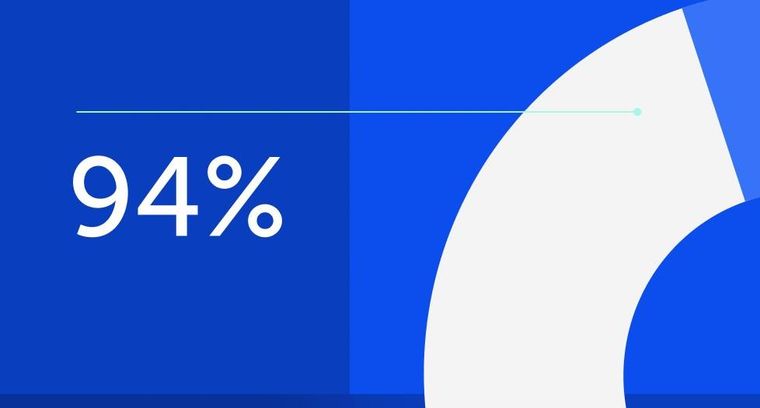
94% of researchers rate our articles as excellent or good
Learn more about the work of our research integrity team to safeguard the quality of each article we publish.
Find out more
ORIGINAL RESEARCH article
Front. Aging Neurosci., 23 September 2022
Sec. Cellular and Molecular Mechanisms of Brain-aging
Volume 14 - 2022 | https://doi.org/10.3389/fnagi.2022.1006473
This article is part of the Research TopicThe UN International Day of Families: Neurodegeneration as a Result of Genetic InheritanceView all 10 articles
Background: Thrombospondin-1 (THBS1) derived from platelets and acted as a critical mediator of hemostasis promoting platelet activation in thrombus formation. The biological connection of genetic variants and mRNA expression of THBS1 with ischemic stroke (IS) warrants further validation with population-based evidence.
Objective: To evaluate the association of single nucleotide polymorphisms (SNPs) and mRNA expression of THBS1 with the risks of IS and long-term death after stroke.
Methods: A case-control study consisted of 4,584 IS patients recruited from five hospitals in Jiangsu, China, and 4,663 age-gender-matched controls free of IS. A cohort study enrolled 4,098 participants free of stroke and lasted from 2009 to 2022. Early collected 3158 IS patients aged between 35 and 80 years were followed up an average of 5.86-year to follow up their long-term death outcomes. Two tagSNPs of the THBS1 gene, rs2236471 and rs3743125, were genotyped in all subjects and THBS1 mRNA expression of peripheral leukocyte was measured using RT-qPCR in 314 IS cases and 314 controls.
Results: There is no significant difference in genotype and haplotype frequencies of rs2236741 and rs3743125 between IS cases and controls (all P > 0.05). Furthermore, the cohort studies did not observe significant associations between THBS1 variants and the risk of IS incidence or long-term death after IS (all P > 0.05). The THBS1 mRNA expression level (2–Δ Δ CT) in IS cases was approximately equal to that in controls (1.01 vs. 0.99, P = 0.833). In addition, THBS1 mRNA expression had no significant association with all-cause death, stroke death, and IS death of IS patients (all P > 0.05).
Conclusion: Therefore, our study suggested that there is no significant association of THBS1 polymorphisms and mRNA expression level with the risk of IS and long-term death after IS.
Stroke affects 13.7 million people worldwide every year and it is the second leading cause of death, with 5.5 million people dying from it annually (Feigin et al., 2018). The main subtypes of stroke include ischemic stroke (IS) and hemorrhagic stroke (HS). IS, making up ∼71% of all strokes, was defined as infarction of the brain, spinal cord, or retina (Virani et al., 2021). As the 2020 report on cardiovascular health and diseases burden in China, the number of patients suffering from stroke in China was about 13 million at present and the deaths of stroke among Chinese residents accounted for 22.33% of the total deaths (Xiao et al., 2021). Thus, exploring the cause of stroke is important as it can guide therapeutic strategies for the prevention of stroke.
Previous studies presented that a history of hypertension or diabetes mellitus, high levels of blood pressure, smoking, high alcohol consumption were considered as the modifiable risk factors for IS (O’Donnell et al., 2010), while age, gender, and genetic factors are non-modifiable risk factors (Campbell et al., 2019). The estimated heritability of IS was about 37.9% when calculated by current genome-wide complex trait analysis (Bevan et al., 2012). Therefore, the missing heritability of IS and gene-environment interaction remain further exploration.
Thrombospondin-1 (THBS1) is a multifunctional glycoprotein released from platelets, macrophages, and adipocytes (Baenziger et al., 1972; Jaffe et al., 1985; Varma et al., 2008). Endogenous THBS1 is necessary for platelet aggregation and adhesion by overcoming the antithrombotic activity of physiologic nitric oxid (NO) (Isenberg et al., 2008). Besides, THBS1 is a critical mediator of hemostasis that promotes platelet activation by modulating inhibitory cyclic adenosine monophosphate (cAMP) signaling at sites of vascular injury (Aburima et al., 2021). Additionally, THBS1 participates in a wide range of physiological and pathological processes such as tissue remodeling, wound healing, angiogenesis, and inflammation (Sweetwyne and Murphy-Ullrich, 2012). THBS1 is also known to regulate the activation of transforming growth factor-β1 (TGF-β1) (Sweetwyne and Murphy-Ullrich, 2012), which makes a difference in restenosis after angioplasty, atherosclerosis, and angiogenesis (August and Suthanthiran, 2006). Above all functions may be involved in the pathophysiology of IS, with common sources of embolism being large artery atherosclerosis (Campbell et al., 2019).
