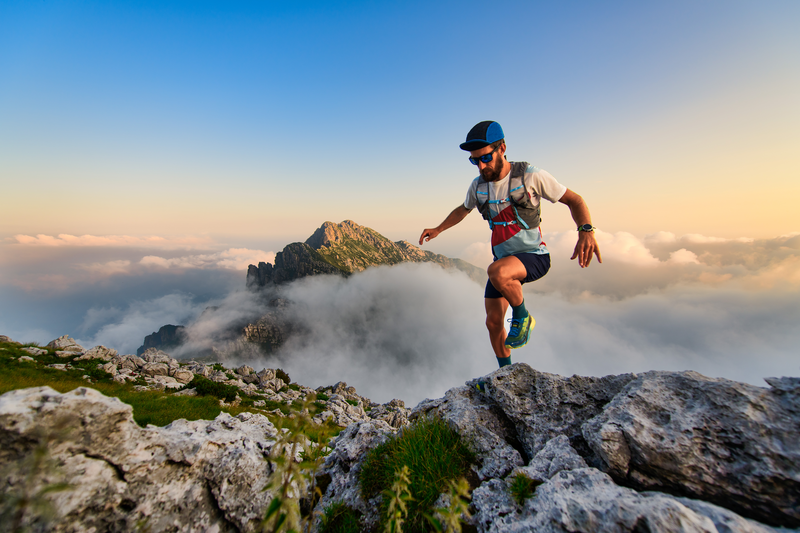
94% of researchers rate our articles as excellent or good
Learn more about the work of our research integrity team to safeguard the quality of each article we publish.
Find out more
ORIGINAL RESEARCH article
Front. Aging Neurosci. , 07 March 2022
Sec. Neurocognitive Aging and Behavior
Volume 14 - 2022 | https://doi.org/10.3389/fnagi.2022.791698
Background: Human brain function declines with aging. In this sense, exercise-based interventions has a promising effect on brain plasticity for older adults. Serum brain-derived neurotrophic factor (BDNF) is a positive biomarker for brain neuroplasticity in healthy older adults also modified by exercise training. Selected features of the exercise prescription for improving brain health are missing; therefore, the aim of this study was to determine the effects of concurrent exercise training frequency on serum BDNF levels in healthy older adults.
Methods: Nineteen volunteers (age: 65 ± 4 year; body mass index: 28.0 ± 4.5 kg/m2) completed either a three times/week (3-t/w) (n = 8) or five times/week (5-t/w) (n = 11) concurrent exercise program. The exercise program lasted 11 weeks and all exercise sessions were performed for 50 min at moderate intensity. Serum BDNF, body composition, cardiovascular, and physical fitness variables were assessed before and after the exercise training program.
Results: Regardless of the group, the serum BDNF increased following the intervention (p < 0.001), and there were no significant group (p = 0.827) or interaction (p = 0.063) effects. The maximal oxygen consumption (VO2max) increased regardless of the group (p = 0.007), with a non-significant group (p = 0.722) or interaction (p = 0.223) effects. Upper- and lower-body strength increased in both groups (p = 0.003); however, there was no effect of the training frequency (p = 0.53). For the skeletal muscle mass, there was a trend in the interaction effect (p = 0.053). Finally, the body fat percentage was unchanged.
Conclusion: Eleven weeks of combined exercise training increased serum BDNF levels in healthy older adults, a response independent of the training frequency. The overall fitness level improved similarly in both exercise groups. These data reveal that a minimal dosage of concurrent exercise enhance functional capacity and a brain health biomarker in older adults.
In humans, aging is a biological condition characterized by a progressive and unstable decline of physiological capacities and core molecular functions (Carmona and Michan, 2016). Immunosenescence (Kohut and Senchina, 2004; Tylutka et al., 2021) and body composition redistribution are deleterious changes observed during aging (Kohrt et al., 1992; St-Onge and Gallagher, 2010). Indeed, in older adults, it is known that these processes elicit high body adiposity stores along with the concomitant reduction in skeletal muscle mass compared to younger adults (Kohrt et al., 1992; Janssen and Ross, 2005). The age-related reduction on skeletal muscle mass has a direct association with muscle function loss (Akima et al., 2001). To address this problem, several guidelines for aging adults recommend to perform strength training regularly (Garber et al., 2011; Fragala et al., 2019). Thus, research has been conducted to identify the optimal exercise dosage to maintain muscle mass and function in older adults (Galvão and Taaffe, 2005; DiFrancisco-Donoghue et al., 2007; Bickel et al., 2011). The evidence suggests that resistance training performed regularly at least once per week provides enough stimulus to maintain the neuromuscular performance in older adults; nevertheless, the same exercise dose was insufficient to maintain an adequate muscle mass (Bickel et al., 2011). Similar findings were reported before (Galvão and Taaffe, 2005; DiFrancisco-Donoghue et al., 2007). Age-related reductions in brain volume are believed to have a direct, negative impact on functional ability and preserved independence (Jack et al., 1998; Erickson et al., 2010). The brain-derived neurotrophic factor (BDNF) is a biomarker of healthy brain function (Erickson et al., 2010; Peng et al., 2011). The BDNF is a member of the neurotrophin family of growth factors (Nofuji et al., 2008), and in the brain, is mainly expressed in the hippocampus (Katoh-Semba et al., 1997) and plays an essential role in the viability and function of neuronal cells (Funakoshi et al., 1993; Mowla et al., 2001). Studies in humans provide evidence that exercise increases BDNF production and secretion from the brain into the periphery in the healthy elderly population (Erickson et al., 2011; Forti et al., 2015). Exercise training is widely recognized as an effective treatment to reduce the age-related cognitive decline, similar to its impact on skeletal muscle mass maintenance (Dishman et al., 2006; Erickson et al., 2010). However, the proper exercise dose-response aimed at improving brain function has not been elucidated as it has been shown in studies of peripheral organs. Therefore, the purpose of the study was to compare two exercise training routines (three or five times for week) on serum BDNF, body composition and selected functional and physiological responses in healthy older adults. The potential data to achieve in the current work might be considered as background to establish and design further studies aimed to identify a threshold of concurrent exercise to strength the brain health in aged population.
