- 1Laizhou City People's Hospital, Laizhou, Yantai, China
- 2Department of Pharmacy, Shanghai Changhai Hospital, Naval Medical University, Shanghai, China
Objective: In order to explore and further understand the efficacy of donepezil (DNP) in the treatment of Alzheimer's disease (AD), this research was conducted based on network pharmacology and molecular docking.
Method: Compounds of DNP and its effective targets were collected using the TCMSP Chinese medicine system pharmacology database. Disease targets were screened and selected utilizing GeneCards, TTD, DrugBank, CTD, and other online databases. Then, Venn diagrams were generated to identify the intersections. A diseases-drug-active ingredient-key target protein interaction (PPI) network was constructed using the STING database. GO and KEGG enrichment analyses were conducted to predict the function and mechanism of DNP, which were visualized by graphs and bubble charts. After the screening, the top five interacting targets in the PPI network and the compound containing the most active target were selected for molecular docking.
Results: The study received 110 potential targeting genes and 155 signaling pathways. A strong association between DNP and modulation of chemical synaptic transmission and the regulation of trans-synaptic signaling is noted. Signaling pathways related to the proliferation, differentiation, and survival of cells are also found positively relative. The results revealed that the mechanism of its therapeutic effect is multi-component, multi-target, and multi-pathway, laying a foundation for the follow-up in-depth study of the mechanism of DNP in the treatment of AD.
Conclusion: This research provides a superior prediction that AD could be treated using DNP which targets the key proteins and essential pathways associated with the recovery of AD.
Introduction
Alzheimer's disease (AD) is a neurodegenerative disorder in the central nervous system, prevalently observed among the elderly and near-elderly, characterized by progressive cognitive impairment, and memory degeneration (Hebert et al., 1995; Alzheimer's, 2016).
Credited as the most common cause of dementia, AD is known for its patients suffering from a long course of the disease, loss of self-care ability, and eventual death from comorbidities, identified by the World Health Organization as a prior global public health concern (Barker et al., 2002; Wilson et al., 2012).
In 2020, an estimated number of AD cases worldwide was reported to be 24 million, which was predicted to increase fourfold by the year 2050 (Breijyeh and Karaman, 2020).
Since the first AD case was reported in 1907 by Alois Alzheimer, significant gains in our understanding of the etiology and pathogenesis of AD have been achieved. Nevertheless, current therapeutic strategies for AD remain considerably limited, with only two alternatives available, including naturally derived and synthetic inhibitors or hybrid analogs to cholinesterase enzyme, and antagonists to N-methyl D-aspartate (NMDA). Despite the effectiveness of the existing therapies in treating the symptoms of AD, no significant influence has been found in curing or preventing the disease (Wang and Reddy, 2017). Acetylcholinesterase inhibitors (AChEIs) are categorized as the former, blocking cholinesterase enzymes from degrading ACh to increase ACh levels in the synaptic cleft. For patients with all levels of AD, whether mild, moderate, or severe (Winblad et al., 2001), donepezil (DNP), an AChEI, is wildly commended in China as the most promising therapeutic candidate for AD (Deardorff and Grossberg, 2016). Studies have confirmed the efficacy and safety of DNP for patients with mild to moderate AD and its possible effectiveness for severe AD (Zhang and Gordon, 2018). However, it should also be noted that DNP is likewise only capable of treating the symptoms of AD but cannot reverse or alter the AD progression (Cacabelos, 2007).
The insufficient understanding of the pathogenesis of AD sets great obstacles in the path of finding a novel breakthrough in the improvement of the existing drugs. Therefore, the investigation of the mechanism of DNP as a drug for AD treatment should be highly prioritized.
Recently, studies concerning the mechanism of DNP are extremely limited. In 2019, Ghosh et al. revealed the mechanistic pathway of DNP-induced cholinergic inhibition of AD via docking and well-tempered metadynamics (WTMtD) stimulations (Duggleby et al., 2019).
