- 1The Key Research Laboratory of Benefiting Qi for Acting Blood Circulation Method to Treat Multiple Sclerosis of State Administration of Traditional Chinese Medicine, Research Center of Neurobiology, Shanxi University of Chinese Medicine, Jinzhong, China
- 2Department of Neurosurgery, Sinopharm Tongmei General Hospital, Datong, China
- 3Department of Physiology, Shanxi Medical University, Taiyuan, China
- 4Shanxi Key Laboratory of Inflammatory Neurodegenerative Diseases, Institute of Brain Science, Shanxi Datong University, Datong, China
In recent years, neurovascular unit (NVU) which is composed of neurons, astrocytes (Ast), microglia (MG), vascular cells and extracellular matrix (ECM), has become an attractive field in ischemic stroke. As the important component of NVU, Ast closely interacts with other constituents, which has been playing double-edged sword roles, beneficial or detrimental after ischemic stroke. Based on the pathophysiological changes, we evaluated some strategies for targeting Ast in treating ischemic stroke. The present review is focused on the roles of Ast in NVU and its complex signaling molecular network after ischemic stroke, which may be a prospective approach to the treatment of ischemic diseases in central nervous system.
Introduction
Ischemic stroke, caused neurological dysfunction due to lack of blood supply to the brain with various reasons, has a high morbidity and mortality (Zhao et al., 2020). In the world, ischemic stroke has reached for nearly 87% of the total stroke patients, and continues to rise annually (Saini et al., 2021). At present, the therapy for ischemic stroke is mainly based on thrombolysis and neuroprotection, but none of them has achieved a satisfactory effect. Increasing clinical studies have shown that neurons, astrocytes (Ast), blood brain barrier (BBB) are suffering different degrees of damage after ischemic stroke, which may explain that targeting neurons or one of other cells seemly exerts almost no significant curative effect. The concept of a neurovascular unit (NVU) was proposed by American scientists Lo et al. (2003), which provided a systematical view on related comprehensive studies. Ast, as one of important NVU components, are accompanied with neurons, microglia (MG) and BBB.
In addition, recent studies have demonstrated the beneficial or detrimental roles of Ast in neurodegenerative diseases (Ding et al., 2021). Therefore, in this review, we will mainly discuss the function of Ast in NVU, and the role of Ast in NVU after ischemic stroke and strategies for targeting Ast in the treatment of ischemic stroke.
The Functions of Astrocytes in Neurovascular Unit
Neurovascular unit refers to a dynamic structural complex composed of neurons, glial cells (Ast and MG), vascular cells (endothelial cells, smooth muscle cells, and pericytes), and extracellular matrix (ECM) that maintains the integrity of brain (Naranjo et al., 2021) (as shown in Figure 1). Physiological and pathological stimuli to NVU are affected by a complex molecular interaction between cell-cell, or cell-ECM, even or paracrine cell-cell communication. Intricate signal connections between each element of NVU may affect the whole function of the NVU if any component is damaged (Lo, 2010).
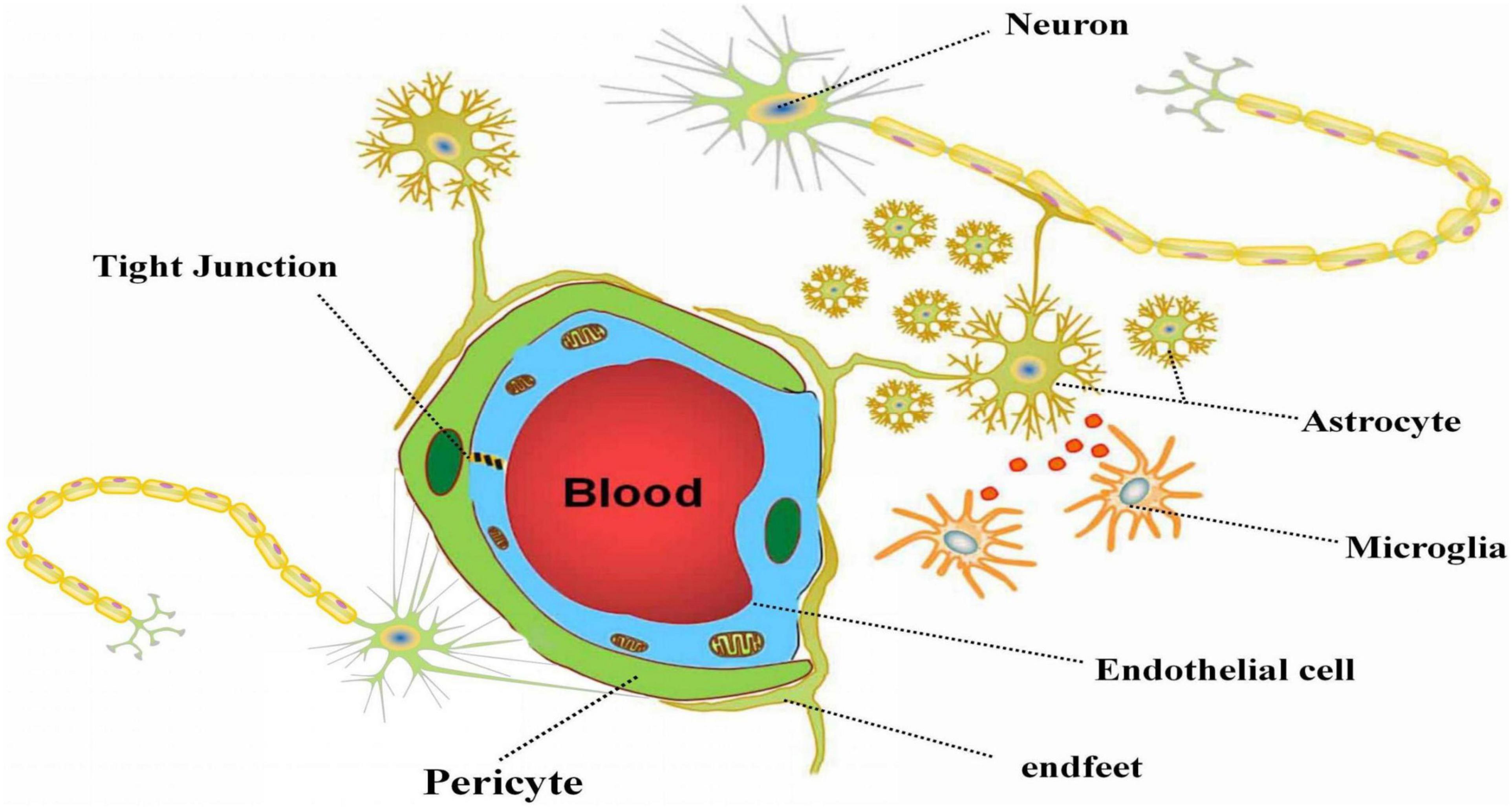
Figure 1. The composition of neurovascular unit. The figure depicts the composition of neurovascular unit and the relationship between the parts in NVU. Neurons, astrocytes, microglia, vascular cells and extracellular matrix together constitute the basic structure of NVU. Astrocytes play a vital role in NVU via connect neurons and vascular cells with the end feet, and carry out information transmission with microglia.
Ast, constituting nearly half of brain cells and outnumbering neurons, are particularly important in the NVU, perform multiple functions to maintain the survival and stability of NVU.
The Interaction Between Astrocytes and Neurons
Astrocytes, five times of neurons in number, play an important role in neuronal nutrition, support, protection and mutual signal transduction. On one hand, the protrusions of Ast surround the neurons and communicate with neurons and synapses. On the other hand, Ast wraps the blood vessels with the end feet and mediates the transmission of the biological information between neurons and blood vessels. Ast can not only regulate the transmission and strength of synapses, but also participate in the synaptic formation and neuronal differentiation (Stogsdill et al., 2017). Studies have shown that estrogen released by cultured primary Ast increased the number of newly formed synapses and enhanced synaptic signal transmission by activating the estrogen receptor-α on neurons (Fester and Rune, 2015). Thrombospondins-1/2 secreted by Ast promote the synaptic formation in the central nervous system (CNS) both in vivo and in vitro (Kim et al., 2017). Cholesterol released by Ast promotes synaptic formation and affects the synapse number during the development of neuron (Mauch et al., 2001). The most interesting is that rat embryonic stem cells could differentiate into neurons only in the medium containing Ast (Nakayama et al., 2003). In addition, Ast can provide energy and nutrients for neurons, and release citrate, an important substance supplying neuronal energy during hypoglycemia (Meshitsuka and Aremu, 2008) and produce lactic acid through glycolysis to provide some energy to neurons (Takahashi, 2020).