Previous studies showed that genetic variants in the THBS1 gene were associated with variation in pulmonary artery systolic pressure (Jacob et al., 2017), and chronic ocular surface inflammation after refractive surgery (Contreras-Ruiz et al., 2014). Besides, the coding polymorphism G1678A (rs2292305) was identified as a susceptible locus for cerebral thrombosis in a Chinese population (Liu et al., 2004) and the THBS1 CT genotype was associated with a reduced risk of developing gastric cancer (Hong et al., 2015). Furthermore, the visceral THBS1 mRNA expression was positively associated with abdominal obesity, hyperglycemia, and hypertension (Matsuo et al., 2015). Additionally, plasma THBS1 level elevated in patients with IS compared to the controls (Gao et al., 2015) as well as at 2h after tPA-treatment (Navarro-Sobrino et al., 2011). Thus, the serum THBS1 level was proposed to be an independent predictor of favorable outcome at baseline and after 6 months (Gao et al., 2015; Al Qawasmeh et al., 2020). Therefore, further population-based studies would be warranted to validate the association between THBS1 and IS.
Therefore, this research aimed to investigate whether the genetic variants and mRNA expression of THBS1 was associated with the susceptibility to developing IS and the long-term death after stroke by conducting the case-control and cohort studies in the Chinese population.
A total of 4,584 IS cases were recruited from five different hospitals in Jiangsu province, China, which included Jurong People’s Hospital, Nanjing Jiangning Hospital, Yixing People’s Hospital, the Affiliated Hospital of Xuzhou Medical University, and Huai’an First People’s Hospital from 2009 to 2021. Included patients were diagnosed according to the computed tomography (CT), magnetic resonance imaging (MRI), or angiography with symptoms lasting more than 24 h. Excluded patients were those who had non-atherosclerotic ischemic stroke, acute coronary syndrome, tumor, autoimmune disease, or severe kidney failure according to the patient’s history of disease, admission diagnosis and discharge diagnosis. Besides, we use the TOAST (Trial of ORG 10172 in Acute Stroke Treatment) criteria to group the IS subjects. The TOAST classification denotes five subtypes of ischemic stroke: large-artery atherosclerosis (LAA), cardioembolism (CE), small-vessel occlusion (SVO), stroke of other determined etiology (SOE), and stroke of undetermined etiology (SUE). 4,663 controls free of stroke were randomly sampled from local communities and matched to the cases on age (±2 years) and gender. Subjects with acute coronary syndrome, tumor, autoimmune disease, and severe kidney failure were also excluded from the study. Additionally, we chose 314 pairs of IS cases and controls to detect THBS1 mRNA expression from 2019 to 2021. IS cases had a definite TOAST type and qualified retention samples. The controls were selected from the cohorts in the same or adjacent areas of IS cases using age- and gender- matching method. For above 314 IS patients, we collected the National Institutes of Health Stroke Scale (NIHSS) score and modified Rankin scale (mRS) score of IS patients when discharge, and followed-up their NIHSS scores and mRS scores at 1, 3, and 6 months after discharge. Demographic and clinical characteristics of the study subjects in above two parts were listed in Table 1.
This research incorporated two prospective cohort studies. The community-based cohort study was conducted from 2009 to 2022, which recruited 4128 participants from Guanlin Town and Xushe Town, Yixing city (Jiangsu, China). 4098 baseline subjects without stroke were followed up until May 25, 2022 for stroke onset. The detailed information about this cohort has been described previously (Dong et al., 2021).
The hospital-based cohort study enrolled 3158 IS patients aged between 35 and 80 years from Yixing People’s Hospital and followed an average of 5.86-year to record their long-term death outcome based on the annual death data from Yixing Center for Disease Control and Prevention. The follow-up period ended on May 25, 2022. The four endpoints were all-cause death, stroke death, IS death, and HS death. Finally, we observed a total of 488 deaths, among which 245 died from stroke and over half of the stroke-induced deaths were attributed to IS (n = 161). Meanwhile, we collected outcome of the long-term death from local Centers for Disease Control and Prevention (CDC) to assessment the prognosis of IS patients comprehensively, including all-cause death, stroke death, and IS death. Followed up to May 13, 2022, we observed a total of 28 deaths. Of the 20 deaths from stroke, 13 deaths were attributed to IS.
Demographic and clinical characteristics of the study population in the two cohort studies were summarized in Supplementary Table 2.
The case-control and cohort studies were all approved by the Research Ethics Committee of Nanjing Medical University (#2018571) and all participants signed the informed consent voluntarily.
Demographic characteristics, smoking and drinking habits, diseases history, and medication history were collected by questionnaire survey. All investigators were trained and qualified uniformly. Subjects who have ever smoked one pack of cigarettes a day in the past were considered smokers. Drinker was defined as individuals drinking alcohol at least three times daily. Physical examination including systolic blood pressure (SBP, mmHg) and diastolic blood pressure (DBP, mmHg) were measured at least three times.
Hypertension was defined as a self-reported history of hypertension, or elevated levels of blood pressure (SBP ≥ 140 mmHg, DBP ≥ 90 mmHg), or taking antihypertensive drugs recently. Those with fasting blood glucose level (GLU) ≥ 7.0 mmol/L, or self-reported history of diabetes, or taking hypoglycemic drugs were considered as diabetes. Dyslipidemia was defined as abnormal changes in lipid levels [total cholesterol (TC) ≥ 6.2 mmol/L, triglyceride (TG) ≥ 2.3 mmol/L, low-density lipoprotein-cholesterol (LDL-C) ≥ 4.1 mmol/L, high-density lipoprotein-cholesterol (HDL-C) < 1.04 mmol/L], or self-reported diagnosis of dyslipidemia, or currently taking lipid-lowering drugs.
Venous peripheral blood was collected from each subject after 8 h from the last meal into vacuum anticoagulation tubes with ethylene diamine tetraacetic acid dipotassium salt (EDTA-K2) and stored at –20°C. Leukocytes were obtained by gradient centrifugation from a subgroup of the study population. GLU was measured using the glucose oxidase method. TC was detected by the cholesterol oxidase-peroxidase method. TG was detected by the glycerophosphate oxidase-peroxidase method. HDL-C was detected by the catalase removal method and LDL-C was measured by sulfuric acid precipitation method.
