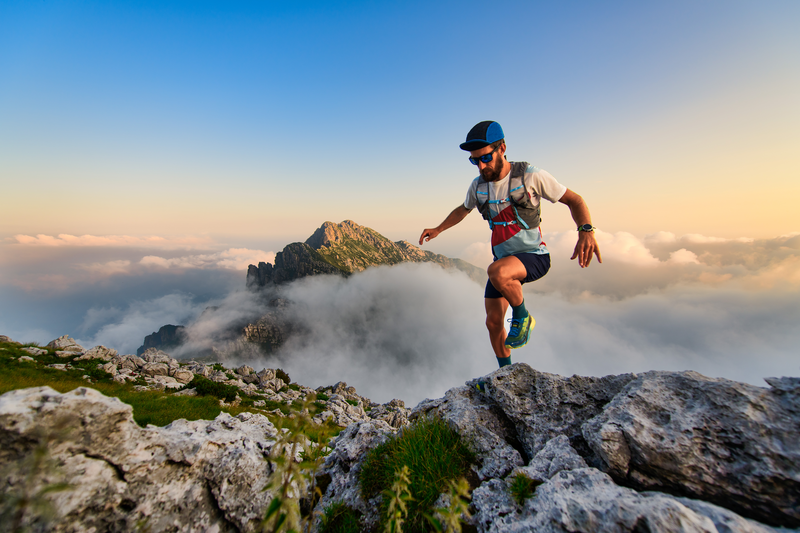
94% of researchers rate our articles as excellent or good
Learn more about the work of our research integrity team to safeguard the quality of each article we publish.
Find out more
REVIEW article
Front. Aging Neurosci. , 26 April 2022
Sec. Cellular and Molecular Mechanisms of Brain-aging
Volume 14 - 2022 | https://doi.org/10.3389/fnagi.2022.838173
This article is part of the Research Topic System Approaches to Advance Drug Discovery Against Aging and Age-Related Diseases View all 6 articles
Metformin, one of the first-line of hypoglycemic drugs, has cardioprotective, anti-inflammatory and anticancer activities, in addition to its proven hypoglycemic effects. Furthermore, the preventive and therapeutic potential of metformin for neurodegenerative diseases has become a topic of concern. Increasing research suggests that metformin can prevent the progression of neurodegenerative diseases. In recent years, many studies have investigated the neuroprotective effect of metformin in the treatment of neurodegenerative diseases. It has been revealed that metformin can play a neuroprotective role by regulating energy metabolism, oxidative stress, inflammatory response and protein deposition of cells, and avoiding neuronal dysfunction and neuronal death. On the contrary, some have hypothesized that metformin has a two-sided effect which may accelerate the progression of neurodegenerative diseases. In this review, the results of animal experiments and clinical studies are reviewed to discuss the application prospects of metformin in neurodegenerative diseases.
Metformin (dimethyl biguanide hydrochloride) is a natural derivative of guanidine, which is found in French cloves (Galega officinalis). It was originally described as a hypoglycemic and anti-malarial drug. The results of a multi-center randomized controlled trial (RCT) in the United Kingdom have profoundly influenced metformin as a treatment for type 2 diabetes (T2D) (No authors listed, 1998). Clinical studies have shown that enhanced glycemic control with metformin reduces the incidence of diabetes-related endpoints and all causes of mortality. In addition, metformin has been shown to extend life span in different animal models (Barini et al., 2016).
Metformin has been shown to decrease intestinal glucose absorption (Pryor and Cabreiro, 2015) and fasting plasma insulin level, increase insulin sensitivity, promotes muscle glucose uptake, activates AMP-activated protein kinase, and reduces liver gluconeogenesis. These helps lower blood glucose concentration without causing obvious hypoglycemia (Inzucchi et al., 2015). Also, metformin can lower the risk of cardiovascular disease (Luo et al., 2019), improve endothelial function in female patients with polycystic ovary syndrome(PCOS) (Heidari et al., 2019), and may prevent or cure age-related diseases (Orchard et al., 2005). More recently, other researches have suggested that metformin could inhibit many cancers, including lung, stomach, and endometrial cancers (Rizos and Elisaf, 2013).
Neurodegenerative diseases are caused by necrosis or loss of function of neurons, including Alzheimer’s disease (AD), Parkinson’s disease (PD) and Huntington’s disease (HD). When neurodegenerative disease occurs, the number of neurons in the brain decreases and synaptic signaling is disrupted. Aging cells become unable to maintain proteins in the correct folding state. This leads to decreased protein homeostasis in the brain, imbalance of epigenetic modifications such as DNA methylation, abnormal signal transduction between cells, inflammatory response and dysfunction of insulin in the brain. All these further aggravate the development of neurodegenerative diseases (Campbell et al., 2018). Increasing evidence shows that metformin can trigger autophagy, prevent oxidation, reduce neuroinflammation, and play a role in neuroprotection and neurorepair for a variety of nervous system diseases. In order to explore the prospect of metformin in neurodegenerative diseases, this paper reviewed the molecular mechanism of metformin, the progress of animal experimental research and some clinical retrospective studies.
The protective effect of metformin had been summarized in Figure 1. AMPK plays an important role of cellular energy metabolism in T2D patients. Activated AMPK regulates glucose and lipid metabolism, autophagy, apoptosis and other cellular processes (Dengler, 2020). Metformin acts on the respiratory chain complex I in the mitochondria, inhibiting ATP production, thereby increasing cytoplasmic ADP/ATP and AMP/ATP ratios. When the balance is disrupted and the ratio is increased, AMPK promotes catabolic pathways, increases ATP production and inhibits ATP consumption to restore cellular homeostasis (Rena et al., 2017). Besides, metformin might activate AMPK through a lysosomal pathway (Jia et al., 2020). Activated AMPK inhibits fat synthesis and promotes fat utilization through phosphorylation of ACC1 and ACC2, thereby reducing hepatic lipid storage and improving hepatic insulin sensitivity (Rena et al., 2017).
Figure 1. Mechanisms of metformin related to neuroprotection for NDD. The progression of neurodegenerative diseases has been shown to be associated with Tau phosphorylation, oxidative stress, amyloid protein accumulation and inflammation. Metformin can inhibit the NF-κB pathway, inhibit the astrocyte inflammation. In neurons, metformin directly inhibits Tau phosphorylation through PP2A. Most importantly, metformin inhibits respiratory chain complex 1, which inhibits energy production. This reduces ROS production, increases the AMP/ATP ratio, and then activates AMPK, which in turn inhibits Aβ plaque, protein synthesis, cell death and autophagy pathways. In general, metformin has great potential for neuroprotection based on current preclinical studies.
