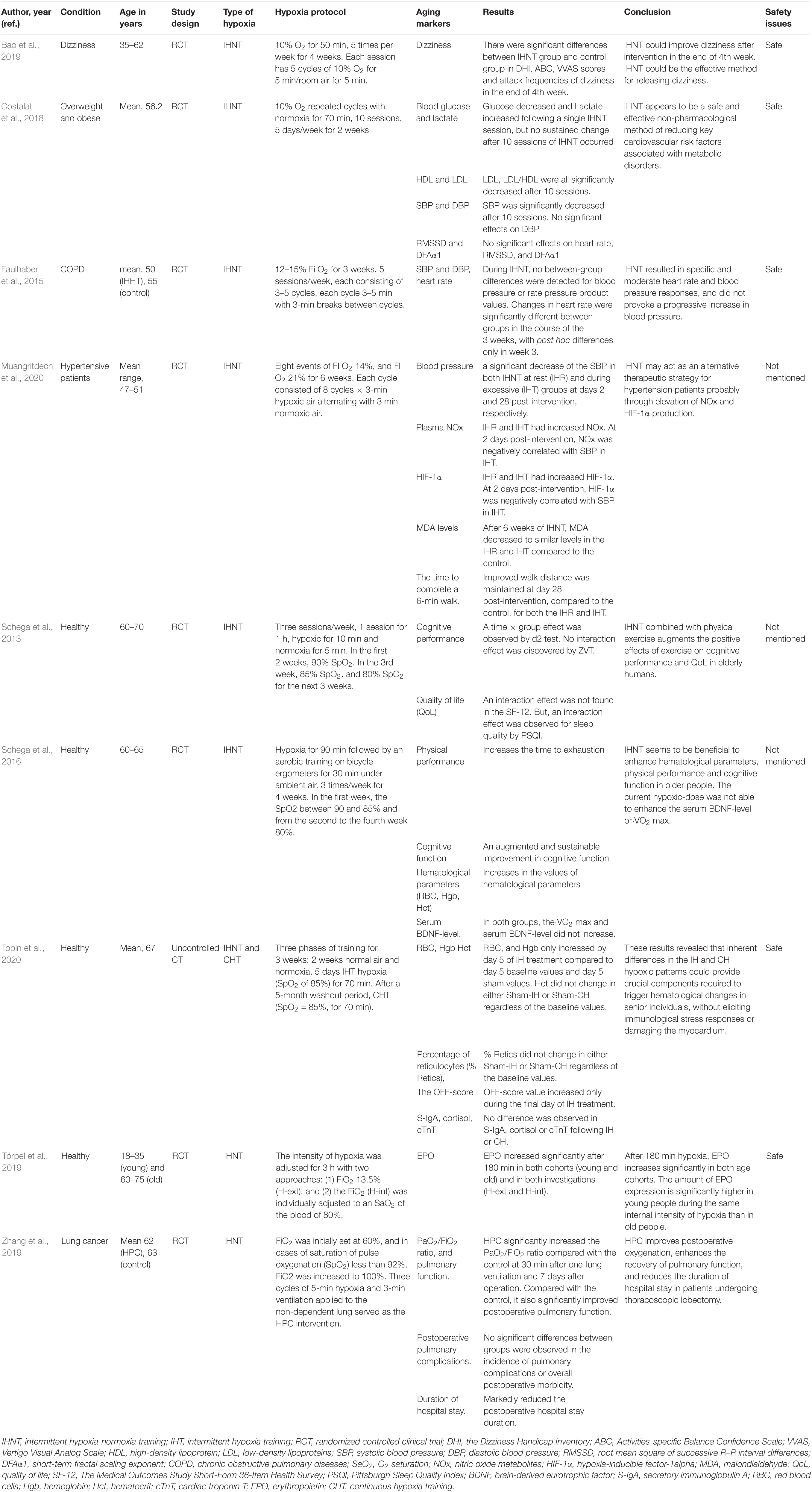
Table 1. The effects of intermittent hypoxia-normoxia training (IHNT) on aging markers and age-related diseases.
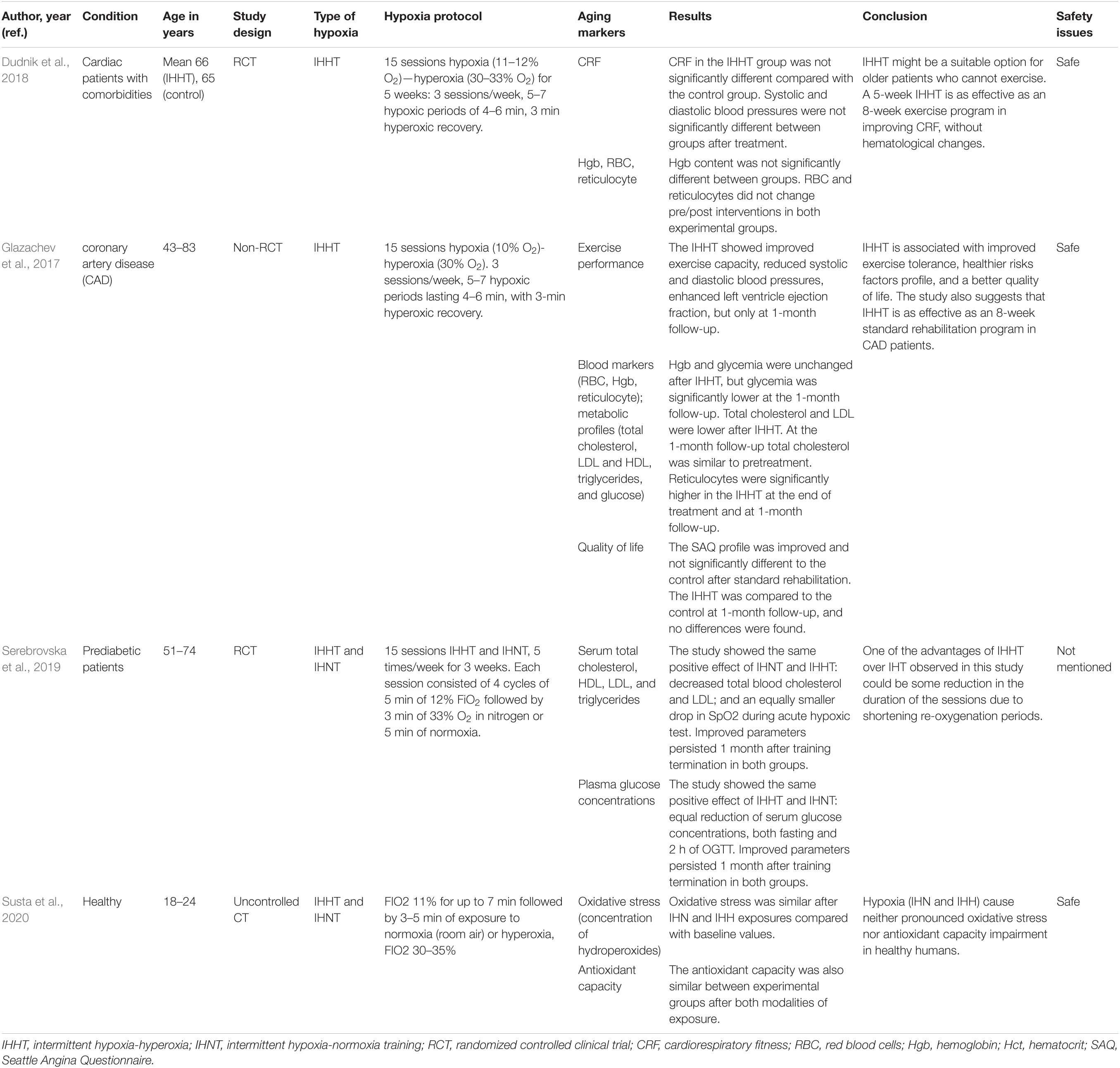
Table 2. The effects of intermittent hypoxia-hyperoxia training (IHHT) on aging markers and age-related diseases.
