- 1Department of Psychiatry, First Hospital/First Clinical Medical College of Shanxi Medical University, Taiyuan, China
- 2Shanxi Key Laboratory of Artificial Intelligence Assisted Diagnosis and Treatment for Mental Disorder, First Hospital of Shanxi Medical University, Taiyuan, China
- 3Department of Mental Health, Shanxi Medical University, Taiyuan, China
Neurodegenerative diseases are closely related to brain function and the progression of the diseases are irreversible. Due to brain tissue being not easy to acquire, the study of the pathophysiology of neurodegenerative disorders has many limitations—lack of reliable early biomarkers and personalized treatment. At the same time, the blood-brain barrier (BBB) limits most of the drug molecules into the damaged areas of the brain, which makes a big drop in the effect of drug treatment. Exosomes, a kind of endogenous nanoscale vesicles, play a key role in cell signaling through the transmission of genetic information and proteins between cells. Because of the ability to cross the BBB, exosomes are expected to link peripheral changes to central nervous system (CNS) events as potential biomarkers, and can even be used as a therapeutic carrier to deliver molecules specifically to CNS. Here we summarize the role of exosomes in pathophysiology, diagnosis, prognosis, and treatment of some neurodegenerative diseases (Alzheimer’s Disease, Parkinson’s Disease, Huntington’s Disease, Amyotrophic Lateral Sclerosis).
Introduction
Neurodegenerative disorders are a group of diseases closely related to brain function. Because brain tissue cannot be histologically examined, we have little understanding of its pathophysiological mechanisms, especially mental disorders. At present, the diagnosis of nervous system disease is mainly based on high-cost neuroimaging and biochemical examination of cerebrospinal fluid. Alzheimer’s Disease, Parkinson’s Disease, Amyotrophic Lateral Sclerosis and Huntington’s Disease are the important parts of the neuropsychiatric diseases. The course of neurodegenerative diseases is long and the onset is slow. Early diagnosis is difficult because of the lack of effective peripheral biomarkers (Cheng et al., 2015; Niu et al., 2020).
The blood-brain barrier (BBB) is a diffusion barrier essential for the normal functioning of CNS, preventing most molecules in the blood from entering the brain, and is composed of endothelial cells, astrocyte end-feet and pericytes (Ballabh et al., 2004). It has been shown that about 98% of small molecules and almost all macromolecules (such as peptides and gene drugs) cannot pass through the BBB, and in contrast, lipophilic small molecules pass more easily (Gabathuler, 2010). Therefore, most molecules must pass through a specific transporter protein or receptor in order to cross the BBB (Gabathuler, 2010). Previous studies have shown that the BBB is disrupted in neurodegenerative diseases, with increased permeability that is more favorable for substances to cross (Sweeney et al., 2018). However, in terms of treatment, the BBB still hinders the delivery of drugs to the CNS, making it difficult for drugs to reach the site of CNS injury because they are confined to the peripheral circulation, thus causing more drug side effects (Lakhal and Wood, 2011; Tian et al., 2018). Many researchers have attempted to increase the efficiency of drug delivery by injecting chemicals into the brain to disrupt the BBB, but this approach simultaneously disrupts the tight junctions of the BBB, leading to the invasion of harmful substances and metabolic waste, and disrupts cerebral blood flow levels, further exacerbating symptoms by affecting metabolism and neuroinflammation (Ouyang et al., 2021). How to transport therapeutic drugs more efficiently across the BBB to target sites has become a critical issue to be addressed in the treatment process.
Exosome contains DNA, RNA, proteins, lipids, and other substances, which can be circularly transmitted to adjacent and distant cells. The transfer of exosomes can lead to phenotypic changes of receptor cells (Cheng et al., 2015). In particular, miRNA is involved in a variety of pathological processes, including the growth and metastasis of tumors. Many recent studies showed that it also plays an important role in nerve inflammation (Steinbichler et al., 2017; Li et al., 2018; Pascual et al., 2020). It has been found that the production and release of exosomes depend largely on the characteristics of the mother cell and the target cell (Pegtel and Gould, 2019). The presence of some signature proteins on their surface can interact with other proteins such as integrins, which leads to the transport and fusion of exosomes, and the connection with target cells to function (Rastogi et al., 2021). Therefore, different exosomes have different contents and physiological functions with good specificity and targeting. Due to its peripheral availability and, ability to cross the BBB and superior targeting, it increases the possibility of being used as a biomarker in neurodegenerative diseases, thus manifesting its application in the diagnosis of ND (Wei et al., 2020). It can even be used as a drug carrier in the treatment of diseases (Haney et al., 2015). In conclusion, the study of exosomes in neurodegenerative diseases contributes to the early detection and diagnosis of diseases and provides new methods for the treatment of diseases.
This review summarizes the role of exosomes in some age-related neurodegenerative diseases (Alzheimer’s Disease, Parkinson’s Disease, Huntington’s Disease, Amyotrophic Lateral Sclerosis) and reveals the present the latest research progress.
Exosome
Exosomes were first described in 1981 by Trams, E. G et al. (Trams et al., 1981). They are nanoscale vesicles (30–100 nm in diameter) released by different types of cells under specific stimuli., including neurons and glial cells. In the early 1980s, the complex process of exosome generation was proposed, including the formation and release of multivesicular bodies (MVB) (Colombo et al., 2014). However, some researchers have pointed out that exosomes are not limited to one mode of generation, but can also be generated by budding of the plasma membrane (Pegtel and Gould, 2019; Figure 1). Exosomes are widely distributed in saliva, plasma, cerebrospinal fluid, milk, and other body fluids found in a variety of body fluids and contain proteins, nucleic acids, and lipids from mother cells (Geng et al., 2019; Saeedi et al., 2019; Sun et al., 2019; Figure 1). The size, shape, and density of exosomes are determined by the proteins, lipids, and other substances they contain, and are highly variable (Pegtel and Gould, 2019). Escola et al. found that co-stimulatory molecule CD86 and several tetraspan proteins (including CD37, CD53, CD63, CD81, and CD82) were highly enriched in exosomes and correlated with their immune effects (Escola et al., 1998), with CD81 and CD63 having become the most commonly used exosome marker proteins (Hemler, 2003). In addition to this, exosomes contain many integral membrane signaling proteins, including epidermal growth factor receptor (EGFR), mast/stem cell growth factor receptor (c-Kit), vascular endothelial growth factor receptor type-2, insulin-like growth factor I receptor, T cell receptor, cytokine receptors, G protein–coupled receptors (GPCRs), Notch receptors and so on, which can act as surface signaling molecules for cellular transmission of functional receptors and signaling pathways (Pegtel and Gould, 2019). In addition, the lipid content of exosomes is not the same as that of their mother cells, with cholesterol, sphingomyelin, glycosphingolipids and phosphatidylserine being enriched in exosomes two to three times as much as in their mother cells (Skotland et al., 2017).
Exosomes have an important biological role in vivo (Pegtel and Gould, 2019), and some researchers have categorized this into the following three points: (1) The organism can selectively remove some protein substances from the plasma membrane through the pathway of exosome generation, such as the vesicular secretion of neurodegenerative amyloidogenic proteins (Quek and Hill, 2017). (2) Exosomes are important components of the extracellular matrix that mediate amyloid aggregation, plaque and tangle formation, growth and spreading in neurodegenerative disease. (3) Exosomes mediate intercellular signaling and messaging. Exosomes can be internalized by other cells through membrane fusion, endocytosis, or specific phagocytosis. By transferring proteins and genetic materials (such as mRNA, miRNA, rRNA, long-chain non-coding RNA, DNA, etc.) to other cells, it can change the function of receptor cells and mediate cell-to-cell communication. And bilayer lipid membrane effectively protects its contents from degradation (Wang et al., 2018; Geng et al., 2019; Koteswara et al., 2019). Exosomes contain a variety of biological substances, among which miRNAs are highly enriched in exosomes. And most miRNAs that can be obtained from serum or saliva are contained in exosomes. Exosome-derived miRNAs are considered to be more stable than cell miRNAs (Wang et al., 2018). The expression profile of exosomal miRNAs is not identical to that of mother cells, and its expression can be changed according to the change of disease status. In recent years, it has been considered as a potential biomarker (Saeedi et al., 2019). More and more attention has been paid to the role of exosomes in neurodegenerative diseases (Fries and Quevedo, 2018). Especially for neurodegenerative diseases, the study of exosomal miRNAs has attracted great attention.
