- 1Eye Center of Xiangya Hospital, Central South University, Changsha, China
- 2Hunan Key Laboratory of Ophthalmology, Changsha, China
- 3Department of Neurology, Xiangya Hospital, Central South University, Changsha, China
- 4National Clinical Research Center for Geriatric Disorders, Xiangya Hospital, Central South University, Changsha, China
- 5Engineering Research Center of Hunan Province in Cognitive Impairment Disorders, Central South University, Changsha, China
- 6Hunan International Scientific and Technological Cooperation Base of Neurodegenerative and Neurogenetic Diseases, Changsha, China
- 7Key Laboratory of Hunan Province in Neurodegenerative Disorders, Central South University, Changsha, China
Background: Retinal microvascular density has been studied in neurodegenerative diseases, whereas patients with Parkinson’s disease (PD) at different clinical stages have been rarely investigated. The present study aimed to evaluate the microvascular variations in superficial retinal capillary plexus (SCP) in patients with PD on different Hoehn-Yahr (H-Y) stages by optical coherence tomography angiography (OCTA), as well as determine their relationships with clinical parameters.
Methods: In total, 115 patients with PD and 67 healthy controls (HCs) were recruited. The PD group was divided into three groups based on the H-Y stage. The OCTA examination was performed in all participants, and the macular vessel density (m-VD), peripapillary vessel density (p-VD), and foveal avascular zone (FAZ) area were measured.
Results: The m-VD in all regions, p-VD in center [6.1 (4.8, 6.95) mm–1 in healthy eyes vs. 5.1 (3.7, 6.4) mm–1 in patients], nasal inner (NI) [18.5 (17.8, 19.3) mm–1 in healthy eyes vs. 17.9 (17.1, 18.7) mm–1 in patients], temporal outer (TO) [19.6 (18.9, 20.2) mm–1 in healthy eyes vs. 19.3 (18.5, 19.7) mm–1 in patients] regions and FAZ area [0.36 (0.32, 0.39) mm2 in healthy eyes vs. 0.29 (0.26, 0.33) mm2 in patients] noticeably decreased in PD groups compared with HC (p < 0.05). Moreover, the FAZ area was suggested to decline significantly in patients with PD with H-Y I stage (p < 0.05), while it was more serious in the H-Y III stage in patients. Furthermore, we found that m-VD exhibited a significant negative correlation with age, disease duration, UPDRS scores, NMSS scores, and H-Y stage.
Conclusion: OCTA has the potential to non-invasively detect the microvascular changes in patients with PD with different clinical stages in vivo, and it may be a valuable tool to monitor the PD progression.
Introduction
Parkinson’s disease (PD) refers to the second most common disease among central neurodegenerative conditions, affecting numerous parts of the brain and showing relationships to motor dysfunction and cognitive impairment in the elderly (Kwapong et al., 2018). This disease is caused by the depletion of dopaminergic neurons. Dopamine (DA) acts as a major neurotransmitter in the retina, which critically regulates the functions of other neurochemical systems (e.g., gamma-aminobutyric acid, glycine, and glutamate in the retina) (Sengupta et al., 2018; Yıldız et al., 2019). PD is recognized as a multisystemic pathology with both motor and non-motor manifestations (de Rijk et al., 1995; de Lau and Breteler, 2006), and patients with PD develop specific visual symptoms (e.g., decreased visual acuity, deteriorated contrast sensitivity, and difficulties in visuospatial orientation) (Archibald et al., 2011; Bodis-Wollner et al., 2014; Mohana Devi et al., 2020).
The eye is regarded as a gateway to the brain, where precise functional/structural relationships are developed. Moreover, PD research primarily aims to develop methods of achieving accurate early diagnosis and to monitor disease progression in individual subjects. For this reason, if the exact cellular function/structure relationship is developed in the retina, the eye can act as a tool to conduct the pathobiological study of PD (MacCormick et al., 2015). The optical coherence tomography angiography (OCTA) enables the capture of direct non-invasive retinal imaging and the study of the microvasculature in the retina qualitatively and quantitatively. Microvascular measurements in OCTA are summarized as vessel density in the respective subfield, which facilitates the detailed study of neuronal and microvascular in PD with high-quality images (Uchida et al., 2018). Meanwhile, only a few studies reported the relationship between retinal microvascular density and PD (Kwapong et al., 2018; Shi et al., 2020). However, no consensus has been reached on retinal vascular variation in patients with PD grouped by Hoehn-Yahr (H-Y) stage with OCTA. It is hypothesized that the microvascular changes in the retina may represent differences between the diverse clinical stages, especially when the patients with PD progress from minimal to severe stage. Accordingly, this study is the first to evaluate the diagnostic significance of macular and peripapillary vessel density (m-VD and p-VD) and FAZ through OCTA in patients with PD at different clinical stages with Hoehn-Yahr staging scores. Moreover, the relationships between quantitative OCTA measures and PD clinical parameters were analyzed.