The Interaction Between Astrocytes and Microglia
As the innate immune cells in the brain, MG monitor the brain microenvironment, serving as the first line of the CNS defense (Li and Barres, 2018). Generally, MG and Ast are activated into two states: the neurontoxin phenotype (M1/A1) and the neuroprotection phenotype (M2/A2), corresponding to either the destructive or reparative functions in the NVU, respectively (Liu et al., 2020). Activated MG induce A1 polarization of Ast by secreting interleukin (IL)-1α, tumor necrosis factor-α (TNF-α) and complement component 1q (C1q). These cytokines are necessary and sufficient to induce A1 Ast, which lose the ability to promote neuronal survival, outgrowth, synaptogenesis and phagocytosis, inducing the death of neurons and oligodendrocytes (Liddelow et al., 2017). Similarly, MG can also release inflammatory factors such as IL-1β to block the production of sonic hedgehog by Asc, but to drive the secretion of CXCL2 and CCL2, further disrupting the integrity of the BBB and increase neuroinflammatory responses. Ast can release a large amount of IL-17A after stroke, and at the same time, IL-17AR is also highly expressed, and the transformation of MG to M1 type can be reduced by inhibiting IL-17A derived from Ast, which suggests that the polarization of M1-type microglia may be mediated by the IL-17 signaling and is closely related to Ast (Ronaldson and Davis, 2020). MG and Ast can also interact in a paracrine manner by secreting cytokines such as transforming growth factor-β (TGF-β), IL-1, ATP, etc. (Liu et al., 2011). Moreover, a specific pyrimidine-receptor related pathway between MG and Ast, may affect chronic inflammation of the nerve and reaction of glia (Quintas et al., 2014). In addition, MG also affect Ast in the NVU upon stimulation by LPS, whereby MG are activated to produce NO, which subsequently induces neuroprotective effects in Ast through the Keap1/Nrf2 pathway (Takahashi, 2020). MG also release IL-10 to act on reactive astrocytes (RAs), which allow Ast to release cytokines and to play an active protective role in NVU (Zagrean et al., 2018).
The Interaction Between Astrocytes and BBB
As a complex composed of the brain endothelial cells, pericytes, extracellular matrix basal lamina and astrocytic end-feet (Najjar et al., 2013), BBB is an important structure in the NVU and a natural barrier for the brain to maintain the microvascular homeostasis. Ast, one of the three types of cells involved in the formation of BBB, stretch out to wrap more than 90% of capillary endothelial cells and pericytes, interact with brain microvascular endothelial cells and maintain the integrity of BBB (Thurgur and Pinteaux, 2018). One of main structures of BBB is the endothelial cells and the tight junctions (TJs) between Ast. Ast can express endothelial transport molecules such as glucose transporter-1, and its end-feet can release the signal molecules to support the formation and maintenance of TJs. Inside BBB, Ast are interconnected with neighboring cells through the channels of gap junction that are regulated by extra- and intra-cellular signals and allow exchange of information (Bell et al., 2020). Ast-endothelial gap junctions are mediated by connexin-43 (CX43) hemichannels that allow cell-cell transfer of nutrients, neurotransmitters and maintain water and ion balance inside and outside blood vessels. In this process, there are complex signal exchanges between Ast, pericytes and endothelial cells (Haj-Yasein et al., 2011; He et al., 2013; Nuriya et al., 2013). Prat et al. (2001) found that cultured endothelial cells alone cannot form BBB unless co-cultured with Ast or glial cell culture fluid. Willis et al. (2004) performed a rat model of Ast injury and found that Ast was injured and died at 4–24 h, while BBB permeability increased after 24 h, and then gradually returned to normal at 6–8 days after BBB injury. Therefore, it can be speculated that Ast play an important role in the regulation of BBB permeability. Moreover, interaction between Ast and endothelial progenitor cells (EPCs) may mediate neurovascular remodeling after stroke (Hayakawa et al., 2012).
Pericytes are very important components of the NVU serving as integrators, coordinators and effectors of neurovascular functions, including the maintenance of BBB integrity (Zheng et al., 2020), regulation of cerebral blood flow (CBF) (Kisler et al., 2017a), clearance and phagocytosis of cellular debris (Sá-Pereira et al., 2012). The contraction and relaxation of pericytes play a key role in the regulation of local microvascular blood flow. Some studies have shown that a proportion of pericytes are contractile, responsive to brain-generated vasoactive signals, and are able to influence capillary diameter both in vitro and in vivo (Hall et al., 2014; Mishra et al., 2016), which can affect the perfusion of local brain and the redistribution of blood flow (Sagare et al., 2013; Kisler et al., 2017b; Montagne et al., 2018). Using two-photon microscopy to observe and manipulate brain capillary pericytes in vivo, Hartmann et al. (2021) found that vasoconstriction is associated with a reduction in red blood cell velocity and flux in the same capillary, suggesting that induced contraction of pericytes can alter CBF. As the key cells that regulate the contraction and the relaxation of brain microvasculature, pericytes can affect the formation and permeability of BBB and participate in the reperfusion of blood flow in the brain, which may be, in this sense, closely related to the fact that Ast can affect the Ca2+ concentration (Iadecola, 2017). Armulik et al. (2010) found that pericyte deficiency increases the permeability of the BBB, indicating that pericytes play an important role by integrating endothelial cells and Ast, and regulation of the BBB in NVU.
The Interaction Between Astrocytes and Extracellular Matrix
Extracellular matrix, secreted by neurons or glial cells, surrounding the dynamic structure of cells, provides support for cell arrangement, and performs a series of physiological functions within NVU (De Luca et al., 2020). As one of the components of NVU, ECM can maintain the homeostasis through constant exchanges of water, irons and nutrients in quantity and quality, which are related to the activities of specific enzymes responsible for the degradation of ECM, such as matrix metalloproteinases (MMPs) (Bonnans et al., 2014), Zn2+ dependent ECM remodeling endopeptidases (Kucera et al., 2016), playing an important role in the degradation of ECM and the regulation of cell-cell adhesion, migration, infiltration, proliferation, apoptosis (Quattromani et al., 2018), and neuronal synapse remodeling (Oohashi et al., 2015; Stanic et al., 2016; Peng et al., 2018). Studies have confirmed that Ast overexpress MMP-2 and MMP-9 after ischemic stroke, which destroy the structure of ECM and increase the permeability of the BBB (Keep et al., 2014). The main function of MMP-2 is to degrade the basement membrane of the ECM, while the MMP-9 is to degrade and remodel the dynamic balance of the ECM. Min et al. (2017) found that heme can stimulate Ast to release TLR2, then increase the expression of MMP9, causing the damage of ECM and BBB. Some cytokines secreted by Ast can cause changes in the composition and structural integrity of the ECM (De Luca et al., 2018), and conversely, the destruction of the ECM can also significantly influence the function of Ast.
The Role of Astrocytes in Neurovascular Unit After Ischemic Stroke
Under physiological conditions, Ast secret a variety of neurotrophic factors, such as glial cell-derived neurotrophic factor (GDNF), brain-derived neurotrophic factor (BDNF) and basic fibroblast growth factor (bFGF) at low levels. In the ischemic state, Ast are activated as demonstrated with its change of the morphology, the expression of marker proteins glial fibrillary acidic protein (GFAP) and the increase of vimentin (Pekny et al., 2019). After activated, on one hand, RAs have a strong tolerance under ischemia and protect neurons through various ways, including the release of neurotrophic factors, the uptake of excitatory amino acids, anti-inflammation, antioxidation and the formation of glial scar; on the other hand, they damage neurons by producing excitatory amino acids, activating MG to release inflammatory mediators, and reducing gap junctions, and thus disrupt the BBB. Therefore, Ast are a double-edged sword in the pathological process of cerebral ischemia, which has both neurotoxic and neuroprotective effects on the CNS (as shown in Figure 2).
