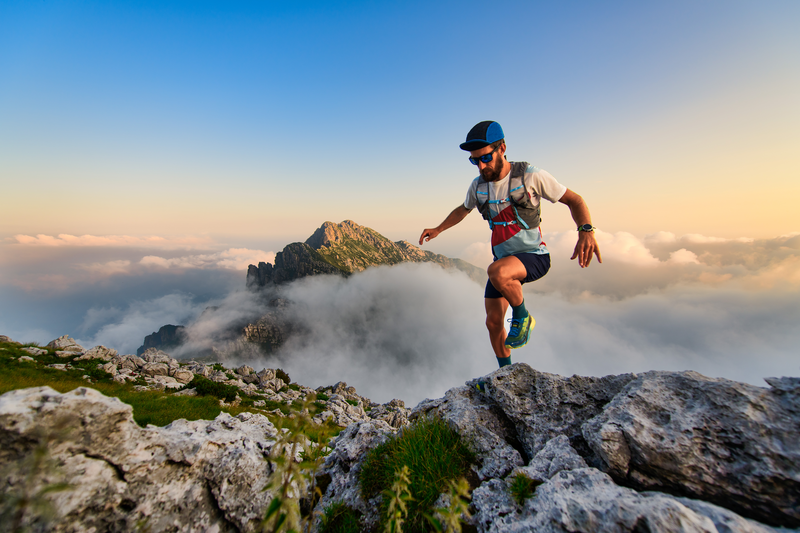
94% of researchers rate our articles as excellent or good
Learn more about the work of our research integrity team to safeguard the quality of each article we publish.
Find out more
REVIEW article
Front. Aging Neurosci. , 29 March 2022
Sec. Parkinson’s Disease and Aging-related Movement Disorders
Volume 14 - 2022 | https://doi.org/10.3389/fnagi.2022.807909
This article is part of the Research Topic Translational Advances in Alzheimer's, Parkinson's, and other Dementia: Molecular Mechanisms, Biomarkers, Diagnosis, and Therapies, Volume II View all 58 articles
Background: Pharmacotherapy is the first-line treatment option for Parkinson’s disease, and levodopa is considered the most effective drug for managing motor symptoms. However, side effects such as motor fluctuation and dyskinesia have been associated with levodopa treatment. For these conditions, alternative therapies, including invasive and non-invasive medical devices, may be helpful. This review sheds light on current progress in the development of devices to alleviate motor symptoms in Parkinson’s disease.
Methods: We first conducted a narrative literature review to obtain an overview of current invasive and non-invasive medical devices and thereafter performed a systematic review of recent randomized controlled trials (RCTs) of these devices.
Results: Our review revealed different characteristics of each device and their effectiveness for motor symptoms. Although invasive medical devices are usually highly effective, surgical procedures can be burdensome for patients and have serious side effects. In contrast, non-pharmacological/non-surgical devices have fewer complications. RCTs of non-invasive devices, especially non-invasive brain stimulation and mechanical peripheral stimulation devices, have proven effectiveness on motor symptoms. Nearly no non-invasive devices have yet received Food and Drug Administration certification or a CE mark.
Conclusion: Invasive and non-invasive medical devices have unique characteristics, and several RCTs have been conducted for each device. Invasive devices are more effective, while non-invasive devices are less effective and have lower hurdles and risks. It is important to understand the characteristics of each device and capitalize on these.
Parkinson’s disease (PD) is a neurodegenerative disorder caused by the progressive loss of dopaminergic neurons in the midbrain (Lang and Lozano, 1998; Kalia and Lang, 2015). Its cardinal motor symptoms include tremor, rigidity, bradykinesia/akinesia, and postural instability (Dubois and Pillon, 1997). Pharmacotherapy is the mainstay treatment for patients with PD, and levodopa is the most effective drug for managing motor symptoms (Poewe and Mahlknecht, 2020). However, side effects such as dyskinesia and the on/off phenomenon have been associated with levodopa treatment (Holloway et al., 2004). Moreover, its effectiveness has decreased over the years (Obeso et al., 2017).
Invasive surgical alternatives to pharmacological treatments include stereotactic thalamotomy, deep brain stimulation (DBS), spinal cord stimulation (SCS), and continuous infusion of levodopa-carbidopa or apomorphine therapy. DBS is a widely used medical device therapy and surgical standard for patients with PD (Kleiner-Fisman et al., 2006). The use of SCS in patients with PD is promising for relieving concurrent pain conditions. The delivery of Duodopa® intestinal gel directly into the small intestine reduces fluctuating motor symptoms and improves the quality of life (Olanow et al., 2014). However, the risk associated with the implantation of these invasive medical devices is a burden for patients with PD. For example, DBS may cause irreversible intracranial hemorrhages (Kleiner-Fisman et al., 2006).
Recently, alternative non-pharmacological/non-surgical approaches have been explored to alleviate PD symptoms. Non-invasive medical devices are mainly effective for improving tremors, freezing of gait (FOG), and gait. Emerging non-invasive medical devices primarily include management devices for tremors, non-invasive brain stimulation (NIBS), cueing devices for FOG, non-invasive vagus nerve stimulation (nVNS), vibrotactile stimulation devices, mechanical/electrical peripheral stimulation, and photobiomodulation devices. Each device adapts to different body parts (Figure 1) and has different properties and effects. In this study, we review emerging therapeutic devices for motor symptoms in PD.
Figure 1. Body parts the medical devices are applied to. The stimulation targets of each device are shown in this figure.
A narrative literature review and a systematic review of randomized control trials (RCTs) were conducted. These reviews summarized recent invasive and non-invasive medical devices and their effects on PD patients in the past 10 years. The search method is shown in Figure 2. All searches were performed on PubMed and Scopus. The study screening was done independently by two reviewers, JF and RM First, we searched for MeSH term “Parkinson’s disease.” English language literature in the past 10 years was reviewed. Next, we added the device name to the search terms and performed a search for each device, as shown in Figure 2. Articles found in this search were screened based on title and abstract, then based on the full text. In the narrative literature review, we selected literature related to motor symptoms. For the systematic review, RCTs were extracted out from the search results. We manually reviewed these papers and selected the ones that fit. The RCT studies selected from the search are shown in Table 1.
Figure 2. Flow diagram of the study selection process in the systematic review. This diagram shows how to search for studies in a systematic review. The numbers in parentheses show the breakdown of the number of search hits, with PubMed and Scopus listed in that order.
DBS is the surgical standard for patients with movement disorders such as PD, essential tremor (ET), and dystonia. DBS is a highly effective and widely used treatment for PD patients and is probably the most important advance in PD treatment since the introduction of levodopa (Kleiner-Fisman et al., 2006; Benabid et al., 2009; Bronstein et al., 2011). The target of stimulation is either the subthalamic nucleus (STN) or internal globus pallidus (GPi) (Sako et al., 2014). The ventral intermediate nucleus of the thalamus (VIM) is also used to improve tremors (Morigaki et al., 2011a,b). Recently, advanced imaging sequences have enabled direct visualization of anatomical targets, and patient-specific DBS has been performed (Neudorfer et al., 2022). DBS has been demonstrated to improve motor function including FOG (Krack et al., 2003; Huang et al., 2018), camptocormia (Sako et al., 2009; Chan et al., 2018), tremor (Morigaki et al., 2011a,b), and cognitive dysfunction. Although DBS has a lower complication rate than stereotactic thalamotomy (Tasker, 1998; Pahwa et al., 2001), intracranial hemorrhage and infection still occur in 3.9 and 1.6% of the patients, respectively (Kleiner-Fisman et al., 2006). There is also a risk of secondary psychiatric effects (Piasecki and Jefferson, 2004; Kinoshita et al., 2018). Long-term effects have been shown to last for more than 10 years for tremors. In many cases, activity of daily living can be maintained for a long time, and patient satisfaction remains high even after 10 years of follow-up (Hitti et al., 2019). However, since DBS is not effective for all patients, it is necessary to determine if the patient is an appropriate candidate. It is also important to confirm the diagnosis of idiopathic PD. A thorough neurological examination is necessary because some neurological disorders can mimic the signs and symptoms of idiopathic PD (Machado et al., 2006). Also, DBS effects for gait impairment, postural instability, postural abnormalities, FOG, and other axial motor signs are under debate (Fasano et al., 2015). Expert opinion showed that the risk/benefit ratio in the elderly for STN DBS is not very favorable; unlike STN DBS, there is no clinical indication that the outcome of VIM DBS is in any way affected by age. It must be determined on an individual basis, considering the need for treatment, risk factors for complications, and general life expectancy (Lang et al., 2006). The precise underlying mechanisms of DBS remain a matter of debate. A leading hypothesis is that DBS restores aberrant neuronal firing to non-pathological rates by applying high-frequency trains of electrical stimulation (Muller and Robinson, 2018). DBS devices are also increasing in functionality as research advances, such as a variety of stimulation methods [interleaved stimulation (Wojtecki et al., 2011; Baumann et al., 2012; Aquino et al., 2019), dual-target
stimulation (Hollingworth et al., 2017), directional stimulation (Schupbach et al., 2017), current steering (Barbe et al., 2014)], and support for magnetic resonance imaging (Larson et al., 2008; McElcheran et al., 2019). Studies have also been conducted to investigate the corresponding effects on stimulus frequency (Karl et al., 2020). Stimulation parameters may change the effects and long-term consequences. In this section, we introduce two emerging technologies in DBS: adaptive DBS (aDBS) and remote programming for DBS adjustment, both of which have seen significant growth and attention in recent years.
DBS devices that operate on the principle of closed-loop interaction are called aDBS. A closed-loop system can sense the effect of stimulation and adjust the stimulation in response to the observed effect. Recently, a closed-loop stimulation technology based on local field potentials (LFPs) in target structures as biomarkers has been used for the treatment of PD subjects (Marceglia et al., 2009; Giannicola et al., 2010; Little et al., 2013; Sasaki et al., 2021). Compared to the cortical neural signals such as electroencephalography (EEG), electrocorticography, and magnetoencephalography, LFP signals from deep electrodes provide direct changes in basal ganglia function (Einevoll et al., 2013). In PD, increased synchrony of neuronal networks in the beta band (13–35 Hz) is associated with reduced segregation of parallel processes, leading to reduced specificity of motor programs (Mink, 1996; Pessiglione et al., 2005). The amplitude of spectral peaks in the beta band correlates with the severity of symptoms (Neumann et al., 2016, 2017) and is reduced by dopaminergic medication and DBS (Kuhn et al., 2009). Beta-power is an ideal candidate for aDBS as a biomarker (Marceglia et al., 2009; Giannicola et al., 2010; Little et al., 2013) and aDBS using LFPs has been confirmed to have advantages over conventional DBS (cDBS). aDBS reportedly prevents several complications related to cDBS, including gait and speech disturbances, and was appropriately modulated by levodopa administration, with a further reduction in stimulation, potentially preventing excessive combined therapy and dyskinesias (Little et al., 2016a,b). Bocci et al. (2021) reported that the Unified PD Rating Scale (UPDRS) part III scores, the Rush scale for dyskinesias, and the total electrical energy delivered to the tissues per second (TEEDs) were significantly lower in the aDBS session during an 8-h stimulation protocol, in conjunction with chronic levodopa assumption and without restriction on patients’ activities. The safety and effectiveness of aDBS stimulation compared to cDBS in a daily session in terms of motor performance and TEEDs in patients with PD are shown. Little et al. (2013) compared the effectiveness of aDBS with that of cDBS. aDBS showed 66% (unblinded) and 50% (blinded) improvements in motor scores, which were 29% (unblind) and 27% (blinded) significantly better than cDBS. These improvements were achieved with a 56% reduction in stimulation time and a significant reduction in energy requirements compared to cDBS.
In the future, a closed-loop system that uses neurotransmitters such as dopamine, histamine, adenosine, serotonin, and glutamate release as a control variable has been proposed (Griessenauer et al., 2010; Shon et al., 2010; Chang et al., 2012a,b; Chang et al., 2013). A 30-minute baseline of dopamine during a DBS surgery was quantified using microdialysis (Kilpatrick et al., 2010). Kishida et al. (2011, 2016) performed the voltammetric measurement of real-time dopamine dynamics in patients with PD using fast scan cyclovoltammetry (FSCV). One study targeted another neurotransmitter, adenosine. Real-time in vivo FSCV neurochemical recordings in patients with tremors showed that DBS induces adenosine release concurrent with tremor arrest (Chang et al., 2012b). It has become clear that although clinical monitoring of neurotransmitters is technically feasible, and these sensing technologies need to be improved to measure dopamine over long periods (days, months, years). This will require the development of technologies that can solve current problems such as biofouling and material degradation to enable reliable long-term recording. Furthermore, the improvement of current biosensors or the development of new biosensors to accommodate chronic implantation and the method for information processing and decision making are also essential.
Remote DBS programming has been implemented in clinical practice (Li et al., 2017; Zhang et al., 2020; Ma et al., 2021; Xu et al., 2021). Unfortunately, many patients do not achieve the expected DBS outcome due to inadequate or suboptimal programming (Farris and Giroux, 2013). Optimization of DBS parameters is usually attained within three to 6 months, during four to five programming sessions (Bronstein et al., 2011). Additionally, the parameters need to be adjusted over the long term to maintain this effect. The physician responsible for postoperative programming should be an expert in both DBS and medical management of PD patients, but patients living in remote areas may have inadequate access and thus may not receive adequate adjustments. Thus, teleprogramming has received increasing attention in recent years, partly because of the coronavirus disease 2019 pandemic. Ma et al. (2021) followed 90 patients for a total of 386 remote programming visits over 27 months. Their questionnaire survey demonstrated that each remote programming visit saved ≥ 2000 Chinese yuan for 76.7% of the patients and ≥ 12 h for 90.0% of the patients, compared with the on-site programming visit. Respondents also rated the acceptability of the remote programming platform highly. Moreover, 89% of the patients were satisfied with the remote programming. Significant improvement in UPDRS Part III was also achieved in another study of 32 patients conducted by Xu et al. (2021). LeMoyne et al. (2019a, b, 2020a, 2020b, 2020c) quantified the tremor of PD patients via a wireless inertial sensor system with connectivity to Cloud computing resources attached to the dorsal side of the hand and successfully classified the stimulus amplitude of DBS. Machine learning was used for classification, with neural networks, J48 decision trees, K-nearest neighbor, support vector machine, logistic regression, and random forest, achieving 100% accuracy in certain conditions. An online system for handling such objective and quantitative data is essential for remote DBS coordination.
The use of SCS in patients with PD is promising for relieving concurrent pain conditions (Fenelon et al., 2012; Cai et al., 2020). Notably, SCS has recently also been shown to be effective for locomotive symptoms in patients with PD (Hassan et al., 2013); therefore, SCS might be a viable alternative therapy to DBS for the management of PD symptoms (Pinto de Souza et al., 2017). Santana et al. (2014) showed that SCS at the upper thoracic level in a primate model of PD improved freezing, hypokinesia, postural instability, and bradykinesia, which is associated with a reduction in beta-frequency oscillation within the cortico-basal ganglia circuitry.
In human case reports, high cervical SCS (C2-C3) yielded incongruent results (Thevathasan et al., 2010; Hassan et al., 2013). Cervical SCS with tonic waveform requires a long latency (more than 3 months) for motor improvement (Mazzone et al., 2019). Contrastingly, the improvement by cervical SCS with burst waveform stimulation showed acute and larger improvements in motor symptoms, including tremor, gait parameters, and pain (Mazzone et al., 2019). Similarly, thoracic SCS (T6-T12) reduced gait impairment, rigidity, abnormal posture, and tremor (Agari and Date, 2012; Fenelon et al., 2012; Landi et al., 2013; Nishioka and Nakajima, 2015; Samotus et al., 2018; Hubsch et al., 2019). Thoracic SCS with burst waveform stimulation also improves pain, gait, and stooping posture (Kobayashi et al., 2018; Furusawa et al., 2020). Some authors have advocated SCS as a possible salvage therapy for DBS with decreased efficacy over the years (Agari and Date, 2012; Landi et al., 2013; Akiyama et al., 2017; Pinto de Souza et al., 2017). However, a 1-year single prospective open-label pilot study revealed no beneficial effect of thoracic SCS in six pain-free advanced PD patients with significant axial symptoms (Prasad et al., 2020). The possibility of quick habituation for tonic stimulation is advocated for the gradual loss of clinical benefit in axial symptoms over time and the ongoing pilot trial of cyclic stimulation (Cury et al., 2020).
The major complications of SCS include infection, lead migration or breakage, subcutaneous hematoma, and discomfort due to the pulse generator (Nissen et al., 2019). The infection rate is 3–6% (Nissen et al., 2019); however, the majority of SCS studies in patients with PD were either uncontrolled or controlled trials with small sample sizes. Thus, well-designed clinical trials, including double-blind and placebo-controlled arms with large sample sizes and specific stimulation protocols are warranted to generate solid evidence regarding the effectiveness of SCS (Fonoff et al., 2019; Rahimpour et al., 2021).
PD patients are mainly treated with oral administration of levodopa and dopamine agonists during the early stage (Fahn and Sulzer, 2004). However, the duration of the response to treatment becomes shorter, and side effects—such as hallucinations—are marked as the progression of the disease stage. Complications such as fluctuations, including the wearing-off phenomenon and dyskinesia, can be seen in patients who have been receiving long-term oral levodopa (Eggert et al., 2008). This fluctuation of symptoms might be caused by the short half-life of levodopa (Nyholm et al., 2005). Continuous drug delivery, allowing for continuous dopaminergic stimulation, is necessary for patients with advanced-stage PD (van Laar, 2003; Nyholm et al., 2005; Gershanik and Jenner, 2012). Dopaminergic stimulation can be prolonged by two methods: the simultaneous administration of enzyme inhibitors to prevent the metabolism of levodopa and continuous administration of dopaminergic agents, not orally. Continuous levodopa administration was reported to be effective for the reduction of dyskinesia and off-time by stabilizing plasma levodopa/carbidopa levels and providing continuous stimulation of dopaminergic receptors in the striatum (Sage et al., 1988; Politis et al., 2017).