Method
First, the structural formula of donepezil was downloaded from PubChem (https://pubchem.ncbi.nlm.nih.gov/). With the structure acquired, data of the donepezil targeted sites were collected from four separate databases: swisstarget (http://www.swisstargetprediction.ch/), pharmmapper (http://lilab-ecust.cn/pharmmapper/), batman-tcm (http://bionet.ncpsb.org.cn/batman-tcm/), and stitch (http://stitch.embl.de/), each with a different standard for data selection. For swisstarget, data with Probability*>0 was included. For batman-tcm, the inclusion standard was set Score cutoff≥ 20. No standard was set for screening in stitch or pharmmapper. The corresponding genes of the donepezil target sites were collected through Uniprot (http://www.uniprot.org/www.uniprot.org/), utilizing data downloaded in pharmmapper.
Three databases were utilized in the searching of pathogenic genes of AD. In GeneCards, genes with a relevance score ≥15 were enrolled. In OMIM, 142 AD-associated genes were included with no screening conducted. In disgenet, genes with score gda ≥0.2 were included. Afterward, data from the three distinct databases were merged and had duplicates removed, leaving 819 distinct genes related to the pathogenesis of AD for subsequent research.
With the screened disease targets, intersections were taken and Venn diagrams were drawn. Four hundred and fourty nine drug targets, 819 disease targets, and 110 intersection targets were obtained. All duplications were removed.
The STRING database was used to construct a disease-drug-active ingredient-key target network, with the minimum required interaction score set as 0.4 and the isolated vertices removed. A target protein interaction network was constructed using Cytoscape3.7.2 software.
With ALB as the brightest in the string hires image and the highest in the string interaction chart, intersections related to ALB are therefore presumed to be of the highest importance.
The intersections of the core genes were collected using the R package with PPI data inputted.
Genes for potential target sites were selected using the R package from the “org.Hs.eg.db” database, and GO enrichment analysis was conducted using DOSE, clusterProfiler, and pathview package (Bioconductor) from three aspects, Biological Process (BP), Cellular Component (CC), and Molecular Function (MF), with p-value cutoff and q-value cutoff both as 0.05. The results from each aspect were visualized with a graph and bubble chart.
With the selected genes, KEGG pathway enrichment analysis was conducted to predict its mechanism of action using DOSE, clusterProfiler, and pathview packages (Bioconductor), with p-value cutoff and q-value cutoff both as 0.05. Columns and bubble charts were generated to visualize the result.
Results
Data Processing of Donepezil
To acquire the structure of donepezil, the associating structure with Probability*>0, score cutoff≥20 was screened and downloaded from the swisstarget and batman-tcm databases. The structure was also searched in the stitch and pharmmapper databases to further verify the results. The structure was visualized using PubChem (Figure 1A) With the selected structure, the associating genes were screened in the Uniprot database (Table 1).