Insulin in the brain can regulate nutrient dynamic balance and cognitive function and has neurotrophic, neuroregulatory and neuroprotective effects. Insulin achieves its function by activating insulin receptor (IR) and its downstream pathway. IR is a family of tyrosine kinase receptors. The binding of islet to IR can phosphorylate IR dimer tyrosine, which in turn phosphorylates insulin receptor substrate (IRS), activating downstream phosphatidylinositol 3-kinase, PI3K/protein kinase B and mitogen-activated protein kinase (MAPK) to produce downstream effects (Manning and Cantley, 2007; Manning and Toker, 2017). Insulin resistance is associated with disturbances of upstream and downstream Akt signaling. During the progression of neurodegenerative changes, insulin gene expression level and IR or IRS protein expression are significantly decreased (Rivera et al., 2005). These results suggest that insulin resistance may play a role in the development of neurodegenerative diseases. Metformin is a commonly used insulin sensitizer in clinic, which can improve the sensitivity of IR to insulin. Metformin inhibits the PI3K/Akt signaling pathway through AMPK (Zakikhani et al., 2010). In addition, metformin down-regulates the expression of insulin and IGF-1 receptors and reduces the phosphorylation of insulin receptors, including IRS-1 (Sarfstein et al., 2013). Epidemiological studies have observed that metformin treatment reduces the risk of dementia in patients with diabetes (Zhou et al., 2015).
Reactive oxygen species (ROS) are by-products of aerobic cell energy metabolism and catecholamine neurotransmitter metabolism, which need to be eliminated in neuron. An imbalance in the production and clearance of ROS may lead to oxidative stress in biological systems, which may contribute to the development of neurodegenerative diseases. As a result, the antioxidant system is crucial to prevent any influence caused by ROS overproduction (Rotermund et al., 2018). Metformin plays a role in these pathways involved in disease progression by inhibiting complex I in mitochondria to slow oxidative phosphorylation and inhibit gluconeogenesis. This helps reduce the oxidative burden on neurons by minimizing Nicotinamide adenine dinucleotide (NADH) utilization (Rotermund et al., 2018). An et al. (2016) found that metformin had antioxidant effects on human umbilical vein endothelial cells (HUVECs) via an AMPK-dependent pathway. Metformin promoted endothelial nitric oxide synthase (eNOS) re-coupling via upregulation of GTPCH1 (guanosine 5′-triphosphate cyclohydrolase 1) and BH4 (tetrahydrobiopterin) levels. In addition, the level of nicotinamide adenine dinucleotide phosphate (NADPH) oxidase subunit p47-phox was down-regulated, thereby reducing ROS generation and BH4 oxidation. Metformin can also act as an antioxidant through other pathways. Metformin can activate fork head box O 3 (FOXO3), reducing ROS/RNS level in immune cells (Hartwig et al., 2021). Importantly, metformin has a concentration-dose effect, and high concentrations of metformin can lead to cell apoptosis through the accumulation of ROS. This metformin-induced effect may depend on its inhibition of nuclear factor erythroid 2 like 1 (NFE2L1) which crucially regulates cellular redox homeostasis (Gou et al., 2021). Cisplatin combined with metformin significantly down-regulated Nrf2 by inhibiting the Ras/Raf/ERK pathway in lung cancer cells, which promoting ROS-mediated apoptosis in the cells (Huang et al., 2020).
Neuroinflammation widely exists in various central nervous system diseases and is closely related to cognitive dysfunction. Studies have shown that neuroinflammation is involved in the pathogenesis of neurodegenerative diseases. Abnormal activation of microglia cells can lead to immune dysfunction and inflammation, in which microglia cells release inflammatory mediators and damage normal surrounding nerve tissue, gradually leading to degeneration and death of neurons (Zajda et al., 2020). Due to the continuous abnormal immunity of microglia, chronic neuroinflammation leads to the destruction of neurons and synaptic structures, resulting in the development of neurodegenerative diseases such as AD, PD, and HD.
Researches have claimed that metformin inhibits NF-κB (nuclear factor kappa-light-chain-enhancer of activated B cells) signaling and the production of pro-inflammatory cytokines of different cell types (Zhou et al., 2021). Docrat et al. (2021) demonstrated that NF plays a neuroprotective role by regulating protein phosphatase 2A (PP2A) dephosphorylation of Tau protein mediated by miR-141. In addition, metformin can be anti-inflammatory by restraining NF-κB mediated NLRP3 inflammase-associated transcription. Metformin may activate the PP2A pathway by decreasing the phosphorylation of PP2Ac at Y307 and disrupting the PP2AC-A4-MID1 interaction. In this way, inflammatory response of primary cardiomyocytes induced by high glucose stimulation were inhibited (Cheng and Li, 2020). Metformin has the ability to activate the AMPK signaling pathway while inhibiting the inflammatory response of bovine mammary epithelial cells. Meanwhile, metformin inhibited the acetylated histone H3 to inactivate the NF-κB, which in turn inhibits the expression of pro-inflammatory genes (Xu T. et al., 2021). Metformin inhibits the interaction between Caveolin1 and AMPKA, thereby reducing hyperglycemic stress and inflammation (Wang et al., 2021). In addition, metformin can reduce inflammatory response by inhibiting translocation of NF-κB, phosphorylation of ERK1/2 and p38 MAPK and reducing the production of inflammatory cytokines (TNFα, IL1β and IL6) (Tao et al., 2018).
Autophagy is an important cellular function that mediates the degradation of dysfunctional proteins and organelles in cells. In neurodegenerative diseases, autophagy is often dysregulated, promoting abnormal protein accumulation and organelle dysfunction (Moujalled et al., 2021). Researches have shown that metformin can promote autophagy in vivo and in vitro, to reduce Tau hyperphosphorylation and improve cognition, through APMK-dependent pathways (Chen et al., 2019). In addition, metformin can inhibit the infection activity of prions by activating autophagy pathways (Abdelaziz et al., 2020).
The traditional pathways of autophagy include PRKA (protein kinase activation)-mTOR-ULK1 signaling and the NADC-the sirtuin histone/protein deacetylase inhibitor (SIRT1) FOXO pathway. Interestingly, metformin has been confirmed to activate both PRKA and SIRT1 (Song et al., 2015). In addition, Bharath et al. (2020) discovered that metformin reduced age-related inflammation by promoting autophagy and normalizing mitochondrial function. It is worth mentioning that the activation of autophagy by metformin is not unidirectional. In tumor cells, metformin can exert anti-tumor effects through other interesting pathways of inhibition of autophagy. The GRP78 is a key driver of bortezomib-induced autophagy. However, studies have shown that metformin can inhibit glucose-regulated protein 78 (GRP78), which breaks the cell defense state and enhances the efficacy of bortezomib-induced autophagy against myeloma (Jagannathan et al., 2015).
Studies have shown that metformin has beneficial effects on metabolic and cellular processes closely related to the development of age-related diseases, such as regulating glucose metabolism, improving insulin sensitivity, and inhibiting oxidative stress, suggesting that metformin may have anti-aging potential (Barzilai et al., 2016). Metformin has been shown to regulate receptors for cytokines, insulin, IGF-1, and adiponectin, all of which are activated with age and associated with longevity. Metformin inhibits intracellular inflammatory pathways, activates AMPK, and increases inhibition of mTOR, which may be a major target for regulating aging. Metformin inhibits mitochondrial complex 1, regulates oxidative stress and clears senescent cells. Together, these processes affect inflammation, cell survival, stress defense, autophagy, and protein synthesis, which are major biological outcomes associated with aging/longevity (Barzilai et al., 2012). Although metformin has been shown to extend life, this may be because it reduces mortality from diseases, not just anti-aging. More studies are needed to clarify metformin’s anti-aging potential.