The methods currently available for continuous infusion are subcutaneous apomorphine, levodopa/carbidopa liquid, and enteral levodopa/carbidopa gel (Duodopa®, Abbott, Allschwil, Switzerland). Continuous subcutaneous apomorphine infusion is effective on motor function and alleviates motor fluctuations in patients with PD and, it can also significantly reduce off-time (Garcia Ruiz et al., 2008). Various types of apomorphine infusion approaches such as inhale, patch pump, and sublingual administrations have been developed (Titova and Chaudhuri, 2016). In recent years, the efficacy of these approaches on motor function has been assessed (Olanow et al., 2020) and sublingual apomorphine has received Food and Drug Administration (FDA) approval (Larson and Simuni, 2022). Duodopa® can be delivered to the jejunum via a percutaneous gastrojejunostomy tube, which is connected to a portable infusion pump filled with Duodopa®. Duodopa® intestinal gel can shorten off-time while extending on-time without dyskinesia (Olanow et al., 2014). Furthermore, Slevin et al. (2015) and Merola et al. (2016) investigated on-time with/without troublesome dyskinesia with Duodopa® treatment. Although there was no significant difference in on-time with troublesome dyskinesia between the control and Duodopa® groups, significant differences were observed in on-time without troublesome dyskinesia and off-time, suggesting that Duodopa® had a positive effect. However, the pooled results showed that Duodopa® intestinal infusion did not significantly improve the UPDRS total score, including parts II and III (Wang et al., 2018). Contrastingly, a cost-utility analysis was performed in the United Kingdom and showed that Duodopa® intestinal infusion increased quality-adjusted life years while also being cost-effective. Thus, it seems that Duodopa® is effective in reducing fluctuating motor symptoms and improving quality of life, and so might be a useful tool to treat patients with advanced-stage PD. The most frequent adverse events associated with Duodopa® were surgery-associated adverse events, such as infection and inflammation, which may be associated with morbidity and mortality in some patients. Tube obstruction, dislocation of the catheter tip, pump failure, and pull-out of the tube were reported to be related to the infusion system. Furthermore, drug-induced events, such as hallucinations, dystonia, worsened dyskinesia, acute peripheral neuropathy, psychosis, weight loss, and homocysteine concentrations have also been reported (Nyholm, 2012).
In recent years, another method for continuous levodopa administration is the continuous subcutaneous infusion of levodopa using ND0612 (NeuroDerm, Rehovot, Israel) (Olanow et al., 2021). ND0612 is a drug/device combination consisting of a subcutaneous pump and a liquid formulation. This formulation contains excipients to allow for low infusion rates. ND0612 administrates a liquid levodopa/carbidopa continuously, that stabilizes plasma levodopa levels compared to standard oral levodopa (Giladi et al., 2021). Olanow et al. (2021) evaluated the potential benefits of a 14-h (waking day) infusion compared to a 24-h infusion. The off-time for the overall population was significantly reduced by 2.0 h compared to baseline. On-time with no troublesome dyskinesia was significantly increased from baseline by 3.3 h, and on-time with moderate/severe dyskinesia was also significantly reduced by 1.2 h. The reduction in off-time was 1.5 h larger in the 24-h group than in the 14-h group. Notably, complete resolution of off-time was observed in 42% of patients in the 24-h group. Therefore, subcutaneous administration might both avoid fluctuations in absorption resulting from delayed gastric emptying and reduce surgical risks compared to Duodopa® (Nilsson et al., 1998; Nyholm and Lennernas, 2008). Poewe et al. (2021) evaluated the 1-year safety data of ND0612. After evaluation by 214 patients, most patients experienced infusion site reactions such as particularly nodules (30.8%) and hematomas (25.2%). However, most cases were mild to moderate, and only 10.3% of the patient discontinued treatment. This indicated that ND0612 is generally safe.
Continuous subcutaneous infusion of apomorphine has been developed by Mitsubishi Tanabe/Neuroderm as ND0701. ND0701 is a novel concentrated apomorphine formulation for apomorphine-based continuous subcutaneous infusion. A study by Ramot et al. (2018) and Larson and Simuni (2022) showed that it was safe and well-tolerated and had similar bioavailability to commercially available apomorphine formulations.
Tremor is the most common movement disorder and is defined as a rhythmic, involuntary oscillating movement (Bhatia et al., 2018). ET is the most common tremor worldwide. Although ET affects any part of the body, this tremor occurs most often in the hands, especially at the time of action—such as drinking and writing. Contrastingly, approximately 70% of PD patients also experience a tremor during the course of illness (Martin et al., 1973; Hughes et al., 1993; Pal et al., 2002), although this resting tremor tends to be a pill-rolling tremor, which looks like holding a pill between the thumb and forefinger and rolling it around. For patients with PD, tremor is one of the most disabling symptoms, in addition to slowed movement, rigid muscles, and postural balance impairment. Although resting tremor is a well-known symptom of PD, postural tremor associated with PD can cause more disability than typical resting tremors (Lance et al., 1963; Koller et al., 1989). Furthermore, tremor can be associated with a certain degree of shame in social situations among PD patients. Thus, the management of tremors may be important for patients with PD to improve their activities of daily living.
Pharmacological treatments can suppress tremors; for instance, levodopa and propranolol are used to alleviate resting tremors; primidone and propranolol for hand postural tremors; and beta-blockers, anticholinergic drugs, and primidone for kinetic tremor (Zesiewicz et al., 2011; Shanker, 2019; Poewe and Mahlknecht, 2020). However, although medication may be useful for suppressing tremors, it may incur mental or physical side effects such as addiction, hypotension, decrement of heart rate, and a feeling of thirst. Approximately 30% of patients with tremors do not respond to pharmacological treatment or experience intolerable secondary effects (Koller and Vetere-Overfield, 1989). Moreover, up to 56% of patients eventually discontinue their medication because of these secondary effects or a lack of efficacy (Diaz and Louis, 2010). For patients with tremors unresponsive to medication, stereotactic surgery—such as DBS, gamma knife radiosurgery, and focused ultrasound—can be a viable alternative. However, considering the severe side effects and complications caused by both medication and surgical intervention, non-surgical device treatments for reducing tremors may represent a good solution.
Active devices transfer external force or energy from devices into nerves and/or muscles to reduce tremors (Table 2). These devices also measure tremor characteristics using a gyroscope, accelerometer, and electromyogram. Peripheral nerve stimulation has been reported to be useful in reducing tremors—especially in patients with ET (Kim et al., 2020; Yu et al., 2020; Reis et al., 2021)—and is thought to evoke central activity via VIM, a thalamic target widely accepted to improve tremor with DBS (Morigaki et al., 2011a,b; Morigaki and Goto, 2015). Lin et al. (2018) reported the effect of median and radial nerve stimulation using a wrist band in patients with PD. They compared the tremor research group’s ET rating assessment scale and spiral drawing tasks pre- and post-stimulation and the scores decreased significantly after stimulation.
The Cala One device (Cala Health, CA, United States) was the first wearable electrical nerve stimulator approved by the FDA (Beringhause et al., 1989). The clinical trial using the new version of the device, Cala TRIO™ (Cala Health, CA, United States), was completed in 2019 (Hendriks et al., 1991). An accelerometer built into this device can assess the frequency of tremors, which enables the individualized calibration of the stimulation intensity. The Cala TRIO™ includes two working electrodes positioned over the median and radial nerves deliver electrical signals that intermittently excite these nerves, while the VIM is stimulated through peripheral sensory nerves of the median and radial nerves, similar to DBS (Bathien et al., 1980; Hanajima et al., 2004a,b). A study with five patients with tremors due to either ET or PD demonstrated its efficacy for tremor suppression (Dosen et al., 2015). Jitkritsadakul et al. (2017) reported the tremor glove to be a medical device that incorporates a tremor detection module and electrical muscle stimulation (EMS) to suppress resting hand tremors. This device included an adjustable glove with embedded inertial sensors and an EMS module, a control box that can be attached to the belt, and a smartphone with the device’s application installed. The glove was worn on the most affected hand. Results showed that the tremor glove effectively suppressed intractable resting hand tremor among patients with PD with no serious adverse events.
The MOTIMOVE system (3F-Fit Fabricando Faber, Serbia) has multiple stimulators that can selectively activate antagonistic muscles in the forearms. This system delivers an out-of-phase stimulation. A pilot study of MOTIMOVE showed 67% tremor suppression in patients with ET and PD (Popovic Maneski et al., 2011). The TREMOR neurorobot is similar to the MOTIMOVE system and comprises electrodes to provide stimulation, sensors to evaluate biomechanical signals of tremor, and a controller. The device uses co-contraction methods that apply continuous stimulation to antagonistic muscles to increase limb stiffness and reportedly showed a 52% tremor suppression (Gallego et al., 2013).
Orthostatic tremor is characterized by unsteadiness in standing and improvement when sitting or walking (Lee et al., 2011), and has been reported in elderly patients with PD. Primary orthostatic tremor (POT) is a rare disorder characterized by 13–18 Hz tremors in the legs when standing and is often refractory to medical treatment. Lamy et al. (2021) investigated the potential beneficial effects of trans-spinal direct current stimulation (tsDCS) in POT. Their results showed that cathodal-tsDCS reduced both tremor amplitude and frequency and lowered corticospinal excitability, whereas anodal-tsDCS reduced tremor frequency only. A single session of tsDCS significantly improved POT-induced instability; thus, Lamy et al. (2021) hypothesized that tsDCS may induce spinal and supraspinal effects via ascending pathways.
Contrastingly, passive devices absorb vibration energy by damping (Table 2). A passive device using a viscous beam and a hand orthosis using air dashpots have been reported (Kotovsky and Rosen, 1998; Takanokura et al., 2011). Buki et al. (2018) reported on a passive absorber, dubbed the vib-bracelet, a tremor attenuation device that was designed to operate as a dynamic vibration absorber. The vib-bracelet does not include any motors or sensors and is relatively light and compact. Meanwhile, the Liftware Steady™ (Liftware, United States) has an electronic stabilizing handle and a variety of attachments such as a spoon, fork, and spork, allowing patients with tremors to eat more easily. The handle contains sensors to detect motion and an onboard computer to differentiate between involuntary and voluntary movements. Sabari et al. (2019) compared the Liftware Steady™, standard spoon, weighted spoon (with standard handle and built-up handle), and swivel spoon. Reportedly, participants tended to select either a weighted spoon with a standard handle or the Liftware Steady™. A positive change in the Fahn-Tolosa-Marin Tremor Rating Scale, indicating improvement in tremors, has been shown in a pilot study (Sabari et al., 2019).
Patients with PD manifest gait disturbances and falls, which cause a significant reduction in their quality of life. The gait of PD patients is characterized by decreased step length, angular displacement, the velocity of the lower and upper limbs, high variability in step timing, poor bilateral coordination, and asymmetrical leg function (Lewis et al., 2003). Patients with PD also have a common and paroxysmal symptom termed FOG. FOG is defined as “an episodic inability (lasting seconds) to generate effective stepping in the absence of any known cause other than parkinsonism or high-level gait disorders” (Giladi and Nieuwboer, 2008). Generally, the initiation of walking requires coordination between locomotion, postural stability, and sensory-motor integration (Hass et al., 2005; Mille et al., 2012). Patients with PD have impaired kinesthesia due to degeneration of this system, resulting in inadequate motor planning to initiate movement, which causes FOG (Konczak et al., 2009). FOG is considered to be a motor manifestation of PD and appears in a significant number of patients as the disease progresses (Giladi and Nieuwboer, 2008), affecting up to 81% of patients after 20 years of disease progression (Hely et al., 2008). It is also one of the main causes of difficulty in walking and deteriorating quality of life (Moore et al., 2007; Perez-Lloret et al., 2014; Walton et al., 2015). Additionally, FOG can increase the risk of falls and cause fractures (Riancho-Zarrabeitia and Delgado-Alvarado, 2017), making freeze control an important issue. The mechanisms underlying FOG are still highly debated. However, impaired sensory processing primarily arising from the proprioceptive system is speculated to be important (Tan et al., 2011). Unfortunately, current pharmacological or surgical treatment has limited efficacy for FOG. Bilateral DBS of the STN may improve FOG during the off-period (Krack et al., 2003) but may be insufficient for long-term benefits (Kim et al., 2019) and FOG in the on-period (Davis et al., 2006). Therefore, alternative non-pharmacological/non-surgical approaches are being explored in an attempt to improve FOG. This section hereafter describes the specific methods for improving gait and FOG.
Among several NIBS techniques, repetitive transcranial magnetic stimulation (rTMS) is particularly effective in modulating corticospinal excitability (Pascual-Leone et al., 1994; Zanjani et al., 2015). rTMS affects cortical networks both during and 1 h after stimulation (Hallett et al., 1999). rTMS can both modify the excitability of local interneurons (local effects) and induce changes in the excitability of spatially distant, but functionally interconnected, cortical areas (network effects) (Hallett et al., 2000).
High-frequency rTMS stimulation (> 5 Hz), applied to the primary motor cortex (M1), efficiently improved the motor symptoms of PD subjects (Khedr et al., 2003; Lefaucheur et al., 2004; Elahi et al., 2009; Zanjani et al., 2015). Specifically, gait performance has been improved by stimulating the leg region of the motor cortex (Maruo et al., 2013; Lee et al., 2014; Kim et al., 2015; Yokoe et al., 2018). In addition to the M1, the supplementary motor area (SMA) and pre-SMA are also used as targets for stimulation. Accumulating evidence suggests that the SMA plays a pivotal role in the pathogenesis of FOG (Snijders et al., 2011; Shine et al., 2013; Peterson et al., 2014). Several studies have explored the clinical efficacy of high-frequency rTMS over the SMA on FOG in patients with PD (Lee et al., 2014; Mi et al., 2019; Mi et al., 2020). Lee et al. (2014) explored the therapeutic effect of rTMS on the SMA and M1. In the study, the number of freezing episodes was significantly decreased after SMA stimulation, and there was a trend for a greater reduction in freezing episodes with SMA stimulation than M1 stimulation. Mi et al. (2019) investigated the effect of rTMS on the SMA with a 4-week follow-up and found significant improvements and interaction effects in the Freezing of Gait Questionnaire score and UPDRS Part III. The pre-SMA is one of the major regions that connect to the basal ganglia and prefrontal cortex and is implicated in the regulation of emotion, motor function, and behavior. A resting-state functional magnetic resonance imaging study indicated that high-frequency rTMS over the SMA confers a beneficial effect jointly by normalizing abnormal brain functional connectivity patterns specifically associated with FOG in addition to normalizing overall disrupted connectivity patterns seen in PD (Mi et al., 2020).
Eye movement disorders have been documented in PD subjects, especially in patients with FOG (Terao et al., 2013). Saccades are controlled by various brain regions and different patterns of saccade impairment reflect pathologies in the corresponding brain regions (Anderson and MacAskill, 2013). Patients with FOG show significantly worse anti-saccade performance, indicating mutually impaired inhibitory control for gait and anti-saccade (Ewenczyk et al., 2017). Okada et al. (2021) confirmed that bilateral 10 Hz rTMS to the leg region of the MC improved MDS-UPDRS motor scores and the anti-saccade success rate, both of which require adequate inhibition of the reflexive response. The improvement in the anti-saccade success rate was correlated with that of the postural instability gait difficulty sub-scores of the MDS-UPDRS (Okada et al., 2021).
Repetitive trans-spinal magnetic stimulation (rTSMS) has been confirmed to improve camptocormia, a treatment-resistant postural abnormality observed in patients with PD. Arii et al. (2014) compared rTSMS (a train of 40 stimuli) groups to sham stimulation groups in patients with PD subjects with camptocormia. The flexion angle in the standing position significantly decreased by a mean of 10.9° after rTSMS but showed no change after sham stimulation. The flexion angle while sitting significantly decreased by 8.1° after rTSMS, whereas the sham treatment had no significant effect. The authors speculated that the effect of rTSMS was possibly due to a blockade of afferent sensory nerve fibers or disruption of akinetic corticostriatal activity.
Transcranial direct current stimulation (tDCS) is a NIBS method that modulates cortical activity. tDCS induces synaptic plasticity changes after stimulation, which has lasting effects (Nitsche and Paulus, 2000) and has some advantages over rTMS. tDCS is less expensive, provides a reliable sham stimulation condition, may lead to longer-lasting modulatory effects of cortical function, and is easy to administer and perform. In animal models, tDCS increased extracellular striatal dopamine levels (Tanaka et al., 2013). Recent systematic reviews have confirmed that tDCS improves motor function in patients with PD (Ferrucci et al., 2016; Lee et al., 2019; Beretta et al., 2020a). In these studies, tDCS protocols primarily targeted motor and prefrontal cortices (e.g., the M1 and dorsolateral prefrontal cortex [DLPFC]) since brain activation patterns in these brain regions are highly involved in successful locomotion performance in patients with PD. Anodal tDCS to the M1 has been shown to significantly improve motor performance and gait in patients with PD. A significant improvement in gait with a reduction in the number and duration of FOG episodes, along with a significant reduction in the UPDRS Part III score, was observed after anodal stimulation (Valentino et al., 2014). Thus, tDCS could potentially be used in walking training and has the potential to enhance its benefits (Kaski et al., 2014b; Schabrun et al., 2016; Costa-Ribeiro et al., 2017; Yotnuengnit et al., 2018). Some studies have shown that tDCS, in addition to gait training, did not produce clinically important effects on gait speed, stride length, or cadence in people with mild to moderate disabilities associated with PD (Nascimento et al., 2021).
The DLPFC plays an essential role in dual-tasking that requires flexibility between the two tasks performed simultaneously (Collette et al., 2005). Therefore, tDCS stimulation of the DLPFC mainly affects cognitive function. Bueno et al. (2019) showed improvements in verbal fluency and reaction time on the Stroop test. A systematic review confirmed that certain cognitive deficits in PD were improved by anterior cranial tDCS (Dinkelbach et al., 2017; Putzolu et al., 2018; Mishra and Thrasher, 2021). Multitarget stimulation of motor (M1) and cognitive (left DLPFC) networks showed greater improvement than M1 stimulation only or sham stimulation in both the Timed Up and Go (TUG) and the Stroop tests (Dagan et al., 2018).
Photobiomodulation (PBM) is also being considered for use in PD therapy. PBM therapy uses a narrow wavelength band of non-thermal light (LED or laser) to modulate the cellular response and it acts at the cellular and mitochondrial levels. In recent years, transcranial PBM therapy for mental disorders such as depression and neurodegenerative diseases such as Alzheimer’s disease and PD has been attracting attention (Hamblin, 2016). Liebert et al. (2021) conducted an RCT to evaluate the efficacy of PBM in reducing the clinical signs of PD. In this study, 12 participants with idiopathic PD underwent PBM therapy for up to 52 weeks. The primary outcome measure was mobility assessment using TUG which showed significant improvements. Moreover, cognition, dynamic balance, and fine motor skills were significantly improved.
As aforementioned, various effects have been reported for each NIBS device. Especially for FOG and postural abnormality, treatment with invasive devices such as DBS is less effective, therefore it is expected to be an alternative or adjunctive treatment for patients with these conditions. However, relatively few studies have been conducted on severely injured patients, which may limit the interpretation of the results.