Nineteen healthy older adults [age: 64.8 ± 4.3 year; body mass index (BMI): 28.0 ± 4.5 kg/m2] enrolled in the “Active Aging” community health program from Facultad de Deportes of Universidad Autónoma de Baja California were recruited in the present study. The volunteers received information about the aim of the study and read and signed an institutionally approved informed consent. Participants completed a medical history questionnaire and reported being free of any musculoskeletal, cardiovascular, mental health problems, cognitive impairment, and metabolic diseases. Volunteers were excluded from the study if any drug or alcohol abuse was reported. Once included in the study, the participants completed an exercise program either three times/week (3-t/w, n = 8) or five times/week (5-t/w, n = 11; Table 1). The experimental group assignment was made according to the preferred participant’s time availability. Participants of both experimental groups performed aerobic and strength training (combined) exercises (see exercise training program for details). The study protocol followed the Helsinki Declaration principles, was approved by the Bioethics and Biosecurity Committee Facultad de Medicina y Psicología of Universidad Autónoma de Baja California and was registered under the code 889/2020-2.
This is a quasi-experimental study with baseline (pre-test) and post-training (post-test) measures of anthropometric, body composition, cardiovascular, functional capacity, and BDNF variables. The participants visited the Laboratorio de Fisiología Aplicada al Ejercicio Fisico twice for familiarization with the experimental procedures and the exercise training protocol. On the first visit, all participants underwent body composition evaluation and fasting venous blood sample collection for BDNF assessment. The second visit was scheduled 24-h before and after the concurrent exercise program for assessment of resting heart rate (HRrest), systolic blood pressure (SBP), diastolic blood pressure (DBP), mean arterial pressure (MAP), 6-min walking test (6MWT), and the Senior Fitness Test (SFT) (Figure 1).
The anthropometric assessments were performed on the first visit to the facilities. Body height (cm) was measured with an electronic stadiometer to the nearest ± 1 mm (Biospace Corporation, Seoul, South Korea). Body composition [i.e., body weight (kg), body fat mass (%), muscle mass (kg), and BMI] was measured with a bioimpedance analyzer Inbody™ model 770 (Inbody, Seoul, South Korea). The participants were instructed to refrain from eating or drinking for at least 2-h before anthropometric and body composition measurements were recorded.
During the second visit, the participants sat for 5-min, and then SBP and SBDP were recorded in mmHg. The SBP and DBP were measured in duplicate using an automatic sphygmomanometer Omron (OMRON Corp., Osaka, Japan). The MAP was estimated with the formula: DBP + (0.33 × [SBP-DBP]) (Sainas et al., 2016). Following the hemodynamic measurements, the 6MWT was performed following a standard procedure (Rikli and Jones, 1998; Guimarães et al., 2002). Briefly, participants wore a Tanita PD-733F pedometer (Tanita Corp., Arlington Heights, IL, United States) hanging from a strap to the neck and set to display the steps. Then, a member of the investigator team followed the manufacturer’s instructions to determine the participant’s stride length. All participants were instructed to walk around a basketball field for 6 min; the aim was to cover the longest possible distance without jogging or running. A member of the investigator team walked alongside the participants encouraging them to walk as fast as possible. Immediately after the 6MWT, the researcher registered the steps recorded to estimate the distance achieved (m). Throughout the 6MWT, all participants wore a chest strapped heart rate monitor Polar FT1 (Polar Electro, Kempele, Finland) for telemetric heart rate monitoring. Finally, the maximal oxygen consumption (VO2max) was estimated following the equation reported by Burr (Burr et al., 2011). After the 6MWT, the participants completed the Senior Fitness Test (Jones and Rikli, 2002).
A resting 4-mL venous blood was drawn from the antecubital vein of the non-dominant arm of the participant by a certified phlebotomist. Blood samples were drawn into 6 mL BD Vacutainer 368175 tubes (Becton, Dickinson and Co., Franklin Lakes, NJ, United States) with no additives for serum collection. Blood was allowed to clot at room temperature for 40-min. Then, the tubes were centrifuged at 1,500 rpm for 10-min at room temperature and the supernatant was isolated and stored into 1.5 mL Eppendorf tubes at −20°C until further analysis. Serum samples were diluted 1:10 with dilution buffer from the manufacture’s kit and BDNF’s concentration was obtained by an enzyme-linked immunoassay (ELISA) kit Abcam ab99978-BDNF (Abcam, Cambridge, United Kingdom) following the manufacturer’s instruction. Detection limits were <80 pg/mL, mean inter-and intra-assay coefficients of variance were <10 and <12%, respectively. The absorbance was read at 450 nm in an iMark microplate absorbance reader BIO-RAD Cat. 168-1130 (Bio-Rad Laboratories, Inc., Hercules, CA, United States), immediately after the stop solution was added.