Materials and Methods
Enrollment of Participants
In total, 115 patients with PD from Xiangya Hospital between October and December 2019 were recruited. 67 age- and gender-matched HC were recruited from the family members of patients with PD or colleagues in the hospital of the identical period. To avoid the correlation of intrasubject inter-eyes, we selected the random eye from each subject. Prior to the participation here, all participants provided written informed consent forms. The diagnosis of PD was diagnosed in accordance with the “Movement Disorder Society (MDS) clinical diagnostic criteria for Parkinson’s disease” (Postuma et al., 2015). The respective patient was assessed at the H-Y staging scale (Goetz et al., 2004) and Unified Parkinson’s Disease Rating Scale (UPDRS; Goetz et al., 2008). Mini-Mental State Examination (MMSE) and Non-motor Symptoms Scale (NMSS) were adopted to assess non-motor symptoms. By complying with the H-Y stage, the patients with PD in this study fell to the following three groups, i.e., the H-Y I stage group, the H-Y II stage group, and the H-Y III stage group.
Demographic and neurologic data consisted of age, sex, and disease duration. All participants were between 50 and 80 years old. The exclusion criteria were presented as the patients with (1) glaucoma, age-related macular degeneration, macular hole, epiretinal membrane, refractive error [> ±3 diopters (D) sphere and ±2D cylinder], macular drusen, as well as pathological myopia, (2) diabetes and uncontrolled hypertension, (3) concomitant neurological disorders, (4) severe cardiac, pulmonary, hepatic, renal diseases, or any types of tumors, and (5) insufficient clinical data. This study was approved by the Research Ethics Committee of Xiangya Hospital, Central South University. The study abided by the Declaration of Helsinki, which was performed by complying with the approved guidelines and regulations.
Optical Coherence Tomography Angiography Imaging
All participants underwent retina microvasculature imaging based on the Cirrus 5000 HD-OCT (Carl Zeiss Meditec, Inc., Dublin, CA, United States). The m-VD and p-VD in superficial retinal capillary plexus (SCP) was analyzed throughout the examined area of 6 mm × 6 mm that was centered on the macula and peripapillary, and they consisted of vessels from the layer of the inner limiting membrane (ILM) to the inner plexiform layer (IPL). In addition, the vessel density was measured in nine subfields of the Early Treatment Diabetic Retinopathy Study (ETDRS) grid (e.g., a central 1-mm circle), respectively, representing the foveal area, and inner and outer rings of diameters 3 and 6 mm, (Figures 1A,B). The inner and outer rings fell to four quadrants, i.e., superior, nasal, inferior, and temporal (Figures 1A,B). The foveal avascular zone (FAZ) area was automatically segmented and then quantified with the OCTA software. The quality of every image was manually checked by a professional ophthalmologist (XB), and the random eye was scanned according to time constraints. Only images of good quality were covered, and images with poor signal strength (signal strength index <7) or motion artifacts visible were excluded here. The observer was blinded to the clinical diagnosis of the participants.
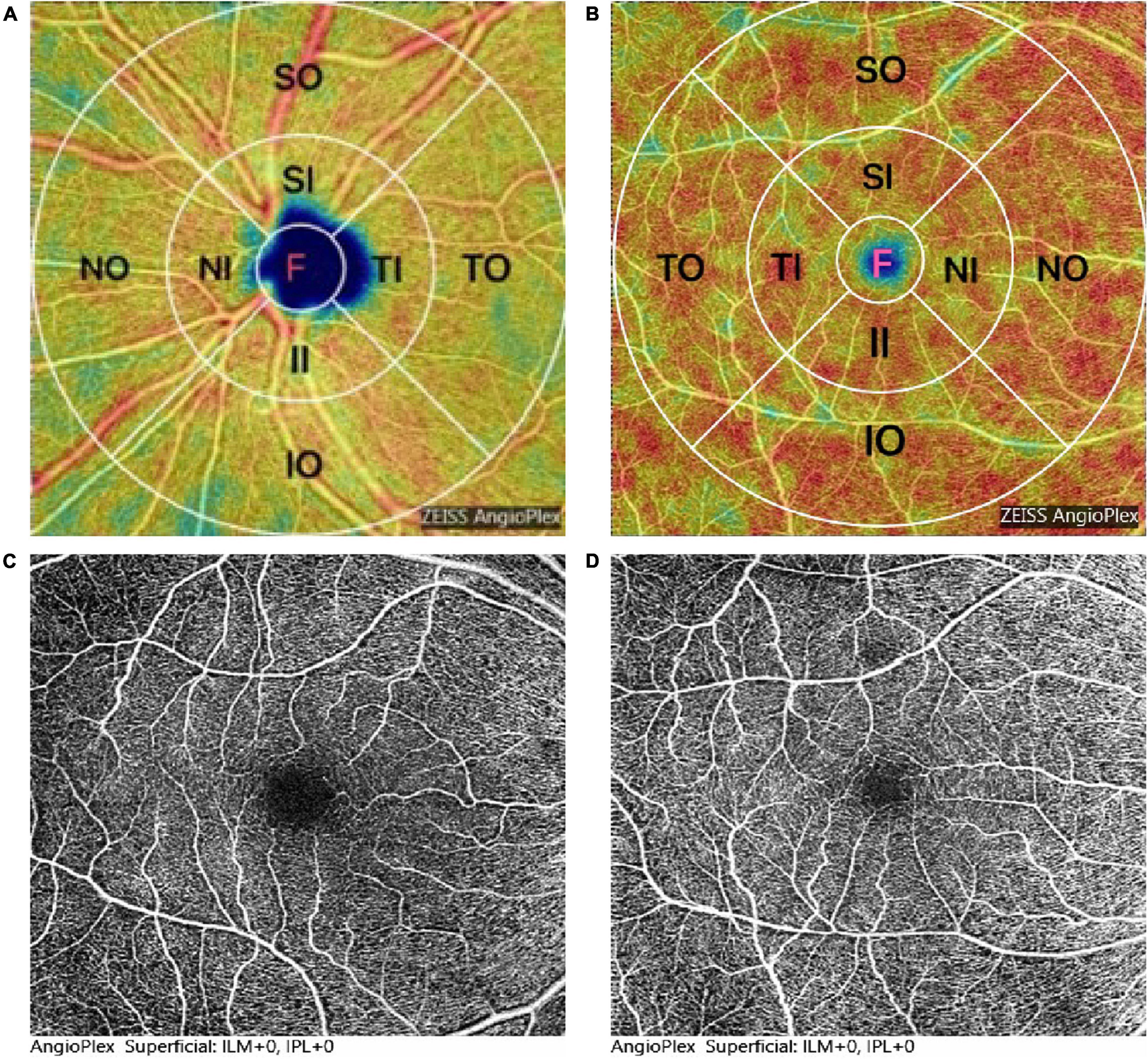