The THBS1 gene (Gene ID: 7057, Locus NC_000015.10) locates on chromosome 15q14 and spans 18,388 bp, and consists of 22 exons. We searched the SNPs from the upstream 2 kb to the downstream 1 kb and selected tagging SNPs (tagSNPs) through the database of the Chinese Han population in Beijing (CHB) and China of the International Hap MAP Project. Three tagSNPs (tagSNPs rs2292305, etc. tagSNP rs2236741, and tagSNP rs2292304) would be available for candidate SNP selection. Included tagSNPs met the criteria of minor allele frequency (MAF) ≥ 0.05 and linkage disequilibrium (LD) r2 ≥ 0.8. A functional candidate strategy was also applied to select potential functional SNPs on the bioinformatics effect prediction website SNPinfo Web Server.1 The SNP function prediction results of rs2292305 showed no informed predictive biological function, therefore, we selected the closely linked tagSNP rs3743125 (r2 = 0.932) with a prior predictive biological function as the substitute. We did not include rs2292304 because its probes and primers were not designed successfully. Finally, two tagSNPs of THBS1 gene, rs2236471 (C > T) and rs3743125 (G > A) were selected and genotyped in this study. Detailed biological information and function prediction were summarized in Supplementary Table 1.
DNA was extracted from the unfrozen venous peripheral blood using the protein precipitation method (Eaglink, EGEN2024, China) and then preserved at –20°C. Each DNA sample was quantified using the NanoDrop 2000 spectrophotometer (Thermo Fisher Scientific, Waltham, MA). The polymerase chain reaction (PCR) TaqMan MGB probe assay was performed to amplify the two SNPs in the GeneAmp® PCR system 9700 thermal cycle (Applied Biosystems, Foster City, CA). The results were post-read on the 7900HT real-time PCR system (Applied Biosystems, Foster City, CA) with the Sequence Detection System (SDS) 2.4 software. The successful call rates of two SNPs genotyping were both 100%.
White blood cells were separated from peripheral blood by gradient centrifugation and stored at –20°C. Peripheral leukocyte was reserved in RNA protective additive (Eaglink, EGEN2026, China) at –20°C. Total RNA was extracted using the Whole Blood RNA Extraction Kit (Yuan, Yu-BR02-1, China) and quantified using a NanoDrop 2000 spectrophotometer (Thermo Fisher Scientific, Waltham, MA). Isolated RNA was reversely transcribed into cDNA using the PrimeScript™ RT Reagent Kit (Takara, RR047A, Japan). THBS1 gene mRNA expression of peripheral leukocyte was measured using SYBR Green quantitative real-time polymerase chain reaction (RT-qPCR), and the housekeeping gene Glyceraldehyde-3-Phosphate Dehydrogenase (GAPDH) was tested as an endogenous control. The qPCR reaction system included Platinum® SYBR® Green qPCR SuperMix-UDG (Invitrogen, 11733-046, USA), RNase free dH2O, forward primer, reverse primer, and cDNA. Samples were incubated at 95°C for 5 min, followed by 40 cycles of 95°C for 10 s, 57°C for 20 s, and 72°C for 20 s on the QuantStudio™ 7 Flex Real-Time PCR System platform (Applied Biosystems, 4485700, CA). All samples were analyzed in three parallels, and cycle threshold values were recorded. The 2–ΔΔCT method was used to calculate relative expression levels of THBS1 normalized by GAPDH. THBS1 mRNA’s forward primer sequence (5′–3′) was AGACTCCGCATCGCAAAGG, and the reverse primer sequence (5′–3′) was TCACCACGTTGTTGTCAAGGG. GAPDH mRNA’s forward primer sequence (5′–3′) was GGAGCGAGATCCCTCCAAAAT, and the reverse primer sequence (5′–3′) was GGCTGTTGTCATACTTCTCATGG.
We used EpiData 3.1 software (The EpiData Association, Odense, Denmark) for duplicate entry and consistency check of the collected data. Continuous variables were presented as median [inter-quartile range (IQR)] for non-parametric data. Categorical variables were presented as frequencies and percentages. For group-wise comparisons, the Kruskal–Wallis test or Mann–Whitney test was used for continuous variables with abnormal distribution. The chi-square test (χ2) was used to compare the differences in categorical variables between case and control groups. The Fisher’s exact test was used to estimate whether the genotype frequencies in controls and IS group met the Hardy–Weinberg equilibrium (HWE) law. Binary logistic regression was applied to calculate the odds ratios (ORs) and corresponding 95% confidence intervals (CIs) for the association of THBS1 variants and IS with adjustment for covariates (age, gender, smoking, drinking, hypertension, diabetes, and dyslipidemia). We used Cox proportional hazard regression to estimate the association with hazard ratios (HRs) and 95% CIs as well as after adjustment for covariates in the cohort study. Kruskal-Wallis H test was conducted to test the trend in mRNA levels among different groups of SNP genotypes. We used Spearman’s rank correlation to evaluate the correlations between THBS1 mRNA expression and NIHSS scores and MRS Scores after discharge in IS cases. We also used restricted cubic splines (RCS) with four knots at the 20th, 40th, 60th, and 80th centiles to flexibly model the association of THBS1 mRNA expression with the risk of long-term deaths in IS patients.
Haplotype association analyses as outlined by Schaid et al. (2002) were performed to test the associations of statistically inferred Haplotype with IS weighted with their estimated probability. All data analyses were carried out using SAS software 9.4 (SAS Inc., Cary, N.C., USA) and R 4.1.1 version.2 A two−tailed P-value < 0.05 was considered statistically significant.
