- 1Guangdong Key Laboratory of Age-Related Cardiac and Cerebral Diseases, Department of Neurology, Institute of Neurology, Affiliated Hospital of Guangdong Medical University, Zhanjiang, China
- 2Department of Neurology, Central People’s Hospital of Zhanjiang, Zhanjiang, China
- 3Department of Neurology, Chenzhou No. 1 People’s Hospital, Chenzhou, China
- 4Department of Neurology, Huizhou Municipal Central Hospital, Huizhou, China
- 5Department of Clinical Research, Affiliated Hospital of Guangdong Medical University, Zhanjiang, China
- 6Department of Clinical Sciences, Liverpool School of Tropical Medicine, Liverpool, United Kingdom
Objectives: We evaluated the effects of long-term/recurrent use of antibiotics in childhood on developing cognitive impairment in middle and old age from UK Biobank Database.
Methods: UK Biobank recruited participants aged 37–73 years. Cognitive impairment was ascertained by fluid intelligence questionnaire. Primary outcome was the occurrence of cognitive impairment in middle and old age. Multivariate logistic regression models were used to explore the relationship between long-term/recurrent use of antibiotics and cognitive impairment.
Results: Over 3.8–10.8 years’ follow-up, 4,781 of the 35,921 participants developed cognitive impairment. The odds of cognitive impairment in middle and old age among long-term/recurrent use of antibiotics in childhood were increased by 18% compared with their counterparts (adjusted odd ratio 1.18, 95% confidence interval 1.08–1.29, p < 0.01). The effect of long-term/recurrent use of antibiotics in childhood on cognitive impairment was homogeneous across different categories of various subgroup variables such as sex, age, APOE4, ethnic groups, income before tax, smoking status, alcohol status, BMI, hypertension and diabetes but the effect of long-term/recurrent use of antibiotics in childhood was modified by the educational qualification (p-value for interaction <0.05).
Conclusion: Long-term/recurrent use of antibiotics in childhood may increase the risk of cognitive impairment in middle and old age.
Introduction
Cognitive impairment is the nomenclature of one or more cognitive impairment areas, ranging from complete cognition to mild cognitive impairment and finally dementia (Courtney, 2013; Ljubenkov and Geschwind, 2016; Rajji, 2018). Cognitive impairment is a serious health problem with high incidence, high disability rate and increasing global costs (Sacuiu, 2016). It is estimated that about 47 million people worldwide suffered from dementia in 2015, and the figure is expected to double every 20 years (Fiest et al., 2016; Livingston et al., 2017). This number will reach 66 million by 2030 and 115 million by 2050 (Fiest et al., 2016; Livingston et al., 2017). A growing body of evidence shows that age, cerebrovascular disease, diabetes, mental illness, etc., are the risk factors of cognitive impairment (Baumgart et al., 2015; Bellou et al., 2017).
Adolescence is a critical period of development, marking the transition from childhood to adulthood. It is in the last stage of development before adulthood that the brain is highly responsive to certain environmental cues (Paus et al., 2008). Compared with adults, the microbiota composition of adolescence is generally simpler and less stable, which is highly diverse and stable (Fouhy et al., 2012; Borre et al., 2014). These differences may be due to the relatively immature intestinal flora during adolescence, making it more susceptible to infection (Fouhy et al., 2012; McVey Neufeld et al., 2016). Therefore, significant changes in the composition of the gut microbiota may lead to the occurrence of neurodegenerative disorders (Verdu, 2006; McVey Neufeld et al., 2016). The use of antibiotics is an important reason for changes in the intestinal flora.
Antibiotics are usually used to remove or prevent bacterial colonization in the human body, and not to target specific types of bacteria (Hadar et al., 2018). Studies have found that antibiotic treatment impairs gut microbiota-brain communication and even cognitive impairment (Fröhlich et al., 2016). Slykerman et al. (2017) identified an association between antibiotic treatment in the first year of life and cognitive, behavior and emotion in childhood. However, there are limited clinical data on the effects of long-term/recurrent use of antibiotics as child or teenager (LRUAC) on cognitive impairment in middle and old age. Thus, the relationship between the LRUAC and the potential risk of developing dementia remains unknown. In the present study, we used a national population data bank in the United Kingdom to explore the associations between LRUAC and cognitive impairment in middle and old age.
Materials and Methods
Study Design and Population
We used the UK Biobank Database to perform the population-based retrospective cohort study with a representative sample of 502,505 participants. The cohorts, with subjects aged 37–73 years, were established from 2006 to 2010 across England, Scotland and Wales. At baseline (between 2006 and 2010), a detailed assessment of their characteristics and a completed cognitive testing were made. The participants were followed until 2014–2015. The study was approved by the North-West Multi-center Research Ethics Committee. The research was carried out in accordance with the Declaration of Helsinki of the World Medical Association, and participants gave informed consent. The data were anonymized and no additional ethical approval was required for the present analyses.
Assessment of Cognitive Impairment
Fluid intelligence (FI) is defined as the ability to reason and to solve new problems independently of previously acquired knowledge, and is related to working memory (Salthouse and Pink, 2008), attention (Cochrane et al., 2019), executive functions (Lyall et al., 2016). FI is used as a tool to evaluate cognitive function (Kindermann et al., 2012; Kendall et al., 2019; Tank et al., 2022). Cognitive impairment was defined as >1 standard deviation (SD) reduction in FI score (Folley et al., 2019). FI score sums the number of correct answers out of 13 verbal and numerical reasoning items that participants were required to answer within 2 min. Baseline assessment of FI was carried out with touch-screen questionnaire in 2006–2010 in UK Biobank Assessment Centre, and follow-up assessment of FI was completed online follow-up remotely in 2014–2015. Primary outcome is the cognitive impairment in middle and old age.