Figure 1. Representation of optical coherence tomography angiography (OCTA) image and measurement of the density analysis of the superficial retinal capillary plexus (SCP). (A,B) Map of macular and peripapillary, 1 mm central circle, representing the center area, and inner and outer rings of diameters 3 and 6 mm, respectively. The inner and outer rings were divided into four quadrants: superior, nasal, inferior, and temporal. (C,D) Foveal avascular zone (FAZ) area was significantly decreased in patients with Parkinson’s disease (PD) (D) compared to healthy controls (HC) (C).
Statistics Analysis
For all analyses in this study, SPSS version 25 (SPSS Inc., Chicago, IL, United States) was employed. Sex differences between the groups were compared by performing the Pearson Chi-square test. The general features including age, systolic blood pressure (SBP), and ophthalmologic parameters (IOP, BCVA, AL) were expressed as mean ± the SDs. The OCTA measures were expressed as median (25% quartile, 75% quartile). Student’s t-test was performed to assess the difference between PD and HC groups. To compare the differences between PD subgroups, the results of this study were analyzed with one-way ANOVA based on the LSD Post Hoc test. The Benjamini–Hochberg Adjusted p-value was calculated to control the false discovery rate (FDR), and the FDR was set at 0.05. Variables not complying with a normal distribution were analyzed by performing a non-parametric test to compare the differences between groups. Correlations between data were tested by Spearman’s correlation. The differences between groups were considered to show statistical significance when p < 0.05.
Results
A total of 182 participants were recruited in this study, which covered 67 HC, 50 H-Y I stage patients, 46 H-Y II stage patients, and 19 H-Y III stage patients. No statistical difference was identified in age distribution (p = 0.151), sex (p = 0.889), SBP (p = 0.645), intraocular pressure (IOP) (p = 0.583), best-corrected VA (BCVA) (p = 0.839), and axial lengths (AL) (p = 0.357) between PD and HC group (Table 1). Moreover, no significant differences were reported among the subgroups of patients with PD. Furthermore, the patients with PD with the H-Y III stage exhibited longer disease duration, higher UPDRS, UPDRS III, NMSS scores, and lower MMSE scores (p < 0.05). Table 1 lists the general demographics, ophthalmic features of all participants, and clinical parameters.
Macular Vessel Density Parameters
Figure 2A and Table 2 presents the m-VD parameters of all participants. The results demonstrated that compared with HC groups, m-VD in the PD group declined noticeably in all subfields (all p < 0.05) (Figure 2A and Table 2). Moreover, quadrantal analyses indicated the FAZ area decreasing significantly in the SCP of the patients with PD in comparison with the HC group [0.36 (0.32, 0.39) mm2 in healthy eyes vs. 0.29 (0.26, 0.33) mm2 in patients, p < 0.001] (Figures 1C,D). As revealed from the results of the one-way ANOVA analysis, the H-Y III stage group showed significantly decreased m-VD in the fovea, SI, II, NI, TI, TO, and lower FAZ area (all p < 0.05). However, a statistically significant difference was not identified in the SO, IO, and NO subfield for the comparison of PD subgroups (Table 2). In addition, results suggested that patients with PD with H-Y I stage showed FAZ area noticeably declining early (p < 0.05). Moreover, the m-VD in all grids in patients with PD with H-Y III were significantly lower than those in healthy eyes. Specific to the whole inner ring and outer ring index, statistically significant differences were reported for the PD and HC group, or PD subgroups (p < 0.05) (Table 2).