The exercise training protocol performed by the participants consisted of the same duration and intensity; the only difference was the weekly frequency (i.e., 3-t/w vs. 5-t/w). The training program was comprised of aerobic and resistance training exercises; the aerobic component included walking on a flat surface, walking up and down a flight of stairs, and skipping exercise performed for 50-min. The resistance exercise component included chair stands for the lower body, and biceps and triceps curls, horizontal adduction, and overhead press for the upper body. All the upper-body exercises were performed with elastic bands for 50-min. The exercise intensity for each exercise session was monitored with the 6–20-point Borg’s rating of perceived exertion (RPE) every 10-min throughout the session. Exercises were adjusted to reach a RPE between 12 and 14 points (Supplementary Figure 1). Furthermore, each exercise session included a warm-up and cool-down recovery including passive stretch exercises for 10-min. The length of the training program was 11 weeks for both experimental groups; all sessions were performed between 08:00 and 10:00 a.m. and were supervised by the investigator team members.
Following the completion of the 11-week exercise program, all anthropometric, body composition, blood samples, cardiovascular, SFT, and 6MWT measurements were performed using the same procedures described above for baseline (Figure 1).
The statistical analyses and figures were completed with the GraphPad Prism 6.0 software (Graphpad Holdings, LLC., La Jolla, CA, United States) and JASP statistical software v. 0.14.1 (University of Amsterdam, Amsterdam, Netherlands). Descriptive statistics are presented as the mean and standard deviation (M ± SD). Independent-samples t-tests compared participant’s demographic, age, BMI, and education years at baseline. Serum BDNF concentration, cardiovascular, SFT, and anthropometric variables were analyzed with a two-way repeated-measures ANOVA (group × time) followed by Bonferroni’s post hoc tests when appropriate. We used the Kolmogorov-Smirnov normality test to analyze the dependent variables. Based on the results, we identified only three variables that departed from the normality assumption; however, ANOVA is considered a robust test that tolerates violations to its normality assumption (Blanca et al., 2017). The η2 effect size was computed and interpreted as small (≤0.01), medium (0.06), and large (≥0.14) (Cohen, 1992). Statistical significance was set a priori at p ≤ 0.05.
Participant characteristics at baseline by experimental group are summarized in Table 1.
The effects of the two exercise training frequencies on serum BDNF are shown in Figure 2. Three participants of the 5-t/w group were excluded from the analysis due to negative absorbance values. There was a small trend for the interaction effect (p = 0.063; η2 = 0.039), non-significant group main effect (p = 0.827; η2 = 0.002), and a significant time main effect (p < 0.001; η2 = 0.328) on the BDNF concentration.
Figure 2. Effects of the exercise training frequency on serum brain-derived neurotrophic factor (BDNF) levels. The chronic concurrent exercise program increased the peripheral BDNF levels in healthy older adults. The response was independent of the exercise training frequency. Two-way ANOVA. ***p < 0.05 vs. Baseline. Data are Mean ± SD.
The heart rate response during the 6MWT before and after the exercise program for the 3- and 5-t/w groups are presented in Figures 3A,B, respectively. There was an interaction between group and time on HRrest (p = 0.042; Table 2). The follow-up analysis showed a reduction trend (p = 0.075) in the HRrest in the 3-t/w exercise group compared to the pre-test scores. For peak heart rate (HRpeak) during the 6MWT there was a time main effect (p = 0.024; η2 = 0.111), and a medium effect for interaction (p = 0.090; η2 = 0.06; Table 2). A main time effect was observed for the heart rate recovery (HRR) (p = 0.003 η2 = 0.222; Figure 3C). There were main time effects for MAP (p = 0.002; η2 = 0.07; Figure 3D), DBP (p = 0.003; η2 = 0.074), and VO2max (p = 0.007; η2 = 0.48; Table 2).
Figure 3. Heart rate response through the 6-min walking test (6MWT) and during 1 min immediately finished the exercise testing. (A) 3-t/w and (B) 5-t/w groups. Absolute values of heart rate recovery during the first minute of immediately finished the 6MWT (C). Baseline mean arterial pressure (MAP) values before and after exercise program (D). Two-way ANOVA. **p < 0.05 vs. Baseline. Data are Mean ± SD.
For the body composition variables, a group effect was observed in fat mass (%) (p = 0.037; η2 = 0.230). A significant time (p = 0.007; η2 = 0.03) and a small interaction effect between group and time (p = 0.053; η2 = 0.013) was found in skeletal muscle mass (Table 3). Regarding the fitness levels, a significant main interaction effect was found on the 6MWT distance (p = 0.031; η2 = 0.04). Only the participants in the 5-t/w group walked a longer distance during the 6MWT following the exercise program (p < 0.001; η2 = 0.233; Table 3). Regardless of the group, a time effect was found on the lower-body strength (p = 0.003; η2 = 0.146), the upper-body strength (p = 0.003; η2 = 0.118), and the agility (p = 0.001). No significant group (p = 0.537) and interaction (p = 0.135) effects were observed on agility (Table 3).
Eleven weeks of concurrent (i.e., aerobic + strength) exercise induced positive changes for fitness and strength and increased serum BDNF; however, the increment of circulating BDNF was independent of the exercise training frequency; similar responses were observed for variables associated with functional capacity. The BDNF is a neurotrophin sensitive to changes elicited by chronic aerobic, strength, or concurrent exercise (Seifert et al., 2010; Schmolesky et al., 2013; Dinoff et al., 2016). The positive impact of acute concurrent exercise session on circulating BDNF has been reported in the young population (Murawska-Cialowicz et al., 2015; Figueiredo et al., 2019). However, there are contradictory findings in older adults (Kim et al., 2014; Nascimento et al., 2014; Ruiz et al., 2015). For instance, there is evidence reporting non-significant effects of exercise on BDNF (Ruiz et al., 2015), whereas others have found high circulating BDNF levels following a long-term concurrent training (Nascimento et al., 2014). Our results agree with the latter (Figure 2). It is worthy to notice that the age of the participants in the study by Nascimento et al. (66 year) and the current work (64 year) was similar; however, the participants in the study by Ruiz et al. were older (92 year). Therefore, it seems that age could be a moderator variable associated with the BDNF regulatory response to a concurrent exercise program. In line with this, other authors agreed that aging is a biological regulator of peripheral BDNF concentration in humans (Lommatzsch et al., 2005; Driscoll et al., 2012). The exercise program prescribed in the current study did not require exercise equipment (i.e., bench press machine, Smith machine); a similar exercise model was reported by others (Nascimento et al., 2014). The previous information emphasizes the efficacy of an exercise program free of expensive equipment to increase the serum BDNF in healthy older adults. Considering this, others have previously demonstrated the efficacy of exercise programs free of expensive equipment to improve cognitive performance in healthy older adults (Eckardt et al., 2020).