Table 1 shows the detailed demographic and clinical characteristics of 4,584 IS cases and 4,663 matched controls in the genetic case-control study. The median age of IS group (66 years) was comparable with that of the control group (66 years, P = 0.355). IS group had higher proportions of male (59.4%) and smokers (22.1%) while a lower proportion of drinkers (13.4%) than control group (41.6, 20.1, and 19.6%; P < 0.05). There were significant differences in SBP, DBP, GLU, TC, HDL-C, and LDL-C levels (P < 0.001) between the IS cases and controls. IS group had higher prevalence of hypertension (84.0%), diabetes (27.4%), and dyslipidemia (41.3%) than controls (50.1, 14.2 and 38.0%; P ≤ 0.001).
In the case-control study for transcriptome level analysis, IS group presented higher level of SBP but lower levels of GLU, TC, and HDL-C than control group (Table 1). Age and gender were both matched for case and control. IS group had lower proportions of smokers (15.3%) and drinkers (9.9%) than control group (26.1% and 29.9%; P ≤ 0.001). IS group had higher prevalence of hypertension (86.6%) while a lower prevalence of dyslipidemia (23.2%) than control group (72.9 and 51.6%; P < 0.001). Table 1 also listed the characteristics of participants in the cohort study for the risk of IS incidence. The clinical characteristic of the follow-up study for the long-term death after IS were presented in Supplementary Table 2.
As shown in Table 2, the genotype and allele distributions of SNP rs2236741 followed HWE both in the control and IS groups (both P-values > 0.05). Even though we have double-checked the genotyping results and controlled the quality by comparing the genotype frequencies of cases and controls of each plate, the allele frequencies of rs3743125 did not accord with the HWE in controls (P = 0.024) but accord with HWE in IS cases (P = 0.098).
Table 2. Association analyses of THBS1 variants and the risk of ischemic stroke in the case-control study.
No significant association of the two tagSNPs at THBS1 with IS was observed in the case-control study (Supplementary Table 3). The adjusted ORs (95%CIs) for the addictive model of rs2236741 and rs3743125 were 0.955 (0.872–1.047) and 0.955 (0.890–1.025) after adjustment for covariates. No significant association was observed for the dominant and recessive models of the two SNPs with IS (Table 2). Further stratification analyses by age, gender, smoking, drinking, hypertension, diabetes, and dyslipidemia did not reveal any significant association (Supplementary Tables 4, 5). There was no significant association between THBS1 variants and TOAST subtypes of IS in the case-control study (Supplementary Table 6).
Compared with C-G haplotype of rs2236741 and rs3743125, the haplotype C-A, T-G, and T-A were identified to have no significant association with IS (Supplementary Table 7), even after adjustment for covariates [Adjusted ORs (95%CIs): 0.974 (0.894–1.062), 1.103 (0.829–1.469), and 0.933 (0.845–1.030), respectively] (Table 3). In addition, the haplotypes C-A, T-G, and T-A were not associated with LAA and SVO (Supplementary Table 8).
Table 3. Haplotype frequencies of rs2236741–rs3743125 and association analyses with ischemic stroke.
After an average of 10.15-year follow-up for 4098 subjects free of stroke at baseline, 319 incident IS cases (10.30%) were observed and the incidence density was 63.08 (per 104 person-year). The variants of rs2236741 and rs3743125 were not associated with the incident risk of IS (Supplementary Table 9), even after adjustment for covariates [Adjusted HRs (95%CIs) for the addictive model: 1.025 (0.821–1.281) and 0.984 (0.829–1.168)] (Table 4).
Table 4. Association analyses of THBS1 variants with the incidence risk of ischemic stroke in the cohort study.
The variants of rs2236741 and rs3743125 were not associated with the long-term death after IS (Supplementary Table 10). Adjusted HRs (95%CIs) of the addictive model of rs2236741 and rs3743125 for all-cause death, stroke death, IS death, and HS death were 1.014 (0.848–1.212), 1.052 (0.820–1.349), 0.990 (0.723–1.356), 0.948 (0.553–1.626), and adjusted HRs (95%CIs) of the addictive model of rs3743125 for all-cause death, stroke death, IS death, and HS death were 1.125 (0.982–1.289), 0.985 (0.809–1.199), 1.030 (0.810–1.310), 0.887 (0.583–1.351), respectively. The dominant and recessive models of the two SNPs had no association with the long-term death either (Table 5).
The mRNA expression level of THBS1 in IS cases was approximately equal to that in controls (1.01 vs. 0.99, P = 0.833). Further subgroup analyses by age, gender, smoking, drinking, hypertension, diabetes, and dyslipidemia did not observed significant differences in THBS1 mRNA expression was detected between IS cases and controls (Supplementary Table 11). Additionally, no significant difference in THBS1 mRNA expression was detected between the three TOAST subgroup of IS and control group (Figure 1). As shown in Figure 2, the THBS1 mRNA expression levels significantly differed across rs3743125 GG, GA, and AA carriers in the control group (P = 0.016), but not in IS group (P = 0.454).
Figure 1. The mRNA expression of THBS1 common variants in IS cases with different TOAST types and controls. The mRNA expression level of THBS1 in IS cases was approximately equal to that in controls (1.01 vs. 0.99, P = 0.833). Additionally, no significant difference in the THBS1 mRNA expression was detected between the three TOAST subgroups of IS cases and control group [LAA vs. controls: (1.09 vs. 0.99, P = 0.205), CE vs. controls: (0.96 vs. 0.99, P = 0.960), SVO vs. controls: (0.96 vs. 0.99, P = 0.183)].
Figure 2. THBS1 gene mRNA expression (2– ΔΔCT) among different genotypes of rs2236741 and rs3743125. The THBS1 mRNA expression levels significantly differed across rs3743125 GG, GA, and AA carriers in the control group (0.91 vs. 1.08 vs. 0.89, P = 0.016) but were not significantly different in IS group (0.97 vs. 1.00 vs. 1.25, P = 0.454). Besides, there were no significant differences across rs2236741 CC, CT, and TT carriers in the control group (1.02 vs. 0.97 vs. 0.54, P = 0.225) or in the IS cases (1.00 vs. 1.04 vs. 0.79, P = 0.709).
