- 1Department of Biochemistry, College of Medicine, Konyang University, Daejeon, South Korea
- 2Research Institute for Dementia Science, Konyang University, Daejeon, South Korea
Alzheimer’s disease (AD) is a neurodegenerative disease that is characterized by irreversible cognitive declines. Senile plaques formed by amyloid-β (Aβ) peptides and neurofibrillary tangles, consisting of hyperphosphorylated tau protein accumulation, are prominent neuropathological features of AD. Impairment of adult neurogenesis is also a well-known pathology in AD. Adult neurogenesis is the process by which neurons are generated from adult neural stem cells. It is closely related to various functions, including cognition, as it occurs throughout life for continuous repair and development of specific neural pathways. Notably, subventricular zone (SVZ) neurogenesis, which occurs in the lateral ventricles, transports neurons to several brain regions such as the olfactory bulb, cerebral cortex, striatum, and hippocampus. These migrating neurons can affect cognitive function and behavior in different neurodegenerative diseases. Despite several studies indicating the importance of adult SVZ neurogenesis in neurodegenerative disorders, the pathological alterations and therapeutic implications of impaired adult neurogenesis in the SVZ in AD have not yet been fully explained. In this review, we summarize recent progress in understanding the alterations in adult SVZ neurogenesis in AD animal models and patients. Moreover, we discuss the potential therapeutic approaches for restoring impaired adult SVZ neurogenesis. Our goal is to impart to readers the importance of adult SVZ neurogenesis in AD and to provide new insights through the discussion of possible therapeutic approaches.
Introduction
Alzheimer’s disease (AD), the most prevalent type of dementia, is a progressive neurodegenerative disease characterized by cognitive impairment and memory loss. Two prominent neuropathological features of AD are senile plaques formed by the deposition of amyloid-β (Aβ) peptide and neurofibrillary tangles produced by the accumulation of abnormal hyperphosphorylation of tau proteins (DeTure and Dickson, 2019). Aggregation of Aβ and hyperphosphorylated tau induces various AD-associated pathologies, such as impaired adult neurogenesis (Kent et al., 2020). There has been much debate as to whether impaired adult neurogenesis contributes to the AD phenotype. Accumulating evidence suggests that the accumulation of Aβ and hyperphosphorylated tau exacerbates adult neurogenesis, and consequently, impaired adult neurogenesis induces cognitive deficits (Li Puma et al., 2020). Indeed, the elimination of adult neurogenesis worsens cognitive function (Hollands et al., 2017; Choi et al., 2018), whereas an increase in adult neurogenesis restores cognitive function in AD mouse models (Wang et al., 2010; Singh et al., 2012; Yan et al., 2014).
Neurogenesis is the process through which neural stem cells (NSCs) divide, migrate, and differentiate into specialized neural phenotypes (Cope and Gould, 2019). Adult neurogenesis progresses throughout life to consistently repair and further develop specific neural tracts (Eriksson et al., 1998; Boldrini et al., 2018), and it prominently occurs in particular regions of the neurogenic niche of the brain: the subgranular zone (SGZ) of the dentate gyrus and the subventricular zone (SVZ) bordering the lateral ventricles (Ming and Song, 2011). In a healthy brain, adult neural progenitor cells (NPCs) of the SVZ migrate through the rostral migratory stream (RMS) and eventually to the olfactory bulb (OB), where they differentiate into periglomerular and granule cells (Belluzzi et al., 2003; Arias-Carrion et al., 2007). Interestingly, several studies have shown that SVZ-derived adult NSCs and NPCs can partially migrate to the OB, cerebral cortex, striatum, and hippocampus (Seaberg and van der Kooy, 2002; Yamashita et al., 2006; Faiz et al., 2008; Motamed et al., 2019). Some evidence shows that new neurons originating from adult SVZ neurogenesis are associated with cognitive function through duplication and filling of multiple neuronal circuit regional connections within the brain (Parent et al., 1995; Bernier et al., 2002; Evans et al., 2002; Dayer et al., 2005). Surprisingly, studies examining the self-renewal ability in SVZ and dentate gyrus primary neurons have reported that self-renewal ability was found only in the SVZ (Seaberg and van der Kooy, 2002). This finding suggests that the SVZ can supply neural precursor cells to the SGZ through its continuous self-renewal capacity (Seaberg and van der Kooy, 2002), so the SVZ can be considered as the radical region of adult neurogenesis and is of comparable importance to the SGZ.
Compared with adult neurogenesis in the SGZ, few studies have focused on adult neurogenesis in the SVZ, but it is evident that the alteration of adult SVZ neurogenesis plays a crucial role in AD (Scopa et al., 2020; Culig et al., 2022). In addition, adult SVZ neurogenesis has been proposed in several studies as a promising therapeutic target for neurodegenerative diseases, including AD (Mu and Gage, 2011; Culig et al., 2022). Furthermore, SVZ-derived newborn neurons could help compensate for the reduced the neuronal population and restore impaired neural circuits in AD (Brinton and Wang, 2006). In this review, we summarize the alterations in adult SVZ neurogenesis in AD mouse models and patients with AD and suggest possible mechanisms. Furthermore, we discuss various pharmacological and non-pharmacological strategies targeting altered adult SVZ neurogenesis in AD, and describe the mechanisms of action of the three agents currently undergoing clinical trials.