There is a plethora of potential molecular mechanisms elicited by combined exercise aimed at increasing the serum BDNF. First, the exercise program prescribed in the present study included moderate-intensity aerobic exercise. It has been suggested -and partially demonstrated-, that aerobic exercise increases the neuronal activity that increases intracellular calcium, which further enhances BDNF synthesis and release (Fernandes et al., 2017). The exercise program also included strength exercises, and recent meta-analytical evidence shows that resistance training exercise increases insulin-like growth factor-1 (IGF-1) synthesis in skeletal muscle in male and female older adults (Jiang et al., 2020; Ye et al., 2021). This anabolic molecule reaches the brain to induce BDNF production (Herold et al., 2019; Pinho et al., 2019).
Additionally, the lactate generated during exercise is another potential molecule to enhance the synthesis of BDNF in brain; consequently, increasing the BDNF concentration in the periphery (Hashimoto et al., 2021; Huang et al., 2021). In this sense, resistance training in older people is a stimulus that increase blood lactate in healthy elderly (Valkeinen et al., 2006; Simões et al., 2013). Therefore, we did not discard that the training program applied in the current work increased the blood lactate, facilitating the raising of serum BDNF. Finally, the BDNF measured in serum is released during by the platelets during their activation (Gejl et al., 2019); that response happened because the platelets (blood cells) are the main reservoir of the circulating neurotrophin (Fujimura et al., 2002). Recent evidence showed that chronic physical exercise did not modify the function of the platelets in healthy elderly population (Haynes et al., 2018). Therefore, we discard that the changes in the serum BDNF showed by the participants can be derived from platelet function more than the brain adaptation to exercise.
The results of the present study showed that there was no significant effect of the training frequency. To the best of our knowledge, this is the first study aimed to determine the impact of different concurrent exercise frequencies on serum BDNF in healthy older adults. Others have studied the effects of different exercise intensities on serum BDNF in a similar population (Forti et al., 2015). For instance, Forti et al. (2015) found that the exercise intensity significantly changed circulating BDNF in males but not in females. In the present study, there was a high proportion of women compared to men (Table 1); therefore, we do not discard the possibility of the majority of female participants in our sample could become a potential factor attenuating the changes in BDNF. Further studies are needed to clarify this effect.
With regard to the cardiovascular adaptations, the HRR, HRpeak, and MAP improved after the concurrent exercise program (Table 2). The attenuated HR response to exercise, named chronotropic incompetence, has been identified as an independent risk marker of mortality (Lauer et al., 1999; Khan et al., 2005). Indeed, the chronotropic incompetence becomes more pronounced with aging, which is prevented by appropriate cardiorespiratory fitness (Ciolac et al., 2014; Ozemek et al., 2016). The high HRpeak found in the current work indicates the efficacy of the concurrent exercise program to improve heart function during exertion (i.e., 6MWT). The greater HRR after the intervention completion reinforces the positive impact of concurrent exercise to strengthen the cardiovascular health, mainly the cardiac function in older adults. Together with the changes mentioned above, the VO2max was also improved with the combined exercise program (Table 2). Our findings agree with other studies with similar duration (Campos et al., 2013; Chapman et al., 2013). The increased distance achieved on the 6MWT by the 5-t/w group after the intervention suggests that the improvement of the VO2max can be dependent on the training frequency in older adults. We consider the null magnitude of VO2max observed between groups in the current study is explained by the indirect estimation method employed (Burr’s equation). Further studies are needed to rectify the lowest amount of combined exercise (i.e., concurrent) frequency to increase VO2max in older adults.
The upper- and lower-limb strength improved after 11 weeks of concurrent exercise. Our findings agree with others using a similar exercise prescription (i.e., 12-week duration, 3-t/w) (Campos et al., 2013). In addition to the increment of segmental strength, agility was also improved (Table 3). However, there was not a significant difference in functional capacity variables between groups after the intervention (Table 3). Our findings are consistent with others who reported no significant effects of the training frequency on the functional capacity in healthy older adults (Taaffe et al., 1999; Turpela et al., 2017). The previous information highlights the potential application of concurrent exercise programs for sedentary older adults. The proposal of strength and aerobic training carried out three times per week for ∼50-min helps to maintain the brain and cardiovascular health during aging.