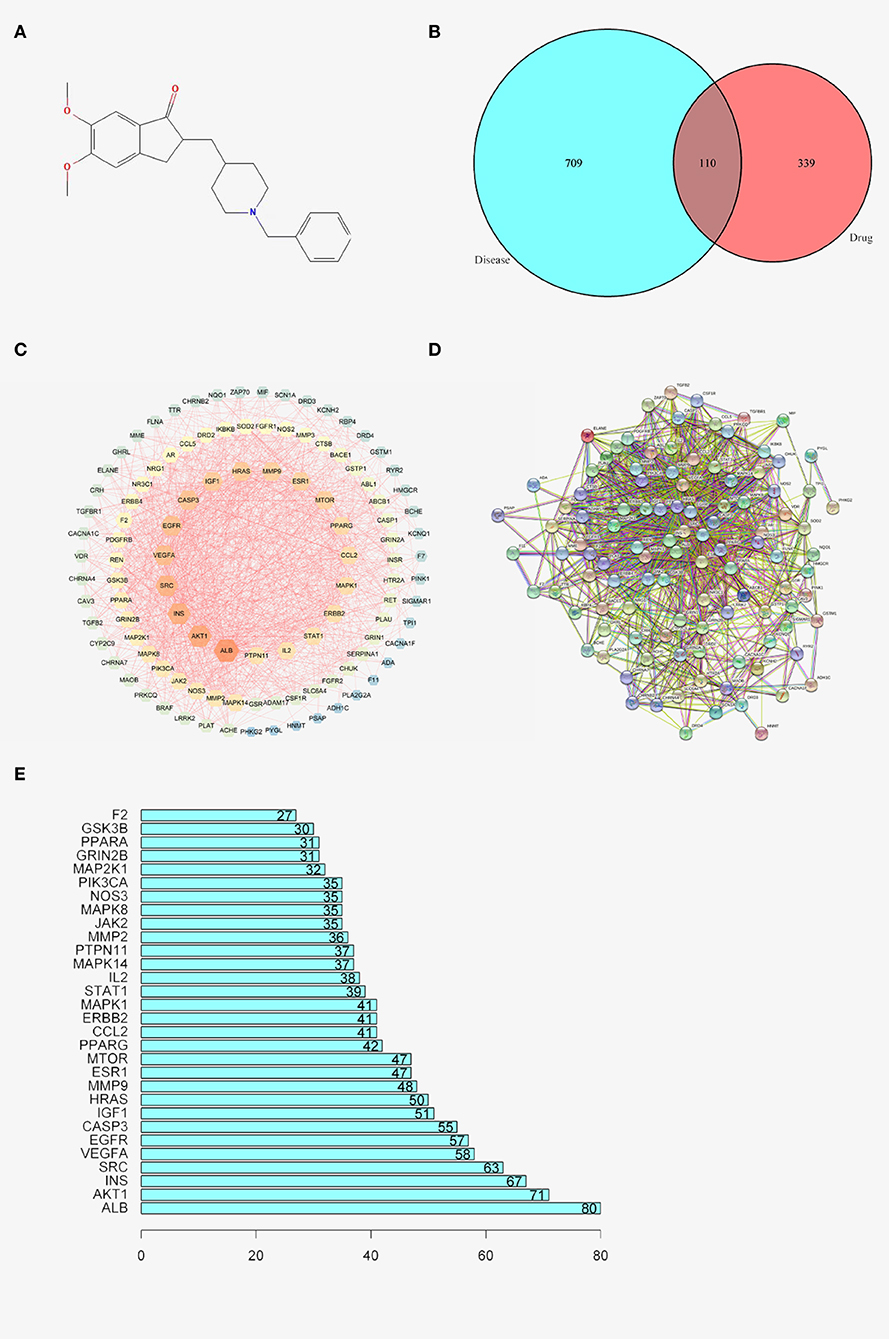
Figure 1. (A) 2D structure of DNP. (B) Venn graph of AD and DNP. (C) Diseases- drug-active ingredient-key target network. (D) PPI network. (E) Bar chart of 30 core genes.
Data Processing of AD
A total of 709, 142, and 154 associating genes are screened from the GeneCards, omim, and disgenet database, with the relevance score≥15 and score gda≥0.2. A final number of 819 genes are included after the duplicates were removed (Table 2). Among these genes, 449 genes are related to the drug donepezil, 819 are related to AD, and 110 intersection genes are noted. All the above research into the genes was conducted with the duplicates removed. Venny2.1 (https://bioinfogp.cnb.csic.es/tools/venny/) was utilized to visualize the intersection result (Figure 1B and Table 3).
Construction and Analysis of Disease-Drug-Active Ingredient-Key Target Network
The STRING database was used to construct a disease-drug-active ingredient-key target network, with the minimum required interaction score set as 0.4 and the isolated vertices removed (Figure 1C). The brightness, the bigger the dots are, the higher the degree is, representing the importance of the spot to the network.