Cueing with external stimuli has also been used to assist patients with PD with FOG. Various effects have been reported, and the European guidelines on PD strongly recommend the use of cues to improve walking speed (Keus et al., 2014). Studies investigating the effect of cues on the disease stage show that the effect of cues can be obtained from the early stages of PD and increases as the disease progresses (Lirani-Silva et al., 2019). External stimulus cues for improving FOG are mainly classified into spatial information that informs the user where to guide the action (e.g., floor lines) and temporal cues that provide information about the timing of movement (e.g., metronome, vibration stimuli). An example of a visual cue is the horizontal line on the floor, which has been shown to improve gait, stride length, and the beginning of walking (Lewis et al., 2000; Jiang and Norman, 2006; Lim et al., 2006). In addition, laser visual cues are often used to improve gait performance, such as stride length and walking speed (Donovan et al., 2011; Buated et al., 2012; Ferraye et al., 2016; Tang et al., 2019). Some laser cues have been developed with lasers built into the cane (Buated et al., 2012) or attached to shoes (Ferraye et al., 2016). Another study comparing the effect of a lateral line on the floor and a wearable laser light showed that the step length increased under both conditions, but the improvement in the sequence effect—which refers to the gradual decrease in amplitude—only occurs with a lateral line on the floor (Cao et al., 2020). The presentation of cues using smart glasses has also been considered as an application of this emerging technology (Zhao et al., 2016; Janssen et al., 2017), but concerns have been expressed about the specifications of smart glasses, such as weight and the reduction of the field of view due to the frame (Janssen et al., 2017).
Some studies have also shown that auditory cues can improve FOG (Delval et al., 2014; Lopez et al., 2014; Ginis et al., 2017a; Spildooren et al., 2017). Patients with PD release their anticipatory postural adjustments more quickly when auditory cueing is applied. Monotonous external inputs, such as a metronome, are often used with/without other non-auditory cues (Bachlin et al., 2010; Espay et al., 2010; Hove et al., 2012; Baram et al., 2016; Ginis et al., 2016, 2017b; Zhao et al., 2016). Hove et al. (2012) used a non-linear limit-cycle oscillator to interactively generate a rhythmic pacing sequence using the information on the number of steps taken from a shoe-mounted foot pressure sensor to improve walking.
However, some side effects of these cueing devices have also been identified. People with PD demonstrated cue dependency, expressed as a decline in movement after cue removal (Nieuwboer et al., 2009; Spildooren et al., 2012; Vercruysse et al., 2012). It has also been suggested that external cues may have a negative effect on increasing gait variability because they increase the cognitive load, as all steps need to be coordinated to synchronize (Yogev et al., 2005). These characteristics limit the effectiveness of simple cueing. To apply appropriate cueing at the required moment, effective FOG detection technologies are warranted. Ginis et al. (2017b) proposed an intelligent system in which an inertial sensor attached to the foot was used to obtain the average cadence, and auditory cues and auditory feedback were triggered only when the cadence deviated from the reference by more than 5%. This method significantly reduced cadence deviation compared to the use of normal cues. Additionally, Bachlin et al. (2010) developed a wearable system that initiated auditory cueing only when FOG was detected. Moreover, some studies have shown self-generating internal cues while singing a song instead of providing cues successfully improves gait externally (Harrison et al., 2019). The effect of internal cueing, in the form of singing, vs. external cueing, in the form of listening to music, gait was examined in people with and without PD. Internal cueing significantly improved cadence compared to external cueing (Harrison et al., 2018), and external cueing increased gait variability whereas internal cues did not (Harrison et al., 2019; Horin et al., 2020). Since higher gait variability is associated with falls, this finding is important for stable walking when addressing PD with FOG.
Invasive vagus nerve stimulation (VNS), delivered by a surgically implanted device, has been approved as an adjunctive neuromodulation therapy for epilepsy (Terry, 2014). VNS is supposed to affect various brain regions through direct effects on the nucleus tractus solitarius and locus coeruleus (Kraus et al., 2007; Oshinsky et al., 2014). Anti-inflammatory properties of VNS have been suggested (Corcoran et al., 2005; Majoie et al., 2011) and potential applications to a wide range of inflammatory disorders have been advocated (Bonaz et al., 2016). Neuroinflammation has been implicated in the pathogenesis of PD (Akiyama et al., 2000); therefore, VNS may be effective in the treatment of PD.
Recently, the development of portable, non-invasive VNS (nVNS) devices has simplified this treatment modality (Yuan and Silberstein, 2016). Notably, the application of nVNS in patients with PD has shown beneficial effects for FOG (Mondal et al., 2019). In the study, the application of 2 × 120 s acute nVNS improved the number of steps taken while turning and reduced UPDRS Part III scores in patients with FOG induced by PD by two-dimensional spatiotemporal gait parameter analysis. These short-term effects are most likely due to indirect activation of central neural circuits, including noradrenergic projections from the locus coeruleus (Johnson and Wilson, 2018), a brain region implicated in the pathogenesis of FOG (Ono et al., 2016). Morris et al. (2019) found significant improvement in step length variability in patients with PD after a single application of cervical nVNS.
A randomized, double-blind, sham-controlled crossover study in which nVNS was applied three times a day for 1 month to confirm the long-term effects of cervical nVNS (Mondal et al., 2021), showed improvement in motor function in patients with PD and a significant reduction in serum inflammatory markers. Intriguingly, the analysis of serum biomarkers after nVNS showed a reduction in neuroinflammatory markers (Tumor Necrosis Factor-α) along with increased brain-derived neurotrophic factor (BDNF), which implicated increased neuroplasticity. Given that neuroinflammation has been implicated in the pathogenesis of PD (Akiyama et al., 2000), nVNS may have disease-modifying effects.
Vibration stimuli to the muscles act as a powerful proprioceptive input and strongly affect the motion perception during extremity movements of healthy people and patients with various neurological disorders (Cohen and Starr, 1985; Han et al., 2014). Peripheral vibrotactile stimulation has been shown to improve bradykinesia, FOG, gait impairment, and postural instability in patients with PD, possibly by affecting the central pattern generator in a desynchronized state (Zehr and Haridas, 2003; Volpe et al., 2014; Pereira et al., 2016; Syrkin-Nikolau et al., 2018; Peppe et al., 2019; Serio et al., 2019; Spolaor et al., 2021; Tan et al., 2021). Three possible mechanisms for how vibration stimuli work against FOG in patients with PD have been laid forth: (i) a cueing effect, (ii) modulation effect against impairment in dealing with conflicting cognitive/attentional resources, and (iii) enhanced proprioceptive processing (Pereira et al., 2016).
Peripheral vibrotactile stimulation delivered by a C-2 tactor glove (Engineering Acoustics Inc., Florida, United States) with high-frequency trains in a patterned sequence acutely improved gait asymmetry, arrhythmicity, and wrist bradykinesia in patients with PD (Syrkin-Nikolau et al., 2018). The study indicated that peripheral vibrotactile stimulation is safe and its effect continues even 1 month after stimulation. The use of light-touch contact is being considered as another approach. Light-touch contact is a phenomenon in which a part of the body lightly (<1 N) touches a stable surface to obtain sensory feedback about the orientation of the body and limbs in space, which can activate the postural muscles and adjust the axial tension of the body’s posture. Light-touch contact has also been found to attenuate postural sway in patients with PD, although this is a commonly occurring effect (Franzen et al., 2012; Rabin et al., 2013). Walking has been found to improve the center of gravity shift and improve gait initiation (Ditthaphongphakdee and Gaogasigam, 2021). A wearable device using vibration stimuli on the fingertips provides tactile feedback reportedly improved body balance in normal subjects (Shima et al., 2021) and may also be effective for PD subjects.
Although the improvement rates were small, muscle vibration therapy in the lower limbs using eight vibrators (60 Hz) made by the study authors, significantly improved stride length and walking speed in patients with PD (Han et al., 2014). Another study reported that hand-made vibratory devices embedded in elastic insoles (70 Hz), where the vibratory device senses the pressure on the sole and delivers the vibration stimulus during walking, decreased stride variability while increasing walking speed, stride duration, stride length, and cadence in patients with PD (Novak and Novak, 2006). Admittedly, these changes were significant but small. Another study placed the CUE1 (Charco Neurotech Ltd., London, United Kingdom) on the patient’s sternum to produce focused vibrotactile pulsatile stimuli (Tan et al., 2021). Since this device was effective for FOG when it was turned on before the FOG episodes, the authors hypothesized that CUE1 has a cueing effect in addition to enhanced proprioceptive stimuli. Another study revealed that focal vibration training using Equistasi® (Equistasi®, Italy) improved postural stability in patients with PD. The Equistasi® is composed of nanotechnological particles that transform the body temperature into a mechanical high-frequency vibration (0.8 N, 9000 Hz). The device is attached over the seventh cervical vertebra and on each soleus muscle-tendon to alleviate gait disturbances and postural instability (Volpe et al., 2014; Peppe et al., 2019; Serio et al., 2019; Spolaor et al., 2021). RCTs using Equistasi® showed significantly improved motor performance in patients with PD.
Another study revealed that a custom-made vibration device (100 Hz and 1.2 mm of amplitude) attached to the less affected limb moderately alleviated FOG in patients with PD when vibration is applied following FOG onset (Pereira et al., 2016). Importantly, no positive effects for FOG were identified when the vibration device was applied before the FOG onset, which indicated that vibration did not serve as an external cue in the study. Additionally, vibration stimuli did not exert a positive effect when the more affected limb triggered attentional processing by vibration, indicating that the modulation effect of choosing conflicting cognitive/attentional resources is unlike the mechanism underpinning the effect (Pereira et al., 2016). Given that improper sensory feedback causes worsening of movements (Nieuwboer et al., 2009), vibration stimuli in the lower extremities strongly enhance proprioceptive information and play an important role in controlling posture and balance (Sorensen et al., 2002; Pereira et al., 2016), and the more preserved side of the basal ganglia seems to properly process the enhanced proprioceptive information (Pereira et al., 2016). Upper limb freezing in PD patients with FOG is associated with decreased activation in the basal ganglia and increased activation in the frontal lobes, including the supplementary and primary motor cortices, which indicated the impairment in the indirect pathway-driven non-selective inhibitory program through the cortico-basal ganglia-thalamo-cortical feedback loop in FOG pathogenesis (Vercruysse et al., 2014). Since local vibration stimuli are reported to evoke focused activation of motor cortical circuits (Rosenkranz and Rothwell, 2003), they may help focus on the desired movements through the thalamus.
Peripheral sensory deficits, particularly reduced plantar sensitivity, have been reported in PD (Pratorius et al., 2003; Nolano et al., 2008). This reduction may result from the loss of cutaneous receptors, encapsulated endings, or free nerve endings (Nolano et al., 2008), and is associated with reduced control of compensatory stepping and impaired balance, which causes falls (Pratorius et al., 2003; Kerr et al., 2010).
Textured insoles significantly decreased mediolateral postural sway, which indicated an improvement in standing balance (Qiu et al., 2013). The continuous use of textured insoles for 1-week increased stride length, and the effect continued for another week without textured insoles (Lirani-Silva et al., 2017). Ribbed insoles produce a significant increase in single-limb support time and normalization of the muscle activation sequence of the tibialis anterior in patients with PD (Jenkins et al., 2009). As described in the previous section, vibration stimulation at the soles increased walking speed and improved stride variability in PD subjects (Novak and Novak, 2006). However, the improvement rates in these studies were usually under 20%.
The automated mechanical peripheral stimulation (AMPS) device Gondola™ (Gondola Medical Technologies SA, Switzerland) has been proven effective for addressing motor impairment in patients with PD. Immediate and long-term increases in stride length and gait speed with Gondola™ have been reported (Kleiner et al., 2015; Stocchi et al., 2015). The benefit was maintained for 10 days after the last treatment (Stocchi et al., 2015). Based on these studies, a randomized controlled clinical trial using Gondola™ was performed involving patients with PD, and Gondola™ promoted faster walking with longer strides after 6–8 stimulation sessions (Galli et al., 2018; Kleiner et al., 2018; Pagnussat et al., 2018, 2020; Pinto et al., 2018). Contrastingly, there was no positive effect in terms of improving static postural control in individuals with PD and FOG (Prusch et al., 2018). Interestingly, patients with PD who underwent treatment with Gondola™ showed higher serum BDNF and lower serum cortisol levels in accordance with improvements in gait parameters (Pagnussat et al., 2018). Gondola™ did increase gait velocity and resting-state brain connectivity between the basal ganglia and sensory-related brain areas (insular and somatosensory cortices) in PD subjects. Gait velocity is positively correlated with increased connectivity between the sensory, motor, and supplementary motor cortices (Pagnussat et al., 2020). These findings may help elucidate the mechanism by which AMPS influences gait.
The electrical stimulation of the common peroneal nerve immediately improved average stride length during a three-min walk and reduced the frequency of falls and FOG during an 8-week stimulation period which diminished 4 weeks after the cessation (Mann et al., 2008). A single randomized controlled trial (RCT) showed significant improvement in 10 m walking speed at week 18 compared to the standard care group (Taylor et al., 2021). However, the decrease in the UPDRS score was not significant and the improvement in 10 m walking speed disappeared 4 weeks following the stimulation session.
To reduce FOG, the use of a “fixed” rhythmic sensory electrical stimulation (sES) cueing strategy has been proposed (Rosenthal et al., 2018). sES cueing was delivered by a voltage-controlled two-channel stimulator and skin surface electrodes were placed over the hamstring or quadriceps muscle. In this study, nine patients with PD walked while receiving sES cueing and their performance was evaluated. Results showed that sES cueing significantly reduces the time taken for the walking task and in the number of FOG episodes by 14.23 and 58.28, respectively.
For the systematic review, we identified RCT studies in the last 10 years (2011–2021). Concerning the invasive devices, RCTs for DBS and LCIG were extracted. These devices have already received FDA certification and a CE mark and are currently being used. The results show a higher efficacy than non-invasive devices. There were no RCTs on SCS and continuous subcutaneous infusion of levodopa.
As represented in Table 1, most RCTs refer to non-invasive devices. RCTs for tremors and cueing devices were few despite many devices having been developed. In contrast, extensive RCTs of rTMS and tDCS for various targets and frequencies have been performed. Most of the rTMS studies showed improvement in motor function as assessed by TUG and UPDRS Part III. rTMS is FDA approved for obsessive-compulsive disorder and depression but has not yet been approved for PD. Several studies have targeted M1 for the improvement of motor function, and most of them have shown that it improves motor function. The second most common target was SMA; some studies showed improvement in FOG as well as improvement in motor function. tDCS has also been used to target M1 and DLPFC. tDCS over M1 has been reported to improve motor function. tDCS over DLPFC may not directly improve motor function, but may improve performance under certain conditions, such as dual-tasking. The effects of tDCS, when used in combination with physical training, have also been studied extensively. There are multiple reports of improved gait performance when stimulated in conjunction with walking. Mechanical/electrical peripheral stimulation devices have also been the subject of many RCTs, which have reported effectiveness in gait performance. Most of the studies have been with Gondola™, a device that is FDA certificated and CE marked. As shown in Table 1, RCTs have been conducted on other non-invasive devices and have shown improvement in motor symptoms, but the number of studies is small, and certification has not been obtained. There is a big difference between invasive and non-invasive treatments in terms of treatment effectiveness. For example, when comparing the improvement of UPDRS Part III, DBS may have an effect of more than 15 points, while non-invasive devices usually have an effect of a few points, and none of them improve by more than 10 points.
This paper describes the progress made in the development of therapeutic devices for motor symptoms in patients with PD. We have conducted a narrative literature review and a systematic review of recent RCTs. Generally, invasive devices are more effective than non-invasive devices in terms of improving motor function in patients with PD. DBS has a striking effect on motor symptoms in PD subjects and, specifically, aDBS is more effective than cDBS, pointing to the importance of instrumental advances. Little et al. (2013) confirmed that motor scores improved during aDBS were 27% better than cDBS. In the future, it is expected that a closed-loop system in which the release of neurotransmitters is a control variable will be realized by FSCV and that a more accurate DBS system might be developed. SCS with burst waveform stimulation showed acute and larger improvements in both motor symptoms, including tremor and gait parameters (Kobayashi et al., 2018; Furusawa et al., 2020). Continuous infusion of levodopa-carbidopa via an intestinal infusion (Duodopa®) system has also demonstrated significantly decreased off-time and increased on-time without troublesome dyskinesia (Olanow et al., 2014). In recent years, as a less invasive method, the continuous subcutaneous infusion of levodopa or apomorphine using ND0612 or ND0701 was also developed (Ramot et al., 2018; Olanow et al., 2021; Larson and Simuni, 2022). The effects of these invasive medical devices are usually drastic.
Regarding non-invasive medical devices, we introduced several devices that are effective for tremors, gait, and FOG. These devices have seen improved efficacy and have the advantage of being non-surgical and easy to use. Thus, each device has its characteristics and effects, and the proper selection and use of these devices will result in more effective treatment. As shown in the systematic review, a growing number of high-evidence studies of non-invasive devices have been conducted in recent years. In particular, rTMS has been subjected to meta-analysis and is more reliable than other devices (Elahi et al., 2009; Zanjani et al., 2015). The meta-analysis revealed that targeting M1 improved UPDRS and that targeting multiple brain regions had a short-term effect on functional locomotion. In addition, improvement in gait was reported when combined with physical training (Kaski et al., 2014b; Costa-Ribeiro et al., 2017; Yotnuengnit et al., 2018; Lee and Kim, 2021). rTMS and tDCS have been studied for different stimulation targets, and different effects were reported for each. A relatively large number of RCTs implies that researchers who performed rTMS and tDCS have some confidence in their efficacy on motor symptoms in patients with PD. Further research is required to determine the best treatment method and target. Significant effects have also been reported for other non-invasive devices, such as nVNS, vibrotactile stimulation devices, mechanical peripheral stimulation, and sensory electrical stimulation by RCT. Non-invasive devices are less effective than invasive devices, but easy-to-use and less invasive characteristics make their use more feasible and easier. They may grow more prevalent in the upcoming decade. They also have the potential to become standard treatments for PD in the next generation.
The devices introduced in this paper include prototypes, which need to be further developed for practical use. Since it is important to develop safe and reliable products, it is very important to make effective, reliable, and easy-to-use gold-standard criteria to evaluate them.
JF, RM, and NY designed and drafted the manuscript. All authors revised and approved the final version of the manuscript.
This work was supported by the Terumo Life Science Foundation, JSPS KAKENHI Grant Numbers 20K17932 and 16KK0182. Department of Advanced Brain Research was supported by the Beauty Life Corporation.
The authors declare that the research was conducted in the absence of any commercial or financial relationships that could be construed as a potential conflict of interest.
