- 1Department of Otorhinolaryngology, Sichuan University Hospital of Sichuan University, Chengdu, China
- 2Department of Otorhinolaryngology-Head and Neck Surgery, West China Hospital of Sichuan University, Chengdu, China
- 3Department of Prevention and Health Care, Sichuan University Hospital of Sichuan University, Chengdu, China
- 4Department of Otorhinolaryngology-Head and Neck Surgery, Sichuan Provincial People’s Hospital, University of Electronic Science and Technology of China, Chengdu, China
Background: Although telomere length has a significant relationship with various age-related diseases, studies on its relationship with hearing status in adults are limited and equivocal. This study investigated the associations between mean telomere length (MTL) and low-, speech-, and high-frequency hearing threshold shifts of adults in the United States.
Methods: A total of 2,027 adults, aged 20-69 years, from the National Health and Nutrition Examination Surveys (NHANES, 1999–2002) were included in the analytic sample. The quantitative polymerase chain reaction method was used for the MTL assay, and MTL was expressed using the telomere-to-single copy gene (T/S) ratio. Hearing loss was defined as a pure-tone average (PTA) for the better ear at ≥ 20 dB HL at frequencies 500, 1,000, 2,000, and 4,000 Hz. Univariate and multivariate linear regression analyses and smooth curve fittings were conducted to evaluate the correlation between MTL and low-, speech-, and high-frequency hearing levels.
Results: The mean age of the participants was 40.60 ± 12.76 years, including 952 men (weighted, 48.67%) and 303 (weighted, 12.88%) participants with hearing loss. After adjusting for potential confounders in the multivariate linear regression model, the relationship between MTL and hearing thresholds was not statistically significant. Smooth curve fittings indicated a non-linear relationship between MTL and high-frequency PTA hearing threshold shifts. MTL was inversely related to high-frequency PTA to the turning point (T/S ratio = 0.82) (adjusted β−21.45, 95% CI −37.28, −5.62; P = 0.008). When the T/S ratio exceeded0.82, MTL was not associated with high-frequency PTA (adjusted β0.18, 95% CI −2.21, 2.57; P = 0.8809).
Conclusion: Our findings revealed that MTL was associated with high-frequency PTA hearing threshold shifts of adults in the United States in a non-linear manner.
Introduction
Hearing loss, which is associated with lifelong adverse health consequences and affects social and economic development, is the most common sensory disability in humans (Bowl et al., 2017; Cunningham and Tucci, 2017). It can originate in childhood (Vos et al., 2017) and is increasingly prevalent in adults (Agrawal et al., 2008). Genetic and environmental factors can cause hearing loss. Although genetic mutations, noise exposure, ototoxic drugs, and aging are the most studied risk factors for hearing loss, understanding of the underlying biological and molecular mechanisms of hearing loss is still limited.
Telomeres are repetitive DNA sequences (TTAGGG in vertebrates) and associated proteins at the ends of chromosomes for chromosomal stability and cellular integrity (Blackburn et al., 2015). They are considered biomarkers of aging because they shorten with every cell division (Chakravarti et al., 2021). Many age-associated diseases and disorders, such as hypertension, cognitive performance disorder, and cancer mortality, have been studied (Huang et al., 2020; Linghui et al., 2020; Shen et al., 2020). Oxidative stress and inflammation, which can promote hearing loss, are associated with telomere loss (von Zglinicki et al., 2001; Chester et al., 2021; Heba et al., 2021). Thus, telomere length may predispose hearing status and telomere loss may be associated with the biological mechanisms of hearing loss.
To date, the results of studies on the relationship between telomere length and hearing level are quite limited and inconsistent (Liu H. et al., 2017; Wang et al., 2019; Zhang et al., 2020). One study showed that longer telomere length was negatively related to hearing loss in older adults (Liu H. et al., 2017). In another study, telomere length was considered a predictive biomarker of hearing loss at an early stage (Zhang et al., 2020). However, telomere length was not correlated with hearing levels in children and midlife adults in a third study (Wang et al., 2019). Therefore, this study investigated the relationship between mean telomere length (MTL) and hearing level of adults in the United States using the National Health and Nutrition Examination Survey (NHANES) database.
Materials and Methods
Ethics Statement
Our study acquired publicly accessible data from the NHANES website1. The NHANES data were approved by the National Center for Health Statistics Institutional Review Board by the revised Declaration of Helsinki. Data collection procedures and examinations were performed after informed consent was obtained from all the eligible participants.