Metformin is a drug with an extensive range of targets, whose neuroprotective effects have been widely reported. Positive results have been achieved in animal models, as shown in Table 1. Metformin not only influences the occurrence and development of neurodegenerative diseases by reducing blood glucose, but it also has promising anti-oxidative, anti-inflammation, anti- senescence, inhibition of Tau protein phosphorylation, and other effects. Even though there have been many researches revealing the neuroprotective function of metformin, it is worth noting that some experimental results have been contradictory. The safety, multi-target, and mechanism of metformin’s effect on neuronal longevity have not been fully elucidated. Different experimental models, different combinations of drugs, and different genetic backgrounds of the experimental population could all contribute to this. We will conduct a systematic review of metformin’s potential protective role in neurodegenerative diseases in part.
Alzheimer’s disease is a progressive neurodegenerative disease among older individuals. The main histopathological feature is the extracellular deposition of amyloid B(Aβ) with microglia and astrocytes surrounding, leading to further neurotoxicity and organelle damage. This eventually leads to mitochondrial dysfunction, neuroinflammation and other pathological events (Yang and Zhang, 2020).
Studies have shown that dysregulation of AMPK is associated with AD. AMPK regulates Aβ production and tau phosphorylation, which is considered to be the molecular pathology of AD. However, there is paradox about the effect of AMPK on AD. On the one hand, in cultured neurons, AMPK activation inhibits Aβ production and tau phosphorylation, and reduces extracellular Aβ accumulation (Greco et al., 2009; Vingtdeux et al., 2010). On the other hand, AMPK-related signaling is activated in APP/PS1 transgenic AD mouse neurons, and AMPK inhibitors reduces ALR-related protein expression levels, thereby ameliorating memory deficits (Du et al., 2019). Oliveira et al. found that metformin activation of AMPK can reduce neuroinflammation and neuronal damage, improve cognition, and improve the symptoms of AD (Oliveira et al., 2021). But the underlying molecular/cellular mechanisms needed further clarification.
Insulin resistance is also thought to be a factor in the development of AD. Insulin resistance leads to dephosphorylation and activation of GSK-3β, resulting in increased production of Aβ, which in turn increases tau phosphorylation associated with NFT formation, contributing to many of the pathological features associated with AD. In addition, metformin is found to reduce Aβ level in APP/PS1 mice through insulin-degrading enzyme (IDE) pathway (Wang et al., 2020). Zhang et al. (2021) found that metformin can improve the AD-like phenotype induced by MC-LR by activating MTOR dependent PP2A and GSK-3β, thereby inhibiting tau phosphorylation at Ser202.
Studies have proved the close relationship between T2D and AD, which are believed to share some common pathophysiological characteristics (Zhou et al., 2015). Metformin therapy has been observed to reduce the risk of dementia in diabetes (Saffari et al., 2020). Elsewhere, metformin can improve the learning and memory capacity and neurological dysfunction of APP/PS1 mice by reducing Aβ (Lu et al., 2020). Farr et al. (2019) obtained the same conclusion that metformin facilitated learning and memory capacity in SAMP8 mice by reducing APPC99 and pTau proteins. High glucose can induce brain astrocyte inflammation and endoplasmic reticulum stress, which can promote the progression of AD. However, inflammation and endoplasmic reticulum stress are inhibited in metformin-pretreated astrocytes. This process may be mediated by metformin through inhibition of the formation of Caveolin1/AMPKα complex (Wang et al., 2021).
Metformin can improve microglia autophagy in APP/PS1 mice. Tau aggregates are injected into APP/PS1 mouse brain to promote the pathological progression of AD. Interestingly, metformin mitigates this pathological process by improving microglial autophagy, increasing the number of microglias around Aβ plaques, and promoting NP Tau phagocytosis (Chen et al., 2021). Xu X. et al. (2021) Found that metformin induces lysosomal dependent autophagy through AK1-IKK α/βHsc70 signaling, reversing the molecular and behavioral phenotypes of AD.
The other causes of AD are abnormal cholinergic systems and hippocampal synaptic transmission. Early AD is usually manifested by abnormal cholinergic function. The apoptosis of cholinergic neurons leads to a decrease in acetylcholine secretion. The deficiency of acetylcholine leads to cortical and hippocampal dysfunction, resulting in abnormal memory and movement of the body. Metformin can improve synaptic defects, memory problems and enhance cognition in animal models of AD (Pilipenko et al., 2020; Saffari et al., 2020). In the STZ-induced rat model, metformin can preserve the synaptic plasticity of the hippocampus, normalize the acetylcholine cleavage in the cortex and hippocampus tissues, and inhibit acetylcholinesterase activity (Pilipenko et al., 2020). This suggests that metformin can prevent neurogenic diseases potentially. In addition, Chen et al. (2020) revealed that metformin treatment can increase glutamate transmission rather than GABA transmission, which may be beneficial for the patients of AD or other relative brain disorders. Cyclin-dependent kinase 5(CDK5) is a serine/threonine kinase regulated by P35/P39 that plays an important role in synaptic plasticity and cognitive behavior. However, abnormal activation of CDK5 can lead to synaptic inhibition, resulting in the early pathological changes of Alzheimer’s disease (AD). A et al. found that metformin prevents Cdk5 overactivation by inhibiting the calpain-dependent cleavage of p35 into p25, thus preventing the onset of AD (Wang et al., 2020).
In the study involving individuals aged over 50 years in a Taiwan database, Hsu et al. (2011) suggested that metformin reduced the risk of dementia by 24% in T2D patients. When combined with sulfonylureas, it reduced that risk by 35%. Aaron et al. investigated its influence on neuroimaging and cognitive ability. Cerebral blood flow wasn’t changed significantly after metformin treatment, but there was significant improvement in executive function and a tendency to improve learning/memory and attention (Koenig et al., 2017). Clinical data suggest that metformin has a dual effect on cognition, which may be caused by B12 deficiency (Kuan et al., 2017). A prospective trial conducted in Australia (Moore et al., 2013) found that metformin is related to cognitive dysfunction, which can be alleviated by vitamin B12 and calcium. Campbell et al. (2018) advised that metformin can be used as the first-line therapy for diabetics to prevent dementia or AD. However, the successive use of metformin reduces vitamin B12 levels, which may account for the failure of metformin’s protective effect. Therefore, it is recommended that elderly patients with diabetes should be supplemented with vitamin B12 when taking metformin. Overall, more definitive experimental data are needed to determine whether metformin protects against dementia in non-diabetic patients.
Parkinson’s disease is a neurodegenerative disease mediated by a variety of factors. The main pathological features are the advanced decrease of dopaminergic neurons in the brain nigra and their projection to neurons in the striatum. Metformin has been shown to alleviate PD progression in a variety of ways.