Assessment of Long-Term/Recurrent Use of Antibiotics as Child or Teenager
Long-term/recurrent use of antibiotics as child or teenager was recorded as “During childhood or as a teenager did you receive long-term or recurrent courses (3 or more per year) of antibiotics (for example for tonsillitis or acne)?” The above information was collected in 2017–2018.
Assessment of Covariates
To control for confounding factors, we included the following baseline variables (2006–2010) as covariates in the analysis: age, sex, educational qualification, apoe4, ethnicity, income before tax, smoking status, alcohol status, Body Mass Index (BMI, classified as underweight: BMI ≤18.5, normal weight: BMI 18.5–24.9, overweight: BMI 25–29.9, and obesity: BMI ≥30.0). History of hypertension was originated from the question: “Has a doctor ever told you that you have had any of the following conditions?” And answers were: 1. Heart attack, 2. Angina, 3. Stroke, 4. High blood pressure, −7. None of the above, −3. “Prefer not to answer.” History of diabetes was derived from the question “Has a doctor ever told you that you have diabetes?”
Statistical Analyses
Using the analysis of Chi-square tests or Fisher’s exact test, we compared the proportion of cognitive impairment by sociodemographic factors (e.g., gender, age, educational qualification, ethnicity, and income level), and clinical conditions and medical histories (e.g., Apoe4, smoking history, drinking history, BMI, history of hypertension, and diabetes). Using multivariate logistic regression models, we calculated adjusted odds ratios (ORs) and 95% confidence intervals (CIs) between LRUAC and non- LRUAC controlling for gender, age, qualification, apoe4, ethnicity, income level, smoking history, drinking history, BMI, history of hypertension, history of diabetes.
P-values less than 0.05 were considered statistically significant. Missing data in covariates were imputed using multiple imputation method (mice package, m = 5, method = “rf,” seed = 12). Sensitivity analysis was performed based on the complete cases without multiple imputation for missing values in covariates. Analyses were conducted with RStudio (Version 1.4.1717, PBC).
Results
A total of 502,505 consecutive cases from UK Biobank were evaluated. 466,584 participants were excluded from the analysis because they had no cognitive assessment, they had cognitive impairment at baseline, they were lost to follow-up at the next wave or the antibiotic data were missing. After exclusions, a total of 35,921 participants without cognitive impairment remained in the study, which included 30,859 participants without long-term recurrent antibiotics as child or teenager. During an average 5.2 years’ follow-up (min 3.8, max 10.8 years), 741 (14.7%) participants with LRUAC and 4040 (13.1%) participants without LRUAC were identified cognitive impairment. Figure 1 shows the process of participant selection.
Table 1 presented potentially modifiable risk factors for cognitive impairment, included LRUAC, female, aged, low qualification, Ethnic groups, low income before tax, current smoking, non-alcohol, higher BMI, hypertension and diabetes.
Table 2 displayed the relationship of LRUAC and cognitive impairment with logistic regression model. Compared with those without LRUAC, participants with LRUAC had a substantially higher odds for cognitive impairment by 20% (OR = 1.20; 95% CI, 1.10–1.31) after adjusting for Model 1 (age, sex, educational qualification, apoe4, and ethnic). The increased odds was slightly attenuated to 18% (OR = 1.18, 95% CI 1.08∼1.29) after adjusting for Model 2 (Model 1 plus income before tax, smoking status, alcohol status, BMI, hypertension, diabetes). The OR was 1.24 (95% CI 1.09–1.41) based on the complete cases without multiple imputation for missing values (Model 3) (Table 2, left). Other than that, after adjusting for Model 2, 60–70 years old, black, Asian, other ethnic group, current smoking increased the risk for cognitive impairment. While male, higher qualification and income, previous alcohol reduced the risk for cognitive impairment.