Study Population
The NHANES is a nationally representative survey approved by the Institutional Review Board of the National Center for Health Statistics. The survey combines a series of interviews, physical examinations, and laboratory tests to collect health-related information from the general population in the United States. The population in this study was enrolled in two cycles of NHANES (1999—2000, 2001–2002), as these are the only cycles containing results of the leukocyte telomere length test. A flow chart for the selection of study participants is shown in Figure 1. Audiometry examinations were performed in adults aged 20–69 years. Participants without complete data on hearing levels, otoscopic test, tympanogram test, or leukocyte telomere length measurement were excluded, as well as participants with abnormal otoscopic results, poor-quality tympanogram results, or tympanogram with compliance of ≤ 0.3ml. Finally, 2,027 adults were included in the study.
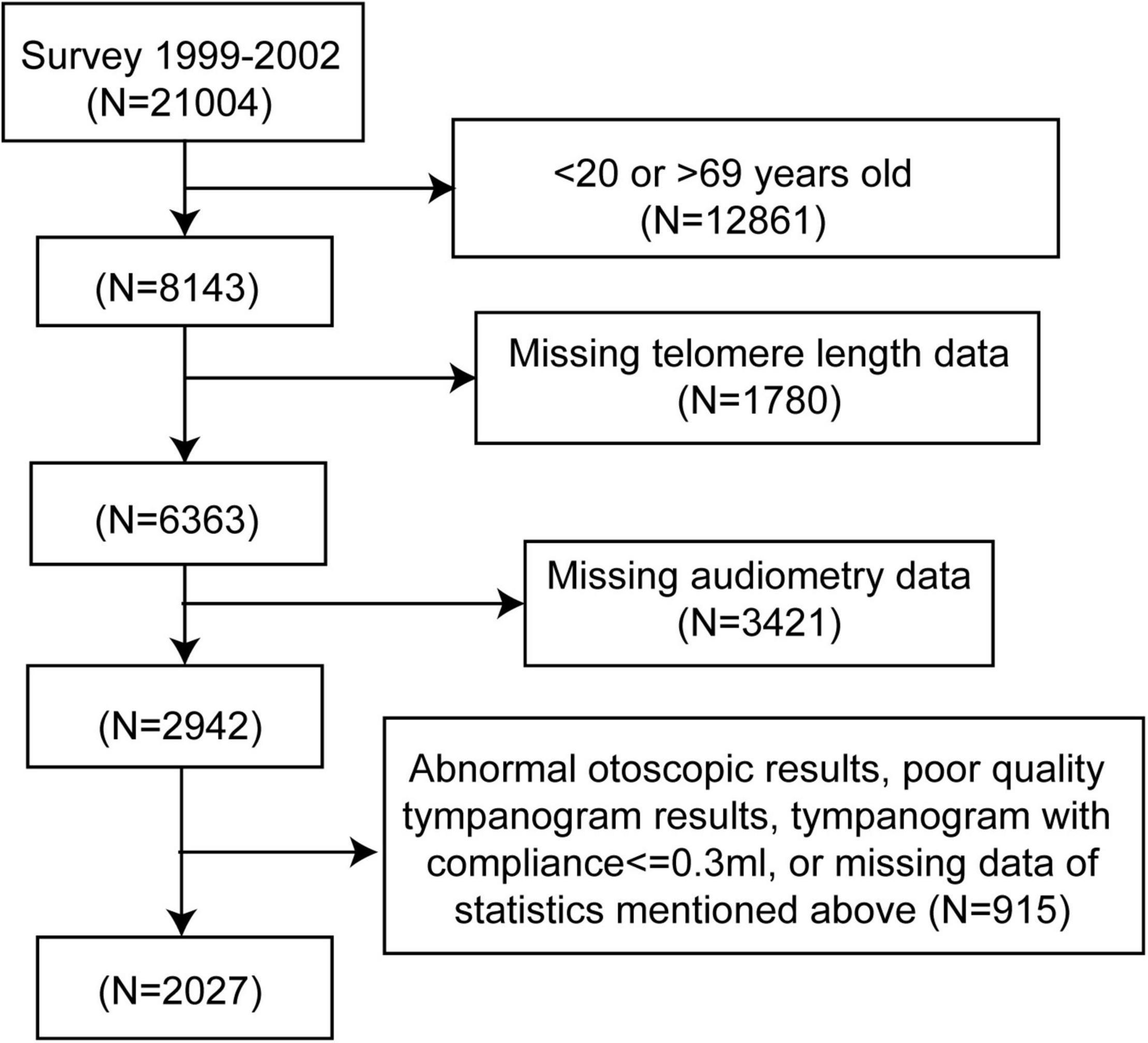
Figure 1. Flow chart of the selection process. NHANES, National Health and Nutrition Examination Survey.