Subventricular zone neurogenesis in Alzheimer’s disease
Alteration of adult subventricular zone neurogenesis in Alzheimer’s disease
Increasing evidence shows alterations in adult SVZ neurogenesis in patients with AD and in animal models (Figure 1 and Table 1). Notably, several studies have reported impairment of adult SVZ neurogenesis during the early stages of AD. A study examining alterations in the SVZ in an early Tg2576 mouse model reported that the proliferation of adult NSCs in the SVZ decreased from 1.5 months of age. In particular, decreased proliferation of adult NSCs is induced by intracellular Aβ oligomers (Scopa et al., 2020). Investigation of adult SVZ neurogenesis in APP/PS1 mice, which are known to exhibit Aβ deposition from 6 weeks of age and cognitive impairment at 7 months of age, showed that the proliferation and migration of NPCs decreased in the SVZ when the mice were 3 and 6 months old (Esteve et al., 2022). Studies using 2-month-old APPswe/PS1ΔE9 mice demonstrated that proliferation of NPCs and migration of neuroblasts significantly decreased in the SVZ (Demars et al., 2010). Reduced adult NSCs, NPCs, and their proliferation in SVZ were confirmed in 4-month-old 5XFAD mice (Park et al., 2016; Liu et al., 2020). In addition, the proliferation of adult NSCs was dramatically reduced in the Aβ-injected cortex compared with the contralateral cortex in Aβ-injected mice (Haughey et al., 2002). Specifically, Aβ inhibits the proliferation of adult NSCs originating from the SVZ by downregulating the expression of positive cell cycle modulators, including CDK5/p35 and cyclin B1, and upregulating the expression of the negative modulator Cdh1 (Esteve et al., 2022). Accumulated findings in an AD mouse model indicate that Aβ is a powerful factor that reduces adult SVZ neurogenesis by inhibiting the cell cycle, thereby inhibiting cell proliferation. Tau inhibits the morphological differentiation of adult NSCs into mature neurons via mediation of Aβ-induced microtubule hyperstabilization in Tg2576 mice (Scopa et al., 2020). The number of adult NSCs in the SVZ was reduced at 3 months of age in a 3xTg mouse model in which Aβ and tau were co-expressed (Rodriguez et al., 2009). Tauopathy mice showed a decrease in the proliferation of adult NSCs in the SVZ from 2 months of age (Komuro et al., 2015). Although tau plays a key role in the impairment of SVZ neuronal proliferation and migration under conditions such as chronic stress (Dioli et al., 2021), its effect on adult SVZ neurogenesis in AD remains unclear. Finally, dysfunction of subependymal lipid metabolism plays a critical role in the deficits of adult SVZ neurogenesis in the early stage of AD via hyperactivation of the protein kinase B signaling pathway (Gontier et al., 2015; Scopa et al., 2020). Decreased adult SVZ neurogenesis with neutral lipid accumulation has been reported in 2-month-old 3xTg mice (Hamilton et al., 2015), and deposition of neutral lipids was also found in the SVZ of post-mortem brains of AD patients (Hamilton et al., 2015). Similarly, proliferation in the recipient region, such as the OB, was reduced in 6 to 7-month-old ApoE4-knock-in mice (Li et al., 2009). These results suggest that the neurogenic niche in the SVZ is impaired in the early stages of AD due to Aβ and tau accumulation and disturbances in lipid metabolism.
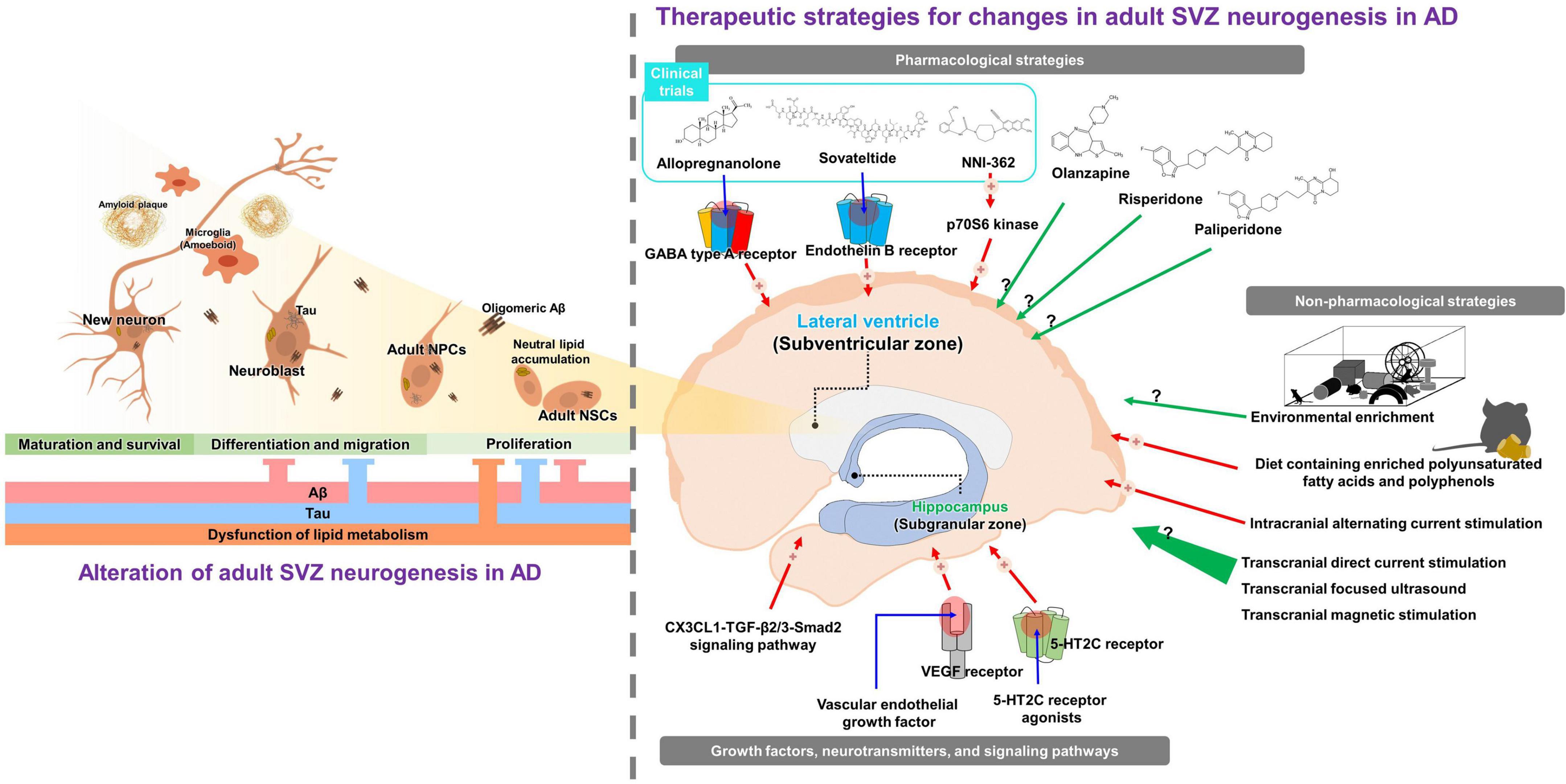
Figure 1. Graphical abstract for pathologic roles and therapeutic implications on adult subventricular neurogenesis in Alzheimer’s disease (AD). The left panel indicates the pathologic roles of adult subventricular neurogenesis in AD. Accumulations of Aβ, tau, and lipid inhibit proliferation, differentiation, and migration of adult neural stem cells. The right panel presents therapeutic strategies for impaired adult subventricular neurogenesis in AD described in the review. Green arrows indicate approaches known to increase adult neurogenesis in healthy animals; however, their efficacy in AD has not yet been investigated. Red arrows indicate approaches known to rescue impaired adult neurogenesis in AD.
