- 1Department of Medical Genetics, School of Medicine, Shahid Beheshti University of Medical Sciences, Tehran, Iran
- 2Department of Medical Biotechnology, School of Advanced Technologies in Medicine, Shahid Beheshti University of Medical Sciences, Tehran, Iran
- 3Department of Pharmacognosy, College of Pharmacy, Hawler Medical University, Erbil, Iraq
- 4Center of Research and Strategic Studies, Lebanese French University, Erbil, Iraq
- 5Department of Pharmacology and Toxicology, College of Pharmacy, Hawler Medical University, Erbil, Iraq
- 6Behbahan Faculty of Medical Sciences, Behbahan, Iran
- 7Institute of Human Genetics, Jena University Hospital, Jena, Germany
- 8Skull Base Research Center, Loghman Hakim Hospital, Shahid Beheshti University of Medical Sciences, Tehran, Iran
- 9Critical Care Quality Improvement Research Center, Loghman Hakim Hospital, Shahid Beheshti University of Medical Sciences, Tehran, Iran
The β-Secretase (BACE1) is widely studied to be particularly involved in amyloid deposition, a process known as the pathogenic pathway in neurodegenerative diseases. Therefore, BACE1 expression is frequently reported to be upregulated in brain samples of the patients with Alzheimer’s disease (AD). BACE1 expression is regulated by BACE1-AS, a long non-coding RNA (lncRNA), which is transcribed in the opposite direction to its locus. BACE1-AS positively regulates the BACE1 expression, and their expression levels are regulated in physiological processes, such as brain and vascular homeostasis, although their roles in the regulation of amyloidogenic process have been studied further. BACE1-AS dysregulation is reported consistent with BACE1 in a number of human diseases, such as AD, Parkinson’s disease (PD), heart failure (HF), and mild cognitive impairment. BACE1 or less BACE1-AS inhibition has shown therapeutic potentials particularly in decreasing manifestations of amyloid-linked neurodegenerative diseases. Here, we have reviewed the role of lncRNA BACE1 and BACE1-AS in a number of human diseases focusing on neurodegenerative disorders, particularly, AD.
Introduction
Although non-coding RNAs (ncRNAs) have been paid less attention compared to protein-coding transcripts, studies in the past decades have unveiled their regulatory roles and widespread biological functions (Hussen et al., 2021b; Najafi et al., 2022b). Long ncRNAs (lncRNAs) are a group of ncRNAs, which are characterized by their length composed of more than 200 nucleotides, and so longer than other ncRNAs (Najafi et al., 2022a). Structurally, lncRNAs can be subdivided into linear and circular transcripts, of which the former class is mainly referred to as conventional transcripts, although each linear transcript can have a circular counterpart, and the latter structures are occasionally found with higher concentration and stability compared to their linear counterparts (Rahmati et al., 2021). They do not possess open reading frames (ORFs) [though exceptions have been reported (Bánfai et al., 2012; Hartford and Lal, 2020)] and thus predominantly not transcribed to protein-encoding transcripts; although studies have revealed protein-encoding potentials for a number of lncRNAs (Rinn and Chang, 2012), however, thousands of genes are identified in association with lncRNAs in mammalian genome, such as humans, which are transcribed to lncRNAs at high levels exceeding tens of thousands of lncRNAs in human cells (Guttman et al., 2009; Iyer et al., 2015). The expression of lncRNAs is mainly conducted from unconventional sequences, such as regulatory promoters, enhancers, sense and antisense transcripts, and introns from eukaryotic genomes (Al-Tobasei et al., 2016). Accordingly, lncRNAs have been divided into 8 subclasses based on their expressing genomic regions, including intergenic, intronic, promoter, enhancer, sense/antisense, bidirectional, small nuclear RNA, and non-polyadenylated lncRNAs (Ma et al., 2013). They are extensively found in all types of eukaryotic cells with specific patterns of expression making some transcripts limited to a specific type of cell or tissue or even a stage of organ development (Sarropoulos et al., 2019). Particularly, sequencing of some lncRNAs has shown that their sequences, although less compared to mRNAs, are conserved among different species, suggesting substantial roles for these non-coding transcripts, which has forced them to evolutionary pressure (Hezroni et al., 2015; Quinn and Chang, 2016). Although RNA sequencing studies have shown that a majority of lncRNAs demonstrate poor DNA conservation with specific expression in primates (Fang and Fullwood, 2016), RNA polymerase II (RNA Pol II) plays the main role in the transcription of lncRNAs, and phosphorylation of its carboxy-terminal domain can affect the lncRNAs expression (Statello et al., 2021). Although this mechanism controls the expression of lncRNAs, however, some lncRNAs escape this regulatory mechanism to be transcribed (Schlackow et al., 2017). Mechanisms responsible for the biogenesis of lncRNAs are not fully elucidated; however, several mechanisms, such as RNase cleavage, RNA and protein complexes recruiting to the ends of transcripts, generation of circular forms, and paraspeckles, are known to be involved in this process (Dahariya et al., 2019). LncRNAs are predominantly located at the cell nucleus and undergo several processes, such as splicing, although with lower efficiency compared to mRNAs, 5′ capping, and 3′ polyadenylation similar to already known protein-encoding mRNAs transcripts (Statello et al., 2021). Although enough evidence is still required to confirm the functionality of a majority of lncRNAs (Statello et al., 2021), however, current findings on a number of lncRNAs support that acting in both cis and trans pathways, lncRNAs play a role in the landscape of cell biology via regulation of a wide variety of substantial biological processes, such as cell proliferation and differentiation, organogenesis, gene expression, and epigenetic regulation (Kazemzadeh et al., 2015). Some of the best-known lncRNAs include X-inactive-specific transcript (XIST), which is known to contribute to epigenetic dosage compensation via regulation of X-chromosome inactivation (Cerase et al., 2015), Kcnq1ot1 in genomic imprinting, and a handful of lncRNAs, such as H19, MALAT1, and HOTAIR, with regulatory functions on cell cycle, proliferation, and tumorigenesis. Deregulation of these processes is already identified in association with pathological conditions, and thus, it is not surprising to know that an increasing number of lncRNAs show deviations in their regulation in association with various human diseases. These include neurodegenerative disorders, diabetes, hereditary diseases, coronary diseases, autoimmune disease, and various types of cancers (Wapinski and Chang, 2011; Sparber et al., 2019; Hussen et al., 2021a). The increasing importance of lncRNAs’ role in the landscape of human diseases has caused the requirement to databases, such as LncRNADisease, for collecting and providing access to findings on the association of lncRNAs with various diseases (Bao et al., 2019). Elucidation of the role of lncRNAs in the development of health disorders can help develop diagnostic and therapeutic approaches for various human disorders. In this study, although we outlined the roles of both BACE1 β-Secretase and its antisense transcript BACE1-AS in a number of human diseases, we have tried to focus on the regulatory role of BACE1-AS in the development of human diseases and its potentials as a biomarker.
In the amyloid cascade, the β-Secretase-1 (BACE1) enzyme is responsible for the cleavage of amyloid precursor protein (APP) resulting in the formation of amyloid-β 1 - 40 (Aβ1 - 40) and Aβ1 - 42, followed by secretase cleavage (Goedert and Spillantini, 2006). This process plays a central role in the pathophysiology of Alzheimer’s disease (AD), in which the biogenesis of amyloid plaques is responsible for the disease manifestations. Changes, either increased or decreased, in BACE1 expression have been associated with pathological conditions, such as AD, memory loss, and disturbance in synaptic plasticity, indicating strict regulatory mechanism controlling its expression (Faghihi et al., 2008). The regulation of BACE1 by a lncRNA was reported for the first time by Faghihi et al. (2008). This lncRNA is a natural antisense transcript transcribed in the opposite direction to the BACE1 locus and thus called BACE1-AS. This strand was initially identified among thousands of sense/antisense transcripts in human cells showing conservation with mice in FANTOM assays (Engström et al., 2006). BACE1-AS has a 2 kb length transcribed from the opposite strand of the sense locus on chromosome 11 (11q 23.3) (Kandalepas and Vassar, 2014). Although the expression pattern of BACE1-AS in normal cells is not determined, however, a few studies have reported that BACE1-AS is expressed in human cells regulating the BACE1 expression unlike BACE1 which is known to be expressed in various cell types. These cells expressing BACE1-AS include neuronal cells with a considerable high number of studies devoted to assess the BACE1-AS role in the AD pathophysiology and myocardial cells (Greco et al., 2017; Li et al., 2019). BACE1-AS expression is shown to be involved in physiological processes, such as apoptosis and neurogenesis (Modarresi et al., 2011; Sadeghi et al., 2018). The regulatory effect of BACE1-AS on BACE1 expression is associated with the pathophysiology of a handful of human diseases, particularly, AD and cancers. Here, we have a glance at an increasing number of evidence reporting the role of lncRNA BACE1-AS in various diseases with a focus on neurodegenerative diseases and malignancies.