The ability to cross BBB (Samanta et al., 2018; Gomez-Molina et al., 2019) makes it possible for exosomes to enter the brain as a drug delivery carrier. Also, due to the targeting effect and immune resistance of exosomes (Bunggulawa et al., 2018), they can further reduce the drug side effects due to drug retention in the periphery during transport as drug carriers, further increasing the drug delivery benefits. In recent years, they are a promising drug carrier. In many types of cancer, whether in vivo or in vitro, it has been shown that they can carry drugs and target delivery to reduce the adverse reactions of drug treatment (Yang et al., 2017; Kalimuthu et al., 2018). While many preclinical studies have demonstrated the potential of exosomes to treat disease, only a handful of companies are currently conducting relevant clinical studies (Perocheau et al., 2021): Codiak Biosciences works to develop therapeutic exosomes carrying siRNAs targeting the KRAS (G12D) mutation in pancreatic cancer (Mendt et al., 2018); Avalon Globocare is developing engineered exosome therapeutics (Wang et al., 2019b).
In conclusion, exosomes, as natural carriers for transferring bioactive molecules between cells, are characterized by low immunogenicity, strong biodegradability, ability to wrap endogenous bioactive molecules, and ability to cross BBB, and are considered as a new endogenous drug delivery system (Sun et al., 2019; Zhang et al., 2019). In the nervous system, exosomes mediate cell-to-cell communication and are thought to be closely related to learning and memory, neuroinflammation, and other aspects (Wang et al., 2018; Saeedi et al., 2019).
Exosomes and Alzheimer’s Disease
Alzheimer’s disease (AD) is a neurodegenerative diseases characterized by decreased levels of amyloid-beta (Aβ) (reduced levels of Aβ in the cerebrospinal fluid due to deposition of Aβ in the brain), increased levels of total tau or phosphorylated tau, and a reduction in the number and function of synapses (Palop et al., 2006). The increased Aβ aggregates into soluble oligomers to activate microglia to produce an inflammatory reaction and oxidative stress. Excessive Aβ produces a cascade reaction to make neurons degenerate. The abnormal phosphorylation of tau protein can form nerve fiber tangles, which leads to a decrease in neuron function and even neuronal apoptosis (Palop et al., 2006). In recent years, a large number of studies have shown that exosomes are closely related to the occurrence and progression of AD, bringing hope for the treatment (Figure 2).
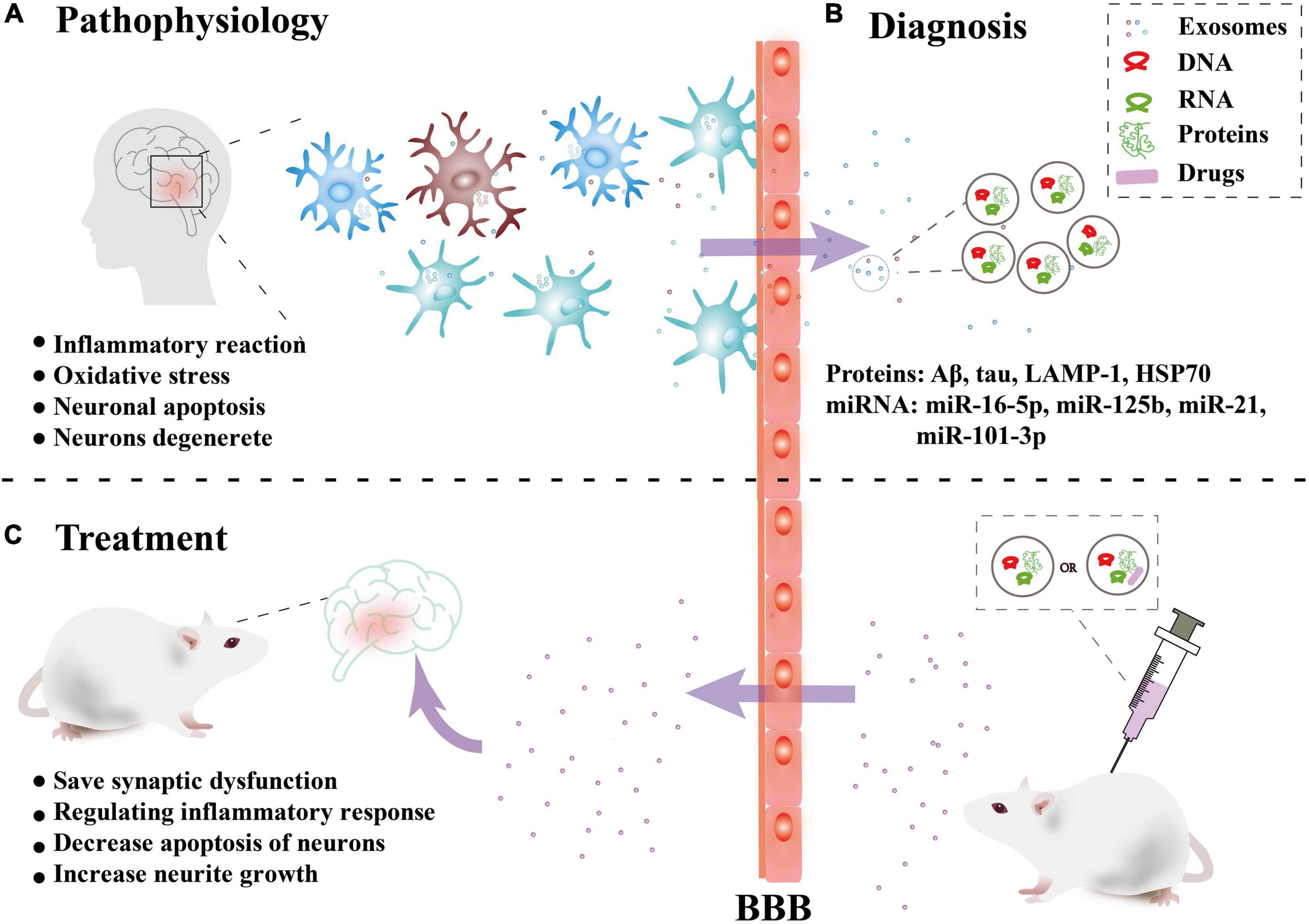
Figure 2. Overview of the role of exosomes in Alzheimer’s disease. (A) Pathophysiology: Nerve cells in brain lesions release and transmit exosomes, leading to local neuroinflammation and neuronal apoptosis, etc. (B) Diagnosis: Released exosomes and entering the peripheral circulation through the blood-brain barrier (BBB) were collected for diagnostic biomarkers. (C) Treatment: In vitro, exosomes combined with drugs or directly can be injected intravenously into mice can cross the BBB and have a certain targeting ability to the damaged areas, thus playing a therapeutic role.
In 2006, some studies found that exosome is associated with the release of Aβ and can aggravate the brain pathology of AD by promoting the aggregation of Aβ (Rajendran et al., 2006; Dinkins et al., 2016). It can be used as a carrier to transfer toxic substances (such as phosphorylated tau protein and Aβ) between neurons (Kaur et al., 2021). Inhibiting exosome synthesis can significantly reduce the proliferation of tau protein (Wang et al., 2017; Sardar Sinha et al., 2018; Winston et al., 2019). However, the cellular origin of the active exosomes has not been determined, and both microglia and astrocytes have been proposed (Asai et al., 2015; Rosas-Hernandez et al., 2019). In addition, it has been proposed that with the progression of AD, the level of functionally specific synaptic proteins in plasma neural-derived exosomes (NDE) decreases. And synaptic proteins in NDE may be effective preclinical indicators and progression indicators of AD (Goetzl et al., 2016, 2018).
