- 1Department of Neuroscience, University of Sheffield, Sheffield, United Kingdom
- 2Department of Life Sciences, Brunel University London, Uxbridge, United Kingdom
Decline in self-awareness is a prevalent symptom in Alzheimer’s disease (AD). Current data suggest that an early breakdown in the brain’s default mode network (DMN) is closely associated with the main symptomatic features in AD patients. In parallel, the integrity of the DMN has been shown to be heavily implicated in retained self-awareness abilities in healthy individuals and AD patients. However, the global contribution to awareness skills of other large-scale networks is still poorly understood. Resting-state functional magnetic resonance imaging (rs-fMRI) scans were acquired and pre-processed from 53 early-stage AD individuals. A group-level independent component analysis was run to isolate and reconstruct four intrinsic connectivity large-scale brain functional networks, namely left and right central executive fronto-parietal networks (FPN), salience network, and anterior and posterior DMN. Hypothesis-driven seed-based connectivity analyses were run to clarify the region-specific underpinnings of multi-domain anosognosia. Multiple regression models were run on large-scale network- and seed-based connectivity maps, including scores of memory, non-memory and total anosognosia obtained via the Measurement of Anosognosia Questionnaire. Memory anosognosia scores were associated with selective lower fronto-temporal connectivity and higher parieto-temporal connectivity. Non-memory anosognosia scores were associated with higher connectivity between the anterior DMN and the cerebellum, between the left medial prefrontal seeds and the contralateral prefrontal cortex, and between the left hippocampal seed and the left insula; lower connectivity was observed between the right prefrontal cortex and the right lingual seed. Lastly, total anosognosia scores were associated with large-scale network alterations, namely reduced left-FPN expression in the left posterior cingulate, reduced right-FPN expression in the left inferior lingual gyrus and adjacent inferior occipital cortex, and increased right-FPN expression in the right anterior cingulate. Seed-based analyses yielded significant connectivity differences only in the connectivity pattern associated with the left hippocampal seed by displaying lower intercommunication with the right prefrontal cortex, but higher connectivity with the left caudate nucleus. These findings support the hypothesis that alterations in functional connectivity of frontal lobe regions involved in executive-related mechanisms represent the neural correlates of domain-specific anosognosia in early AD. Up-regulated connectivity with subcortical structures appears to contribute to changes in the network dynamics interplay and fosters the appearance of anosognosia.
Introduction
Anosognosia in Alzheimer’s disease (AD) is a prevalent symptom that can be defined as the inability of a patient to recognize decline in their own cognitive functioning (Hanseeuw et al., 2020). The clinical manifestations of anosognosia at an individual level affect efficiency in the various cognitive domains in a highly heterogeneous way (Starkstein, 2014). The onset of this symptom, however, tends to occur quite early on throughout the clinical timeline, either at the stage of mild cognitive impairment (MCI), or even before that, during the preclinical period when individuals who are still psychometrically normal but report subjective cognitive complaints might show poorer awareness of the extent of their decline than their informant would report (Cacciamani et al., 2017; Hanseeuw et al., 2020). Anosognosia might not manifest only as lack of awareness of a memory impairment but can also apply to other cognitive domains, such as unawareness of executive dysfunction, of socio-emotional deficits or of difficulties with daily life activities (Lacerda et al., 2021). This heterogeneity highlights the multidimensionality of this phenomenon and warrants the necessity for a multi-domain clinical assessment (Leicht et al., 2010; de Ruijter et al., 2020).
The use of resting-state fMRI (rs-fMRI) in AD holds great potential as an early diagnostic biomarker, as evidenced by studies that found disruptions of intrinsic large-scale network connectivity in the prodromal and mild AD stages (Sorg et al., 2007; Vemuri et al., 2012; Antoine et al., 2019). It is now well recognized that three of the brain main large-scale functional networks play a crucial role in efficient human cognition, namely the default mode network (DMN), the nodes of which are centered in the medial prefrontal cortex, posterior cingulate cortex/precuneus and medial temporal cortices, including the hippocampus (Raichle, 2015; Alves et al., 2019), the salience network, of which the anterior cingulate cortex and insula are the most representative hubs, and the central executive fronto-parietal network, with its computational regions found predominantly in the dorsolateral prefrontal and posterior parietal cortices (Bressler and Menon, 2010). Published evidence suggests that DMN down-regulation is significantly associated with cognitive decline during the early clinical stages of AD (Greicius et al., 2004; Badhwar et al., 2017; Grieder et al., 2018). At the same time, however, functional integrity of DMN hubs is implicated in self-awareness abilities, as evidenced in healthy individuals (Northoff et al., 2006; Davey et al., 2016). Consequently, AD patients with alterations affecting the DMN might have difficulties with self-awareness or may manifest anosognosia very early in the course of the disease.