Leukocyte Telomere Length Measurement
DNA from the participants was purified from whole blood and stored at −80°C using standardized procedures before the MTL assay measurement. The MTL assay was performed in the laboratory at the University of California, San Francisco, using the quantitative polymerase chain reaction-based method to measure telomere length relative to standard reference DNA (telomere-to-single copy gene [T/S] ratio), as previously described in detail (Cawthon, 2002; Lin et al., 2010; Needham et al., 2013). More details regarding the MTL quantification procedure and analytical methods are available on the NHANES website2.
Audiometric Measurement
Standardized air conduction pure-tone audiometric measurements were conducted in a sound-isolated room by trained and certificated audiologists. Air-conduction thresholds were tested in both ears of the participants at frequencies between 500 and 8,000 Hz, each with an intensity range of −10 to 120 dB. The hearing threshold was defined as the level at which participants were able to detect 50% of the signal. The 1,000 Hz frequency was tested twice in each ear to ensure quality and reliability (Su and Chan, 2017). Pure-tone average (PTA) hearing thresholds were regarded as: 0.5, 1, and 2 kHz low-frequency; 0.5, 1, 2, and 4 kHz speech-frequency; 4, 6, and 8 kHz high-frequency. Hearing impairment was defined as a hearing threshold of 20 dB or greater at speech-frequency PTA in the better ear (World Health Organization [WHO], 2021).
Covariates
Potential covariates considered in the analyses included age, body mass index (BMI), sex, race/ethnicity, education level, diabetes, hypertension, cigarette smoking, and noise exposure. Information on age, sex, race/ethnicity, education level, diabetes, and hypertension were obtained during the in-home interviews. Information on noise exposure was obtained from a pre-exam audiometric questionnaire. BMI data were recorded during the physical examination.
Diabetes was determined if the participants answered responded ‘yes’ to “other than during pregnancy, ever been told by a doctor or health professional had diabetes or sugar diabetes.” The answer of “borderline” was also considered diabetes (Szeto et al., 2021). Hypertension was determined by a positive reply to “ever been told by a doctor or other health professional had hypertension, also called high blood pressure” (Szeto et al., 2021). Smoking status was categorized as “ever” or “never” from the answers to the questions, “Have you smoked at least 100 cigarettes in your entire life?” and “Do you now smoke cigarettes?” (Szeto et al., 2021). Noise exposure was defined as “exposed to loud noise or listening to music with headphones in the past 24 h” (Ding and Park, 2020).
Statistical Analysis
The 1999/2000 and 2001/2002 cycles were combined, and audiometry subsample of 4-year Mobile Examination Center weights (WTSAU4YR) of the two cycles were used to estimate more representative measures for the general population of the United States following the NHANES analytic guidelines (Zipf et al., 2013). Categorical data are shown as percentages and continuous data are presented as means ± standard deviation (SD) according to baseline MTL in quartiles (Table 1). The P-value of continuous data was calculated by the weighted linear regression model and the P-value of categorical data was calculated by the weighted chi-square test. A univariate analysis was conducted to estimate potential variables (Table 2). A multivariate linear regression analysis was used to determine regression coefficients (β) and 95% confidence intervals (CIs) between MTL and low-, speech-, and high-frequency PTAs (Table 3). Three regression models were built to adjust for the relevant covariates. In the crude mode, no adjustments were included. In the model I, we adjusted for sex and age. In model II, sex, age, race, education level, BMI, noise exposure, hypertension, diabetes, and cigarette smoking status were adjusted. The βs and 95% CIs of low-, speech-, and high-frequency PTAs across each MTL/age, MTL/sex, and MTL/race subgroup were analyzed and their interactions were estimated. Smooth curve fittings were used to explore the relationship between MTL and hearing threshold shifts, with an adjustment for potential confounders (Figure 2). A two piecewise linear regression model was then conducted to examine the threshold effect of MTL on hearing threshold shifts in terms of the smoothing plot. The log-likelihood ratio test was performed to determine whether a threshold existed. The inflection point was calculated using the recursive method, and the maximum model likelihood was used. Statistical significance was set at P < 0.05. Statistical analyses were performed using the R statistical programming language 3.6.1 (R Foundation for Statistical Computing), and EmpowerStats software (X&Y Solutions, Inc.).