Construction and Analysis of PPI Network
To further investigate the mechanism of donepezil's therapeutic effect on AD, 110 intersecting genes were enrolled into the STRING database for PPI network analysis. One hundred and nine core genes and their interacting lines were screened and visualized (Figure 1D and Table 4). The intersections of the core genes were collected using the R package with inputted PPI data. Thirty core genes were finally screened and visualized (Figure 1E).
GO and KEGG Enrichment Analysis
Genes for potential target sites were selected using the R package from “org.Hs.eg.db” database, and GO enrichment analysis was conducted using the DOSE, clusterProfiler, and pathview packages (Bioconductor) from three aspects, Biological Process (BP), Cellular Component (CC), and Molecular Function (MF), with p-value cutoff and q-value cutoff both as 0.05. The results from each aspect were visualized with a bubble and bar chart (Figures 2A,B). 30 GO lists are acquired (P < 0.05, FDR < 0.05), including 10 BP, 10CC, and 10 MF. Therefore, it can be concluded that the mechanism of the therapeutic effect of donepezill to AD could be associated with biological processes such as positive regulation of RNA polymerase II promoter, signal transduction, and positive regulation of DNA template transcription. At the same time, a variety of substances such as plasma membrane, cytosol, and extracellular space are involved, such as protein binding, enzyme binding, transcription factor activity, sequence-specific DNA binding, same protein binding, and other molecular functions.
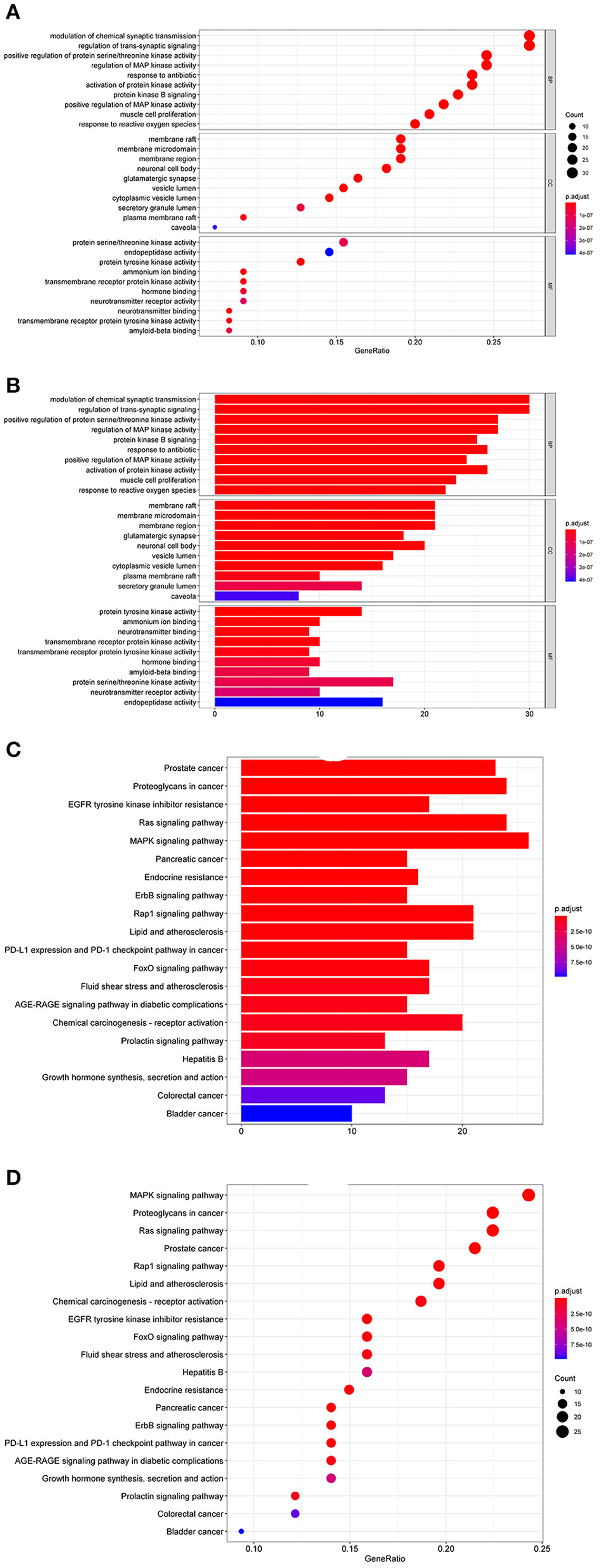
Figure 2. (A) Bubble graph of GO enrichment analysis. (B) Bar chart of GO enrichment analysis. (C) Bar chart of KEGG enrichment analysis. (D) Bubble graph of KEGG enrichment analysis.