In recent years, there have been many studies on AD biomarkers in exosomes in CSF, blood, and in vitro cultures, mainly involving proteins and miRNAs (Table 1). In terms of proteins, HSP70 (Goetzl et al., 2015; Chanteloup et al., 2019) has received a great deal of attention in addition to the various forms of Aβ extracted in various body fluids (Watson et al., 2019). In addition, the findings of exosomal miRNAs as biomarkers are variable and lack uniform and authoritative conclusions.
In treatment, because exosomes can cross the BBB, its application in targeted therapy has attracted much attention. Relative to conventional AAV (adeno-associated virus), exo-AAV (AAV combine with exosomes) is more efficient at gene delivery to the brain at low vector doses. The ability of exo-AAV of evading neutralizing antibodies and transducing CNS after peripheral delivery makes it electively ingested by neurons (Hudry et al., 2016; Perets et al., 2019). In addition, some researchers combined curcumin and exosomes to inhibit tau phosphorylation and activate GSK-3/AKT pathway, to prevent neuronal death and relief symptoms (Wang et al., 2019a).
Besides, exosomes injected into the mouse models from different cells play different roles in treatment. For example:
(1) The exosomes from mesenchymal stromal cells (MSCs) or hypoxia-preconditioned MSCs could improve cognitive function (the learning and memory) by saving synaptic dysfunction and regulating inflammatory response through regulation of miR-21 (Cui et al., 2018).
(2) Treatment with exosomes from adipose-derived stem cells (ADSC-Exo) resulted in decreased Aβ42 and Aβ40 levels, increased apoptotic molecule levels (such as p53, pro-caspase-3, decreased Bcl-2 protein), and decreased apoptosis of neurons. In addition, neurite growth was also increased during the treatment (Lee et al., 2018).
(3) Exosomes from human umbilical cord mesenchymal stem cells showed the ability to repair cognitive dysfunction, help clear Aβ deposition in the brain, and reduce neuroinflammation and relief symptoms by regulating the activation of mouse brain microglia cells (Ding et al., 2018).
Exosomes and Parkinson’s Disease
Parkinson’s disease (PD) is the second most common neurodegenerative diseases in the world after Alzheimer’s disease (Lebouvier et al., 2009). The main pathological changes were degeneration and death of dopaminergic neurons in substantia nigra, a significant decrease of DA in the striatum, and eosinophilic inclusion bodies appearance in the cytoplasm of residual neurons in substantia nigra, namely Lewy body, α-synuclein (α-syn) is its main ingredients. Recent studies have shown that exosomes play a certain role in the pathogenesis, diagnosis, and treatment of PD (Howitt and Hill, 2016).
As an important component of the Lewy bodies, α-syn are transferred from intracellular to extracellular by being packaged into exosomes or directly released (Lee, 2005). Exosomes provide an ideal environment for α-syn polymerization (Grey et al., 2015). Exosomes can transfer toxic forms of α-syn between a variety of cells, such as astrocytes and microglia, which can lead to the exacerbation of PD. α-syn deposited in glial cells induces inflammation and its further transmission promotes the degeneration of neurons and exacerbates the development of PD (Chistiakov and Chistiakov, 2017). α-syn in NDE is considered an important biomarker of PD and associated with deterioration of motor symptoms (Si et al., 2019; Niu et al., 2020). Some researchers have found that the content of α-syn in NDE of non-tremor patients is higher than that of tremor patients, so it may also be used to identify different types of motor types in PD (Si et al., 2019).
In terms of biomarkers, the value of α-syn in exosomes in diagnosis has been pointed out in many studies (Si et al., 2019; Fu et al., 2020; Niu et al., 2020; Zheng et al., 2021), and it has also been shown that exosomal α-syn can be used to differentiate PD from multiple system atrophy (Dutta et al., 2021). There are some other studies on exosomes in serum and CSF of PD patients (Table 2).
In terms of treatment, exosomes can be used as drug therapy carriers for PD and have a natural brain targeted ability. By using the blood exosomes as a delivery system, the distribution of dopamine in the brain has increased more than 15-fold. Compared with free dopamine after intravenous administration, dopamine-loaded exosomes show better therapeutic efficacy and lower systemic toxicity in a PD mouse model (Qu et al., 2018). Otherwise, the release of catalase can reduce cell death by protecting neurons from oxidative damage. In vitro and in vivo trials of PD, therapeutic catalase mRNA delivery by exosomes attenuated neurotoxicity and neuroinflammation (Haney et al., 2015; Kojima et al., 2018).
Moreover, the study on exosomes also provided a theoretical basis for other known causes of PD and clarified the specific pathological mechanism. For instance:
(1) Glucocerebrosidase gene (GBA) mutation is the most common genetic pathogenic factor in PD and is associated with decreased glucocerebrosidase activity in PD. Overexpression of GBA in vitro resulted in significantly reduced exosome secretion which is associated with the α-syn oligomers. And the reduction of glucocerebrosidase activity in vivo induced a significant increase in the number of exosomes released in the brain, thus promoting the pathological changes of PD (Papadopoulos et al., 2018).
(2) Manganese exposure is considered to be an important factor in the susceptibility of PD. It can increase the protein Rab27a and some miRNAs that regulate the release of exosomes, leading to protein aggregation, autophagy, inflammation, and hypoxia, thereby potentially promoting progressive neurodegeneration (Harischandra et al., 2018). In addition, manganese exposure promotes the secretion of α-syn in exosomes, accelerates the intercellular transmission of α-syn, and leads to dopaminergic neurotoxicity, thus causing proinflammatory and neurodegenerative reactions (Harischandra et al., 2019).
(3) Mutations in leucine-rich repetitive kinase 2 (LRRK2) enhance the level of self-phosphorylated LRRK2 protein, the most commonly known cause of hereditary PD. The level of Ser(P)-1292 LRRK2 in Urinary exosomes were found to be elevated in idiopathic Parkinson’s disease and correlated with the severity of cognitive impairment and difficulty in daily activities (Fraser et al., 2016b). Its proportion in total LRRK2 predicted LRRK2 mutation status in LRRK2 mutation carriers (Fraser et al., 2016a).
Exosomes and Huntington’s Disease
Huntington’s disease (HD) is an autosomal dominant neurodegenerative disorder. In 1993, the international Huntington’s Disease Cooperative Research Group cloned the disease-causing gene IT15, its metabolite mutant Huntington’s protein (mHTT) which has many repeated glutamines, is easy adhesion, aggregation, and eventually lead to the death of nerve cells. Exosomes can carry and spread mHTT between cells, triggering HD-related behaviors and pathological performance (Jeon et al., 2016).
Previous studies in different HD mouse models have shown that exosomes from astrocytes (ASC-EXO) carry heat shock proteins and other neuroprotective substances, which can reduce the cytotoxicity of misfolded proteins and prevent neurodegeneration. And dysfunction of astrocytes can lead to neuronal vulnerability (Hajrasouliha et al., 2013; Nafar et al., 2016). Although mHTT does not exist in ASC-Exo, it can reduce the secretion of ASC-Exo in HD mice (Hong et al., 2017). The specific possible mechanism is as follows: (1) The n-terminal of mHTT can form aggregation in the nucleus, leading to the decrease of exosomes in astrocytes; (2) mHTT reduces the expression of the small heat shock protein αB-crystallin [a protein mainly expressed in glial cells, mediating exosome secretion (Gangalum et al., 2016)] in astrocytes, thus reducing the secretion of exosomes in the brain and promoting the acellular autonomic neurotoxicity of HD. And when ASC-Exo is injected into the striatum of HD mice, the density of mHTT aggregation could be reduced and the overexpression of αB-crystallin could reduce the exosome deficiency and neuropathological changes, which provided ideas for the treatment of Huntington’s disease (Hong et al., 2017).
It has been found that ASC-EXO could significantly reduce mHTT aggregation of nerve cells, up-regulate the expression of PGC-1 and phosphorylated CREB, and reduce mitochondrial dysfunction and cell apoptosis, indicating that ASC-EXO has the potential to treat HD (Lee et al., 2016b). In addition, it has been found that exosome-mediated hydrophobic modification of siRNA can silence HTT mRNA, which is expected to promote the development of treatment methods for Huntington’s disease and other neurodegenerative diseases (Didiot et al., 2016). Some researchers have injected exosomes loaded with excess miR-124 into the striatum of HD model mice in an attempt to improve HD-like behavior, and although the results were not satisfactory, this approach still resulted in downregulation of the target gene RE1-Silencing Transcription Factor (Lee et al., 2017). Some researchers recently found that transferring serum exosomes from young mice into an in vitro model of HD can effectively ameliorate mHTT mutations, slow down apoptosis, and promote mitochondrial biogenesis, while the shared blood circulation through parabiosis experiment confirmed the above idea (Lee et al., 2021).