Finally, for the skeletal muscle mass, there was a strong trend of the interaction effect, having the 5-t/w the group with more skeletal muscle mass increase compared to the 3-t/w group after 11 weeks of training. Body weight, BMI, and body fat percentage were unaffected by the exercise program (Table 3). The results regarding body composition are unclear; some components showed a trend for improvement whereas the variables associated with obesity did not change. The current findings agree with previous studies that used chronic exercise interventions (Taaffe et al., 1999; Murlasits et al., 2012; Campos et al., 2013; Turpela et al., 2017; Marcos-Pardo et al., 2019). In these studies, including the current work, the diet was not controlled or accounted for. The previous information leads us to consider the diet as a key variable to modify the body composition in healthy older adults. Our study has important limitations. One of them is the small sample size analyzed, even though we recruited small participants in our work, similar studies did involve similar sample sizes (Kim et al., 2014; Ruiz et al., 2015). Another limitation was that the work did not included a non-exercise control group. The study was carried out in a community health program focused to promote the regular exercise practice in elderly. Therefore, the limit or reduce the participation of elderly to recruit a control group will be have ethical issue for the community outreach program. A second limitation is that our work did not included cognitive testing. Although the BDNF is considered a direct marker of brain health (mainly hippocampus function in elderly) (Erickson et al., 2010), this neurotrophin only cover one of the multiple levels to analyze the neurocognitive function (Stillman et al., 2016; Herold et al., 2019), studies employing techniques to determine the structural brain changes and cognitive testing are needed to clarify the impact of the concurrent training on neurocognitive function in healthy elderly population.
The current work demonstrates that a moderate-length concurrent exercise training program increases fasting serum BDNF levels in healthy older adults. This response seems independent of the training frequency, which suggests a plateau for the CNS response to the concurrent exercise stimulus. The present evidence emphasizes the unnecessary excessive training to achieve a healthier brain in older adults. Moreover, the physical fitness level was improved in the same magnitude in both exercise groups. These data reveal a minimal dose-response of concurrent exercise to improve functional capacity in older adults.
The raw data supporting the conclusions of this article will be made available by the authors, without undue reservation.
The studies involving human participants were reviewed and approved by Bioethics and Biosecurity Committee Facultad de Medicina y Psicología of Universidad Autónoma de Baja California and was registered under the code 889/2020-2. The patients/participants provided their written informed consent to participate in this study.
AJ-M, IR, and EC-M: conceptualization. PG-S, JM-P, and EC-M: methodology. JM-J and PG-S: statistical analysis. AJ-M: writing—original draft preparation. FL and DJ: writing—review and editing. PG-S, IR, and JM-J: manuscript preparation and editing. All authors have read and agreed to the published version of the manuscript.
The authors declare that the research was conducted in the absence of any commercial or financial relationships that could be construed as a potential conflict of interest.
All claims expressed in this article are solely those of the authors and do not necessarily represent those of their affiliated organizations, or those of the publisher, the editors and the reviewers. Any product that may be evaluated in this article, or claim that may be made by its manufacturer, is not guaranteed or endorsed by the publisher.
We greatly appreciate the time and effort invested by the participants. We thank the staff of the “Active Aging” community health program of Facultad de Deportes Campus Ensenada for their collaboration. We also thank our research staff for their skillful support during the exercise sessions.
The Supplementary Material for this article can be found online at: https://www.frontiersin.org/articles/10.3389/fnagi.2022.791698/full#supplementary-material
Supplementary Figure 1 | Values of the rating of perceived exertion (Borg scale-20) for each exercise training session. Data are M ± SD.
Akima, H., Kano, Y., Enomoto, Y., Ishizu, M., Okada, M., Oishi, Y., et al. (2001). Muscle function in 164 men and women aged 20-84 yr. Med. Sci. Sports Exerc. 33, 220–226. doi: 10.1097/00005768-200102000-00008
Bickel, C. S., Cross, J. M., and Bamman, M. M. (2011). Exercise dosing to retain resistance training adaptations in young and older adults. Med. Sci. Sports Exerc. 43, 1177–1187. doi: 10.1249/MSS.0b013e318207c15d
Blanca, M. J., Alarcón, R., Arnau, J., Bono, R., and Bendayan, R. (2017). Datos no normales: ¿es el ANOVA una opción válida? Psicothema 29, 552–557. doi: 10.7334/psicothema2016.383
Burr, J. F., Bredin, S. S. D., Faktor, M. D., and Warburton, D. E. R. (2011). The 6-minute walk test as a predictor of objectively measured aerobic fitness in healthy working-aged adults. Phys. Sportsmed. 39, 133–139. doi: 10.3810/psm.2011.05.1904
Campos, A. L. P., dos Santos Del Ponte, L., Schuler Cavalli, A., da Rosa, Afonso, M., Gomes Schild, J. F., et al. (2013). Effects of concurrent training on health aspects of elderly women Efeitos. Brazilian J. Kinanthropometry Hum. Perform. 15, 437–447.
Carmona, J. J., and Michan, S. (2016). Biology of healthy aging and longevity. Rev. Invest. Clin. 68, 7–16.