RCS regression analyses did not identify significant linear or non-linear correlation between THBS1 mRNA expression and the risk of all-cause death, stroke death, and IS death in IS patients (Figure 3). The adjusted HRs (95%CIs) for all-cause death, stroke death, and IS death were 0.962 (0.842–1.099), 0.986 (0.908–1.071), 0.975 (0.821–1.158), respectively (Supplementary Table 12). Furthermore, THBS1 mRNA expression has no significant relevance to NIHSS scores of discharges (ρ = –0.042, P = 0.462), 1 month after discharge (ρ = 0.064, P = 0.445), 3 months after discharge (ρ = 0.044, P = 0.601), and 6 months after discharge (ρ = 0.065, P = 0.441) in IS cases (Figure 4). Similarly, there is no correlation between THBS1 mRNA expression and mRS scores of discharges (ρ = –0.010, P = 0.864), 1 month after discharge (ρ = 0.030, P = 0.716), 3 months after discharge (ρ = 0.043, P = 0.608), and 6 months after discharge (ρ = 0.066, P = 0.434) in IS cases (Figure 5).
Figure 3. THBS1 mRNA expression with the HRs with 95%CIs for long-term deaths of IS patients. The HRs with 95%CIs for all-cause death, stroke death, and IS death had no correlation to THBS1 mRNA expression in IS patients. (all P-values > 0.05).
Figure 4. The correlation between THBS1 mRNA expression and NIHSS scores of different periods after discharge in IS patients. THBS1 mRNA expression has no significant relevance to NIHSS scores of different periods after discharge in IS patients (all P-values > 0.05).
Figure 5. The correlation between THBS1 mRNA expression and mRS scores of different periods after discharge in IS patients. THBS1 mRNA expression has no significant relevance to mRS scores of different periods after discharge in IS patients (all P-values > 0.05).
In this research, we investigated the association of variations and mRNA expression of THBS1 with the risk of IS and long-term death after stroke. Our results demonstrated that there was no significant association between tagSNPs of THBS1 and IS as following aspects. In the case control study, genotype and haplotype analyses identified no significant association with IS and TOAST subtypes of IS. Furthermore, cohort studies did not indicate significant associations between THBS1 variants and the risk of IS incidence or long-term death after stroke. Previous study showed that the coding polymorphism rs2292305 which is closely linked with rs3743125 we selected (r2 = 0.932) was identified no significant with cerebral infarction in a Chinese population (Liu et al., 2004). This result was consistent with our results. In addition, the THBS1 mRNA expression in IS cases was approximately equal to that in controls not only in the total population but also in different subgroups. THBS1 mRNA expression had no significant association with the prognosis of IS, including NIHSS scores, mRS scores, and long-term deaths after discharge. Thus, there is no significant association of THBS1 variants and leukocyte mRNA expression with the risk of IS and long-term death after stroke.
Ischemic stroke is one of the most vital causes of neurological morbidity and mortality in the world (Virani et al., 2021). Numerous studies confirmed that the inflammatory reaction that occurs in the cerebral tissue takes part in acute pathologies of ischemic stroke. The function damage to the brain following ischemic stroke results in necrosis and apoptosis; all of these could trigger the inflammatory reaction controlled by the release of ROS, chemokines, and cytokines (Brea et al., 2009). The thrombospondin (THBS) family of secreted matricellular glycoproteins consisting of five members (THBS1-5) are stress and injury mediators of cellular attachment dynamics and extracellular matrix protein production (Schips et al., 2019). Della-Morte et al. (2012) observed that variation in the von Willebrand factor (VWF), THBS1, and SERPINE1 genes may involve in the pathogenesis of atherosclerotic plaque and that suggested THBS1 had the ability to interact with VWF, to be an candidate gene for arterial thrombosis (Bonnefoy et al., 2006). Nonetheless, no strong evidence supported the role of THBS1 in the occurrence of arterial thrombosis (van Schie et al., 2011), and the correlation between peripheral THBS1 levels and long-term outcome of IS (Gao et al., 2015; Al Qawasmeh et al., 2020) merely displayed a concomitant effect rather than sequential relationship of causality. Therefore, the causal relationship between THBS1 and IS was still lack of sufficient evidence.
Isenberg and Roberts (2020) concluded that the THBS1 mutation alone may not be sufficient to cause pulmonary artery hypertension (PAH), and THBS1 was proposed to be a modifier gene for familial PAH. The frequency of common THBS1 polymorphisms did not differ between PAH and control cohorts (Isenberg and Roberts, 2020). THBS1 induced lethal cardiac atrophy and played a vital role in intermittent hypoxia-induced fibroblast activation and cardiac fibrosis when overexpressed (Bao et al., 2020; Vanhoutte et al., 2021). Furthermore, hsa-miR-4443 protected atrial fibrillation (AF) by targeting THBS1 (Xiao et al., 2021), as a biomarker in the development of AF and AF-related complications (Liu et al., 2021). Cardioembolic stroke caused by the embolus forming in the heart and occluding cerebral arteries is rising largely (Maida et al., 2020). AF is one of the risk factors for cardioembolic stroke. A previous study verified that subjects affected by AF have a risk of cerebral ischemia 3–5 times higher than the average (Wolf et al., 1991). Consequently, THBS1 may have relation to cardioembolic stroke. However, our research did not observe a positive association between THBS1 and IS not only in the IS case-control study but also in the cohort studies. The reason may be that cardioembolic stroke accounts for only 5.4% of all IS cases in our study.