As AD progresses, the increased interaction between Aβ and tau induces synergistic neurotoxicity (Zhang et al., 2021). Several other factors, along with Aβ and tau, strongly inhibit adult neurogenesis during the progression of AD. In this respect, dysregulations of inflammatory factors such as IL-6 and factors contributing to protein homeostasis may contribute to the impairment of adult SVZ neurogenesis in neurodegenerative disease (Wang et al., 2016). One study showed that adult NPC proliferation was significantly reduced by Aβ-induced dysregulation of calcium homeostasis in the SVZ of 11 to 12-month-old APP mutant mice (Haughey et al., 2002). Moreover, 7-month-old 5XFAD mice had higher Aβ deposition and microgliosis in the cerebral cortex than those in 2-month-old 5XFAD mice. SVZ-derived NPC from these 7-month-old 5XFAD mice exhibited impairments in general functions, including expansion of the neurosphere, immunomodulation, neurotrophic regulation, recovery from neurotoxic stimuli, and migration to the defective region (Fainstein et al., 2018). Adult SVZ neurogenesis, such as generating proliferating and progenitor cells, considerably decreased with AD progression in 11-and 18-month-old 3xTg mice compared with that in 2-month-old 3xTg mice (Hamilton et al., 2010, 2015). Consistent with the AD animal model, alterations in adult neurogenesis have also been reported in the brains of AD patients (Moreno-Jimenez et al., 2019). A post-mortem study of patients with AD showed that adult NPCs were reduced compared to those in healthy controls (Ziabreva et al., 2006). Levels of adult NPCs decrease with progressing Braak stages in AD patients (Perry et al., 2012). An in vitro study on human cortical NPCs showed that Aβ can inhibit NPC proliferation and differentiation and induce apoptosis even by disrupting calcium homeostasis (Haughey et al., 2002). In addition, a multimodal magnetic resonance imaging study performed on MCI and AD patients suggests that adult SVZ neurogenesis may be continuously impaired in MCI (Cherubini et al., 2010). Many studies have demonstrated that adult SVZ neurogenesis deteriorates due to various complex factors, including calcium homeostasis dysfunction and microgliosis, during AD progression. Therefore, approaches that target the impairment of adult SVZ neurogenesis are important in both asymptomatic and symptomatic stages of AD.
Therapeutic strategies for changes in adult subventricular zone neurogenesis in Alzheimer’s disease
Adult neurogenesis is important in compensating for neuronal loss and restoring cognitive function. Various pharmacological and non-pharmacological strategies have been investigated to enhance or restore adult neurogenesis (Figure 1). Several antipsychotic drugs have been reported to increase adult neurogenesis in the SVZ. One study has shown that antidepressants, such as olanzapine, increase adult SVZ neurogenesis (Green et al., 2006). In addition, administration of atypical neuroleptics, such as risperidone and paliperidone, increases adult SVZ neurogenesis in rats (Wakade et al., 2002; Nasrallah et al., 2010). It has been shown that, like pharmacological strategies, non-pharmacological therapeutic strategies can restore SVZ neurogenesis in AD. Environmental reinforcement is known to upregulate both adult SVZ and SGZ neurogenesis (van Praag et al., 1999; Mastrorilli et al., 2017). Although environmental enrichment enhances adult hippocampal neurogenesis in APP23 and APPSw, Ind mice (Mirochnic et al., 2009; Valero et al., 2011), few studies have reported the effect of environmental enrichment on adult SVZ neurogenesis. In apoE4-transgenic mice, environmental enrichment only increased adult neurogenesis in the SGZ (Levi and Michaelson, 2007). Further studies are needed to determine the effects of environmental enrichment on adult SVZ neurogenesis in other AD transgenic models. In addition, the LMN diet, a diet containing enriched polyunsaturated fatty acids and polyphenols, increased adult SVZ neurogenesis and alleviated cognitive impairment in Tg2576 mice (Fernandez-Fernandez et al., 2012). Intracranial alternating current stimulation has been reported to increase neurogenesis in the adult SVZ and SGZ in 5XFAD mice (Liu et al., 2020). Although there are an increasing number of studies in which non-invasive stimulation, such as transcranial direct current stimulation, transcranial focused ultrasound, and transcranial magnetic stimulation, increases adult neurogenesis in normal mice (Ueyama et al., 2011; Scarcelli et al., 2014; Pikhovych et al., 2016), there have been no investigations into whether these stimulations rescue impaired adult neurogenesis in AD.
Adult SVZ neurogenesis is modulated by various growth factors, neurotransmitters, and signaling pathways. Serotonin neurotransmission is important in the early stages of SVZ cell proliferation because serotonin (5-HT) terminals play an important role in forming the dense plexus that regulates ventricle and type B1 cells (Banasr et al., 2004; Tong et al., 2014). In particular, the level of the serotonin receptors decreased in the brains of patients with AD (Lorke et al., 2006). In practice, stimulation of 5-HT2C receptors via the administration of agonists increases adult SVZ neurogenesis in rats (Banasr et al., 2004). A strategy to modulate the level of vascular endothelial growth factor (VEGF) has also been proposed as a promising approach for restoring impaired neurogenesis (Sawada et al., 2014). The role of VEGF in adult neurogenesis in the neurogenic niche, including the SVZ, is well-established (Jin et al., 2002; Sawada et al., 2014). Implantation of VEGF through nanospheres effectively increased adult neurogenesis in APP/PS1 mice (Antequera et al., 2012; Herran et al., 2015). Finally, modulating activation of the signaling pathways involved in adult neurogenesis can enhance neurogenesis in the SVZ. For instance, stimulation of the CX3CL1-TGF-β2/3-Smad2 signaling pathway increases adult SVZ neurogenesis and ameliorates tau pathology-induced cognitive decline (Fan et al., 2019, 2020).