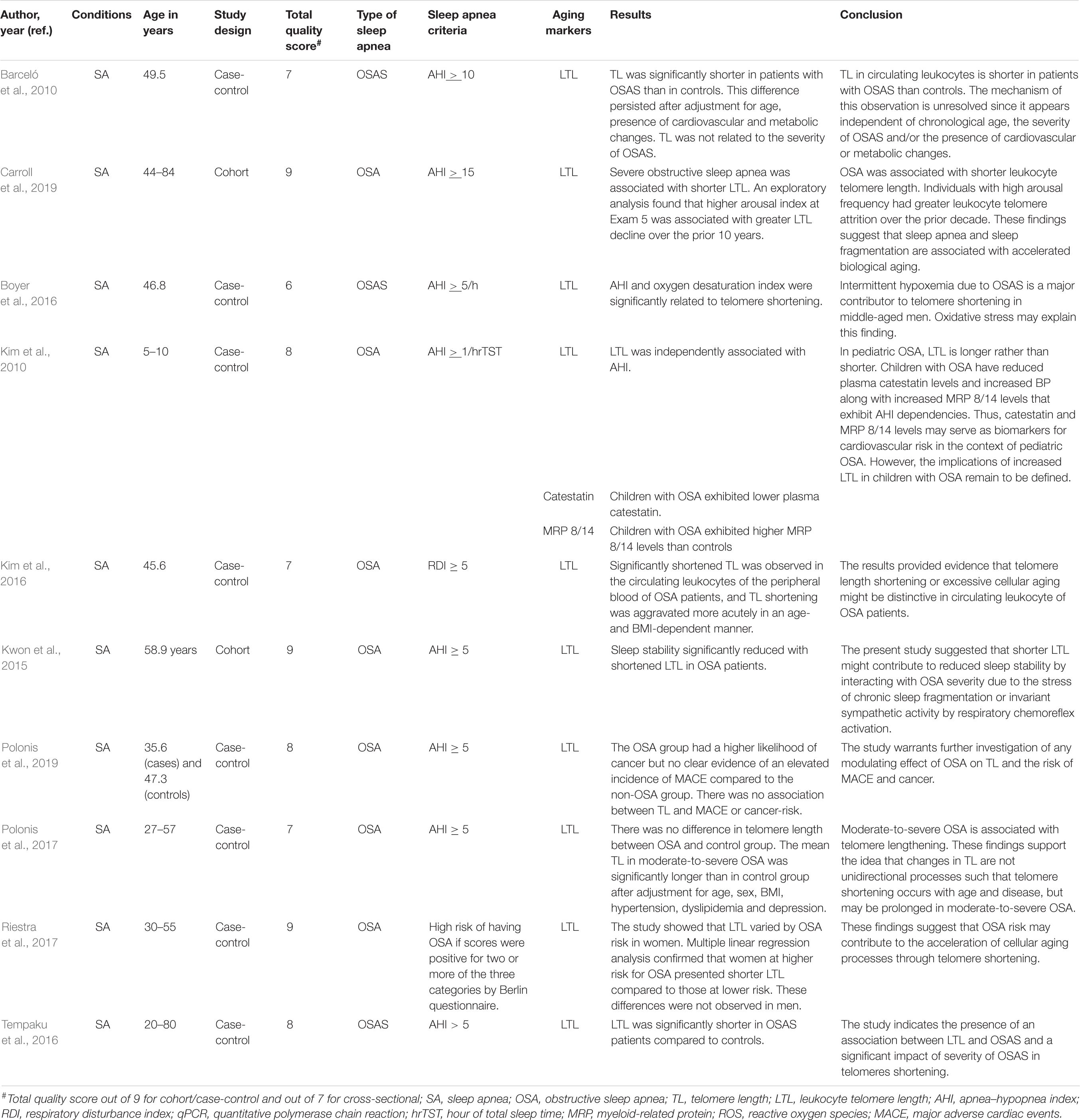
Table 5. The effects of sleep apnea on aging markers (leukocyte telomere length) and age-related diseases.
Among 18 studies on IHT, the study designs were randomized controlled clinical trial (RCT) for 10 studies, non-randomized clinical trial for 7 studies, and uncontrolled clinical trial for one study. Of the total 20 studies on OSA, 13 studies were case-control, 4 cohort, and 3 cross-sectional studies. The age groups of human study participants in the IHT studies range from 18 to 83 years (Tables 1, 2), while for SA studies the age groups of the study participants range from 5 to 93 years (Tables 4, 5).
Quality Assessment
The results of the risk of bias assessment for 18 IHT studies showed that the risk of selective reporting bias, incomplete outcome data bias (long term), and incomplete outcome data bias (short term) were low in all studies. However, one study had unclear risk of allocation concealment bias, two studies had unclear blinding of outcome assessment bias (all case mortality), two studies in human and five studies in cell culture model had unclear risks of random sequence generation bias, one study had unclear and another one study had high risk of blinding of outcome assessment bias (patient reported). Moreover, two studies had high risks of blinding of participants and personnel bias. Similarly, the results of risk of bias assessment by NOS for the 20 OSA studies showed that the overall quality scores for cohort and case-control studies ranged from 6 to 9, while the overall quality scores ranged from 6 to 7 for cross-sectional studies. Overall, 7/20 OSA studies scored the highest quality scores (Tables 4, 5).
The Effects of Intermittent Hypoxia-Normoxia Training and Intermittent Hypoxia-Hyperoxia Training on Age-Related Diseases
Randomized clinical trial studies have demonstrated the beneficial effects of IHNT and IHHT on age-related diseases including cardiovascular diseases, cognitive disorders, lung diseases, diabetes, hematological disorders, oxidative stress, inflammation, and quality of life (Tables 1, 2 and Supplementary Tables 1, 2).
Cardiovascular Diseases
A study aimed to investigate the effects of moderate IHNT on key cardio-metabolic risk factors in overweight and obese subjects showed decreased glucose and increased lactate levels following a single IHNT session, but no sustained change after 10 sessions of IHNT occurred. Conversely, LDL, LDL/HDL ratio, and SBP were all significantly decreased after 10 sessions (Costalat et al., 2018). During IHNT intervention (15 sessions), variations for heart rate and rate pressure product were significantly higher in the hypoxia group and tended to be increased for systolic blood pressure (Faulhaber et al., 2015). A study by Muangritdech et al. (2020) showed a significant decrease of the SBP in both IHNT at rest and IHNT during exercise groups at days 2 and 28 post-intervention, respectively, compared with control (Table 1). Dudnik et al. (2018) demonstrated the positive effect of IHHT on cardiorespiratory fitness compared with a standard exercise-based rehabilitation program, whereas no difference in blood pressure and in hemoglobin content was observed. In the study of Glazachev et al. (2017), IHHT also improved exercise capacity, enhanced left ventricle ejection fraction, reduced SBP and DBP, and reduced glycemia at 1-month follow-up. Angina was also significantly reduced after treatment and at 1-month follow-up (Table 2).
Cognitive Function and Physical Improvement
Bao et al. (2019) reported that dizziness scores and attack frequencies of dizziness were significantly decreased after IHNT intervention at the end of the 4th week. Moreover, Schega et al. (2013) suggested that an additional intermittent hypoxic training combined with physical exercise augments the positive effects of exercise on cognitive performance in elderly humans. IHNT also increases the values of the time to exhaustion in the load test and an augmented and sustainable improvement in cognitive function in older people (Schega et al., 2016) (Table 1).
Lung Diseases (Pulmonary Function)
A prospective randomized controlled trial study showed that hypoxic preconditioning (HPC) of patients with lung cancer undergoing elective thoracoscopic lobectomy significantly increased the PaO2/FiO2 ratio compared with patients without HPC at 30 min after one-lung ventilation and 7 days after operation. HPC also markedly decreased the duration of postoperative hospital stay and significantly improved postoperative pulmonary function (Zhang et al., 2019) (Table 1).
Hematological Parameters
Several studies reported the positive effects of IHNT and IHHT on hematological parameters. RBC, Hgb, and Hct increased after IHNT intervention (Schega et al., 2016). Similarly, RBC and Hgb increased by day 5 of IHNT treatment compared with day 5 baseline values and day 5 sham values. Likewise, Hct increased during the final day of IHNT treatment (Tobin et al., 2020). EPO increased significantly after 180 min of IHNT intervention in both old and young cohorts. EPO expression was significantly higher in young than in old people after 180 min of hypoxic exposure and 30 min afterward (Törpel et al., 2019) (Table 1).
Oxidative Stress and Inflammation
A study by Susta et al. (2020) measured oxidative stress and antioxidant capacity in healthy humans after being acutely exposed to both IHNT and IHHT. After IHNT and IHHT, neither oxidative stress nor antioxidant capacity was significantly different compared with baseline. Oxidative stress and antioxidant capacity were not significantly different between IHNT and IHHT groups. As a result, authors concluded that IHNT and IHHT cause neither antioxidant capacity impairment nor pronounced oxidative stress in healthy humans (Susta et al., 2020). An in vitro cell culture study by Tantingco and Ryou (2020) also reported that intermittent hypoxic training protects the microglia from oxygen–glucose deprivation/re-oxygenation stress. ROS in the oxygen–glucose deprivation/re-oxygenation group is increased, but intermittent hypoxic training lowers the ROS generation by oxygen–glucose deprivation/re-oxygenation. Moreover, intermittent hypoxic training regulates the polarization of the microglial phenotype toward anti-inflammatory type M2 (Tantingco and Ryou, 2020).
Quality of Life and Diabetes
The findings of the study by Schega et al. (2013) suggested that an additional intermittent hypoxic training combined with physical exercise augments the positive effects of exercise on quality of life (QoL) in elderly humans (Schega et al., 2013). Similarly, Glazachev et al. (2017) showed that quality of life, risk factors profile, and exercise tolerance were improved in the IHHT group in the same manner as identified in the standard rehabilitation group immediately after the course and at 1-month follow-up. Authors also suggested IHHT as an effective intervention as a standard rehabilitation program in coronary artery patients. A placebo-controlled clinical trial study of the effects of IHNT and IHHT interventions on metabolic variables in prediabetes patients showed the positive effects of both types of training, namely, reduction of serum glucose level, at fasting point and 2 h after glucose tolerance test, decrease of total blood cholesterol and LDL, and increase in SpO2 during acute hypoxic test. Improved parameters persisted 1 month after training termination in both groups (Serebrovska et al., 2019) (Table 2).