Although previous study indicated that THBS1 may related to cardioembolic stroke, there was no significant difference of THBS1 mRNA expression between CE cases and controls in this study. Consequently, these findings did not support the etiological role of THBS1 in IS. However, Li et al. found several key genomic expressions (CCL20, THBS1, EREG, and IL6 etc.) were dramatically down-regulated in 5 and 24 h after ischemic stroke compared to controls (Li et al., 2017). On the contrary, expression of THBS1 was noted in the ischemic brain with different temporal expression profiles from different cellular origins after focal cerebral ischemia/reperfusion (Lin et al., 2003). Besides, an animal study suggested that THBS1 may increase the recruitment of monocytes into the clot and promote the transformation of monocytes into macrophages (Zhou et al., 2010). In advance, it has been reported that THBS1 could block vascular endothelial growth factor (VEGF)-induced angiogenesis (Iruela-Arispe et al., 1999), so the change of THBS1 expression in ischemic brains may confer a negative-feedback mechanism in angiogenesis (Kyriakides et al., 1998). In this study, THBS1 mRNA expression levels significantly differed across rs3743125 GG, GA, and AA carriers in the control group but not in IS group. The results may imply the latent biological function of rs3743125 on regulating mRNA expression in the population free of stroke while the effect disappears at IS occurrence. Since no significant association of THBS1 genetic variations and mRNA level was observed with IS or long-term death after IS, the value of this difference is limited to verify the pathogenesis of IS.
Though our current data did not suggest any significant association between the THBS1 variants or mRNA expression and IS, this research with no doubt has notable strengths. First of all, our study differs from previous THBS1 relevant studies that focused on the relationship between THBS1 protein levels in plasma and IS (Gao et al., 2015; Moin et al., 2021). This study is unique since we integrated analyses of the genomic level and the transcriptomic level of THBS1. Moreover, we explored the association of THBS1 candidate SNPs with not only the future new-onset IS but also the long-term outcomes of IS by combining the case-control study and the cohort study. In addition, the large sample size and the experiments with high-quality control ensured the accuracy and reliability of our results. However, some limitations are also worth mentioning. First, we did not detect serum THBS1 protein. Second, we selected candidate SNPs at the THBS1 gene with the criterion of MAF ≥ 0.05, so could have missed the rare variants with MAF < 0.05 that may have substantial biological effects on the IS occurrence and long-term death after IS.
In conclusion, although these findings need to be validated, our results indicated that there was no significant association between THBS1 polymorphisms and the risk of IS incidence or long-term death of IS, and no significant difference in THBS1 mRNA expression observed between IS patients and controls. Our findings, though negative, might as well contribute to verifying that the THBS1variants and expression do not affect the susceptibility to IS, and may provide useful evidence and guidance regarding the correlation between THBS1 and IS.
The data analyzed in this study is subject to the following licenses/restrictions: “The datasets presented in this article are not available due to participants’ confidentiality.” Requests to access these datasets should be directed to corresponding author.
Informed consent was obtained from each participant and this study was approved by the Research Ethics Committee of Nanjing Medical University (#2018571). The patients/participants provided their written informed consent to participate in this study.
CS designed the study, edited, and proofed the manuscript. CC performed the experiment work, analyzed the data, and wrote the manuscript. XC edited and proofed the manuscript. SY, LW, YJ, LL, and YW collected the data. QL, ZR, JL, and YY collected the samples. XG, FL, and JM performed the experiment work. All authors agreed to be accountable for the content of the work.
This work was supported by the National Natural Science Foundation of China (Grant Nos. 81872686 and 82173611) and the Priority Academic Program for the Development of Jiangsu Higher Education Institutions (Public Health and Preventive Medicine).
We would like to thank Hankun Xie for helping to edit the English language.
The authors declare that the research was conducted in the absence of any commercial or financial relationships that could be construed as a potential conflict of interest.
All claims expressed in this article are solely those of the authors and do not necessarily represent those of their affiliated organizations, or those of the publisher, the editors and the reviewers. Any product that may be evaluated in this article, or claim that may be made by its manufacturer, is not guaranteed or endorsed by the publisher.
The Supplementary Material for this article can be found online at: https://www.frontiersin.org/articles/10.3389/fnagi.2022.1006473/full#supplementary-material
Aburima, A., Berger, M., Spurgeon, B. E. J., Webb, B. A., Wraith, K. S., Febbraio, M., et al. (2021). Thrombospondin-1 promotes hemostasis through modulation of cAMP signaling in blood platelets. Blood 137, 678–689. doi: 10.1182/blood.2020005382
Al Qawasmeh, M., Alhusban, A., and Alfwaress, F. (2020). An evaluation of the ability of thrombospondin-1 to predict stroke outcomes and mortality after ischemic stroke. Int. J. Neurosci. 1–4. [Epub ahead of print]. doi: 10.1080/00207454.2020.1825417
August, P., and Suthanthiran, M. (2006). Transforming growth factor beta signaling, vascular remodeling, and hypertension. N. Engl. J. Med. 354, 2721–2723. doi: 10.1056/NEJMcibr062143
Baenziger, N. L., Brodie, G. N., and Majerus, P. W. (1972). Isolation and properties of a thrombin-sensitive protein of human platelets. J. Biol. Chem. 247, 2723–2731.