Three pharmaceutical candidates targeting adult neurogenesis are currently undergoing clinical trials for the treatment of AD (Cummings et al., 2022). Allopregnanolone is a neurosteroid that promotes adult SVZ neurogenesis by modulating GABA type A receptors (Wang et al., 2005; Brinton, 2013; Karout et al., 2016). Interestingly, allopregnanolone significantly decreased in brains with AD (Weill-Engerer et al., 2002). Studies in the 3xTg mouse model demonstrated that allopregnanolone increased adult neurogenesis in the SVZ and SGZ, and significantly recovered cognitive function in 3xTg mice (Wang et al., 2010; Singh et al., 2012). Moreover, a relevant study revealed that allopregnanolone stimulates NPC proliferation (Wang et al., 2005; Wang and Brinton, 2008). These results suggest that allopregnanolone promotes adult SVZ neurogenesis in AD and may be a therapeutic agent for AD. Allopregnanolone is currently in phase 1 and 2 clinical trials (NCT03748303 and NCT 04838301). Second, sovateltide (IRL-1620 or PMZ-1620) is an endothelin B receptor (ETBR) agonist that promotes adult SVZ neurogenesis. Previous studies have shown that the ETBR system is involved in neuronal cell survival and the restoration of adult neurogenesis in neurodegenerative disease (Leonard et al., 2011, 2012; Ranjan et al., 2020). Moreover, some studies have revealed that elevated levels of ETBR in AD are correlated with Aβ levels (Palmer et al., 2009; Koyama, 2013). In addition, a study in Aβ-injected rats suggested that sovateltide, an ETBR agonist, restored adult neurogenesis by promoting nerve growth factor and VEGF (Briyal et al., 2015). Furthermore, treatment of Aβ-injected rats with sovateltide significantly restored cognitive function (Briyal et al., 2014). Based on these neurogenesis-related effects, sovateltide for AD is undergoing a phase 2 clinical trial (NCT 04052737). NNI-362 is a small molecule that enhances adult SGZ neurogenesis by stimulating allosteric p70S6 kinase (Sumien et al., 2021). In addition, the administration of NNI-362 significantly restored cognitive function in Ts65Dn mice (Sumien et al., 2021). NNI-362 is a phase 1 clinical trial for AD (NCT 04074837). Unfortunately, NNI-362 is restricted to adult SGZ neurogenesis, and its effect on adult SVZ neurogenesis has not yet been confirmed. Although adult neurogenesis compensates for neuronal loss and restores cognitive function, studies, and clinical trials targeting AD are limited.
Discussion
In this review, we summarize the changes in adult SVZ neurogenesis in AD mouse models and patients with AD. Furthermore, we describe the possibility of therapeutic approaches to target altered adult SVZ neurogenesis in AD. Since SVZ-derived neuronal cells can migrate to the neocortex, striatum, hippocampus, and OB to compensate for neuronal loss, stimulating adult SVZ neurogenesis may provide a potential benefit in the restoration of impaired cognitive function in AD (Yamashita et al., 2006; Rodriguez et al., 2009; Rodriguez and Verkhratsky, 2011). In addition, adult SVZ neurogenesis is impaired from an early stage of AD and may contribute to the onset and progression of AD via various mechanisms (Rodriguez et al., 2009; Scopa et al., 2020). In particular, the striatum, which receives neuronal cells originating from the SVZ, is important for cognitive functions, including learning and memory, and neuronal loss in the ventral tegmental area is associated with memory dysfunction in AD (Ernst et al., 2014; Nobili et al., 2017). Therefore, alterations in adult SVZ neurogenesis have important implications as promising therapeutic targets in AD.
Despite many studies reporting the impairment of adult SVZ neurogenesis in AD, few studies have shown increased adult SVZ neurogenesis in AD (Table 1; Jin et al., 2004; Mastroeni et al., 2016). It can be speculated that Aβ could transiently induce hormetic effects in adult neurogenesis at subthreshold concentrations that induce neurotoxicity in early AD (Kent et al., 2020). For instance, Aβ-induced neurogenic effects in hippocampal NSCs are caused exclusively by Aβ42 but not by Aβ25–35 or Aβ40, and these effects are mediated by the extracellular signal-regulated kinase (ERK) pathway (Lopez-Toledano and Shelanski, 2004). Aβ42 is involved in adult neurogenesis by increasing DNA polymerase-β (Calafiore et al., 2012). Furthermore, adult SVZ neurogenesis could be temporarily enhanced as a compensatory response to massive nerve loss and brain injury during AD progression (Jin et al., 2004; Mu and Gage, 2011). Although adult SVZ neurogenesis is an attractive therapeutic target for AD, further studies are required. First, the migration pathways of SVZ-derived immature neurons in the human brain are not fully understood. Although the migration of neuroblasts has been observed in animals, the presence of RMS and OB neurogenesis in humans is controversial (Arellano and Rakic, 2011; Sanai et al., 2011; Bergmann et al., 2015). In addition, more studies are required to determine the pathological implications of tau in adult SVZ neurogenesis in AD. Although some studies have reported the inhibitory effects of tau pathology on adult SVZ neurogenesis (Rodriguez et al., 2009; Komuro et al., 2015; Scopa et al., 2020), the mechanisms of tau pathology in adult SVZ neurogenesis in the AD brain are still limited. Interspecies differences in adult SVZ neurogenesis between humans and mice should also be considered when interpreting the results obtained from AD mouse models (Lazarov and Marr, 2013). Thus, more mechanistic and translational studies on adult SVZ neurogenesis in AD should be performed.
Author contributions
HK, SS, SK, and YN drafted the initial version of the manuscript. AY conceived the tables. YN made the figure. MM conceived the outline of the manuscript, provided critical input, and revised the initial version of manuscript. All authors approved the final version of the manuscript.
Funding
This research was funded by Basic Science Research Program of the National Research Foundation of Korea, which is funded by the Ministry of Science, ICT and Future Planning (NRF-2018R1D1A3B07041059 to MM), and funded by the Ministry of Education (NRF-2022R1A6A3A13053190 to YN). This research was supported by a grant of the Korea Health Technology R&D Project through the Korea Health Industry Development Institute, funded by the Ministry of Health and Welfare, Republic of Korea (grant number: HF21C0021 to MM).
Conflict of interest
The authors declare that the research was conducted in the absence of any commercial or financial relationships that could be construed as a potential conflict of interest.
Publisher’s note
All claims expressed in this article are solely those of the authors and do not necessarily represent those of their affiliated organizations, or those of the publisher, the editors and the reviewers. Any product that may be evaluated in this article, or claim that may be made by its manufacturer, is not guaranteed or endorsed by the publisher.
References
Antequera, D., Portero, A., Bolos, M., Orive, G., Hernandez, R. M., Pedraz, J. L., et al. (2012). Encapsulated VEGF-secreting cells enhance proliferation of neuronal progenitors in the hippocampus of AbetaPP/Ps1 mice. J. Alzheimers Dis. 29, 187–200. doi: 10.3233/JAD-2011-111646
Arellano, J. I., and Rakic, P. (2011). Neuroscience: Gone with the wean. Nature 478, 333–334. doi: 10.1038/478333a
Arias-Carrion, O., Freundlieb, N., Oertel, W. H., and Hoglinger, G. U. (2007). Adult neurogenesis and Parkinson’s disease. CNS Neurol. Disord. Drug Targets 6, 326–335.