The two cardinal pathologies of PD are degeneration of SNc-DA neurons and the accumulation of αSyn aggregates in Lewy bodies. AMPK subunits α-1 and α-2 have neuroprotective effects against αSyn toxicity, almost completely preventing the loss of dopaminergic neurons. Metformin affects neuronal function in rodent PD models through AMPK-mediated phosphorylation of αSyn (Perez-Revuelta et al., 2014). However, AMPK seems to be a double-edged sword in PD progress. Overactivated AMPK promotes the accumulation of α-SYN (184), resulting in SYN binding to GTPase pike-L and dopaminergic cell death (Ng et al., 2012). Sardoiwala et al. (2020) constructed polynanocarrier for Metformin delivery (Met Encapsulated PDANPs) to facilitate metformin to cross the blood-brain barrier. Then they found that metformin Encapsulated PDANPs promotes EZH2-mediated ubiquitination of α-SYN at PSer129, triggering the ubiquitin-proteasome degradation pathway of α-SYN, enhancing the neuroprotective effect of metformin and providing a new idea for optimization of metformin (Sardoiwala et al., 2020).
The BCAT-1 gene knockout Caenorhabditis elegans showed PD-like symptoms such as neurodegeneration with age, advanced dyskinesia and increased mitochondrial respiration (Mor et al., 2020; Sohrabi et al., 2021). Metformin can reduce mitochondrial respiration of BCAT-1 knockout Caenorhabditis elegans to a controlled level while improving motor function and neuronal activity (Mor et al., 2020). This suggests that metformin may offer a new treatment strategy against PD by inhibiting mitochondrial respiration. Metformin has neuroprotective effects on 6-hydroxydopamine-induced dopaminergic neurodegeneration and β -synuclein aggregation in nematode models of Parkinson’s disease. However, metformin do not significantly increase the mean or maximum lifespan of model animals, and the exact mechanism remains to be further studied (Saewanee et al., 2021).
Rotenone can cause oxidative stress and mitochondrial dysfunction, which are the main pathologic features of Parkinson’s disease. Therefore, it is often used to induce experimental Parkinson’s disease in cells and animals. Katila et al. (2021) found that metformin induces antioxidant system through NRF2-mediated transcriptional up egestion, thereby inhibiting intracellular oxidative stress, thereby restoring mitochondrial dysfunction and energy loss, and avoiding rotenone-induced SH-SY5Y cell death. In another rotenone-induced mouse model of PD, MTF reduces VEGF and Caspase3 activity by activating the AMPK-FOXO3 pathway, improving antioxidant capacity in the striatum and improving motor function in mice. In addition, MTF increases the number of tyrosine hydroxylase (TH) staining neurons in substantia nigra and striatum neurons, delaying the progression of PD (El-Ghaiesh et al., 2020).
Some studies have shown that neurotrophic factors such as glial cell-derived neurotrophic factor (GDNF) and brain-derived neurotrophic factor (BDNF) upregulate tyrosine hydroxylase (TH), improve movement disorders and provide neuroprotection in animal models of Parkinson’s disease (Gash et al., 2005; Lindholm and Saarma, 2021). Katila et al. (2020) indicated that by promoting the phosphorylation of tyrosine hydroxylase (TH), metformin simultaneously increases brain-derived and glial cell-derived neurotrophic factor (GDNF), thereby activating the cell survival signaling pathways.
Tayara et al. (2018) proposed that using metformin in diabetic populations at risk of PD be adequately evaluated. Their findings suggest that metformin has a paradoxical effect on nerves, with both beneficial and harmful effects. The authors further demonstrated the its anti-inflammatory function and overall immunosuppressive influence on microglia. However, Tayara et al. (2018) suggested that metformin was harmful to the dopamine system (Wahlqvist et al., 2012). According to the study, patients of T2D doubled the risk of PD, and with anti-hyperglycemia treatment, the risk of PD decreased to 1.3-fold. When sulfonylureas were used in combination with metformin, the HR decreased to 0.4, suggesting that sulfonylureas, in combination with metformin, had a protective effect on PD. A 12-year retrospective cohort study demonstrated that metformin increased risk of dementia and PD. Therefore, the authors suggest that successive metformin treatment in type 2 diabetes may contribute to the progress of neurodegenerative diseases (Kuan et al., 2017). However, the authors did not take into account vitamin B12 levels in the population, which may have influenced their final conclusions.
Huntington’s disease (HD) is a dominant inherited neurodegenerative disorder in which patients express high levels of Htt due to abnormal amplification of the first exon CAG of the gene encoding Huntington protein. When the CAG triplet of Htt is repeated more than 35 times, the mutant Huntingtin(mHtt) containing an abnormally long polyQ chain is produced. Improper folding and aggregation of mHtt produce toxic effects on striatum neurons, resulting in impaired motor coordination, chorea and progressive deterioration of cognitive function (Walker, 2007). There is no resultful therapy, but studies have shown that metformin has great therapeutic potential for this disease. A global observational clinical study of HD has assessed the effects of metformin on cognition, with linear analysis finding that metformin was related to the better cognitive ability of patients with HD (Hervas et al., 2017).
Animal experiments have shown that metformin can prolong the survival time of HD transgenic male mice (Ma et al., 2007). Dysfunction of the visual cortical circuits is one manifestation of the early effects of HD. Arnoux et al. (2018) found that in presymptomatic HD mice, neurons in the visual cortex were hyperactive and increased simultaneously, accompanied by behavioral abnormalities. Interestingly, metformin reduced mHtt via the MID1/PP2A complex and reduced S6 phosphorylation, which completely restored the mice’s early network activity pattern and behavioral abnormalities. These results show the perspective on the early prevention and treatment of HD with metformin.
The regulation of energy homeostasis by adenosine monophosphate activated kinase (AMPK) is crucial in cell survival and biological longevity, and its activation was proved in vivo and in vitro to be beneficial to the recovery of HD. Vazquez-Manrique et al. (2016) confirmed that the AMPK pathway is over activated in Caenorhabditis elegans and mouse HD models, and they suggested that metformin may work as a protective role in the early progression of HD. This conclusion was confirmed by Jin et al. (2016). They found that metformin may weaken the toxic effect of Htt through activating the AMPK pathway. In addition, Gomez-Escribano et al. (2020) found that the low doses of metformin and salicylate could synergistically activate AMPK and alleviate the neurotoxic effects of extended polyQ and α-synuclein, which provided a new idea for the combination of metformin.
Sanchis et al. (2019) observed that metformin alleviated HD symptoms in animal models in many ways. In the zQ175 Mouse model of HD, they found that metformin reduced the nuclear aggregation of mHtt in the striatum, partial recovery of neurotrophic factor expression, and decreased glial cell activation. In addition, metformin reduced polyglutamine aggregation and neuronal damage in a nematode model by an AMPK and lysosome dependent mechanism (Sanchis et al., 2019).
Sporadic cerebral amyloidosis (CAA) is caused by the deposition of amyloid (Aβ) protein in the blood vessels of the brain. It is the main cause of recurrent cerebral hemorrhage and dementia in the elderly (Viswanathan and Greenberg, 2011). Inoue et al. gave CAA and T2 DM mixed mouse model (APP23-ob/ob) metformin treatment, that Aβ in the cerebral cortex and hippocampus of APP23ob/ob mice significantly decreased at the 15th month. But IDE (Insulin-degrading enzyme) were significantly increased in the hippocampus, which is a kind of a Aβ-degrading enzyme. These results suggest that metformin can promote degradation of Aβ and reduce AB deposition in the cerebrovascular system (Inoue et al., 2021).