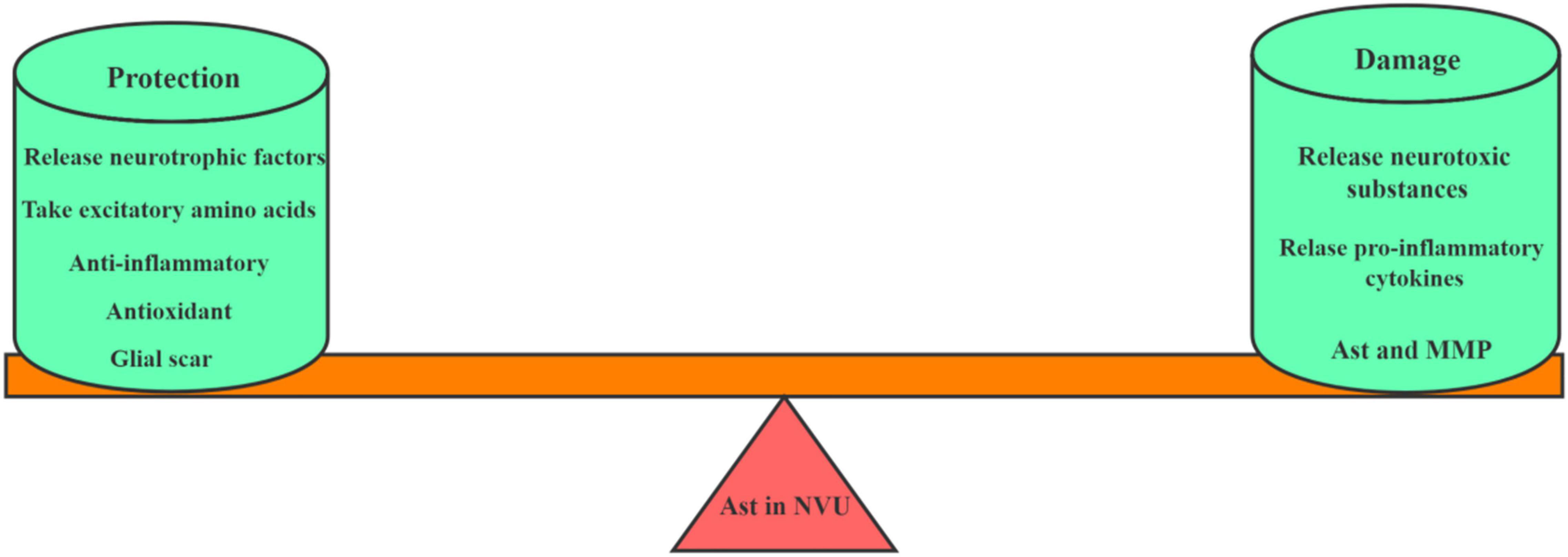
Figure 2. The role of Ast in NVU after ischemic stroke. This picture depicts the dual role of astrocytes in NVU after cerebral ischemia. On the one hand, Ast plays a protective role by secreting neurotrophic factors, taking excitatory amino acids, exerting anti-inflammatory effects and forming glial scars. On the other hand, Ast also plays a damaging role by releasing neurotoxic substances, secreting MMP, and aggravating pro-inflammatory reactions.
Protective Effects
The Release of Neurotrophic Factors
Ast are the main source of neurotrophic factors in the CNS. After cerebral ischemia, RAs secrete a variety of nutritional factors, including nerve growth factor (NGF), bFGF, BDNF, GDNF, vascular endothelial growth factor (VEGF) (Liu and Chopp, 2016), erythropoietin (EPO) (Roe, 2017) and neurotrophin-3 (NT-3) (Jurič et al., 2011), which can promote the regeneration of axons and blood vessels, the formation of myelin sheath, and synaptic plasticity. Among them, NGF can antagonize the activities of TNF-α and IL-1β to induce synaptogenesis and inhibit cell apoptosis, therefore exerting neuroprotective functions. bFGF can not only down-regulate the expression of GFAP and other markers to weaken the activation of Ast, but also regulate the upstream TLR4/NF-κB signal pathway to reduce the levels of pro-inflammatory cytokines such as IL-6 and TNF-α (Ye et al., 2015). The injection of bFGF into the lateral ventricle after cerebral ischemia can prevent neuronal damage (Liu et al., 2018). In ischemic stroke, BDNF plays a neuroprotective role in the regulation of signal pathways, the release of cytokines and the inhibition of apoptosis (Li et al., 2021). There is evidence indicating that lower BDNF serum level is associated with increased risk of stroke or transient ischemic attack (TIA) incidence (Pikula et al., 2013). Recent studies have shown that BDNF can also be used as a diagnostic marker for post-stroke complications and a prognostic parameter to predict the functional status of the patients with ischemic stroke (Lasek-Bal et al., 2015; Stanne et al., 2016). GDNF can alleviate learning and memory impairment caused by ischemia via promoting cell survival, neurite outgrowth and synapse formation. In addition, GDNF can also regulate Ast proliferation and inflammation in the ischemic penumbra, preventing premature and delayed neuronal death (Steliga et al., 2020). Ast may secrete VEGF, which helps to regenerate microvessels in nerve tissue, increase the density and number of blood vessels and regenerate nerves, and promote the repair of ischemic area. Besides, Ast also produce EPO, which protects hippocampal neurons from ischemic damage by activating Jak2 (Sola et al., 2005). Related studies have shown that EPO can reduce damage to neurons in vivo and in vitro.
The Uptake of Excitatory Amino Acids
Excitotoxic effect is one of the main mechanisms leading to neuronal death during cerebral ischemia. Glutamate (Glu) is mainly produced by Ast and is the main excitatory amino acid in the CNS. However, after the release of Glu, the superfluous Glu in the synaptic cleft can be uptaken by means of glutamate transporter-1 (GLT-1) and glutamate and aspartate transporter (GLAST) to play a neuroprotective effect (Becerra-Calixto and Cardona-Gómez, 2017; Mahmoud et al., 2019). Glu is ingested into Ast and quickly converted to glutamine, which returns to neurons through the glutamate-glutamine cycle (Lau et al., 2010), terminates the signal transduction of excitatory neurons, and maintains low Glu levels. After cerebral ischemia, the ability of RAs to uptake and transform Glu is enhanced, which reduces the accumulation of extracellular Glu, thereby reducing the secondary neurotoxicity caused by excitatory amino acids.
Anti-inflammation
Cerebral ischemia usually causes a series of inflammatory reactions, and Ast have a certain anti-inflammatory effect, which can protect the CNS and reduce inflammatory damage. At the peak of inflammation, Ast act as regulatory cells to secrete anti-inflammatory cytokines such as TGF-β and IL-10 to inhibit inflammatory response (Norden et al., 2014). Studies have shown that TGF-β1 is significantly upregulated in the human penumbra region after ischemic stroke. This might be because hypoxia induced stress of neuronal and astroglial cells increases growth factor expression (Krupinski et al., 1996). TGF-β1 upregulates BDNF and ciliary neuronal trophic factor (CNTF) and also induces autosynthesis of TGF-β1 in neurons, which may further explain the existence of the ischemic penumbra, where neurons survive longer because of a protective action of TGF-β1 (Krupinski et al., 1996). Meanwhile, Ast also release lipoxins (Lipoxin A4, LXA4) and (Lipoxin B4, LXB4) to inhibit neuroinflammation, and LXB4 is more effective in neuroprotection than LXA4 (Livne-Bar et al., 2017). Cerebral ischemia and hypoxia may induce the phenotype of A2, which up-regulates a variety of neurotrophic factors and inhibits the spread of inflammatory cells in the CNS to play a protective role in the brain (Li et al., 2019; Jing et al., 2021). In addition, Ast indirectly regulate the function of MG. By inhibiting the MG-mediated inflammatory response, limiting the infiltration of peripheral inflammatory cells and certain bacteria to cross the BBB, Ast repair the damaged BBB, inhibit apoptosis and degranulation of neutrophils, and enhance the phagocytic ability of neutrophils to inhibit the inflammation (Xie et al., 2010). Studies have shown that MG cultured with Ast-conditioned medium increase the expression of their anti-inflammatory gene named CX3CL1 (Li et al., 2018). In addition, Hung et al. (2016) showed that RAs released growth-related protein 43 (GAP-43) via the activation of the TLR4/NF-κB/STAT3 signal to inhibit MG and the inflammatory response.