Banasr, M., Hery, M., Printemps, R., and Daszuta, A. (2004). Serotonin-induced increases in adult cell proliferation and neurogenesis are mediated through different and common 5-HT receptor subtypes in the dentate gyrus and the subventricular zone. Neuropsychopharmacology 29, 450–460. doi: 10.1038/sj.npp.1300320
Belluzzi, O., Benedusi, M., Ackman, J., and Loturco, J. J. (2003). Electrophysiological differentiation of new neurons in the olfactory bulb. J. Neurosci. 23, 10411–10418.
Bergmann, O., Spalding, K. L., and Frisen, J. (2015). Adult Neurogenesis in Humans. Cold Spring Harb. Perspect. Biol. 7:a018994.
Bernier, P. J., Bedard, A., Vinet, J., Levesque, M., and Parent, A. (2002). Newly generated neurons in the amygdala and adjoining cortex of adult primates. Proc. Natl. Acad. Sci. U.S.A. 99, 11464–11469. doi: 10.1073/pnas.172403999
Boldrini, M., Fulmore, C. A., Tartt, A. N., Simeon, L. R., Pavlova, I., Poposka, V., et al. (2018). Human hippocampal neurogenesis persists throughout aging. Cell Stem Cell 22:e585.
Brinton, R. D. (2013). Neurosteroids as regenerative agents in the brain: Therapeutic implications. Nat. Rev. Endocrinol. 9, 241–250. doi: 10.1038/nrendo.2013.31
Brinton, R. D., and Wang, J. M. (2006). Therapeutic potential of neurogenesis for prevention and recovery from Alzheimer’s disease: Allopregnanolone as a proof of concept neurogenic agent. Curr. Alzheimer. Res. 3, 185–190. doi: 10.2174/156720506777632817
Briyal, S., Nguyen, C., Leonard, M., and Gulati, A. (2015). Stimulation of endothelin B receptors by IRL-1620 decreases the progression of Alzheimer’s disease. Neuroscience 301, 1–11.
Briyal, S., Shepard, C., and Gulati, A. (2014). Endothelin receptor type B agonist. IRL-1620, prevents beta amyloid (Abeta) induced oxidative stress and cognitive impairment in normal and diabetic rats. Pharmacol. Biochem. Behav. 120, 65–72. doi: 10.1016/j.pbb.2014.02.008
Calafiore, M., Copani, A., and Deng, W. (2012). DNA polymerase-beta mediates the neurogenic effect of beta-amyloid protein in cultured subventricular zone neurospheres. J. Neurosci. Res. 90, 559–567. doi: 10.1002/jnr.22780
Cherubini, A., Spoletini, I., Peran, P., Luccichenti, G., Di Paola, M., Sancesario, G., et al. (2010). A multimodal MRI investigation of the subventricular zone in mild cognitive impairment and Alzheimer’s disease patients. Neurosci. Lett. 469, 214–218. doi: 10.1016/j.neulet.2009.11.077
Choi, S. H., Bylykbashi, E., Chatila, Z. K., Lee, S. W., Pulli, B., Clemenson, G. D., et al. (2018). Combined adult neurogenesis and BDNF mimic exercise effects on cognition in an Alzheimer’s mouse model. Science 361, eaan8821. doi: 10.1126/science.aan8821
Cope, E. C., and Gould, E. (2019). Adult neurogenesis. glia, and the extracellular matrix. Cell Stem Cell 24, 690–705.
Culig, L., Chu, X., and Bohr, V. A. (2022). Neurogenesis in aging and age-related neurodegenerative diseases. Ageing Res. Rev. 78:101636.
Cummings, J., Lee, G., Nahed, P., Kambar, M., Zhong, K., Fonseca, J., et al. (2022). Alzheimer’s disease drug development pipeline: 2022. Alzheimers Dement (N Y) 8:e12295.
Dayer, A. G., Cleaver, K. M., Abouantoun, T., and Cameron, H. A. (2005). New GABAergic interneurons in the adult neocortex and striatum are generated from different precursors. J. Cell Biol. 168, 415–427. doi: 10.1083/jcb.200407053
Demars, M., Hu, Y. S., Gadadhar, A., and Lazarov, O. (2010). Impaired neurogenesis is an early event in the etiology of familial Alzheimer’s disease in transgenic mice. J. Neurosci. Res. 88, 2103–2117.
DeTure, M. A., and Dickson, D. W. (2019). The neuropathological diagnosis of Alzheimer’s disease. Mol. Neurodegener. 14:32.
Dioli, C., Patricio, P., Pinto, L. G., Marie, C., Morais, M., Vyas, S., et al. (2021). Adult neurogenic process in the subventricular zone-olfactory bulb system is regulated by Tau protein under prolonged stress. Cell Prolif. 54:e13027. doi: 10.1111/cpr.13027
Eriksson, P. S., Perfilieva, E., Bjork-Eriksson, T., Alborn, A. M., Nordborg, C., Peterson, D. A., et al. (1998). Neurogenesis in the adult human hippocampus. Nat. Med. 4, 1313–1317.
Ernst, A., Alkass, K., Bernard, S., Salehpour, M., Perl, S., Tisdale, J., et al. (2014). Neurogenesis in the striatum of the adult human brain. Cell 156, 1072–1083.