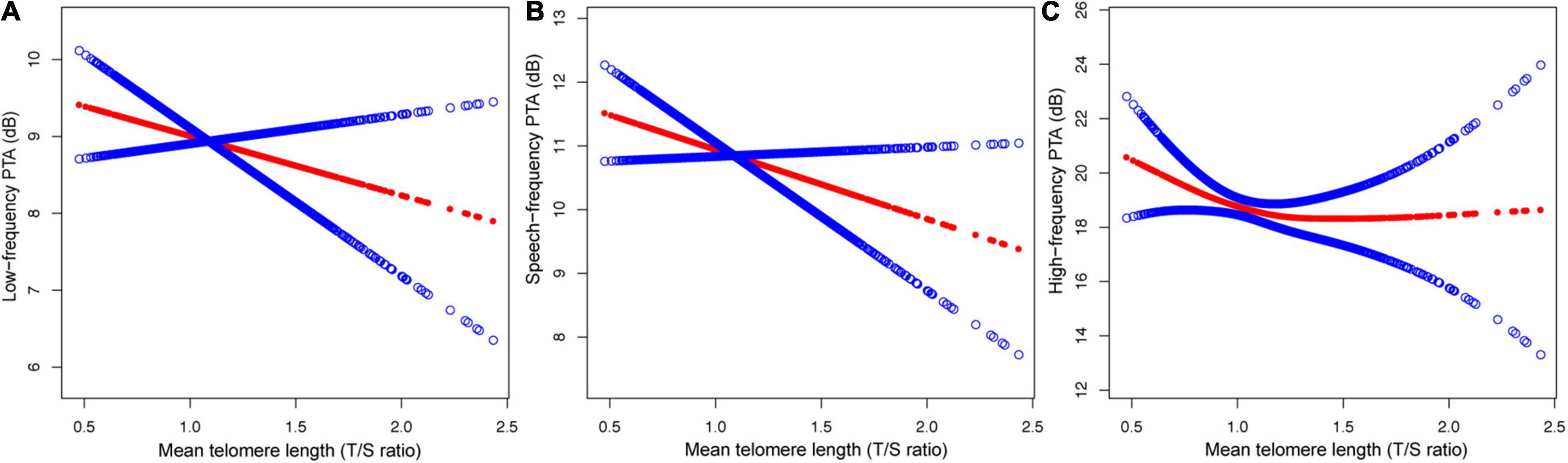
Figure 2. The relationship between MTL and hearing threshold shifts: (A) low-frequency PTA, (B) speech-frequency PTA and (C) high-frequency PTA.
Results
Characteristics of Participants
The baseline characteristics of the 2,027 participants (weighted mean, aged 40.60 ± 12.76 years) enrolled in this study were stratified by quartiles for MTL and are shown in Table 1. This study included 952 men (weighted, 48.67%) and 1,075 women (weighted, 51.33%). Of the participants, 1,367 (weighted, 64.2%) were above normal weight, 438 (weighted, 19.59%) with hypertension, and 148 (weighted, 5.94%) with diabetes. The means ± SD of low-frequency, speech-frequency, and high-frequency PTA hearing thresholds were 8.44 ± 7.30, 10.34 ± 8.70, and 18.25 ± 16.68 dB, respectively. There were 303 (weighted, 12.88%) participants with hearing loss. In addition, there were significant subgroup differences in age, low-, speech, and high-frequency PTAs, BMI, race/ethnicity, noise exposure, hypertension, diabetes, smoking status, and hearing loss rate (all P < 0.01).