All claims expressed in this article are solely those of the authors and do not necessarily represent those of their affiliated organizations, or those of the publisher, the editors and the reviewers. Any product that may be evaluated in this article, or claim that may be made by its manufacturer, is not guaranteed or endorsed by the publisher.
aDBS, adaptive deep brain stimulation; AMPS, automated mechanical peripheral stimulation; BDNF, brain-derived neurotrophic factor; cDBS, conventional deep brain stimulation; DBS, deep brain stimulation; DLPFC, dorsolateral prefrontal cortex; EEG, electroencephalography; EMG, electromyography; EMS, electrical muscle stimulation; ET, essential tremor; FDA, Food and Drug Administration; fMRI, functional magnetic resonance imaging; FTM-TRS, Fahn-Tolosa-Marin Tremor Rating Scale; GPi, internal globus pallidus; FOG, freezing of gait; FOGQ, Freezing of Gait Questionnaire score; FSCV, fast scan cyclovoltammetry; LCIG, continuous infusion of levodopa-carbidopa gel; LFPs, local field potentials; M1, primary motor cortex; NIBS, non-invasive brain stimulation; nVNS, non-invasive vagus nerve stimulation; PBM, photobiomodulation; PD, Parkinson’s disease; POT, primary orthostatic tremor; RCT, randomized controlled trial; rTMS, repetitive transcranial magnetic stimulation; PMC, premotor cortex; RMS, root mean square; ROM, range of motion; rTSMS, repetitive trans-spinal magnetic stimulation; SCS, spinal cord stimulation; SEMG, surface electromyography; sES, sensory electrical stimulation; SMA, supplementary motor area; STN, subthalamic nucleus; tDCS, transcranial direct current stimulation; TEEDs, total electrical energy delivered to the tissues per second; TETRAS, the Tremor Research Group’s Essential Tremor Rating Assessment Scale; tsDCS, trans-spinal direct current stimulation; TUG, timed up and go; UPDRS, the Unified Parkinson’s Disease Rating Scale; VIM, ventral intermediate nucleus of the thalamus; VNS, vagus nerve stimulation.
Agari, T., and Date, I. (2012). Spinal cord stimulation for the treatment of abnormal posture and gait disorder in patients with Parkinson’s disease. Neurol. Med. Chir. 52, 470–474. doi: 10.2176/nmc.52.470
Akiyama, H., Barger, S., Barnum, S., Bradt, B., Bauer, J., Cole, G. M., et al. (2000). Inflammation and Alzheimer’s disease. Neurobiol. Aging 21, 383–421. doi: 10.1016/s0197-4580(00)00124-x
Akiyama, H., Nukui, S., Akamatu, M., Hasegawa, Y., Nishikido, O., and Inoue, S. (2017). Effectiveness of spinal cord stimulation for painful camptocormia with Pisa syndrome in Parkinson’s disease: a case report. BMC Neurol. 17:148. doi: 10.1186/s12883-017-0926-y
Anderson, T. J., and MacAskill, M. R. (2013). Eye movements in patients with neurodegenerative disorders. Nat. Rev. Neurol. 9, 74–85. doi: 10.1038/nrneurol.2012.273
Aquino, C. C., Duffley, G., Hedges, D. M., Vorwerk, J., House, P. A., Ferraz, H. B., et al. (2019). Interleaved deep brain stimulation for dyskinesia management in Parkinson’s disease. Mov. Disord. 34, 1722–1727. doi: 10.1002/mds.27839
Arii, Y., Sawada, Y., Kawamura, K., Miyake, S., Taichi, Y., Izumi, Y., et al. (2014). Immediate effect of spinal magnetic stimulation on camptocormia in Parkinson’s disease. J. Neurol. Neurosurg. Psychiatry 85, 1221–1226. doi: 10.1136/jnnp-2014-307651
Bachlin, M., Plotnik, M., Roggen, D., Giladi, N., Hausdorff, J. M., and Troster, G. (2010). A wearable system to assist walking of Parkinson’s disease patients. Methods Inf. Med. 49, 88–95. doi: 10.3414/ME09-02-0003
Baijens, L. W., Speyer, R., Passos, V. L., Pilz, W., van der Kruis, J., Haarmans, S., et al. (2013). Surface electrical stimulation in dysphagic Parkinson patients: a randomized clinical trial. Laryngoscope 123, E38–E44. doi: 10.1002/lary.24119
Baram, Y., Aharon-Peretz, J., Badarny, S., Susel, Z., and Schlesinger, I. (2016). Closed-loop auditory feedback for the improvement of gait in patients with Parkinson’s disease. J. Neurol. Sci. 363, 104–106. doi: 10.1016/j.jns.2016.02.021
Barbe, M. T., Maarouf, M., Alesch, F., and Timmermann, L. (2014). Multiple source current steering–a novel deep brain stimulation concept for customized programming in a Parkinson’s disease patient. Parkinsonism Relat. Disord. 20, 471–473. doi: 10.1016/j.parkreldis.2013.07.021
Barbic, F., Galli, M., Dalla Vecchia, L., Canesi, M., Cimolin, V., Porta, A., et al. (2014). Effects of mechanical stimulation of the feet on gait and cardiovascular autonomic control in Parkinson’s disease. J. Appl. Physiol. (1985) 116, 495–503. doi: 10.1152/japplphysiol.01160.2013
Bathien, N., Rondot, P., and Toma, S. (1980). Inhibition and synchronisation of tremor induced by a muscle twitch. J. Neurol. Neurosurg. Psychiatry 43, 713–718. doi: 10.1136/jnnp.43.8.713
Baumann, C. R., Imbach, L. L., Baumann-Vogel, H., Uhl, M., Sarnthein, J., and Surucu, O. (2012). Interleaving deep brain stimulation for a patient with both Parkinson’s disease and essential tremor. Mov. Disord. 27, 1700–1701. doi: 10.1002/mds.25221
Benabid, A. L., Chabardes, S., Mitrofanis, J., and Pollak, P. (2009). Deep brain stimulation of the subthalamic nucleus for the treatment of Parkinson’s disease. Lancet Neurol. 8, 67–81. doi: 10.1016/S1474-4422(08)70291-6
Benninger, D. H., Iseki, K., Kranick, S., Luckenbaugh, D. A., Houdayer, E., and Hallett, M. (2012). Controlled study of 50-Hz repetitive transcranial magnetic stimulation for the treatment of Parkinson disease. Neurorehabil. Neural Repair. 26, 1096–1105. doi: 10.1177/1545968312445636
Beretta, V. S., Conceicao, N. R., Nobrega-Sousa, P., Orcioli-Silva, D., Dantas, L., Gobbi, T. B., et al. (2020a). Transcranial direct current stimulation combined with physical or cognitive training in people with Parkinson’s disease: a systematic review. J. Neuroeng. Rehabil. 17:74. doi: 10.1186/s12984-020-00701-6
Beretta, V. S., Vitorio, R., Nobrega-Sousa, P., Conceicao, N. R., Orcioli-Silva, D., Pereira, M. P., et al. (2020b). Effect of different intensities of transcranial direct current stimulation on postural response to external perturbation in patients with Parkinson’s disease. Neurorehabil. Neural Repair. 34, 1009–1019. doi: 10.1177/1545968320962513
Beringhause, S., Rosen, M., and Huang, S. (1989). “Evaluation of a damped joystick for people disabled by intention tremor,” in Proceedings of the 12th Annual Conference on Rehabilitation Technology, New Orleans, LA.
Bhatia, K. P., Bain, P., Bajaj, N., Elble, R. J., Hallett, M., Louis, E. D., et al. (2018). Consensus statement on the classification of tremors. from the task force on tremor of the International Parkinson and Movement Disorder Society. Mov. Disord. 33, 75–87. doi: 10.1002/mds.27121
Bocci, T., Prenassi, M., Arlotti, M., Cogiamanian, F. M., Borrellini, L., Moro, E., et al. (2021). Eight-hours conventional versus adaptive deep brain stimulation of the subthalamic nucleus in Parkinson’s disease. NPJ Parkinsons Dis. 7:88. doi: 10.1038/s41531-021-00229-z
Bonaz, B., Sinniger, V., and Pellissier, S. (2016). Anti-inflammatory properties of the vagus nerve: potential therapeutic implications of vagus nerve stimulation. J. Physiol. 594, 5781–5790. doi: 10.1113/JP271539
Broeder, S., Heremans, E., Pinto Pereira, M., Nackaerts, E., Meesen, R., Verheyden, G., et al. (2019). Does transcranial direct current stimulation during writing alleviate upper limb freezing in people with Parkinson’s disease? A pilot study. Hum. Mov. Sci. 65, 142–153. doi: 10.1016/j.humov.2018.02.012
Bronstein, J. M., Tagliati, M., Alterman, R. L., Lozano, A. M., Volkmann, J., Stefani, A., et al. (2011). Deep brain stimulation for Parkinson disease: an expert consensus and review of key issues. Arch. Neurol. 68:165. doi: 10.1001/archneurol.2010.260
Brys, M., Fox, M. D., Agarwal, S., Biagioni, M., Dacpano, G., Kumar, P., et al. (2016). Multifocal repetitive TMS for motor and mood symptoms of Parkinson disease: a randomized trial. Neurology 87, 1907–1915. doi: 10.1212/WNL.0000000000003279
Buated, W., Sriyudthsak, M., Sribunruangrit, N., and Bhidayasiri, R. (2012). A low-cost intervention for improving gait in Parkinson’s disease patients: a cane providing visual cues. Eur. Geriatr. Med. 3, 126–130. doi: 10.1016/j.eurger.2012.01.006
Bueno, M. E. B., do Nascimento Neto, L. I., Terra, M. B., Barboza, N. M., Okano, A. H., and Smaili, S. M. (2019). Effectiveness of acute transcranial direct current stimulation on non-motor and motor symptoms in Parkinson’s disease. Neurosci. Lett. 696, 46–51. doi: 10.1016/j.neulet.2018.12.017
Buki, E., Katz, R., Zacksenhouse, M., and Schlesinger, I. (2018). Vib-bracelet: a passive absorber for attenuating forearm tremor. Med. Biol. Eng. Comput. 56, 923–930. doi: 10.1007/s11517-017-1742-7
Bunting-Perry, L., Spindler, M., Robinson, K. M., Noorigian, J., Cianci, H. J., and Duda, J. E. (2013). Laser light visual cueing for freezing of gait in Parkinson disease: a pilot study with male participants. J. Rehabil. Res. Dev. 50, 223–230. doi: 10.1682/jrrd.2011.12.0255
Cai, Y., Reddy, R. D., Varshney, V., and Chakravarthy, K. V. (2020). Spinal cord stimulation in Parkinson’s disease: a review of the preclinical and clinical data and future prospects. Bioelectron. Med. 6:5. doi: 10.1186/s42234-020-00041-9
Cao, S. S., Yuan, X. Z., Wang, S. H., Taximaimaiti, R., and Wang, X. P. (2020). Transverse strips instead of wearable laser lights alleviate the sequence effect toward a destination in Parkinson’s disease patients with freezing of gait. Front. Neurol. 11:838. doi: 10.3389/fneur.2020.00838
Chan, A. K., Chan, A. Y., Lau, D., Durcanova, B., Miller, C. A., Larson, P. S., et al. (2018). Surgical management of camptocormia in Parkinson’s disease: systematic review and meta-analysis. J. Neurosurg. 131, 368–375. doi: 10.3171/2018.4.JNS173032
Chang, S. Y., Jay, T., Munoz, J., Kim, I., and Lee, K. H. (2012a). Wireless fast-scan cyclic voltammetry measurement of histamine using WINCS–a proof-of-principle study. Analyst 137, 2158–2165. doi: 10.1039/c2an16038b
Chang, S. Y., Kim, I., Marsh, M. P., Jang, D. P., Hwang, S. C., Van Gompel, J. J., et al. (2012b). Wireless fast-scan cyclic voltammetry to monitor adenosine in patients with essential tremor during deep brain stimulation. Mayo Clin. Proc. 87, 760–765. doi: 10.1016/j.mayocp.2012.05.006
Chang, S. Y., Kimble, C. J., Kim, I., Paek, S. B., Kressin, K. R., Boesche, J. B., et al. (2013). Development of the Mayo Investigational Neuromodulation Control System: toward a closed-loop electrochemical feedback system for deep brain stimulation. J. Neurosurg. 119, 1556–1565. doi: 10.3171/2013.8.JNS122142
Chang, W. H., Kim, M. S., Cho, J. W., Youn, J., Kim, Y. K., Kim, S. W., et al. (2016). Effect of cumulative repetitive transcranial magnetic stimulation on freezing of gait in patients with atypical Parkinsonism: a pilot study. J. Rehabil. Med. 48, 824–828. doi: 10.2340/16501977-2140
Chung, C. L., Mak, M. K., and Hallett, M. (2020). Transcranial magnetic stimulation promotes gait training in Parkinson disease. Ann. Neurol. 88, 933–945. doi: 10.1002/ana.25881
Cohen, L. G., and Starr, A. (1985). Vibration and muscle contraction affect somatosensory evoked potentials. Neurology 35, 691–698. doi: 10.1212/wnl.35.5.691
Cohen, O. S., Rigbi, A., Yahalom, G., Warman-Alaluf, N., Nitsan, Z., Zangen, A., et al. (2018). Repetitive deep TMS for Parkinson disease: a 3-month double-blind, randomized sham-controlled study. J. Clin. Neurophysiol. 35, 159–165. doi: 10.1097/WNP.0000000000000455
Collette, F., Olivier, L., Van der Linden, M., Laureys, S., Delfiore, G., Luxen, A., et al. (2005). Involvement of both prefrontal and inferior parietal cortex in dual-task performance. Brain Res. Cogn. Brain Res. 24, 237–251. doi: 10.1016/j.cogbrainres.2005.01.023
Conceicao, N. R., Gobbi, L. T. B., Nobrega-Sousa, P., Orcioli-Silva, D., Beretta, V. S., Lirani-Silva, E., et al. (2021). Aerobic exercise combined with transcranial direct current stimulation over the prefrontal cortex in Parkinson disease: effects on cortical activity, gait, and cognition. Neurorehabil. Neural Repair. 35, 717–728. doi: 10.1177/15459683211019344
Corcoran, C., Connor, T. J., O’Keane, V., and Garland, M. R. (2005). The effects of vagus nerve stimulation on pro- and anti-inflammatory cytokines in humans: a preliminary report. Neuroimmunomodulation 12, 307–309. doi: 10.1159/000087109
Cosentino, G., Valentino, F., Todisco, M., Alfonsi, E., Davi, R., Savettieri, G., et al. (2017). Effects of more-affected vs. less-affected motor cortex tDCS in Parkinson’s disease. Front. Hum. Neurosci. 11:309. doi: 10.3389/fnhum.2017.00309
Costa-Ribeiro, A., Maux, A., Bosford, T., Aoki, Y., Castro, R., Baltar, A., et al. (2017). Transcranial direct current stimulation associated with gait training in Parkinson’s disease: a pilot randomized clinical trial. Dev. Neurorehabil. 20, 121–128. doi: 10.3109/17518423.2015.1131755
Cury, R. G., Carra, R. B., Capato, T. T. C., Teixeira, M. J., and Barbosa, E. R. (2020). Spinal cord stimulation for Parkinson’s disease: dynamic habituation as a mechanism of failure? Mov. Disord. 35, 1882–1883. doi: 10.1002/mds.28271
da Silva, D. C. L., Lemos, T., de Sa, F. A., Horsczaruk, C. H. R., Pedron, C. A., de Carvalho, R. E., et al. (2018). Effects of acute transcranial direct current stimulation on gait kinematics of individuals with Parkinson disease. Top. Geriatr. Rehabil. 34, 262–268.