Antioxidation
Under pathological conditions such as cerebral ischemia, a large amount of ROS and free radicals will be produced in the brain, causing oxidative damage to cells (Rinaldi et al., 2021). Reduced glutathione (GSH) in the brain is mainly derived from Ast, which scavenges oxygen free radicals, and participates in redox reactions, playing a vital role in anti-oxidative stress (Mashima et al., 2018). Firstly, Ast secrete insulin-like growth factor I and play an anti-oxidative stress effect by activating protein kinase B (Dávila et al., 2016). Secondly, Ast promote NF-E2-related factor 2 (Nrf2) to translocate into the nucleus and activate antioxidant response element (ARE), protecting Ast and adjacent neurons from oxidative damage (Zhou et al., 2015). Thirdly, Ast express Cx43 to form gap junctions counteracting oxidation, and secrete G protein-coupled receptor GPR37-like 1 to protect Ast from oxidative injury (Jolly et al., 2018).
The Formation of Glial Scar
After cerebral infarction, the inflammatory response in the brain promotes the activation of Ast and induces the formation of tight junctions of BBB. Meanwhile, RAs around the infarct area secrete extracellular matrix components, especially chondroitin sulfate proteoglycans. Together with the help of microglia/macrophages, glial scars are formed (Huang L. et al., 2014). In this way, a molecular barrier separates the injured area from the surrounding normal tissues, inhibiting the diffusion of toxic substances, and reducing neuronal death caused by excitatory amino acids and neurotoxic substances such as inflammatory mediators (Sato et al., 2008). Studies have found that although glial scars are powerful physical and endocytic barriers, they are unable to completely isolate the liquefied necrotic area from the viable brain tissue, and could not inhibit the neurotoxic factors present in the liquefied necrotic area. This permeability of glial scars may account for the fact that some stroke patients develop neurodegeneration after stroke (Zbesko et al., 2018).
Damaging Effects
The Release of Neurotoxic Substances and Pro-inflammatory Cytokines
Glutamate is an excitatory neurotransmitter. After cerebral ischemia, neurotoxic glutamate accumulates in the intercellular space in a large amount, causing intracellular calcium overloading, and aggravating neuronal damage or death. Ast may secrete a large number of inflammatory mediators such as TNF-α and IL-1β, activating MG to aggravate the degree of cerebral edema, and the inflammatory response of cerebral ischemia (Kadhim et al., 2008; Xin et al., 2021). Thus, activated glial cells, in turn, produce numerous inflammatory mediators which further stimulate the activation and proliferation of glial cells, thereby intensifying the inflammatory response and forming a vicious circle. In this process, TNF-α plays a pivotal role, which induces the release of neurotoxic substances such as glutamate and nitric oxide, and participates in the inflammatory response, while IL-β directly damages neurons.
The Secretion of Matrix Metalloproteinases by Astrocytes
Matrix metalloproteinases (MMPs) are composed of a family of zinc-dependent extracellular matrix remodeling endopeptidases (Kucera et al., 2016). In cerebral ischemic diseases, MMPs, secreted by Ast, may not only degrade extracellular matrix, but also destroy the integrity of BBB. Studies have confirmed that there is a certain relationship between acute ischemic stroke and MMPs. Among them, the level of MMP-9 is directly related to the area of cerebral infarction and the hemorrhagic transformation after cerebral ischemia. It was found the serum MMPs levels in the patients with acute ischemic stroke were significantly increased, which aggravated neuronal damage. Knocking out the MMPs gene or adding MMPs inhibitors significantly reduce the damage caused by stroke (Bae et al., 2016). In addition, a variety of drugs targeting MMP-2 and MMP-9 have protective effects on the brain in the mice with cerebral ischemia/reperfusion injury (Zeng et al., 2018).
Strategies for Targeting Astrocytes in the Treatment of Ischemic Stroke
Ast participate in the pathological process of ischemic stroke by activating or inhibiting a variety of signal pathways, and form a complex molecular signal network to the damaging and repairing process of local NVU at certain stages after ischemic stroke. Given that Ast plays a double-edged sword role on neurons in the process of ischemic stroke, a variety of drugs targeting Ast may have beneficial effects and reduce CNS damage, where ERK1/2 and TLR4/NF-κB pathways are mainly involved. Table 1 lists several drugs or molecules that can potentially target Ast to tackle cerebral ischemia and related mechanisms.
ERK1/2 Signal Pathway
ERK1/2 is a key regulator in the MARK signal pathway and plays an important role in cell proliferation and differentiation. Studies have shown that activation of the ERK1/2 signal pathway also has dual effects to ischemic stroke (Maddahi and Edvinsson, 2010). On one hand, inflammatory factors or oxidative stress activates ERK1/2, which aggravates NVU damage; on the other hand, after the activation of ERK1/2 signal pathway, neurotrophic factors secreted by Ast play a neuroprotective role (Sawe et al., 2008). Some drugs targeting Ast can affect ischemic stroke through the ERK1/2 pathway. For example, Potassium2-(1-hydroxypentyl)-benzoate (D, L-PHPB), a new drug candidate for ischemic stroke at the phase II clinic trial. By up-regulating ERK activity, D, L-PHPB significantly increases the levels of BDNF and NGF secreted by Ast to promote neuronal survival and inhibit neuronal apoptosis under OGD/R conditions in the co-culture system of neurons and Ast (Liu et al., 2017). D, L-PHPB treatment suggests that the neuroprotective effects may be related to Ast (Zhang et al., 2006; Hu et al., 2012; Zhao et al., 2013; Peng et al., 2014), but the mechanisms of D, L-PHPB remain to be further studied. Tanshinone IIA inhibits the accumulation of hypoxia inducible factor-1α and stromal cell derived factor-1 in Ast under OGD conditions, the activation of ERK1/2 and AKT, and the proliferation of Ast (Huang X. et al., 2014), thereby reducing the degree of injury.
TLR4/NF-κB Signal Pathway
TLR4 is the earliest discovered Toll-like protein. After cerebral ischemia, TLR4 is firstly activated in both Ast and MG. NF-κB is one of the important downstream signaling nuclear transcription factors of TLR4. Some cytokines such as IL-2, IL-1β and TNF-α in the cell can activate the NF-κB in turn, thereby stimulating further cascade amplification of inflammatory signals. Inhibiting the activation of the TLR4/NF-κB signal pathway improves the damage of ischemic stroke (Zhao et al., 2018). Studies have shown that ginkgo diterpene lactones attenuates ischemic injury by inhibiting the TLR4/NF-κB pathway, inhibiting platelet aggregation, astrocyte activation and pro-inflammatory cytokine production (Li et al., 2020). Pien Tze Huang (PTH) significantly reduces cerebral infarct volume in MCAO rats, improves neurological function scores and pathological damage, which may be related to reducing the expression of IL-1b, IL-6, TNF-α and MCP-1 by downregulating TLR4, MyD88 and TRAF6 and reducing the expression and nuclear translocation of NF-κB. In addition, PTH can also reduce the levels of p-ERK1/2 to exert neuroprotective effect after ischemia (Zhang et al., 2021). Fasudil inhibits the activation of TLR4/NF-κB signal pathway and inflammatory response, exerting a neuroprotective effect (Zhang H. et al., 2018). In addition, Enriched environment (EE) is a method of exogenous intervention on the nervous system through behavioral intervention, which promotes neurogenesis and functional recovery through NF-kB-mediated secretion of IL-17A by Ast in stroke patients (Zhang Y. et al., 2018).
Summary and Prospects
In summary, Ast, an important component in the NVU and closely connected to neurons, BBB and other glial cells, are activated in ischemic stroke and play an crucial double-edged sword role. On one hand, Ast play a protective role through anti-inflammatory, antioxidant, and release of neurotrophic factors. On the other hand, Ast release neurotoxic substances that damage BBB and increase the area of cerebral infarction. Various signal pathways form a complex signaling molecular network in the NVU, and Ast participate in this exquisite network and play an important regulatory role.