The Effects of Intermittent Hypoxia-Normoxia Training and Intermittent Hypoxia-Hyperoxia Training on Aging Markers
A cell culture study by Minamino et al. (2001) reported that the growth of human vascular smooth muscle cell (VSMC) could be prolonged by chronic hypoxia (1% O2) via inducing telomerase activity and telomere stabilization. Authors demonstrated hypoxia-induced sustained high levels of TERT protein expression and phosphorylation of the telomerase catalytic component compared with normoxia (Minamino et al., 2001). Another cell culture study by Casciaro et al. (2020) showed that hypoxia (1% O2) delays induction of senescence-associated markers (p16 and p21) expression and senescence-associated β-galactosidase, upregulates pluripotent marker (Oct4), activates a metabolic shift, and raises resistance to pro-apoptotic stimuli in human amniotic fluid stem cells. Similarly, Tantingco and Ryou (2020) showed that intermittent hypoxic training (3.5–4% O2) protects the microglia from oxygen–glucose deprivation/re-oxygenation stress. Hypoxia also preserves osteogenesis for extended periods of time, indicating a more youthful phenotype (Casciaro et al., 2020).
Damiani et al. (2021) demonstrated that aging markers such as β-galactosidase activity, p16 expression, and proliferation rate, as well as expression of miRNA-181a, were significantly increased in human dermal fibroblast under 21% O2 compared with those under 5% O2. Despite the positive effects on cell aging markers, incubation under 5% O2 was associated with higher levels of total GSH, mitochondrial superoxide anion, CoQ10, SOD1, and SOD3, lower levels of intracellular ROS, MMP1 expression, and DNA damage (Damiani et al., 2021). Muangritdech et al. (2020) showed that NOx and HIF-1α were upregulated after IHNT at rest and during exercise compared with the control group. The dynamics of NOx and HIF-1α were negatively associated with systolic blood pressure in IHNT during exercise 2 days post-intervention (Muangritdech et al., 2020). However, a cell culture study on human white preadipocytes (HWPs) demonstrated that chronic intermittent hypoxia at a very low oxygen concentration (0.1% O2) is related to a higher level of ROS and increased number of cells with nuclear localization of γH2AX and p16. A higher number of cells positive for senescence-associated β-galactosidase activity were also observed in chronic intermittent hypoxia exposure (Polonis et al., 2020).
In general, intermittent hypoxia of moderate level and duration provides a positive effect on several age-related parameters including quality of life, cognitive and physical functions, plasma level of glucose and cholesterol/LDL, systolic blood pressure, red blood cells, and inflammation. Moreover, moderate intermittent hypoxia induces TERT activity and telomere stabilization, delays induction of senescence-associated markers (p16 and p21) expression and senescence-associated β-galactosidase, upregulates pluripotent marker (Oct4), activates a metabolic shift, and raises resistance to pro-apoptotic stimuli (Table 3).
The Effects of Obstructive Sleep Apnea on Age-Related Diseases
Several investigators have documented the effects of OSA on age-related diseases such as cardiovascular diseases, cognitive disorders, hematological disorder, oxidative stress, and inflammation (Table 4 and Supplementary Table 4).
Cardiovascular Diseases
A study by Vgontzas et al. (2019) reported that mild-to-moderate OSA was significantly related to a higher risk of incident hypertension and metabolic syndrome in young and middle-aged persons, but not in adults older than 60 years. Similarly, Kim et al. (2010) reported that vascular function impairment and arterial stiffness were associated with OSA in younger (≤50 years), but not in older, men. In children, OSA increased BP and decreased plasma catestatin levels together with increased multidrug-resistance-associated protein 8/14 levels (Kim et al., 2010).
Cognitive Function, Physical Improvement, and Quality of Life
Addison-Brown et al. (2014) assessed cognitive function in OSA high-risk patients with standardized fluency and recall measures. Patients at high risk for OSA had significantly reduced quality of life (depressive symptoms and health-related quality of life) and decreased cognitive scores. However, some of the associations were age-dependent. During middle age, differences in quality of life and cognition between high and low OSA risk were most pronounced, with attenuated effects after 70 years of age (Addison-Brown et al., 2014). Middle-aged OSA patients showed slower reaction time during sustained attention and decreased performance for immediate word recall compared with the middle-aged control, young control, and young sleep apnea. For both functions, reduced activation was detected for middle-aged OSA cases relative to the other groups in task-related brain regions (Ayalon et al., 2010). GSH/Cr level increased during lower oxygen desaturation and more severe apnea-hypopnea index (AHI) scores. Poorer executive functioning was also correlated with GSH/Cr level (Duffy et al., 2016). Moreover, men with OSA were significantly sleepier than controls, while women with OSA showed comparable objective sleepiness with controls (Kritikou et al., 2014).
According to Sajkov et al. (1998)’s report, neuropsychological variables in patients with tetraplegia were significantly correlated with measures of sleep hypoxia, but not with the apnea-hypopnea index and the frequency of sleep arousals. The most affected neuropsychological functions by sleep hypoxia were immediate and short-term memory, cognitive flexibility, verbal attention and concentration, internal scanning, and working memory. The authors concluded that sleep apnea is common in patients with tetraplegia and may be associated with significant oxygen desaturation. The oxygen desaturation weakens daytime cognitive function in these patients, mainly memory, learning skills, attention, and concentration. Cognitive disturbances resulting from OSA might adversely affect rehabilitation in patients with tetraplegia (Sajkov et al., 1998). A cohort study by Khan et al. (2011) showed that OSA patients did not suffer from anemia of aging when living without OSA treatment, whereas symptoms started to appear 1 year after treatment. Researchers suggest that OSA inflammatory progressions are related to OSA hypoxia-induced erythropoiesis (Khan et al., 2011).
Hematological Parameters
A study by Khan et al. (2011) investigated OSA and anemia of aging (AoA) coexistence, and OSA treatment-AoA interaction. The study showed that Hct changed significantly post OSA treatment. Moreover, Hct changed significantly among both men and women. The change in Hgb after OSA treatment was not significant. However, the change in Hgb was large enough to reach WHO standards for AoA (Table 4).
Oxidative Stress, Inflammation, and Diabetics
Increased levels of GSH/Cr were associated with lower oxygen desaturation and more severe apnea-hypopnea index scores during rapid eye movement sleep. In addition, GSH/Cr correlated with poorer executive functioning (Duffy et al., 2016). Serum concentration of hydrogen peroxide was also considerably higher in OSA patients, and it was closely related to the severity of OSA (Kim et al., 2016). Men with OSA had significantly higher IL-6, hsCRP, insulin resistance, and leptin than controls, while women with OSA had significantly elevated level of hsCRP; however, TNFR-1, IL-6, insulin resistance (Homeostatic Model Assessment index), adiponectin, and leptin were similar to controls (Kritikou et al., 2014) (Table 4).
The Effects of Obstructive Sleep Apnea on Aging Markers
A number of studies have reported the effects of OSA on aging using mainly leukocyte telomere length (LTL) as a marker of aging (Table 5 and Supplementary Table 5). Barceló et al. (2010) reported that TL was significantly shorter in patients with OSA than in controls. This difference continued after adjustment for body mass index, age, glucose, cholesterol, uric acid and triglycerides levels, the presence of arterial hypertension, and smoking status. The severity of OSA was not related to LTL as assessed by nocturnal oxygen saturation, AHI, and daytime sleepiness (Barceló et al., 2010). However, severe OSA (OSA; AHI >30) was associated with shorter TL. A higher arousal index at exam 5 was correlated with higher LTL shortening over the prior 10 years (Carroll et al., 2019). TL was also independently associated with oxygen desaturation index (Boyer et al., 2016).
In OSA patients, TL of circulating leukocytes of the peripheral blood was significantly shortened, and TL shortening was aggravated more acutely in age- and BMI-dependent manner. The concentration of hydrogen peroxide and the TL of OSA patients were inversely correlated, and the severity of OSA was associated with excessive TL shortening. The findings indicate that TL shortening or extreme cellular aging might be typical in peripheral leukocytes of OSA patients. TL shortening may be a biomarker to reveal the problem of oxidative stress in the circulating blood of OSA patients (Kim et al., 2016). Increased narrow-band low-frequency coupling by relating with OSA severity showed a significant association with TL shortening. Particularly, in OSA patients, sleep stability significantly decreased with reduced TL (AHI ≥ 15) (Kwon et al., 2015). Polonis et al. (2019) reported that OSA patients had a higher likelihood of cancer but no clear evidence of an elevated incidence of major adverse cardiac events and no association with TL or cancer risk.
Telomere shortening was observed in women at higher risk for OSA compared with those at lower risk, independent of income, age, obesity, smoking, hypertension, alcohol consumption, and education. This change was not seen in men (Riestra et al., 2017). Tempaku et al. (2016) demonstrated that in OSAS patients, TL was significantly reduced compared with controls. There were negative associations between TL and desaturation index, AHI, wake after sleep onset, and respiratory disturbance index. LTL was directly related to basal minimum and maximum oxygen saturation, total sleep time, and sleep efficiency (Tempaku et al., 2016).
A J-shaped association was shown between TL and OSA severity. In moderate-to-severe OSA, the mean TL was significantly larger than that in the control group. These results strengthen the idea that variations in TL are not unidirectional processes such that TL reduction occurs with disease and age, but may be elongated in moderate-to-severe OSA (Polonis et al., 2017). In children with OSA, TL is increased rather than decreased (Kim et al., 2010) (Table 5).