NPC1(Niemann–Pick disease type C1) is a neurodegenerative inherited recessive disorder caused by mutations in the NPC1 or NPC2 genes, characterized by disturbed intracellular transport of cholesterol and other lipids, resulting in the accumulation of unesterified cholesterol in the central nervous system (Vanier, 2015). HPβCD (2-Hydroxypropyl-β-cyclodextrin) is being developed to treat NPC1. Du et al. (2021) found that combination therapy of HPβCD and metformin in NPC1−/− mice reduced inflammatory responses in NPC1−/− mice and inhibited the release of pro-inflammatory cytokines in the organs. Notably, although the combination therapy did not prolong the lifespan of the mice and metformin did not reduce free cholesterol levels in NPC1 –/– brain tissue or fibroblasts, it provided a valuable exploration for the treatment of NPC1.
Nowadays, preventive and therapeutic function of metformin on neurodegenerative diseases have been extensively studied. Metformin can promote autophagy, prevent mitochondrial dysfunction, reduce neuroinflammation, and play a role in neuroprotection and neurorepair. However, some scholars believe that the efficacy and safety of metformin applying to neurodegenerative diseases needs further investigation. This may be due to differences in animal models and different drug combination effects. The clinical research progress of metformin in the therapy of neurodegenerative diseases is slow, most of which are the prospective or retrospective clinical studies. Large multicenter randomized controlled trials are still lacking. Metformin has two roles in the treatment of neurodegenerative diseases, according to current clinical research findings. This might be because Metformin can cause vitamin B12 deficiency among T2D patients which presents with diverse clinical presentations like cognitive impairment and dementia. Therefore, the neuroprotective ability of metformin may be enhanced by higher levels of vitamin B12. This review supports that metformin can potentially serve for the prevention and therapy of neurodegenerative diseases, which has been demonstrated by the existing literature on mechanism studies and animal experiments. However, good animal models and large sample randomized controlled trials are urgently needed to provide more adequate clinical evidence support.
M-RD and Q-YG: writing the original version. C-LL and L-YB: critical revision and corrections. F-LW and TL: idea, revision, and supervision. All authors contributed to the article and approved the submitted version.
This study was supported by Tangdu Hospital Seed Talent Program (F-LW).
The authors declare that the research was conducted in the absence of any commercial or financial relationships that could be construed as a potential conflict of interest.
All claims expressed in this article are solely those of the authors and do not necessarily represent those of their affiliated organizations, or those of the publisher, the editors and the reviewers. Any product that may be evaluated in this article, or claim that may be made by its manufacturer, is not guaranteed or endorsed by the publisher.
We would like to thank Tangdu Hospital, Fourth Military Medical University for supporting our work. We also would like to thank Home for Researchers (www.home-for-researchers.com) for a language polishing service.
Abdelaziz, D. H., Thapa, S., Abdulrahman, B., Vankuppeveld, L., and Schatzl, H. M. (2020). Metformin reduces prion infection in neuronal cells by enhancing autophagy. Biochem. Biophys. Res. Commun. 523, 423–428. doi: 10.1016/j.bbrc.2019.12.074
An, H., Wei, R., Ke, J., Yang, J., Liu, Y., Wang, X., et al. (2016). Metformin attenuates fluctuating glucose-induced endothelial dysfunction through enhancing GTPCH1-mediated eNOS recoupling and inhibiting NADPH oxidase. J. Diabetes Complications 30, 1017–1024. doi: 10.1016/j.jdiacomp.2016.04.018
Arnoux, M., Griesche, N., Krummeich, J., Watari, H., Offermann, N., Weber, S., et al. (2018). Metformin reverses early cortical network dysfunction and behavior changes in Huntington’s disease. Elife 7:e38744. doi: 10.7554/eLife.38744
Barini, E., Antico, O., Zhao, Y., Asta, F., Tucci, V., Catelani, T., et al. (2016). Metformin promotes tau aggregation and exacerbates abnormal behavior in a mouse model of tauopathy. Mol. Neurodegener. 11:16. doi: 10.1186/s13024-016-0082-7
Barzilai, N., Crandall, J. P., Kritchevsky, S. B., and Espeland, M. A. (2016). Metformin as a tool to target aging. Cell Metab. 23, 1060–1065. doi: 10.1016/j.cmet.2016.05.011
Barzilai, N., Huffman, D. M., Muzumdar, R. H., and Bartke, A. (2012). The critical role of metabolic pathways in aging. Diabetes 61, 1315–1322. doi: 10.2337/db11-1300
Bharath, L. P., Agrawal, M., McCambridge, G., Nicholas, D. A., Hasturk, H., Liu, J., et al. (2020). Metformin enhances autophagy and normalizes mitochondrial function to alleviate aging-associated inflammation. Cell Metab. 32, 44–55.e6. doi: 10.1016/j.cmet.2020.04.015
Campbell, J. M., Stephenson, M. D., de Courten, B., Chapman, I., Bellman, S. M., and Aromataris, E. (2018). Metformin use associated with reduced risk of dementia in patients with diabetes: a systematic review and meta-analysis. J. Alzheimers Dis. 65, 1225–1236. doi: 10.3233/JAD-180263
Chen, J. L., Luo, C., Pu, D., Zhang, G. Q., Zhao, Y. X., Sun, Y., et al. (2019). Metformin attenuates diabetes-induced tau hyperphosphorylation in vitro and in vivo by enhancing autophagic clearance. Exp. Neurol. 311, 44–56. doi: 10.1016/j.expneurol.2018.09.008
Chen, W. B., Chen, J., Liu, Z. Y., Luo, B., Zhou, T., and Fei, E. K. (2020). Metformin enhances excitatory synaptic transmission onto hippocampal CA1 pyramidal neurons. Brain Sci. 10:706. doi: 10.3390/brainsci10100706
Chen, Y., Zhao, S., Fan, Z., Li, Z., Zhu, Y., Shen, T., et al. (2021). Metformin attenuates plaque-associated tau pathology and reduces amyloid-beta burden in APP/PS1 mice. Alzheimers Res. Ther. 13:40. doi: 10.1186/s13195-020-00761-9
Cheng, G., and Li, L. (2020). High-glucose-induced apoptosis, ROS production and pro-inflammatory response in cardiomyocytes is attenuated by metformin treatment via PP2A activation. J. Biosci. 45:126.