To clarify the role of dysfunction in regions of the DMN when anosognosia is present in AD, partial insight is provided by fMRI studies that investigated hemodynamic activation in patients with AD during tasks tapping self-awareness abilities. These studies reported activation of fronto-parietal (Ruby et al., 2009), fronto-cingulate (Ries et al., 2007; Amanzio et al., 2011), and fronto-temporal (Zamboni et al., 2013) regions during tasks of self-reflection and perspective-changing, with an additional, yet limited, activation of posteromedial parietal regions (Amanzio et al., 2011). When resting-state activity within the DMN was studied in relation to anosognosia in this patient population, Berlingeri et al. (2015) found that anosognosia for memory impairment was associated with reduced connectivity of the DMN network, the left lateral temporal cortex, the hippocampus and the insula. Perrotin et al. (2015) found reduced connectivity between the medial temporal lobe and both orbitofrontal cortex and posterior cingulate. Vannini et al. (2017) showed reduced connectivity between the precuneus and the bilateral inferior parietal lobe, the left posterior cingulate and orbitofrontal cortex, and between the right hippocampus and the fusiform gyrus. Lastly, Mondragón et al. (2021) showed that stronger connectivity of the bilateral anterior cingulate cortex was associated with anosognosia in prodromal AD. Notably, regardless of the applied methodology, all task-based and rs-fMRI studies reported an involvement of the frontal lobe cortex and/or anterior cingulate cortex as the essential neural substrates modulating multi-dimensional awareness and self-appraisal abilities (Ries et al., 2007; Amanzio et al., 2011; Zamboni et al., 2013; Perrotin et al., 2015; Vannini et al., 2017; Antoine et al., 2019; Mondragón et al., 2021).
At the cognitive level, the most influential theoretical framework at the basis of disorders of awareness is the Cognitive Awareness Model (Agnew and Morris, 1998). This model posits that a “Mnemonic Comparator” based on executive resources plays a major role in sustaining awareness. It has, thus, been proposed that loss of functional integrity within fronto-temporo-parietal networks (including the DMN) may result in dysfunctional changes to the comparator system, and this, in turn, may lead to deficits in self-awareness abilities (Tagai et al., 2020). In this respect, an association between anosognosia and the integrity of the DMN has been reported by multiple studies (Zamboni and Wilcock, 2011; Antoine et al., 2019; Mondragon et al., 2019). Furthermore, the presence of anosognosia at the MCI stage has been found to predict hypometabolism in regions of the DMN and progression to dementia at a 2-year follow up (Therriault et al., 2018). The role that other, non-DMN large-scale networks play in the genesis and persistence of this symptom, however, is still poorly understood. Clarifying in more detail the changes to the overall neurofunctional circuitry (i.e., which networks are involved and in what direction the association is observed) that are associated with domain-specific anosognosia would provide valuable insights into the mechanisms fostering the presence of this symptom in AD.
Although the reviewed literature provides some evidence of the involvement of resting-state functional connectivity in the mechanisms of anosognosia in early AD, no study has yet investigated seed-based and data-driven (i.e., as informed by latent-variable models) pathways in a systematic way, including non-DMN networks, and by differentiating anosognosia for memory and non-memory impairments. Relying on the “Mnemonic Comparator” notion introduced as part of the Cognitive Awareness Model (Agnew and Morris, 1998), we hypothesized that connectivity alterations ascribable to fronto-limbic dysfunction would be associated with single-domain (i.e., “memory”/”non-memory”) and multi-domain (i.e., memory plus non-memory, or “total”) anosognosia in the early stage of AD. In addition, we also hypothesized that alterations to the DMN of adaptive and compensatory nature, such as increased abnormal network-to-network interplay, would be deployed (and, thus, would emerge as statistically significant) in the presence of anosognosia.
Materials and Methods
Experimental Design
This study followed a correlational design in which measures of domain-specific (memory and non-memory) and total anosognosia and statistical maps of brain activity extracted from fMRI images acquired in resting state were entered into linear regression models to explore the relationship between different brain network activities and patients’ levels of cognitive awareness.