Exosomes and Amyotrophic Lateral Sclerosis
Amyotrophic lateral sclerosis (ALS) is caused by progressive weakness and atrophy of the muscles innervated by the medulla oblongata and muscles in the limbs and trunk after injury to upper and lower motor neurons. According to family history, it can be divided into sporadic ALS (sALS) and familial ALS (fALS), among which only 5 ∼ 10% are fALS. So far, more than 20 genes related to the pathogenesis of ALS have been found, among which superoxide dismutase gene (SODl) and ubiquitinated TAR-DNA binding protein 43(TDP43) are the most studied (Chen et al., 2019).
Some ALS are caused by the misfolding of mutated SOD1, and the misfolded SOD1 is transferred through exosome dependent or independent way. In cell culture, once SOD1 misfold happens, it can still induce misfolding of the immature cell after the initial misfolded template has been degraded for a long time (Gomes et al., 2007; Grad et al., 2014a,b; Silverman et al., 2016). Other researchers have found that NDE in the brain of ALS can cause the cytoplasmic redistribution of TDP-43, suggesting that exosomes may be involved in the transmission of TDP-43 protein lesions (Yohei et al., 2016). By analyzing serum miR-27a-3b in ALS and healthy subjects, Xu Qian et al. found that exosomal miR-27a-3b expression was down-regulated in ALS, which may serve as a detection indicator for ALS, but the exact mechanism is unclear (Xu et al., 2018).
Noriko Hayashi et al. analyzed the proteomics of exosomes from patients with sALS, it is found that three proteins increased and 11 proteins decreased in exosomes of ALS patients. The most increased protein was a new that inhibitor (NIR), which was closely related to nucleolar function. The decrease of NIR in the motor neuron nucleus of ALS patients suggested that nucleolar stress might play a role in the pathogenesis of sporadic ALS through NIR dysfunction (Hayashi et al., 2019). Chen et al. (2019) found that the level of IL-6 in ASC-Exo of sALS patients was increased, which was positively correlated with the rate of disease progression (only for patients with disease course less than 12 months), indicating that inflammation of CNS was increased, and exosomes derived from nerve cell might help to reveal the neuroinflammation of CNS of ALS patients. Besides, exosome proteins have been proved to be mainly involved in the negative regulation of cell adhesion and apoptosis. In the exosomes of ALS patients, the pro-apoptotic protein Bax and caspase-3 are down-regulated, and the antiapoptotic protein Bcl-2α is up-regulated. The protein content is related to the antiapoptotic effect of exosomes (Bonafede et al., 2019). Moreover, TDP-43 from the exosomes of patients can induce the increase of monocytes, which may increase the neuroinflammatory effect (Zondler et al., 2017). In addition, exosomes mediated the interaction mechanism between muscle and bone at the cellular level, promoted the mineralization of osteoblasts, participated in the occurrence and development of ALS, and had potential reference value for the clinical diagnosis of ALS (Xu et al., 2018).
In the treatment of ALS, ASC-Exo has been shown to save mitochondria-related dysfunction, which can reduce intracellular SOD1 aggregation, regulate the cell phenotype of ALS, and can be used as a candidate drug for ALS treatment (Lee et al., 2016a). Investigators treated an in vitro cell model of ALS with ASC-Exo and found that ASC-Exo rescued mitochondrial dysfunction and the mitochondrial membrane potential, thus suggesting that ASC-Exo may be used to treat diseases characterized by mitochondrial adaptations, such as ALS (Calabria et al., 2019). Subsequently, Roberta et al. tried to inject ASC-Exo into ALS mice transgenic for SOD1 (G93A) and found that this treatment significantly improved motor performance, protected lumbar motor neurons, neuromuscular junctions and muscles in ALS mice (Bonafede et al., 2020).
Discussion
Although there are a large number of studies on exosomes in nervous system disease, mainly focusing on the pathophysiological mechanism, the disease development, biomarkers, and treatment (as carriers or themselves), in addition to the biomarker studies, most of the rest in the nervous system research is in the animal model or in vitro cell culture data, especially in the treatment. Applying exosomes to therapeutic safety and technical issues is a major challenge (Chen and Chopp, 2018): (1) Cell culture conditions and storage methods may have a significant impact on the contents and functions of exosomes, which requires standardization of exosome extraction methods, storage, and functional read-out systems; (2) The content, function, and activity of exosomes depend on the generating cells of origin. So, it is necessary to optimize exosome cell sources, including age, gender, comorbidities, and other factors related to the exosome-generating cells; (3) As a therapeutic method, exosomes are mainly focused on functional aspects, but the negative effects are rarely studied. In previous studies, we can see that exosomes play a certain role in promoting neurogenesis, inhibiting neuroinflammation, promoting angiogenesis, and promoting synaptic plasticity. However, in the treatment of a disease, not all the effects are meaningful for the treatment, some even bring great risks, such as whether the treatment of other non-tumor diseases will increase the risk of cancer promotion; (4) Exosome therapy is still in the preclinical trial stage. Although it can relieve symptoms, it is still a problem whether it can promote the prognosis.
In a word, exosomes bring hope for patients with neurodegenerative diseases, which has great clinical research value, provides a new method for the diagnosis and treatment of diseases, and better clarifies the pathophysiological mechanism.
Author Contributions
PG was responsible for reviewing the literature and writing the manuscript. XL and XD participated in providing ideas for the article. SL and YX participated in revising the manuscript. All authors contributed to the article and approved the submitted version.
Funding
This work was supported by the National Natural Science Foundation of China (81971601), the National Key Research and Development Program of China (2016YFC1307004), the Multidisciplinary Team for Cognitive Impairment of Shanxi Science and Technology Innovation Training Team (201705D131027), and the Fund Program for the Scientific Activities of Selected Overseas Professionals in Shanxi Province (20200038).
Conflict of Interest
The authors declare that the research was conducted in the absence of any commercial or financial relationships that could be construed as a potential conflict of interest.
Publisher’s Note
All claims expressed in this article are solely those of the authors and do not necessarily represent those of their affiliated organizations, or those of the publisher, the editors and the reviewers. Any product that may be evaluated in this article, or claim that may be made by its manufacturer, is not guaranteed or endorsed by the publisher.