A study that investigated the correlation between advanced brain aging and OSA revealed that oxygen desaturation index (ODI) and AHI were positively correlated with brain age. The correlations persisted stable in the presence of a number of confounders including diabetes, and the correlations were partially mediated by the white blood cell count, showing a subclinical inflammation process. These findings show a correlation between OSA and brain age, showing subtle but extensive age-related changes in regional brain structures (Weihs et al., 2021). Edwards et al. (2014) assessed the physiological traits responsible for OSA in a group of young and old patients with OSA to understand the pathological mechanisms underlying OSA. In comparison with younger patients with OSA, older patients with OSA had a more collapsible airway but lower Veupnea and a lower loop gain. These findings suggest that a sensitive ventilatory control system is a greater prominent trait in younger adults, while airway anatomy/collapsibility has a relatively higher pathogenic role in older adults with OSA (Edwards et al., 2014).
In general, intermittent hypoxia in OSA causes hypertension, metabolic syndrome, vascular function impairment, quality of life and cognitive scores reduction, advanced brain aging, and increase in insulin resistance, plasma hydrogen peroxide, GSH, IL-6, hsCRP, leptin, and LTL shortening.
Safety of Intermittent Hypoxia-Normoxia Training and Intermittent Hypoxia-Hyperoxia Training Protocols
Several researchers have assessed the safety of IHNT and IHHT protocols when used as an intervention for age-related diseases and during investigations of their effects on aging markers (Tables 1, 2 and Supplementary Tables 1, 2). Studies included in this review and that assessed the safety of IHNT and IHHT protocols in human have shown that both IHNT and IHHT protocols are safe interventions to use in humans (Faulhaber et al., 2015; Glazachev et al., 2017; Costalat et al., 2018; Dudnik et al., 2018; Bao et al., 2019; Törpel et al., 2019; Susta et al., 2020; Tobin et al., 2020).
Discussion
Main Findings
In this review, we have systematically summarized the available literature on the effects of IHT (IHNT and IHHT) and OSA on aging and age-related diseases according to the standard PRISMA guidelines. The IHNT and IHHT have similar effects on aging markers and age-related diseases, while OSA creates different and even opposite results compared with IHNT and IHHT. The main influencing factors in all studied groups of hypoxia are intensity and duration of hypoxic exposure. IHHT and IHNT provide 3–5 training per week with 3–5 hypoxic episodes (2–5 min of duration, SaO2 > 86–88%). OSA is diagnosed when a person regularly suffers 5–10 episodes (approximately 2 min of duration, SaO2 < 86%). Unlike IHHT and IHNT, OSA occurs at night time in a supine position and in a state of sleep accompanied by hypercapnia. Similarly, a review by Navarrete-Opazo and Mitchell (2014) showed that the adverse and beneficial effects of intermittent hypoxia depend on its dose. The severity of hypoxemia, the duration of hypoxia, the number of cycles/day, the pattern of intermittent hypoxia (consecutive days vs. alternating days), and the total protocol duration are crucial factors that determine its effects and even direction. In intermittent hypoxia with high cycle numbers and/or severe hypoxic episodes, adverse effects are more common, while in intermittent hypoxia with low cycle numbers per day and/or mild to moderate hypoxic episodes, apparently beneficial effects are more common. As the result, the finding of the review suggests that low-dose intermittent hypoxia has considerable therapeutic potential to treat multiple clinical conditions (Navarrete-Opazo and Mitchell, 2014).
The most pronounced effects of IHHT and IHNT on age-related diseases are cognitive and physical improvement (Schega et al., 2013, 2016; Glazachev et al., 2017; Bao et al., 2019), increased quality of life (Schega et al., 2013; Glazachev et al., 2017), and downregulation of glucose and cholesterol (LDL) (Glazachev et al., 2017; Costalat et al., 2018; Serebrovska et al., 2019), especially in persons with metabolic disorders. Blood pressure, especially systolic blood pressure, decrease is another positive effect of IHHT and IHNT described in several studies (Costalat et al., 2018; Muangritdech et al., 2020). While a slight increase in EPO was mentioned in only one study, no effect on circulating red blood cells (Hb and Hct) was found in several articles (Törpel et al., 2019).
Intermittent and chronic hypoxia influence aging biomarkers in vitro. Chronic exposure to 1% O2 prolongs the proliferation of human VSMC, upregulates telomerase (Minamino et al., 2001), downregulates the expression of senescence-associated markers, activates a metabolic shift, and increases resistance to pro-apoptotic stimuli (Casciaro et al., 2020). In the same manner, incubation under 5% O2 caused significant downregulation of senescent markers expression, mitochondrial superoxide anion, total GSH, CoQ10, SOD1, SOD3, and reduced levels of intracellular ROS in comparison with 21% O2. Moreover, miRNA-181a expression, which is known to be upregulated in cellular cycle arrest, was significantly increased in cells at 21% O2 compared with those at 5% O2. Increased DNA damage, upregulation of MMP1, and downregulation of COL1A1 were also observed under 21% O2 compared with cells at 5% O2 (Damiani et al., 2021). Similarly, intermittent hypoxic training (3.5–4% O2) protects the microglia from oxygen–glucose deprivation/re-oxygenation stress (Tantingco and Ryou, 2020). However, a cell culture study on HWPs demonstrated that chronic intermittent hypoxia exposure at a very low oxygen concentration (0.1% O2) is associated with an increased generation of mitochondrial ROS along with increased prevalence of cells with nuclear localization of γH2AX and p16 and a higher prevalence of cells positive for senescence-associated β-galactosidase activity (Polonis et al., 2020).
When speaking of OSA, it changes almost all parameters listed above for age-related diseases and aging markers in a negative direction. OSA is responsible for the cognitive and physical decline, even for brain damage, as well as for increased blood pressure, cholesterol/LDL, glucose, and insulin resistance, and lower health-related quality of life (Sajkov et al., 1998; Ayalon et al., 2010; Addison-Brown et al., 2014; Kritikou et al., 2014). Moreover, OSA significantly shortened LTL, common marker of aging (Barceló et al., 2010; Boyer et al., 2016; Kim et al., 2016; Tempaku et al., 2016; Riestra et al., 2017; Carroll et al., 2019), and brain aging (Weihs et al., 2021). The pleasant exceptions from negative OSA effects are the increase of Hb/Hct and prevention of age-related anemia (Khan et al., 2011), and in pediatric (age group 5–10 years) OSA cases, LTL is longer rather than shorter (Kim et al., 2010).
The explanation of dose-dependent effects of intermittent hypoxia should be found more likely in mechanisms of action. It is well known that hypoxia causes cell damage followed by inflammation and HIF-1α accumulation (Chen and Gaber, 2021; Shao et al., 2021). Inflammation is described many times as a significant pathogenic factor of OSA (Khalyfa et al., 2018; Wang et al., 2022). At the same time, intermittent hypoxia in IHHT, IHNT, and OSA circumstances is able to increase HIF-1α expression in white blood cells (Serebrovska et al., 2017; Tregub et al., 2020; Fitzpatrick et al., 2021; Nanduri et al., 2021). Thus, both mechanisms of action of hypoxia are active in intermittent regimes, and the direction and modality of effect are dependent on the level of inflammation and interaction of multiple HIF-1α targets.
The effects of intermittent hypoxia depending on the dose of hypoxia and the mechanism of actions are presented in Figure 2. Endothelial nitric oxide synthase (eNOS) is activated by HIF-1α; in the regime of IHHT and IHNT, its activity is upregulated, which causes more intensive NO production followed by vassal tonus and blood pressure decrease. At the same time, NOx is also the HIF-1α target. NOx is activated in OSA circumstances, which causes ROS production, NO inactivation, and blood pressure increase. It looks like the effect of NOx stimulation overwhelms the effect of NOS stimulation by the same transcriptional factors under more severe hypoxia. We can also speculate that HIF-1α → EPO → Hb axis is too weak in the training regime, whereas it gains strength under more severe hypoxia. It is very important to find factors that mediate the effect of intermittent hypoxia. HIFs are certainly candidates for the role of mediators, although other groups of molecules cannot be excluded. It is a fact that HIF-1a expression is upregulated in both OSA and IHT; however, it is not well known whether the oxygen-dependent pathway or hypoxic tissue destruction, accumulation of pro-inflammatory molecules, or activation of MAPK-1 pathway is the main factor in HIF-1a accumulation. The role of HIF-1a downstream is not clear either. On the one hand, the activation of glycolysis ensures the adaptation of the whole organism to hypoxic conditions; on the other hand, the accumulation of HIF-1a initiates the Warburg effect in leukocytes, increasing the rate of ATP-dependent processes tenfold. It can be speculated that exacerbation of inflammation in chronic conditions may be one of the mechanisms of the therapeutic effect of intermittent hypoxia. On the other hand, HIF-1a-dependent activation of inflammation can act destructively. It can be assumed that the direction of the effect of intermittent hypoxia depends on the balance between the metabolic and pro-inflammatory effects of HIF-1a.