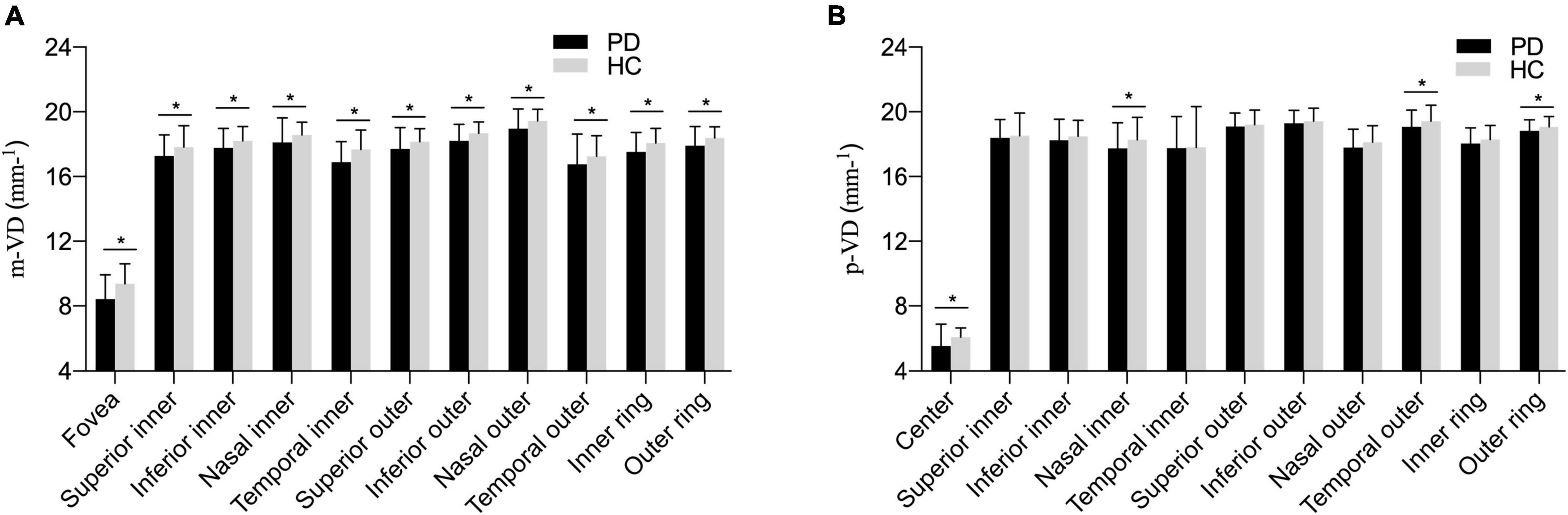
Figure 2. The comparison of vessel density parameters among different PD subgroups (A) in the macula area; (B) in the peripapillary area. Compared with HC groups, macular vessel density (m-VD) in the PD group was significantly declined in all quadrants. Additionally, peripapillary vessel density (p-VD) was found significantly decreased in the fovea, nasal inner (NI), temporal outer (TO), and outer ring regions in the PD group, *p < 0.05.
Peripapillary Vessel Density Parameters
The p-VD parameters of all participants are presented in Figure 2B and Table 3. As indicated from the result, no significant difference of p-VD was found in all the subfields of the peripapillary scan between PD and HC groups (Figure 2B and Table 3). Meanwhile, no statistically significant difference was reported in p-VD among PD subgroups (p > 0.05) (Table 3).
Correlation Between Vessel Density and Other Parameters
The correlation of m-VD of patients with PD with clinical parameters was analyzed, (e.g., age, sex, disease duration, UPDRS, UPDRS III, NMSS, MMSE scores, as well as H-Y stage). Spearman’s correlation coefficients and corresponding p-value are presented in Figure 3. As indicated from the results, all subfields m-VD indicated a significant negative correlation with age. In addition, we observed that there was a negative correlation was identified between the m-VD of some subfields and disease duration, UPDRS total, UPDRS III, NMSS scores, and H-Y stage (p < 0.05). However, no statistically significant differences were noted for the correlation between the m-VD and sex and MMSE scores (both p > 0.05).