Participants
Fifty-three patients with cognitive impairment were included in this study. Recruitment of patients and administration of study procedures were carried out as an ancillary study of the EU-funded Virtual Physiological Human---DementiA Research Enabled by IT initiative1, a multicenter project coordinated by the Department of Neuroscience, University of Sheffield, United Kingdom. All eligible patients for the VPH-DARE initiative at our sites were also approached to take part in this ancillary study. All patients were recruited consecutively and all were approached if they also met the eligibility criterion for this additional study, i.e., having a reliable informant. Our intention was to cover the entire continuum of early AD clinical severity (i.e., from MCI to mild dementia) in order to maximize numerical variability of outcome variables (i.e., voxel-by-voxel indices of connectivity) as well as of the main predictors (i.e., the anosognosia scores). Of the total sample, n = 24 had a clinical diagnosis of mild AD, as per the National Institute of Aging diagnostic criteria typically implemented in clinical settings (McKhann et al., 2011) and n = 29 had a diagnosis of MCI due to AD (Albert et al., 2011) based on clinical, neuropsychological and structural neuroimaging biomarkers of neuronal injury (Albert et al., 2011, p. 8). Patient diagnoses were corroborated by regular clinical longitudinal follow ups that were carried out for at least 4 years after recruitment. In all cases included in this report the clinical course and structural imaging findings were supportive of an AD etiology. A series of exclusion criteria (applied at recruitment and, retrospectively, at each clinical follow up examination) was defined to rule out signs and symptoms suggestive of a condition incompatible with the objective of this study. This included: significant neurological conditions (e.g., history of acute or chronic cerebrovascular disease or transient ischemic attacks), history of epilepsy or presence of uncontrolled brain seizures, peripheral neuropathy, significant neuropsychiatric symptoms or evidence of radiological abnormalities (other than those seen as part of the typical AD profile); cardiovascular and gastroenterological conditions (e.g., sick-sinus syndrome or peptic ulcer), metabolic disorders (e.g., abnormal levels of vitamin B12, folates or thyroid stimulating hormone), major pharmacological interventions (e.g., treatment with psychotropic medication other than AD-related drugs, pharmacological interventions displaying important organic adverse effects or medications used in other research protocols) and presence of major disabilities significantly affecting daily life activities. Since the indices of anosognosia were obtained from patient-caregiver dyads, patients from the primary study without a reliable informant were not offered participation. A brief screening of caregivers’ neurological status was completed by a senior clinician to rule out neurological or psychological factors that could have prevented them from providing reliable information. This study received ethical approval by the Regional Ethics Committee of Yorkshire and Humber (Ref No: 12/YH/0474). In accordance with data-collection procedures approved by the European Union, ethical approval was reiterated by the relevant local ethics committees at recruitment sites. Written informed consent was obtained from all participants.
Anosognosia Assessment
The Measurement of Anosognosia Instrument (Stewart et al., 2010) was used to quantify the patients’ level of (or lack of) awareness of difficulties in a set of daily life scenarios. This questionnaire consists of 15 dichotomical “yes/no” questions on cognitive deficits that may manifest during daily life routines and the responses are split into two functional domains: “memory” (9 items) and “non-memory” (including anosognosia for executive dysfunction and anosognosia for deficits in daily life activities; 6 items). All 15 questions must be answered independently by the patient and by the informant. As a result, two set of scores are obtained: that provided by the informant (construed as the “standard-of-truth” of the patient’s abilities) and that provided by the patient as a self-evaluative measure. Individual informant-based and patient-based responses were compared to quantify the number of discrepant answers (i.e., higher discrepancy scores indicate a higher degree of anosognosia). Discrepancy scores were used to quantify the presence of domain-specific anosognosia (“memory,” max score: 9; “non-memory,” max score: 6) and a “total” score that was also obtained by the sum of the memory and non-memory scores (max score: 15) (Migliorelli et al., 1995; Stewart et al., 2010).
Functional Magnetic Resonance Imaging Acquisition and Pre-Processing
Brain MRI data were acquired following the protocol for multicenter harmonization for Philips MRI scanners defined by the VPH-DARE@IT consortium. Images were acquired on two Philips scanners. The following parameters were used for acquisition of resting state functional MRI (rs-fMRI) images on the 3 T Philips Ingenia scanner: 35 axial slices, reconstructed in-plane voxel dimensions = 1.8 × 1.8 mm2, slice thickness = 4.0 mm, 128 × 128 matrix size, 230 mm field of view, repetition time = 2.6 s, echo time = 35 ms, flip angle = 90°, number of temporal dynamics = 125. Acquisitions of rs-fMRI images on the Philips Achieva 1.5 T scanner had the following specifications: voxel size: 3.28 × 3.28 mm2, slice thickness = 6.00 mm, 64 × 64 matrix size, 230 mm field of view, repetition time = 2 s, echo time = 50 ms and flip angle = 90°, number of temporal dynamics = 240. As per protocol, 20 s of dummy acquisitions were set at the beginning of each sequence to enable the scanner to reach electromagnetic equilibrium. A T1-weighted image was also obtained for each participant as part of the MRI protocol.
Functional brain images were processed with Statistical Parametric Mapping (SPM) 12 software running in MATLAB R2014a (V.8.3) through a standardized fMRI data pre-processing pipeline that included slice-timing, realignment, normalization, temporal band-pass filtering (0.01–0.1 Hz) and a 6-mm full-width at half-maximum Gaussian kernel smoothing (Postema et al., 2019). In addition, total intracranial volumes were computed from the T1-weighted scans using the get_totals MATLAB script2 and summing the maps of gray matter, white matter and cerebrospinal fluid volumes.