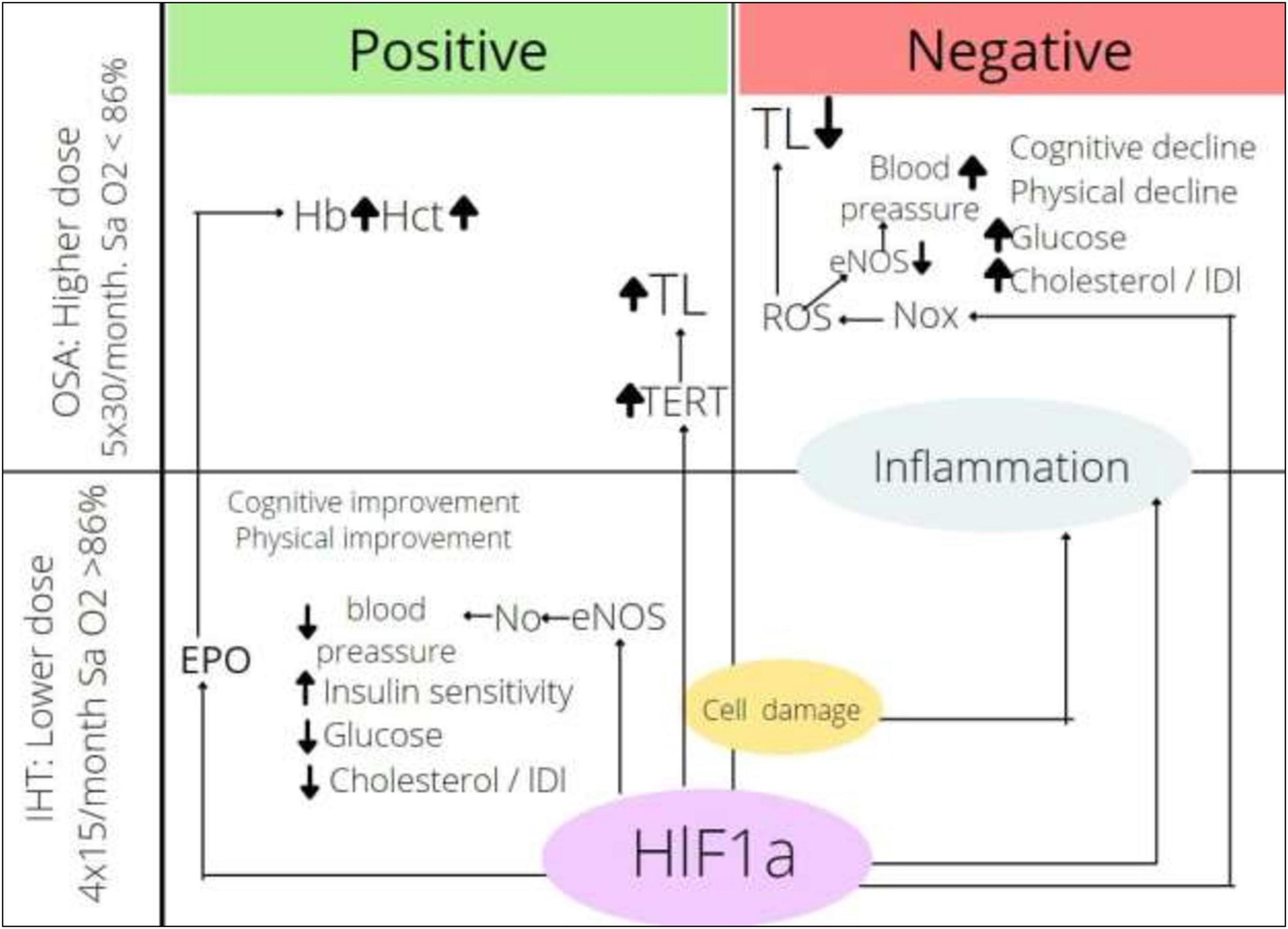
Figure 2. Effects of intermittent hypoxia depending on dose. HIF-1α, hypoxia inducible factor-1α; ROS, reactive oxygen species; Nox, nicotinamide adenine dinucleotide phosphate oxidase; eNOS, endothelial nitric oxide synthase; TERT, telomerase reverse transcriptase; TL, telomere length; EPO, erythropoietin; Hb, hemoglobin content; Hct, hematocrit; IHT, intermittent hypoxic training; OSA, obstructive sleep apnea.
It should be noted that not very much is known about the effects of intermittent hypoxia on rejuvenation markers. Nevertheless, the dose of hypoxia appears to be a key when an important aging marker, TL, is discussed. Telomeres protect chromosomes from end-to-end fusions and that is why they influence many processes in cellular metabolism. There is a discrepancy in the pull of data dedicated to TL in OSA. The majority of studies point out the decrease of TL and correlation between the severity of OSA or/and age of persons and TL (Boyer et al., 2016). Other authors stated that telomere shortening in OSA is not dependent on syndrome severity, age, or co-morbidity (Barceló et al., 2010). On the contrary, OSA in children causes rather telomere elongation than shortening (Kim et al., 2010). The implications of OSA impact on TL remain to be defined; nevertheless, some speculations can be formulated. The length of telomeres decreases in each cycle of division due to the inability of DNA polymerase to completely replicate the terminal region. Also, telomeres can be damaged independent of the cell cycle directly by oxidative stress. The length of telomeres in different tissues depends on the proliferation of this tissue, but always correlates with age (Vaiserman and Krasnienkov, 2021). The restoration of TL is provided by telomerase reverse transcriptase (TERT). There is a lot of evidence that HIF-1α is involved in the upregulation of TERT expression (Lou et al., 2007; Tsai et al., 2011; Liu et al., 2016; Mahfouz et al., 2017; Lu et al., 2021; Song et al., 2021).
Hypoxia affects TL in at least two ways: (1) It causes telomere shortening due to direct damage by oxidative stress products and (2) it increases the expression and activity of telomerase, which lead to the lengthening of telomeres. The balance between the two processes determines the result. In adults suffering from age-related pathologies, the sensitivity to oxidative stress is high, and severe hypoxic exposure to OSA causes telomere shortening. When children or younger people suffer from OSA, telomere shortening is not pronounced. High altitude hypoxia (5,000 m) causes both tissue damage due to oxidative stress and telomere elongation due to HIF-1α-TERT axis activation (Wang et al., 2018). Physical exercise, which is always accompanied by moderate intermittent hypoxia, increases TL in older persons (Sánchez-González et al., 2021; Sellami et al., 2021); it can be speculated that when intermittent hypoxia is applied in a training regime, oxidative stress is minimal; an increase in telomerase expression would lead to telomeres elongation, thereby increasing rejuvenation.
Limitations
There are some limitations in our review. First, although we observed the effects of IHNT, IHHT, and OSA on some aging markers, the strength of evidence might be weak due to insufficient data, especially in human studies. Second, variations in the study design include the use of human and cell line models; the human models include the use of different study participants such as healthy individuals or patients with different age-related diseases and different age groups; and variations in biomarkers measured hindered us to pool the data for meta-analysis and may also account for some of the conflicting results.
Conclusion
Intensive literature search was a necessary step in understanding what IHT (IHNT and IHHT) can really do for rejuvenation and in planning research. IHHT and IHNT show positive effects on age-related diseases including cognitive and physical improvement, increased quality of life, downregulation of glucose and cholesterol/LDL, decreased blood pressure, expression of senescence-associated markers; increase resistance to pro-apoptotic stimuli; prolong periods of osteogenesis; and protect the cells from oxygen–glucose deprivation/re-oxygenation stress. On the contrary, OSA causes hypertension, metabolic syndrome, vascular function impairment, quality of life and cognitive scores reduction, advanced brain aging, and increase in insulin resistance, plasma hydrogen peroxide, GSH, IL-6, hsCRP, leptin, and LTL shortening. Thus, it can be speculated that the main factors that determine the direction of the intermittent hypoxia action are intensity and duration of exposure. There is no direct study to prove that IHNT and IHHT actually increase life expectancy in humans. Therefore, further study is needed to investigate the actual effects of IHNT and IHHT on aging in humans.
Data Availability Statement
The original data presented in the study are included in the article/Supplementary Material, further inquiries can be directed to the corresponding author.
Author Contributions
BT, ZS, and EE designed the review. BT searched articles and drafted the manuscript. BT and BK selected relevant articles and extracted the data. ZS, US, BK, and EE reviewed the manuscript. All authors contributed to the article and approved the submitted version.
Funding
The article processing charge was funded by InterHypox e.V., Germany. This study received funding from CellAir Construction GmbH. The funder was not involved in the study design, collection, analysis, interpretation of data, the writing of this article or the decision to submit it for publication.
Conflict of Interest
EE is a co-owner of CellAir Construction GmbH.
The remaining authors declare that the research was conducted in the absence of any commercial or financial relationships that could be construed as a potential conflict of interest.
Publisher’s Note
All claims expressed in this article are solely those of the authors and do not necessarily represent those of their affiliated organizations, or those of the publisher, the editors and the reviewers. Any product that may be evaluated in this article, or claim that may be made by its manufacturer, is not guaranteed or endorsed by the publisher.