In KEGG pathway enrichment analysis, 155 signaling pathways are screened (P < 0.05, FDR < 0.05), and the top 20 are listed by Count number (Figure 2C). The KEGG analysis indicates that the genes are especially enriched in the MAPK signaling pathway, Ras signaling pathway, and Proteoglycan in cancer. An advanced bubble graph is formed based on the above results, with the signaling pathways as the ordinate and the gene ratio as the abscissa. The count value represents the number of targets enriched on the signal path. The larger the bubble, the more targets enriched, and the color of the bubble represents the p-value (Figure 2D).
Discussion
The development of drugs with clinical value has met with extreme obstacles (Becker et al., 2008). Over the past 50 years, only two types of drugs are approved for the treatment of AD, which are cholinesterase inhibitors and memantine. Approximately 200 drugs were advanced to at least phase 2 development. However, the therapeutic effects of the approved drugs are limited and there have been disputes on whether they are clinically useful (Schneider et al., 2014).
DNP is a kind of AChEI, which functions as an inhibitor to the cholinesterase enzyme and sustains the activity of acetylcholine at cholinergic synapses. DNP has been shown to significantly improve cognition and daily function and some behavior manifestations of patients with AD (Massoud and Gauthier, 2010). In this study, DNP was taken as the research object. By using the methods of network pharmacology and molecular docking, through the establishment of a disease-drug-active ingredient-key target network, the possible targets and signaling pathways in the treatment of AD were analyzed. The results revealed that the mechanism of its therapeutic effect is multi-component, multi-target, and multi-pathway, laying a foundation for the follow-up in-depth study of the mechanism of DNP in the treatment of AD.
The study received 110 potential targeting genes and 155 signaling pathways in total. The diversity of the associated genes and pathways demonstrate that the mechanism of DNP in the treatment of AD is multifactorial. A strong association between DNP and modulation of chemical synaptic transmission and the regulation of trans-synaptic signaling is noted, further confirming the ability of DNP in sustaining the activity of synapses. In addition, signaling pathway related to the proliferation, differentiation, and survival of cells are also found positively relative, such as Ras and MAPK signaling pathways, suggesting a potential of promoting the growth and proliferation of neurons using DNP.
The cholinergic system plays an indispensable role in neuronal function in memory, learning, and other essential aspects of cognition and plays a wider role in the promotion of neuronal plasticity (Drachman and Leavitt, 1974). Human studies of AD have shown that lesion of the cholinergic system, emerging as early as asymptomatic or prodromal stages of the disease, is one of the most important factors (Schroder et al., 1991). It has been discovered that promotion of cholinergic function in patients with AD may also have durable beneficial biological effects on the brain besides a temporary augmentation of cognitive function (Cavedo et al., 2016, 2017). Cholinesterase inhibitors are designed to inhibit the acetylcholine breakdown and sustain its activity at synapses. There has been evidence that DNP can restore cholinergic function by blocking the enzymes that break down acetylcholine (Lovestone and Howard, 1995). DNP belongs to the most currently available FDA-approved cholinesterase inhibitors for the treatment of AD (Hogan, 2007; Massoud and Gauthier, 2010). Since the number of patients with AD has been on the rise in recent decades, and is becoming a severe social concern, the need to develop an effective drug or therapeutic management for AD is urgent. It is indicated that the implication of cholinesterase inhibitor significantly reduces the risk for nursing home placement by ~30% annually (Feldman et al., 2009). In future studies, more attention should be paid on the mechanism of the pathogenesis of AD and the mechanism of DNP for AD treatment.