Independent Component Analysis
Pre-processed rs-fMRI images were analyzed with a group independent component analysis (ICA), to extract functional large-scale network connectivity maps. The GIFT toolbox (v1.3i)3 was used to this end. The Infomax optimization principle was applied and the number of components to be extracted set at 20, as a reliable number that typically separates human resting-state connectivity into its fundamental networks (Wang and Li, 2015). Individual independent component maps were reconstructed for each of the five main brain functional networks of interest and these were selected via visual inspection that was carried out independently by the three co-authors with 100% agreement. These five pathways were the anterior DMN (aDMN), the posterior DMN (pDMN), the left frontoparietal network (l-FPN), the right frontoparietal network (r-FPN), and the salience network. Large-scale network connectivity beta-score maps were then extracted for each participant for group-level inferential modeling.
Seed-Signal Extraction and Seed-Based First Level Analyses
Region of interest (ROI) analyses were carried out on each individual patient’s rs-fMRI acquisition to define the association between the hemodynamic signal extracted from a series of seed regions and the signal throughout the entire brain. The resulting maps are typically interpreted as the functional connectivity of those seed regions. Cytoarchitectonically-defined seed regions were created using the WFU PickAtlas toolbox and AAL human brain atlas (Tzourio-Mazoyer et al., 2002; Maldjian et al., 2003). Informed by the Cognitive Awareness Model and based on previous neuroimaging research (Mondragon et al., 2019), the following 9 ROIs were chosen: anterior cingulate cortex, medial prefrontal cortex, medial orbitofrontal cortex, precuneus, and posterior cingulate cortex were selected for their established role in self-awareness (Northoff et al., 2006), while precentral gyrus, lingual gyrus, fusiform gyrus, and hippocampus were selected based on published evidence suggesting that these are part of the neural substrate associated with levels of anosognosia in the early stages of AD (Valera-Bermejo et al., 2020). Seed time-courses were extracted independently for the left and right hemispheres (18 seeds in total) with the SPM-based MarsBaR toolbox4 (Brett et al., 2002).
First-level analyses were devised in the form of general linear models with the seed time-course as predictor. The physiological signal from the global maps of white matter and cerebrospinal fluid was regressed out to minimize the impact of non-neural sources of variability. Twenty-four parameters related to head-motion i.e., the six rigid-body transformation regressors generated during realignment, their temporal derivatives, and all squared values were also regressed out (max. translational motion 3 mm and max. rotational motion 3°). This methodology also allowed for functional scans to address and minimize the impact of the differences in magnetic field strength as there is evidence that signal extent and intensity are not affected by this effect, but signal-to-noise ratio may variate depending on magnetic field-strength (Voss et al., 2006).
Group-Level Data Modeling
Multiple regression analyses were carried out at group level to test the functional associations of domain-specific (i.e., memory and non-memory) and total anosognosia scores with large-scale network connectivity maps extracted through ICA and individual ROI maps obtained through seed-based first-level analysis. The cluster-forming threshold of significance was set at p = 0.005 uncorrected. Clusters were considered significant only if they survived a family-wise error (FWE)-corrected threshold of p < 0.05 (Whitwell, 2009). All statistical models were controlled for age, years of education, total intracranial volume, and normalized hippocampal volumes (Cardoso et al., 2013). The latter served to control for an index of neurodegeneration-informed disease severity. Peak regions were transposed from Montreal Neurological Institute coordinates to the Talairach space, to permit identification through the Talairach Daemon Client, version 2.4.3 (Lancaster et al., 2000).
Results
Demographics
Demographic and sample characteristics are reported in Table 1.
Large-Scale Network Results
Significant associations emerged in some of the networks of interest (the extent of the clusters in voxels is indicated with k). Negative associations were found between expression of the l-FPN and both memory (k = 298, p = 0.02) and total anosognosia scores (k = 375, p = 0.006). These were in limbic-occipital regions, namely the left lingual gyrus and left posterior cingulate (Table 2 and Figure 1). Conversely, a significant positive association was found between expression of the r-FPN and total anosognosia (k = 264, p = 0.036). This was located in the left lingual gyrus and left inferior occipital gyrus (Table 2 and Figure 2). Along the same directional lines, positive associations were found between non-memory anosognosia scores and aDMN expression in the cerebellar culmen, bilaterally (k = 263, p = 0.038), and between total anosognosia and aDMN expression in the right anterior cingulate (k = 261, p = 0.039; Table 2 and Figure 3). No significant associations were found in the pDMN or salience network.