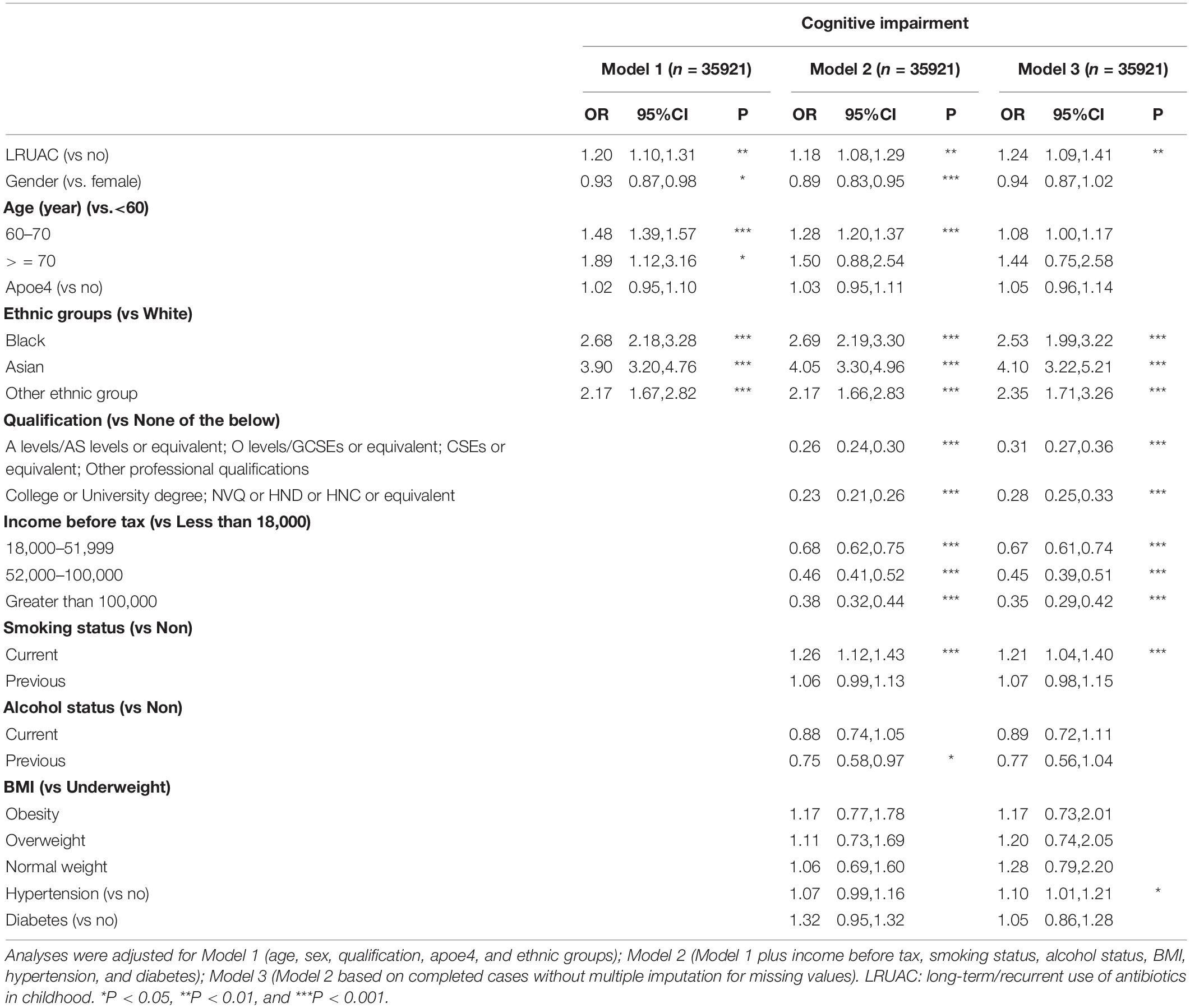
Table 2. Association between long-term/recurrent use of antibiotics and cognitive impairment and fluid intelligence (FI) difference.
We also evaluated the risk of cognitive impairment after LRUAC in each variable subgroup and found that the effect of long-term/recurrent use of antibiotics in childhood on cognitive impairment was homogeneous across different categories of subgroup variables such as sex, age, APOE4, ethnic groups, income before tax, smoking status, alcohol status, BMI, hypertension and diabetes but the effect of LRUAC was modified by higher qualification (p-value for interaction <0.05, Table 3), with the decreased effect of LRUAC on cognitive impairment being found only among participants with a higher qualification of College or University degree; NVQ or HND or HNC or equivalent.
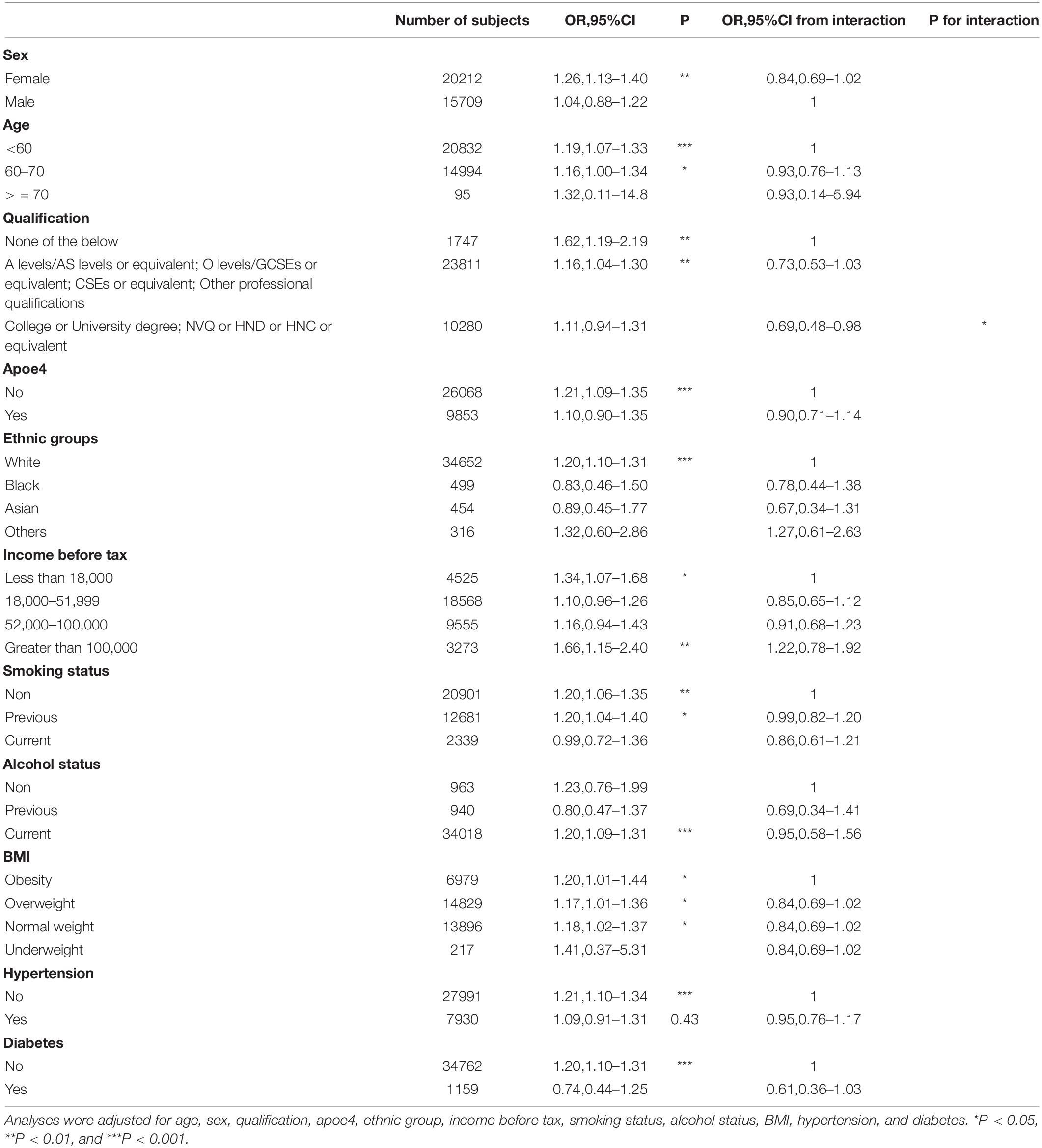
Table 3. Long-term/recurrent use of antibiotics and risk of cognitive impairment: Subgroup analysis.