Dengler, F. (2020). Activation of AMPK under hypoxia: many roads leading to rome. Int. J. Mol. Sci. 21:2428. doi: 10.3390/ijms21072428
Docrat, T. F., Nagiah, S., and Chuturgoon, A. A. (2021). Metformin protects against neuroinflammation through integrated mechanisms of miR-141 and the NF-kB-mediated inflammasome pathway in a diabetic mouse model. Eur. J. Pharmacol. 903:174146. doi: 10.1016/j.ejphar.2021.174146
Du, J., Liu, X., Zhang, Y., Han, X., Ma, C., Liu, Y., et al. (2021). The effects of combined therapy with metformin and hydroxypropyl-beta-cyclodextrin in a mouse model of niemann-pick disease type C1. Front. Pharmacol. 12:825425. doi: 10.3389/fphar.2021.825425
Du, S., Deng, Y., Yuan, H., and Sun, Y. (2019). Safflower yellow b protects brain against cerebral ischemia reperfusion injury through AMPK/NF-kB pathway. Evid. Based Complement Alternat. Med. 2019:7219740. doi: 10.1155/2019/7219740
El-Ghaiesh, S. H., Bahr, H. I., Ibrahiem, A. T., Ghorab, D., Alomar, S. Y., Farag, N. E., et al. (2020). Metformin protects from rotenone-induced nigrostriatal neuronal death in adult mice by activating AMPK-FOXO3 signaling and mitigation of angiogenesis. Front. Mol. Neurosci. 13:84. doi: 10.3389/fnmol.2020.00084
Farr, S. A., Roesler, E., Niehoff, M. L., Roby, D. A., McKee, A., and Morley, J. E. (2019). Metformin improves learning and memory in the SAMP8 mouse model of alzheimer’s disease. J. Alzheimers Dis. 68, 1699–1710. doi: 10.3233/JAD-181240
Gash, D. M., Zhang, Z., Ai, Y., Grondin, R., Coffey, R., and Gerhardt, G. A. (2005). Trophic factor distribution predicts functional recovery in parkinsonian monkeys. Ann. Neurol. 58, 224–233. doi: 10.1002/ana.20549
Gomez-Escribano, A. P., Bono-Yague, J., Garcia-Gimeno, M. A., Sequedo, M. D., Hervas, D., Fornes-Ferrer, V., et al. (2020). Synergistic activation of AMPK prevents from polyglutamine-induced toxicity in Caenorhabditis elegans. Pharmacol. Res. 161:105105. doi: 10.1016/j.phrs.2020.105105
Gou, S., Qiu, L., Yang, Q., Li, P., Zhou, X., Sun, Y., et al. (2021). Metformin leads to accumulation of reactive oxygen species by inhibiting the NFE2L1 expression in human hepatocellular carcinoma cells. Toxicol. Appl. Pharmacol. 420:115523. doi: 10.1016/j.taap.2021.115523
Greco, S. J., Sarkar, S., Johnston, J. M., and Tezapsidis, N. (2009). Leptin regulates tau phosphorylation and amyloid through AMPK in neuronal cells. Biochem. Biophys. Res. Commun. 380, 98–104. doi: 10.1016/j.bbrc.2009.01.041
Hartwig, J., Loebel, M., Steiner, S., Bauer, S., Karadeniz, Z., Roeger, C., et al. (2021). Metformin attenuates ROS via FOXO3 activation in immune cells. Front. Immunol. 12:581799. doi: 10.3389/fimmu.2021.581799
Heidari, B., Lerman, A., Lalia, A. Z., Lerman, L. O., and Chang, A. Y. (2019). Effect of metformin on microvascular endothelial function in polycystic ovary syndrome. Mayo Clin. Proc. 94, 2455–2466. doi: 10.1016/j.mayocp.2019.06.015
Hervas, D., Fornes-Ferrer, V., Gomez-Escribano, A. P., Sequedo, M. D., Peiro, C., Millan, J. M., et al. (2017). Metformin intake associates with better cognitive function in patients with Huntington’s disease. PLoS One 12:e0179283. doi: 10.1371/journal.pone.0179283
Hsu, C. C., Wahlqvist, M. L., Lee, M. S., and Tsai, H. N. (2011). Incidence of dementia is increased in type 2 diabetes and reduced by the use of sulfonylureas and metformin. J. Alzheimers Dis. 24, 485–493. doi: 10.3233/JAD-2011-101524
Huang, S., He, T., Yang, S., Sheng, H., Tang, X., Bao, F., et al. (2020). Metformin reverses chemoresistance in non-small cell lung cancer via accelerating ubiquitination-mediated degradation of Nrf2. Transl. Lung Cancer Res. 9, 2337–2355. doi: 10.21037/tlcr-20-1072
Inoue, Y., Masuda, T., Misumi, Y., Ando, Y., and Ueda, M. (2021). Metformin attenuates vascular pathology by increasing expression of insulin-degrading enzyme in a mixed model of cerebral amyloid angiopathy and type 2 diabetes mellitus. Neurosci. Lett. 762:136136. doi: 10.1016/j.neulet.2021.136136
Inzucchi, S. E., Bergenstal, R. M., Buse, J. B., Diamant, M., Ferrannini, E., Nauck, M., et al. (2015). Management of hyperglycemia in type 2 diabetes, 2015: a patient-centered approach: update to a position statement of the american diabetes association and the european association for the study of diabetes. Diabetes Care 38, 140–149. doi: 10.2337/dc14-2441
Jagannathan, S., Abdel-Malek, M. A., Malek, E., Vad, N., Latif, T., Anderson, K. C., et al. (2015). Pharmacologic screens reveal metformin that suppresses GRP78-dependent autophagy to enhance the anti-myeloma effect of bortezomib. Leukemia 29, 2184–2191. doi: 10.1038/leu.2015.157
Jia, J., Bissa, B., Brecht, L., Allers, L., Choi, S. W., Gu, Y., et al. (2020). AMPK, a regulator of metabolism and autophagy, is activated by lysosomal damage via a novel galectin-directed ubiquitin signal transduction system. Mol. Cell 77, 951–969.e9. doi: 10.1016/j.molcel.2019.12.028
Jin, H., Gu, N., Anders, M., Ren, T., Jiang, M., Tao, M., et al. (2016). Metformin protects cells from mutant huntingtin toxicity through activation of AMPK and modulation of mitochondrial dynamics. Neuromol. Med. 18, 581–592. doi: 10.1007/s12017-016-8412-z
Katila, N., Bhurtel, S., Park, P. H., Hong, J. T., and Choi, D. Y. (2020). Activation of AMPK/aPKCzeta/CREB pathway by metformin is associated with upregulation of GDNF and dopamine. Biochem. Pharmacol. 180:114193. doi: 10.1016/j.bcp.2020.114193
Katila, N., Bhurtel, S., Park, P.-H., and Choi, D.-Y. (2021). Metformin attenuates rotenone-induced oxidative stress and mitochondrial damage via the AKT/Nrf2 pathway. Neurochem. Int. 148:105120. doi: 10.1016/j.neuint.2021.105120
Koenig, A. M., Mechanic-Hamilton, D., Xie, S. X., Combs, M. F., Cappola, A. R., Xie, L., et al. (2017). Effects of the insulin sensitizer metformin in alzheimer disease: pilot data from a randomized placebo-controlled crossover study. Alzheimer Dis. Assoc. Disord. 31, 107–113. doi: 10.1097/WAD.0000000000000202
Kuan, Y. C., Huang, K. W., Lin, C. L., Hu, C. J., and Kao, C. H. (2017). Effects of metformin exposure on neurodegenerative diseases in elderly patients with type 2 diabetes mellitus. Prog. Neuropsychopharmacol. Biol. Psychiatry 79, 77–83. doi: 10.1016/j.pnpbp.2017.06.002
Lindholm, P., and Saarma, M. (2021). Cerebral dopamine neurotrophic factor protects and repairs dopamine neurons by novel mechanism. Mol. Psychiatry. doi: 10.1038/s41380-021-01394-6 [Epub ahead of print].