Esteve, D., Molina-Navarro, M. M., Giraldo, E., Martinez-Varea, N., Blanco-Gandia, M. C., Rodriguez-Arias, M., et al. (2022). Adult neural stem cell migration is impaired in a mouse model of Alzheimer’s Disease. Mol. Neurobiol. 59, 1168–1182. doi: 10.1007/s12035-021-02620-6
Evans, J., Sumners, C., Moore, J., Huentelman, M. J., Deng, J., Gelband, C. H., et al. (2002). Characterization of mitotic neurons derived from adult rat hypothalamus and brain stem. J. Neurophysiol. 87, 1076–1085. doi: 10.1152/jn.00088.2001
Fainstein, N., Dan-Goor, N., and Ben-Hur, T. (2018). Resident brain neural precursor cells develop age-dependent loss of therapeutic functions in Alzheimer’s mice. Neurobiol. Aging 72, 40–52. doi: 10.1016/j.neurobiolaging.2018.07.020
Faiz, M., Acarin, L., Villapol, S., Schulz, S., Castellano, B., and Gonzalez, B. (2008). Substantial migration of SVZ cells to the cortex results in the generation of new neurons in the excitotoxically damaged immature rat brain. Mol. Cell. Neurosci. 38, 170–182. doi: 10.1016/j.mcn.2008.02.002
Fan, Q., Gayen, M., Singh, N., Gao, F., He, W., Hu, X., et al. (2019). The intracellular domain of CX3CL1 regulates adult neurogenesis and Alzheimer’s amyloid pathology. J. Exp. Med. 216, 1891–1903. doi: 10.1084/jem.20182238
Fan, Q., He, W., Gayen, M., Benoit, M. R., Luo, X., Hu, X., et al. (2020). Activated CX3CL1/Smad2 signals prevent neuronal loss and Alzheimer’s tau pathology-mediated cognitive dysfunction. J. Neurosci. 40, 1133–1144. doi: 10.1523/JNEUROSCI.1333-19.2019
Fernandez-Fernandez, L., Comes, G., Bolea, I., Valente, T., Ruiz, J., Murtra, P., et al. (2012). LMN diet, rich in polyphenols and polyunsaturated fatty acids, improves mouse cognitive decline associated with aging and Alzheimer’s disease. Behav. Brain Res. 228, 261–271. doi: 10.1016/j.bbr.2011.11.014
Gontier, G., Lin, K., and Villeda, S. A. (2015). Fat chance for neural stem cells in Alzheimer’s Disease. Cell Stem Cell 17, 373–374. doi: 10.1016/j.stem.2015.09.006
Green, W., Patil, P., Marsden, C. A., Bennett, G. W., and Wigmore, P. M. (2006). Treatment with olanzapine increases cell proliferation in the subventricular zone and prefrontal cortex. Brain Res. 1070, 242–245.
Hamilton, L. K., Aumont, A., Julien, C., Vadnais, A., Calon, F., and Fernandes, K. J. (2010). Widespread deficits in adult neurogenesis precede plaque and tangle formation in the 3xTg mouse model of Alzheimer’s disease. Eur. J. Neurosci. 32, 905–920. doi: 10.1111/j.1460-9568.2010.07379.x
Hamilton, L. K., Dufresne, M., Joppe, S. E., Petryszyn, S., Aumont, A., Calon, F., et al. (2015). aberrant lipid metabolism in the forebrain niche suppresses adult neural stem cell proliferation in an animal model of Alzheimer’s Disease. Cell Stem Cell 17, 397–411. doi: 10.1016/j.stem.2015.08.001
Haughey, N. J., Liu, D., Nath, A., Borchard, A. C., and Mattson, M. P. (2002). Disruption of neurogenesis in the subventricular zone of adult mice, and in human cortical neuronal precursor cells in culture, by amyloid beta-peptide: Implications for the pathogenesis of Alzheimer’s disease. Neuromolecular. Med. 1, 125–135. doi: 10.1385/NMM:1:2:125
Herran, E., Perez-Gonzalez, R., Igartua, M., Pedraz, J. L., Carro, E., and Hernandez, R. M. (2015). Enhanced Hippocampal Neurogenesis in APP/Ps1 Mouse Model of Alzheimer’s Disease After Implantation of VEGF-loaded PLGA Nanospheres. Curr. Alzheimer. Res. 12, 932–940. doi: 10.2174/1567205012666151027121622
Hollands, C., Tobin, M. K., Hsu, M., Musaraca, K., Yu, T. S., Mishra, R., et al. (2017). Depletion of adult neurogenesis exacerbates cognitive deficits in Alzheimer’s disease by compromising hippocampal inhibition. Mol. Neurodegener. 12:64. doi: 10.1186/s13024-017-0207-7
Jin, K., Galvan, V., Xie, L., Mao, X. O., Gorostiza, O. F., Bredesen, D. E., et al. (2004). Enhanced neurogenesis in Alzheimer’s disease transgenic (PDGF-APPSw,Ind) mice. Proc. Natl. Acad. Sci. U.S.A. 101, 13363–13367. doi: 10.1073/pnas.0403678101
Jin, K., Zhu, Y., Sun, Y., Mao, X. O., Xie, L., and Greenberg, D. A. (2002). Vascular endothelial growth factor (VEGF) stimulates neurogenesis in vitro and in vivo. Proc. Natl. Acad. Sci. U.S.A. 99, 11946–11950.
Karout, M., Miesch, M., Geoffroy, P., Kraft, S., Hofmann, H. D., Mensah-Nyagan, A. G., et al. (2016). Novel analogs of allopregnanolone show improved efficiency and specificity in neuroprotection and stimulation of proliferation. J. Neurochem. 139, 782–794. doi: 10.1111/jnc.13693
Kent, S. A., Spires-Jones, T. L., and Durrant, C. S. (2020). The physiological roles of tau and Abeta: Implications for Alzheimer’s disease pathology and therapeutics. Acta Neuropathol. 140, 417–447.
Komuro, Y., Xu, G., Bhaskar, K., and Lamb, B. T. (2015). Human tau expression reduces adult neurogenesis in a mouse model of tauopathy. Neurobiol. Aging 36, 2034–2042. doi: 10.1016/j.neurobiolaging.2015.03.002
Koyama, Y. (2013). Endothelin systems in the brain: Ivolvement in pathophysiological responses of damaged nerve tissues. Biomol. Concepts 4, 335–347. doi: 10.1515/bmc-2013-0004
Lazarov, O., and Marr, R. A. (2013). Of mice and men: Neurogenesis, cognition and Alzheimer’s disease. Front. Aging Neurosci. 5:43. doi: 10.3389/fnagi.2013.00043
Leonard, M. G., Briyal, S., and Gulati, A. (2011). Endothelin B receptor agonist, IRL-1620, reduces neurological damage following permanent middle cerebral artery occlusion in rats. Brain Res. 1420, 48–58.
Leonard, M. G., Briyal, S., and Gulati, A. (2012). Endothelin B receptor agonist, IRL-1620, provides long-term neuroprotection in cerebral ischemia in rats. Brain Res. 1464, 14–23. doi: 10.1016/j.brainres.2012.05.005
Levi, O., and Michaelson, D. M. (2007). Environmental enrichment stimulates neurogenesis in apolipoprotein E3 and neuronal apoptosis in apolipoprotein E4 transgenic mice. J. Neurochem. 100, 202–210.