Neurodegenerative Diseases
Alzheimer’s Disease
The AD is the most common dementia disorder affecting 29 million people in the past decades of life (Cole and Vassar, 2008). The formation of senile plaques and neurofibrillary tangles are the characteristic findings described in brain autopsies (Flaten et al., 2014). The amyloid hypothesis as the most popular model described for AD pathogenesis introduced in the period of 1991–1992 (Beyreuther and Masters, 1991; Hardy and Allsop, 1991; Selkoe, 1991; Hardy and Higgins, 1992) states that Aβ deposition is responsible for AD pathogenesis and the corresponding disease manifestations through triggering a cascade damaging the neurons and synapses (Morris et al., 2014). Aβ is produced from APP, which normally acts as a transmembrane protein, in a process of sequential cleavages by secretase enzymes (Zhang et al., 2011). Although APP can be affected by α-secretase, in the amyloidogenic process, two other secretases play the main role. First, β-Secretase cleaves the APP producing β-carboxyl-terminal fragments (bCTFs) sequentially affected by γ-secretase and finally generating Aβ (Zhang et al., 2011; Zhao et al., 2020).
Through APP cleavage and, consequently, generation of Aβ plaques, BACE1 plays a promoting role in the pathogenesis of AD (Cole and Vassar, 2008). BACE1 naturally plays a role in the processing of neuregulin-1 (NRG1), which is involved in development, and signaling pathways of neural cells (Fleck et al., 2012). Its levels have been reported to be elevated in brain samples of subjects with AD supporting other evidence indicating the association of BACE1 with AD pathogenesis (Sathya et al., 2012). BACE1 is shown to regulate the voltage-gated sodium channels controlling the neuronal activity involved in AD pathophysiology (Kim et al., 2007). Kandalepas et al. (2013) demonstrated that BACE1 expression is localized to the presynaptic terminals surrounding amyloid plaques in AD mouse models. Accordingly, Luo et al. (2001) showed that BACE1-deficient mice exhibit healthy phenotype and suppressed production of Aβ. Following advances in understanding the BACE1 mechanisms of action, several inhibitors have been suggested with potential application in AD treatment via targeting the enzymatic activity in AD development (Vassar and Kandalepas, 2011; Sathya et al., 2012). The lncRNA BACE1-AS has been shown to be upregulated in AD specimens by positively regulating the BACE1 expression using a feed-forward mechanism (Faghihi et al., 2008; Figure 1). Liu et al. (2014) showed that exogenous Aβ1–42 induces the expression of both BACE1 and BACE1-AS in the SH–SY5Y cell model for AD. BACE1-AS was demonstrated to enhance the BACE1 mRNA stability via forming RNA duplexes. Conversely, siRNA-mediated BACE1-AS knockdown caused to diminish in BACE1 potential for APP cleavage and formation of senile plaques. Furthermore, Zeng et al. (2019) in another study showed that BACE1-AS acts as a competing endogenous RNA (ceRNA) stabilizing the BACE1 expression via repressing several microRNAs (miRNAs) and targeting BACE1 mRNA. Accordingly, the levels of these miRNAs were increased upon BACE1-AS knockdown. Functionally, BACE1-AS is shown to promote autophagy contributing to neuronal damage (Zhou et al., 2021). Zhou et al. (2021) found that autophagy was increased consistent with BACE1-AS expression that led to neuron injury, while BACE1-AS knockdown improved damage injury via modulation of autophagy. The dual-luciferase assay revealed that BACE1-AS targets miR-214-3p and upregulates ATG5 as a ceRNA. The same effect on BACE1-AS was observed when miR-214-3p was inhibited. These findings indicated that lncRNA BACE1-AS exerts its role in the development of AD phenotype through an axis mediated by miRNAs. This class of ncRNAs has been studied to act as players and suggested as biomarkers for several neurodegenerative and psychiatric disorders, such as AD, Parkinson’s disease (PD), and schizophrenia (Angelucci et al., 2019; Fyfe, 2020; Davarinejad et al., 2021), and, therefore, it is not surprising to see miRNAs involved in BACE1-AS-mediated pathogenesis in AD. Accordingly, another miRNA miR-214-3p was shown to perform the BACE1-AS impact on enhancing the isoflurane-induced neurotoxicity in AD SK-N-SH and SK-N-AS cell models (He et al., 2020). Taken together, an increasing number of evidence clearly confirms the role of lncRNA BACE1-AS in the pathophysiology of AD via regulation of BACE1 expression. This function has suggested BACE1-AS with diagnostic and therapeutic applications. Repeatedly, BACE1-AS has been reported to serve as a biomarker in the differentiation of patients with AD from healthy individuals. Through the comparison of BACE1-AS mRNA levels in plasma and plasma-derived exosomes retrieved from patients with AD and healthy controls, Fotuhi et al. (2019) found that although no association can be observed between BACE1-AS expression and state of disease, BACE1-AS showed higher expression in patients with AD compared to those at the pre-AD stage. In addition, receiver operating characteristic (ROC) curve analysis showed good sensitivity and specificity of BACE1-AS in the differentiation of patients with pre-AD and AD from healthy individuals. BACE1-AS in combination with traditionally used paraclinical diagnostic parameters has also shown an improving effect on diagnostic power (Wang et al., 2020). In this study, Wang et al. (2020) demonstrated that BACE1-AS levels in plasma exosomes in combination with right entorhinal cortex volume and thickness promote sensitivity and specificity of both parameters to 90.91 and 96.15%, respectively, in the detection of patients with AD compared to the condition when MRI parameters are used alone. Furthermore, targeting BACE1-AS has shown therapeutic potentials in improving AD symptoms and pathological manifestations in cell and animal studies. For instance, Modarresi et al. (2011) demonstrated that BACE1-AS knockdown ameliorates Aβ-associated hippocampal neurogenesis. Improved memory and learning behaviors in siRNA-mediated BACE1-AS-silenced AD mouse models were reported in another study described by Zhang et al. (2018). Ge et al. (2020) also found that BACE1-AS depletion in combination with berberine ameliorates Aβ25–35-associated neuronal injury in human primary neuron and SK-N-SH AD model cells. Curcumin as a polyphenol extracted from plant Curcuma longa Linn. has also shown a repressing effect on BACE1 expression in SH-SY5Y cell lines, suggesting therapeutic potentials for AD (Huang et al., 2020). The same effect on amyloidogenesis has been reported for ginsenoside or combined taxifolin and cilostazol (Cao et al., 2016; Park et al., 2016). Bioinformatics analyses have also demonstrated therapeutic potentials for Lupeol and several compounds extracted from Cajanus cajan and Citrus reticulata by the inhibition of BACE1 in situ (Koirala et al., 2017; Adewole and Ishola, 2021). Moreover, miRNAs, such as miR-34a-5p, miR-125b-5p, miR-15b, and miR-149, have been demonstrated in distinctive studies to inhibit BACE1 expression, decrease amyloid accumulation, and ameliorate neuronal injury (Gong et al., 2017; Li P. et al., 2020; Du et al., 2021). Overall, although the precise mechanism of action is not elucidated, however, BACE1-AS as a lncRNA is reportedly involved in the pathophysiology of AD rather than another disease, which is believed to play a role via the regulation of mRNA stability and expression of BACE1 β-Secretase. Accordingly, diagnostic and therapeutic potentials of BACE1-AS in AD have been paid more attention compared to other diseases hopefully suggesting that this lncRNA can bring beneficial potentials, particularly, in diagnosis and treatment of this disease.