Discussion
In this population-based study, we found LRUAC was associated with higher risk of cognition impairment in middle and old age, independently of age, sex, educational qualification, apoe4, ethnic, income before tax, smoking status, alcohol status, Body Mass Index (BMI), history of hypertension, and history of diabetes.
Antibiotics changed the world and saved the lives of many patients who would have died of infection (Hadar et al., 2018). In fact, more than 10% of European children use antibiotics each year (Sturkenboom et al., 2008). In the United States, antibiotics account for 25% of all prescriptions for pediatricians (Finkelstein et al., 2003). However, a large body of evidence has begun to suggest that such frequent use of antibiotics can also come at a price. There is indeed evidence that antibiotic treatment has significantly changed the microflora composition of adults and infants (Francino, 2015; Azad et al., 2016; Yassour et al., 2016). Even short-term antibiotic treatment can have a long-term effect on the composition of microflora (Isaac et al., 2017). Stable microflora composition plays a role in regulating the immune system, hormone secretion and response, and metabolism (Spor et al., 2011; Neuman et al., 2015; Blaser, 2016; Shamriz et al., 2016). Therefore, changes in the composition of microflora caused by antibiotics may lead to disease. Exposure to antibiotics in young children is associated with an increased risk of excessive weight gain, asthma, allergies and autoimmune diseases, such as inflammatory bowel disease (IBD) (Cho et al., 2012; Cox et al., 2014; Bokulich et al., 2016; Korpela et al., 2016; Turta and Rautava, 2016). The impact of antibiotic use on future health and disease has caused widespread concern (Diamond et al., 2011; Diaz Heijtz et al., 2011).
Although no direct clinical studies prove that antibiotics are associated with cognition impairment, there are several cohort studies reveal effects of antibiotics on central nervous system diseases. Antibiotic exposure in the first 2 years of life increase risk of attention-deficit/hyperactivity disorder (ADHD) with average 8.8 years follow-up (Aversa et al., 2021). Even prenatal antibiotic exposure is associated with increased risk of ADHD (Hamad et al., 2020), cerebral palsy or epilepsy (Meeraus et al., 2015) in the child. Quinolone or d cephalosporins increase stroke risk slightly in older person(s) in 2 years (Luchsinger et al., 2001). In this study, we found LRUAC’s effect on cognition impairment more than 30 years later in middle and old age. These studies suggest that antibiotics could cause long-term brain damage. In this study, age, sex, apoe4, income before tax, smoking status, alcohol status, BMI, hypertension, and diabetes had no interaction with LRUAC but qualification (college or university degree; NVQ or HND or HNC or equivalent) had a quantitative interaction with LRUAC on cognitive impairment: the effect of LRUAC on cognitive impairment diminished in participants with high qualification, which is consistent with a previous study (Ko et al., 2021).
There are some animal studies exhibiting the effects of antibiotics on cognition and the mechanics in the brain. Lach’s study shows that microbiota depletion with antibiotic treatment during the adolescent period cause enduring neurobehavioral disfunction, with obvious change of gene expression in the amygdala (Lach et al., 2020). Study by Wang et al. (2015) showed that rats taking ampicillin increased serum corticosterone, increased anxiety-like behavior, and impaired spatial memory. Further support to this notion is the fact that antibiotics, such as streptozotocin, have been used to induce sporadic AD forms in animal models, thereby affecting learning and memory performance (Cattaneo et al., 2017). Antibiotic treatment from early adolescence significantly decreased hippocampal BDNF and enhanced learning and memory impairments in a mouse model of multiple sclerosis (Zeraati et al., 2019), and prevented the development of anxiety- and depression-related behaviors, oxidative stress and hypothalamic-pituitary-adrenal axis dysregulation in AD mice (Mosaferi et al., 2021). The evidence suggests that the use of antibiotic cocktails in adolescent mice can lead to permanent changes in intestinal microflora and an increase in pro-inflammatory cytokines (Desbonnet et al., 2014, 2015; Cattaneo et al., 2017). In humans, some antibiotics, cefepime, can cross the blood-brain barrier, leading to changes in mental state, loss of consciousness, myoclonus and insanity (Payne et al., 2017). fMRI study also shows that antibiotic influences insular cortex functional connectivity that could involve cognitive flexibility and memory processing (Sometti et al., 2021).