Dagan, M., Herman, T., Harrison, R., Zhou, J., Giladi, N., Ruffini, G., et al. (2018). Multitarget transcranial direct current stimulation for freezing of gait in Parkinson’s disease. Mov. Disord. 33, 642–646. doi: 10.1002/mds.27300
Davis, J. T., Lyons, K. E., and Pahwa, R. (2006). Freezing of gait after bilateral subthalamic nucleus stimulation for Parkinson’s disease. Clin. Neurol. Neurosurg. 108, 461–464. doi: 10.1016/j.clineuro.2005.07.008
Delval, A., Moreau, C., Bleuse, S., Tard, C., Ryckewaert, G., Devos, D., et al. (2014). Auditory cueing of gait initiation in Parkinson’s disease patients with freezing of gait. Clin. Neurophysiol. 125, 1675–1681. doi: 10.1016/j.clinph.2013.12.101
Diaz, N. L., and Louis, E. D. (2010). Survey of medication usage patterns among essential tremor patients: movement disorder specialists vs. general neurologists. Parkinsonism Relat. Disord. 16, 604–607. doi: 10.1016/j.parkreldis.2010.07.011
Dinkelbach, L., Brambilla, M., Manenti, R., and Brem, A. K. (2017). Non-invasive brain stimulation in Parkinson’s disease: exploiting crossroads of cognition and mood. Neurosci. Biobehav. Rev. 75, 407–418. doi: 10.1016/j.neubiorev.2017.01.021
Ditthaphongphakdee, S., and Gaogasigam, C. (2021). The effects of light touch cue on gait initiation in patients with Parkinson’s disease. J. Bodyw. Mov. Ther. 26, 187–192. doi: 10.1016/j.jbmt.2020.08.009
Donovan, S., Lim, C., Diaz, N., Browner, N., Rose, P., Sudarsky, L. R., et al. (2011). Laserlight cues for gait freezing in Parkinson’s disease: an open-label study. Parkinsonism Relat. Disord. 17, 240–245. doi: 10.1016/j.parkreldis.2010.08.010
Dosen, S., Muceli, S., Dideriksen, J. L., Romero, J. P., Rocon, E., Pons, J., et al. (2015). Online tremor suppression using electromyography and low-level electrical stimulation. IEEE Trans. Neural. Syst. Rehabil. Eng. 23, 385–395. doi: 10.1109/TNSRE.2014.2328296
Dubois, B., and Pillon, B. (1997). Cognitive deficits in Parkinson’s disease. J. Neurol. 244, 2–8. doi: 10.1007/pl00007725
Eggert, K., Schrader, C., Hahn, M., Stamelou, M., Russmann, A., Dengler, R., et al. (2008). Continuous jejunal levodopa infusion in patients with advanced Parkinson disease: practical aspects and outcome of motor and non-motor complications. Clin. Neuropharmacol. 31, 151–166. doi: 10.1097/wnf.0b013e31814b113e
Einevoll, G. T., Kayser, C., Logothetis, N. K., and Panzeri, S. (2013). Modelling and analysis of local field potentials for studying the function of cortical circuits. Nat. Rev. Neurosci. 14, 770–785. doi: 10.1038/nrn3599
Elahi, B., Elahi, B., and Chen, R. (2009). Effect of transcranial magnetic stimulation on Parkinson motor function–systematic review of controlled clinical trials. Mov. Disord. 24, 357–363. doi: 10.1002/mds.22364
Espay, A. J., Baram, Y., Dwivedi, A. K., Shukla, R., Gartner, M., Gaines, L., et al. (2010). At-home training with closed-loop augmented-reality cueing device for improving gait in patients with Parkinson disease. J. Rehabil. Res. Dev. 47, 573–581. doi: 10.1682/jrrd.2009.10.0165
Ewenczyk, C., Mesmoudi, S., Gallea, C., Welter, M. L., Gaymard, B., Demain, A., et al. (2017). Antisaccades in Parkinson disease: a new marker of postural control? Neurology 88, 853–861. doi: 10.1212/WNL.0000000000003658
Fahn, S., and Sulzer, D. (2004). Neurodegeneration and neuroprotection in Parkinson disease. NeuroRx 1, 139–154. doi: 10.1602/neurorx.1.1.139
Farris, S., and Giroux, M. (2013). Retrospective review of factors leading to dissatisfaction with subthalamic nucleus deep brain stimulation during long-term management. Surg. Neurol. Int. 4:69. doi: 10.4103/2152-7806.112612
Fasano, A., Aquino, C. C., Krauss, J. K., Honey, C. R., and Bloem, B. R. (2015). Axial disability and deep brain stimulation in patients with Parkinson disease. Nat. Rev. Neurol. 11, 98–110. doi: 10.1038/nrneurol.2014.252
Fenelon, G., Goujon, C., Gurruchaga, J. M., Cesaro, P., Jarraya, B., Palfi, S., et al. (2012). Spinal cord stimulation for chronic pain improved motor function in a patient with Parkinson’s disease. Parkinsonism Relat. Disord. 18, 213–214. doi: 10.1016/j.parkreldis.2011.07.015
Fernandez-Lago, H., Bello, O., Mora-Cerda, F., Montero-Camara, J., and Fernandez-Del-Olmo, M. A. (2017). Treadmill walking combined with anodal transcranial direct current stimulation in Parkinson disease: a pilot study of kinematic and neurophysiological effects. Am. J. Phys. Med. Rehabil. 96, 801–808. doi: 10.1097/PHM.0000000000000751
Ferraye, M. U., Fraix, V., Pollak, P., Bloem, B. R., and Debu, B. (2016). The laser-shoe: a new form of continuous ambulatory cueing for patients with Parkinson’s disease. Parkinsonism Relat. Disord. 29, 127–128. doi: 10.1016/j.parkreldis.2016.05.004
Ferrucci, R., Mameli, F., Ruggiero, F., and Priori, A. (2016). Transcranial direct current stimulation as treatment for Parkinson’s disease and other movement disorders. Basal Ganglia 6, 53–61. doi: 10.1016/j.baga.2015.12.002
Fonoff, E. T., de Lima-Pardini, A. C., Coelho, D. B., Monaco, B. A., Machado, B., Pinto de Souza, C., et al. (2019). Spinal cord stimulation for freezing of gait: from bench to bedside. Front. Neurol. 10:905. doi: 10.3389/fneur.2019.00905
Franzen, E., Paquette, C., Gurfinkel, V., and Horak, F. (2012). Light and heavy touch reduces postural sway and modifies axial tone in Parkinson’s disease. Neurorehabil. Neural Repair. 26, 1007–1014. doi: 10.1177/1545968312437942
Furusawa, Y., Matsui, A., Kobayashi-Noami, K., Kojima, Y., Tsubouchi, A., Todoroki, D., et al. (2020). Burst spinal cord stimulation for pain and motor function in Parkinson’s disease: a case series. Clin. Park. Relat. Disord. 3, 100043. doi: 10.1016/j.prdoa.2020.100043
Gallego, J. A., Rocon, E., Belda-Lois, J. M., and Pons, J. L. (2013). A neuroprosthesis for tremor management through the control of muscle co-contraction. J. Neuroeng. Rehabil. 10:36. doi: 10.1186/1743-0003-10-36
Galli, M., Vicidomini, C., Rozin Kleiner, A. F., Vacca, L., Cimolin, V., Condoluci, C., et al. (2018). Peripheral neurostimulation breaks the shuffling steps patterns in Parkinsonian gait: a double blind randomized longitudinal study with automated mechanical peripheral stimulation. Eur. J. Phys. Rehabil. Med. 54, 860–865. doi: 10.23736/S1973-9087.18.05037-2
Garcia Ruiz, P. J., Sesar Ignacio, A., Ares Pensado, B., Castro Garcia, A., Alonso Frech, F., Alvarez Lopez, M., et al. (2008). Efficacy of long-term continuous subcutaneous apomorphine infusion in advanced Parkinson’s disease with motor fluctuations: a multicenter study. Mov. Disord. 23, 1130–1136. doi: 10.1002/mds.22063
Gershanik, O., and Jenner, P. (2012). Moving from continuous dopaminergic stimulation to continuous drug delivery in the treatment of Parkinson’s disease. Eur. J. Neurol. 19, 1502–1508. doi: 10.1111/j.1468-1331.2011.03593.x
Giannicola, G., Marceglia, S., Rossi, L., Mrakic-Sposta, S., Rampini, P., Tamma, F., et al. (2010). The effects of levodopa and ongoing deep brain stimulation on subthalamic beta oscillations in Parkinson’s disease. Exp. Neurol. 226, 120–127. doi: 10.1016/j.expneurol.2010.08.011
Giladi, N., Gurevich, T., Djaldetti, R., Adar, L., Case, R., Leibman-Barak, S., et al. (2021). ND0612 (levodopa/carbidopa for subcutaneous infusion) in patients with Parkinson’s disease and motor response fluctuations: a randomized, placebo-controlled phase 2 study. Parkinsonism Relat. Disord. 91, 139–145. doi: 10.1016/j.parkreldis.2021.09.024
Giladi, N., and Nieuwboer, A. (2008). Understanding and treating freezing of gait in Parkinsonism, proposed working definition, and setting the stage. Mov. Disord. 23(Suppl. 2), S423–S425. doi: 10.1002/mds.21927
Ginis, P., Heremans, E., Ferrari, A., Bekkers, E. M. J., Canning, C. G., and Nieuwboer, A. (2017a). External input for gait in people with Parkinson’s disease with and without freezing of gait: one size does not fit all. J. Neurol. 264, 1488–1496. doi: 10.1007/s00415-017-8552-6
Ginis, P., Heremans, E., Ferrari, A., Dockx, K., Canning, C. G., and Nieuwboer, A. (2017b). Prolonged walking with a wearable system providing intelligent auditory input in people with Parkinson’s disease. Front. Neurol. 8:128. doi: 10.3389/fneur.2017.00128
Ginis, P., Nieuwboer, A., Dorfman, M., Ferrari, A., Gazit, E., Canning, C. G., et al. (2016). Feasibility and effects of home-based smartphone-delivered automated feedback training for gait in people with Parkinson’s disease: a pilot randomized controlled trial. Parkinsonism Relat. Disord. 22, 28–34. doi: 10.1016/j.parkreldis.2015.11.004
Griessenauer, C. J., Chang, S. Y., Tye, S. J., Kimble, C. J., Bennet, K. E., Garris, P. A., et al. (2010). Wireless Instantaneous Neurotransmitter Concentration System: electrochemical monitoring of serotonin using fast-scan cyclic voltammetry–a proof-of-principle study. J. Neurosurg. 113, 656–665. doi: 10.3171/2010.3.JNS091627
Hallett, M., Epstein, C. M., Berardelli, A., Sackeim, H., and Maccabee, P. (2000). Topics in transcranial magnetic stimulation. Suppl. Clin. Neurophysiol. 53, 301–311. doi: 10.1016/s1567-424x(09)70173-4
Hallett, M., Wassermann, E. M., Pascual-Leone, A., and Valls-Sole, J. (1999). Repetitive transcranial magnetic stimulation. The International Federation of Clinical Neurophysiology. Electroencephalogr. Clin. Neurophysiol. Suppl. 52, 105–113.
Hamblin, M. R. (2016). Shining light on the head: photobiomodulation for brain disorders. BBA Clin. 6, 113–124. doi: 10.1016/j.bbacli.2016.09.002
Han, J., Kim, E., Jung, J., Lee, J., Sung, H., and Kim, J. (2014). Effect of muscle vibration on spatiotemporal gait parameters in patients with Parkinson’s disease. J. Phys. Ther. Sci. 26, 671–673. doi: 10.1589/jpts.26.671
Hanajima, R., Chen, R., Ashby, P., Lozano, A. M., Hutchison, W. D., Davis, K. D., et al. (2004a). Very fast oscillations evoked by median nerve stimulation in the human thalamus and subthalamic nucleus. J. Neurophysiol. 92, 3171–3182. doi: 10.1152/jn.00363.2004
Hanajima, R., Dostrovsky, J. O., Lozano, A. M., Hutchison, W. D., Davis, K. D., Chen, R., et al. (2004b). Somatosensory evoked potentials (SEPs) recorded from deep brain stimulation (DBS) electrodes in the thalamus and subthalamic nucleus (STN). Clin. Neurophysiol. 115, 424–434. doi: 10.1016/j.clinph.2003.09.027
Harrison, E. C., Horin, A. P., and Earhart, G. M. (2018). Internal cueing improves gait more than external cueing in healthy adults and people with Parkinson disease. Sci. Rep. 8:15525. doi: 10.1038/s41598-018-33942-6
Harrison, E. C., Horin, A. P., and Earhart, G. M. (2019). Mental singing reduces gait variability more than music listening for healthy older adults and people with Parkinson disease. J. Neurol. Phys. Ther. 43, 204–211. doi: 10.1097/NPT.0000000000000288
Hass, C. J., Waddell, D. E., Fleming, R. P., Juncos, J. L., and Gregor, R. J. (2005). Gait initiation and dynamic balance control in Parkinson’s disease. Arch. Phys. Med. Rehabil. 86, 2172–2176. doi: 10.1016/j.apmr.2005.05.013
Hassan, S., Amer, S., Alwaki, A., and Elborno, A. (2013). A patient with Parkinson’s disease benefits from spinal cord stimulation. J. Clin. Neurosci. 20, 1155–1156. doi: 10.1016/j.jocn.2012.08.018
Hely, M. A., Reid, W. G., Adena, M. A., Halliday, G. M., and Morris, J. G. (2008). The Sydney multicenter study of Parkinson’s disease: the inevitability of dementia at 20 years. Mov. Disord. 23, 837–844. doi: 10.1002/mds.21956
Hendriks, J., Rosen, M., and Berube, N. and Aisen M. A. (1991). “A second-generation joystick for people disabled by tremor,” in Proceedings of the 14th Annual RESNA Conference, Kansas, MO.
Hitti, F. L., Ramayya, A. G., McShane, B. J., Yang, A. I., Vaughan, K. A., and Baltuch, G. H. (2019). Long-term outcomes following deep brain stimulation for Parkinson’s disease. J. Neurosurg. 132, 205–210. doi: 10.3171/2018.8.JNS182081
Hollingworth, M., Sims-Williams, H. P., Pickering, A. E., Barua, N., and Patel, N. K. (2017). Single electrode deep brain stimulation with dual targeting at dual frequency for the treatment of chronic pain: a case series and review of the literature. Brain Sci. 7:9. doi: 10.3390/brainsci7010009
Holloway, R. G., Shoulson, I., Fahn, S., Kieburtz, K., Lang, A., Marek, K., et al. (2004). Pramipexole vs levodopa as initial treatment for Parkinson disease: a 4-year randomized controlled trial. Arch. Neurol. 61, 1044–1053. doi: 10.1001/archneur.61.7.1044
Horiba, M., Ueki, Y., Nojima, I., Shimizu, Y., Sahashi, K., Itamoto, S., et al. (2019). Impaired motor skill acquisition using mirror visual feedback improved by transcranial direct current stimulation (tDCS) in patients with Parkinson’s disease. Front. Neurosci. 13:602. doi: 10.3389/fnins.2019.00602
Horin, A. P., Harrison, E. C., Rawson, K. S., and Earhart, G. M. (2020). People with Parkinson disease with and without freezing of gait respond similarly to external and self-generated cues. Gait Posture 82, 161–166. doi: 10.1016/j.gaitpost.2020.09.005
Hove, M. J., Suzuki, K., Uchitomi, H., Orimo, S., and Miyake, Y. (2012). Interactive rhythmic auditory stimulation reinstates natural 1/f timing in gait of Parkinson’s patients. PLoS One 7:e32600. doi: 10.1371/journal.pone.0032600
Huang, C., Chu, H., Zhang, Y., and Wang, X. (2018). Deep brain stimulation to alleviate freezing of gait and cognitive dysfunction in Parkinson’s disease: update on current research and future perspectives. Front. Neurosci. 12:29. doi: 10.3389/fnins.2018.00029
Hubsch, C., D’Hardemare, V., Ben Maacha, M., Ziegler, M., Patte-Karsenti, N., Thiebaut, J. B., et al. (2019). Tonic spinal cord stimulation as therapeutic option in Parkinson disease with axial symptoms: effects on walking and quality of life. Parkinsonism Relat. Disord. 63, 235–237. doi: 10.1016/j.parkreldis.2019.02.044
Hughes, A. J., Daniel, S. E., Blankson, S., and Lees, A. J. (1993). A clinicopathologic study of 100 cases of Parkinson’s disease. Arch. Neurol. 50, 140–148. doi: 10.1001/archneur.1993.00540020018011
Janssen, S., Bolte, B., Nonnekes, J., Bittner, M., Bloem, B. R., Heida, T., et al. (2017). Usability of three-dimensional augmented visual cues delivered by smart glasses on (Freezing of) gait in Parkinson’s disease. Front. Neurol. 8:279. doi: 10.3389/fneur.2017.00279
Jenkins, M. E., Almeida, Q. J., Spaulding, S. J., van Oostveen, R. B., Holmes, J. D., Johnson, A. M., et al. (2009). Plantar cutaneous sensory stimulation improves single-limb support time, and EMG activation patterns among individuals with Parkinson’s disease. Parkinsonism Relat. Disord. 15, 697–702. doi: 10.1016/j.parkreldis.2009.04.004
Ji, G. J., Liu, T., Li, Y., Liu, P., Sun, J., Chen, X., et al. (2021). Structural correlates underlying accelerated magnetic stimulation in Parkinson’s disease. Hum. Brain Mapp. 42, 1670–1681. doi: 10.1002/hbm.25319
Jiang, Y., and Norman, K. E. (2006). Effects of visual and auditory cues on gait initiation in people with Parkinson’s disease. Clin. Rehabil. 20, 36–45. doi: 10.1191/0269215506cr925oa
Jitkritsadakul, O., Thanawattano, C., Anan, C., and Bhidayasiri, R. (2017). Tremor’s glove-an innovative electrical muscle stimulation therapy for intractable tremor in Parkinson’s disease: a randomized sham-controlled trial. J. Neurol. Sci. 381, 331–340. doi: 10.1016/j.jns.2017.08.3246
Johnson, R. L., and Wilson, C. G. (2018). A review of vagus nerve stimulation as a therapeutic intervention. J. Inflamm. Res. 11, 203–213. doi: 10.2147/JIR.S163248
Kalia, L. V., and Lang, A. E. (2015). Parkinson’s disease. Lancet 386, 896–912. doi: 10.1016/S0140-6736(14)61393-3
Karl, J. A., Ouyang, B., Goetz, S., and Metman, L. V. (2020). A novel DBS paradigm for axial features in Parkinson’s disease: a randomized crossover study. Mov. Disord. 35, 1369–1378. doi: 10.1002/mds.28048
Kaski, D., Dominguez, R. O., Allum, J. H., Islam, A. F., and Bronstein, A. M. (2014b). Combining physical training with transcranial direct current stimulation to improve gait in Parkinson’s disease: a pilot randomized controlled study. Clin. Rehabil. 28, 1115–1124. doi: 10.1177/0269215514534277
Kaski, D., Allum, J. H., Bronstein, A. M., and Dominguez, R. O. (2014a). Applying anodal tDCS during tango dancing in a patient with Parkinson’s disease. Neurosci. Lett. 568, 39–43. doi: 10.1016/j.neulet.2014.03.043
Kerr, G. K., Worringham, C. J., Cole, M. H., Lacherez, P. F., Wood, J. M., and Silburn, P. A. (2010). Predictors of future falls in Parkinson disease. Neurology 75, 116–124. doi: 10.1212/WNL.0b013e3181e7b688
Keus, S., Munneke, M., Graziano, M., Paltamaa, J., Pelosin, E., Domingos, J., et al. (2014). On Behalf of the Guideline Development Group. 2014. European Physiotherapy Guideline for Parkinson’s Disease. Nijmegen: KNGF/ParkinsonNet.