Lu, X. Y., Huang, S., Chen, Q. B., Zhang, D., Li, W., Ao, R., et al. (2020). Metformin ameliorates abeta pathology by insulin-degrading enzyme in a transgenic mouse model of alzheimer’s disease. Oxid. Med. Cell Longev. 2020:2315106. doi: 10.1155/2020/2315106
Luo, F., Das, A., Chen, J., Wu, P., Li, X., and Fang, Z. (2019). Metformin in patients with and without diabetes: a paradigm shift in cardiovascular disease management. Cardiovasc. Diabetol. 18:54. doi: 10.1186/s12933-019-0860-y
Ma, T. C., Buescher, J. L., Oatis, B., Funk, J. A., Nash, A. J., Carrier, R. L., et al. (2007). Metformin therapy in a transgenic mouse model of Huntington’s disease. Neurosci. Lett. 411, 98–103. doi: 10.1016/j.neulet.2006.10.039
Manning, B. D., and Cantley, L. C. (2007). AKT/PKB signaling: navigating downstream. Cell 129, 1261–1274. doi: 10.1016/j.cell.2007.06.009
Manning, B. D., and Toker, A. (2017). AKT/PKB Signaling: navigating the network. Cell 169, 381–405. doi: 10.1016/j.cell.2017.04.001
Moore, E. M., Mander, A. G., Ames, D., Kotowicz, M. A., Carne, R. P., Brodaty, H., et al. (2013). Increased risk of cognitive impairment in patients with diabetes is associated with metformin. Diabetes Care 36, 2981–2987. doi: 10.2337/dc13-0229
Mor, D. E., Sohrabi, S., Kaletsky, R., Keyes, W., Tartici, A., Kalia, V., et al. (2020). Metformin rescues Parkinson’s disease phenotypes caused by hyperactive mitochondria. Proc. Natl. Acad. Sci. U.S.A. 117, 26438–26447. doi: 10.1073/pnas.2009838117
Moujalled, D., Strasser, A., and Liddell, J. R. (2021). Molecular mechanisms of cell death in neurological diseases. Cell Death Differ. 28, 2029–2044. doi: 10.1038/s41418-021-00814-y
Ng, C. H., Guan, M. S., Koh, C., Ouyang, X., Yu, F., Tan, E. K., et al. (2012). AMP kinase activation mitigates dopaminergic dysfunction and mitochondrial abnormalities in Drosophila models of Parkinson’s disease. J. Neurosci. 32, 14311–14317. doi: 10.1523/JNEUROSCI.0499-12.2012
No authors listed (1998). Effect of intensive blood-glucose control with metformin on complications in overweight patients with type 2 diabetes (UKPDS 34). Lancet 352, 854–865. doi: 10.1016/s0140-6736(98)07037-8
Oliveira, W. H., Braga, C. F., Los, D. B., Araujo, S. M. R., Franca, M. R., Duarte-Silva, E., et al. (2021). Metformin prevents p-tau and amyloid plaque deposition and memory impairment in diabetic mice. Exp. Brain Res. 239, 2821–2839. doi: 10.1007/s00221-021-06176-8
Orchard, T. J., Temprosa, M., Goldberg, R., Haffner, S., Ratner, R., Marcovina, S., et al. (2005). The effect of metformin and intensive lifestyle intervention on the metabolic syndrome: the diabetes prevention program randomized trial. Ann. Intern. Med. 142, 611–619. doi: 10.7326/0003-4819-142-8-200504190-00009
Perez-Revuelta, B. I., Hettich, M. M., Ciociaro, A., Rotermund, C., Kahle, P. J., Krauss, S., et al. (2014). Metformin lowers Ser-129 phosphorylated alpha-synuclein levels via mTOR-dependent protein phosphatase 2A activation. Cell Death Dis. 5:e1209. doi: 10.1038/cddis.2014.175
Pilipenko, V., Narbute, K., Pupure, J., Langrate, I. K., Muceniece, R., and Klusa, V. (2020). Neuroprotective potential of antihyperglycemic drug metformin in streptozocin-induced rat model of sporadic Alzheimer’s disease. Eur. J. Pharmacol. 881:173290. doi: 10.1016/j.ejphar.2020.173290
Pryor, R., and Cabreiro, F. (2015). Repurposing metformin: an old drug with new tricks in its binding pockets. Biochem. J. 471, 307–322. doi: 10.1042/BJ20150497
Rena, G., Hardie, D. G., and Pearson, E. R. (2017). The mechanisms of action of metformin. Diabetologia 60, 1577–1585. doi: 10.1007/s00125-017-4342-z
Rivera, E. J., Goldin, A., Fulmer, N., Tavares, R., Wands, J. R., and de la Monte, S. M. (2005). Insulin and insulin-like growth factor expression and function deteriorate with progression of Alzheimer’s disease: link to brain reductions in acetylcholine. J. Alzheimers Dis. 8, 247–268. doi: 10.3233/jad-2005-8304
Rotermund, C., Machetanz, G., and Fitzgerald, J. C. (2018). The therapeutic potential of metformin in neurodegenerative diseases. Front. Endocrinol. 9:400 doi: 10.3389/fendo.2018.00400
Saewanee, N., Praputpittaya, T., Malaiwong, N., Chalorak, P., and Meemon, K. (2021). Neuroprotective effect of metformin on dopaminergic neurodegeneration and alpha-synuclein aggregation in C. elegans model of Parkinson’s disease. Neurosci. Res. 162, 13–21. doi: 10.1016/j.neures.2019.12.017
Saffari, P. M., Alijanpour, S., Takzaree, N., Sahebgharani, M., Etemad-Moghadam, S., Noorbakhsh, F., et al. (2020). Metformin loaded phosphatidylserine nanoliposomes improve memory deficit and reduce neuroinflammation in streptozotocin-induced Alzheimer’s disease model. Life Sci. 255:117861. doi: 10.1016/j.lfs.2020.117861
Sanchis, A., Garcia-Gimeno, M. A., Canada-Martinez, A. J., Sequedo, M. D., Millan, J. M., Sanz, P., et al. (2019). Metformin treatment reduces motor and neuropsychiatric phenotypes in the zQ175 mouse model of Huntington disease. Exp. Mol. Med. 51, 1–16. doi: 10.1038/s12276-019-0264-9
Sardoiwala, M. N., Srivastava, A. K., Kaundal, B., Karmakar, S., and Choudhury, S. R. (2020). Recuperative effect of metformin loaded polydopamine nanoformulation promoting EZH2 mediated proteasomal degradation of phospho-α-synuclein in Parkinson’s disease model. Nanomedicine 24:102088. doi: 10.1016/j.nano.2019.102088
Sarfstein, R., Friedman, Y., Attias-Geva, Z., Fishman, A., Bruchim, I., and Werner, H. (2013). Metformin downregulates the insulin/IGF-I signaling pathway and inhibits different uterine serous carcinoma (USC) cells proliferation and migration in p53-dependent or -independent manners. PLoS One 8:e61537. doi: 10.1371/journal.pone.0061537
Sohrabi, S., Mor, D. E., Kaletsky, R., Keyes, W., and Murphy, C. T. (2021). High-throughput behavioral screen in C. elegans reveals Parkinson’s disease drug candidates. Commun. Biol. 4:203. doi: 10.1038/s42003-021-01731-z
Song, Y. M., Lee, Y. H., Kim, J. W., Ham, D. S., Kang, E. S., Cha, B. S., et al. (2015). Metformin alleviates hepatosteatosis by restoring SIRT1-mediated autophagy induction via an AMP-activated protein kinase-independent pathway. Autophagy 11, 46–59. doi: 10.4161/15548627.2014.984271