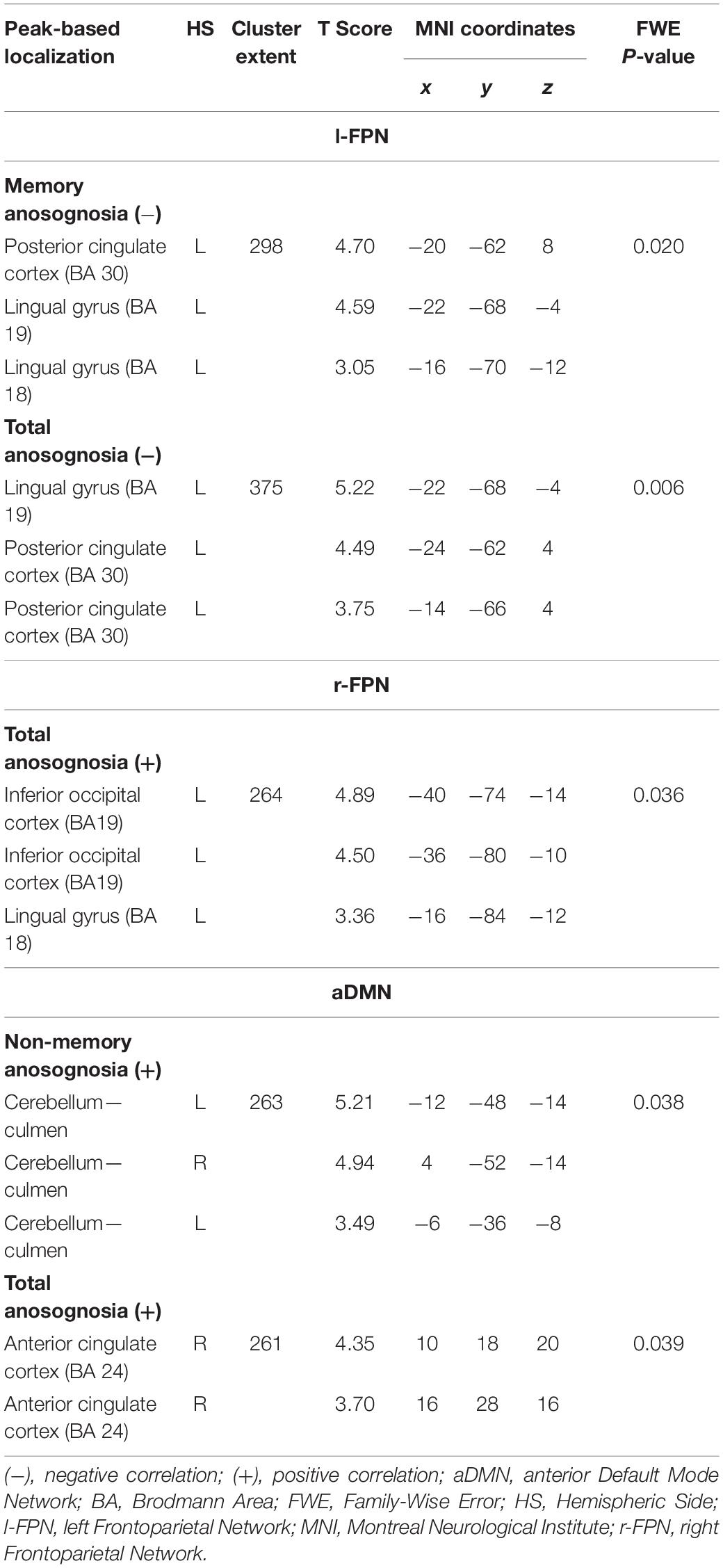
Table 2. Results emerging from large-scale brain functional networks obtained via independent component analysis.
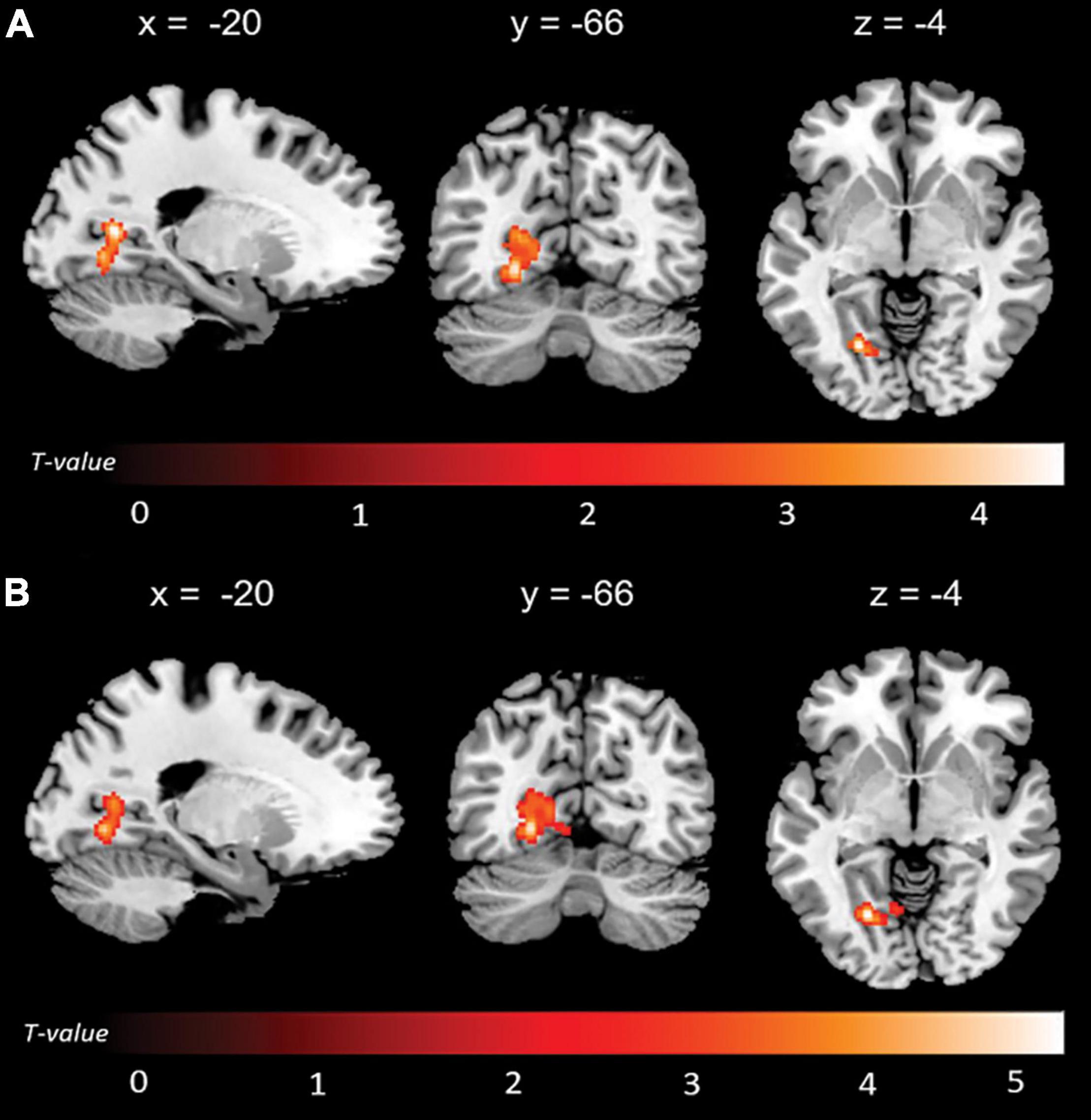
Figure 1. Negative correlations between functional connectivity