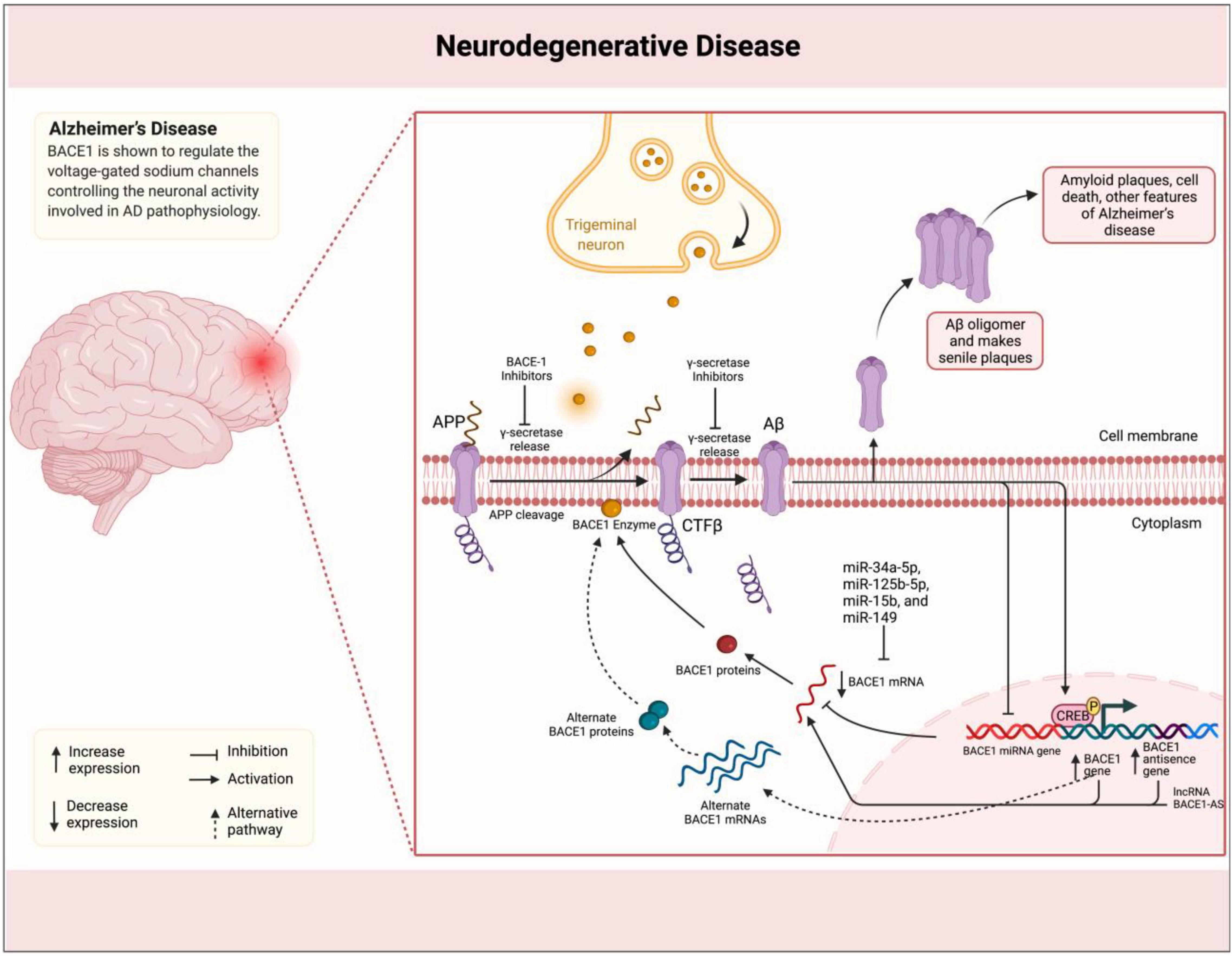
Figure 1. BACE1-AS promotes the synthesis of β-secretase in the brain of patients with AD. Amyloid plaques in the brain of patients with AD are linked to the illness. Plaques are formed by the successive breakdown of the APP via β-secretase (BACE1) and γ-secretase. In individuals with BACE1 deficiency, the mRNA is stabilized by binding to its antisense non-coding RNA (BACE1-AS). miRNAs, such as miR-34a-5p, miR-125b-5p, miR-15b, and miR-149, inhibit BACE1 expression, decrease amyloid accumulation, and ameliorate neuronal injury.
Parkinson’s Disease
The PD is the second common neurodegenerative disease after AD. PD is characterized by motor manifestations, such as tremor, rigidity, and bradykinesia (Schneider et al., 2017). Several lncRNAs have been found to be dysregulated in the PD pathophysiology, such as MALAT1 through facilitating the inflammasome activation (Cai et al., 2020), BDNF-AS and NEAT1 via promoting autophagy and apoptosis in vitro and in vivo (Fan et al., 2020; Dong et al., 2021), and SNHG1 via promoting neuronal injury (Wang H. et al., 2021). BACE1 and its antisense transcript BACE1-AS have been less studied in PD compared to those in AD. Bekris et al. (2015) reported a correlation between two single nucleotide polymorphisms in the APP gene and Aβ42 levels in cerebrospinal fluid (CSF) of patients with PD. Lange et al. (2015) also demonstrated an association between rs638405 polymorphism in the BACE1 gene and enhanced risk of PD in a Norwegian population of patients with PD. The role of lncRNA BACE1-AS in PD was reported by Li Y. et al. (2020) in 2019, although, currently, further investigations are required to suggest BACE1-AS potentials in PD. In the latter study, the authors showed that BACE1-AS silencing ameliorates dopamine-dependent oxidative stress in PD model rats. The isolated substantia nigra tissues in rats with downregulated BACE1-AS had decreased α-synuclein and inducible nitric oxide synthase (iNOS). Diminished BACE1-AS also repressed neuronal injury and apoptosis in animals. The promoting effect of BACE1-AS on BACE1 expression was shown to be exerted through “sponging” miRNA miR-34b-5p.
Cancer
Today, cancer is among the most common causes of human diseases and related deaths with 19 newly diagnosed patients and 10 million deaths reported in 2020 (Ferlay et al., 2018). Several features are required to be achieved for the development of cancer known as “hallmarks of cancer.” These include limitless proliferative potential, self-sufficiency in growth signals, insensitivity to anti-growth signals, tissue invasion and metastasis, evading apoptosis, and sustained angiogenesis (Su et al., 2019). Several classes of ncRNAs, including lncRNAs, miRNAs, and circular RNAs, have been widely studied in various human malignancies in association with cancer development and progression (Visone and Croce, 2009; Huarte, 2015; Sayad et al., 2022). Although the precise functions of ncRNAs leading to cancer promotion are not completely elucidated, circRNAs, for instance, are found to regulate critical cellular processes via “sponging” miRNAs within axes of action leading to enhanced malignant behaviors (Taheri et al., 2021).
Although BACE1 and BACE1-AS have been extensively studied in patients with AD, however, their role in tumorigenesis is poorly understood. The putative role of β-secretase BACE1 and its antisense transcript BACE1-AS in angiogenesis has suggested that they can play a role in tumorigenesis (Esfandi et al., 2019). It is also found to regulate signaling pathways involved in tumorigenesis (He et al., 2014). The APP plays a role in regulating cell proliferation in cancer cells, particularly, in breast cancer (Lim et al., 2014). Accordingly, elevated β-secretases BACE1 and BACE2 have been shown to play a role in a number of human malignancies (Farris et al., 2021). They were reported for the first time to enhance cell survival in pancreatic cancer cells, and also the effects of several inhibitors were compared with suppression of cell survival (Peters et al., 2012). Through affecting tumor microenvironment features, such as neutrophil extracellular traps (NETs), BACE1/2 leads to promoted cancer development and progression and, therefore, it is not surprising to see that their inhibition causes and modulates tumor growth (Munir et al., 2021). In another study, Zhai et al. (2021) very recently have demonstrated that pharmacological inhibition of BACE1 using MK-8931 causes reprogramming of tumor-promoting macrophages (pTAMs) into tumor-suppressive macrophages (sTAMs) and suppression of tumor growth in glioblastoma and, therefore, using AD drugs in the treatment of human cancers can be predicted (Martinez-Usatorre and De Palma, 2021; Figure 2). Conversely, miR-574-3p is shown to promote the migratory and invasive potentials of non-small cell lung cancer (NSCLC) cells (Yuan et al., 2019), which also particularly suggests potentials in fighting against human malignancies where silencing miRNAs using antisense oligonucleotides have shown a potent effect in cancer therapy (Dean and Bennett, 2003). Esfandi et al. (2019) reported downregulation of both BACE1 and BACE1-AS in 30 tissue samples of patients with gastric cancer in consistent with findings for BACE1 in breast ductal carcinoma (Yaghoobi et al., 2019).