At present, there are still many difficulties in the treatment of ischemic stroke, and the interaction between signal molecules needs to be further elucidated. Although a variety of drugs have shown that targeting Ast has a certain positive effect on recovery after stroke, due to the complexity and diversity of the underlying mechanisms, most drugs are still in experimental research or phase I and phase II clinical trials. Given that Ast play a dual role in the integrity of the NVU, addressing homeostasis of Ast would be critical to tackle ischemic stroke-related conditions. Targeting Ast to treat ischemic stroke needs continuous attentions in the future.
Author Contributions
GH, LS, and QW outlined and drafted the manuscript. CM helped coordinate and draft the manuscript. ZD and YY were involved in the revisions. CM and JH revised and finalized the manuscript. All authors read and approved the final manuscript.
Funding
This work was supported by research grants from the National Natural Science Foundation of China (82004028 to LS and 81473577 to CM), Young Scientists Cultivation Project of Shanxi University of Chinese Medicine (2021PY-QN-09 to LS), the Department of Science and Technology, Shanxi Province of China (201803D421073 to YY and 201805D111009 to CM), Shanxi Applied Basic Research Project (201901D211538 to LS), Datong Municipal Science and Technology Bureau (2019198 to CM), Leading Team of Medical Science and Technology, Shanxi Province (2020TD05 to CM), China Postdoctoral Science Foundation (2020M680912 to LS), Cardiovascular special fund project of national regional traditional Chinese medicine medical center of Affiliated Hospital of Shanxi University of Chinese Medicine in 2021 (XGZX202115 to LS), and “Basic research project” of the cultivation plan of scientific and technological innovation ability of Shanxi University of Chinese Medicine (2020PY-JC-02 to LS).
Conflict of Interest
The authors declare that the research was conducted in the absence of any commercial or financial relationships that could be construed as a potential conflict of interest.
Publisher’s Note
All claims expressed in this article are solely those of the authors and do not necessarily represent those of their affiliated organizations, or those of the publisher, the editors and the reviewers. Any product that may be evaluated in this article, or claim that may be made by its manufacturer, is not guaranteed or endorsed by the publisher.
References
Armulik, A., Genové, G., Mäe, M., Nisancioglu, M. H., Wallgard, E., Niaudet, C., et al. (2010). Pericytes regulate the blood-brain barrier. Nature 468, 557–561. doi: 10.1038/nature09522
Bae, M. J., Karadeniz, F., Lee, S. G., Seo, Y., and Kong, C. S. (2016). Inhibition of MMP-2 and MMP-9 Activities by Limonium tetragonum Extract. Prev. Nutr. Food Sci. 21, 38–43. doi: 10.3746/pnf.2016.21.1.38
Becerra-Calixto, A., and Cardona-Gómez, G. P. (2017). The role of astrocytes in neuroprotection after brain stroke: potential in cell therapy. Front. Mol. Neurosci. 10:88. doi: 10.3389/fnmol.2017.00088
Bell, A. H., Miller, S. L., Castillo-Melendez, M., and Malhotra, A. (2020). The neurovascular unit: effects of brain insults during the perinatal period. Front. Neurosci. 13:1452. doi: 10.3389/fnins.2019.01452
Bonnans, C., Chou, J., and Werb, Z. (2014). Remodelling the extracellular matrix in development and disease. Nat. Rev. Mol. Cell Biol. 15, 786–801. doi: 10.1038/nrm3904
Dávila, D., Fernández, S., and Torres-Alemán, I. (2016). Astrocyte resilience to oxidative stress induced by Insulin-Like Growth Factor I (IGF-I) Involves Preserved AKT (Protein Kinase B) activity. J. Biol. Chem. 291, 2510–2523. doi: 10.1074/jbc.M115.695478
De Luca, C., Colangelo, A. M., Alberghina, L., and Papa, M. (2018). Neuro-immune hemostasis: homeostasis and diseases in the central nervous system. Front. Cell. Neurosci. 12:459. doi: 10.3389/fncel.2018.00459
De Luca, C., Colangelo, A. M., Virtuoso, A., Alberghina, L., and Papa, M. (2020). Neurons, glia, extracellular matrix and neurovascular unit: a systems biology approach to the complexity of synaptic plasticity in health and disease. Int. J. Mol. Sci. 21:1539. doi: 10.3390/ijms21041539
Ding, Z. B., Song, L. J., Wang, Q., Kumar, G., Yan, Y. Q., and Ma, C. G. (2021). Astrocytes: a double-edged sword in neurodegenerative diseases. Neural Regen. Res. 16, 1702–1710. doi: 10.4103/1673-5374.306064
Dong, C., Wen, S., Zhao, S., Sun, S., Zhao, S., Dong, W., et al. (2021). Salidroside inhibits reactive astrogliosis and glial scar formation in late cerebral ischemia via the Akt/GSK-3β Pathway. Neurochem. Res. 46, 755–769. doi: 10.1007/s11064-020-03207-8
Fester, L., and Rune, G. M. (2015). Sexual neurosteroids and synaptic plasticity in the hippocampus. Brain Res. 1621, 162–169. doi: 10.1016/j.brainres.2014.10.033
Haj-Yasein, N. N., Vindedal, G. F., Eilert-Olsen, M., Gundersen, G. A., Skare, Ø., Laake, P., et al. (2011). Glial-conditional deletion of aquaporin-4 (Aqp4) reduces blood-brain water uptake and confers barrier function on perivascular astrocyte endfeet. Proc. Natl. Acad. Sci. U.S.A. 108, 17815–17820. doi: 10.1073/pnas.1110655108
Hall, C. N., Reynell, C., Gesslein, B., Hamilton, N. B., Mishra, A., Sutherland, B. A., et al. (2014). Capillary pericytes regulate cerebral blood flow in health and disease. Nature 508, 55–60. doi: 10.1038/nature13165
Hartmann, D. A., Berthiaume, A. A., Grant, R. I., Harrill, S. A., Koski, T., Tieu, T., et al. (2021). Brain capillary pericytes exert a substantial but slow influence on blood flow. Nat. Neurosci. 24, 633–645. doi: 10.1038/s41593-020-00793-2
Hayakawa, K., Pham, L. D., Katusic, Z. S., Arai, K., and Lo, E. H. (2012). Astrocytic high-mobility group box 1 promotes endothelial progenitor cell-mediated neurovascular remodeling during stroke recovery. Proc. Natl. Acad. Sci. U.S.A. 109, 7505–7510. doi: 10.1073/pnas.1121146109
He, Q. W., Xia, Y. P., Chen, S. C., Wang, Y., Huang, M., Huang, Y., et al. (2013). Astrocyte-derived sonic hedgehog contributes to angiogenesis in brain microvascular endothelial cells via RhoA/ROCK pathway after oxygen-glucose deprivation. Mol. Neurobiol. 47, 976–987. doi: 10.1007/s12035-013-8396-8
Hu, Y., Peng, Y., Long, Y., Xu, S., Feng, N., Wang, L., et al. (2012). Potassium 2-(1-hydroxypentyl)-benzoate attenuated hydrogen peroxide-induced apoptosis in neuroblastoma SK-N-SH cells. Eur. J. Pharmacol. 680, 49–54. doi: 10.1016/j.ejphar.2012.01.031
Huang, L., Wu, Z. B., Zhuge, Q., Zheng, W., Shao, B., Wang, B., et al. (2014). Glial scar formation occurs in the human brain after ischemic stroke. Int. J. Med. Sci. 11, 344–348. doi: 10.7150/ijms.8140