Chapman, S. B., Aslan, S., Spence, J. S., DeFina, L. F., Keebler, M. W., Didehbani, N., et al. (2013). Shorter term aerobic exercise improves brain, cognition, and cardiovascular fitness in aging. Front. Aging Neurosci. 5:75. doi: 10.3389/fnagi.2013.00075
Ciolac, E. G., Roberts, C. K., Rodrigues Da Silva, J. M., and Guimarães, G. V. (2014). Age affects exercise-induced improvements in heart rate response to exercise. Int. J. Sports Med. 35, 371–378. doi: 10.1055/s-0033-1351332
DiFrancisco-Donoghue, J., Werner, W., and Douris, P. C. (2007). Comparison of once-weekly and twice-weekly strength training in older adults. Br. J. Sports Med. 41, 19–22. doi: 10.1136/bjsm.2006.029330
Dinoff, A., Herrmann, N., Swardfager, W., Liu, C. S., Sherman, C., Chan, S., et al. (2016). The effect of exercise training on resting concentrations of peripheral brain-derived neurotrophic factor (BDNF): a meta-analysis. PLoS One 11:e0163037. doi: 10.1371/journal.pone.0163037
Dishman, R. K., Berthoud, H. R., Booth, F. W., Cotman, C. W., Edgerton, V. R., Fleshner, M. R., et al. (2006). Neurobiology of exercise. Obesity 14, 345–356. doi: 10.1038/oby.2006.46
Driscoll, I., Martin, B., An, Y., Maudsley, S., Ferrucci, L., Mattson, M. P., et al. (2012). Plasma BDNF is associated with age-related white matter atrophy but not with cognitive function in older, non-demented adults. PLoS One 7:e35217. doi: 10.1371/journal.pone.0035217
Eckardt, N., Braun, C., and Kibele, A. (2020). Instability resistance training improves working memory, processing speed and response inhibition in healthy older adults: a double-blinded randomised controlled trial. Sci. Rep. 10:2506. doi: 10.1038/s41598-020-59105-0
Erickson, K. I., Prakash, R. S., Voss, M. W., Chaddock, L., Heo, S., McLaren, M., et al. (2010). Brain-derived neurotrophic factor is associated with age-related decline in hippocampal volume. J. Neurosci. 30, 5368–5375. doi: 10.1523/JNEUROSCI.6251-09.2010
Erickson, K. I., Voss, M. W., Prakash, R. S., Basak, C., Szabo, A., Chaddock, L., et al. (2011). Exercise training increases size of hippocampus and improves memory. Proc. Natl. Acad. Sci. 108, 3017–3022.
Fernandes, J., Arida, R. M., and Gomez-Pinilla, F. (2017). Physical exercise as an epigenetic modulator of brain plasticity and cognition. Neurosci. Biobehav. Rev. 80, 443–456. doi: 10.1016/j.neubiorev.2017.06.012
Figueiredo, C., Antunes, B. M., Giacon, T. R., Vanderlei, L. C. M., Campos, E. Z., Peres, F. P., et al. (2019). Influence of acute and chronic high-intensity intermittent aerobic plus strength exercise on BDNF, lipid and autonomic parameters. J. Sport. Sci. Med. 18, 359–368.
Forti, L. N., Van Roie, E., Njemini, R., Coudyzer, W., Beyer, I., Delecluse, C., et al. (2015). Dose-and gender-specific effects of resistance training on circulating levels of brain derived neurotrophic factor (BDNF) in community-dwelling older adults. Exp. Gerontol. 70, 144–149. doi: 10.1016/j.exger.2015.08.004
Fragala, M. S., Cadore, E. L., Dorgo, S., Izquierdo, M., Kraemer, W. J., Peterson, M. D., et al. (2019). Resistance training for older adults: position statement from the national strength and conditioning association. J. Strength Cond. Res. 33, 2019–2052. doi: 10.1519/jsc.0000000000003230
Fujimura, H., Altar, C. A., Chen, R., Nakamura, T., Nakahashi, T., Kambayashi, J. I., et al. (2002). Brain-derived neurotrophic factor is stored in human platelets and released by agonist stimulation. Thromb. Haemost. 87, 728–734. doi: 10.1055/s-0037-1613072
Funakoshi, H., Frisén, J., Barbany, G., Timmusk, T., Zachrisson, O., Verge, V. M. K., et al. (1993). Differential expression of mRNAs for neurotrophins and their receptors after axotomy of the sciatic nerve. J. Cell Biol. 123, 455–465. doi: 10.1083/jcb.123.2.455
Galvão, D. A., and Taaffe, D. R. (2005). Resistance exercise dosage in older adults: single- versus multiset effects on physical performance and body composition. J. Am. Geriatr. Soc. 53, 2090–2097. doi: 10.1111/j.1532-5415.2005.00494.x
Garber, C. E., Blissmer, B., Deschenes, M. R., Franklin, B. A., Lamonte, M. J., Lee, I. M., et al. (2011). Quantity and quality of exercise for developing and maintaining cardiorespiratory, musculoskeletal, and neuromotor fitness in apparently healthy adults: guidance for prescribing exercise. Med. Sci. Sports Exerc. 43, 1334–1359. doi: 10.1249/MSS.0b013e318213fefb
Gejl, A. K., Enevold, C., Bugge, A., Andersen, M. S., Nielsen, C. H., and Andersen, L. B. (2019). Associations between serum and plasma brain-derived neurotrophic factor and influence of storage time and centrifugation strategy. Sci. Rep. 9:9655. doi: 10.1038/s41598-019-45976-5
Guimarães, G. V., Bellotti, G., Bacal, F., Mocelin, A., and Bocchi, E. A. (2002). Can the cardiopulmonary 6-minute walk test reproduce the usual activities of patients with heart failure? Arq. Bras. Cardiol. 78, 553–560. doi: 10.1590/s0066-782x2002000600003
Hashimoto, T., Tsukamoto, H., Ando, S., and Ogoh, S. (2021). Effect of exercise on brain health: the potential role of lactate as a myokine. Metabolites 11:813. doi: 10.3390/metabo11120813
Haynes, A., Linden, M. D., Robey, E., Naylor, L. H., Ainslie, P. N., Cox, K. L., et al. (2018). Beneficial impacts of regular exercise on platelet function in sedentary older adults: evidence from a randomized 6-mo walking trial. J. Appl. Physiol. 125, 401–408. doi: 10.1152/japplphysiol.00079.2018
Herold, F., Törpel, A., Schega, L., and Müller, N. G. (2019). Functional and/or structural brain changes in response to resistance exercises and resistance training lead to cognitive improvements - a systematic review. Eur. Rev. Aging Phys. Act. 16, 1–33. doi: 10.1186/s11556-019-0217-2
Huang, Z., Zhang, Y., Zhou, R., Yang, L., and Pan, H. (2021). Lactate as potential mediators for exercise-induced positive effects on neuroplasticity and cerebrovascular plasticity. Front. Physiol. 12:656455. doi: 10.3389/fphys.2021.656455
Jack, C. R., Petersen, R. C., Xu, Y., O’Brien, P. C., Smith, G. E., Ivnik, R. J., et al. (1998). Rate of medial temporal lobe atrophy in typical aging and Alzheimer’s disease. Neurology 51, 993–999. doi: 10.1212/WNL.51.4.993
Janssen, I., and Ross, R. (2005). Linking age-related changes in skeletal muscle mass and composition with metabolism and disease. J. Nutr. Heal. Aging 9, 408–419.