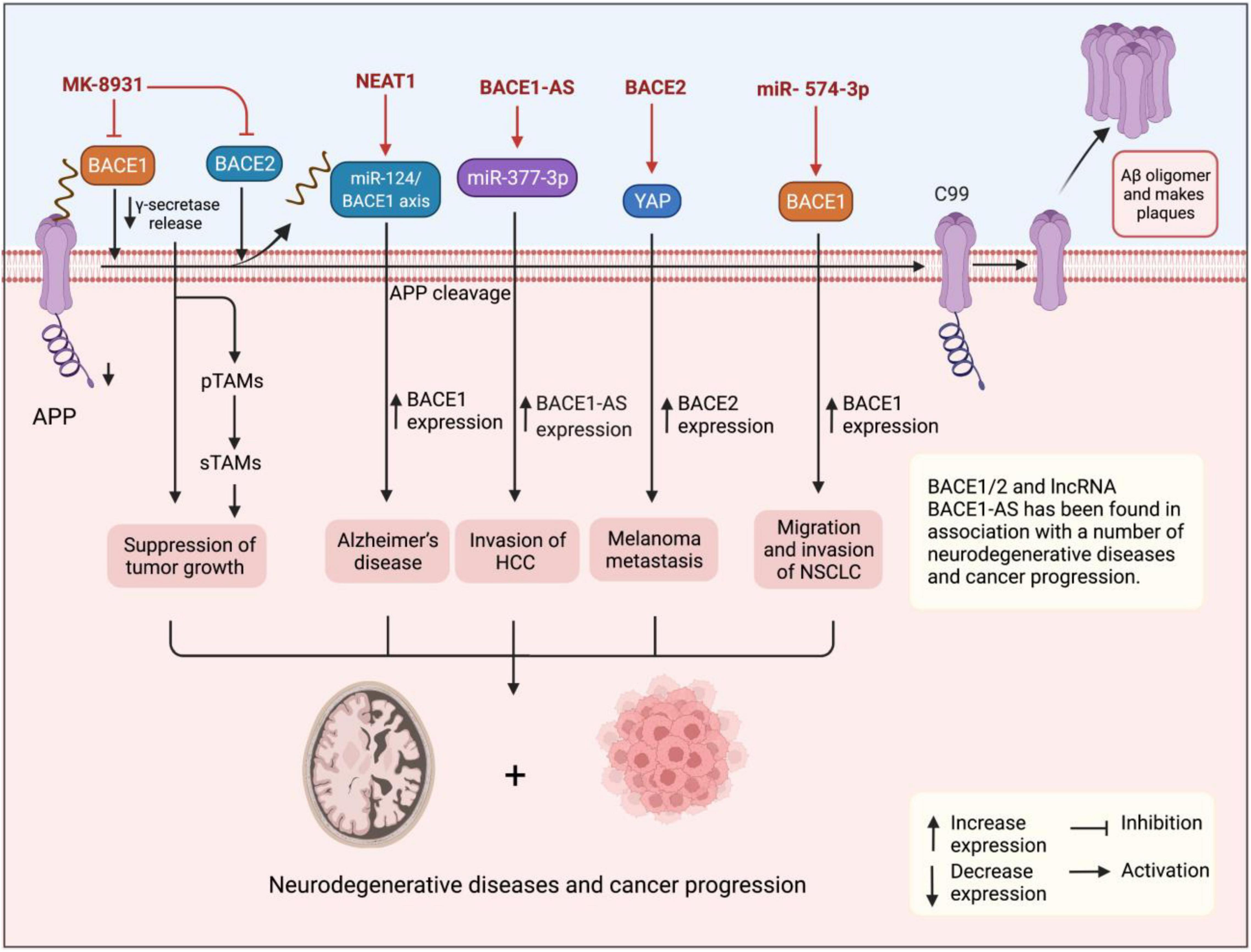
Figure 2. Figure shows the upregulation of BACE1 and BACE1-AS in cancer tissues and cells suggesting them with oncogenic functions. BACE1 and lncRNA BACE1-AS have also been found in association with progression for several cancers and neurodegenerative diseases. Pharmacological inhibition of BACE1 using MK-8931 causes reprogramming of pTAMs into sTAMs and suppression of tumor growth in glioblastoma.
Similar to BACE1, the lncRNA BACE1-AS has also been found in association with a number of cancers in a series of evidence. For instance, Wang M. et al. (2021) using expression patterns and survival data in 32 cancer types retrieved from the cBioPortal1 and Cancer Genome Atlas2 found that BACE1-AS is significantly upregulated in various human malignancies, such as glioblastoma, liver hepatocellular carcinoma (HCC), kidney renal clear cell carcinoma, and colon adenocarcinoma. Moreover, high BACE1-AS expression was correlated with poor survival in head and neck squamous cell carcinoma and liver carcinoma. BACE1-AS also showed a mutation in 0.9% of cancers mainly in melanoma cases. Importantly, according to the databases, BACE1-AS demonstrated a negative correlation with the tumor microenvironment. Experimentally, Liu et al. (2021) have recently reported the role of BACE1-AS in promoting the malignant features of HCC cells. They found that BACE1-AS expression is elevated in YY-8103, MHCC-97L, MHCC-97H, HCCLM3, and Huh-7 HCC cell lines compared to L02 healthy hepatic cell line and also in cancer tissues isolated from patients diagnosed with HCC compared to healthy adjacent tissues in the quantitative real-time polymerase chain reaction (qRT-PCR) assay, which was consistent with in situ findings. The dual-luciferase assay demonstrated that BACE1-AS sponges miR-377-3p confirming the bioinformatics predictions. Importantly, BACE1-AS knockdown suppressed the migration and invasion of HCC cells in wound healing and transwell assays, while BACE1-AS overexpression reversed the inhibitory effect on migratory and invasive potentials of cancer cells. Moreover, experiments in xenograft animal models revealed that BACE1-AS knockdown also inhibits tumor invasion and metastasis in vivo. Accordingly, elevated expression of BACE1-AS in cancer cells and tissues in addition to its promoting effects on their malignant features suggests BACE1-AS as an oncogenic lncRNA. In another study, Chen et al. (2016) demonstrated that BACE1-AS is responsible for antiproliferative and anti-invasive effects of anisomycin on ovarian cancer cells, while its knockdown ameliorates the chemotherapy-associated tumor suppressor effects and so concluded that BACE1-AS is a target for anisomycin in suppression of cancer cell proliferation and invasion. More than the evaluation of BACE1-AS potentials in cancer development and progression, this lncRNA has been studied as a biomarker with potential applications in the differentiation of several human cancers. For example, Nie et al. (2020), by analyzing the RNA-Seq expression data retrieved from the Cancer Genome Atlas database for 370 patients, found that the expression levels of upregulated BACE1-AS in liver cancer tissues were associated with clinicopathological features, such as tumor histological types and age of patients. BACE1-AS was shown to differentiate cancerous patients with high sensitivity, specificity, and area under the curve (AUC) values of 0.94, 0.836, and 0.949, respectively. Moreover, high BACE1-AS expression predicted poor overall survival (OS) and recurrence-free survival (RFS) for patients with liver cancer. In another study, Esfandi et al. (2019) reported diagnostic power of 75% for BACE1-AS in 30 patients with gastric cancer, while Yeganeh et al. (2020) also reported that increased expression of BACE1-AS along with 4 other lncRNAs in 30 tissues of breast cancer suggested them as potential biomarkers for breast cancer. Taken together, studies show upregulation of BACE1 and BACE1-AS in cancer tissues and cells suggesting them with oncogenic functions. Furthermore, the lncRNA BACE1-AS has shown diagnostic potentials in the differentiation of cancer patients from healthy individuals and also between different types of cancer.
Heart Failure
Heart failure (HF) is a major cardiac disease with more than 37 million people estimated with the disease with high morbidity and mortality in the patients (Ziaeian and Fonarow, 2016). In association with amyloidogenic disorders, cardiac amyloidogenesis occurs upon heart involvement in systemic amyloidogenesis (Banypersad et al., 2012). Cardiac accumulation of transthyretin deposits in the affected patients seen in amyloidosis due to transthyretin deposition (ATTR) cause clinical manifestations of HF (Kittleson et al., 2020). Other types of amyloidoses with cardiac involvement include hereditary amyloidosis resulting from mutations in several genes, i.e., transthyretin and fibrinogen, and senile systemic amyloidosis (Banypersad et al., 2012). The role and diagnostic potentials of lncRNAs in the landscape of HF have been evaluated in a number of studies (Pang et al., 2016; Xu et al., 2020; Zhao et al., 2021). Zhuang et al. (2021) showed that lncRNA OIP5-AS1 that is highly found in striated muscles endures regulation in heart development, and mice models showed more severe HF under induction by pressure overload compared to healthy controls. In another study, Zhao et al. (2021) demonstrated that lncRNA MALAT1 is significantly upregulated in serum samples of 57 patients with HF and 40 HF rat models with an AUC of 0.918 in the prediction of patients with HF. Interestingly, MALAT1 inhibition ameliorated the degree of myocardial injury, improved the lipid metabolism, decreased inflammation in the rat models, and also relieved myocardial injury in the H9C2 cells. Similar results have been reported for SOX2-OT in H9C2 cells where it’s silencing reduced cell injury via promotion of cell viability, suppression of apoptosis, and ameliorating productions of collagens (Tu et al., 2021). Unlike cardiac amyloidogenesis, amyloid deposits are not common in cardiac muscle tissue in patients with HF; however, the involvement of ncRNAs in HF may demonstrate their complex role in the disease pathogenesis. The effect of BACE1-AS on HF was shown in a study by Greco et al. (2017). The authors measured the expression of BACE1 and BACE1-AS in the left ventricle biopsies retrieved from 18 patients with ischemic HF. The qRT-PCR results showed the elevated expression of both BACE1 and BACE1-AS in patient tissues compared to matched controls similar to the findings for mice models. BACE1 overexpression caused accumulation of intracellular Aβ consistent with results in patients with HF. Interestingly, HAOEC cells or HL-1 cells overexpressing BACE1-AS demonstrated transcriptomic changes similar to those treated with Aβ, which were seen particularly on cell proliferation, apoptosis, inflammatory responses, and signaling pathways. Finally, BACE1-AS overexpression induced apoptosis in endothelial cells, which was reversed by its silencing. Overall, BACE1-AS can act as a potential target for treating HF since accumulated Aβ is observed in patients with HF and BACE1-AS reduces Aβ accumulation and BACE1 levels.