Li Puma, D. D., Piacentini, R., and Grassi, C. (2020). Does impairment of adult neurogenesis contribute to pathophysiology of Alzheimer’s Disease? A still open question. Front. Mol. Neurosci. 13:578211. doi: 10.3389/fnmol.2020.578211
Li, G., Bien-Ly, N., Andrews-Zwilling, Y., Xu, Q., Bernardo, A., Ring, K., et al. (2009). GABAergic interneuron dysfunction impairs hippocampal neurogenesis in adult apolipoprotein E4 knockin mice. Cell Stem Cell 5, 634–645. doi: 10.1016/j.stem.2009.10.015
Liu, Q., Jiao, Y., Yang, W., Gao, B., Hsu, D. K., Nolta, J., et al. (2020). Intracranial alternating current stimulation facilitates neurogenesis in a mouse model of Alzheimer’s disease. Alzheimers Res. Ther. 12:89. doi: 10.1186/s13195-020-00656-9
Lopez-Toledano, M. A., and Shelanski, M. L. (2004). Neurogenic effect of beta-amyloid peptide in the development of neural stem cells. J. Neurosci. 24, 5439–5444.
Lorke, D. E., Lu, G., Cho, E., and Yew, D. T. (2006). Serotonin 5-HT2A and 5-HT6 receptors in the prefrontal cortex of Alzheimer and normal aging patients. BMC Neurosci. 7:36. doi: 10.1186/1471-2202-7-36
Mastroeni, D., Chouliaras, L., Van Den Hove, D. L., Nolz, J., Rutten, B. P. F., Delvaux, E., et al. (2016). Increased 5-hydroxymethylation levels in the sub ventricular zone of the Alzheimer’s brain. Neuroepigenetics 6, 26–31.
Mastrorilli, V., Scopa, C., Saraulli, D., Costanzi, M., Scardigli, R., Rouault, J. P., et al. (2017). Physical exercise rescues defective neural stem cells and neurogenesis in the adult subventricular zone of Btg1 knockout mice. Brain Struct. Funct. 222, 2855–2876. doi: 10.1007/s00429-017-1376-4
Ming, G. L., and Song, H. (2011). Adult neurogenesis in the mammalian brain: Significant answers and significant questions. Neuron 70, 687–702.
Mirochnic, S., Wolf, S., Staufenbiel, M., and Kempermann, G. (2009). Age effects on the regulation of adult hippocampal neurogenesis by physical activity and environmental enrichment in the APP23 mouse model of Alzheimer disease. Hippocampus 19, 1008–1018. doi: 10.1002/hipo.20560
Moreno-Jimenez, E. P., Flor-Garcia, M., Terreros-Roncal, J., Rabano, A., Cafini, F., Pallas-Bazarra, N., et al. (2019). Adult hippocampal neurogenesis is abundant in neurologically healthy subjects and drops sharply in patients with Alzheimer’s disease. Nat. Med. 25, 554–560. doi: 10.1038/s41591-019-0375-9
Motamed, S., Del Borgo, M. P., Zhou, K., Kulkarni, K., Crack, P. J., Merson, T. D., et al. (2019). Migration and differentiation of neural stem cells diverted from the subventricular zone by an injectable self-assembling beta-peptide hydrogel. Front. Bioeng. Biotechnol. 7:315. doi: 10.3389/fbioe.2019.00315
Mu, Y., and Gage, F. H. (2011). Adult hippocampal neurogenesis and its role in Alzheimer’s disease. Mol. Neurodegener. 6:85.
Nasrallah, H. A., Hopkins, T., and Pixley, S. K. (2010). Differential effects of antipsychotic and antidepressant drugs on neurogenic regions in rats. Brain Res. 1354, 23–29. doi: 10.1016/j.brainres.2010.07.075
Nobili, A., Latagliata, E. C., Viscomi, M. T., Cavallucci, V., Cutuli, D., Giacovazzo, G., et al. (2017). Dopamine neuronal loss contributes to memory and reward dysfunction in a model of Alzheimer’s disease. Nat. Commun. 8:14727. doi: 10.1038/ncomms14727
Palmer, J. C., Baig, S., Kehoe, P. G., and Love, S. (2009). Endothelin-converting enzyme-2 is increased in Alzheimer’s disease and up-regulated by Abeta. Am. J. Pathol. 175, 262–270. doi: 10.2353/ajpath.2009.081054
Parent, A., Cicchetti, F., and Beach, T. G. (1995). Calretinin-immunoreactive neurons in the human striatum. Brain Res. 674, 347–351.
Park, S. E., Lee, J., Chang, E. H., Kim, J. H., Sung, J. H., Na, D. L., et al. (2016). Activin A secreted by human mesenchymal stem cells induces neuronal development and neurite outgrowth in an in vitro model of Alzheimer’s disease: Neurogenesis induced by MSCs via activin A. Arch. Pharm. Res. 39, 1171–1179. doi: 10.1007/s12272-016-0799-4
Perry, E. K., Johnson, M., Ekonomou, A., Perry, R. H., Ballard, C., and Attems, J. (2012). Neurogenic abnormalities in Alzheimer’s disease differ between stages of neurogenesis and are partly related to cholinergic pathology. Neurobiol. Dis. 47, 155–162. doi: 10.1016/j.nbd.2012.03.033
Pikhovych, A., Stolberg, N. P., Jessica Flitsch, L., Walter, H. L., Graf, R., Fink, G. R., et al. (2016). Transcranial direct current stimulation modulates neurogenesis and microglia activation in the mouse brain. Stem Cells Int. 2016, 2715196. doi: 10.1155/2016/2715196
Ranjan, A. K., Briyal, S., and Gulati, A. (2020). Sovateltide (IRL-1620) activates neuronal differentiation and prevents mitochondrial dysfunction in adult mammalian brains following stroke. Sci. Rep. 10:12737. doi: 10.1038/s41598-020-69673-w
Rodriguez, J. J., and Verkhratsky, A. (2011). Neurogenesis in Alzheimer’s disease. J. Anat. 219, 78–89.
Rodriguez, J. J., Jones, V. C., and Verkhratsky, A. (2009). Impaired cell proliferation in the subventricular zone in an Alzheimer’s disease model. Neuroreport 20, 907–912. doi: 10.1097/WNR.0b013e32832be77d
Sanai, N., Nguyen, T., Ihrie, R. A., Mirzadeh, Z., Tsai, H. H., Wong, M., et al. (2011). Corridors of migrating neurons in the human brain and their decline during infancy. Nature 478, 382–386. doi: 10.1038/nature10487
Sawada, M., Matsumoto, M., and Sawamoto, K. (2014). Vascular regulation of adult neurogenesis under physiological and pathological conditions. Front. Neurosci. 8:53. doi: 10.3389/fnins.2014.00053
Scarcelli, T., Jordao, J. F., O’reilly, M. A., Ellens, N., Hynynen, K., and Aubert, I. (2014). Stimulation of hippocampal neurogenesis by transcranial focused ultrasound and microbubbles in adult mice. Brain Stimul. 7, 304–307. doi: 10.1016/j.brs.2013.12.012
Scopa, C., Marrocco, F., Latina, V., Ruggeri, F., Corvaglia, V., La Regina, F., et al. (2020). Impaired adult neurogenesis is an early event in Alzheimer’s disease neurodegeneration, mediated by intracellular Abeta oligomers. Cell Death Differ. 27, 934–948.