References
Agliardi, C., Guerini, F. R., Zanzottera, M., Bianchi, A., Nemni, R., and Clerici, M. (2019). SNAP-25 in Serum Is Carried by Exosomes of Neuronal Origin and Is a Potential Biomarker of Alzheimer’s Disease. Mol. Neurobiol. 56, 5792–5798. doi: 10.1007/s12035-019-1501-x
Asai, H., Ikezu, S., Tsunoda, S., Medalla, M., Luebke, J., Haydar, T., et al. (2015). Depletion of microglia and inhibition of exosome synthesis halt tau propagation. Nat. Neurosci. 18, 1584–1593. doi: 10.1038/nn.4132
Ballabh, P., Braun, A., and Nedergaard, M. (2004). The blood-brain barrier: an overview: structure, regulation, and clinical implications. Neurobiol. Dis. 16, 1–13. doi: 10.1016/j.nbd.2003.12.016
Bonafede, R., Brandi, J., Manfredi, M., Scambi, I., Schiaffino, L., Merigo, F., et al. (2019). The Anti-Apoptotic Effect of ASC-Exosomes in an In Vitro ALS Model and Their Proteomic Analysis. Cells 8:1087. doi: 10.3390/cells8091087
Bonafede, R., Turano, E., Scambi, I., Busato, A., Bontempi, P., Virla, F., et al. (2020). ASC-Exosomes Ameliorate the Disease Progression in SOD1(G93A) Murine Model Underlining Their Potential Therapeutic Use in Human ALS. Int. J. Mol. Sci. 21:3651. doi: 10.3390/ijms21103651
Bunggulawa, E., Wang, W., Yin, T., Wang, N., Durkan, C., Wang, Y., et al. (2018). Recent advancements in the use of exosomes as drug delivery systems. J. Nanobiotechnol. 16:81. doi: 10.1186/s12951-018-0403-9
Calabria, E., Scambi, I., Bonafede, R., Schiaffino, L., Peroni, D., Potrich, V., et al. (2019). ASCs-Exosomes Recover Coupling Efficiency and Mitochondrial Membrane Potential in an in vitro Model of ALS. Front. Neurosci. 13:1070. doi: 10.3389/fnins.2019.01070
Cao, X.-Y., Lu, J.-M., Zhao, Z.-Q., Li, M.-C., Lu, T., An, X.-S., et al. (2017). MicroRNA biomarkers of Parkinson’s disease in serum exosome-like microvesicles. Neurosci. Lett. 644, 94–99. doi: 10.1016/j.neulet.2017.02.045
Chanteloup, G., Cordonnier, M., Moreno-Ramos, T., Pytel, V., Matias-Guiu, J., Gobbo, J., et al. (2019). Exosomal HSP70 for Monitoring of Frontotemporal Dementia and Alzheimer’s Disease: Clinical and FDG-PET Correlation. J. Alzheimers Dis. 71, 1263–1269. doi: 10.3233/JAD-190545
Chen, J., and Chopp, M. (2018). Exosome Therapy for Stroke. Stroke 49, 1083–1090. doi: 10.1161/STROKEAHA.117.018292
Chen, Y., Xia, K., Chen, L., and Fan, D. (2019). Increased Interleukin-6 Levels in the Astrocyte-Derived Exosomes of Sporadic Amyotrophic Lateral Sclerosis Patients. Front. Neurosci. 13:574. doi: 10.3389/fnins.2019.00574
Cheng, L., Doecke, J. D., Sharples, R. A., Villemagne, V. L., Fowler, C. J., Rembach, A., et al. (2015). Prognostic serum miRNA biomarkers associated with Alzheimer’s disease shows concordance with neuropsychological and neuroimaging assessment. Mol. Psychiatry 20, 1188–1196. doi: 10.1038/mp.2014.127
Chistiakov, D. A., and Chistiakov, A. A. (2017). alpha-Synuclein-carrying extracellular vesicles in Parkinson’s disease: deadly transmitters. Acta Neurol. Belg. 117, 43–51. doi: 10.1007/s13760-016-0679-1
Colombo, M., Raposo, G., Théry, C. J. A. R. O. C., and Biology, D. (2014). Biogenesis, secretion, and intercellular interactions of exosomes and other extracellular vesicles. Annu. Rev. Cell Dev. Biol. 30, 255–289. doi: 10.1146/annurev-cellbio-101512-122326
Cui, G. H., Wu, J., Mou, F. F., Xie, W. H., Wang, F. B., Wang, Q. L., et al. (2018). Exosomes derived from hypoxia-preconditioned mesenchymal stromal cells ameliorate cognitive decline by rescuing synaptic dysfunction and regulating inflammatory responses in APP/PS1 mice. FASEB J. 32, 654–668. doi: 10.1096/fj.201700600R
Didiot, M.-C., Hall, L. M., Coles, A. H., Haraszti, R. A., Godinho, B. M., Chase, K., et al. (2016). Exosome-mediated Delivery of Hydrophobically Modified siRNA for Huntingtin mRNA Silencing. Mol. Ther. 24, 1836–1847. doi: 10.1038/mt.2016.126
Ding, M., Shen, Y., Wang, P., Xie, Z., Xu, S., Zhu, Z., et al. (2018). Exosomes Isolated From Human Umbilical Cord Mesenchymal Stem Cells Alleviate Neuroinflammation and Reduce Amyloid-Beta Deposition by Modulating Microglial Activation in Alzheimer’s Disease. Neurochem. Res. 43, 2165–2177. doi: 10.1007/s11064-018-2641-5
Dinkins, M. B., Enasko, J., Hernandez, C., Wang, G., Kong, J., Helwa, I., et al. (2016). Neutral Sphingomyelinase-2 Deficiency Ameliorates Alzheimer’s Disease Pathology and Improves Cognition in the 5XFAD Mouse. J. Neurosci. 36, 8653–8667. doi: 10.1523/JNEUROSCI.1429-16.2016
Dutta, S., Hornung, S., Kruayatidee, A., Maina, K., Del Rosario, I., Paul, K., et al. (2021). α-Synuclein in blood exosomes immunoprecipitated using neuronal and oligodendroglial markers distinguishes Parkinson’s disease from multiple system atrophy. Acta Neuropathol. 142, 495–511. doi: 10.1007/s00401-021-02324-0
Goetzl, E. J., Boxer, A., Schwartz, J. B., Abner, E. L., Petersen, R. C., Miller, B. L., et al. (2015). Altered lysosomal proteins in neural-derived plasma exosomes in preclinical Alzheimer disease. Neurology 85, 40–47. doi: 10.1212/wnl.0000000000001702
Escola, J., Kleijmeer, M., Stoorvogel, W., Griffith, J., Yoshie, O., and Geuze, H. J. (1998). Selective enrichment of tetraspan proteins on the internal vesicles of multivesicular endosomes and on exosomes secreted by human B-lymphocytes. J. Biol. Chem. 273, 20121–20127. doi: 10.1074/jbc.273.32.20121
Fernandes, A., Ribeiro, A. R., Monteiro, M., Garcia, G., Vaz, A. R., and Brites, D. (2018). Secretome from SH-SY5Y APPSwe cells trigger time-dependent CHME3 microglia activation phenotypes, ultimately leading to miR-21 exosome shuttling. Biochimie 155, 67–82. doi: 10.1016/j.biochi.2018.05.015
Fraser, K. B., Moehle, M. S., Alcalay, R. N., and West, A. B. LRRK2 Cohort Consortium. (2016a). Urinary LRRK2 phosphorylation predicts parkinsonian phenotypes in G2019S LRRK2 carriers. Neurology 86, 994–999.