Other Diseases
Rather than the role of BACE1 and the regulatory function of BACE1-AS on amyloidogenic process clearly involved in the pathogenesis of particularly neurodegenerative diseases discussed earlier, both BACE1 and BACE1-AS have been reported with dysregulation in a number of other diseases, which can’t be easily associated with the amylogenic processes. These can be interpreted as impacts of these couple on gene expression and proteins known to play a role in the pathogenesis of several human disorders. Ghafouri-Fard et al. (2020) reported increased expression of BACE1 in children with autism spectrum disorder (ASD) compared to healthy children. Furthermore, BACE1-AS expression was correlated with the patient’s age. BACE1 and BACE1-AS also demonstrated diagnostic power of 0.762, and 0.795, respectively, for AUC in the ROC curve. In another study, Zhong et al. (2007) evaluated the BACE1 levels in the CSF specimens of 45 patients with mild cognitive impairment (MCI) via ELISA and Western blot analysis. The results demonstrated that patients with MCI have elevated BACE1 expression levels and β-Secretase enzymatic activity compared to healthy controls, which are also significantly higher than patients with AD. Interestingly, these findings suggest that BACE1, which is traditionally considered as an AD biomarker, can be used in the differentiation of patients with MCI from AD individuals. In addition, Cai et al. (2012) reported the expression of BACE1 in mice retina, and to explore its role on retinal health, they developed BACE1–/– knockout animals. The results demonstrated distinct thinning in the neural retina, increased apoptosis in nuclei, and increased lipofuscin in addition to thinning and atrophy at the retinal pigment epithelium (RPE) in favor of retinal pathology. Furthermore, BACE1 inhibition using β-secretase inhibitor IV (β-SI) was shown to promote angiogenesis in mice retinas. These findings revealed the role of BACE1 in eye health and its dysregulation can cause retinal pathogenesis via disturbance in vascular homeostasis and accumulation of aging lipofuscin pigments beyond its traditional roles in amyloidogenesis.
Concluding Remarks
β-Secretase (BACE1) particularly plays a role in amyloid-linked neurodegenerative diseases and its expression is known to be upregulated in brain samples of patients with AD. BACE1 expression is regulated by BACE1-AS, a lncRNA, which is transcribed in the opposite direction to its locus. BACE1-AS positively regulates the BACE1 expression and, therefore, their dysregulation is consistently reported in a number of human diseases, such as AD, PD, HF, and MCI. It is known that lncRNAs have been found with their regulatory functions on gene expression and their dysregulations are being increasingly reported with involvement in human diseases. Accordingly, enhanced expression of BACE1-AS is also reported particularly in amyloid-linked neurodegenerative diseases consistent with BACE1 expression. Both are reported with diagnostic potentials in the differentiation of some diseases, and although BACE1 inhibition has been further explored with therapeutical effects, however, BACE1-AS also suggests potentials. Taken together, detection of BACE1-AS or BACE1 as an easy, reliable, and minimally invasive approach with applications in diagnosis of particularly neurodegenerative diseases or their targeting as a therapeutic approach for diminishing amyloidogenesis requires further studies, guaranteeing their usages in the promotion of human health in future. Among the efficient approaches for targeting nucleic acids, antisense oligonucleotides may suggest potentials for inhibition of BACE1 or lncRNA BACE1-AS.
Author Contributions
MT designed and supervised the study. SN wrote the draft and revised it. BH, SA, MH, AM, and AS collected the data and designed the figures and tables. All authors read and approved the submitted version.
Conflict of Interest
The authors declare that the research was conducted in the absence of any commercial or financial relationships that could be construed as a potential conflict of interest.
Publisher’s Note
All claims expressed in this article are solely those of the authors and do not necessarily represent those of their affiliated organizations, or those of the publisher, the editors and the reviewers. Any product that may be evaluated in this article, or claim that may be made by its manufacturer, is not guaranteed or endorsed by the publisher.
Footnotes
References
Adewole, K. E., and Ishola, A. A. (2021). BACE1 and cholinesterase inhibitory activities of compounds from Cajanus cajan and Citrus reticulata: an in silico study. In Silico Pharmacol. 9:14. doi: 10.1007/s40203-020-00067-6
Al-Tobasei, R., Paneru, B., and Salem, M. (2016). Genome-wide discovery of long non-coding RNAs in rainbow trout. PLoS One 11:e0148940. doi: 10.1371/journal.pone.0148940
Angelucci, F., Cechova, K., Valis, M., Kuca, K., Zhang, B., and Hort, J. (2019). MicroRNAs in Alzheimer’s disease: diagnostic markers or therapeutic agents? Front. Pharmacol. 10:665. doi: 10.3389/fphar.2019.00665
Bánfai, B., Jia, H., Khatun, J., Wood, E., Risk, B., Gundling, W. E., et al. (2012). Long noncoding RNAs are rarely translated in two human cell lines. Genome Res. 22, 1646–1657. doi: 10.1101/gr.134767.111
Banypersad, S. M., Moon, J. C., Whelan, C., Hawkins, P. N., and Wechalekar, A. D. (2012). Updates in cardiac amyloidosis: a review. J. Am. Heart Assoc. 1:e000364. doi: 10.1161/JAHA.111.000364
Bao, Z., Yang, Z., Huang, Z., Zhou, Y., Cui, Q., and Dong, D. (2019). LncRNADisease 2.0: an updated database of long non-coding RNA-associated diseases. Nucleic Acids Res. 47, D1034–D1037. doi: 10.1093/nar/gky905
Bekris, L. M., Tsuang, D. W., Peskind, E. R., Yu, C. E., Montine, T. J., Zhang, J., et al. (2015). Cerebrospinal fluid Aβ42 levels and APP processing pathway genes in Parkinson’s disease. Mov. Disord. 30, 936–944. doi: 10.1002/mds.26172
Beyreuther, K., and Masters, C. L. (1991). Amyloid precursor protein (APP) and B ZA4 amyloid in the etiology of Alzheimer’s disease: precursor-product relationships in the derangement of neuronal function. Brain Pathol. 1, 241–251. doi: 10.1111/j.1750-3639.1991.tb00667.x
Cai, J., Qi, X., Kociok, N., Skosyrski, S., Emilio, A., Ruan, Q., et al. (2012). β-Secretase (BACE1) inhibition causes retinal pathology by vascular dysregulation and accumulation of age pigment. EMBO Mol. Med. 4, 980–991. doi: 10.1002/emmm.201101084
Cai, L. J., Tu, L., Huang, X. M., Huang, J., Qiu, N., Xie, G. H., et al. (2020). LncRNA MALAT1 facilitates inflammasome activation via epigenetic suppression of Nrf2 in Parkinson’s disease. Mol. Brain 13:130. doi: 10.1186/s13041-020-00656-8
Cao, G., Su, P., Zhang, S., Guo, L., Zhang, H., Liang, Y., et al. (2016). Ginsenoside Re reduces Aβ production by activating PPARγ to inhibit BACE1 in N2a/APP695 cells. Eur. J. Pharmacol. 793, 101–108. doi: 10.1016/j.ejphar.2016.11.006
Cerase, A., Pintacuda, G., Tattermusch, A., and Avner, P. (2015). Xist localization and function: new insights from multiple levels. Genome Biol. 16:166. doi: 10.1186/s13059-015-0733-y
Chen, Q., Liu, X., Xu, L., Wang, Y., Wang, S., Li, Q., et al. (2016). Long non-coding RNA BACE1-AS is a novel target for anisomycin-mediated suppression of ovarian cancer stem cell proliferation and invasion. Oncol. Rep. 35, 1916–1924. doi: 10.3892/or.2016.4571
Cole, S. L., and Vassar, R. (2008). The role of amyloid precursor protein processing by BACE1, the β-Secretase, in alzheimer disease pathophysiology *. J. Biol. Chem. 283, 29621–29625.