Bao, Q., Zhang, B., Suo, Y., Liu, C., Yang, Q., Zhang, K., et al. (2020). Intermittent hypoxia mediated by TSP1 dependent on STAT3 induces cardiac fibroblast activation and cardiac fibrosis. Elife 9:e49923. doi: 10.7554/eLife.49923
Bevan, S., Traylor, M., Adib-Samii, P., Malik, R., Paul, N. L., Jackson, C., et al. (2012). Genetic heritability of ischemic stroke and the contribution of previously reported candidate gene and genomewide associations. Stroke 43, 3161–3167. doi: 10.1161/strokeaha.112.665760
Bonnefoy, A., Daenens, K., Feys, H. B., De Vos, R., Vandervoort, P., Vermylen, J., et al. (2006). Thrombospondin-1 controls vascular platelet recruitment and thrombus adherence in mice by protecting (sub)endothelial VWF from cleavage by ADAMTS13. Blood 107, 955–964. doi: 10.1182/blood-2004-12-4856
Brea, D., Sobrino, T., Ramos-Cabrer, P., and Castillo, J. J. C. D. (2009). Inflammatory and neuroimmunomodulatory changes in acute cerebral ischemia. Cerebrovasc. Dis. 27, 48–64. doi: 10.1159/000200441
Campbell, B. C. V., De Silva, D. A., Macleod, M. R., Coutts, S. B., Schwamm, L. H., Davis, S. M., et al. (2019). Ischaemic stroke. Nat. Rev. Dis. Primers 5:70. doi: 10.1038/s41572-019-0118-8
Contreras-Ruiz, L., Ryan, D. S., Sia, R. K., Bower, K. S., Dartt, D. A., and Masli, S. (2014). Polymorphism in THBS1 gene is associated with post-refractive surgery chronic ocular surface inflammation. Ophthalmology 121, 1389–1397. doi: 10.1016/j.ophtha.2014.01.033
Della-Morte, D., Beecham, A., Dong, C., Wang, L., McClendon, M. S., Gardener, H., et al. (2012). Association between variations in coagulation system genes and carotid plaque. J. Neurol. Sci. 323, 93–98. doi: 10.1016/j.jns.2012.08.020
Dong, J., Yang, S., Zhuang, Q., Sun, J., Wei, P., Zhao, X., et al. (2021). The Associations of Lipid Profiles With Cardiovascular Diseases and Death in a 10-Year Prospective Cohort Study. Front. Cardiovasc. Med. 8:745539. doi: 10.3389/fcvm.2021.745539
Feigin, V. L., Nguyen, G., Cercy, K., Johnson, C. O., Alam, T., Parmar, P. G., et al. (2018). Global, Regional, and Country-Specific Lifetime Risks of Stroke, 1990 and 2016. N. Engl. J. Med. 379, 2429–2437. doi: 10.1056/NEJMoa1804492
Gao, J. B., Tang, W. D., Wang, H. X., and Xu, Y. (2015). Predictive value of thrombospondin-1 for outcomes in patients with acute ischemic stroke. Clin. Chim. Acta 450, 176–180. doi: 10.1016/j.cca.2015.08.014
Hong, B. B., Chen, S. Q., Qi, Y. L., Zhu, J. W., and Lin, J. Y. (2015). Association of THBS1 rs1478605 T>C in 5′-untranslated regions with the development and progression of gastric cancer. Biomed. Rep. 3, 207–214. doi: 10.3892/br.2015.414
Iruela-Arispe, M. L., Lombardo, M., Krutzsch, H. C., Lawler, J., and Roberts, D. D. (1999). Inhibition of angiogenesis by thrombospondin-1 is mediated by 2 independent regions within the type 1 repeats. Circulation 100, 1423–1431. doi: 10.1161/01.cir.100.13.1423
Isenberg, J. S., and Roberts, D. D. (2020). THBS1 (thrombospondin-1). Atlas Genet. Cytogenet. Oncol. Haematol. 24, 291–299. doi: 10.4267/2042/70774
Isenberg, J. S., Romeo, M. J., Yu, C., Yu, C. K., Nghiem, K., Monsale, J., et al. (2008). Thrombospondin-1 stimulates platelet aggregation by blocking the antithrombotic activity of nitric oxide/cGMP signaling. Blood 111, 613–623. doi: 10.1182/blood-2007-06-098392
Jacob, S. A., Novelli, E. M., Isenberg, J. S., Garrett, M. E., Chu, Y., Soldano, K., et al. (2017). Thrombospondin-1 gene polymorphism is associated with estimated pulmonary artery pressure in patients with sickle cell anemia. Am. J. Hematol. 92, E31–E34. doi: 10.1002/ajh.24635
Jaffe, E. A., Ruggiero, J. T., and Falcone, D. J. (1985). Monocytes and macrophages synthesize and secrete thrombospondin. Blood 65, 79–84.
Kyriakides, T. R., Zhu, Y. H., Smith, L. T., Bain, S. D., Yang, Z., Lin, M. T., et al. (1998). Mice that lack thrombospondin 2 display connective tIS casesue abnormalities that are associated with disordered collagen fibrillogenesis, an increased vascular density, and a bleeding diathesis. J. Cell. Biol. 140, 419–430. doi: 10.1083/jcb.140.2.419
Li, W. X., Qi, F., Liu, J. Q., Li, G. H., Dai, S. X., Zhang, T., et al. (2017). Different impairment of immune and inflammation functions in short and long-term after ischemic stroke. Am. J. Transl. Res. 9, 736–745.
Lin, T. N., Kim, G. M., Chen, J. J., Cheung, W. M., He, Y. Y., and Hsu, C. Y. (2003). Differential regulation of thrombospondin-1 and thrombospondin-2 after focal cerebral ischemia/reperfusion. Stroke 34, 177–186. doi: 10.1161/01.str.0000047100.84604.ba
Liu, H., Yang, J., Zhang, J., Zheng, T., and Zhai, Y. (2021). THBS1: a potential biomarker for atrial fibrillation. Int. J. Cardiol. 345:129. doi: 10.1016/j.ijcard.2021.10.152
Liu, X. N., Song, L., Wang, D. W., Liao, Y. H., Ma, A. Q., Zhu, Z. M., et al. (2004). [Correlation of thrombospondin-1 G1678A polymorphism to stroke: a study in Chinese population]. Zhonghua Yi Xue Za Zhi 84, 1959–1962.