Fraser, K. B., Rawlins, A. B., Clark, R. G., Alcalay, R. N., Standaert, D. G., Liu, N., et al. (2016b). Ser(P)-1292 LRRK2 in urinary exosomes is elevated in idiopathic Parkinson’s disease. Mov. Disord. 31, 1543–1550. doi: 10.1002/mds.26686
Fu, Y., Jiang, C., Tofaris, G., and Davis, J. J. (2020). Facile Impedimetric Analysis of Neuronal Exosome Markers in Parkinson’s Disease Diagnostics. Anal. Chem. 92, 13647–13651. doi: 10.1021/acs.analchem.0c03092
Gabathuler, R. (2010). Approaches to transport therapeutic drugs across the blood-brain barrier to treat brain diseases. Neurobiol. Dis. 37, 48–57. doi: 10.1016/j.nbd.2009.07.028
Gangalum, R. K., Bhat, A. M., Kohan, S. A., and Bhat, S. P. (2016). Inhibition of the Expression of the Small Heat Shock Protein alphaB-Crystallin Inhibits Exosome Secretion in Human Retinal Pigment Epithelial Cells in Culture. J. Biol. Chem. 291, 12930–12942. doi: 10.1074/jbc.M115.698530
Goetzl, E. J., Abner, E. L., Jicha, G. A., Kapogiannis, D., and Schwartz, J. B. (2018). Declining levels of functionally specialized synaptic proteins in plasma neuronal exosomes with progression of Alzheimer’s disease. FASEB J. 32, 888–893. doi: 10.1096/fj.201700731R
Goetzl, E. J., Kapogiannis, D., Schwartz, J. B., Lobach, I. V., Goetzl, L., Abner, E. L., et al. (2016). Decreased synaptic proteins in neuronal exosomes of frontotemporal dementia and Alzheimer’s disease. FASEB J. 30, 4141–4148. doi: 10.1096/fj.201600816R
Gomes, C., Keller, S., Altevogt, P., and Costa, J. (2007). Evidence for secretion of Cu, Zn superoxide dismutase via exosomes from a cell model of amyotrophic lateral sclerosis. Neurosci. Lett. 428, 43–46. doi: 10.1016/j.neulet.2007.09.024
Gomez-Molina, C., Sandoval, M., Henzi, R., Ramirez, J. P., Varas-Godoy, M., Luarte, A., et al. (2019). Small Extracellular Vesicles in Rat Serum Contain Astrocyte-Derived Protein Biomarkers of Repetitive Stress. Int. J. Neuropsychopharmacol. 22, 232–246. doi: 10.1093/ijnp/pyy098
Fries, G. R., and Quevedo, J. (2018). Exosomal MicroRNAs as Potential Biomarkers in Neuropsychiatric Disorders. Methods Mol. Biol. 1733, 79–85. doi: 10.1007/978-1-4939-7601-0_6
Grad, L. I., Pokrishevsky, E., Silverman, J. M., and Cashman, N. R. J. P. (2014a). Exosome-dependent and independent mechanisms are involved in prion-like transmission of propagated Cu/Zn superoxide dismutase misfolding. Prion 8, 331–335. doi: 10.4161/19336896.2014.983398
Grad, L. I., Yerbury, J. J., Turner, B. J., Guest, W. C., Pokrishevsky, E., O’Neill, M. A., et al. (2014b). Intercellular propagated misfolding of wild-type Cu/Zn superoxide dismutase occurs via exosome-dependent and -independent mechanisms. Proc. Natl. Acad. Sci. U. S. A. 111, 3620–3625. doi: 10.1073/pnas.1312245111
Grey, M., Dunning, C. J., Gaspar, R., Grey, C., Brundin, P., Sparr, E., et al. (2015). Acceleration of alpha-synuclein aggregation by exosomes. J. Biol. Chem. 290, 2969–2982. doi: 10.1074/jbc.M114.585703
Gui, Y., Liu, H., Zhang, L., Lv, W., and Hu, X. J. O. (2015). Altered microRNA profiles in cerebrospinal fluid exosome in Parkinson disease and Alzheimer disease. Oncotarget 6, 37043–37053. doi: 10.18632/oncotarget.6158
Rosas-Hernandez, H., Cuevas, E., Raymick, J. B., Robinson, B. L., Ali, S. F., Hanig, J., et al. (2019). Characterization of Serum Exosomes from a Transgenic Mouse Model of Alzheimer’s Disease. Curr. Alzheimer Res. 16, 388–395. doi: 10.2174/1567205016666190321155422
Hajrasouliha, A. R., Jiang, G., Lu, Q., Lu, H., Kaplan, H. J., Zhang, H. G., et al. (2013). Exosomes from retinal astrocytes contain antiangiogenic components that inhibit laser-induced choroidal neovascularization. J. Biol. Chem. 288, 28058–28067. doi: 10.1074/jbc.M113.470765
Haney, M. J., Klyachko, N. L., Zhao, Y., Gupta, R., Plotnikova, E. G., He, Z., et al. (2015). Exosomes as drug delivery vehicles for Parkinson’s disease therapy. J. Control Rel. 207, 18–30. doi: 10.1016/j.jconrel.2015.03.033
Harischandra, D. S., Ghaisas, S., Rokad, D., Zamanian, M., Jin, H., Anantharam, V., et al. (2018). Environmental neurotoxicant manganese regulates exosome-mediated extracellular miRNAs in cell culture model of Parkinson’s disease: Relevance to alpha-synuclein misfolding in metal neurotoxicity. Neurotoxicology 64, 267–277. doi: 10.1016/j.neuro.2017.04.007
Harischandra, D. S., Rokad, D., Neal, M. L., Ghaisas, S., Manne, S., Sarkar, S., et al. (2019). Manganese promotes the aggregation and prion-like cell-to-cell exosomal transmission of alpha-synuclein. Sci. Signal. 12:eaau4543. doi: 10.1126/scisignal.aau4543
Hemler, M. E. (2003). Tetraspanin proteins mediate cellular penetration, invasion, and fusion events and define a novel type of membrane microdomain. Annu. Rev. Cell. Dev. Biol. 19, 397–422. doi: 10.1146/annurev.cellbio.19.111301.153609
Hong, Y., Zhao, T., Li, X. J., and Li, S. (2017). Mutant Huntingtin Inhibits alphaB-Crystallin Expression and Impairs Exosome Secretion from Astrocytes. J. Neurosci. 37, 9550–9563. doi: 10.1523/JNEUROSCI.1418-17.2017
Howitt, J., and Hill, A. F. (2016). Exosomes in the Pathology of Neurodegenerative Diseases. J. Biol. Chem. 291, 26589–26597. doi: 10.1074/jbc.R116.757955
Hudry, E., Martin, C., Gandhi, S., Gyorgy, B., Scheffer, D. I., Mu, D., et al. (2016). Exosome-associated AAV vector as a robust and convenient neuroscience tool. Gene Ther. 23, 380–392. doi: 10.1038/gt.2016.11
Jeon, I., Cicchetti, F., Cisbani, G., Lee, S., Li, E., Bae, J., et al. (2016). Human-to-mouse prion-like propagation of mutant huntingtin protein. Acta Neuropathol. 132, 577–592. doi: 10.1007/s00401-016-1582-9
Li, J. J., Wang, B., Kodali, M. C., Chen, C., Kim, E., Patters, B. J., et al. (2018). In vivo evidence for the contribution of peripheral circulating inflammatory exosomes to neuroinflammation. J. Neuroinflam. 15:8. doi: 10.1186/s12974-017-1038-8
Kaur, S., Verma, H., Dhiman, M., Tell, G., Gigli, G., Janes, F., et al. (2021). Brain Exosomes: Friend or Foe in Alzheimer’s Disease? Mol. Neurobiol. 58, 6610–6624. doi: 10.1007/s12035-021-02547-y [Epub ahead of print].