However, controversial results have been obtained in some clinical and animal trials. Studies have shown that antibiotics also have beneficial effects on Alzheimer’s disease. Through the triple eradication of antibiotics (omeprazole, clarithromycin, and amoxicillin) to eliminate Helicobacter pylori and other pathogens, the cognitive function parameters of patients with AD were positively improved (Kountouras et al., 2009). The possible mechanism is that some antibiotics can have a beneficial effect on Alzheimer’s disease by reducing neuroinflammation caused by biological disorders. Antibiotics have been shown to reduce inflammation and improve cognitive impairment in AD animal models. The use of rifampicin in the animal model of AD can reduce the levels of A β and inflammatory cytokines in the brain (Yulug et al., 2018). Minocycline has a similar effect on Aβ and reduces the activation of microglia in rodent AD model (Budni et al., 2016). In 2004, patients with suspected Alzheimer’s disease and mild to moderate dementia who took a combination of doxycycline and rifampicin significantly improved the cognitive subscale (SADAScog) of the Standard Alzheimer’s Disease Assessment scale at 6 months of age (Loeb et al., 2004). In contrast, in 2013, a multicenter, double-blind, randomized, 2 × 2 factor-controlled trial of mild to moderate AD patients showed no significant effect on cognitive ability after 12 months of treatment with doxycycline or rifampicin alone or in combination (Molloy et al., 2013). Similarly, in 1999, d-cycloserine was found to effectively improve cognitive impairment in patients with AD (Tsai et al., 1999), but these positive effects were not found in subsequent cochrane review (Laake and Oeksengaard, 2002).
The strengths of this study are the large sample size, the cohort study with long follow-up time, and the robust result for the effect of LRUAC on cognitive impairment. Also, the study has some limitations. Firstly, the records of LRUAC were collected during 2017–2018, which may be subject to recall bias. The cognition normal participants were more likely to recall they did not expose to LRUAC, which might exaggerate the effect of LRUAC. Secondly, the FI score was collected from different ways: basic collection in UK Biobank Assessment Centre during 2006–2010 and follow-up collection online remotely during 2014–2015, which would cause measurement bias toward the score. Lastly, this was an observational study and the observed results may still be subject to possible unobserved confounding factors.
In this population-based retrospective cohort study, we observed the impact of the LRUAC on the development of the cognitive impairment. We hope that our results could provide useful clues for assessing the lifelong effects of inappropriate use of antibiotics.
Data Availability Statement
The original contributions presented in the study are included in the article/supplementary material, further inquiries can be directed to the corresponding authors.
Ethics Statement
The studies involving human participants were reviewed and approved by the North-West Multi-center Research Ethics Committee. The patients/participants provided their written informed consent to participate in this study.
Author Contributions
ZLiu: full access to the UK Biobank data and statistical analysis. HZ and DW: concept and design. ZLiu, SW, XC, and LL: drafting of the manuscript. All authors acquisition, analysis, or interpretation of data and critical revision of the manuscript for important intellectual content
Funding
ZLiu reported receiving grants from the Discipline Construction Project of Guangdong Medical University (No.4SG21234G) and Competitive Allocation Project of Zhanjiang Science and Technology Development Special Fund (No. 2021A05071) during the conduct of the study.
Conflict of Interest
The authors declare that the research was conducted in the absence of any commercial or financial relationships that could be construed as a potential conflict of interest.
Publisher’s Note
All claims expressed in this article are solely those of the authors and do not necessarily represent those of their affiliated organizations, or those of the publisher, the editors and the reviewers. Any product that may be evaluated in this article, or claim that may be made by its manufacturer, is not guaranteed or endorsed by the publisher.
References
Aversa, Z., Atkinson, E. J., Schafer, M. J., Theiler, R. N., Rocca, W. A., Blaser, M. J., et al. (2021). Association of infant antibiotic exposure with childhood health outcomes. Mayo Clin. Proc. 96, 66–77. doi: 10.1016/j.mayocp.2020.07.019
Azad, M., Konya, T., Persaud, R., Guttman, D., Chari, R., Field, C., et al. (2016). Impact of maternal intrapartum antibiotics, method of birth and breastfeeding on gut microbiota during the first year of life: a prospective cohort study. BJOG 6, 983–993. doi: 10.1111/1471-0528.13601