Relationship Between MTL and Hearing Threshold Shifts
In a univariate analysis, sex, age, education level, BMI, hypertension, diabetes, cigarette smoking, and MTL (both continuous MTL and MTL in quartiles) were significantly associated with low-, speech, and high-frequency PTA hearing thresholds (all P < 0.01) (Table 2). To further explore the association between MTL and hearing threshold shifts, a multivariate regression analysis was conducted. As shown in Table 3, when treating MTL as a continuous variable (per 1 T/S increment) in the non-adjusted model (crude model), MTL was significantly associated with low-frequency (β = −5.01, 95% CI: −6.19, −3.84; P < 0.01), speech-frequency (β = −6.90, 95% CI: −8.29, −5.51; P < 0.01), and high-frequency (β = −12.99, 95% CI: −15.66, −10.31; P < 0.01) PTA hearing threshold shifts. However, in model II, there was no association between MTL and low-frequency (β = −1.12, 95% CI: −2.22, −0.01; P = 0.0474), speech-frequency (β = −1.20, 95% CI: −2.40, −0.00; P = 0.0503), and high-frequency (β = −1.09, 95% CI: −3.27, −1.09; P = 0.3278) PTA hearing threshold shifts. When the lowest quartiles of MTL was the referent, multivariate linear (low-, speech-, high-frequency PTAs) regression analyses demonstrated the βs for low-frequency PTA (βs0.46, 0.46, and −0.42 from the second to the fourth quartiles, respectively; P = 0.2938 for trend), speech-frequency PTA (βs0.33, 0.20, and −0.43, respectively, from the second to the fourth quartiles; P = 0.2962 for trend) and high-frequency PTA (βs −0.05, −0.46, and −0.25, from the second to the fourth quartiles, respectively; P = 0.6687 for trend) in the fully adjusted model (model II). MTL showed no statistically significant relationship with hearing threshold shifts after stratification by either age, sex, or race (Supplementary Tables 1–3).
Analyses of a Non-linear Relationship Between MTL and Hearing Threshold Shifts
After adjusting for potential confounders, including sex, age, race, education level, BMI, noise exposure, hypertension, diabetes, and cigarette smoking, a non-linear relationship between MTL and high-frequency PTA but not low-, or speech-frequency PTAs was observed (Figure 2). The inflection point was calculated using a two-piecewise linear regression model in the T/S ratio for the association with low-, speech-, and high-frequency PTA hearing threshold shifts to 1.14, 1.06, and 0.82, respectively. On the left of the inflection point, the βs for low-, speech-, and high frequency PTAs were −0.79 (95% CI: −1.45, 3.02; P = 0.4913), 0.26 (95% CI: −2.73, 3.25; P = 0.8661), and −21.45 (95% CI: −37.28, −5.62; P = 0.0080), respectively. The corresponding effect estimates at the right of inflection point were −2.56 (95% CI: −4.41, −0.72; P = 0.0065), −1.88 (95% CI: −3.64, −0.13; P = −0.0358), and0.18 (95% CI: −2.21, 2.57; P = 0.8809), respectively. The high-frequency PTA decreased with the T/S ratio up to the turning point (0.82). When the T/S ratio exceeded0.82, MTL was not associated with the high-frequency PTA (Table 4).
Discussion
The present nationwide cross-sectional study identified a relationship between telomere length and hearing threshold shifts of adults residing in the United States and indicated that MTL was inversely associated with the high-frequency PTA in a non-linear manner after adjusting for sex, age, race, education level, BMI, noise exposure, hypertension, diabetes, and cigarette smoking (Figure 2 and Table 4). To the best of our knowledge, it is the first cross-sectional study on the relationship between telomere length and hearing threshold shifts of adults in the United States. The findings of this study suggest that telomere length might be a potential predictive biomarker of hearing threshold shifts.
Mammalian telomeres are composed of tandem repeats of the hexanucleotide sequence TTAGGG and several DNA-binding proteins (Blackburn et al., 2015). Telomeres shorten during every cell division with the shortening rate varying among the species, suggesting that they are determinants of species’ life spans and have hallmarks of aging (Whittemore et al., 2019; Chakravarti et al., 2021). A large number of studies have been conducted to prove the correlation between MTL and age-related chronic diseases or disorders, such as cardiovascular disease, cognitive performance, and cancer mortality (Huang et al., 2020; Linghui et al., 2020; Shen et al., 2020; Hoffmann et al., 2021). However, studies on the relationship between MTL and hearing loss, which is a very common age-related chronic disorder, are quite limited, with results of inverse or negative associations between them (Liu H. et al., 2017; Wang et al., 2019; Zhang et al., 2020). Our results are rather consistent with the results of two previous case-control studies of the Chinese population (Liu X. et al., 2017; Zhang et al., 2020). However, no sex difference was observed in the role of telomere shortening in hearing loss in our study, which is inconsistent with a previous report (Zhang et al., 2020). Study designs and methods may be one of the reasons for the inconsistent results among different studies. Multiple factors, such as racial heterogeneity and environmental factors, different methods of auditory and MTL measurements, and different sample sizes should be considered (Aubert and Lansdorp, 2008; Ishikawa et al., 2016; Wang et al., 2019).