Kojima, R., Bojar, D., Rizzi, G., Hamri, C. E., El-Baba, M. D., Saxena, P., et al. (2018). Designer exosomes produced by implanted cells intracerebrally deliver therapeutic cargo for Parkinson’s disease treatment. Nat. Commun. 9:1305. doi: 10.1038/s41467-018-03733-8
Koteswara, R., Nalamolu, I., and Venkatesh, A. (2019). Exosomes Treatment Mitigates Ischemic Brain Damage but Does Not Improve Post-Stroke Neurological Outcome. Cell. Physiol. Biochem. 52, 1280–1291. doi: 10.33594/000000090
Rajendran, L., Honsho, M., Zahn, T. R., Keller, P., Geiger, K. D., Verkade, P., et al. (2006). Alzheimer’s disease beta-amyloid peptides are released in association with exosomes. Proc. Natl. Acad. Sci. U. S. A. 103, 11172–11177. doi: 10.1073/pnas.0603838103
Lebouvier, T., Chaumette, T., Paillusson, S., Duyckaerts, C., Bruley des Varannes, S., Neunlist, M., et al. (2009). The second brain and Parkinson’s disease. Eur. J. Neurosci. 30, 735–741. doi: 10.1111/j.1460-9568.2009.06873.x
Lee, H. J. (2005). Intravesicular Localization and Exocytosis of -Synuclein and its Aggregates. J. Neurosci. 25, 6016–6024. doi: 10.1523/jneurosci.0692-05.2005
Lee, M., Ban, J. J., Kim, K. Y., Jeon, G. S., Im, W., Sung, J. J., et al. (2016a). Adipose-derived stem cell exosomes alleviate pathology of amyotrophic lateral sclerosis in vitro. Biochem. Biophys. Res. Commun. 479, 434–439. doi: 10.1016/j.bbrc.2016.09.069
Lee, M., Ban, J. J., Yang, S., Im, W., and Kim, M. (2018). The exosome of adipose-derived stem cells reduces beta-amyloid pathology and apoptosis of neuronal cells derived from the transgenic mouse model of Alzheimer’s disease. Brain Res. 1691, 87–93. doi: 10.1016/j.brainres.2018.03.034
Lee, M., Im, W., and Kim, M. (2021). Exosomes as a potential messenger unit during heterochronic parabiosis for amelioration of Huntington’s disease. Neurobiol. Dis. 155:105374. doi: 10.1016/j.nbd.2021.105374
Lee, M., Liu, T., Im, W., and Kim, M. (2016b). Exosomes from adipose-derived stem cells ameliorate phenotype of Huntington’s disease in vitro model. Eur. J. Neurosci. 44, 2114–2119. doi: 10.1111/ejn.13275
Lee, S., Im, W., Ban, J., Lee, M., Jung, K., Lee, S., et al. (2017). Exosome-Based Delivery of miR-124 in a Huntington’s Disease Model. J. Mov. Disord. 10, 45–52. doi: 10.14802/jmd.16054
Leng, B., Sun, H., Zhao, J., Liu, Y., Shen, T., Liu, W., et al. (2020). Plasma exosomal prion protein levels are correlated with cognitive decline in PD patients. Neurosci. Lett. 723:134866. doi: 10.1016/j.neulet.2020.134866
Lugli, G., Cohen, A. M., Bennett, D. A., Shah, R. C., Fields, C. J., Hernandez, A. G., et al. (2015). Plasma Exosomal miRNAs in Persons with and without Alzheimer Disease: Altered Expression and Prospects for Biomarkers. PLoS One 10:e0139233. doi: 10.1371/journal.pone.0139233
Pascual, M., Ibáñez, F., and Guerri, C. (2020). Exosomes as mediators of neuron-glia communication in neuroinflammation. Neural. Regen. Res. 15, 796–801. doi: 10.4103/1673-5374.268893
McKeever, P. M., Schneider, R., Taghdiri, F., Weichert, A., Multani, N., Brown, R. A., et al. (2018). MicroRNA Expression Levels Are Altered in the Cerebrospinal Fluid of Patients with Young-Onset Alzheimer’s Disease. Mol. Neurobiol. 55, 8826–8841. doi: 10.1007/s12035-018-1032-x
Mendt, M., Kamerkar, S., Sugimoto, H., McAndrews, K., Wu, C., Gagea, M., et al. (2018). Generation and testing of clinical-grade exosomes for pancreatic cancer. JCI Insight 3:e99263. doi: 10.1172/jci.insight.99263
Hayashi, N., Doi, H., Kurata, Y., Kagawa, H., Atobe, Y., Funakoshi, K., et al. (2019). Proteomic analysis of exosome-enriched fractions derived from cerebrospinal fluid of amyotrophic lateral sclerosis patients. Neurosci. Res. 160, 43–49. doi: 10.1016/j.neures.2019.10.010
Nafar, F., Williams, J. B., and Mearow, K. M. (2016). Astrocytes release HspB1 in response to amyloid-beta exposure in vitro. J. Alzheimers Dis. 49, 251–263. doi: 10.3233/JAD-150317
Niu, M., Li, Y., Li, G., Zhou, L., Luo, N., Yao, M., et al. (2020). A longitudinal study on alpha-synuclein in plasma neuronal exosomes as a biomarker for Parkinson’s disease development and progression. Eur. J. Neurol. 27, 967–974. doi: 10.1111/ene.14208
Ouyang, Q., Meng, Y., Zhou, W., Tong, J., Cheng, Z., and Zhu, Q. (2021). New advances in brain-targeting nano-drug delivery systems for Alzheimer’s disease. J. Drug Target 1–21. doi: 10.1080/1061186x.2021.1927055 [Epub ahead of print].
Palop, J. J., Chin, J., and Mucke, L. (2006). A network dysfunction perspective on neurodegenerative diseases. Nature 443, 768–773. doi: 10.1038/nature05289
Papadopoulos, V. E., Nikolopoulou, G., Antoniadou, I., Karachaliou, A., Arianoglou, G., Emmanouilidou, E., et al. (2018). Modulation of beta-glucocerebrosidase increases alpha-synuclein secretion and exosome release in mouse models of Parkinson’s disease. Hum. Mol Genet. 27, 1696–1710. doi: 10.1093/hmg/ddy075
Pegtel, D., and Gould, S. J. (2019). Exosomes. Annu. Rev. Biochem. 88, 487–514. doi: 10.1146/annurev-biochem-013118-111902
Perets, N., Betzer, O., Shapira, R., Brenstein, S., Angel, A., Sadan, T., et al. (2019). Golden Exosomes Selectively Target Brain Pathologies in Neurodegenerative and Neurodevelopmental Disorders. Nano. Lett. 19, 3422–3431. doi: 10.1021/acs.nanolett.8b04148
Perocheau, D., Touramanidou, L., Gurung, S., Gissen, P., and Baruteau, J. (2021). Clinical applications for exosomes: Are we there yet? Br. J. Pharmacol. 178, 2375–2392. doi: 10.1111/bph.15432
Qu, M., Lin, Q., Huang, L., Fu, Y., Wang, L., He, S., et al. (2018). Dopamine-loaded blood exosomes targeted to brain for better treatment of Parkinson’s disease. J. Control Rel. 287, 156–166. doi: 10.1016/j.jconrel.2018.08.035
Quek, C., and Hill, A. F. (2017). The role of extracellular vesicles in neurodegenerative diseases. Biochem. Biophys. Res. Commun. 483, 1178–1186. doi: 10.1016/j.bbrc.2016.09.090
Rastogi, S., Sharma, V., Bharti, P., Rani, K., Modi, G., Nikolajeff, F., et al. (2021). The Evolving Landscape of Exosomes in Neurodegenerative Diseases: Exosomes Characteristics and a Promising Role in Early Diagnosis. Int. J. Mol. Sci. 22:440. doi: 10.3390/ijms22010440
Riancho, J., Vazquez-Higuera, J. L., Pozueta, A., Lage, C., Kazimierczak, M., Bravo, M., et al. (2017). MicroRNA Profile in Patients with Alzheimer’s Disease: Analysis of miR-9-5p and miR-598 in Raw and Exosome Enriched Cerebrospinal Fluid Samples. J. Alzheimers Dis. 57, 483–491. doi: 10.3233/JAD-161179
Kalimuthu, S., Gangadaran, P., Rajendran, R. L., Zhu, L., Oh, J. M., Lee, H. W., et al. (2018). A New Approach for Loading Anticancer Drugs Into Mesenchymal Stem Cell-Derived Exosome Mimetics for Cancer Therapy. Front. Pharmacol. 9:1116. doi: 10.3389/fphar.2018.01116
Lakhal, S., and Wood, M. J. (2011). Exosome nanotechnology: an emerging paradigm shift in drug delivery: exploitation of exosome nanovesicles for systemic in vivo delivery of RNAi heralds new horizons for drug delivery across biological barriers. Bioessays 33, 737–741. doi: 10.1002/bies.201100076
Saeedi, S., Israel, S., Nagy, C., and Turecki, G. (2019). The emerging role of exosomes in mental disorders. Transl. Psychiatry 9:122. doi: 10.1038/s41398-019-0459-9
Samanta, S., Rajasingh, S., Drosos, N., Zhou, Z., Dawn, B., and Rajasingh, J. (2018). Exosomes: new molecular targets of diseases. Acta Pharmacol. Sin. 39, 501–513. doi: 10.1038/aps.2017.162
Sardar Sinha, M., Ansell-Schultz, A., Civitelli, L., Hildesjo, C., Larsson, M., Lannfelt, L., et al. (2018). Alzheimer’s disease pathology propagation by exosomes containing toxic amyloid-beta oligomers. Acta Neuropathol. 136, 41–56. doi: 10.1007/s00401-018-1868-1
Si, X., Tian, J., Chen, Y., Yan, Y., Pu, J., and Zhang, B. (2019). Central Nervous System-Derived Exosomal Alpha-Synuclein in Serum May Be a Biomarker in Parkinson’s Disease. Neuroscience 413, 308–316. doi: 10.1016/j.neuroscience.2019.05.015
Silverman, J. M., Fernando, S. M., Grad, L. I., Hill, A. F., Turner, B. J., Yerbury, J. J., et al. (2016). Disease Mechanisms in ALS: Misfolded SOD1 Transferred Through Exosome-Dependent and Exosome-Independent Pathways. Cell Mol. Neurobiol. 36, 377–381. doi: 10.1007/s10571-015-0294-3
Skotland, T., Sandvig, K., and Llorente, A. (2017). Lipids in exosomes: Current knowledge and the way forward. Prog. Lipid Res. 66, 30–41. doi: 10.1016/j.plipres.2017.03.001
Sun, X., Jung, J. H., Arvola, O., Santoso, M. R., Giffard, R. G., Yang, P. C., et al. (2019). Stem Cell-Derived Exosomes Protect Astrocyte Cultures From in vitro Ischemia and Decrease Injury as Post-stroke Intravenous Therapy. Front. Cell. Neurosci. 13:394. doi: 10.3389/fncel.2019.00394
Sweeney, M., Sagare, A., and Zlokovic, B. V. (2018). Blood-brain barrier breakdown in Alzheimer disease and other neurodegenerative disorders. Nat. Rev. Neurol. 14, 133–150. doi: 10.1038/nrneurol.2017.188
Steinbichler, T. B., Dudás, J., Riechelmann, H., and Skvortsova, I. I. (2017). The role of exosomes in cancer metastasis. Semin. Cancer Biol. 44, 170–181. doi: 10.1016/j.semcancer.2017.02.006
Tian, T., Zhang, H. X., He, C. P., Fan, S., Zhu, Y. L., Qi, C., et al. (2018). Surface functionalized exosomes as targeted drug delivery vehicles for cerebral ischemia therapy. Biomaterials 150, 137–149. doi: 10.1016/j.biomaterials.2017.10.012
Trams, E., Lauter, C., Salem, N., and Heine, U. (1981). Exfoliation of membrane ecto-enzymes in the form of micro-vesicles. Biochim. Biophys. Acta 645, 63–70. doi: 10.1016/0005-2736(81)90512-5
Geng, W., Tang, H., Luo, S., Lv, Y., Liang, D., Kang, X., et al. (2019). Exosomes from miRNA-126-modified ADSCs promotes functional recovery after stroke in rats by improving neurogenesis and suppressing microglia activation. Am. J. Transl. Res. 11, 780–792.