Huang, X., Li, Y., Li, J., Feng, Y., and Xu, X. (2014). Tanshinone IIA dampens the cell proliferation induced by ischemic insult in rat astrocytes via blocking the activation of HIF-1α/SDF-1 signaling. Life Sci. 112, 59–67. doi: 10.1016/j.lfs.2014.07.020
Hung, C. C., Lin, C. H., Chang, H., Wang, C. Y., Lin, S. H., Hsu, P. C., et al. (2016). Astrocytic GAP43 Induced by the TLR4/NF-κB/STAT3 Axis Attenuates Astrogliosis-Mediated Microglial Activation and Neurotoxicity. J. Neurosci. 36, 2027–2043. doi: 10.1523/JNEUROSCI.3457-15.2016
Iadecola, C. (2017). The neurovascular unit coming of age: a journey through neurovascular coupling in health and disease. Neuron 96, 17–42. doi: 10.1016/j.neuron.2017.07.030
Jing, Y., Ma, R., Chu, Y., Dou, M., Wang, M., Li, X., et al. (2021). Matrine treatment induced an A2 astrocyte phenotype and protected the blood-brain barrier in CNS autoimmunity. J. Chem. Neuroanat. 117:102004. doi: 10.1016/j.jchemneu.2021.102004
Jolly, S., Bazargani, N., Quiroga, A. C., Pringle, N. P., Attwell, D., Richardson, W. D., et al. (2018). G protein-coupled receptor 37-like 1 modulates astrocyte glutamate transporters and neuronal NMDA receptors and is neuroprotective in ischemia. Glia 66, 47–61. doi: 10.1002/glia.23198
Jurič, D. M., Mele, T., and Carman-Kržan, M. (2011). Involvement of histaminergic receptor mechanisms in the stimulation of NT-3 synthesis in astrocytes. Neuropharmacology 60, 1309–1317. doi: 10.1016/j.neuropharm.2011.01.019
Kadhim, H. J., Duchateau, J., and Sébire, G. (2008). Cytokines and brain injury: invited review. J. Intensive Care Med. 23, 236–249. doi: 10.1177/0885066608318458
Keep, R. F., Zhou, N., Xiang, J., Andjelkovic, A. V., Hua, Y., and Xi, G. (2014). Vascular disruption and blood-brain barrier dysfunction in intracerebral hemorrhage. Fluids Barriers CNS 11:18. doi: 10.1186/2045-8118-11-18
Kim, S. K., Nabekura, J., and Koizumi, S. (2017). Astrocyte-mediated synapse remodeling in the pathological brain. Glia 65, 1719–1727. doi: 10.1002/glia.23169
Kisler, K., Nelson, A. R., Montagne, A., and Zlokovic, B. V. (2017a). Cerebral blood flow regulation and neurovascular dysfunction in Alzheimer disease. Nat. Rev. Neurosci. 18, 419–434. doi: 10.1038/nrn.2017.48
Kisler, K., Nelson, A. R., Rege, S. V., Ramanathan, A., Wang, Y., Ahuja, A., et al. (2017b). Pericyte degeneration leads to neurovascular uncoupling and limits oxygen supply to brain. Nat. Neurosci. 20, 406–416. doi: 10.1038/nn.4489
Krupinski, J., Kumar, P., Kumar, S., and Kaluza, J. (1996). Increased expression of TGF-beta 1 in brain tissue after ischemic stroke in humans. Stroke 27, 852–857. doi: 10.1161/01.str.27.5.852
Kucera, R., Smid, D., Topolcan, O., Karlikova, M., Fiala, O., Slouka, D., et al. (2016). Searching for New Biomarkers and the Use of Multivariate Analysis in Gastric Cancer Diagnostics. Anticancer Res. 36, 1967–1971.
Lasek-Bal, A., Jędrzejowska-Szypułka, H., Różycka, J., Bal, W., Holecki, M., Duława, J., et al. (2015). Low Concentration of BDNF in the acute phase of ischemic stroke as a factor in poor prognosis in terms of functional status of patients. Med. Sci. Monit. 21, 3900–3905. doi: 10.12659/msm.895358
Lau, C. L., Beart, P. M., and O’Shea, R. D. (2010). Transportable and non-transportable inhibitors of L-glutamate uptake produce astrocytic stellation and increase EAAT2 cell surface expression. Neurochem. Res. 35, 735–742. doi: 10.1007/s11064-010-0130-6
Li, C., Sui, C., Wang, W., Yan, J., Deng, N., Du, X., et al. (2021). Baicalin Attenuates Oxygen-Glucose Deprivation/Reoxygenation-Induced Injury by Modulating the BDNF-TrkB/PI3K/Akt and MAPK/Erk1/2 Signaling Axes in Neuron-Astrocyte Cocultures. Front. Pharmacol. 12:599543. doi: 10.3389/fphar.2021.599543
Li, D., Lang, W., Zhou, C., Wu, C., Zhang, F., Liu, Q., et al. (2018). Upregulation of Microglial ZEB1 Ameliorates Brain Damage after Acute Ischemic Stroke. Cell Rep. 22, 3574–3586. doi: 10.1016/j.celrep.2018.03.011
Li, Q., and Barres, B. A. (2018). Microglia and macrophages in brain homeostasis and disease. Nat. Rev. Immunol. 18, 225–242. doi: 10.1038/nri.2017.125
Li, T., Chen, X., Zhang, C., Zhang, Y., and Yao, W. (2019). An update on reactive astrocytes in chronic pain. J. Neuroinflammation 16:140. doi: 10.1186/s12974-019-1524-2
Li, X., Huang, L., Liu, G., Fan, W., Li, B., Liu, R., et al. (2020). Ginkgo diterpene lactones inhibit cerebral ischemia/reperfusion induced inflammatory response in astrocytes via TLR4/NF-κB pathway in rats. J. Ethnopharmacol. 249:112365. doi: 10.1016/j.jep.2019.112365
Liddelow, S. A., Guttenplan, K. A., Clarke, L. E., Bennett, F. C., Bohlen, C. J., Schirmer, L., et al. (2017). Neurotoxic reactive astrocytes are induced by activated microglia. Nature 54, 481–487. doi: 10.1038/nature21029
Lin, C. H., Chen, H. Y., and Wei, K. C. (2020). Role of HMGB1/TLR4 Axis in Ischemia/Reperfusion-Impaired Extracellular Glutamate Clearance in Primary Astrocytes. Cells 9:2585. doi: 10.3390/cells9122585
Liu, D. M., Zhang, M., Rong, X. F., Li, J., and Wang, X. (2017). Potassium 2-(1-hydroxypentyl)-benzoate attenuates neuronal apoptosis in neuron-astrocyte co-culture system through neurotrophy and neuroinflammation pathway. Acta Pharm. Sin. B 7, 554–563. doi: 10.1016/j.apsb.2017.06.006
Liu, L. R., Liu, J. C., Bao, J. S., Bai, Q. Q., and Wang, G. Q. (2020). Interaction of Microglia and Astrocytes in the Neurovascular Unit. Front. Immunol. 11:1024. doi: 10.3389/fimmu.2020.01024
Liu, M., Wu, Y., Liu, Y., Chen, Z., He, S., Zhang, H., et al. (2018). Basic fibroblast growth factor protects astrocytes against ischemia/reperfusion injury by upregulating the caveolin-1/VEGF Signaling Pathway. J. Mol. Neurosci. 64, 211–223. doi: 10.1007/s12031-017-1023-9
Liu, W., Tang, Y., and Feng, J. (2011). Cross talk between activation of microglia and astrocytes in pathological conditions in the central nervous system. Life Sci. 89, 141–146. doi: 10.1016/j.lfs.2011.05.011
Liu, Z., and Chopp, M. (2016). Astrocytes, therapeutic targets for neuroprotection and neurorestoration in ischemic stroke. Prog. Neurobiol. 144, 103–120. doi: 10.1016/j.pneurobio.2015.09.008
Livne-Bar, I., Wei, J., Liu, H. H., Alqawlaq, S., Won, G. J., Tuccitto, A., et al. (2017). Astrocyte-derived lipoxins A4 and B4 promote neuroprotection from acute and chronic injury. J. Clin. Invest. 127, 4403–4414. doi: 10.1172/JCI77398
Lo, E. H. (2010). Degeneration and repair in central nervous system disease. Nat. Med. 16, 1205–1209. doi: 10.1038/nm.2226
Lo, E. H., Dalkara, T., and Moskowitz, M. A. (2003). Mechanisms, challenges and opportunities in stroke. Nat. Rev. Neurosci. 4, 399–415. doi: 10.1038/nrn1106
Maddahi, A., and Edvinsson, L. (2010). Cerebral ischemia induces microvascular pro-inflammatory cytokine expression via the MEK/ERK pathway. J. Neuroinflammation 7:14. doi: 10.1186/1742-2094-7-14