of the left fronto-parietal network (l-FPN) and (A) memory and (B) total anosognosia.
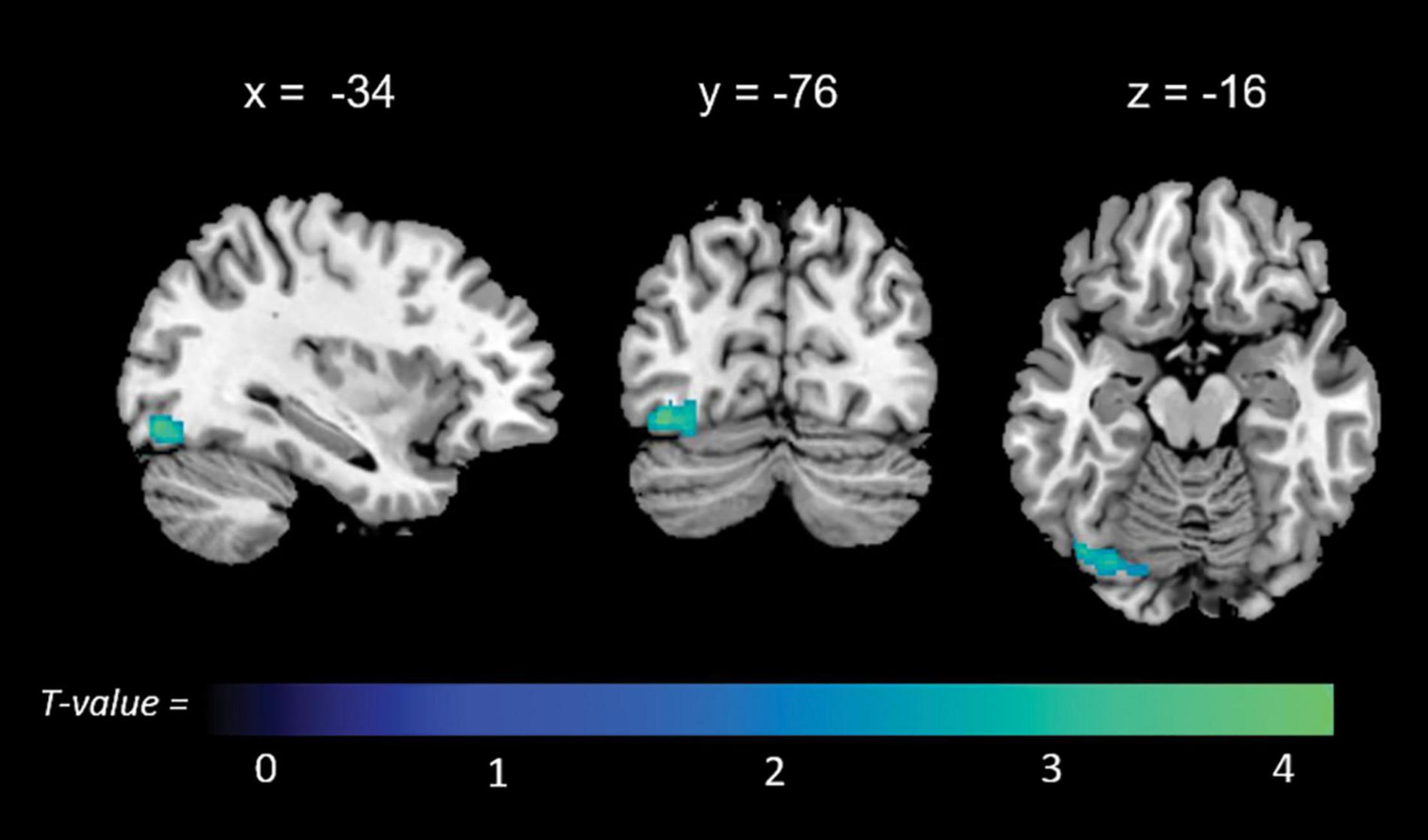
Figure 2. Positive correlations between functional connectivity of the right frontoparietal network (r-FPN) and total anosognosia.