Khedr, E. M., Al-Fawal, B., Abdel Wraith, A., Saber, M., Hasan, A. M., Bassiony, A., et al. (2019). The effect of 20 Hz versus 1 Hz repetitive transcranial magnetic stimulation on motor dysfunction in Parkinson’s disease: which is more beneficial? J. Parkinsons Dis. 9, 379–387. doi: 10.3233/JPD-181540
Khedr, E. M., Farweez, H. M., and Islam, H. (2003). Therapeutic effect of repetitive transcranial magnetic stimulation on motor function in Parkinson’s disease patients. Eur. J. Neurol. 10, 567–572. doi: 10.1046/j.1468-1331.2003.00649.x
Kilpatrick, M., Church, E., Danish, S., Stiefel, M., Jaggi, J., Halpern, C., et al. (2010). Intracerebral microdialysis during deep brain stimulation surgery. J. Neurosci. Methods 190, 106–111. doi: 10.1016/j.jneumeth.2010.04.013
Kim, J., Wichmann, T., Inan, O. T., and Deweerth, S. P. (2020). A wearable system for attenuating essential tremor based on peripheral nerve stimulation. IEEE J. Transl. Eng. Health Med. 8:2000111. doi: 10.1109/JTEHM.2020.2985058
Kim, M. S., Chang, W. H., Cho, J. W., Youn, J., Kim, Y. K., Kim, S. W., et al. (2015). Efficacy of cumulative high-frequency rTMS on freezing of gait in Parkinson’s disease. Restor. Neurol. Neurosci. 33, 521–530. doi: 10.3233/RNN-140489
Kim, R., Kim, H. J., Shin, C., Park, H., Kim, A., Paek, S. H., et al. (2019). Long-term effect of subthalamic nucleus deep brain stimulation on freezing of gait in Parkinson’s disease. J. Neurosurg. 131, 1797–1804. doi: 10.3171/2018.8.JNS18350
Kinoshita, M., Nakataki, M., Morigaki, R., Sumitani, S., Goto, S., Kaji, R., et al. (2018). Turning on the left side electrode changed depressive state to manic state in a Parkinson’s disease patient who received bilateral subthalamic nucleus deep brain stimulation: a case report. Clin. Psychopharmacol. Neurosci. 16, 494–496. doi: 10.9758/cpn.2018.16.4.494
Kishida, K. T., Saez, I., Lohrenz, T., Witcher, M. R., Laxton, A. W., Tatter, S. B., et al. (2016). Subsecond dopamine fluctuations in human striatum encode superposed error signals about actual and counterfactual reward. Proc. Natl. Acad. Sci. U.S.A. 113, 200–205. doi: 10.1073/pnas.1513619112
Kishida, K. T., Sandberg, S. G., Lohrenz, T., Comair, Y. G., Saez, I., Phillips, P. E., et al. (2011). Sub-second dopamine detection in human striatum. PLoS One 6:e23291. doi: 10.1371/journal.pone.0023291
Kleiner, A., Galli, M., Gaglione, M., Hildebrand, D., Sale, P., Albertini, G., et al. (2015). The Parkinsonian gait spatiotemporal parameters quantified by a single inertial sensor before and after automated mechanical peripheral stimulation treatment. Parkinsons Dis. 2015:390512. doi: 10.1155/2015/390512
Kleiner, A. F. R., Souza Pagnussat, A., Pinto, C., Redivo Marchese, R., Salazar, A. P., and Galli, M. (2018). Automated mechanical peripheral stimulation effects on gait variability in individuals with Parkinson disease and freezing of gait: a double-blind, randomized controlled trial. Arch. Phys. Med. Rehabil. 99, 2420–2429. doi: 10.1016/j.apmr.2018.05.009
Kleiner-Fisman, G., Herzog, J., Fisman, D. N., Tamma, F., Lyons, K. E., Pahwa, R., et al. (2006). Subthalamic nucleus deep brain stimulation: summary and meta-analysis of outcomes. Mov. Disord. 21(Suppl. 14), S290–S304. doi: 10.1002/mds.20962
Kobayashi, R., Kenji, S., Taketomi, A., Murakami, H., Ono, K., and Otake, H. (2018). New mode of burst spinal cord stimulation improved mental status as well as motor function in a patient with Parkinson’s disease. Parkinsonism Relat. Disord. 57, 82–83. doi: 10.1016/j.parkreldis.2018.07.002
Koller, W. C., and Vetere-Overfield, B. (1989). Acute and chronic effects of propranolol and primidone in essential tremor. Neurology 39, 1587–1588. doi: 10.1212/wnl.39.12.1587
Koller, W. C., Vetere-Overfield, B., and Barter, R. (1989). Tremors in early Parkinson’s disease. Clin. Neuropharmacol. 12, 293–297. doi: 10.1097/00002826-198908000-00006
Konczak, J., Corcos, D. M., Horak, F., Poizner, H., Shapiro, M., Tuite, P., et al. (2009). Proprioception and motor control in Parkinson’s disease. J. Mot. Behav. 41, 543–552. doi: 10.3200/35-09-002
Kotovsky, J., and Rosen, M. J. (1998). A wearable tremor-suppression orthosis. J. Rehabil. Res. Dev. 35, 373–387.
Krack, P., Batir, A., Van Blercom, N., Chabardes, S., Fraix, V., Ardouin, C., et al. (2003). Five-year follow-up of bilateral stimulation of the subthalamic nucleus in advanced Parkinson’s disease. N. Engl. J. Med. 349, 1925–1934. doi: 10.1056/NEJMoa035275
Kraus, T., Hosl, K., Kiess, O., Schanze, A., Kornhuber, J., and Forster, C. (2007). BOLD fMRI deactivation of limbic and temporal brain structures and mood enhancing effect by transcutaneous vagus nerve stimulation. J. Neural Transm. (Vienna) 114, 1485–1493. doi: 10.1007/s00702-007-0755-z
Kuhn, A. A., Tsui, A., Aziz, T., Ray, N., Brucke, C., Kupsch, A., et al. (2009). Pathological synchronisation in the subthalamic nucleus of patients with Parkinson’s disease relates to both bradykinesia and rigidity. Exp. Neurol. 215, 380–387. doi: 10.1016/j.expneurol.2008.11.008
Lamy, J. C., Varriale, P., Apartis, E., Mehdi, S., Blancher-Meinadier, A., Kosutzka, Z., et al. (2021). Trans-spinal direct current stimulation for managing primary orthostatic tremor. Mov. Disord. 36, 1835–1842. doi: 10.1002/mds.28581
Lance, J. W., Schwab, R. S., and Peterson, E. A. (1963). Action tremor and the cogwheel phenomenon in Parkinson’s disease. Brain 86, 95–110. doi: 10.1093/brain/86.1.95
Landi, A., Trezza, A., Pirillo, D., Vimercati, A., Antonini, A., and Sganzerla, E. P. (2013). Spinal cord stimulation for the treatment of sensory symptoms in advanced Parkinson’s disease. Neuromodulation 16, 276–279. doi: 10.1111/ner.12005
Lang, A. E., Houeto, J. L., Krack, P., Kubu, C., Lyons, K. E., Moro, E., et al. (2006). Deep brain stimulation: preoperative issues. Mov. Disord. 21(Suppl. 14), S171–S196. doi: 10.1002/mds.20955
Lang, A. E., and Lozano, A. M. (1998). Parkinson’s disease. Second of two parts. N. Engl. J. Med. 339, 1130–1143. doi: 10.1056/NEJM199810153391607
Larson, D., and Simuni, T. (2022). New dopaminergic therapies for PD motor complications. Neuropharmacology 204:108869. doi: 10.1016/j.neuropharm.2021.108869
Larson, P. S., Richardson, R. M., Starr, P. A., and Martin, A. J. (2008). Magnetic resonance imaging of implanted deep brain stimulators: experience in a large series. Stereotact. Funct. Neurosurg. 86, 92–100. doi: 10.1159/000112430
Lattari, E., Costa, S. S., Campos, C., de Oliveira, A. J., Machado, S., and Maranhao Neto, G. A. (2017). Can transcranial direct current stimulation on the dorsolateral prefrontal cortex improves balance and functional mobility in Parkinson’s disease? Neurosci. Lett. 636, 165–169. doi: 10.1016/j.neulet.2016.11.019
Lee, H. K., Ahn, S. J., Shin, Y. M., Kang, N., and Cauraugh, J. H. (2019). Does transcranial direct current stimulation improve functional locomotion in people with Parkinson’s disease? A systematic review and meta-analysis. J. Neuroeng. Rehabil. 16:84. doi: 10.1186/s12984-019-0562-4
Lee, S. A., and Kim, M. K. (2021). The effect of transcranial direct current stimulation combined with visual cueing training on motor function, balance, and gait ability of patients with Parkinson’s disease. Medicina (Kaunas) 57, 1146. doi: 10.3390/medicina57111146
Lee, S. Y., Chung, E. J., Kim, Y. J., and Kim, S. J. (2011). Dopa responsive slow orthostatic tremor in Parkinson’s disease. J. Mov. Disord. 4, 82–84. doi: 10.14802/jmd.11019
Lee, S. Y., Kim, M. S., Chang, W. H., Cho, J. W., Youn, J. Y., and Kim, Y. H. (2014). Effects of repetitive transcranial magnetic stimulation on freezing of gait in patients with Parkinsonism. Restor. Neurol. Neurosci. 32, 743–753. doi: 10.3233/RNN-140397
Lefaucheur, J. P., Drouot, X., Von Raison, F., Menard-Lefaucheur, I., Cesaro, P., and Nguyen, J. P. (2004). Improvement of motor performance and modulation of cortical excitability by repetitive transcranial magnetic stimulation of the motor cortex in Parkinson’s disease. Clin. Neurophysiol. 115, 2530–2541. doi: 10.1016/j.clinph.2004.05.025
LeMoyne, R., Mastroianni, T., Whiting, D., and Tomycz, N. (2019a). Preliminary network centric therapy for machine learning classification of deep brain stimulation status for the treatment of Parkinson’s disease with a conformal wearable and wireless inertial sensor. Adv. Parkinsons Dis. 8, 75–91. doi: 10.4236/apd.2019.84007
LeMoyne, R., Mastroianni, T., Whiting, D., and Tomycz, N. (2019b). “Wearable and wireless systems with internet connectivity for quantification of Parkinson’s disease and essential tremor characteristics,” in Wearable and Wireless Systems for Healthcare II. Smart Sensors, Measurement and Instrumentation, ed. S. C. Mukhopadhyay (Singapore: Springer), 79–97. doi: 10.1007/978-981-13-5808-1_7
LeMoyne, R., Mastroianni, T., Whiting, D., and Tomycz, N. (2020a). “Application of deep learning to distinguish multiple deep brain stimulation parameter configurations for the treatment of Parkinson’s disease,” in Proceedings of the 19th IEEE International Conference on Machine Learning and Applications (ICMLA), Miami, FL. doi: 10.1109/ICMLA51294.2020.00178
LeMoyne, R., Mastroianni, T., Whiting, D., and Tomycz, N. (2020b). Distinction of an assortment of deep brain stimulation parameter configurations for treating Parkinson’s disease using machine learning with quantification of tremor response through a conformal wearable and wireless inertial sensor. Adv. Parkinsons Dis. 9, 21–39. doi: 10.4236/apd.2020.93003
LeMoyne, R., Mastroianni, T., Whiting, D., and Tomycz, N. (2020c). Parametric evaluation of deep brain stimulation parameter configurations for Parkinson’s disease using a conformal wearable and wireless inertial sensor system and machine learning. Annu. Int. Conf. IEEE Eng. Med. Biol. Soc. 2020, 3606–3611. doi: 10.1109/EMBC44109.2020.9175408
Lewis, G. N., Byblow, W. D., and Walt, S. E. (2000). Stride length regulation in Parkinson’s disease: the use of extrinsic, visual cues. Brain 123(Pt 10), 2077–2090. doi: 10.1093/brain/123.10.2077
Lewis, S. J., Dove, A., Robbins, T. W., Barker, R. A., and Owen, A. M. (2003). Cognitive impairments in early Parkinson’s disease are accompanied by reductions in activity in frontostriatal neural circuitry. J. Neurosci. 23, 6351–6356. doi: 10.1523/JNEUROSCI.23-15-06351.2003
Li, D., Zhang, C., Gault, J., Wang, W., Liu, J., Shao, M., et al. (2017). Remotely programmed deep brain stimulation of the bilateral subthalamic nucleus for the treatment of primary parkinson disease: a randomized controlled trial investigating the safety and efficacy of a novel deep brain stimulation system. Stereotact. Funct. Neurosurg. 95, 174–182. doi: 10.1159/000475765
Li, Z. J., Wu, Q., and Yi, C. J. (2015). Clinical efficacy of istradefylline versus rTMS on Parkinson’s disease in a randomized clinical trial. Curr. Med. Res. Opin. 31, 2055–2058. doi: 10.1185/03007995.2015.1086994
Liebert, A., Bicknell, B., Laakso, E. L., Heller, G., Jalilitabaei, P., Tilley, S., et al. (2021). Improvements in clinical signs of Parkinson’s disease using photobiomodulation: a prospective proof-of-concept study. BMC Neurol. 21:256. doi: 10.1186/s12883-021-02248-y
Lim, E. C. H., Tan, T. M., and Seet, R. C. S. (2006). LASER-assisted device (LAD) for start hesitation and freezing in Parkinson’s disease. Case Rep. Clin. Pract. Rev. 7, 92–95.
Lima de Albuquerque, L., Pantovic, M., Clingo, M., Fischer, K., Jalene, S., Landers, M., et al. (2020). An acute application of cerebellar transcranial direct current stimulation does not improve motor performance in Parkinson’s disease. Brain Sci. 10:735. doi: 10.3390/brainsci10100735
Lin, P. T., Ross, E. K., Chidester, P., Rosenbluth, K. H., Hamner, S. R., Wong, S. H., et al. (2018). Noninvasive neuromodulation in essential tremor demonstrates relief in a sham-controlled pilot trial. Mov. Disord. 33, 1182–1183. doi: 10.1002/mds.27350
Lirani-Silva, E., Lord, S., Moat, D., Rochester, L., and Morris, R. (2019). Auditory cueing for gait impairment in persons with Parkinson disease: a pilot study of changes in response with disease progression. J. Neurol. Phys. Ther. 43, 50–55. doi: 10.1097/NPT.0000000000000250
Lirani-Silva, E., Vitorio, R., Barbieri, F. A., Orcioli-Silva, D., Simieli, L., and Gobbi, L. T. B. (2017). Continuous use of textured insole improve plantar sensation and stride length of people with Parkinson’s disease: a pilot study. Gait Posture 58, 495–497. doi: 10.1016/j.gaitpost.2017.09.017
Little, S., Beudel, M., Zrinzo, L., Foltynie, T., Limousin, P., Hariz, M., et al. (2016a). Bilateral adaptive deep brain stimulation is effective in Parkinson’s disease. J. Neurol. Neurosurg. Psychiatry 87, 717–721. doi: 10.1136/jnnp-2015-310972
Little, S., Tripoliti, E., Beudel, M., Pogosyan, A., Cagnan, H., Herz, D., et al. (2016b). Adaptive deep brain stimulation for Parkinson’s disease demonstrates reduced speech side effects compared to conventional stimulation in the acute setting. J. Neurol. Neurosurg. Psychiatry 87, 1388–1389. doi: 10.1136/jnnp-2016-313518
Little, S., Pogosyan, A., Neal, S., Zavala, B., Zrinzo, L., Hariz, M., et al. (2013). Adaptive deep brain stimulation in advanced Parkinson disease. Ann. Neurol. 74, 449–457. doi: 10.1002/ana.23951
Lopez, W. O., Higuera, C. A., Fonoff, E. T., Souza Cde, O., Albicker, U., and Martinez, J. A. (2014). Listenmee and Listenmee smartphone application: synchronizing walking to rhythmic auditory cues to improve gait in Parkinson’s disease. Hum. Mov. Sci. 37, 147–156. doi: 10.1016/j.humov.2014.08.001
Ma, J., Gao, L., Mi, T., Sun, J., Chan, P., and Wu, T. (2019). Repetitive transcranial magnetic stimulation does not improve the sequence effect in freezing of gait. Parkinsons Dis. 2019:2196195. doi: 10.1155/2019/2196195
Ma, Y., Miao, S., Zhou, R., Zhang, Q., Chen, H., and Liang, Y. (2021). Application of remote deep brain stimulation programming for Parkinson’s disease patients. World Neurosurg. 147, e255–e261. doi: 10.1016/j.wneu.2020.12.030
Machado, A., Rezai, A. R., Kopell, B. H., Gross, R. E., Sharan, A. D., and Benabid, A. L. (2006). Deep brain stimulation for Parkinson’s disease: surgical technique and perioperative management. Mov. Disord. 21(Suppl. 14), S247–S258. doi: 10.1002/mds.20959
Majoie, H. J., Rijkers, K., Berfelo, M. W., Hulsman, J. A., Myint, A., Schwarz, M., et al. (2011). Vagus nerve stimulation in refractory epilepsy: effects on pro- and anti-inflammatory cytokines in peripheral blood. Neuroimmunomodulation 18, 52–56. doi: 10.1159/000315530
Mann, G. E., Finn, S. M., and Taylor, P. N. (2008). A pilot study to investigate the feasibility of electrical stimulation to assist gait in Parkinson’s disease. Neuromodulation 11, 143–149. doi: 10.1111/j.1525-1403.2008.00157.x
Manor, B., Dagan, M., Herman, T., Gouskova, N. A., Vanderhorst, V. G., Giladi, N., et al. (2021). Multitarget transcranial electrical stimulation for freezing of gait: a randomized controlled trial. Mov. Disord. 36, 2693–2698. doi: 10.1002/mds.28759
Marceglia, S., Fiorio, M., Foffani, G., Mrakic-Sposta, S., Tiriticco, M., Locatelli, M., et al. (2009). Modulation of beta oscillations in the subthalamic area during action observation in Parkinson’s disease. Neuroscience 161, 1027–1036. doi: 10.1016/j.neuroscience.2009.04.018
Martin, W. E., Loewenson, R. B., Resch, J. A., and Baker, A. B. (1973). Parkinson’s disease. Clinical analysis of 100 patients. Neurology 23, 783–790. doi: 10.1212/wnl.23.8.783
Maruo, T., Hosomi, K., Shimokawa, T., Kishima, H., Oshino, S., Morris, S., et al. (2013). High-frequency repetitive transcranial magnetic stimulation over the primary foot motor area in Parkinson’s disease. Brain Stimul. 6, 884–891. doi: 10.1016/j.brs.2013.05.002
Mazzone, P., Viselli, F., Ferraina, S., Giamundo, M., Marano, M., Paoloni, M., et al. (2019). High cervical spinal cord stimulation: a one year follow-up study on motor and non-motor functions in Parkinson’s disease. Brain Sci. 9:78. doi: 10.3390/brainsci9040078
McElcheran, C. E., Golestanirad, L., Iacono, M. I., Wei, P. S., Yang, B., Anderson, K. J. T., et al. (2019). Numerical simulations of realistic lead trajectories and an experimental verification support the efficacy of parallel radiofrequency transmission to reduce heating of deep brain stimulation implants during MRI. Sci. Rep. 9:2124. doi: 10.1038/s41598-018-38099-w
Merola, A., Espay, A. J., Romagnolo, A., Bernardini, A., Rizzi, L., Rosso, M., et al. (2016). Advanced therapies in Parkinson’s disease: long-term retrospective study. Parkinsonism Relat. Disord. 29, 104–108. doi: 10.1016/j.parkreldis.2016.05.015
Mi, T. M., Garg, S., Ba, F., Liu, A. P., Liang, P. P., Gao, L. L., et al. (2020). Repetitive transcranial magnetic stimulation improves Parkinson’s freezing of gait via normalizing brain connectivity. NPJ Parkinsons Dis. 6:16. doi: 10.1038/s41531-020-0118-0
Mi, T. M., Garg, S., Ba, F., Liu, A. P., Wu, T., Gao, L. L., et al. (2019). High-frequency rTMS over the supplementary motor area improves freezing of gait in Parkinson’s disease: a randomized controlled trial. Parkinsonism Relat. Disord. 68, 85–90. doi: 10.1016/j.parkreldis.2019.10.009
Mille, M. L., Creath, R. A., Prettyman, M. G., Johnson Hilliard, M., Martinez, K. M., Mackinnon, C. D., et al. (2012). Posture and locomotion coupling: a target for rehabilitation interventions in persons with Parkinson’s disease. Parkinsons Dis. 2012:754186. doi: 10.1155/2012/754186
Mink, J. W. (1996). The basal ganglia: focused selection and inhibition of competing motor programs. Prog. Neurobiol. 50, 381–425. doi: 10.1016/s0301-0082(96)00042-1
Mishra, R. K., and Thrasher, A. T. (2021). Transcranial direct current stimulation of dorsolateral prefrontal cortex improves dual-task gait performance in patients with Parkinson’s disease: a double blind, sham-controlled study. Gait Posture 84, 11–16. doi: 10.1016/j.gaitpost.2020.11.012
Mondal, B., Choudhury, S., Banerjee, R., Roy, A., Chatterjee, K., Basu, P., et al. (2021). Non-invasive vagus nerve stimulation improves clinical and molecular biomarkers of Parkinson’s disease in patients with freezing of gait. NPJ Parkinsons Dis. 7:46. doi: 10.1038/s41531-021-00190-x
Mondal, B., Choudhury, S., Simon, B., Baker, M. R., and Kumar, H. (2019). Noninvasive vagus nerve stimulation improves gait and reduces freezing of gait in Parkinson’s disease. Mov. Disord. 34, 917–918. doi: 10.1002/mds.27662
Moore, O., Peretz, C., and Giladi, N. (2007). Freezing of gait affects quality of life of peoples with Parkinson’s disease beyond its relationships with mobility and gait. Mov. Disord. 22, 2192–2195. doi: 10.1002/mds.21659
Morigaki, R., and Goto, S. (2015). “Deep brain stimulation for essential tremor,” in Deep Brain Stimulation for Neurological Disorders, ed. T. Itakura (Cham: Springer), 135–155. doi: 10.1007/978-3-319-08476-3_11
Morigaki, R., Nagahiro, S., Kaji, R., and Goto, S. (2011a). “Current use of thalamic surgeries for treating movement disorders,” in Thalamus: Anatomy, Functions and Disorders, ed. J. L. Song (New York, NY: Nova Science Publishers, Inc), 1–31.