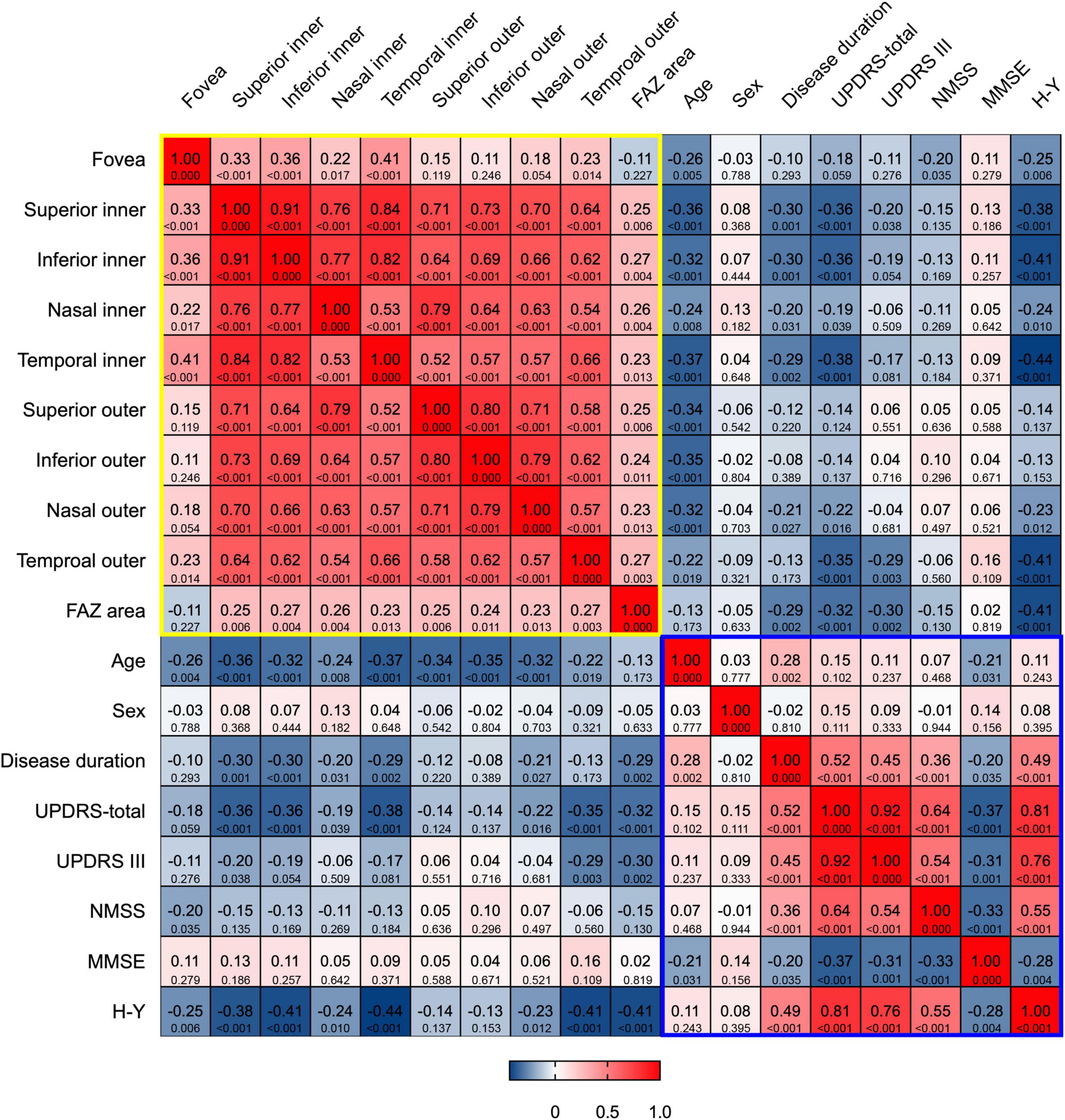
Figure 3. Heat map of correlation between m-VD and clinical parameters. There was a significant negative correlation between all subfields m-VD and age. In addition, there was a negative correlation between the m-VD of some subfields and disease duration, UPDRS-total, UPDRS III, NMSS scores, and H-Y stage. The top row of each cell is the Spearman correlation coefficients, and the bottom row is the corresponding p-value. The yellow and blue box represented the correlations among OCT parameters, and among clinical parameters, respectively.
Discussion
Retinal microvasculature variations in patients with PD have been rarely studied, and the relevant results are inconsistent. As reported by Shi et al. (2020), PD displayed a lower retinal capillary skeleton of SCP in all the quadrants as compared with HC in our study. However, Gulmez Sevim et al. (2019) found that the diameter of the retinal vessel showed no change in patients with PD. Our study is the initial study to explore the change of retinal vessel density in patients with PD divided into three stages based on the H-Y stage.
In this study, compared with the HC group, the m-VD in SCP in all subfields was suggested to significantly decrease in patients with PD. Moreover, as revealed from the in-depth analysis, the H-Y III stage group had lower m-VD in the fovea, SI, II, NI, TI, TO, and lower FAZ area, whereas no statistically significant difference was identified in p-VD for PD subgroups. The FAZ size significantly declined in patients with PD with H-Y I stage compared with HC. Thereby demonstrating that the abnormal change could be detected at the early stage of patients with PD. These suggested that the FAZ area may be a more sensitive indicator to detect PD at the early stage (H-Y I stage). Moreover, as suggested from the results here, mid-stage (H-Y II and H-Y III) patients with PD tended to show greater generalized microvascular variations. Furthermore, a significant negative correlation was identified between m-VD and both the age and H-Y stage score.
Vascular impairment may account for the pathogenesis of PD (Guan et al., 2013). As reported by earlier studies, several neurological disorders including PD are associated with ischemia or cerebrovascular lesions (Alavi et al., 1998; Kalaria, 2000). Since the retina is a “window” to the brain, they share an analogous pathophysiological mechanism. Cerebral vascular variations in PD might spread to the retina and lead to retinal angiopathy in the microvascular network. Guan et al. (2013) revealed the disruption of capillary network in patients with PD (e.g., shorter length, less number, and fewer branches). It was inferred that intracellular oxidative stress (Cuenca et al., 2014) might critically contribute to capillary variations. According to existing studies, decreased dopamine (Harnois and Di Paolo, 1990) levels and pathologic α-synuclein deposits (Zhang et al., 2015) were found in the wall of the blood vessels in the retina, especially in the ganglion cells located in SCP (Ortuño-Lizarán et al., 2018). As suggested from quantification studies of the inner retinal capillary in PD, microvascular variation in patients with PD displays an association with the neuronal loss in the inner retina (Miri et al., 2015; Kromer et al., 2016; Kwapong et al., 2018), which may comply with this study and explain why m-VD was found to decrease in SCP and retinal neurodegeneration.