Maida, C. D., Norrito, R. L., Daidone, M., Tuttolomondo, A., and Pinto, A. (2020). Neuroinflammatory Mechanisms in Ischemic Stroke: Focus on Cardioembolic Stroke, Background, and Therapeutic Approaches. Int. J. Mol. Sci. 21:6454. doi: 10.3390/ijms21186454
Matsuo, Y., Tanaka, M., Yamakage, H., Sasaki, Y., Muranaka, K., Hata, H., et al. (2015). Thrombospondin 1 as a novel biological marker of obesity and metabolic syndrome. Metabolism 64, 1490–1499. doi: 10.1016/j.metabol.2015.07.016
Moin, A. S. M., Nandakumar, M., Al-Qaissi, A., Sathyapalan, T., Atkin, S. L., and Butler, A. E. (2021). Potential Biomarkers to Predict Acute Ischemic Stroke in Type 2 Diabetes. Front. Mol. Biosci. 8:744459. doi: 10.3389/fmolb.2021.744459
Navarro-Sobrino, M., Rosell, A., Hernández-Guillamon, M., Penalba, A., Boada, C., Domingues-Montanari, S., et al. (2011). A large screening of angiogenesis biomarkers and their association with neurological outcome after ischemic stroke. Atherosclerosis 216, 205–211. doi: 10.1016/j.atherosclerosis.2011.01.030
O’Donnell, M. J., Xavier, D., Liu, L., Zhang, H., Chin, S. L., Rao-Melacini, P., et al. (2010). Risk factors for ischaemic and intracerebral haemorrhagic stroke in 22 countries (the INTERSTROKE study): a case-control study. Lancet 376, 112–123. doi: 10.1016/s0140-6736(10)60834-3
Schaid, D. J., Rowland, C. M., Tines, D. E., Jacobson, R. M., and Poland, G. A. (2002). Score tests for association between traits and haplotypes when linkage phase is ambiguous. Am. J. Hum. Genet. 70, 425–434. doi: 10.1086/338688
Schips, T. G., Vanhoutte, D., Vo, A., Correll, R. N., Brody, M. J., Khalil, H., et al. (2019). Thrombospondin-3 augments injury-induced cardiomyopathy by intracellular integrin inhibition and sarcolemmal instability. Nat. Commun. 10:76. doi: 10.1038/s41467-018-08026-8
Sweetwyne, M. T., and Murphy-Ullrich, J. E. (2012). Thrombospondin1 in tissue repair and fibrosis: TGF-β-dependent and independent mechanisms. Matrix Biol. 31, 178–186. doi: 10.1016/j.matbio.2012.01.006
van Schie, M. C., van Loon, J. E., de Maat, M. P., and Leebeek, F. W. (2011). Genetic determinants of von Willebrand factor levels and activity in relation to the risk of cardiovascular disease: a review. J. Thromb. Haemost. 9, 899–908. doi: 10.1111/j.1538-7836.2011.04243.x
Vanhoutte, D., Schips, T. G., Vo, A., Grimes, K. M., Baldwin, T. A., Brody, M. J., et al. (2021). Thbs1 induces lethal cardiac atrophy through PERK-ATF4 regulated autophagy. Nat. Commun. 12:3928. doi: 10.1038/s41467-021-24215-4
Varma, V., Yao-Borengasser, A., Bodles, A. M., Rasouli, N., Phanavanh, B., Nolen, G. T., et al. (2008). Thrombospondin-1 is an adipokine associated with obesity, adipose inflammation, and insulin resistance. Diabetes 57, 432–439. doi: 10.2337/db07-0840
Virani, S. S., Alonso, A., Aparicio, H. J., Benjamin, E. J., Bittencourt, M. S., Callaway, C. W., et al. (2021). Heart Disease and Stroke Statistics-2021 Update: A Report From the American Heart Association. Circulation 143, e254–e743. doi: 10.1161/cir.0000000000000950
Wolf, P. A., Abbott, R. D., and Kannel, W. B. (1991). Atrial fibrillation as an independent risk factor for stroke: the Framingham Study. Stroke 22, 983–988. doi: 10.1161/01.str.22.8.983
Xiao, J., Zhang, Y., Tang, Y., Dai, H., OuYang, Y., Li, C., et al. (2021). hsa-miR-4443 inhibits myocardial fibroblast proliferation by targeting THBS1 to regulate TGF-β1/α-SMA/collagen signaling in atrial fibrillation. Braz. J. Med. Biol. Res. 54:e10692. doi: 10.1590/1414-431x202010692
Keywords: thrombospondin-1, mRNA expression, ischemic stroke, case control study, cohort study
Citation: Chen C, Chen X, Yang S, Li Q, Ren Z, Wang L, Jiang Y, Gu X, Liu F, Mu J, Liu L, Wang Y, Li J, Yu Y, Zhang J and Shen C (2022) Association of THBS1 genetic variants and mRNA expression with the risks of ischemic stroke and long-term death after stroke. Front. Aging Neurosci. 14:1006473. doi: 10.3389/fnagi.2022.1006473
Received: 29 July 2022; Accepted: 05 September 2022;
Published: 23 September 2022.
Edited by:
Xiao-Qiao Dong, Hangzhou First People’s Hospital, ChinaReviewed by:
Jiemiao Hu, Ningbo Hangzhou Bay Hospital, ChinaCopyright © 2022 Chen, Chen, Yang, Li, Ren, Wang, Jiang, Gu, Liu, Mu, Liu, Wang, Li, Yu, Zhang and Shen. This is an open-access article distributed under the terms of the Creative Commons Attribution License (CC BY). The use, distribution or reproduction in other forums is permitted, provided the original author(s) and the copyright owner(s) are credited and that the original publication in this journal is cited, in accordance with accepted academic practice. No use, distribution or reproduction is permitted which does not comply with these terms.
*Correspondence: Chong Shen, c2NAbmptdS5lZHUuY24=
†These authors have contributed equally to this work and share first authorship
Disclaimer: All claims expressed in this article are solely those of the authors and do not necessarily represent those of their affiliated organizations, or those of the publisher, the editors and the reviewers. Any product that may be evaluated in this article or claim that may be made by its manufacturer is not guaranteed or endorsed by the publisher.
Research integrity at Frontiers
Learn more about the work of our research integrity team to safeguard the quality of each article we publish.