Supplementary Material
The Supplementary Material for this article can be found online at: https://www.frontiersin.org/articles/10.3389/fnagi.2022.878278/full#supplementary-material
References
Addison-Brown, K. J., Letter, A. J., Yaggi, K., Mcclure, L. A., Unverzagt, F. W., Howard, V. J., et al. (2014). Age differences in the association of obstructive sleep apnea risk with cognition and quality of life. J. Sleep Res. 23, 69–76. doi: 10.1111/jsr.12086
Antikainen, H., Driscoll, M., Haspel, G., and Dobrowolski, R. (2017). TOR -mediated regulation of metabolism in aging. Aging Cell. 16, 1219–1233. doi: 10.1111/acel.12689
Arkhipenko, Y. V., Sazontova, T. G., and Zhukova, A. G. (2005). Adaptation to periodic hypoxia and hyperoxia improves resistance of membrane structures in heart, liver, and brain. Bull. Exp. Biol. Med. 140, 278–281. doi: 10.1007/s10517-005-0466-0
Armanios, M., Alder, J. K., Parry, E. M., Karim, B., Strong, M. A., and Greider, C. W. (2009). Short telomeres are sufficient to cause the degenerative defects associated with aging. Am. J. Hum. Genet. 85, 823–832. doi: 10.1016/j.ajhg.2009.10.028
Ayalon, L., Ancoli-Israel, S., and Drummond, S. P. A. (2010). Obstructive sleep apnea and age: a double insult to brain function? Am. J. Respir Crit. Care Med. 182, 413–419. doi: 10.1164/rccm.200912-1805OC
Bao, X., Tan, J. W., Long, Y., Liu, H., and Liu, H. Y. (2019). Effect of intermittent hypoxia training for dizziness: a randomized controlled trial. J. Sport Rehabil. 28, 540–543. doi: 10.1123/jsr.2017-0341
Barceló, A., Piérola, J., López-Escribano, H., de la Peña, M., Soriano, J. B., Alonso-Fernández, A., et al. (2010). Telomere shortening in sleep apnea syndrome. Respir Med. 104, 1225–1229. doi: 10.1016/j.rmed.2010.03.025
Bayer, U., Likar, R., Pinter, G., Stettner, H., Demschar, S., Trummer, B., et al. (2017). Intermittent hypoxic-hyperoxic training on cognitive performance in geriatric patients. Alzheimer’s Dement Trans. Res. Clin. Int. 3, 114–122.
Beaudin, A. E., Waltz, X., Hanly, P. J., and Poulin, M. J. (2017). Impact of obstructive sleep apnoea and intermittent hypoxia on cardiovascular and cerebrovascular regulation. Exp. Physiol. 102, 743–763. doi: 10.1113/EP086051
Bianchi, G., Di Giulio, C., Rapino, C., Rapino, M., Antonucci, A., and Cataldi, A. (2006). p53 and p66 proteins compete for hypoxia-inducible factor 1 alpha stabilization in young and old rat hearts exposed to intermittent hypoxia. Gerontology 52, 17–23. doi: 10.1159/000089821
Blackburn, E. H. (2001). Switching and signaling at the telomere. Cell 106, 661–673. doi: 10.1016/s0092-8674(01)00492-5
Boyer, L., Audureau, E., Margarit, L., Marcos, E., Bizard, E., Le Corvoisier, P., et al. (2016). Telomere shortening in middle-aged men with sleep-disordered breathing. Ann. Am. Thorac. Soc. 13, 1136–1143. doi: 10.1513/AnnalsATS.201510-718OC
Bradley, T. D., and Floras, J. S. (2009). Obstructive sleep apnoea and its cardiovascular consequences. Lancet 373, 82–93.
Burtscher, M., Pachinger, O., Ehrenbourg, I., Mitterbauer, G., Faulhaber, M., Pühringer, R., et al. (2004). Intermittent hypoxia increases exercise tolerance in elderly men with and without coronary artery disease. Int. J. Cardiol. 96, 247–254. doi: 10.1016/j.ijcard.2003.07.021
Carroll, J. E., Irwin, M. R., Seeman, T. E., Diez-Roux, A. V., Prather, A. A., Olmstead, R., et al. (2019). Obstructive sleep apnea, nighttime arousals, and leukocyte telomere length: the multi-ethnic study of atherosclerosis. Sleep 42:546. doi: 10.1093/sleep/zsz089
Casciaro, F., Borghesan, M., and Beretti, F. (2020). MZ- of A and, 2020 U. Prolonged Hypoxia Delays Aging And Preserves Functionality Of Human Amniotic Fluid Stem Cells. Elsevier. Available online at: https://www.sciencedirect.com/science/article/pii/S004763742030124X (accessed September 19, 2021)
Chen, Y., and Gaber, T. (2021). Hypoxia/HIF modulates immune responses. Biomedicines 9:260. doi: 10.3390/biomedicines9030260
Costalat, G., Lemaitre, F., Tobin, B., and Renshaw, G. (2018). Intermittent hypoxia revisited: a promising non-pharmaceutical strategy to reduce cardio-metabolic risk factors? Sleep Breath 22, 267–271. doi: 10.1007/s11325-017-1459-8
Damiani, E., Brugè, F., and Cirilli, I. (2021). FM-…medicine and cellular, 2018 U. Modulation of oxidative status by normoxia and hypoxia on cultures of human dermal fibroblasts: how does it affect cell aging?. Available online at: https://www.hindawi.com/journals/omcl/2018/5469159/ (accessed May 7, 2021)
Debevec, T., Millet, G. P., and Pialoux, V. (2017). Hypoxia-induced oxidative stress modulation with physical activity. Front. Physiol. 8:84. doi: 10.3389/fphys.2017.00084/full
Debevec, T., Pialoux, V., Saugy, J., Schmitt, L., Cejuela, R., Mury, P., et al. (2015). Prooxidant/antioxidant balance in hypoxia: a cross-over study on normobaric vs. hypobaric “live high-train low. PLoS One 10:e0137957. doi: 10.1371/journal.pone.0137957
Dempsey, J. A., and Morgan, B. J. (2015). Humans in hypoxia: a conspiracy of maladaptation? Physiology 30, 304–316. doi: 10.1152/physiol.00007.2015
Dudnik, E., Zagaynaya, E., Glazachev, O. S., and Susta, D. (2018). Intermittent hypoxia–hyperoxia conditioning improves cardiorespiratory fitness in older comorbid cardiac outpatients without hematological changes: a randomized controlled trial. High Alt. Med. Biol. 19, 339–343. doi: 10.1089/ham.2018.0014
Duffy, S. L., Lagopoulos, J., Terpening, Z., Lewis, S. J. G., Grunstein, R., Mowszowski, L., et al. (2016). Association of anterior cingulate glutathione with sleep apnea in older adults at-risk for dementia. Sleep. 39, 899–906. doi: 10.5665/sleep.5650
Edwards, B. A., Wellman, A., Sands, S. A., Owens, R. L., Eckert, D. J., White, D. P., et al. (2014). Obstructive sleep apnea in older adults is a distinctly different physiological phenotype. Sleep 37, 1227–1236.
Faulhaber, M., Gatterer, H., Haider, T., Linser, T., Netzer, N., and Burtscher, M. (2015). Heart rate and blood pressure responses during hypoxic cycles of a 3-week intermittent hypoxia breathing program in patients at risk for or with;mild COPD. Int. J. Chron. Obstr. Pulmon Dis. 2015:339. doi: 10.2147/COPD.S75749
Fitzpatrick, S. F., King, A. D., O’Donnell, C., Roche, H. M., and Ryan, S. (2021). Mechanisms of intermittent hypoxia-mediated macrophage activation – potential therapeutic targets for obstructive sleep apnoea. J. Sleep Res. 30:13202. doi: 10.1111/jsr.13202
Fyhrquist, F., and Saijonmaa, O. (2012). Telomere length and cardiovascular aging. Ann. Med. 44, S138–S142. doi: 10.3109/07853890.2012.660497
Gabryelska, A., Chrzanowski, J., Sochal, M., Kaczmarski, P., Turkiewicz, S., Ditmer, M., et al. (2021). Nocturnal oxygen saturation parameters as independent risk factors for type 2 diabetes mellitus among obstructive sleep apnea patients. J. Clin. Med. 10:3770. doi: 10.3390/jcm10173770
Glazachev, O., Kopylov, P., Susta, D., Dudnik, E., and Zagaynaya, E. (2017). Adaptations following an intermittent hypoxia-hyperoxia training in coronary artery disease patients: a controlled study. Clin. Cardiol. 40, 370–376. doi: 10.1002/clc.22670
Hancock, J. T., Desikan, R., and Neill, S. J. (2001). Role of reactive oxygen species in cell signalling pathways. Biochem. Soc. Trans. 29:345. doi: 10.1042/0300-5127:0290345
Heß, V., Kasim, M., Mathia, S., Persson, P. B., Rosenberger, C., and Fähling, M. (2019). Episodic hypoxia promotes defence against cellular stress. Cell Physiol. Biochem. 52, 1075–1091. doi: 10.33594/000000073
Higgins, J. P. T., Altman, D. G., Gotzsche, P. C., Juni, P., Moher, D., Oxman, A. D., et al. (2011). The cochrane collaboration’s tool for assessing risk of bias in randomised trials. BMJ 343:d5928.