Dahariya, S., Paddibhatla, I., Kumar, S., Raghuwanshi, S., Pallepati, A., and Gutti, R. K. (2019). Long non-coding RNA: classification, biogenesis and functions in blood cells. Mol. Immunol. 112, 82–92. doi: 10.1016/j.molimm.2019.04.011
Davarinejad, O., Najafi, S., Zhaleh, H., Golmohammadi, F., Radmehr, F., Alikhani, M., et al. (2021). MiR-574-5P, miR-1827, and miR-4429 as potential biomarkers for schizophrenia. J. Mol. Neurosci. 72, 226–238. doi: 10.1007/s12031-021-01945-0
Dean, N. M., and Bennett, C. F. (2003). Antisense oligonucleotide-based therapeutics for cancer. Oncogene 22, 9087–9096. doi: 10.1038/sj.onc.1207231
Dong, L. I., Zheng, Y., Gao, L., and Luo, X. (2021). lncRNA NEAT1 prompts autophagy and apoptosis in MPTP-induced Parkinson’s disease by impairing miR-374c-5p. Acta Biochim. Biophys. Sin. 53, 870–882. doi: 10.1093/abbs/gmab055
Du, W., Lei, C., and Dong, Y. (2021). MicroRNA-149 is downregulated in Alzheimer’s disease and inhibits β-amyloid accumulation and ameliorates neuronal viability through targeting BACE1. Genet. Mol. Biol. 44:e20200064. doi: 10.1590/1678-4685-GMB-2020-0064
Engström, P. G., Suzuki, H., Ninomiya, N., Akalin, A., Sessa, L., Lavorgna, G., et al. (2006). Complex Loci in human and mouse genomes. PLoS Genet. 2:e47. doi: 10.1371/journal.pgen.0020047
Esfandi, F., Ghafouri-Fard, S., Oskooei, V. K., and Taheri, M. (2019). β-Secretase 1 and its naturally occurring anti-sense RNA are down-regulated in gastric cancer. Pathol. Oncol. Res. 25, 1627–1633. doi: 10.1007/s12253-019-00621-4
Faghihi, M. A., Modarresi, F., Khalil, A. M., Wood, D. E., Sahagan, B. G., Morgan, T. E., et al. (2008). Expression of a noncoding RNA is elevated in Alzheimer’s disease and drives rapid feed-forward regulation of beta-secretase. Nat. Med. 14, 723–730. doi: 10.1038/nm1784
Fan, Y., Zhao, X., Lu, K., and Cheng, G. (2020). LncRNA BDNF-AS promotes autophagy and apoptosis in MPTP-induced Parkinson’s disease via ablating microRNA-125b-5p. Brain Res. Bull. 157, 119–127. doi: 10.1016/j.brainresbull.2020.02.003
Fang, Y., and Fullwood, M. J. (2016). Roles, functions, and mechanisms of long non-coding RNAs in cancer. Genom. Proteom. Bioinform. 14, 42–54. doi: 10.1016/j.gpb.2015.09.006
Farris, F., Matafora, V., and Bachi, A. (2021). The emerging role of β-secretases in cancer. J. Exp. Clin. Cancer Res. 40:147.
Ferlay, J., Ervik, M., Lam, F., Colombet, M., Mery, L., Piñeros, M., et al. (2018). Global Cancer Observatory: Cancer Today. Lyon: International Agency for Research on Cancer, 1–6.
Flaten, V., Laurent, C., Coelho Joana, E., Sandau, U., Batalha Vânia, L., Burnouf, S., et al. (2014). From epidemiology to pathophysiology: what about caffeine in Alzheimer’s disease? Biochem. Soc. Trans. 42, 587–592. doi: 10.1042/BST20130229
Fleck, D., Garratt, A. N., Haass, C., and Willem, M. (2012). BACE1 dependent neuregulin processing: review. Curr. Alzheimer Res. 9, 178–183. doi: 10.2174/156720512799361637
Fotuhi, S. N., Khalaj-Kondori, M., Hoseinpour Feizi, M. A., and Talebi, M. (2019). Long Non-coding RNA BACE1-AS may serve as an Alzheimer’s disease blood-based biomarker. J. Mol. Neurosci. 69, 351–359. doi: 10.1007/s12031-019-01364-2
Fyfe, I. (2020). MicroRNAs — diagnostic markers in Parkinson disease? Nat. Rev. Neurol. 16, 65–69. doi: 10.1038/s41582-019-0305-y
Ge, Y., Song, X., Liu, J., Liu, C., and Xu, C. (2020). The combined therapy of berberine treatment with lncRNA BACE1-AS depletion attenuates Aβ(25-35) induced neuronal injury through regulating the expression of miR-132-3p in neuronal cells. Neurochem. Res. 45, 741–751. doi: 10.1007/s11064-019-02947-6
Ghafouri-Fard, S., Namvar, A., Arsang-Jang, S., Komaki, A., and Taheri, M. (2020). Expression analysis of BDNF, BACE1, and their natural occurring antisenses in autistic patients. J. Mol. Neurosci. 70, 194–200. doi: 10.1007/s12031-019-01432-7
Goedert, M., and Spillantini, M. G. (2006). A century of Alzheimer’s disease. Science 314, 777–781. doi: 10.1126/science.1132814
Gong, G., An, F., Wang, Y., Bian, M., Yu, L.-J., and Wei, C. (2017). miR-15b represses BACE1 expression in sporadic Alzheimer’s disease. Oncotarget 8, 91551–91557. doi: 10.18632/oncotarget.21177
Greco, S., Zaccagnini, G., Fuschi, P., Voellenkle, C., Carrara, M., Sadeghi, I., et al. (2017). Increased BACE1-AS long noncoding RNA and β-amyloid levels in heart failure. Cardiovasc. Res. 113, 453–463. doi: 10.1093/cvr/cvx013
Guttman, M., Amit, I., Garber, M., French, C., Lin, M. F., Feldser, D., et al. (2009). Chromatin signature reveals over a thousand highly conserved large non-coding RNAs in mammals. Nature 458, 223–227.
Hardy, J., and Allsop, D. (1991). Amyloid deposition as the central event in the aetiology of Alzheimer’s disease. Trends Pharmacol. Sci. 12, 383–388. doi: 10.1016/0165-6147(91)90609-v
Hardy, J. A., and Higgins, G. A. (1992). Alzheimer’s disease: the amyloid cascade hypothesis. Science 256, 184–185.
Hartford, C. C. R., and Lal, A. (2020). When long noncoding becomes protein coding. Mol. Cell. Biol. 40:e00528-19. doi: 10.1128/MCB.00528-19
He, W., Chi, S., Jin, X., Lu, J., Zheng, W., Yan, J., et al. (2020). Long Non-Coding RNA BACE1-AS modulates isoflurane-induced neurotoxicity to Alzheimer’s disease through sponging miR-214-3p. Neurochem. Res. 45, 2324–2335. doi: 10.1007/s11064-020-03091-2
He, W., Hu, J., Xia, Y., and Yan, R. (2014). β-Site amyloid precursor protein cleaving enzyme 1(BACE1) regulates notch signaling by controlling the cleavage of jagged 1 (Jag1) and jagged 2 (Jag2) proteins. J. Biol. Chem. 289, 20630–20637. doi: 10.1074/jbc.M114.579862
Hezroni, H., Koppstein, D., Schwartz, M. G., Avrutin, A., Bartel, D. P., and Ulitsky, I. (2015). Principles of long noncoding RNA evolution derived from direct comparison of transcriptomes in 17 species. Cell Rep. 11, 1110–1122. doi: 10.1016/j.celrep.2015.04.023
Huang, P., Zheng, N., Zhou, H.-B., and Huang, J. (2020). Curcumin inhibits BACE1 expression through the interaction between ERβ and NFκB signaling pathway in SH-SY5Y cells. Mol. Cell. Biochem. 463, 161–173. doi: 10.1007/s11010-019-03638-0
Hussen, B. M., Hidayat, H. J., Salihi, A., Sabir, D. K., Taheri, M., and Ghafouri-Fard, S. (2021b). MicroRNA: a signature for cancer progression. Biomed. Pharmacother. 138:111528. doi: 10.1016/j.biopha.2021.111528
Hussen, B. M., Azimi, T., Hidayat, H. J., Taheri, M., and Ghafouri-Fard, S. (2021a). Long Non-coding RNA RMRP in the pathogenesis of human disorders. Front. Cell Dev. Biol. 9:676588. doi: 10.3389/fcell.2021.676588
Iyer, M. K., Niknafs, Y. S., Malik, R., Singhal, U., Sahu, A., Hosono, Y., et al. (2015). The landscape of long noncoding RNAs in the human transcriptome. Nat. Genet. 47, 199–208. doi: 10.1038/ng.3192
Kandalepas, C. P., and Vassar, R. (2014). The normal and pathologic roles of the Alzheimer’s β-secretase, BACE1. Curr. Alzheimer Res. 11, 441–449. doi: 10.2174/1567205011666140604122059
Kandalepas, P. C., Sadleir, K. R., Eimer, W. A., Zhao, J., Nicholson, D. A., and Vassar, R. (2013). The Alzheimer’s β-secretase BACE1 localizes to normal presynaptic terminals and to dystrophic presynaptic terminals surrounding amyloid plaques. Acta Neuropathol. 126, 329–352. doi: 10.1007/s00401-013-1152-3
Kazemzadeh, M., Safaralizadeh, R., and Orang, A. V. (2015). LncRNAs: emerging players in gene regulation and disease pathogenesis. J. Genet. 94, 771–784. doi: 10.1007/s12041-015-0561-6
Kim, D. Y., Carey, B. W., Wang, H., Ingano, L. A., Binshtok, A. M., Wertz, M. H., et al. (2007). BACE1 regulates voltage-gated sodium channels and neuronal activity. Nat. Cell Biol. 9, 755–764. doi: 10.1038/ncb1602
Kittleson, M. M., Maurer, M. S., Ambardekar, A. V., Bullock-Palmer, R. P., Chang, P. P., Eisen, H. J., et al. (2020). Cardiac amyloidosis: evolving diagnosis and management: a scientific statement from the american heart association. Circulation 142, e7–e22.