Baumgart, M., Snyder, H. M., Carrillo, M. C., Fazio, S., Kim, H., and Johns, H. (2015). Summary of the evidence on modifiable risk factors for cognitive decline and dementia: a population-based perspective. Alzheimers Dement. J. Alzheimers Assoc. 6, 718–726. doi: 10.1016/j.jalz.2015.05.016
Bellou, V., Belbasis, L., Tzoulaki, I., Middleton, L. T., Ioannidis, J. P. A., and Evangelou, E. (2017). Systematic evaluation of the associations between environmental risk factors and dementia: an umbrella review of systematic reviews and meta-analyses. Alzheimers Dement. 4, 406–418. doi: 10.1016/j.jalz.2016.07.152
Blaser, M. J. (2016). Antibiotic use and its consequences for the normal microbiome. Science 6285, 544–545. doi: 10.1126/science.aad9358
Bokulich, N. A., Chung, J., Battaglia, T., Henderson, N., Jay, M., Li, H., et al. (2016). Antibiotics, birth mode, and diet shape microbiome maturation during early life. Sci. Transl. Med. 343:343ra82. doi: 10.1126/scitranslmed.aad7121
Borre, Y. E., O’Keeffe, G. W., Clarke, G., Stanton, C., Dinan, T. G., and Cryan, J. F. (2014). Microbiota and neurodevelopmental windows: implications for brain disorders. Trends Mol. Med. 9, 509–518. doi: 10.1016/j.molmed.2014.05.002
Budni, J., Garcez, M. L., de Medeiros, J., Cassaro, E., Bellettini-Santos, T., Mina, F., et al. (2016). The anti-inflammatory role of minocycline in Alzheimer’s disease. Curr. Alzheimer Res. 12, 1319–1329. doi: 10.2174/1567205013666160819124206
Cattaneo, A., Cattane, N., Galluzzi, S., Provasi, S., Lopizzo, N., Festari, C., et al. (2017). Association of brain amyloidosis with pro-inflammatory gut bacterial taxa and peripheral inflammation markers in cognitively impaired elderly. Neurobiol. Aging 49, 60–68. doi: 10.1016/j.neurobiolaging.2016.08.019
Cho, I., Yamanishi, S., Cox, L., Methé, B. A., Zavadil, J., Li, K., et al. (2012). Antibiotics in early life alter the murine colonic microbiome and adiposity. Nature 7413, 621–626. doi: 10.1038/nature11400
Cochrane, A., Simmering, V., and Green, C. S. (2019). Fluid intelligence is related to capacity in memory as well as attention: evidence from middle childhood and adulthood. PLoS One 8:e0221353. doi: 10.1371/journal.pone.0221353
Courtney, D. L. (2013). Dealing with mild cognitive impairment: help for patients and caregivers. Clin. Geriatr. Med. 4, 895–905. doi: 10.1016/j.cger.2013.07.010
Cox, L. M., Yamanishi, S., Sohn, J., Alekseyenko, A. V., Leung, J. M., Cho, I., et al. (2014). Altering the intestinal microbiota during a critical developmental window has lasting metabolic consequences. Cell 4, 705–721. doi: 10.1016/j.cell.2014.05.052
Desbonnet, L., Clarke, G., Shanahan, F., Dinan, T. G., and Cryan, J. F. (2014). Microbiota is essential for social development in the mouse. Mol. Psychiatry 2, 146–148. doi: 10.1038/mp.2013.65
Desbonnet, L., Clarke, G., Traplin, A., O’Sullivan, O., Crispie, F., Moloney, R. D., et al. (2015). Gut microbiota depletion from early adolescence in mice: implications for brain and behaviour. Brain Behav. Immun. 48, 165–173.
Diamond, B., Huerta, P. T., Tracey, K., and Volpe, B. T. (2011). It takes guts to grow a brain: increasing evidence of the important role of the intestinal microflora in neuro- and immune-modulatory functions during development and adulthood. BioEssays 8, 588–591. doi: 10.1002/bies.201100042
Diaz Heijtz, R., Wang, S., Anuar, F., Qian, Y., Björkholm, B., Samuelsson, A., et al. (2011). Normal gut microbiota modulates brain development and behavior. Proc. Natl. Acad. Sci. U.S.A. 7, 3047–3052. doi: 10.1073/pnas.1010529108
Fiest, K. M., Roberts, J. I., Maxwell, C. J., Hogan, D. B., Smith, E. E., Frolkis, A., et al. (2016). The prevalence and incidence of dementia due to Alzheimer’s disease: a systematic review and meta-analysis. Can. J. Neurol. Sci. 43(Suppl. 1), S51–S82. doi: 10.1017/cjn.2016.36
Finkelstein, J. A., Stille, C., Nordin, J., Davis, R., Raebel, M. A., Roblin, D., et al. (2003). Reduction in antibiotic use among US children, 1996-2000. Pediatrics 3(Pt 1), 620–627. doi: 10.1542/peds.112.3.620
Folley, S., Zhou, A., Llewellyn, D. J., and Hypponen, E. (2019). Physical activity, APOE genotype, and cognitive decline: exploring gene-environment interactions in the UK biobank. J. Alzheimers Dis. 3, 741–750. doi: 10.3233/JAD-181132
Fouhy, F., Ross, R. P., Fitzgerald, G. F., Stanton, C., and Cotter, P. D. (2012). Composition of the early intestinal microbiota: knowledge, knowledge gaps and the use of high-throughput sequencing to address these gaps. Gut Microbes 3, 203–220. doi: 10.4161/gmic.20169
Francino, M. P. (2015). Antibiotics and the human gut microbiome: dysbioses and accumulation of resistances. Front. Microbiol. 6:1543. doi: 10.3389/fmicb.2015.01543