The high oxygen consumption determines the vessel structure in the macular region (Yu et al., 2019), meanwhile, the energy metabolism in the retina is mainly on the neurons. In addition, the blood from the relatively high density and sophisticated capillary network around the foveola, supplying to the macula (Yu et al., 2019). It means neuron loss associated with the decrease of m-VD, and macula especially in the fovea VD might be a more sensitive region to the change in patients with PD.
The foveal avascular zone (FAZ) located in the fovea, surrounded by the continuous capillary network of the retina, had no capillary structure. Such regions contained DA neurons in the human retina. Berkowitz and Patel (2020) reported that patients with PD have smaller FAZ areas than healthy controls, complying with our findings. The mechanism underlying the FAZ area reduction is suggested to damage foveal DA neurons, which promotes vasculogenesis from the capillary surround (Miri et al., 2015). On the contrary, whether alterations of capillary morphology and pathology act in parallel on DA neurons and microglial cells surrounding the FAZ, was not confirmed. Same with most neurodegenerative diseases, vascular variation may contribute to the neuronal loss (Guan et al., 2013). This is consistent with our results that the deficiency of DA (Harnois and Di Paolo, 1990) and smaller FAZ size accompanied by the reduction in foveal vessel density indicated the damaged and incomplete parafoveal capillary arcade in the macula.
According to the results of this study, macular vascular variation might occur before the variation in p-VD. This might mainly be attributed to the fact that the macular SCP largely originates from the retinal circulation, complying with the results here. However, the peripapillary radial peripapillary capillary (RPC) receives perfusion, and the circulation from the choroidal ciliary vessels (Zhang et al., 2019). Moreover, the loss of the capillaries might not be detected by OCTA, as impacted by the overlap of the multilayered capillaries in RPC. Previous research has demonstrated, thinner nerve fibers concentrate on the temporal side of the optic nerve head (ONH), the thinner of the nerve fibers (Mikelberg et al., 1989), the shallower decline of the retinal nerve fiber layer (RNFL), this would be common to the vascular structure of blood circulation of these regions (Jo et al., 2019). This might be another reason. To date, this is the first study that analyzed peripapillary VD in patients with PD.
An inverse correlation between m-VD in the SCP and age, disease duration, UPDRS scores, NMSS scores, and H-Y stage was another novel finding in the report here. It is therefore predicted that the higher the age, UPDRS scores, NMSS scores, H-Y stage, and longer disease duration, the lower the density in the SCP. Existing studies reported no association between microvascular densities and H-Y scales (Kwapong et al., 2018; Shi et al., 2020). The possible explanation for this discrepancy with the measures here might result from our smaller study population, comprising the early and mid-stage of PD in this study. Meanwhile, age substantially has an impact on VD in the macula. The relationship between the m-VD and age reflected the vessel density decreased with age, as previous studies reported (Jo et al., 2019).
However, some limitations remained in our investigation. Due to poor patient cooperation and OCTA high inspection requirements for subjects, this study lacked advanced patients with PD, however, we can still observe a decreasing trend of m-VD in this study as the severity of PD increased. Meanwhile, this is consistent with the results of our correlation analysis that m-VD is inversely related to UPDRS scores. In addition, the use of anti-Parkinson’s disease medicine was not considered in patients with PD, and this assessment of disease severity would be biased. Moreover, this study was cross-sectional, and the m-VD changes could not be captured over time. Thus, further longitudinal, larger sample study (e.g., the advanced stage patients with PD were included) should be performed subsequently.
Conclusion
The results here suggested that abnormal variations could be detected with OCTA at the early stage of patients with PD. In addition, as the disease progresses, m-VD showed a broader decrease, which is associated with age and disease severity. Furthermore, m-VD and FAZ might be a more sensitive index than p-VD to detect the variations. As revealed from the mentioned results, OCTA is a potential biomarker to detect the changes in different clinical stages of PD by visualizing retinal structure in vivo directly. OCTA can act as a valuable tool for early diagnosis and progression for PD.
Data Availability Statement
The raw data supporting the conclusions of this article will be made available by the authors, without undue reservation.
Ethics Statement
The studies involving human participants were reviewed and approved by the Research Ethics Committee of Xiangya Hospital, Central South University. The patients/participants provided their written informed consent to participate in this study.
Author Contributions
BX and XW collected and analyzed the data and wrote the manuscript. BX, XW, JG, HX, and BT collected participants’ data and discussed the results. BX performed an OCT images scan of all participants. LS and BJ conceived the project, designed the research, supervised the data collection and analysis, and revised the manuscript. All authors contributed to the article and approved the submitted version.
Funding
This study was supported by the National Key R&D Program of China (Nos. 2020YFC2008500, 2017YFC0840100, 2017YFC0840104, 2018YFC1312003, and 2016YFC1306000), National Natural Science Foundation of China (Nos. 81671075, 81971029, 82071216, and 81901171), and Hunan Innovative Province Construction Project (No. 2019SK2335).