Mahmoud, S., Gharagozloo, M., Simard, C., and Gris, D. (2019). Astrocytes maintain glutamate homeostasis in the CNS by controlling the balance between glutamate uptake and release. Cells 8:184. doi: 10.3390/cells8020184
Mashima, K., Takahashi, S., Minami, K., Izawa, Y., Abe, T., Tsukada, N., et al. (2018). Neuroprotective role of astroglia in parkinson disease by reducing oxidative stress through dopamine-induced activation of pentose-phosphate pathway. ASN Neuro 10:1759091418775562. doi: 10.1177/1759091418775562
Mauch, D. H., Nägler, K., Schumacher, S., Göritz, C., Müller, E. C., Otto, A., et al. (2001). CNS synaptogenesis promoted by glia-derived cholesterol. Science 294, 1354–1357. doi: 10.1126/science.294.5545.1354
Meshitsuka, S., and Aremu, D. A. (2008). (13)C heteronuclear NMR studies of the interaction of cultured neurons and astrocytes and aluminum blockade of the preferential release of citrate from astrocytes. J. Biol. Inorg. Chem. 13, 241–247. doi: 10.1007/s00775-007-0317-8
Min, H., Choi, B., Jang, Y. H., Cho, I. H., and Lee, S. J. (2017). Heme molecule functions as an endogenous agonist of astrocyte TLR2 to contribute to secondary brain damage after intracerebral hemorrhage. Mol. Brain 10:27. doi: 10.1186/s13041-017-0305-z
Mishra, A., Reynolds, J. P., Chen, Y., Gourine, A. V., Rusakov, D. A., and Attwell, D. (2016). Astrocytes mediate neurovascular signaling to capillary pericytes but not to arterioles. Nat. Neurosci. 19, 1619–1627. doi: 10.1038/nn.4428
Montagne, A., Nikolakopoulou, A. M., Zhao, Z., Sagare, A. P., Si, G., Lazic, D., et al. (2018). Pericyte degeneration causes white matter dysfunction in the mouse central nervous system. Nat. Med. 24, 326–337. doi: 10.1038/nm.4482
Najjar, S., Pearlman, D. M., Devinsky, O., Najjar, A., and Zagzag, D. (2013). Neurovascular unit dysfunction with blood-brain barrier hyperpermeability contributes to major depressive disorder: a review of clinical and experimental evidence. J. Neuroinflammation 10:142. doi: 10.1186/1742-2094-10-142
Nakayama, T., Momoki-Soga, T., and Inoue, N. (2003). Astrocyte-derived factors instruct differentiation of embryonic stem cells into neurons. Neurosci. Res. 46, 241–249. doi: 10.1016/s0168-0102(03)00063-4
Naranjo, O., Osborne, O., Torices, S., and Toborek, M. (2021). In vivo targeting of the neurovascular unit: challenges and advancements. Cell. Mol. Neurobiol. doi: 10.1007/s10571-021-01113-3 [Epub ahed of print].
Norden, D. M., Fenn, A. M., Dugan, A., and Godbout, J. P. T. G. F. (2014). β produced by IL-10 redirected astrocytes attenuates microglial activation. Glia 62, 881–895. doi: 10.1002/glia.22647
Nuriya, M., Shinotsuka, T., and Yasui, M. (2013). Diffusion properties of molecules at the blood-brain interface: potential contributions of astrocyte endfeet to diffusion barrier functions. Cereb. Cortex 23, 2118–2126. doi: 10.1093/cercor/bhs198
Oohashi, T., Edamatsu, M., Bekku, Y., and Carulli, D. (2015). The hyaluronan and proteoglycan link proteins: organizers of the brain extracellular matrix and key molecules for neuronal function and plasticity. Exp. Neurol. 274, 134–144. doi: 10.1016/j.expneurol.2015.09.010
Pekny, M., Wilhelmsson, U., Tatlisumak, T., and Pekna, M. (2019). Astrocyte activation and reactive gliosis-A new target in stroke? Neurosci. Lett. 689, 45–55. doi: 10.1016/j.neulet.2018.07.021
Peng, M., Wang, J., Zhang, D., Jin, H., Li, J., Wu, X. R., et al. (2018). PHLPP2 stabilization by p27 mediates its inhibition of bladder cancer invasion by promoting autophagic degradation of MMP2 protein. Oncogene 37, 5735–5748. doi: 10.1038/s41388-018-0374-1
Peng, Y., Hu, Y., Xu, S., Rong, X., Li, J., Li, P., et al. (2014). Potassium 2-(1-hydroxypentyl)-benzoate improves memory deficits and attenuates amyloid and τ pathologies in a mouse model of Alzheimer’s disease. J. Pharmacol. Exp. Ther. 350, 361–374. doi: 10.1124/jpet.114.213140
Pikula, A., Beiser, A. S., Chen, T. C., Preis, S. R., Vorgias, D., DeCarli, C., et al. (2013). Serum brain-derived neurotrophic factor and vascular endothelial growth factor levels are associated with risk of stroke and vascular brain injury: framingham Study. Stroke 44, 2768–2775. doi: 10.1161/STROKEAHA.113.001447
Prat, A., Biernacki, K., Wosik, K., and Antel, J. P. (2001). Glial cell influence on the human blood-brain barrier. Glia 36, 145–155. doi: 10.1002/glia.1104
Quattromani, M. J., Pruvost, M., Guerreiro, C., Backlund, F., Englund, E., Aspberg, A., et al. (2018). Extracellular matrix modulation is driven by experience-dependent plasticity during stroke recovery. Mol. Neurobiol. 55, 2196–2213. doi: 10.1007/s12035-017-0461-2
Quintas, C., Pinho, D., Pereira, C., Saraiva, L., Gonçalves, J., and Queiroz, G. (2014). Microglia P2Y6 receptors mediate nitric oxide release and astrocyte apoptosis. J. Neuroinflammation 11:141. doi: 10.1186/s12974-014-0141-3
Rinaldi, C., Donato, L., Alibrandi, S., Scimone, C., D’Angelo, R., and Sidoti, A. (2021). Oxidative stress and the neurovascular unit. Life 11:767. doi: 10.3390/life11080767
Roe, C. (2017). Unwrapping neurotrophic cytokines and histone modification. Cell. Mol. Neurobiol. 37, 1–4. doi: 10.1007/s10571-016-0330-y
Ronaldson, P. T., and Davis, T. P. (2020). Regulation of blood-brain barrier integrity by microglia in health and disease: a therapeutic opportunity. J. Cereb. Blood Flow Metab. 40, S6–S24. doi: 10.1177/0271678X20951995
Sagare, A. P., Bell, R. D., Zhao, Z., Ma, Q., Winkler, E. A., and Ramanathan, A. (2013). Pericyte loss influences Alzheimer-like neurodegeneration in mice. Nat. Commun. 4:2932. doi: 10.1038/ncomms3932
Saini, V., Guada, L., and Yavagal, D. R. (2021). Global epidemiology of stroke and access to acute ischemic stroke interventions. Neurology 97, S6–S16. doi: 10.1212/WNL.0000000000012781
Sá-Pereira, I., Brites, D., and Brito, M. A. (2012). Neurovascular unit: a focus on pericytes. Mol. Neurobiol. 45, 327–347. doi: 10.1007/s12035-012-8244-2
Sato, Y., Nakanishi, K., Tokita, Y., Kakizawa, H., Ida, M., Maeda, H., et al. (2008). A highly sulfated chondroitin sulfate preparation, CS-E, prevents excitatory amino acid-induced neuronal cell death. J. Neurochem. 104, 1565–1576. doi: 10.1111/j.1471-4159.2007.05107.x
Sawe, N., Steinberg, G., and Zhao, H. (2008). Dual roles of the MAPK/ERK1/2 cell signaling pathway after stroke. J. Neurosci. Res. 86, 1659–1669. doi: 10.1002/jnr.21604
Sola, A., Rogido, M., Lee, B. H., Genetta, T., and Wen, T. C. (2005). Erythropoietin after focal cerebral ischemia activates the Janus kinase-signal transducer and activator of transcription signaling pathway and improves brain injury in postnatal day 7 rats. Pediatr. Res. 57, 481–487. doi: 10.1203/01
Stanic, K., Saldivia, N., Förstera, B., Torrejón, M., Montecinos, H., and Caprile, T. (2016). Expression patterns of extracellular matrix proteins during posterior commissure development. Front. Neuroanat. 10:89. doi: 10.3389/fnana.2016.00089