The MTL was inversely related to high-frequency PTA before a turning point (T/S ratio = −0.82). The possible mechanisms are as follows: first, telomere length is inversely associated with aging, and high-frequency PTA increases with age; and second, telomere length is closely related to inflammation, oxidative stress, and inhibition of DNA repair, which play an important role in the development of age-related hearing loss (Rizvi et al., 2014; Zhang et al., 2016). Oxidation damage, including cochlear DNA damage caused by reactive oxygen species (ROS), plays a causal role in the development of hearing loss (Someya et al., 2009). Due to the enrichment of the GGG triplet, telomeres are also highly sensitive to damage by ROS (Houben et al., 2008). Further studies among longitudinal cohorts across different life stages and measurement of MTL in the ear tissue are necessary to confirm these findings. Further experimental studies are needed to explore the mechanisms underlying these findings.
Our study has several strengths. The data in this study were obtained from a large and nationally representative sample from the NHANES, which were standardized and reliable. Participants with abnormal results of the otoscopic examination and poor-quality results in tympanogram or tympanogram compliance of ≤0.3 ml were excluded to avoid analyzing data for conductive or mixed hearing loss.
Despite these strengths, the limitations of this study should be considered. The results of this study were not validated because the NHANES is a cross-sectional study. The data representing noise exposure was from a pre-exam audiometric questionnaire involving noise exposure 24 h before the audiometric examination, which may not have reflected the accurate noise exposure status of participants. Some potential confounders were not calculated in the models.
Conclusion
According to the results of the NHANES data analyses, MTL was associated with high-frequency PTA hearing threshold shifts of adults in the United States in a non-linear manner. MTL might be a potential predictive biomarker for hearing loss.
Data Availability Statement
The original contributions presented in the study are included in the article/Supplementary Material, further inquiries can be directed to the corresponding author/s.
Ethics Statement
The studies involving human participants were reviewed and approved by National Center for Health Statistics Institutional Review Board. The patients/participants provided their written informed consent to participate in this study.
Author Contributions
LL made formal analysis of data and wrote the original draft. ZM completed the methodology. ZJ and XT completed the conceptualization, review and editing, revising, and final approval, and are accountable for all aspects. All authors have approved the final manuscript as submitted.
Conflict of Interest
The authors declare that the research was conducted in the absence of any commercial or financial relationships that could be construed as a potential conflict of interest.
The reviewer DL declared a shared affiliation with the author ZM to the handling editor at the time of review.
Publisher’s Note
All claims expressed in this article are solely those of the authors and do not necessarily represent those of their affiliated organizations, or those of the publisher, the editors and the reviewers. Any product that may be evaluated in this article, or claim that may be made by its manufacturer, is not guaranteed or endorsed by the publisher.
Supplementary Material
The Supplementary Material for this article can be found online at: https://www.frontiersin.org/articles/10.3389/fnagi.2022.770159/full#supplementary-material
Footnotes
References