Morigaki, R., Nagahiro, S., Kaji, R., and Goto, S. (2011b). “Thalamic deep brain stimulation for Parkinson’s disease,” in Diagnosis and Treatment of Parkinson’s Disease, ed. A. Q. Rana (London: InTech), 233–252. doi: 10.1007/s007010100024
Morris, R., Yarnall, A. J., Hunter, H., Taylor, J. P., Baker, M. R., and Rochester, L. (2019). Noninvasive vagus nerve stimulation to target gait impairment in Parkinson’s disease. Mov. Disord. 34, 918–919. doi: 10.1002/mds.27664
Muller, E. J., and Robinson, P. A. (2018). Quantitative theory of deep brain stimulation of the subthalamic nucleus for the suppression of pathological rhythms in Parkinson’s disease. PLoS Comput. Biol. 14:e1006217. doi: 10.1371/journal.pcbi.1006217
Nascimento, L. R., do Carmo, W. A., de Oliveira, G. P., Arêas, F. Z. D. S., and Dias, F. M. V. (2021). Transcranial direct current stimulation provides no clinically important benefits over walking training for improving walking in Parkinson’s disease: a systematic review. J. Physiother. 67, 190–196. doi: 10.1016/j.jphys.2021.06.003
Neudorfer, C., Kroneberg, D., Al-Fatly, B., Goede, L., Kubler, D., Faust, K., et al. (2022). Personalizing deep brain stimulation using advanced imaging sequences. Ann. Neurol. 1–15. doi: 10.1002/ana.26326
Neumann, W. J., Degen, K., Schneider, G. H., Brucke, C., Huebl, J., Brown, P., et al. (2016). Subthalamic synchronized oscillatory activity correlates with motor impairment in patients with Parkinson’s disease. Mov. Disord. 31, 1748–1751. doi: 10.1002/mds.26759
Neumann, W. J., Staub-Bartelt, F., Horn, A., Schanda, J., Schneider, G. H., Brown, P., et al. (2017). Long term correlation of subthalamic beta band activity with motor impairment in patients with Parkinson’s disease. Clin. Neurophysiol. 128, 2286–2291. doi: 10.1016/j.clinph.2017.08.028
Nieuwboer, A., Rochester, L., Muncks, L., and Swinnen, S. P. (2009). Motor learning in Parkinson’s disease: limitations and potential for rehabilitation. Parkinsonism Relat. Disord. 15(Suppl. 3), S53–S58. doi: 10.1016/S1353-8020(09)70781-3
Nilsson, D., Hansson, L. E., Johansson, K., Nystrom, C., Paalzow, L., and Aquilonius, S. M. (1998). Long-term intraduodenal infusion of a water based levodopa-carbidopa dispersion in very advanced Parkinson’s disease. Acta Neurol. Scand. 97, 175–183. doi: 10.1111/j.1600-0404.1998.tb00633.x
Nishioka, K., and Nakajima, M. (2015). Beneficial Therapeutic effects of spinal cord stimulation in advanced cases of Parkinson’s disease with intractable chronic pain: a case series. Neuromodulation 18, 751–753. doi: 10.1111/ner.12315
Nissen, M., Ikaheimo, T. M., Huttunen, J., Leinonen, V., and von Und Zu Fraunberg, M. (2019). Long-Term outcome of spinal cord stimulation in failed back surgery syndrome: 20 years of experience with 224 consecutive patients. Neurosurgery 84, 1011–1018. doi: 10.1093/neuros/nyy194
Nitsche, M. A., and Paulus, W. (2000). Excitability changes induced in the human motor cortex by weak transcranial direct current stimulation. J. Physiol. 527(Pt 3), 633–639. doi: 10.1111/j.1469-7793.2000.t01-1-00633.x
Nolano, M., Provitera, V., Estraneo, A., Selim, M. M., Caporaso, G., Stancanelli, A., et al. (2008). Sensory deficit in Parkinson’s disease: evidence of a cutaneous denervation. Brain 131(Pt 7), 1903–1911. doi: 10.1093/brain/awn102
Novak, P., and Novak, V. (2006). Effect of step-synchronized vibration stimulation of soles on gait in Parkinson’s disease: a pilot study. J. Neuroeng. Rehabil. 3:9. doi: 10.1186/1743-0003-3-9
Nyholm, D. (2012). Duodopa(R) treatment for advanced Parkinson’s disease: a review of efficacy and safety. Parkinsonism Relat. Disord. 18, 916–929. doi: 10.1016/j.parkreldis.2012.06.022
Nyholm, D., and Lennernas, H. (2008). Irregular gastrointestinal drug absorption in Parkinson’s disease. Expert Opin. Drug Metab. Toxicol. 4, 193–203. doi: 10.1517/17425255.4.2.193
Nyholm, D., Nilsson Remahl, A. I., Dizdar, N., Constantinescu, R., Holmberg, B., Jansson, R., et al. (2005). Duodenal levodopa infusion monotherapy vs oral polypharmacy in advanced Parkinson disease. Neurology 64, 216–223. doi: 10.1212/01.WNL.0000149637.70961.4C
Obeso, J. A., Stamelou, M., Goetz, C. G., Poewe, W., Lang, A. E., Weintraub, D., et al. (2017). Past, present, and future of Parkinson’s disease: a special essay on the 200th Anniversary of the Shaking Palsy. Mov. Disord. 32, 1264–1310. doi: 10.1002/mds.27115
Okada, K. I., Takahira, M., Mano, T., Uga, T., Konaka, K., Hosomi, K., et al. (2021). Concomitant improvement in anti-saccade success rate and postural instability gait difficulty after rTMS treatment for Parkinson’s disease. Sci. Rep. 11:2472. doi: 10.1038/s41598-021-81795-3
Olanow, C. W., Espay, A. J., Stocchi, F., Ellenbogen, A. L., Leinonen, M., Adar, L., et al. (2021). Continuous subcutaneous levodopa delivery for Parkinson’s disease: a randomized study. J. Parkinsons Dis. 11, 177–186. doi: 10.3233/JPD-202285
Olanow, C. W., Factor, S. A., Espay, A. J., Hauser, R. A., Shill, H. A., Isaacson, S., et al. (2020). Apomorphine sublingual film for off episodes in Parkinson’s disease: a randomised, double-blind, placebo-controlled phase 3 study. Lancet Neurol. 19, 135–144. doi: 10.1016/S1474-4422(19)30396-5
Olanow, C. W., Kieburtz, K., Odin, P., Espay, A. J., Standaert, D. G., Fernandez, H. H., et al. (2014). Continuous intrajejunal infusion of levodopa-carbidopa intestinal gel for patients with advanced Parkinson’s disease: a randomised, controlled, double-blind, double-dummy study. Lancet Neurol. 13, 141–149. doi: 10.1016/S1474-4422(13)70293-X
Ono, S. A., Sato, T., and Muramatsu, S. (2016). Freezing of gait in Parkinson’s disease is associated with reduced 6-[(18)F]Fluoro-l-m-tyrosine uptake in the locus coeruleus. Parkinsons Dis. 2016:5430920. doi: 10.1155/2016/5430920
Oshinsky, M. L., Murphy, A. L., Hekierski, H. Jr., Cooper, M., and Simon, B. J. (2014). Noninvasive vagus nerve stimulation as treatment for trigeminal allodynia. Pain 155, 1037–1042. doi: 10.1016/j.pain.2014.02.009
Pagnussat, A. S., Kleiner, A. F. R., Rieder, C. R. M., Frantz, A., Ehlers, J., Pinto, C., et al. (2018). Plantar stimulation in parkinsonians: from biomarkers to mobility – randomized-controlled trial. Restor. Neurol. Neurosci. 36, 195–205. doi: 10.3233/RNN-170744
Pagnussat, A. S., Salazar, A. P., Pinto, C., Redivo Marchese, R., Rieder, C. R. M., Alves Filho, J. O., et al. (2020). Plantar stimulation alters brain connectivity in idiopathic Parkinson’s disease. Acta Neurol. Scand. 142, 229–238. doi: 10.1111/ane.13253
Pahwa, R., Lyons, K. E., Wilkinson, S. B., Troster, A. I., Overman, J., Kieltyka, J., et al. (2001). Comparison of thalamotomy to deep brain stimulation of the thalamus in essential tremor. Mov. Disord. 16, 140–143. doi: 10.1002/1531-8257(200101)16:1<140::aid-mds1025<3.0.co;2-t
Pal, P. K., Samii, A., and Calne, D. B. (2002). “Cardinal features of early Parkinson’s disease,” in Parkinson’s Disease Diagnosis and Clinical Management, eds S. Factor and W. J. Weiner (New York, NY: Demos Medical Publishing, Inc), 41–56.
Pascual-Leone, A., Valls-Sole, J., Wassermann, E. M., and Hallett, M. (1994). Responses to rapid-rate transcranial magnetic stimulation of the human motor cortex. Brain 117(Pt 4), 847–858. doi: 10.1093/brain/117.4.847
Peppe, A., Paravati, S., Baldassarre, M. G., Bakdounes, L., Spolaor, F., Guiotto, A., et al. (2019). Proprioceptive focal stimulation (Equistasi®) may improve the quality of gait in middle-moderate Parkinson’s disease patients. Double-Blind, double-dummy, randomized, crossover, Italian multicentric study. Front. Neurol. 10:998. doi: 10.3389/fneur.2019.00998
Pereira, M. P., Gobbi, L. T., and Almeida, Q. J. (2016). Freezing of gait in Parkinson’s disease: evidence of sensory rather than attentional mechanisms through muscle vibration. Parkinsonism Relat. Disord. 29, 78–82. doi: 10.1016/j.parkreldis.2016.05.021
Perez-Lloret, S., Negre-Pages, L., Damier, P., Delval, A., Derkinderen, P., Destee, A., et al. (2014). Prevalence, determinants, and effect on quality of life of freezing of gait in Parkinson disease. JAMA Neurol. 71, 884–890. doi: 10.1001/jamaneurol.2014.753
Pessiglione, M., Guehl, D., Rolland, A. S., Francois, C., Hirsch, E. C., Feger, J., et al. (2005). Thalamic neuronal activity in dopamine-depleted primates: evidence for a loss of functional segregation within basal ganglia circuits. J. Neurosci. 25, 1523–1531. doi: 10.1523/JNEUROSCI.4056-04.2005
Peterson, D. S., Pickett, K. A., Duncan, R., Perlmutter, J., and Earhart, G. M. (2014). Gait-related brain activity in people with Parkinson disease with freezing of gait. PLoS One 9:e90634. doi: 10.1371/journal.pone.0090634
Piasecki, S. D., and Jefferson, J. W. (2004). Psychiatric complications of deep brain stimulation for Parkinson’s disease. J. Clin. Psychiatry 65, 845–849. doi: 10.4088/jcp.v65n0617
Pinto, C., Pagnussat, A. S., Rozin Kleiner, A. F., Marchese, R. R., Salazar, A. P., Rieder, C. R. M., et al. (2018). Automated mechanical peripheral stimulation improves gait parameters in subjects with Parkinson disease and freezing of gait: a randomized clinical trial. Am. J. Phys. Med. Rehabil. 97, 383–389. doi: 10.1097/PHM.0000000000000890
Pinto de Souza, C., Hamani, C., Oliveira Souza, C., Lopez Contreras, W. O., Dos Santos Ghilardi, M. G., Cury, R. G., et al. (2017). Spinal cord stimulation improves gait in patients with Parkinson’s disease previously treated with deep brain stimulation. Mov. Disord. 32, 278–282. doi: 10.1002/mds.26850
Poewe, W., and Mahlknecht, P. (2020). Pharmacologic treatment of motor symptoms associated with Parkinson disease. Neurol. Clin. 38, 255–267. doi: 10.1016/j.ncl.2019.12.002
Poewe, W., Stocchi, F., Arkadir, D., Ebersbach, G., Ellenbogen, A. L., Giladi, N., et al. (2021). Subcutaneous levodopa infusion for Parkinson’s disease: 1-year data from the open-label BeyoND study. Mov. Disord. 36, 2687–2692. doi: 10.1002/mds.28758
Politis, M., Sauerbier, A., Loane, C., Pavese, N., Martin, A., Corcoran, B., et al. (2017). Sustained striatal dopamine levels following intestinal levodopa infusions in Parkinson’s disease patients. Mov. Disord. 32, 235–240. doi: 10.1002/mds.26848
Popovic Maneski, L., Jorgovanovic, N., Ilic, V., Dosen, S., Keller, T., Popovic, M. B., et al. (2011). Electrical stimulation for the suppression of pathological tremor. Med. Biol. Eng. Comput. 49, 1187–1193. doi: 10.1007/s11517-011-0803-6
Prasad, S., Aguirre-Padilla, D. H., Poon, Y. Y., Kalsi-Ryan, S., Lozano, A. M., and Fasano, A. (2020). Spinal cord stimulation for very advanced Parkinson’s disease: a 1-year prospective trial. Mov. Disord. 35, 1082–1083. doi: 10.1002/mds.28065
Pratorius, B., Kimmeskamp, S., and Milani, T. L. (2003). The sensitivity of the sole of the foot in patients with Morbus Parkinson. Neurosci. Lett. 346, 173–176. doi: 10.1016/s0304-3940(03)00582-2
Prusch, J. S., Kleiner, A. F. R., Salazar, A. P., Pinto, C., Marchese, R. R., Galli, M., et al. (2018). Automated mechanical peripheral stimulation and postural control in subjects with Parkinson’s disease and freezing of gait: a randomized controlled trial. Funct. Neurol. 33, 206–212.