Conflict of Interest
The authors declare that the research was conducted in the absence of any commercial or financial relationships that could be construed as a potential conflict of interest.
Publisher’s Note
All claims expressed in this article are solely those of the authors and do not necessarily represent those of their affiliated organizations, or those of the publisher, the editors and the reviewers. Any product that may be evaluated in this article, or claim that may be made by its manufacturer, is not guaranteed or endorsed by the publisher.
Acknowledgments
We are grateful to all participants in the present study.
References
Alavi, A., Clark, C., and Fazekas, F. (1998). Cerebral ischemia and alzheimer’s disease: critical role of pet and implications for therapeutic intervention. J. Nucl. Med. 39, 1363–1365.
Archibald, N. K., Clarke, M. P., Mosimann, U. P., and Burn, D. J. (2011). Visual symptoms in parkinson’s disease and parkinson’s disease dementia. Mov. Disord. 26, 2387–2395. doi: 10.1002/mds.23891
Berkowitz, S., and Patel, S. (2020). Pilot study for neurological and retinal imaging as biomarkers for parkinson’s disease using optical coherence tomography-angiography. Invest. Ophth. Vis. Sci. 61:4831.
Bodis-Wollner, I., Kozlowski, P. B., Glazman, S., and Miri, S. (2014). A -synuclein in the inner retina in parkinson disease. Ann. Neurol. 75, 964–966. doi: 10.1002/ana.24182
Cuenca, N., Fernández-Sánchez, L., Campello, L., Maneu, V., De La Villa, P., Lax, P., et al. (2014). Cellular responses following retinal injuries and therapeutic approaches for neurodegenerative diseases. Prog. Retin. Eye Res. 43, 17–75. doi: 10.1016/j.preteyeres.2014.07.001
de Lau, L. M., and Breteler, M. M. (2006). Epidemiology of parkinson’s disease. Lancet Neurol. 5, 525–535. doi: 10.1016/s1474-4422(06)70471-9
de Rijk, M. C., Breteler, M. M., Graveland, G. A., Ott, A., Grobbee, D. E., Van Der Meché, F. G., et al. (1995). Prevalence of parkinson’s disease in the elderly: the rotterdam study. Neurology 45, 2143–2146. doi: 10.1212/wnl.45.12.2143
Goetz, C. G., Poewe, W., Rascol, O., Sampaio, C., Stebbins, G. T., Counsell, C., et al. (2004). Movement disorder society task force report on the hoehn and yahr staging scale: status and recommendations. Mov. Disord. 19, 1020–1028. doi: 10.1002/mds.20213
Goetz, C. G., Tilley, B. C., Shaftman, S. R., Stebbins, G. T., Fahn, S., Martinez-Martin, P., et al. (2008). Movement disorder society-sponsored revision of the unified parkinson’s disease rating scale (mds-updrs): scale presentation and clinimetric testing results. Mov. Disord. 23, 2129–2170. doi: 10.1002/mds.22340
Guan, J., Pavlovic, D., Dalkie, N., Waldvogel, H. J., O’carroll, S. J., Green, C. R., et al. (2013). Vascular degeneration in parkinson’s disease. Brain Pathol. 23, 154–164. doi: 10.1111/j.1750-3639.2012.00628.x
Gulmez Sevim, D., Unlu, M., Sonmez, S., Gultekin, M., Karaca, C., and Ozturk Oner, A. (2019). Retinal vessel diameter obtained by optical coherence tomography is spared in parkinson’s disease. Int. Ophthalmol. 39, 813–819. doi: 10.1007/s10792-018-0873-7
Harnois, C., and Di Paolo, T. (1990). Decreased dopamine in the retinas of patients with parkinson’s disease. Invest. Ophthalmol. Vis. Sci. 31, 2473–2475. doi: 10.1016/0042-6989(89)90165-X
Jo, Y. H., Sung, K. R., and Shin, J. W. (2019). Effects of age on peripapillary and macular vessel density determined using optical coherence tomography angiography in healthy eyes. Invest. Ophthalmol. Vis. Sci. 60, 3492–3498. doi: 10.1167/iovs.19-26848
Kalaria, R. N. (2000). The role of cerebral ischemia in alzheimer’s disease. Neurobiol. Aging 21, 321–330. doi: 10.1016/s0197-4580(00)00125-1
Kromer, R., Buhmann, C., Hidding, U., Keserü, M., Keserü, D., Hassenstein, A., et al. (2016). Evaluation of retinal vessel morphology in patients with parkinson’s disease using optical coherence tomography. PLoS One 11:e0161136. doi: 10.1371/journal.pone.0161136
Kwapong, W. R., Ye, H., Peng, C., Zhuang, X., Wang, J., Shen, M., et al. (2018). Retinal microvascular impairment in the early stages of parkinson’s disease. Invest. Ophthalmol. Vis. Sci. 59, 4115–4122. doi: 10.1167/iovs.17-23230
MacCormick, I. J., Czanner, G., and Faragher, B. (2015). Developing retinal biomarkers of neurological disease: an analytical perspective. Biomark Med. 9, 691–701. doi: 10.2217/bmm.15.17