Koirala, P., Seong, S. H., Jung, H. A., and Choi, J. S. (2017). Comparative molecular docking studies of lupeol and lupenone isolated from Pueraria lobata that inhibits BACE1: probable remedies for Alzheimer’s disease. Asian Pac. J. Trop. Med. 10, 1117–1122. doi: 10.1016/j.apjtm.2017.10.018
Lange, J., Lunde, K. A., Sletten, C., Møller, S. G., Tysnes, O. B., Alves, G., et al. (2015). Association of a BACE1 gene polymorphism with Parkinson’s disease in a Norwegian population. Parkinsons. Dis. 2015:973298. doi: 10.1155/2015/973298
Li, F., Wang, Y., Yang, H., Xu, Y., Zhou, X., Zhang, X., et al. (2019). The effect of BACE1-AS on β-amyloid generation by regulating BACE1 mRNA expression. BMC Mol. Biol. 20:23. doi: 10.1186/s12867-019-0140-0
Li, P., Xu, Y., Wang, B., Huang, J., and Li, Q. (2020). miR-34a-5p and miR-125b-5p attenuate Aβ-induced neurotoxicity through targeting BACE1. J. Neurol. Sci. 413:116793. doi: 10.1016/j.jns.2020.116793
Li, Y., Fang, J., Zhou, Z., Zhou, Q., Sun, S., Jin, Z., et al. (2020). Downregulation of lncRNA BACE1-AS improves dopamine-dependent oxidative stress in rats with Parkinson’s disease by upregulating microRNA-34b-5p and downregulating BACE1. Cell Cycle 19, 1158–1171. doi: 10.1080/15384101.2020.1749447
Lim, S., Yoo, B. K., Kim, H.-S., Gilmore, H. L., Lee, Y., and Lee, H. - (2014). Amyloid-β precursor protein promotes cell proliferation and motility of advanced breast cancer. BMC Cancer 14:928. doi: 10.1186/1471-2407-14-928
Liu, C., Wang, H., Tang, L., Huang, H., Xu, M., Lin, Y., et al. (2021). LncRNA BACE1-AS enhances the invasive and metastatic capacity of hepatocellular carcinoma cells through mediating miR-377-3p/CELF1 axis. Life Sci. 275:119288. doi: 10.1016/j.lfs.2021.119288
Liu, T., Huang, Y., Chen, J., Chi, H., Yu, Z., Wang, J., et al. (2014). Attenuated ability of BACE1 to cleave the amyloid precursor protein via silencing long noncoding RNA BACE1-AS expression. Mol. Med. Rep. 10, 1275–1281. doi: 10.3892/mmr.2014.2351
Luo, Y., Bolon, B., Kahn, S., Bennett, B. D., Babu-Khan, S., Denis, P., et al. (2001). Mice deficient in BACE1, the Alzheimer’s β-secretase, have normal phenotype and abolished β-amyloid generation. Nat. Neurosci. 4, 231–232. doi: 10.1038/85059
Ma, L., Bajic, V. B., and Zhang, Z. (2013). On the classification of long non-coding RNAs. RNA Biol. 10, 925–933.
Martinez-Usatorre, A., and De Palma, M. (2021). Alzheimer’s drug turns macrophages against cancer. Nat. Cancer 2, 1119–1121. doi: 10.1038/s43018-021-00284-8
Modarresi, F., Faghihi, M. A., Patel, N. S., Sahagan, B. G., Wahlestedt, C., and Lopez-Toledano, M. A. (2011). Knockdown of BACE1-AS nonprotein-coding transcript modulates beta-amyloid-related hippocampal neurogenesis. Int. J. Alzheimer Dis. 2011:929042. doi: 10.4061/2011/929042
Morris, G. P., Clark, I. A., and Vissel, B. (2014). Inconsistencies and controversies surrounding the amyloid hypothesis of Alzheimer’s disease. Acta Neuropathol. Commun. 2:135. doi: 10.1186/s40478-014-0135-5
Munir, H., Jones, J. O., Janowitz, T., Hoffmann, M., Euler, M., Martins, C. P., et al. (2021). Stromal-driven and Amyloid β-dependent induction of neutrophil extracellular traps modulates tumor growth. Nat. Commun. 12:683. doi: 10.1038/s41467-021-20982-2
Najafi, S., Tan, S. C., Raee, P., Rahmati, Y., Asemani, Y., Lee, E. H. C., et al. (2022b). Gene regulation by antisense transcription: a focus on neurological and cancer diseases. Biomed. Pharmacother. 145:112265. doi: 10.1016/j.biopha.2021.112265
Najafi, S., Ghafouri-Fard, S., Hussen, B. M., Hidayat, H., Taheri, M., and Hallajnejad, M. (2022a). Oncogenic roles of small nucleolar RNA host gene 7 (SNHG7) long non-coding RNA in human cancers and potentials. Front. Cell Dev. Biol. 9:809345. doi: 10.3389/fcell.2021.809345
Nie, Y., Li, Y., Xu, Y., Jiao, Y., and Li, W. (2020). Long non-coding RNA BACE1-AS is an independent unfavorable prognostic factor in liver cancer. Oncol. Lett. 20:202. doi: 10.3892/ol.2020.12065
Pang, L., Hu, J., Zhang, G., Li, X., Zhang, X., Yu, F., et al. (2016). Dysregulated long intergenic non-coding RNA modules contribute to heart failure. Oncotarget 7, 59676–59690. doi: 10.18632/oncotarget.10834
Park, S. Y., Kim, H. Y., Park, H. J., Shin, H. K., Hong, K. W., and Kim, C. D. (2016). Concurrent treatment with taxifolin and cilostazol on the lowering of β-amyloid accumulation and neurotoxicity via the suppression of P-JAK2/P-STAT3/NF-κB/BACE1 signaling pathways. PLoS One 11:e0168286. doi: 10.1371/journal.pone.0168286
Peters, H. L., Tuli, A., Wang, X., Liu, C., Pan, Z., Ouellette, M. M., et al. (2012). Relevance of amyloid precursor-like protein 2 C-terminal fragments in pancreatic cancer cells. Int. J. Oncol. 41, 1464–1474. doi: 10.3892/ijo.2012.1553
Quinn, J. J., and Chang, H. Y. (2016). Unique features of long non-coding RNA biogenesis and function. Nat. Rev. Genet. 17, 47–62. doi: 10.1038/nrg.2015.10
Rahmati, Y., Asemani, Y., Aghamiri, S., Ezzatifar, F., and Najafi, S. (2021). CiRS-7/CDR1as; an oncogenic circular RNA as a potential cancer biomarker. Pathol. Res. Pract. 227:153639. doi: 10.1016/j.prp.2021.153639
Rinn, J. L., and Chang, H. Y. (2012). Genome regulation by long noncoding RNAs. Annu. Rev. Biochem. 81, 145–166.
Sadeghi, I., Bakhshinejad, B., Moradi Rehabdarkolaee, H., and Sadeghizadeh, M. (2018). BACE1-AS non-coding RNA increases apoptosis in HAOEC endothelial cells. Pathobiol. Res. 20, 53–66.
Sarropoulos, I., Marin, R., Cardoso-Moreira, M., and Kaessmann, H. (2019). Developmental dynamics of lncRNAs across mammalian organs and species. Nature 571, 510–514. doi: 10.1038/s41586-019-1341-x
Sathya, M., Premkumar, P., Karthick, C., Moorthi, P., Jayachandran, K. S., and Anusuyadevi, M. (2012). BACE1 in Alzheimer’s disease. Clin. Chim. Acta 414, 171–178.