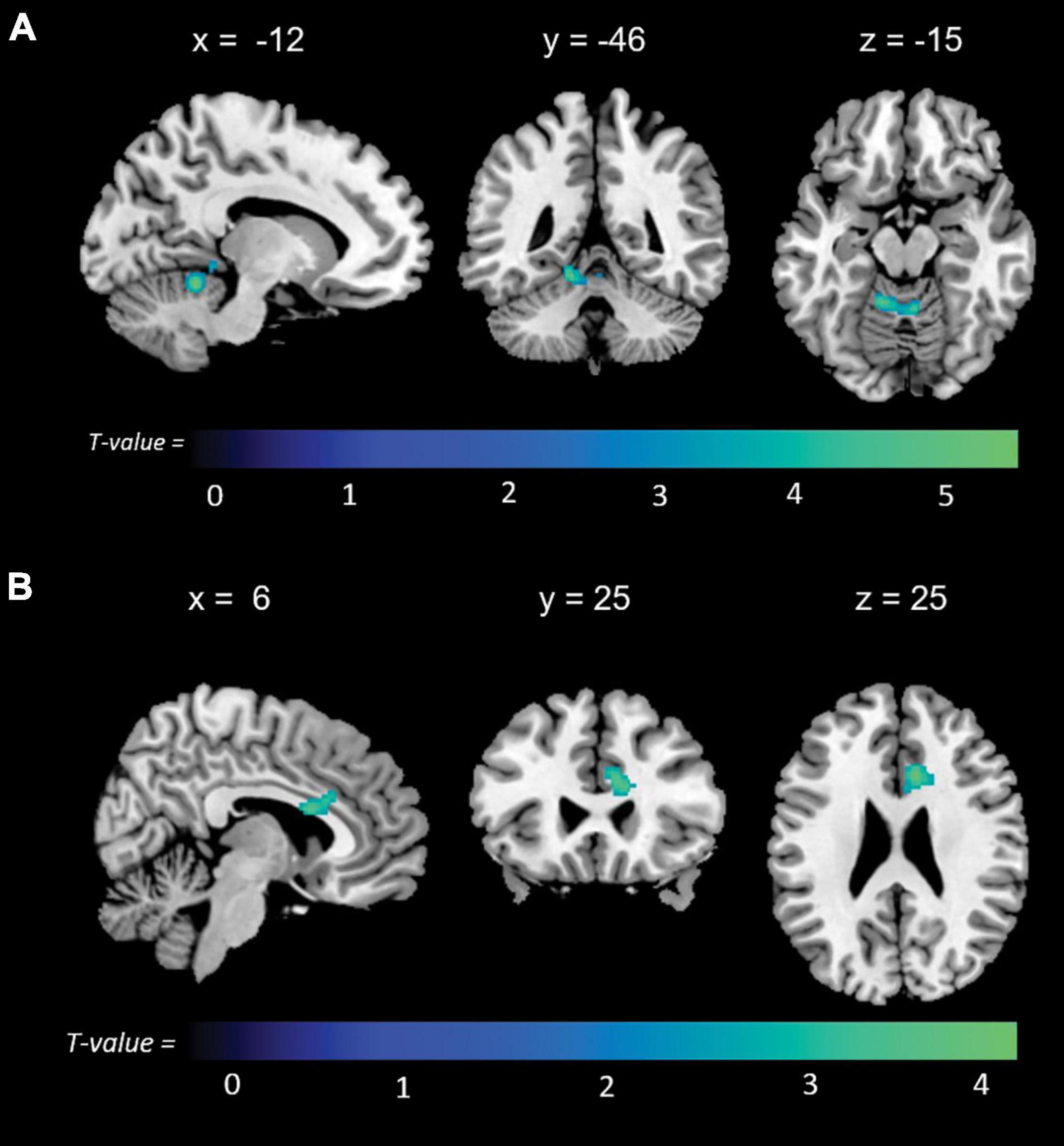
Figure 3. Positive correlations between functional connectivity of the anterior default mode network (aDMN) and (A) non-memory and (B) total anosognosia.