Agrawal, Y., Platz, E. A., and Niparko, J. K. (2008). Prevalence of hearing loss and differences by demographic characteristics among US adults: data from the National Health and Nutrition Examination Survey, 1999–2004. Arch. Intern. Med. 168, 1522–1530. doi: 10.1001/archinte.168.14.1522
Aubert, G., and Lansdorp, P. M. (2008). Telomeres and aging. Physiol. Rev. 88, 557–579. doi: 10.1152/physrev.00026.2007
Blackburn, E. H., Epel, E. S., and Lin, J. (2015). Human telomere biology: a contributory and interactive factor in aging, disease risks, and protection. Science 350, 1193–1198. doi: 10.1126/science.aab3389
Bowl, M. R., Simon, M. M., Ingham, N. J., Greenaway, S., Santos, L., Cater, H., et al. (2017). A large scale hearing loss screen reveals an extensive unexplored genetic landscape for auditory dysfunction. Nat. Commun. 8:886. doi: 10.1038/s41467-017-00595-4
Cawthon, R. M. (2002). Telomere measurement by quantitative PCR. Nucleic Acids Res. 30:e47. doi: 10.1093/nar/30.10.e47
Chakravarti, D., LaBella, K. A., and DePinho, R. A. (2021). Telomeres: history, health, and hallmarks of aging. Cell 184, 306–322. doi: 10.1016/j.cell.2020.12.028
Chester, J., Johnston, E., Walker, D., Jones, M., Ionescu, C. M., Wagle, S. R., et al. (2021). A Review on Recent Advancement on Age-Related Hearing Loss: the Applications of Nanotechnology, Drug Pharmacology, and Biotechnology. Pharmaceutics 13:1041. doi: 10.3390/pharmaceutics13071041
Cunningham, L. L., and Tucci, D. L. (2017). Hearing Loss in Adults. N. Engl. J. Med. 377, 2465–2473. doi: 10.1056/NEJMra1616601
Ding, N., and Park, S. K. (2020). Perfluoroalkyl substances exposure and hearing impairment in US adults. Environ. Res. 187:109686. doi: 10.1016/j.envres.2020.109686
Heba, A.-C., Toupance, S., Arnone, D., Peyrin-Biroulet, L., Benetos, A., and Ndiaye, N. C. (2021). Telomeres: new players in immune-mediated inflammatory diseases? J. Autoimmun. 123:102699. doi: 10.1016/j.jaut.2021.102699
Hoffmann, J., Richardson, G., Haendeler, J., Altschmied, J., Andrés, V., and Spyridopoulos, I. (2021). Telomerase as a Therapeutic Target in Cardiovascular Disease. Arterioscler. Thromb. Vasc. Biol. 41, 1047–1061. doi: 10.1161/ATVBAHA.120.315695
Houben, J. M. J., Moonen, H. J. J., van Schooten, F. J., and Hageman, G. J. (2008). Telomere length assessment: biomarker of chronic oxidative stress? Free Radic. Biol. Med. 44, 235–246. doi: 10.1016/j.freeradbiomed.2007.10.001
Huang, Y.-Q., Liu, L., Lo, K., Huang, J.-Y., Zhang, B., and Feng, Y.-Q. (2020). The relationship between mean telomere length and blood pressure: results from the National Health and Nutrition Examination Surveys. Ann. Transl. Med. 8, 535–535. doi: 10.21037/atm.2020.03.205
Ishikawa, N., Nakamura, K.-I., Izumiyama-Shimomura, N., Aida, J., Matsuda, Y., Arai, T., et al. (2016). Changes of telomere status with aging: an update. Geriatr. Gerontol. Int. 16, 30–42. doi: 10.1111/ggi.12772
Lin, J., Epel, E., Cheon, J., Kroenke, C., Sinclair, E., Bigos, M., et al. (2010). Analyses and comparisons of telomerase activity and telomere length in human T and B cells: insights for epidemiology of telomere maintenance. J. Immunol. Methods 352, 71–80. doi: 10.1016/j.jim.2009.09.012
Linghui, D., Shi, Q., Chi, C., Xiaolei, L., Lixing, Z., Zhiliang, Z., et al. (2020). The Association Between Leukocyte Telomere Length and Cognitive Performance Among the American Elderly. Front. Aging Neurosci. 12:527658. doi: 10.3389/fnagi.2020.527658
Liu, H., Luo, H., Yang, T., Wu, H., and Chen, D. (2017). Association of leukocyte telomere length and the risk of age-related hearing impairment in Chinese Hans. Sci. Rep. 7:10106. doi: 10.1038/s41598-017-10680-9
Liu, X., Yao, L. U., and H, Liu, and Yun, H. E. (2017). Correlation between telomere length of peripheral blood leukocytes and noise-induced hearing loss. Carcinog. Teratogen. Mutagen. 29, 222–225.