Stanne, T. M., Åberg, N. D., Nilsson, S., Jood, K., Blomstrand, C., Andreasson, U., et al. (2016). Low circulating acute brain-derived neurotrophic factor levels are associated with poor long-term functional outcome after ischemic stroke. Stroke 47, 1943–1945. doi: 10.1161/STROKEAHA.115.012383
Steliga, A., Kowiański, P., Czuba, E., Waśkow, M., Moryś, J., and Lietzau, G. (2020). Neurovascular unit as a source of ischemic stroke biomarkers-limitations of experimental studies and perspectives for clinical application. Transl. Stroke Res. 11, 553–579. doi: 10.1007/s12975-019-00744-5
Stogsdill, J. A., Ramirez, J., Liu, D., Kim, Y. H., Baldwin, K. T., Enustun, E., et al. (2017). Astrocytic neuroligins control astrocyte morphogenesis and synaptogenesis. Nature 551, 192–197. doi: 10.1038/nature24638
Takahashi, S. (2020). Metabolic compartmentalization between astroglia and neurons in physiological and pathophysiological conditions of the neurovascular unit. Neuropathology 40, 121–137. doi: 10.1111/neup.12639
Thurgur, H., and Pinteaux, E. (2018). Microglia in the Neurovascular Unit: Blood-Brain Barrier-microglia Interactions After Central Nervous System Disorders. Neuroscience 405, 55–67. doi: 10.1016/j.neuroscience.2018.06.046
Willis, C. L., Nolan, C. C., Reith, S. N., Lister, T., Prior, M. J., Guerin, C. J., et al. (2004). Focal astrocyte loss is followed by microvascular damage, with subsequent repair of the blood-brain barrier in the apparent absence of direct astrocytic contact. Glia 45, 325–337. doi: 10.1002/glia.10333
Xie, L., Poteet, E. C., Li, W., Scott, A. E., Liu, R., Wen, Y., et al. (2010). Modulation of polymorphonuclear neutrophil functions by astrocytes. J. Neuroinflammation 7:53. doi: 10.1186/1742-2094-7-53
Xin, W. Q., Wei, W., Pan, Y. L., Cui, B. L., Yang, X. Y., Bähr, M., et al. (2021). Modulating poststroke inflammatory mechanisms: Novel aspects of mesenchymal stem cells, extracellular vesicles and microglia. World J. Stem Cells 13, 1030–1048. doi: 10.4252/wjsc.v13.i8.1030
Yang, J., Shao, C., Li, W., Wan, H., He, Y., and Yang, J. (2021). Protective effects of Astragaloside IV against oxidative injury and apoptosis in cultured astrocytes by regulating Nrf2/JNK signaling. Exp. Brain Res. 239, 1827–1840. doi: 10.1007/s00221-021-06096-7
Ye, L., Yang, Y., Zhang, X., Cai, P., Li, R., Chen, D., et al. (2015). The Role of bFGF in the excessive activation of astrocytes is related to the inhibition of TLR4/NFκB Signals. Int. J. Mol. Sci. 17:37. doi: 10.3390/ijms17010037
Zagrean, A. M., Hermann, D. M., Opris, I., Zagrean, L., and Popa-Wagner, A. (2018). Multicellular crosstalk between exosomes and the neurovascular unit after cerebral Ischemia, Therapeutic Implications. Front. Neurosci. 12:811. doi: 10.3389/fnins.2018.00811
Zbesko, J. C., Nguyen, T. V., Yang, T., Frye, J. B., Hussain, O., Hayes, M., et al. (2018). Glial scars are permeable to the neurotoxic environment of chronic stroke infarcts. Neurobiol. Dis. 112, 63–78. doi: 10.1016/j.nbd.2018.01.007
Zeng, G., Ding, W., Li, Y., Sun, M., and Deng, L. (2018). Morroniside protects against cerebral ischemia/reperfusion injury by inhibiting neuron apoptosis and MMP2/9 expression. Exp. Ther. Med. 16, 2229–2234. doi: 10.3892/etm.2018.6457
Zhang, H., Guo, M., Yu, J., Li, Y., Zhao, Y., and Zhang, J. (2018). [Inhibitory effect of Fasudil on activation and inflammatory response of mouse astrocytes induced by lipopolysaccharide and its mechanism]. Xi Bao Yu Fen Zi Mian Yi Xue Za Zhi 34, 505–510.
Zhang, X., Zhang, Q., Huang, L., Liu, M., Cheng, Z., Zheng, Y., et al. (2021). Pien-Tze-Huang attenuates neuroinflammation in cerebral ischaemia-reperfusion injury in rats through the TLR4/NF-κB/MAPK pathway. Pharm. Biol. 59, 828–839. doi: 10.1080/13880209.2021.1942926
Zhang, Y., and Miao, J. M. (2018). Ginkgolide K promotes astrocyte proliferation and migration after oxygen-glucose deprivation via inducing protective autophagy through the AMPK/mTOR/ULK1 signaling pathway. Eur. J. Pharmacol. 832, 96–103. doi: 10.1016/j.ejphar.2018.05.029
Zhang, Y., Wang, L., Li, J., and Wang, X. L. (2006). 2-(1-Hydroxypentyl)-benzoate increases cerebral blood flow and reduces infarct volume in rats model of transient focal cerebral ischemia. J. Pharmacol. Exp. Ther. 317, 973–979. doi: 10.1124/jpet.105.098517
Zhang, Y., Xu, D., Qi, H., Yuan, Y., Liu, H., Yao, S., et al. (2018). Enriched environment promotes post-stroke neurogenesis through NF-κB-mediated secretion of IL-17A from astrocytes. Brain Res. 1687, 20–31. doi: 10.1016/j.brainres.2018.02.030
Zhao, H., Chen, Z., Xie, L. J., and Liu, G. F. (2018). Suppression of TLR4/NF-κB signaling pathway improves cerebral ischemia-reperfusion injury in Rats. Mol. Neurobiol. 55, 4311–4319. doi: 10.1007/s12035-017-0552-0
Zhao, W., Xu, S., Peng, Y., Ji, X., Cao, D., Li, J., et al. (2013). Potassium 2-(1-hydroxypentyl)-benzoate improves learning and memory deficits in chronic cerebral hypoperfused rats. Neurosci. Lett. 541, 155–160. doi: 10.1016/j.neulet.2013.01.053
Zhao, Y., Yang, J., Li, C., Zhou, G., Wan, H., Ding, Z., et al. (2020). Role of the neurovascular unit in the process of cerebral ischemic injury. Pharmacol. Res. 160:105103. doi: 10.1016/j.phrs.2020.105103
Zheng, Z., Chopp, M., and Chen, J. (2020). Multifaceted roles of pericytes in central nervous system homeostasis and disease. J. Cereb. Blood Flow Metab. 40, 1381–1401. doi: 10.1177/0271678X20911331
Keywords: astrocytes, neurovascular units, ischemic stroke, BBB, double-edged role
Citation: Han G, Song L, Ding Z, Wang Q, Yan Y, Huang J and Ma C (2022) The Important Double-Edged Role of Astrocytes in Neurovascular Unit After Ischemic Stroke. Front. Aging Neurosci. 14:833431. doi: 10.3389/fnagi.2022.833431
Received: 13 December 2021; Accepted: 16 February 2022;
Published: 07 April 2022.
Edited by:
Fengquan Zhou, Johns Hopkins Medicine, United StatesReviewed by:
Yoon Kyung Choi, Konkuk University, South KoreaPatrick T. Ronaldson, University of Arizona, United States
Copyright © 2022 Han, Song, Ding, Wang, Yan, Huang and Ma. This is an open-access article distributed under the terms of the Creative Commons Attribution License (CC BY). The use, distribution or reproduction in other forums is permitted, provided the original author(s) and the copyright owner(s) are credited and that the original publication in this journal is cited, in accordance with accepted academic practice. No use, distribution or reproduction is permitted which does not comply with these terms.
*Correspondence: Lijuan Song, slj_0354@126.com; Yuqing Yan, 779734216@qq.com; Jianjun Huang, hjj1958@126.com; Cungen Ma, macungen@sxtcm.edu.cn
†These authors have contributed equally to this work