Hong, S., Zhao, B., Lombard, D. B., Fingar, D. C., and Inoki, K. (2014). Cross-talk between Sirtuin and mammalian target of rapamycin complex 1 (mTORC1) signaling in the regulation of S6 kinase 1 (S6K1) phosphorylation. J. Biol. Chem. 289, 13132–13141. doi: 10.1074/jbc.M113.520734
Hunyor, I., and Cook, K. M. (2018). Models of intermittent hypoxia and obstructive sleep apnea: molecular pathways and their contribution to cancer. Am. J. Physiol. Integr. Comput. Phys. 315, R669–R687. doi: 10.1152/ajpregu.00036.2018
Joanny, P., Steinberg, J., Robach, P., Richalet, J. P., Gortan, C., Gardette, B., et al. (2001). Operation everest III (Comex’97): the effect of simulated severe hypobaric hypoxia on lipid peroxidation and antioxidant defence systems in human blood at rest and after maximal exercise. Resuscitation 49, 307–314. doi: 10.1016/s0300-9572(00)00373-7
Khalyfa, A., Kheirandish-Gozal, L., and Gozal, D. (2018). Exosome and macrophage crosstalk in sleep-disordered breathing-induced metabolic dysfunction. Int. J. Mol. Sci. 19:3383. doi: 10.3390/ijms19113383
Khan, A. M., Ashizawa, S., Hlebowicz, V., and Appel, D. W. (2011). Anemia of aging and obstructive sleep apnea. Sleep Breath 15, 29–34. doi: 10.1007/s11325-010-0326-7
Kim, J., Lee, S., Bhattacharjee, R., Khalyfa, A., Kheirandish-Gozal, L., and Gozal, D. (2010). Leukocyte telomere length and plasma catestatin and myeloid-related protein 8/14 concentrations in children with obstructive sleep apnea. Chest 138, 91–99. doi: 10.1378/chest.09-2832
Kim, K. S., Kwak, J. W., Lim, S. J., Park, Y. K., Yang, H. S., and Kim, H. J. (2016). Oxidative stress-induced telomere length shortening of circulating leukocyte in patients with obstructive sleep apnea. Aging Dis. 7, 604–613. doi: 10.14336/AD.2016.0215
Kritikou, I., Basta, M., Vgontzas, A. N., Pejovic, S., Liao, D., Tsaoussoglou, M., et al. (2014). Sleep apnoea, sleepiness, inflammation and insulin resistance in middle-aged males and females. Eur. Respir J. 43, 145–155. doi: 10.1183/09031936.00126712
Kwon, A. M., Baik, I., Thomas, R. J., and Shin, C. (2015). The association between leukocyte telomere lengths and sleep instability based on cardiopulmonary coupling analysis. Sleep Breath 19, 963–968. doi: 10.1007/s11325-014-1110-x
Lévy, P., Kohler, M., McNicholas, W. T., Barbé, F., McEvoy, R. D., Somers, V. K., et al. (2015). Obstructive sleep apnoea syndrome. Nat. Rev. Dis. Prim 1:15015.
Liu, Q., Sun, Y., Lv, Y., Le, Z., Xin, Y., Zhang, P., et al. (2016). TERT alleviates irradiation-induced late rectal injury by reducing hypoxia-induced ROS levels through the activation of NF-κB and autophagy. Int. J. Mol. Med. 38, 785–793. doi: 10.3892/ijmm.2016.2673
López-Otín, C., Blasco, M. A., Partridge, L., Serrano, M., and Kroemer, G. (2013). The hallmarks of aging. Cell 153, 1194–1217.
Lou, F., Chen, X., Jalink, M., Zhu, Q., Ge, N., Zhao, S., et al. (2007). The opposing effect of hypoxia-inducible factor-2α on expression of telomerase reverse transcriptase. Mol. Cancer Res. 5, 793–800. doi: 10.1158/1541-7786.MCR-07-0065
Lu, H., Lyu, Y., Tran, L., Lan, J., Xie, Y., Yang, Y., et al. (2021). HIF-1 recruits NANOG as a coactivator for TERT gene transcription in hypoxic breast cancer stem cells. Cell Rep. 36:109757. doi: 10.1016/j.celrep.2021.109757
Lukyanova, L. D., and Kirova, Y. I. (2011). Effect of hypoxic preconditioning on free radical processes in tissues of rats with different resistance to hypoxia. Bull. Exp. Biol. Med. 151, 292–296. doi: 10.1007/s10517-011-1312-1
Lukyanova, L. D., Germanova, E. L., and Kopaladze, R. A. (2009). Development of resistance of an organism under various conditions of hypoxic preconditioning: role of the hypoxic period and reoxygenation. Bull. Exp. Biol. Med. 147, 400–404. doi: 10.1007/s10517-009-0529-8
Mackenzie, R. W. A., and Watt, P. A. (2016). Molecular and whole body insight of the mechanisms surrounding glucose disposal and insulin resistance with hypoxic treatment in skeletal muscle. J. Diabetes Res. 2016, 1–10. doi: 10.1155/2016/6934937
Magalhães, J., Ascensão, A., Viscor, G., Soares, J., Oliveira, J., Marques, F., et al. (2004). Oxidative stress in humans during and after 4 hours of hypoxia at a simulated altitude of 5500 m. Aviat Space Environ. Med. 75, 16–22.
Mahfouz, N., Tahtouh, R., Alaaeddine, N., El Hajj, J., Sarkis, R., Hachem, R., et al. (2017). Gastrointestinal cancer cells treatment with bevacizumab activates a VEGF autoregulatory mechanism involving telomerase catalytic subunit hTERT via PI3K-AKT, HIF-1α and VEGF receptors. PLoS One 12:e0179202. doi: 10.1371/journal.pone.0179202
Masi, S., Salpea, K. D., Li, K., Parkar, M., Nibali, L., Donos, N., et al. (2011). Oxidative stress, chronic inflammation, and telomere length in patients with periodontitis. Free Radic Biol. Med. 50, 730–735. doi: 10.1016/j.freeradbiomed.2010.12.031
Minamino, T., Mitsialis, S. A., and Kourembanas, S. (2001). Hypoxia extends the life span of vascular smooth muscle cells through telomerase activation. Mol. Cell Biol. 21, 3336–3342. doi: 10.1128/MCB.21.10.3336-3342.2001
Moher, D., Liberati, A., Tetzlaff, J., and Altman, D. G. (2009). Preferred reporting items for systematic reviews and meta-analyses: the PRISMA statement. BMJ 339:b2535.
Mokros, Ł, Kuczynski, W., Gabryelska, A., Franczak, Ł, Spałka, J., and Białasiewicz, P. (2018). High negative predictive value of normal body mass index for obstructive sleep apnea in the lateral sleeping position. J. Clin. Sleep Med. 14, 985–990. doi: 10.5664/jcsm.7166
Muangritdech, N., Hamlin, M. J., Sawanyawisuth, K., Prajumwongs, P., Saengjan, W., Wonnabussapawich, P., et al. (2020). Hypoxic training improves blood pressure, nitric oxide and hypoxia-inducible factor-1 alpha in hypertensive patients. Eur. J. Appl. Phys. 120, 1815–1826. doi: 10.1007/s00421-020-04410-9
Nanduri, J., Wang, N., Wang, B. L., and Prabhakar, N. R. (2021). Lysine demethylase KDM6B regulates HIF-1α-mediated systemic and cellular responses to intermittent hypoxia. Phys. Geno. 53, 385–394. doi: 10.1152/physiolgenomics.00045.2021
Navarrete-Opazo, A., and Mitchell, G. S. (2014). Therapeutic potential of intermittent hypoxia: a matter of dose. Am. J. Phys. Int. Comput. Phys. 307, R1181–R1197. doi: 10.1152/ajpregu.00208.2014
Pan, H., and Finkel, T. (2017). Key proteins and pathways that regulate lifespan. J. Biol. Chem. 292, 6452–6460. doi: 10.1074/jbc.R116.771915
Polonis, K., Becari, C., Chahal, C. A. A., Zhang, Y., Allen, A. M., Kellogg, T. A., et al. (2020). Chronic intermittent hypoxia triggers a senescence-like phenotype in human white preadipocytes. Sci. Rep. 10, 1–14. doi: 10.1038/s41598-020-63761-7
Polonis, K., Somers, V. K., Becari, C., Covassin, N., Schulte, P. J., Druliner, B. R., et al. (2017). Moderate-to-severe obstructive sleep apnea is associated with telomere lengthening. Am. J. Phys. Circ. Physiol 313, H1022–H1030. doi: 10.1152/ajpheart.00197.2017
Polonis, K., Sompalli, S., Becari, C., Xie, J., Covassin, N., Schulte, P. J., et al. (2019). Telomere length and risk of major adverse cardiac events and cancer in obstructive sleep apnea patients. Cells. 8:381.
Prasad, K. N., Wu, M., and Bondy, S. C. (2017). Telomere shortening during aging: attenuation by antioxidants and anti-inflammatory agents. Mech Ageing Dev. 164, 61–66. doi: 10.1016/j.mad.2017.04.004
Rains, J. L., and Jain, S. K. (2011). Oxidative stress, insulin signaling, and diabetes. Free Radic. Biol. Med. 50, 567–575.