Seaberg, R. M., and van der Kooy, D. (2002). Adult rodent neurogenic regions: The ventricular subependyma contains neural stem cells, but the dentate gyrus contains restricted progenitors. J. Neurosci. 22, 1784–1793. doi: 10.1523/JNEUROSCI.22-05-01784.2002
Singh, C., Liu, L., Wang, J. M., Irwin, R. W., Yao, J., Chen, S., et al. (2012). Allopregnanolone restores hippocampal-dependent learning and memory and neural progenitor survival in aging 3xTgAD and nonTg mice. Neurobiol. Aging 33, 1493–1506. doi: 10.1016/j.neurobiolaging.2011.06.008
Sumien, N., Wells, M. S., Sidhu, A., Wong, J. M., Forster, M. J., Zheng, Q. X., et al. (2021). Novel pharmacotherapy: NNI-362, an allosteric p70S6 kinase stimulator, reverses cognitive and neural regenerative deficits in models of aging and disease. Stem Cell Res. Ther. 12:59. doi: 10.1186/s13287-020-02126-3
Tong, C. K., Chen, J., Cebrian-Silla, A., Mirzadeh, Z., Obernier, K., Guinto, C. D., et al. (2014). Axonal control of the adult neural stem cell niche. Cell Stem Cell 14, 500–511.
Ueyama, E., Ukai, S., Ogawa, A., Yamamoto, M., Kawaguchi, S., Ishii, R., et al. (2011). Chronic repetitive transcranial magnetic stimulation increases hippocampal neurogenesis in rats. Psychiatry Clin. Neurosci. 65, 77–81.
Valero, J., Espana, J., Parra-Damas, A., Martin, E., Rodriguez-Alvarez, J., and Saura, C. A. (2011). Short-term environmental enrichment rescues adult neurogenesis and memory deficits in APP(Sw,Ind) transgenic mice. PLoS One 6:e16832. doi: 10.1371/journal.pone.0016832
van Praag, H., Kempermann, G., and Gage, F. H. (1999). Running increases cell proliferation and neurogenesis in the adult mouse dentate gyrus. Nat. Neurosci. 2, 266–270.
Wakade, C. G., Mahadik, S. P., Waller, J. L., and Chiu, F. C. (2002). Atypical neuroleptics stimulate neurogenesis in adult rat brain. J. Neurosci. Res. 69, 72–79.
Wang, J. M., and Brinton, R. D. (2008). Allopregnanolone-induced rise in intracellular calcium in embryonic hippocampal neurons parallels their proliferative potential. BMC Neurosci. 9 Suppl 2:S11. doi: 10.1186/1471-2202-9-S2-S11
Wang, J. M., Johnston, P. B., Ball, B. G., and Brinton, R. D. (2005). The neurosteroid allopregnanolone promotes proliferation of rodent and human neural progenitor cells and regulates cell-cycle gene and protein expression. J. Neurosci. 25, 4706–4718.
Wang, J. M., Singh, C., Liu, L., Irwin, R. W., Chen, S., Chung, E. J., et al. (2010). Allopregnanolone reverses neurogenic and cognitive deficits in mouse model of Alzheimer’s disease. Proc. Natl. Acad. Sci. U.S.A. 107, 6498–6503.
Wang, X., Dong, C., Sun, L., Zhu, L., Sun, C., Ma, R., et al. (2016). Quantitative proteomic analysis of age-related subventricular zone proteins associated with neurodegenerative disease. Sci. Rep. 6:37443. doi: 10.1038/srep37443
Weill-Engerer, S., David, J. P., Sazdovitch, V., Liere, P., Eychenne, B., Pianos, A., et al. (2002). Neurosteroid quantification in human brain regions: Comparison between Alzheimer’s and nondemented patients. J. Clin. Endocrinol. Metab. 87, 5138–5143. doi: 10.1210/jc.2002-020878
Yamashita, T., Ninomiya, M., Hernandez Acosta, P., Garcia-Verdugo, J. M., Sunabori, T., Sakaguchi, M., et al. (2006). Subventricular zone-derived neuroblasts migrate and differentiate into mature neurons in the post-stroke adult striatum. J. Neurosci. 26, 6627–6636.
Yan, Y., Ma, T., Gong, K., Ao, Q., Zhang, X., and Gong, Y. (2014). Adipose-derived mesenchymal stem cell transplantation promotes adult neurogenesis in the brains of Alzheimer’s disease mice. Neural. Regen. Res. 9, 798–805.
Zhang, H., Wei, W., Zhao, M., Ma, L., Jiang, X., Pei, H., et al. (2021). Interaction between Abeta and Tau in the Pathogenesis of Alzheimer’s Disease. Int. J. Biol. Sci. 17, 2181–2192.
Keywords: Alzheimer’s disease, amyloid beta, tau, adult neurogenesis, subventricular zone
Citation: Kim HS, Shin SM, Kim S, Nam Y, Yoo A and Moon M (2022) Relationship between adult subventricular neurogenesis and Alzheimer’s disease: Pathologic roles and therapeutic implications. Front. Aging Neurosci. 14:1002281. doi: 10.3389/fnagi.2022.1002281
Received: 25 July 2022; Accepted: 24 August 2022;
Published: 14 September 2022.
Edited by:
Ana Lloret, University of Valencia, SpainReviewed by:
Daniel Esteve Moreno, University of Wisconsin-Madison, United StatesCopyright © 2022 Kim, Shin, Kim, Nam, Yoo and Moon. This is an open-access article distributed under the terms of the Creative Commons Attribution License (CC BY). The use, distribution or reproduction in other forums is permitted, provided the original author(s) and the copyright owner(s) are credited and that the original publication in this journal is cited, in accordance with accepted academic practice. No use, distribution or reproduction is permitted which does not comply with these terms.
*Correspondence: Minho Moon, hominmoon@konyang.ac.kr
†These authors have contributed equally to this work