Needham, B. L., Adler, N., Gregorich, S., Rehkopf, D., Lin, J., Blackburn, E. H., et al. (2013). Socioeconomic status, health behavior, and leukocyte telomere length in the National Health and Nutrition Examination Survey, 1999–2002. Soc. Sci. Med. 85, 1–8. doi: 10.1016/j.socscimed.2013.02.023
Rizvi, S., Raza, S. T., and Mahdi, F. (2014). Telomere length variations in aging and age-related diseases. Curr. Aging Sci. 7, 161–167. doi: 10.2174/1874609808666150122153151
Shen, G., Huang, J.-Y., Huang, Y.-Q., and Feng, Y. (2020). The Relationship between Telomere Length and Cancer Mortality: data from the 1999–2002 National Healthy and Nutrition Examination Survey (NHANES). J. Nutr. Health Aging 24, 9–15. doi: 10.1007/s12603-019-1265-z
Someya, S., Xu, J., Kondo, K., Ding, D., Salvi, R. J., Yamasoba, T., et al. (2009). Age-related hearing loss in C57BL/6J mice is mediated by Bak-dependent mitochondrial apoptosis. Proc. Natl. Acad. Sci. U. S. A. 106, 19432–19437. doi: 10.1073/pnas.0908786106
Su, B. M., and Chan, D. K. (2017). Prevalence of Hearing Loss in US Children and Adolescents: findings From NHANES 1988-2010. JAMA Otolaryngol. Head Neck Surg. 143, 920–927. doi: 10.1001/jamaoto.2017.0953
Szeto, B., Valentini, C., and Lalwani, A. K. (2021). Low vitamin D status is associated with hearing loss in the elderly: a cross-sectional study. Am. J. Clin. Nutr. 113, 456–466. doi: 10.1093/ajcn/nqaa310
von Zglinicki, T., Bürkle, A., and Kirkwood, T. B. (2001). Stress, DNA damage and ageing—An integrative approach. Exp. Gerontol. 36, 1049–1062. doi: 10.1016/s0531-5565(01)00111-5
Vos, T., Abajobir, A. A., Abate, K. H., Abbafati, C., Abbas, K. M., Abd-Allah, F., et al. (2017). Global, regional, and national incidence, prevalence, and years lived with disability for 328 diseases and injuries for 195 countries, 1990–2016: a systematic analysis for the Global Burden of Disease Study 2016. Lancet 390, 1211–1259. doi: 10.1016/S0140-6736(17)32154-2
Wang, J., Nguyen, M. T., Sung, V., Grobler, A., Burgner, D., Saffery, R., et al. (2019). Associations Between Telomere Length and Hearing Status in Mid-Childhood and Midlife: population-Based Cross-Sectional study. Ear Hear. 40, 1256–1259. doi: 10.1097/AUD.0000000000000705
Whittemore, K., Vera, E., Martínez-Nevado, E., Sanpera, C., and Blasco, M. A. (2019). Telomere shortening rate predicts species life span. Proc. Natl. Acad. Sci. U. S. A. 116, 15122–15127. doi: 10.1073/pnas.1902452116
Zhang, H., Wang, D., Ma, H., Li, C., Wang, S., Wang, Y., et al. (2020). Association between Leucocyte Telomere Length and Risk of Hearing Loss in the General Population: a Case-Control Study in Zhejiang Province, China. Int. J. Environ. Res. Public Health 17:1881. doi: 10.3390/ijerph17061881
Zhang, J., Rane, G., Dai, X., Shanmugam, M. K., Arfuso, F., Samy, R. P., et al. (2016). Ageing and the telomere connection: an intimate relationship with inflammation. Ageing Res. Rev. 25, 55–69. doi: 10.1016/j.arr.2015.11.006
Keywords: telomere length, hearing threshold shift, National Health and Nutrition Examination Survey, cross-sectional study, adults
Citation: Long L, Meng Z, Jia Z and Tang X (2022) Exploring the Association of Leukocyte Telomere Length and Hearing Threshold Shifts of Adults in the United States. Front. Aging Neurosci. 14:770159. doi: 10.3389/fnagi.2022.770159
Received: 03 September 2021; Accepted: 25 April 2022;
Published: 03 June 2022.
Edited by:
Jiehui Jiang, Shanghai University, ChinaReviewed by:
Deng Linghui, Sichuan University, ChinaWoon-Man Kung, Chinese Culture University, Taiwan
Can Sheng, Capital Medical University, China
Copyright © 2022 Long, Meng, Jia and Tang. This is an open-access article distributed under the terms of the Creative Commons Attribution License (CC BY). The use, distribution or reproduction in other forums is permitted, provided the original author(s) and the copyright owner(s) are credited and that the original publication in this journal is cited, in accordance with accepted academic practice. No use, distribution or reproduction is permitted which does not comply with these terms.
*Correspondence: Zhenchao Jia, emhlbmNoYW8tMUAxNjMuY29t; Xinghua Tang, dGFuZ3hpbmdodWFAbWVkLnVlc3RjLmVkdS5jbg==