Tao, L., Li, D., Liu, H., Jiang, F., Xu, Y., Cao, Y., et al. (2018). Neuroprotective effects of metformin on traumatic brain injury in rats associated with NF-kappaB and MAPK signaling pathway. Brain Res. Bull. 140, 154–161. doi: 10.1016/j.brainresbull.2018.04.008
Tayara, K., Espinosa-Oliva, A. M., Garcia-Dominguez, I., Ismaiel, A. A., Boza-Serrano, A., Deierborg, T., et al. (2018). Divergent effects of metformin on an inflammatory model of parkinson’s disease. Front. Cell Neurosci. 12:440. doi: 10.3389/fncel.2018.00440
Tourette, C., Farina, F., Vazquez-Manrique, R. P., Orfila, A. M., Voisin, J., Hernandez, S., et al. (2014). The Wnt receptor Ryk reduces neuronal and cell survival capacity by repressing FOXO activity during the early phases of mutant huntingtin pathogenicity. PLoS Biol. 12:e1001895. doi: 10.1371/journal.pbio.1001895
Vanier, M. T. (2015). Complex lipid trafficking in niemann-pick disease type C. J. Inherit. Metab. Dis. 38, 187–199. doi: 10.1007/s10545-014-9794-4
Vazquez-Manrique, R. P., Farina, F., Cambon, K., Dolores Sequedo, M., Parker, A. J., Millan, J. M., et al. (2016). AMPK activation protects from neuronal dysfunction and vulnerability across nematode, cellular and mouse models of Huntington’s disease. Hum. Mol. Genet. 25, 1043–1058. doi: 10.1093/hmg/ddv513
Vingtdeux, V., Giliberto, L., Zhao, H., Chandakkar, P., Wu, Q., Simon, J. E., et al. (2010). AMP-activated protein kinase signaling activation by resveratrol modulates amyloid-β peptide metabolism. J. Biol. Chem. 285, 9100–9113. doi: 10.1074/jbc.M109.060061
Viswanathan, A., and Greenberg, S. M. (2011). Cerebral amyloid angiopathy in the elderly. Ann. Neurol. 70, 871–880.
Wahlqvist, M. L., Lee, M. S., Hsu, C. C., Chuang, S. Y., Lee, J. T., and Tsai, H. N. (2012). Metformin-inclusive sulfonylurea therapy reduces the risk of Parkinson’s disease occurring with Type 2 diabetes in a Taiwanese population cohort. Parkinsonism Relat. Disord. 18, 753–758. doi: 10.1016/j.parkreldis.2012.03.010
Wang, G., Cui, W., Chen, S., Shao, Z., Li, Y., Wang, W., et al. (2021). Metformin alleviates high glucose-induced ER stress and inflammation by inhibiting the interaction between caveolin1 and AMPKalpha in rat astrocytes. Biochem. Biophys. Res. Commun. 534, 908–913. doi: 10.1016/j.bbrc.2020.10.075
Wang, Y., Zhao, J., Guo, F. L., Gao, X., Xie, X., Liu, S., et al. (2020). Metformin ameliorates synaptic defects in a mouse model of AD by inhibiting Cdk5 activity. Front. Cell Neurosci. 14:170. doi: 10.3389/fncel.2020.00170
Xu, T., Wu, X., Lu, X., Liang, Y., Mao, Y., Loor, J. J., et al. (2021). Metformin activated AMPK signaling contributes to the alleviation of LPS-induced inflammatory responses in bovine mammary epithelial cells. BMC Vet. Res. 17:97. doi: 10.1186/s12917-021-02797-x
Xu, X., Sun, Y., Cen, X., Shan, B., Zhao, Q., Xie, T., et al. (2021). Metformin activates chaperone-mediated autophagy and improves disease pathologies in an Alzheimer disease mouse model. Protein Cell 12, 769–787. doi: 10.1007/s13238-021-00858-3
Yang, Y., and Zhang, L. (2020). The effects of caloric restriction and its mimetics in Alzheimer’s disease through autophagy pathways. Food Funct. 11, 1211–1224. doi: 10.1039/c9fo02611h
Zajda, K. M., Sikora, H. J., Podsiedlik, M., and Markowicz-Piasecka, M. (2020). Is metformin a geroprotector? A peek into the current clinical and experimental data. Mech. Ageing Dev. 191:111350. doi: 10.1016/j.mad.2020.111350
Zakikhani, M., Blouin, M. J., Piura, E., and Pollak, M. N. (2010). Metformin and rapamycin have distinct effects on the AKT pathway and proliferation in breast cancer cells. Breast Cancer Res. Treat. 123, 271–279.
Zhang, Y., Fan, X., Su, Z., Yuan, T., Yin, H., Gu, H., et al. (2021). Pretreatment with metformin prevents microcystin-LR-induced tau hyperphosphorylation via mTOR-dependent PP2A and GSK-3beta activation. Environ. Toxicol. 36, 2414–2425. doi: 10.1002/tox.23354
Zhou, Y., Fang, R., Liu, L. H., Chen, S. D., and Tang, H. D. (2015). Clinical characteristics for the relationship between type-2 diabetes mellitus and cognitive impairment: a cross-sectional study. Aging Dis. 6, 236–244. doi: 10.14336/AD.2014.1004
Keywords: metformin, neurodegeneration, AMP-activated protein kinase, narrative review, pharmacology
Citation: Du M-R, Gao Q-Y, Liu C-L, Bai L-Y, Li T and Wei F-L (2022) Exploring the Pharmacological Potential of Metformin for Neurodegenerative Diseases. Front. Aging Neurosci. 14:838173. doi: 10.3389/fnagi.2022.838173
Received: 17 December 2021; Accepted: 11 March 2022;
Published: 26 April 2022.
Edited by:
Jinhui Wu, Sichuan University, ChinaReviewed by:
Ilia Stambler, Vetek (Seniority) Association – the Movement for Longevity and Quality of Life, IsraelCopyright © 2022 Du, Gao, Liu, Bai, Li and Wei. This is an open-access article distributed under the terms of the Creative Commons Attribution License (CC BY). The use, distribution or reproduction in other forums is permitted, provided the original author(s) and the copyright owner(s) are credited and that the original publication in this journal is cited, in accordance with accepted academic practice. No use, distribution or reproduction is permitted which does not comply with these terms.
*Correspondence: Fei-Long Wei, Zmx3ZWlzcGluZUBmbW11LmVkdS5jbg==; ZmVpbG9uZ3NwaW5lQDE2My5jb20=; Tian Li, dGlhbkBmbW11LmVkdS5jbg==
†These authors have contributed equally to this work
Disclaimer: All claims expressed in this article are solely those of the authors and do not necessarily represent those of their affiliated organizations, or those of the publisher, the editors and the reviewers. Any product that may be evaluated in this article or claim that may be made by its manufacturer is not guaranteed or endorsed by the publisher.
Research integrity at Frontiers
Learn more about the work of our research integrity team to safeguard the quality of each article we publish.