Wang, H., Sui, H., Zheng, Y., Jiang, Y., Shi, Y., Liang, J., et al. (2019a). Curcumin-primed exosomes potently ameliorate cognitive function in AD mice by inhibiting hyperphosphorylation of the Tau protein through the AKT/GSK-3beta pathway. Nanoscale 11, 7481–7496. doi: 10.1039/c9nr01255a
Wang, L., Yin, P., Wang, J., Wang, Y., Sun, Z., Zhou, Y., et al. (2019b). Delivery of mesenchymal stem cells-derived extracellular vesicles with enriched miR-185 inhibits progression of OPMD. Artif Cells Nanomed. Biotechnol. 47, 2481–2491. doi: 10.1080/21691401.2019.1623232
Wang, W., Li, D. B., Li, R. Y., Zhou, X., Yu, D. J., Lan, X. Y., et al. (2018). Diagnosis of Hyperacute and Acute Ischaemic Stroke: The Potential Utility of Exosomal MicroRNA-21-5p and MicroRNA-30a-5p. Cerebrovasc. Dis. 45, 204–212. doi: 10.1159/000488365
Wang, Y., Balaji, V., Kaniyappan, S., Kruger, L., Irsen, S., Tepper, K., et al. (2017). The release and trans-synaptic transmission of Tau via exosomes. Mol. Neurodegener. 12:5. doi: 10.1186/s13024-016-0143-y
Watson, L., Hamlett, E., Stone, T., and Sims-Robinson, C. J. M. (2019). Neuronally derived extracellular vesicles: an emerging tool for understanding Alzheimer’s disease. Mol. Neurodegener. 14:22. doi: 10.1186/s13024-019-0317-5
Wei, Z. X., Xie, G. J., Mao, X., Zou, X. P., Liao, Y. J., Liu, Q. S., et al. (2020). Exosomes from patients with major depression cause depressive-like behaviors in mice with involvement of miR-139-5p-regulated neurogenesis. Neuropsychopharmacology 45, 1050–1058. doi: 10.1038/s41386-020-0622-2
Winston, C. N., Aulston, B., Rockenstein, E. M., Adame, A., Prikhodko, O., Dave, K. N., et al. (2019). Neuronal Exosome-Derived Human Tau is Toxic to Recipient Mouse Neurons in vivo. J. Alzheimers Dis. 67, 541–553. doi: 10.3233/JAD-180776
Xu, Q., Zhao, Y., Zhou, X., Luan, J., Cui, Y., and Han, J. (2018). Comparison of the extraction and determination of serum exosome and miRNA in serum and the detection of miR-27a-3p in serum exosome of ALS patients. Intractable Rare Dis. Res. 7, 13–18. doi: 10.5582/irdr.2017.01091
Yao, Y. F., Gao, Q., Li, J. L., Xue, C. X., Fang, W., Jing, J. H., et al. (2018). Circulating exosomal miRNAs as diagnostic biomarkers in Parkinson’s disease. Eur. Rev. Med. Pharmacol. Sci. 22, 5278–5283.
Yang, J., Zhang, X., Chen, X., Wang, L., and Yang, G. (2017). Exosome Mediated Delivery of miR-124 Promotes Neurogenesis after Ischemia. Mol. Ther. Nucleic Acids 7, 278–287. doi: 10.1016/j.omtn.2017.04.010
Yohei, I., Lara, E., Martin, P., Geneviève, S., Christine, B., Yuichi, R., et al. (2016). Exosome secretion is a key pathway for clearance of pathological TDP-43. Brain 139, 3187–3201. doi: 10.1093/brain/aww237
Zhang, H., Wu, J., Wu, J., Fan, Q., Zhou, J., Wu, J., et al. (2019). Exosome-mediated targeted delivery of miR-210 for angiogenic therapy after cerebral ischemia in mice. J. Nanobiotechnol. 17, 29. doi: 10.1186/s12951-019-0461-7
Zhao, Z. H., Chen, Z. T., Zhou, R. L., Zhang, X., Ye, Q. Y., and Wang, Y. Z. (2018). Increased DJ-1 and alpha-Synuclein in Plasma Neural-Derived Exosomes as Potential Markers for Parkinson’s Disease. Front. Aging Neurosci. 10:438. doi: 10.3389/fnagi.2018.00438
Zheng, H., Xie, Z., Zhang, X., Mao, J., Wang, M., Wei, S., et al. (2021). Investigation of α-Synuclein Species in Plasma Exosomes and the Oligomeric and Phosphorylated α-Synuclein as Potential Peripheral Biomarker of Parkinson’s Disease. Neuroscience 469, 79–90. doi: 10.1016/j.neuroscience.2021.06.033
Keywords: exosomes, neurodegenerative diseases, pathophysiology, biomarkers, treatment
Citation: Gao P, Li X, Du X, Liu S and Xu Y (2021) Diagnostic and Therapeutic Potential of Exosomes in Neurodegenerative Diseases. Front. Aging Neurosci. 13:790863. doi: 10.3389/fnagi.2021.790863
Received: 07 October 2021; Accepted: 02 December 2021;
Published: 16 December 2021.
Edited by:
Anamitra Ghosh, Wave Life Sciences Ltd., United StatesReviewed by:
Wen Xiong, Baylor College of Medicine, United StatesVinod Kumar, The University of Queensland, Australia
An Phu Tran Nguyen, Van Andel Institute, United States
Copyright © 2021 Gao, Li, Du, Liu and Xu. This is an open-access article distributed under the terms of the Creative Commons Attribution License (CC BY). The use, distribution or reproduction in other forums is permitted, provided the original author(s) and the copyright owner(s) are credited and that the original publication in this journal is cited, in accordance with accepted academic practice. No use, distribution or reproduction is permitted which does not comply with these terms.
*Correspondence: Sha Liu, bGl1c2hhQHN4bXUuZWR1LmNu; Yong Xu, eHV5b25nQHN4bXUuZWR1LmNu; eHV5b25nc211QHZpcC4xNjMuY29t
†These authors have contributed equally to this work