Jiang, Q., Lou, K., Hou, L., Lu, Y., Sun, L., Tan, S. C., et al. (2020). The effect of resistance training on serum insulin-like growth factor 1(IGF-1): a systematic review and meta-analysis. Complement. Ther. Med. 50:102360. doi: 10.1016/j.ctim.2020.102360
Jones, C. J., and Rikli, R. E. (2002). Measuring functional fitness of older adults. J. Act. Aging 24–30. doi: 10.1016/0021-8502(77)90078-7
Katoh-Semba, R., Takeuchi, I. K., Semba, R., and Kato, K. (1997). Distribution of brain-derived neurotrophic factor in rats and its changes with development in the brain. J. Neurochem. 69, 34–42. doi: 10.1046/j.1471-4159.1997.69010034.x
Khan, M. N., Pothier, C. E., and Lauer, M. S. (2005). Chronotropic incompetence as a predictor of death among patients with normal electrograms taking beta blockers (Metoprolol or Atenolol). Am. J. Cardiol. 96, 1328–1333. doi: 10.1016/j.amjcard.2005.06.082
Kim, H. J., Song, B. K., So, B., Lee, O., Song, W., and Kim, Y. (2014). Increase of circulating BDNF levels and its relation to improvement of physical fitness following 12 weeks of combined exercise in chronic patients with schizophrenia: a pilot study. Psychiatry Res. 220, 792–796. doi: 10.1016/j.psychres.2014.09.020
Kohrt, W. M., Malley, M. T., Dalsky, G. P., and Holloszy, J. O. (1992). Body composition of healthy sedentary and trained, young and older men and women. Med. Sci. Sport. Exerc. 24, 832–837.
Kohut, M. L., and Senchina, D. S. (2004). Reversing age-associated immunosenescence via exercise. Exerc. Immunol. Rev. 10, 6–41.
Lauer, M. S., Francis, G. S., Okin, P. M., Pashkow, F. J., Snader, C. E., and Marwick, T. H. (1999). Impaired chronotropic response to exercise stress testing as a predictor of mortality. J. Am. Med. Assoc. 281, 524–529. doi: 10.1001/jama.281.6.524
Lommatzsch, M., Zingler, D., Schuhbaeck, K., Schloetcke, K., Zingler, C., Schuff-Werner, P., et al. (2005). The impact of age, weight and gender on BDNF levels in human platelets and plasma. Neurobiol. Aging 26, 115–123. doi: 10.1016/j.neurobiolaging.2004.03.002
Marcos-Pardo, P. J., Orquin-Castrillón, F. J., Gea-García, G. M., Menayo-Antúnez, R., González-Gálvez, N., Vale, R. G., et al. (2019). Effects of a moderate-to-high intensity resistance circuit training on fat mass, functional capacity, muscular strength, and quality of life in elderly: a randomized controlled trial. Sci. Rep. 9:7830. doi: 10.1038/s41598-019-44329-6
Mowla, S. J., Farhadi, H. F., Pareek, S., Atwal, J. K., Morris, S. J., Seidah, N. G., et al. (2001). Biosynthesis and post-translational processing of the precursor to brain-derived neurotrophic factor. J. Biol. Chem. 276, 12660–12666. doi: 10.1074/jbc.M008104200
Murawska-Cialowicz, E., Wojna, J., and Zuwala-Jagiello, J. (2015). Crossfit training changes brain-derived neurotrophic factor and irisin levels at rest, after wingate and progressive tests, and improves aerobic capacity and body composition of young physically active men and women. J. Physiol. Pharmacol. 66, 811–821.
Murlasits, Z., Reed, J., and Wells, K. (2012). Effect of resistance training frequency on physiological adaptations in older adults. J. Exerc. Sci. Fit. 10, 28–32. doi: 10.1016/j.jesf.2012.04.006
Nascimento, C., Pereira, J., Andrade, L., Garuffi, M., Talib, L., Forlenza, O., et al. (2014). Physical exercise in MCI elderly promotes reduction of pro-inflammatory cytokines and improvements on cognition and bdnf peripheral levels. Curr. Alzheimer Res. 11, 799–805. doi: 10.2174/156720501108140910122849
Nofuji, Y., Suwa, M., Moriyama, Y., Nakano, H., Ichimiya, A., Nishichi, R., et al. (2008). Decreased serum brain-derived neurotrophic factor in trained men. Neurosci. Lett. 437, 29–32. doi: 10.1016/j.neulet.2008.03.057
Ozemek, C., Whaley, M. H., Holmes Finch, W., and Kaminsky, L. A. (2016). High cardiorespiratory fitness levels slow the decline in peak heart rate with age. Med. Sci. Sports Exerc. 48, 73–81. doi: 10.1249/MSS.0000000000000745
Peng, C., Aron, L., Klein, R., Li, M., Wurst, W., Prakash, N., et al. (2011). Pitx3 is a critical mediator of GDNF-Induced BDNF expression in nigrostriatal dopaminergic neurons. J. Neurosci. 31, 12802–12815. doi: 10.1523/JNEUROSCI.0898-11.2011
Pinho, R. A., Aguiar, A. S., and Radák, Z. (2019). Effects of resistance exercise on cerebral redox regulation and cognition: an interplay between muscle and brain. Antioxidants 8:529. doi: 10.3390/antiox8110529
Rikli, R. E., and Jones, C. J. (1998). The reliability and validity of a 6 minute walk. J. Aging Phys. Act. 6, 363–375.