Putzolu, M., Pelosin, E., Ogliastro, C., Lagravinese, G., Bonassi, G., Ravaschio, A., et al. (2018). Anodal tDCS over prefrontal cortex improves dual-task walking in Parkinsonian patients with freezing. Mov. Disord. 33, 1972–1973. doi: 10.1002/mds.27533
Qiu, F., Cole, M. H., Davids, K. W., Hennig, E. M., Silburn, P. A., Netscher, H., et al. (2013). Effects of textured insoles on balance in people with Parkinson’s disease. PLoS One 8:e83309. doi: 10.1371/journal.pone.0083309
Quattrocchi, C. C., de Pandis, M. F., Piervincenzi, C., Galli, M., Melgari, J. M., Salomone, G., et al. (2015). Acute modulation of brain connectivity in Parkinson Disease after automatic mechanical peripheral stimulation: a pilot study. PLoS One 10:e0137977. doi: 10.1371/journal.pone.0137977
Rabin, E., Chen, J., Muratori, L., DiFrancisco-Donoghue, J., and Werner, W. G. (2013). Haptic feedback from manual contact improves balance control in people with Parkinson’s disease. Gait Posture 38, 373–379. doi: 10.1016/j.gaitpost.2012.12.008
Rahimpour, S., Gaztanaga, W., Yadav, A. P., Chang, S. J., Krucoff, M. O., Cajigas, I., et al. (2021). Freezing of gait in Parkinson’s disease: invasive and noninvasive neuromodulation. Neuromodulation 24, 829–842. doi: 10.1111/ner.13347
Ramot, Y., Nyska, A., Adar, L., Durlach, C., Fishelovitch, D., Sacco, G., et al. (2018). ND0701, a novel formulation of apomorphine for subcutaneous infusion, in comparison to a commercial apomorphine formulation: 28-day pharmacokinetic study in minipigs and a phase I Study in healthy volunteers to assess the safety, tolerability, pharmacokinetics and relative bioavailability. CNS Drugs 32, 443–454. doi: 10.1007/s40263-018-0512-x
Reis, C., Arruda, B. S., Pogosyan, A., Brown, P., and Cagnan, H. (2021). Essential tremor amplitude modulation by median nerve stimulation. Sci. Rep. 11:17720. doi: 10.1038/s41598-021-96660-6
Riancho-Zarrabeitia, L., and Delgado-Alvarado, M. (2017). Bone health in Parkinson’s disease. Clin. Rev. Bone Miner. Metab. 15, 99–114. doi: 10.1007/s12018-017-9232-x
Rosenkranz, K., and Rothwell, J. C. (2003). Differential effect of muscle vibration on intracortical inhibitory circuits in humans. J. Physiol. 551(Pt 2), 649–660. doi: 10.1113/jphysiol.2003.043752
Rosenthal, L., Sweeney, D., Cunnington, A. L., Quinlan, L. R., and ÓLaighin, G. (2018). Sensory electrical stimulation cueing may reduce freezing of gait episodes in Parkinson’s disease. J. Healthc. Eng. 2018:4684925. doi: 10.1155/2018/4684925
Sabari, J., Stefanov, D. G., Chan, J., Goed, L., and Starr, J. (2019). Adapted feeding utensils for people with Parkinson’s-related or essential tremor. Am J Occup Ther 73, 7302205120p1–7302205120p9. doi: 10.5014/ajot.2019.030759
Sage, J. I., Trooskin, S., Sonsalla, P. K., Heikkila, R., and Duvoisin, R. C. (1988). Long-term duodenal infusion of levodopa for motor fluctuations in parkinsonism. Ann. Neurol. 24, 87–89. doi: 10.1002/ana.410240116
Sako, W., Miyazaki, Y., Izumi, Y., and Kaji, R. (2014). Which target is best for patients with Parkinson’s disease? A meta-analysis of pallidal and subthalamic stimulation. J. Neurol. Neurosurg. Psychiatry 85, 982–986. doi: 10.1136/jnnp-2013-306090
Sako, W., Nishio, M., Maruo, T., Shimazu, H., Matsuzaki, K., Tamura, T., et al. (2009). Subthalamic nucleus deep brain stimulation for camptocormia associated with Parkinson’s disease. Mov. Disord. 24, 1076–1079. doi: 10.1002/mds.22529
Samotus, O., Parrent, A., and Jog, M. (2018). Spinal cord stimulation therapy for gait dysfunction in advanced Parkinson’s disease patients. Mov. Disord. 33, 783–792. doi: 10.1002/mds.27299
Santana, M. B., Halje, P., Simplicio, H., Richter, U., Freire, M. A. M., Petersson, P., et al. (2014). Spinal cord stimulation alleviates motor deficits in a primate model of Parkinson disease. Neuron 84, 716–722. doi: 10.1016/j.neuron.2014.08.061
Santos, L., Olmo-Aguado, S. D., Valenzuela, P. L., Winge, K., Iglesias-Soler, E., Arguelles-Luis, J., et al. (2019). Photobiomodulation in Parkinson’s disease: a randomized controlled trial. Brain Stimul. 12, 810–812. doi: 10.1016/j.brs.2019.02.009
Sasaki, F., Oyama, G., Sekimoto, S., Nuermaimaiti, M., Iwamuro, H., Shimo, Y., et al. (2021). Closed-loop programming using external responses for deep brain stimulation in Parkinson’s disease. Parkinsonism Relat. Disord. 84, 47–51. doi: 10.1016/j.parkreldis.2021.01.023
Sayin, S., Cakmur, R., Yener, G. G., Yaka, E., Ugurel, B., and Uzunel, F. (2014). Low-frequency repetitive transcranial magnetic stimulation for dyskinesia and motor performance in Parkinson’s disease. J. Clin. Neurosci. 21, 1373–1376. doi: 10.1016/j.jocn.2013.11.025
Schabrun, S. M., Lamont, R. M., and Brauer, S. G. (2016). Transcranial direct current stimulation to enhance dual-task gait training in Parkinson’s disease: a pilot RCT. PLoS One 11:e0158497. doi: 10.1371/journal.pone.0158497
Schlick, C., Ernst, A., Botzel, K., Plate, A., Pelykh, O., and Ilmberger, J. (2016). Visual cues combined with treadmill training to improve gait performance in Parkinson’s disease: a pilot randomized controlled trial. Clin. Rehabil. 30, 463–471. doi: 10.1177/0269215515588836
Schoellmann, A., Scholten, M., Wasserka, B., Govindan, R. B., Kruger, R., Gharabaghi, A., et al. (2019). Anodal tDCS modulates cortical activity and synchronization in Parkinson’s disease depending on motor processing. Neuroimage Clin. 22:101689. doi: 10.1016/j.nicl.2019.101689
Schupbach, W. M. M., Chabardes, S., Matthies, C., Pollo, C., Steigerwald, F., Timmermann, L., et al. (2017). Directional leads for deep brain stimulation: opportunities and challenges. Mov. Disord. 32, 1371–1375. doi: 10.1002/mds.27096
Serio, F., Minosa, C., De Luca, M., Conte, P., Albani, G., and Peppe, A. (2019). Focal vibration training (Equistasi§) to improve posture stability. a retrospective study in Parkinson’s disease. Sensors (Basel) 19:2101. doi: 10.3390/s19092101
Shanker, V. (2019). Essential tremor: diagnosis and management. BMJ 366, l4485. doi: 10.1136/bmj.l4485
Shima, K., Shimatani, K., and Sakata, M. (2021). A wearable light-touch contact device for human balance support. Sci. Rep. 11:7324. doi: 10.1038/s41598-021-85687-4
Shine, J. M., Matar, E., Ward, P. B., Bolitho, S. J., Pearson, M., Naismith, S. L., et al. (2013). Differential neural activation patterns in patients with Parkinson’s disease and freezing of gait in response to concurrent cognitive and motor load. PLoS One 8:e52602. doi: 10.1371/journal.pone.0052602
Shirota, Y., Ohtsu, H., Hamada, M., Enomoto, H., Ugawa, Y., and Research Committee on rTMS Treatment of Parkinson’s Disease (2013). Supplementary motor area stimulation for Parkinson disease: a randomized controlled study. Neurology 80, 1400–1405. doi: 10.1212/WNL.0b013e31828c2f66
Shon, Y. M., Chang, S. Y., Tye, S. J., Kimble, C. J., Bennet, K. E., Blaha, C. D., et al. (2010). Comonitoring of adenosine and dopamine using the wireless instantaneous neurotransmitter concentration system: proof of principle. J. Neurosurg. 112, 539–548. doi: 10.3171/2009.7.JNS09787
Slevin, J. T., Fernandez, H. H., Zadikoff, C., Hall, C., Eaton, S., Dubow, J., et al. (2015). Long-term safety and maintenance of efficacy of levodopa-carbidopa intestinal gel: an open-label extension of the double-blind pivotal study in advanced Parkinson’s disease patients. J. Parkinsons Dis. 5, 165–174. doi: 10.3233/JPD-140456
Snijders, A. H., Leunissen, I., Bakker, M., Overeem, S., Helmich, R. C., Bloem, B. R., et al. (2011). Gait-related cerebral alterations in patients with Parkinson’s disease with freezing of gait. Brain 134(Pt 1), 59–72. doi: 10.1093/brain/awq324
Sorensen, K. L., Hollands, M. A., and Patla, E. (2002). The effects of human ankle muscle vibration on posture and balance during adaptive locomotion. Exp. Brain Res. 143, 24–34. doi: 10.1007/s00221-001-0962-z
Spagnolo, F., Fichera, M., Chieffo, R., Dalla Costa, G., Pisa, M., Volonte, M. A., et al. (2020). Bilateral repetitive transcranial magnetic stimulation with the H-Coil in Parkinson’s disease: a randomized, sham-controlled study. Front. Neurol. 11:584713. doi: 10.3389/fneur.2020.584713
Spildooren, J., Vercruysse, S., Heremans, E., Galna, B., Verheyden, G., Vervoort, G., et al. (2017). Influence of cueing and an attentional strategy on freezing of gait in parkinson disease during turning. J. Neurol. Phys. Ther. 41, 129–135. doi: 10.1097/NPT.0000000000000178
Spildooren, J., Vercruysse, S., Meyns, P., Vandenbossche, J., Heremans, E., Desloovere, K., et al. (2012). Turning and unilateral cueing in Parkinson’s disease patients with and without freezing of gait. Neuroscience 207, 298–306. doi: 10.1016/j.neuroscience.2012.01.024
Spolaor, F., Romanato, M., Annamaria, G., Peppe, A., Bakdounes, L., To, D. K., et al. (2021). Relationship between muscular activity and postural control changes after proprioceptive focal stimulation (Equistasi§) in middle-moderate Parkinson’s disease patients: an explorative study. Sensors (Basel) 21:560. doi: 10.3390/s21020560
Stocchi, F., Sale, P., Kleiner, A. F., Casali, M., Cimolin, V., de Pandis, F., et al. (2015). Long-term effects of automated mechanical peripheral stimulation on gait patterns of patients with Parkinson’s disease. Int. J. Rehabil. Res. 38, 238–245. doi: 10.1097/MRR.0000000000000120
Swank, C., Mehta, J., and Criminger, C. (2016). Transcranial direct current stimulation lessens dual task cost in people with Parkinson’s disease. Neurosci. Lett. 626, 1–5. doi: 10.1016/j.neulet.2016.05.010
Syrkin-Nikolau, J., Neuville, R., O’Day, J., Anidi, C., Miller Koop, M., Martin, T., et al. (2018). Coordinated reset vibrotactile stimulation shows prolonged improvement in Parkinson’s disease. Mov. Disord. 33, 179–180. doi: 10.1002/mds.27223
Takanokura, M., Sugahara, R., Miyake, N., Ishiguro, K., Muto, T., and Sakamoto, K. (2011). “Upper-limb orthoses implemented with airdashpot for suppression of a pathological tremor in daily activities,” in Proceedings of the ISB Conference, Brussels.
Tan, T., Almeida, Q. J., and Rahimi, F. (2011). Proprioceptive deficits in Parkinson’s disease patients with freezing of gait. Neuroscience 192, 746–752. doi: 10.1016/j.neuroscience.2011.06.071
Tan, X. S., Pierres, F., Dallman-Porter, A., Hardie-Brown, W., and Kwon, K. Y. (2021). Focused vibrotactile stimulation with cueing effect on freezing of gait in Parkinson’s disease: two case reports. J. Mov. Disord. 14, 236–238. doi: 10.14802/jmd.21076
Tanaka, T., Takano, Y., Tanaka, S., Hironaka, N., Kobayashi, K., Hanakawa, T., et al. (2013). Transcranial direct-current stimulation increases extracellular dopamine levels in the rat striatum. Front. Syst. Neurosci. 7:6. doi: 10.3389/fnsys.2013.00006
Tang, L., Xu, W., Li, Z., Chen, Y., Chen, H., Yu, R., et al. (2019). Quantitative gait analysis for laser cue in Parkinson’s disease patients with freezing of gait. Ann. Transl. Med. 7:324. doi: 10.21037/atm.2019.05.87
Tasker, R. R. (1998). Deep brain stimulation is preferable to thalamotomy for tremor suppression. Surg. Neurol. 49, 145–53; discussion153–4. doi: 10.1016/s0090-3019(97)00459-x
Taylor, P. N., Sampson, T., Beare, B., Donavon-Hall, M., Thomas, P. W., Marques, E., et al. (2021). The effectiveness of peroneal nerve functional electrical simulation for the reduction of bradykinesia in Parkinson’s disease: a feasibility study for a randomised control trial. Clin. Rehabil. 35, 546–557. doi: 10.1177/0269215520972519
Terao, Y., Fukuda, H., Ugawa, Y., and Hikosaka, O. (2013). New perspectives on the pathophysiology of Parkinson’s disease as assessed by saccade performance: a clinical review. Clin. Neurophysiol. 124, 1491–1506. doi: 10.1016/j.clinph.2013.01.021
Terry, R. S. Jr. (2014). “Vagus nerve stimulation therapy for epilepsy,” in Epilepsy Topics, ed. M. D. Holms (London: InTech), 139–160. doi: 10.5772/58332
Thevathasan, W., Mazzone, P., Jha, A., Djamshidian, A., Dileone, M., Di Lazzaro, V., et al. (2010). Spinal cord stimulation failed to relieve akinesia or restore locomotion in Parkinson disease. Neurology 74, 1325–1327. doi: 10.1212/WNL.0b013e3181d9ed58
Titova, N., and Chaudhuri, K. R. (2016). Apomorphine therapy in Parkinson’s and future directions. Parkinsonism Relat. Disord. 33(Suppl. 1), S56–S60. doi: 10.1016/j.parkreldis.2016.11.013
Valentino, F., Cosentino, G., Brighina, F., Pozzi, N. G., Sandrini, G., Fierro, B., et al. (2014). Transcranial direct current stimulation for treatment of freezing of gait: a cross-over study. Mov. Disord. 29, 1064–1069. doi: 10.1002/mds.25897
van Laar, T. (2003). Levodopa-induced response fluctuations in patients with Parkinson’s disease: strategies for management. CNS Drugs 17, 475–489. doi: 10.2165/00023210-200317070-00002
Vercruysse, S., Gilat, M., Shine, J. M., Heremans, E., Lewis, S., and Nieuwboer, A. (2014). Freezing beyond gait in Parkinson’s disease: a review of current neurobehavioral evidence. Neurosci. Biobehav. Rev. 43, 213–227. doi: 10.1016/j.neubiorev.2014.04.010
Vercruysse, S., Spildooren, J., Heremans, E., Vandenbossche, J., Wenderoth, N., Swinnen, S. P., et al. (2012). Abnormalities and cue dependence of rhythmical upper-limb movements in Parkinson patients with freezing of gait. Neurorehabil. Neural Repair. 26, 636–645. doi: 10.1177/1545968311431964
Volpe, D., Giantin, M. G., and Fasano, A. (2014). A wearable proprioceptive stabilizer (Equistasi§) for rehabilitation of postural instability in Parkinson’s disease: a phase II randomized double-blind, double-dummy, controlled study. PLoS One 9:e112065. doi: 10.1371/journal.pone.0112065
Walton, C. C., Shine, J. M., Hall, J. M., O’Callaghan, C., Mowszowski, L., Gilat, M., et al. (2015). The major impact of freezing of gait on quality of life in Parkinson’s disease. J. Neurol. 262, 108–115. doi: 10.1007/s00415-014-7524-3
Wang, L., Li, J., and Chen, J. (2018). Levodopa-carbidopa intestinal gel in Parkinson’s disease: a systematic review and meta-analysis. Front. Neurol. 9:620. doi: 10.3389/fneur.2018.00620
Wojtecki, L., Vesper, J., and Schnitzler, A. (2011). Interleaving programming of subthalamic deep brain stimulation to reduce side effects with good motor outcome in a patient with Parkinson’s disease. Parkinsonism Relat. Disord. 17, 293–294. doi: 10.1016/j.parkreldis.2010.12.005
Xu, J., Wang, J., Keith, S., Zhang, M., Yang, C., Yuan, Q., et al. (2021). Management of Parkinson’s disease patients after DBS by remote programming: preliminary application of single center during quarantine of 2019-nCoV. J. Neurol. 268, 1295–1303. doi: 10.1007/s00415-020-10273-z
Yang, Y. R., Tseng, C. Y., Chiou, S. Y., Liao, K. K., Cheng, S. J., Lai, K. L., et al. (2013). Combination of rTMS and treadmill training modulates corticomotor inhibition and improves walking in Parkinson disease: a randomized trial. Neurorehabil. Neural Repair. 27, 79–86. doi: 10.1177/1545968312451915
Yogev, G., Giladi, N., Peretz, C., Springer, S., Simon, E. S., and Hausdorff, J. M. (2005). Dual tasking, gait rhythmicity, and Parkinson’s disease: which aspects of gait are attention demanding? Eur. J. Neurosci. 22, 1248–1256. doi: 10.1111/j.1460-9568.2005.04298.x
Yokoe, M., Mano, T., Maruo, T., Hosomi, K., Shimokawa, T., Kishima, H., et al. (2018). The optimal stimulation site for high-frequency repetitive transcranial magnetic stimulation in Parkinson’s disease: a double-blind crossover pilot study. J. Clin. Neurosci. 47, 72–78. doi: 10.1016/j.jocn.2017.09.023
Yotnuengnit, P., Bhidayasiri, R., Donkhan, R., Chaluaysrimuang, J., and Piravej, K. (2018). Effects of transcranial direct current stimulation plus physical therapy on gait in patients with Parkinson disease: a randomized controlled trial. Am. J. Phys. Med. Rehabil. 97, 7–15. doi: 10.1097/PHM.0000000000000783
Yu, J. Y., Rajagopal, A., Syrkin-Nikolau, J., Shin, S., Rosenbluth, K. H., Khosla, D., et al. (2020). Transcutaneous afferent patterned stimulation therapy reduces hand tremor for one hour in essential tremor patients. Front. Neurosci. 14:530300. doi: 10.3389/fnins.2020.530300
Yuan, H., and Silberstein, S. D. (2016). Vagus nerve and vagus nerve stimulation, a comprehensive review: part II. Headache 56, 259–266. doi: 10.1111/head.12650
Zanjani, A., Zakzanis, K. K., Daskalakis, Z. J., and Chen, R. (2015). Repetitive transcranial magnetic stimulation of the primary motor cortex in the treatment of motor signs in Parkinson’s disease: a quantitative review of the literature. Mov. Disord. 30, 750–758. doi: 10.1002/mds.26206
Zehr, E. P., and Haridas, C. (2003). Modulation of cutaneous reflexes in arm muscles during walking: further evidence of similar control mechanisms for rhythmic human arm and leg movements. Exp. Brain Res. 149, 260–266. doi: 10.1007/s00221-003-1377-9
Zesiewicz, T. A., Elble, R. J., Louis, E. D., Gronseth, G. S., Ondo, W. G., Dewey, R. B. Jr., et al. (2011). Evidence-based guideline update: treatment of essential tremor: report of the quality standards subcommittee of the American Academy of Neurology. Neurology 77, 1752–1755. doi: 10.1212/WNL.0b013e318236f0fd
Zhang, J., Hu, W., Chen, H., Meng, F., Li, L., and Okun, M. S. (2020). Implementation of a novel bluetooth technology for remote deep brain stimulation programming: the pre- and post-COVID-19 Beijing experience. Mov. Disord. 35, 909–910. doi: 10.1002/mds.28098
Zhao, Y., Nonnekes, J., Storcken, E. J., Janssen, S., van Wegen, E. E., Bloem, B. R., et al. (2016). Feasibility of external rhythmic cueing with the Google Glass for improving gait in people with Parkinson’s disease. J. Neurol. 263, 1156–1165. doi: 10.1007/s00415-016-8115-2
Keywords: Parkinson’s disease, tremor, freezing of gait (FOG), gait, stimulation, invasive medical devices, non-invasive medical device
Citation: Fujikawa J, Morigaki R, Yamamoto N, Oda T, Nakanishi H, Izumi Y and Takagi Y (2022) Therapeutic Devices for Motor Symptoms in Parkinson’s Disease: Current Progress and a Systematic Review of Recent Randomized Controlled Trials. Front. Aging Neurosci. 14:807909. doi: 10.3389/fnagi.2022.807909
Received: 02 November 2021; Accepted: 14 January 2022;
Published: 29 March 2022.
Edited by:
Woon-Man Kung, Chinese Culture University, TaiwanReviewed by:
Joan Cabestany, Universitat Politecnica de Catalunya, SpainCopyright © 2022 Fujikawa, Morigaki, Yamamoto, Oda, Nakanishi, Izumi and Takagi. This is an open-access article distributed under the terms of the Creative Commons Attribution License (CC BY). The use, distribution or reproduction in other forums is permitted, provided the original author(s) and the copyright owner(s) are credited and that the original publication in this journal is cited, in accordance with accepted academic practice. No use, distribution or reproduction is permitted which does not comply with these terms.
*Correspondence: Ryoma Morigaki, bW9yaWdha2kucml5b21hLjFAdG9rdXNoaW1hLXUuYWMuanA=
Disclaimer: All claims expressed in this article are solely those of the authors and do not necessarily represent those of their affiliated organizations, or those of the publisher, the editors and the reviewers. Any product that may be evaluated in this article or claim that may be made by its manufacturer is not guaranteed or endorsed by the publisher.
Research integrity at Frontiers
Learn more about the work of our research integrity team to safeguard the quality of each article we publish.