Mikelberg, F. S., Drance, S. M., Schulzer, M., Yidegiligne, H. M., and Weis, M. M. (1989). The normal human optic nerve. Axon count and axon diameter distribution. Ophthalmology 96, 1325–1328. doi: 10.1016/s0161-6420(89)32718-7
Miri, S., Shrier, E. M., Glazman, S., Ding, Y., Selesnick, I., Kozlowski, P. B., et al. (2015). The avascular zone and neuronal remodeling of the fovea in parkinson disease. Ann. Clin. Transl. Neurol. 2, 196–201. doi: 10.1002/acn3.146
Mohana Devi, S., Mahalaxmi, I., Aswathy, N. P., Dhivya, V., and Balachandar, V. (2020). Does retina play a role in parkinson’s disease? Acta Neurol. Belg. 120, 257–265. doi: 10.1007/s13760-020-01274-w
Ortuño-Lizarán, I., Beach, T. G., Serrano, G. E., Walker, D. G., Adler, C. H., and Cuenca, N. (2018). Phosphorylated α-synuclein in the retina is a biomarker of parkinson’s disease pathology severity. Mov. Disord. 33, 1315–1324. doi: 10.1002/mds.27392
Postuma, R. B., Berg, D., Stern, M., Poewe, W., Olanow, C. W., Oertel, W., et al. (2015). Mds clinical diagnostic criteria for parkinson’s disease. Mov. Disord. 30, 1591–1601. doi: 10.1002/mds.26424
Sengupta, P., Dutta, K., Ghosh, S., Mukherjee, A., Pal, S., and Basu, D. (2018). Optical coherence tomography findings in patients of parkinson’s disease: an indian perspective. Ann. Indian Acad. Neurol. 21, 150–155. doi: 10.4103/aian.AIAN_152_18
Shi, C., Chen, Y., Kwapong, W. R., Tong, Q., Wu, S., Zhou, Y., et al. (2020). Characterization by fractal dimension analysis of the retinal capillary network in parkinson disease. Retina 40, 1483–1491. doi: 10.1097/iae.0000000000002641
Uchida, A., Pillai, J. A., Bermel, R., Bonner-Jackson, A., Rae-Grant, A., Fernandez, H., et al. (2018). Outer retinal assessment using spectral-domain optical coherence tomography in patients with alzheimer’s and parkinson’s disease. Invest. Ophthalmol. Vis. Sci. 59, 2768–2777. doi: 10.1167/iovs.17-23240
Yıldız, D., Pekel, N. B., Yener, N. P., Seferoğlu, M., Günes, A., and Sığırlı, D. (2019). Assessment of neurodegeneration by optical coherence tomography and mini-mental test in parkinson’s disease. Ann. Indian Acad. Neurol. 22, 212–216. doi: 10.4103/aian.AIAN_424_17
Yu, D. Y., Cringle, S. J., Yu, P. K., Balaratnasingam, C., Mehnert, A., Sarunic, M. V., et al. (2019). Retinal capillary perfusion: spatial and temporal heterogeneity. Prog. Retin Eye Res. 70, 23–54. doi: 10.1016/j.preteyeres.2019.01.001
Zhang, X., Iverson, S. M., Tan, O., and Huang, D. (2015). Effect of signal intensity on measurement of ganglion cell complex and retinal nerve fiber layer scans in fourier-domain optical coherence tomography. Transl. Vis. Sci. Technol. 4:7. doi: 10.1167/tvst.4.5.7
Zhang, Y. S., Zhou, N., Knoll, B. M., Samra, S., Ward, M. R., Weintraub, S., et al. (2019). Parafoveal vessel loss and correlation between peripapillary vessel density and cognitive performance in amnestic mild cognitive impairment and early alzheimer’s disease on optical coherence tomography angiography. PLoS One 14:e0214685. doi: 10.1371/journal.pone.0214685
Keywords: diagnosis, Hoehn-Yahr stage, optical coherence tomography angiography (OCTA), Parkinson’s disease, vessel density
Citation: Xu B, Wang X, Guo J, Xu H, Tang B, Jiao B and Shen L (2022) Retinal Microvascular Density Was Associated With the Clinical Progression of Parkinson’s Disease. Front. Aging Neurosci. 14:818597. doi: 10.3389/fnagi.2022.818597
Received: 19 November 2021; Accepted: 21 January 2022;
Published: 17 February 2022.
Edited by:
Jee-Young Lee, Seoul Metropolitan Government – Seoul National University Boramae Medical Center, South KoreaReviewed by:
Jeeyun Ahn, Seoul Metropolitan Government – Seoul National University Boramae Medical Center, South KoreaSteven Gunzler, University Hospitals Cleveland Medical Center, United States
Copyright © 2022 Xu, Wang, Guo, Xu, Tang, Jiao and Shen. This is an open-access article distributed under the terms of the Creative Commons Attribution License (CC BY). The use, distribution or reproduction in other forums is permitted, provided the original author(s) and the copyright owner(s) are credited and that the original publication in this journal is cited, in accordance with accepted academic practice. No use, distribution or reproduction is permitted which does not comply with these terms.
*Correspondence: Lu Shen, c2hlbmx1QGNzdS5lZHUuY24=; Bin Jiao, amJpbjA5MTFAMTYzLmNvbQ==
†These authors have contributed equally to this work