Ruiz, J. R., Gil-Bea, F., Bustamante-Ara, N., Rodríguez-Romo, G., Fiuza-Luces, C., Serra-Rexach, J. A., et al. (2015). Resistance training does not have an effect on cognition or related serum biomarkers in nonagenarians: a randomized controlled trial. Int. J. Sports Med. 36, 54–60. doi: 10.1055/s-0034-1375693
Sainas, G., Milia, R., Palazzolo, G., Ibba, G., Marongiu, E., Roberto, S., et al. (2016). Mean blood pressure assessment during post-exercise: result from two different methods of calculation. J. Sport. Sci. Med. 15, 424–433.
Schmolesky, M. T., Webb, D. L., and Hansen, R. A. (2013). The effects of aerobic exercise intensity and duration on levels of brain- derived neurotrophic factor in healthy men. J. Sport. Sci. Med. 12, 502–511.
Seifert, T., Brassard, P., Wissenberg, M., Rasmussen, P., Nordby, P., Stallknecht, B., et al. (2010). Endurance training enhances BDNF release from the human brain. Am. J. Physiol.Regul. Integr. Comp. Physiol. 298, 372–377. doi: 10.1152/ajpregu.00525.2009
Simões, R. P., Castello-Simões, V., Mendes, R. G., Archiza, B., Santos, D. A., Machado, H. G., et al. (2013). Lactate and heart rate variability threshold during resistance exercise in the young and elderly. Int. J. Sports Med. 34, 991–996. doi: 10.1055/s-0033-1337946
Stillman, C. M., Cohen, J., Lehman, M. E., and Erickson, K. I. (2016). Mediators of physical activity on neurocognitive function: a review at multiple levels of analysis. Front. Hum. Neurosci. 10, 1–17. doi: 10.3389/fnhum.2016.00626
St-Onge, M.-P., and Gallagher, D. (2010). Body composition changes with aging: the cause or the result of alterations in metabolic rate and macronutrient oxidation? Marie-Pierre. Nutrition 26, 152–155. doi: 10.1016/j.nut.2009.07.004
Taaffe, D. R., Duret, C., Wheeler, S., and Marcus, R. (1999). Once-weekly resistance exercise improves muscle strength and neuromuscular performance in older adults. J. Am. Geriatr. Soc. 47, 1208–1214. doi: 10.1111/j.1532-5415.1999.tb05201.x
Turpela, M., Häkkinen, K., Haff, G. G., and Walker, S. (2017). Effects of different strength training frequencies on maximum strength, body composition and functional capacity in healthy older individuals. Exp. Gerontol. 98, 13–21. doi: 10.1016/j.exger.2017.08.013
Tylutka, A., Morawin, B., Gramacki, A., and Zembron-Lacny, A. (2021). Lifestyle exercise attenuates immunosenescence; flow cytometry analysis. BMC Geriatr. 21:200. doi: 10.1186/s12877-021-02128-7
Valkeinen, H., Häkkinen, A., Hannonen, P., Häkkinen, K., and Alén, M. (2006). Acute heavy-resistance exercise-induced pain and neuromuscular fatigue in elderly women with fibromyalgia and in healthy controls: effects of strength training. Arthritis Rheum. 54, 1334–1339. doi: 10.1002/art.21751
Keywords: concurrent training, heart rate, brain derived neurotrophic factor, body fat, elderly
Citation: Canton-Martínez E, Rentería I, García-Suárez PC, Moncada-Jiménez J, Machado-Parra JP, Lira FS, Johnson DK and Jiménez-Maldonado A (2022) Concurrent Training Increases Serum Brain-Derived Neurotrophic Factor in Older Adults Regardless of the Exercise Frequency. Front. Aging Neurosci. 14:791698. doi: 10.3389/fnagi.2022.791698
Received: 08 October 2021; Accepted: 09 February 2022;
Published: 07 March 2022.
Edited by:
Christina Maria Pabelick, Mayo Clinic, United StatesReviewed by:
Matea Nikolac Perković, Rudjer Boskovic Institute, CroatiaCopyright © 2022 Canton-Martínez, Rentería, García-Suárez, Moncada-Jiménez, Machado-Parra, Lira, Johnson and Jiménez-Maldonado. This is an open-access article distributed under the terms of the Creative Commons Attribution License (CC BY). The use, distribution or reproduction in other forums is permitted, provided the original author(s) and the copyright owner(s) are credited and that the original publication in this journal is cited, in accordance with accepted academic practice. No use, distribution or reproduction is permitted which does not comply with these terms.
*Correspondence: Alberto Jiménez-Maldonado, amltZW5lei5hbGJlcnRvODZAdWFiYy5lZHUubXg=
†These authors share first authorship
Disclaimer: All claims expressed in this article are solely those of the authors and do not necessarily represent those of their affiliated organizations, or those of the publisher, the editors and the reviewers. Any product that may be evaluated in this article or claim that may be made by its manufacturer is not guaranteed or endorsed by the publisher.
Research integrity at Frontiers
Learn more about the work of our research integrity team to safeguard the quality of each article we publish.