Riestra, P., Gebreab, S. Y., Xu, R., Khan, R. J., Quarels, R., Gibbons, G., et al. (2017). Obstructive sleep apnea risk and leukocyte telomere length in african americans from the MH-GRID study. Sleep Breath 21, 751–757. doi: 10.1007/s11325-016-1451-8
Ruderman, N. B., Julia, X., Nelson, L., Cacicedo, J. M., Saha, A. K., Lan, F., et al. (2010). AMPK and SIRT1: a long-standing partnership? Am. J. Physiol. Metab. 298, E751–E760. doi: 10.1152/ajpendo.00745.2009
Sajkov, D., Marshall, R., Walker, P., Mykytyn, I., McEvoy, R. D., Wale, J., et al. (1998). Sleep apnoea related hypoxia is associated with cognitive disturbances in patients with tetraplegia. Spinal Cord 36, 231–239. doi: 10.1038/sj.sc.3100563
Sánchez-González, J. L., Sánchez-Rodríguez, J. L., Martín-Vallejo, J., Martel-Martel, A., and González-Sarmiento, R. (2021). Effects of physical exercise on cognition and telomere length in healthy older women. Brain Sci. 11:1417. doi: 10.3390/brainsci11111417
Sazontova, T. G., Stryapko, N. V., and Arkhipenko, Y. V. (2017). Correction of abnormalities provoked by long-term alcoholization with hypoxic-hyperoxic training. Bull. Exp. Biol. Med. 162, 624–628. doi: 10.1007/s10517-017-3672-7
Schega, L., Peter, B., Brigadski, T., Leßmann, V., Isermann, B., Hamacher, D., et al. (2016). Effect of intermittent normobaric hypoxia on aerobic capacity and cognitive function in older people. J. Sci. Med. Sport 19, 941–945. doi: 10.1016/j.jsams.2016.02.012
Schega, L., Peter, B., Törpel, A., Mutschler, H., Isermann, B., and Hamacher, D. (2013). Effects of intermittent hypoxia on cognitive performance and quality of life in elderly adults: a pilot study. Gerontology 59, 316–323. doi: 10.1159/000350927
Sellami, M., Bragazzi, N., Prince, M. S., Denham, J., and Elrayess, M. (2021). Regular, intense exercise training as a healthy aging lifestyle strategy: preventing DNA damage, telomere shortening and adverse DNA methylation changes over a lifetime. Front. Genet. 12:652497. doi: 10.3389/fgene.2021.652497/full
Semenza, G. L. (2000). HIF-1: mediator of physiological and pathophysiological responses to hypoxia. J. Appl. Physiol. 88, 1474–1480. doi: 10.1152/jappl.2000.88.4.1474
Sen, C. K., and Packer, L. (1996). Antioxidant and redox regulation of gene transcription. FASEB J. 10, 709–720. doi: 10.1096/fasebj.10.7.8635688
Serebrovska, T. V., Grib, O. N., Portnichenko, V. I., Serebrovska, Z. O., Egorov, E., and Shatylo, V. B. (2019). Intermittent hypoxia/hyperoxia versus intermittent hypoxia/normoxia: comparative study in prediabetes. High Alt. Med. Biol. 20, 383–391. doi: 10.1089/ham.2019.0053
Serebrovska, T. V., Portnychenko, A. G., Drevytska, T. I., Portnichenko, V. I., Xi, L., Egorov, E., et al. (2017). Intermittent hypoxia training in prediabetes patients: beneficial effects on glucose homeostasis, hypoxia tolerance and gene expression. Exp. Biol. Med. 242, 1542–1552. doi: 10.1177/1535370217723578
Shao, R., Sun, D., Hu, Y., and Cui, D. (2021). White matter injury in the neonatal hypoxic-ischemic brain and potential therapies targeting microglia. J. Neurosci. Res. 99, 991–1008. doi: 10.1002/jnr.24761
Song, H., Chen, X., Jiao, Q., Qiu, Z., Shen, C., Zhang, G., et al. (2021). HIF-1α-mediated telomerase reverse transcriptase activation inducing autophagy through mammalian target of rapamycin promotes papillary thyroid carcinoma progression during hypoxia stress. Thyroid 31, 233–246. doi: 10.1089/thy.2020.0023
Stang, A. (2010). Critical evaluation of the newcastle-ottawa scale for the assessment of the quality of nonrandomized studies in meta-analyses. Eur. J. Epidemiol. 25, 603–605. doi: 10.1007/s10654-010-9491-z
Susta, D., Glazachev, O. S., Zapara, M. A., Dudnik, E. N., and Samartseva, V. G. (2020). Redox homeostasis in humans exposed to intermittent hypoxia–normoxia and to intermittent hypoxia–hyperoxia. High Alt. Med. Biol. 21, 45–51. doi: 10.1089/ham.2019.0059
Tantingco, G., and Ryou, M. G. (2020). Normobaric intermittent hypoxic training regulates microglia phenotype and enhances phagocytic activity. Exp. Biol. Med. 245, 740–747. doi: 10.1177/1535370220919361
Taylor, C. T. (2008). Mitochondria and cellular oxygen sensing in the HIF pathway. Biochem. J. 409, 19–26. doi: 10.1042/BJ20071249
Tempaku, P. F., Mazzotti, D. R., Hirotsu, C., Andersen, M. L., Xavier, G., Maurya, P. K., et al. (2016). The effect of the severity of obstructive sleep apnea syndrome on telomere length. Oncotarget 7, 69216–69224. doi: 10.18632/oncotarget.12293
Tobin, B., Costalat, G., and Renshaw, G. M. C. (2020). Intermittent not continuous hypoxia provoked haematological adaptations in healthy seniors: hypoxic pattern may hold the key. Eur. J. Appl. Phys. 120, 707–718. doi: 10.1007/s00421-020-04310-y
Törpel, A., Peter, B., Hamacher, D., and Schega, L. (2019). Dose–response relationship of intermittent normobaric hypoxia to stimulate erythropoietin in the context of health promotion in young and old people. Eur. J. Appl. Physiol. 119, 1065–1074. doi: 10.1007/s00421-019-04096-8
Tregub, P. P., Malinovskaya, N. A., Morgun, A. V., Osipova, E. D., Kulikov, V. P., Kuzovkov, D. A., et al. (2020). Hypercapnia potentiates HIF-1α activation in the brain of rats exposed to intermittent hypoxia. Res. Phys. Neurobiol. 278:103442. doi: 10.1016/j.resp.2020.103442
Tsai, C.-C., Chen, Y.-J., Yew, T.-L., Chen, L.-L., Wang, J.-Y., Chiu, C.-H., et al. (2011). Hypoxia inhibits senescence and maintains mesenchymal stem cell properties through down-regulation of E2A-p21 by HIF-TWIST. Blood 117, 459–469. doi: 10.1182/blood-2010-05-287508
Vaiserman, A., and Krasnienkov, D. (2021). Telomere length as a marker of biological age: state-of-the-art, open issues, and future perspectives. Front. Genet. 11:630183. doi: 10.3389/fgene.2020.630186/full
Vgontzas, A. N., Li, Y., He, F., Fernandez-Mendoza, J., Gaines, J., Liao, D., et al. (2019). Mild-to-moderate sleep apnea is associated with incident hypertension: age effect. Sleep 42:884. doi: 10.1093/sleep/zsy265
Wang, R., Mihaicuta, S., Tiotiu, A., Corlateanu, A., Ioan, I. C., and Bikov, A. (2022). Asthma and obstructive sleep apnoea in adults and children – an up-to-date review. Sleep Med. Rev. 61:101564. doi: 10.1016/j.smrv.2021.101564
Wang, Y., Zhao, Z., Zhu, Z., Li, P., Li, X., Xue, X., et al. (2018). Telomere elongation protects heart and lung tissue cells from fatal damage in rats exposed to severe hypoxia. J. Physiol. Anthr. 37:5. doi: 10.1186/s40101-018-0165-y
Weihs, A., Frenzel, S., Wittfeld, K., Obst, A., Stubbe, B., Habes, M., et al. (2021). Associations between sleep apnea and advanced brain aging in a large-scale population study. Sleep 44:17994. doi: 10.1093/sleep/zsaa204
Yim-Yeh, S., Rahangdale, S., Nguyen, A. T. D., Jordan, A. S., Novack, V., Veves, A., et al. (2010). Obstructive sleep apnea and aging effects on macrovascular and microcirculatory function. Sleep 33, 1177–1183. doi: 10.1093/sleep/33.9.1177
Zepeda, A. B., Pessoa, A., Castillo, R. L., Figueroa, C. A., Pulgar, V. M., and Farías, J. G. (2013). Cellular and molecular mechanisms in the hypoxic tissue: role of HIF-1 and ROS. Cell Biochem. Funct. 31, 451–459. doi: 10.1002/cbf.2985
Keywords: IHNT, IHHT, OSA, aging biomarkers, age-related diseases
Citation: Tessema B, Sack U, König B, Serebrovska Z and Egorov E (2022) Effects of Intermittent Hypoxia in Training Regimes and in Obstructive Sleep Apnea on Aging Biomarkers and Age-Related Diseases: A Systematic Review. Front. Aging Neurosci. 14:878278. doi: 10.3389/fnagi.2022.878278
Received: 17 February 2022; Accepted: 21 April 2022;
Published: 23 May 2022.
Edited by:
Aurel Popa-Wagner, University of Medicine and Pharmacy of Craiova, RomaniaReviewed by:
Jihyun Song, The University of Utah, United StatesJ. Thomas Cunningham, University of North Texas Health Science Center, United States
Copyright © 2022 Tessema, Sack, König, Serebrovska and Egorov. This is an open-access article distributed under the terms of the Creative Commons Attribution License (CC BY). The use, distribution or reproduction in other forums is permitted, provided the original author(s) and the copyright owner(s) are credited and that the original publication in this journal is cited, in accordance with accepted academic practice. No use, distribution or reproduction is permitted which does not comply with these terms.
*Correspondence: Belay Tessema, bt1488@yahaoo.com, Belay.Belay@medizin.uni-leipzig.de