Fröhlich, E. E., Farzi, A., Mayerhofer, R., Reichmann, F., Jaèan, A., Wagner, B., et al. (2016). Cognitive impairment by antibiotic-induced gut dysbiosis: analysis of gut microbiota-brain communication. Brain Behav. Immun. 56, 140–155. doi: 10.1016/j.bbi.2016.02.020
Hadar, N., Paul, F., Atara, U., Orly, A., and Omry, K. (2018). Antibiotics in early life: dysbiosis and the damage done. FEMS Microbiol. Rev. 42, 489–499. doi: 10.1093/femsre/fuy018
Hamad, A. F., Alessi-Severini, S., Mahmud, S., Brownell, M., and Kuo, I. F. (2020). Prenatal antibiotic exposure and risk of attention-deficit/hyperactivity disorder: a population-based cohort study. CMAJ 20, E527–E535. doi: 10.1503/cmaj.190883
Isaac, S., Scher, J. U., Djukovic, A., Jimenez, N., Littman, D. R., Abramson, S. B., et al. (2017). Short- and long-term effects of oral vancomycin on the human intestinal microbiota. J. Antimicrob. Chemother. 1, 128–136. doi: 10.1093/jac/dkw383
Kendall, K. M., Bracher-Smith, M., Fitzpatrick, H., Lynham, A., Rees, E., Escott-Price, V., et al. (2019). Cognitive performance and functional outcomes of carriers of pathogenic copy number variants: analysis of the UK Biobank. Br. J. Psychiatry 5, 297–304. doi: 10.1192/bjp.2018.301
Kindermann, I., Fischer, D., Karbach, J., Link, A., Walenta, K., Barth, C., et al. (2012). Cognitive function in patients with decompensated heart failure: the cognitive impairment in heart failure (CogImpair-HF) study. Eur. J. Heart Fail. 4, 404–413. doi: 10.1093/eurjhf/hfs015
Ko, H., Kim, S., Kim, K., Jung, S. H., Shim, I., Cha, S., et al. (2021). Genome-wide association study of occupational attainment as a proxy for cognitive reserve. Brain [Online ahead of print]. doi: 10.1093/brain/awab351
Korpela, K., Salonen, A., Virta, L. J., Kekkonen, R. A., Forslund, K., Bork, P., et al. (2016). Intestinal microbiome is related to lifetime antibiotic use in Finnish pre-school children. Nat. Commun. 7:10410. doi: 10.1038/ncomms10410
Kountouras, J., Boziki, M., Gavalas, E., Zavos, C., Grigoriadis, N., Deretzi, G., et al. (2009). Eradication of Helicobacter pylori may be beneficial in the management of Alzheimer’s disease. J. Neurol. 5, 758–767. doi: 10.1007/s00415-009-5011-z
Laake, K., and Oeksengaard, A. R. (2002). D-cycloserine for Alzheimer’s disease. Cochrane Database Syst. Rev. 2:CD003153. doi: 10.1002/14651858.CD003153
Lach, G., Fulling, C., Bastiaanssen, T. F. S., Fouhy, F., Donovan, A. N. O., Ventura-Silva, A. P., et al. (2020). Enduring neurobehavioral effects induced by microbiota depletion during the adolescent period. Transl. Psychiatry 10:382. doi: 10.1038/s41398-020-01073-0
Livingston, G., Sommerlad, A., Orgeta, V., Costafreda, S. G., and Mukadam, N. (2017). Dementia prevention, intervention, and care. Lancet 390, 2673–2734. doi: 10.1016/S0140-6736(17)31363-6
Ljubenkov, P. A., and Geschwind, M. D. (2016). Dementia. Semin. Neurol. 36, 397–404. doi: 10.1055/s-0036-1585096
Loeb, M. B., Molloy, D. W., Smieja, M., Standish, T., Goldsmith, C. H., Mahony, J., et al. (2004). A randomized, controlled trial of doxycycline and rifampin for patients with Alzheimer’s disease. J. Am. Geriatr. Soc. 52, 381–387. doi: 10.1111/j.1532-5415.2004.52109.x
Luchsinger, J. A., Pablos-Mendez, A., Knirsch, C., Rabinowitz, D., and Shea, S. (2001). Antibiotic use and risk of ischemic stroke in the elderly. Am. J. Med. 5, 361–366. doi: 10.1016/s0002-9343(01)00871-3
Lyall, D. M., Cullen, B., Allerhand, M., Smith, D. J., Mackay, D., Evans, J., et al. (2016). Cognitive test scores in UK biobank: data reduction in 480,416 participants and longitudinal stability in 20,346 participants. PLoS One 4:e0154222. doi: 10.1371/journal.pone.0154222
McVey Neufeld, K. A., Luczynski, P., Seira Oriach, C., Dinan, T. G., and Cryan, J. F. (2016). What’s bugging your teen?-the microbiota and adolescent mental health. Neurosci. Biobehav. Rev. 70, 300–312. doi: 10.1016/j.neubiorev.2016.06.005
Meeraus, W. H., Petersen, I., and Gilbert, R. (2015). Association between antibiotic prescribing in pregnancy and cerebral palsy or epilepsy in children born at term: a cohort study using the health improvement network. PLoS One 3:e0122034. doi: 10.1371/journal.pone.0122034
Molloy, D. W., Standish, T. I., Zhou, Q., Guyatt, G., and Group, D. S. (2013). A multicenter, blinded, randomized, factorial controlled trial of doxycycline and rifampin for treatment of Alzheimer’s disease: the DARAD trial. Int. J. Geriatr. Psychiatry 5, 463–470. doi: 10.1002/gps.3846
Mosaferi, B., Jand, Y., and Salari, A. A. (2021). Gut microbiota depletion from early adolescence alters anxiety and depression-related behaviours in male mice with Alzheimer-like disease. Sci. Rep. 11:22941. doi: 10.1038/s41598-021-02231-0
Neuman, H., Debelius, J. W., Knight, R., and Koren, O. (2015). Microbial endocrinology: the interplay between the microbiota and the endocrine system. FEMS Microbiol. Rev. 4, 509–521. doi: 10.1093/femsre/fuu010