In this study, due to the incomplete information in the database and unclear interaction between DNP and other factors, the prediction results have certain limitations. This study did not verify the possible molecular mechanism of DNP in the treatment of AD syndromes either in vivo or in vitro, and deficiencies remain noticeable. However, this study can provide new ideas and directions for further exploration of relevant experiments.
Data Availability Statement
The simulation experiment data used to support the findings of this study are available from the corresponding author upon request.
Author Contributions
LL and PF prepared the figures. JY and YZ finished the paper. All authors contributed to the article and approved the submitted version.
Funding
This work was supported in part by the Science and Technology Commission of Shanghai Municipality, General Program, No.20ZR1456400.
Conflict of Interest
The authors declare that the research was conducted in the absence of any commercial or financial relationships that could be construed as a potential conflict of interest.
Publisher's Note
All claims expressed in this article are solely those of the authors and do not necessarily represent those of their affiliated organizations, or those of the publisher, the editors and the reviewers. Any product that may be evaluated in this article, or claim that may be made by its manufacturer, is not guaranteed or endorsed by the publisher.
References
Alzheimer's (2016). Alzheimer's disease facts and figures. Alzheimers Dement. 12, 459–509. doi: 10.1016/j.jalz.2016.03.001
Barker, W. W., Luis, C. A., Kashuba, A., Luis, M., Harwood, D. G., Loewenstein, D., et al. (2002). Relative frequencies of Alzheimer disease, lewy body, vascular and frontotemporal dementia, and hippocampal sclerosis in the State of Florida Brain Bank. Alzheimer. Dis. Assoc. Disord. 16, 203–212. doi: 10.1097/00002093-200210000-00001
Becker, R. E., Greig, N. H., and Giacobini, E. (2008). Why do so many drugs for Alzheimer's disease fail in development? Time for new methods and new practices? J. Alzheimers Dis. 15, 303–325. doi: 10.3233/JAD-2008-15213
Breijyeh, Z., and Karaman, R. (2020). Comprehensive review on Alzheimer's disease: causes and treatment. Molecules 25:5789. doi: 10.3390/molecules25245789
Cacabelos, R. (2007). Donepezill in Alzheimer's disease: from conventional trials to pharmacogenetics. Neuropsychiatr. Dis. Treat. 3, 303–333.
Cavedo, E., Dubois, B., Colliot, O., Lista, S., Croisile, B., Tisserand, G. L., et al. (2016). Reduced regional cortical thickness rate of change in donepezill-treated subjects with suspected prodromal Alzheimer's disease. J. Clin. Psychiatry 77:e1631–e1638. doi: 10.4088/JCP.15m10413
Cavedo, E., Grothe, M. J., Colliot, O., Lista, S., Chupin, M., Dormont, D., et al. (2017). Reduced basal forebrain atrophy progression in a randomized donepezill trial in prodromal Alzheimer's disease. Sci. Rep. 7:11706. doi: 10.1038/s41598-017-09780-3
Deardorff, W. J., and Grossberg, G. T. (2016). Fixed-dose combination of memantine extended-release and donepezill in the treatment of moderate-to-severe Alzheimer's disease. Drug Des. Devel. Ther. 10, 3267–3279. doi: 10.2147/DDDT.S86463
Drachman, A., and Leavitt, J. (1974). Human memory and the cholinergic system. A relationship to aging? Arch. Neurol. 30, 113–121. doi: 10.1001/archneur.1974.00490320001001
Duggleby, W., Ploeg, J., McAiney, C., Fisher, K., Jovel Ruiz, K., Ghosh, S., et al. (2019). A comparison of users and nonusers of a web-based intervention for carers of older persons with alzheimer disease and related dementias: mixed methods secondary analysis. J. Med. Internet. Res. 21:e14254. doi: 10.2196/14254
Feldman, H. H., Pirttila, T., Dartigues, J. F., Everitt, B., Van Baelen, B., Schwalen, S., et al. (2009). Treatment with galantamine and time to nursing home placement in Alzheimer's disease patients with and without cerebrovascular disease. Int. J. Geriatr. Psychiatry 24, 479–488. doi: 10.1002/gps.2141
Hebert, L. E., Scherr, P. A., Beckett, L. A., Albert, M. S., Pilgrim, D. M., Chown, M. J., et al. (1995). Age-specific incidence of Alzheimer's disease in a community population. JAMA 273, 1354–1359. doi: 10.1001/jama.1995.03520410048025
Hogan, D. B. (2007). Progress update: pharmacological treatment of Alzheimer's disease. Neuropsychiatr. Dis. Treat. 3, 569–578.