Sayad, A., Najafi, S., Kashi, A. H., Hosseini, S. J., Akrami, S. M., Taheri, M., et al. (2022). Circular RNAs in renal cell carcinoma: functions in tumorigenesis and diagnostic and prognostic potentials. Pathol. Res. Pract. 229:153720. doi: 10.1016/j.prp.2021.153720
Schlackow, M., Nojima, T., Gomes, T., Dhir, A., Carmo-Fonseca, M., and Proudfoot, N. J. (2017). Distinctive patterns of transcription and RNA processing for human lincRNAs. Mol. Cell. 65, 25–38. doi: 10.1016/j.molcel.2016.11.029
Schneider, R. B., Iourinets, J., and Richard, I. H. (2017). Parkinson’s disease psychosis: presentation, diagnosis and management. Neurodegener. Dis. Manag. 7, 365–376. doi: 10.2217/nmt-2017-0028
Sparber, P., Filatova, A., Khantemirova, M., and Skoblov, M. (2019). The role of long non-coding RNAs in the pathogenesis of hereditary diseases. BMC Med. Genom. 12:42. doi: 10.1186/s12920-019-0487-6
Statello, L., Guo, C.-J., Chen, L.-L., and Huarte, M. (2021). Gene regulation by long non-coding RNAs and its biological functions. Nat. Rev. Mol. Cell Biol. 22, 96–118.
Su, M., Xiao, Y., Ma, J., Tang, Y., Tian, B., Zhang, Y., et al. (2019). Circular RNAs in cancer: emerging functions in hallmarks, stemness, resistance and roles as potential biomarkers. Mol. Cancer 18:90. doi: 10.1186/s12943-019-1002-6
Taheri, M., Najafi, S., Basiri, A., Hussen, B. M., Baniahmad, A., Jamali, E., et al. (2021). The Role and clinical potentials of circular RNAs in prostate cancer. Front. Oncol. 11:781414. doi: 10.3389/fonc.2021.781414
Tu, J., Ma, L., Zhang, M., and Zhang, J. (2021). Long non-coding RNA SOX2 overlapping transcript aggravates H9c2 cell injury via the miR-215-5p/ZEB2 axis and promotes ischemic heart failure in a rat model. Tohoku J. Exp. Med. 254, 221–231. doi: 10.1620/tjem.254.221
Vassar, R., and Kandalepas, P. C. (2011). The β-secretase enzyme BACE1 as a therapeutic target for Alzheimer’s disease. Alzheimers Res. Ther. 3:20. doi: 10.1186/alzrt82
Wang, D., Wang, P., Bian, X., Xu, S., Zhou, Q., Zhang, Y., et al. (2020). Elevated plasma levels of exosomal BACE1-AS combined with the volume and thickness of the right entorhinal cortex may serve as a biomarker for the detection of Alzheimer’s disease. Mol. Med. Rep. 22, 227–238. doi: 10.3892/mmr.2020.11118
Wang, H., Wang, X., Zhang, Y., and Zhao, J. (2021). LncRNA SNHG1 promotes neuronal injury in Parkinson’s disease cell model by miR-181a-5p/CXCL12 axis. J. Mol. Histol. 52, 153–163. doi: 10.1007/s10735-020-09931-3
Wang, M., Chen, D., Xu, Y., Qiu, M., Jiang, X., and Xiong, Z. (2021). Identification and validation of the lncRNA BACE1-AS as immune-related influencing factor in tumorigenesis following pan-carcinoma analysis. J. Immunol. Res. 2021:1589864. doi: 10.1155/2021/1589864
Wapinski, O., and Chang, H. Y. (2011). Long noncoding RNAs and human disease. Trends Cell Biol. 21, 354–361.
Xu, Y. L., Liu, Y., Cai, R. P., He, S. R., Dai, R. X., Yang, X. H., et al. (2020). Long non-coding RNA CASC7 is associated with the pathogenesis of heart failure via modulating the expression of miR-30c. J. Cell. Mol. Med. 24, 11500–11511. doi: 10.1111/jcmm.15764
Yaghoobi, H., Azizi, H., Banitalebi-Dehkordi, M., Mohammad Rezaei, F., Arsang-Jnag, S., Taheri, M., et al. (2019). Beta-secretase 1 (BACE1) is down-regulated in invasive ductal carcinoma of breast. Rep. Biochem. Mol. Biol. 8, 200–207.
Yeganeh, Z., Nabi, M., Solymani, S., Yazdian, S., Razavinia, A., and Khalili, A. (2020). The role of long-range non-coding RNAs NeST, NTT, BACE1-AS, CCAT2, FOXCUT and HULC as predictor biomarkers in breast cancer. Gene Rep. 20:100687.
Yuan, C., Wei, Y., Hu, L., Tian, Y., and Liu, Y. (2019). MiR-574-3p accelerates cell migration and invasion through regulating BACE1 in non-small cell lung cancer. Panminerva Med. [Epub ahead of print]. doi: 10.23736/S0031-0808.19.03734-0
Zeng, T., Ni, H., Yu, Y., Zhang, M., Wu, M., Wang, Q., et al. (2019). BACE1-AS prevents BACE1 mRNA degradation through the sequestration of BACE1-targeting miRNAs. J. Chem. Neuroanat. 98, 87–96. doi: 10.1016/j.jchemneu.2019.04.001
Zhai, K., Huang, Z., Huang, Q., Tao, W., Fang, X., Zhang, A., et al. (2021). Pharmacological inhibition of BACE1 suppresses glioblastoma growth by stimulating macrophage phagocytosis of tumor cells. Nat. Cancer 2, 1136–1151. doi: 10.1038/s43018-021-00267-9
Zhang, W., Zhao, H., Wu, Q., Xu, W., and Xia, M. (2018). Knockdown of BACE1-AS by siRNA improves memory and learning behaviors in Alzheimer’s disease animal model. Exp. Ther. Med. 16, 2080–2086. doi: 10.3892/etm.2018.6359
Zhang, Y.-W., Thompson, R., Zhang, H., and Xu, H. (2011). APP processing in Alzheimer’s disease. Mol. Brain 4:3.
Zhao, J., Liu, X., Xia, W., Zhang, Y., and Wang, C. (2020). Targeting amyloidogenic processing of APP in Alzheimer’s disease. Front. Mol. Neurosci. 13:137. doi: 10.3389/fnmol.2020.00137
Zhao, P., Wang, Y., Zhang, L., Zhang, J., Liu, N., and Wang, H. (2021). Mechanism of long non-coding RNA metastasis-associated lung adenocarcinoma transcript 1 in lipid metabolism and inflammation in heart failure. Int. J. Mol. Med. 47:5. doi: 10.3892/ijmm.2020.4838
Zhong, Z., Ewers, M., Teipel, S., Bürger, K., Wallin, A., Blennow, K., et al. (2007). Levels of β-secretase (BACE1) in cerebrospinal fluid as a predictor of risk in mild cognitive impairment. Arch. Gen. Psychiatry 64, 718–726. doi: 10.1001/archpsyc.64.6.718
Zhou, Y., Ge, Y., Liu, Q., Li, Y.-X., Chao, X., Guan, J.-J., et al. (2021). LncRNA BACE1-AS promotes autophagy-mediated neuronal damage through the miR-214-3p/ATG5 signalling axis in Alzheimer’s disease. Neuroscience 455, 52–64. doi: 10.1016/j.neuroscience.2020.10.028
Zhuang, A., Calkin, A. C., Lau, S., Kiriazis, H., Donner, D. G., Liu, Y., et al. (2021). Loss of the long non-coding RNA OIP5-AS1 exacerbates heart failure in a sex-specific manner. iScience 24:102537. doi: 10.1016/j.isci.2021.102537
Keywords: BACE1, BACE1-AS, lncRNA, Alzheimer’s disease, cancer
Citation: Sayad A, Najafi S, Hussen BM, Abdullah ST, Movahedpour A, Taheri M and Hajiesmaeili M (2022) The Emerging Roles of the β-Secretase BACE1 and the Long Non-coding RNA BACE1-AS in Human Diseases: A Focus on Neurodegenerative Diseases and Cancer. Front. Aging Neurosci. 14:853180. doi: 10.3389/fnagi.2022.853180
Received: 12 January 2022; Accepted: 07 February 2022;
Published: 21 March 2022.
Edited by:
Yi Guo, Central South University, ChinaCopyright © 2022 Sayad, Najafi, Hussen, Abdullah, Movahedpour, Taheri and Hajiesmaeili. This is an open-access article distributed under the terms of the Creative Commons Attribution License (CC BY). The use, distribution or reproduction in other forums is permitted, provided the original author(s) and the copyright owner(s) are credited and that the original publication in this journal is cited, in accordance with accepted academic practice. No use, distribution or reproduction is permitted which does not comply with these terms.
*Correspondence: Mohammad Taheri, TW9oYW1tYWQudGFoZXJpQHVuaS1qZW5hLmRl; Mohammadreza Hajiesmaeili, bXJoYWppZXNtYWVpbGlAc2JtdS5hYy5pcg==