Paus, T., Keshavan, M., and Giedd, J. N. (2008). Why do many psychiatric disorders emerge during adolescence? Nat. Rev. Neurosci. 12, 947–957. doi: 10.1038/nrn2513
Payne, L. E., Gagnon, D. J., Riker, R. R., Seder, D. B., Glisic, E. K., Morris, J. G., et al. (2017). Cefepime-induced neurotoxicity: a systematic review. Crit. Care 21:276. doi: 10.1186/s13054-017-1856-1
Rajji, T. K. (2018). Neurophysiology and cognitive reserve: a promising path. Clin. Neurophysiol. 129, 286–287. doi: 10.1016/j.clinph.2017.12.007
Sacuiu, S. F. (2016). Dementias. Handb. Clin. Neurol. 138, 123–151. doi: 10.1016/B978-0-12-802973-2.00008-2
Salthouse, T. A., and Pink, J. E. (2008). Why is working memory related to fluid intelligence? Psychon. Bull. Rev. 15, 364–371. doi: 10.3758/pbr.15.2.364
Shamriz, O., Mizrahi, H., Werbner, M., Shoenfeld, Y., Avni, O., and Koren, O. (2016). Microbiota at the crossroads of autoimmunity. Autoimmun. Rev. 9, 859–869. doi: 10.1016/j.autrev.2016.07.012
Slykerman, R. F., Thompson, J., Waldie, K. E., Murphy, R., Wall, C., and Mitchell, E. A. (2017). Antibiotics in the first year of life and subsequent neurocognitive outcomes. Acta Paediatr. 106, 87–94. doi: 10.1111/apa.13613
Sometti, D., Ballan, C., Wang, H., Braun, C., and Enck, P. (2021). Effects of the antibiotic rifaximin on cortical functional connectivity are mediated through insular cortex. Sci. Rep. 11:4479. doi: 10.1038/s41598-021-83994-4
Spor, A., Koren, O., and Ley, R. (2011). Unravelling the effects of the environment and host genotype on the gut microbiome. Nat. Rev. Microbiol. 4, 279–290. doi: 10.1038/nrmicro2540
Sturkenboom, M. C., Verhamme, K. M., Nicolosi, A., Murray, M. L., Neubert, A., Caudri, D., et al. (2008). Drug use in children: cohort study in three European countries. BMJ 337:a2245. doi: 10.1136/bmj.a2245
Tank, R., Ward, J., Flegal, K. E., Smith, D. J., Bailey, M. E. S., Cavanagh, J., et al. (2022). Association between polygenic risk for Alzheimer’s disease, brain structure and cognitive abilities in UK Biobank. Neuropsychopharmacology 2, 564–569. doi: 10.1038/s41386-021-01190-4
Tsai, G. E., Falk, W. E., Gunther, J., and Coyle, J. T. (1999). Improved cognition in Alzheimer’s disease with short-term D-cycloserine treatment. Am. J. Psychiatry 3, 467–469. doi: 10.1176/ajp.156.3.467
Turta, O., and Rautava, S. (2016). Antibiotics, obesity and the link to microbes - what are we doing to our children? BMC Med. 14:57. doi: 10.1186/s12916-016-0605-7
Verdu, F. E. (2006). Specific probiotic therapy attenuates antibiotic induced visceral hypersensitivity in mice. Gut 55, 182–190. doi: 10.1136/gut.2005.066100
Wang, T., Hu, X., Liang, S., Li, W., Wu, X., Wang, L., et al. (2015). Lactobacillus fermentum NS9 restores the antibiotic induced physiological and psychological abnormalities in rats. Benef. Microbes 5, 707–717. doi: 10.3920/bm2014.0177
Yassour, M., Vatanen, T., Siljander, H., Hamalainen, A. M., Harkonen, T., Ryhanen, S. J., et al. (2016). Natural history of the infant gut microbiome and impact of antibiotic treatment on bacterial strain diversity and stability. Sci. Transl. Med. 343:343ra81. doi: 10.1126/scitranslmed.aad0917
Yulug, B., Hanoglu, L., Ozansoy, M., Isık, D., Kilic, U., Kilic, E., et al. (2018). Therapeutic role of rifampicin in Alzheimer’s disease. Psychiatry Clin. Neurosci. 3, 152–159. doi: 10.1111/pcn.12637
Keywords: antibiotics, children, adolescents, cognitive impairment, middle-aged and older person(s), cohort study
Citation: Liu Z, Wei S, Chen X, Liu L, Wei Z, Liao Z, Wu J, Li Z, Zhou H and Wang D (2022) The Effect of Long-Term or Repeated Use of Antibiotics in Children and Adolescents on Cognitive Impairment in Middle-Aged and Older Person(s) Adults: A Cohort Study. Front. Aging Neurosci. 14:833365. doi: 10.3389/fnagi.2022.833365
Received: 11 December 2021; Accepted: 21 February 2022;
Published: 23 March 2022.
Edited by:
Robert Friedland, University of Louisville, United StatesReviewed by:
Ali-Akbar Salari, Radboud University Medical Center, NetherlandsYumi Watanabe, Niigata University, Japan
Copyright © 2022 Liu, Wei, Chen, Liu, Wei, Liao, Wu, Li, Zhou and Wang. This is an open-access article distributed under the terms of the Creative Commons Attribution License (CC BY). The use, distribution or reproduction in other forums is permitted, provided the original author(s) and the copyright owner(s) are credited and that the original publication in this journal is cited, in accordance with accepted academic practice. No use, distribution or reproduction is permitted which does not comply with these terms.
*Correspondence: Haihong Zhou, bWFvbWFvMDUzOEAxMjYuY29t; Duolao Wang, ZHVvbGFvLndhbmdAbHN0bWVkLmFjLnVr
†These authors have contributed equally to this work and share first authorship