Lovestone, S., and Howard, R. (1995). Alzheimer's disease: a treatment in sight? J. Neurol. Neurosurg. Psychiatry 59, 566–567. doi: 10.1136/jnnp.59.6.566
Massoud, F., and Gauthier, S. (2010). Update on the pharmacological treatment of Alzheimer's disease. Curr. Neuropharmacol. 8, 69–80. doi: 10.2174/157015910790909520
Schneider, L. S., Mangialasche, F., Andreasen, N., Feldman, H., Giacobini, E., Jones, R., et al. (2014). Clinical trials and late-stage drug development for Alzheimer's disease: an appraisal from 1984 to 2014. J. Intern. Med. 275, 251–283. doi: 10.1111/joim.12191
Schroder, H., Giacobini, E., Struble, R. G., Zilles, K., and Maelicke, A. (1991). Nicotinic cholinoceptive neurons of the frontal cortex are reduced in Alzheimer's disease. Neurobiol. Aging. 12, 259–262. doi: 10.1016/0197-4580(91)90107-U
Wang, R., and Reddy, P. H. (2017). Role of glutamate and NMDA receptors in alzheimer's disease. J. Alzheimers Dis. 57, 1041–1048. doi: 10.3233/JAD-160763
Wilson, R. S., Segawa, E., Boyle, P. A., Anagnos, S. E., Hizel, L. P., Bennett, D. A., et al. (2012). The natural history of cognitive decline in Alzheimer's disease. Psychol. Aging. 27, 1008–1017. doi: 10.1037/a0029857
Winblad, B., Engedal, K., Soininen, H., Verhey, F., Waldemar, G., Wimo, A., et al. (2001). A 1-year, randomized, placebo-controlled study of donepezill in patients with mild to moderate AD. Neurology 57, 489–495. doi: 10.1212/WNL.57.3.489
Keywords: donepezil, Alzheimer's disease, network pharmacolgy, mechanism, prognosis
Citation: Liu L, Zhu Y, Fu P and Yang J (2022) A Network Pharmacology Based Research on the Mechanism of Donepezil in Treating Alzheimer's Disease. Front. Aging Neurosci. 14:822480. doi: 10.3389/fnagi.2022.822480
Received: 25 November 2021; Accepted: 11 February 2022;
Published: 08 April 2022.
Edited by:
Yuanpeng Zhang, Nantong University, ChinaReviewed by:
Hao Chen, Hunan University, ChinaLuobing Dong, Xidian University, China
Lin Haifeng, McMaster University, Canada
Copyright © 2022 Liu, Zhu, Fu and Yang. This is an open-access article distributed under the terms of the Creative Commons Attribution License (CC BY). The use, distribution or reproduction in other forums is permitted, provided the original author(s) and the copyright owner(s) are credited and that the original publication in this journal is cited, in accordance with accepted academic practice. No use, distribution or reproduction is permitted which does not comply with these terms.
*Correspondence: Jundong Yang, 1295218809@qq.com; Peng Fu, fucaofan@163.com
†These authors have contributed equally to this work and share first authorship