Seed-Based Analyses Results
Memory anosognosia scores were negatively associated with: right anterior cingulate connectivity in a cluster extending from the right fusiform gyrus (BA 20) to the caudate (k = 383, p = 0.025); right hippocampal connectivity in the territory of the bilateral caudate and right thalamus (k = 394, p = 0.016); and left hippocampal connectivity in the right dorsolateral prefrontal cortex (k = 333, p = 0.040). Memory anosognosia scores were also positively associated with: right precuneal connectivity in the left fusiform and lingual gyri (k = 358, p = 0.028); right posterior cingulate connectivity in the right inferior occipital gyrus and cuneus (k = 407, p = 0.025); and left anterior cingulate connectivity in the precentral and postcentral gyri (k = 346, p = 0.029). All these results are summarized in Table 3 and Figure 4.
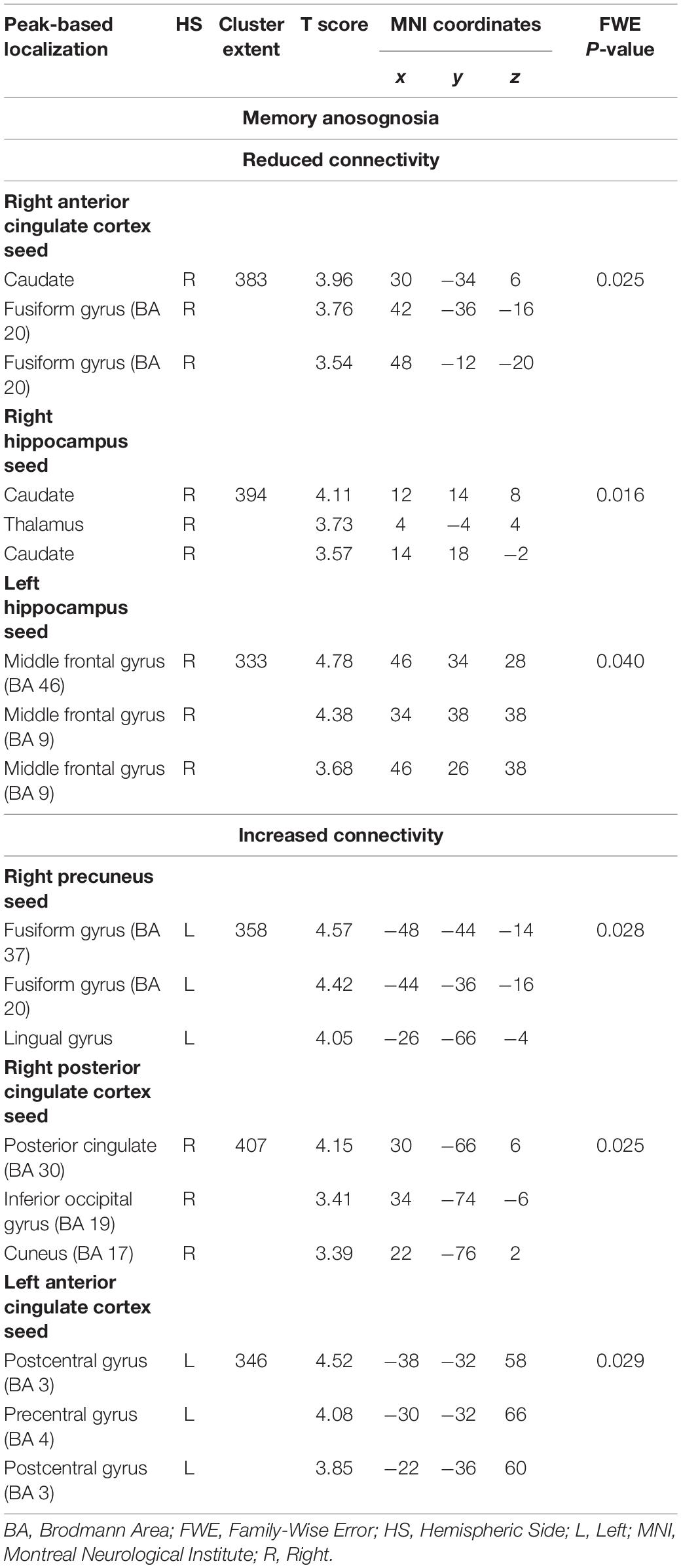
Table 3. Neural correlates of memory anosognosia emerging from functional-connectivity patterns obtained via seed-based analysis.
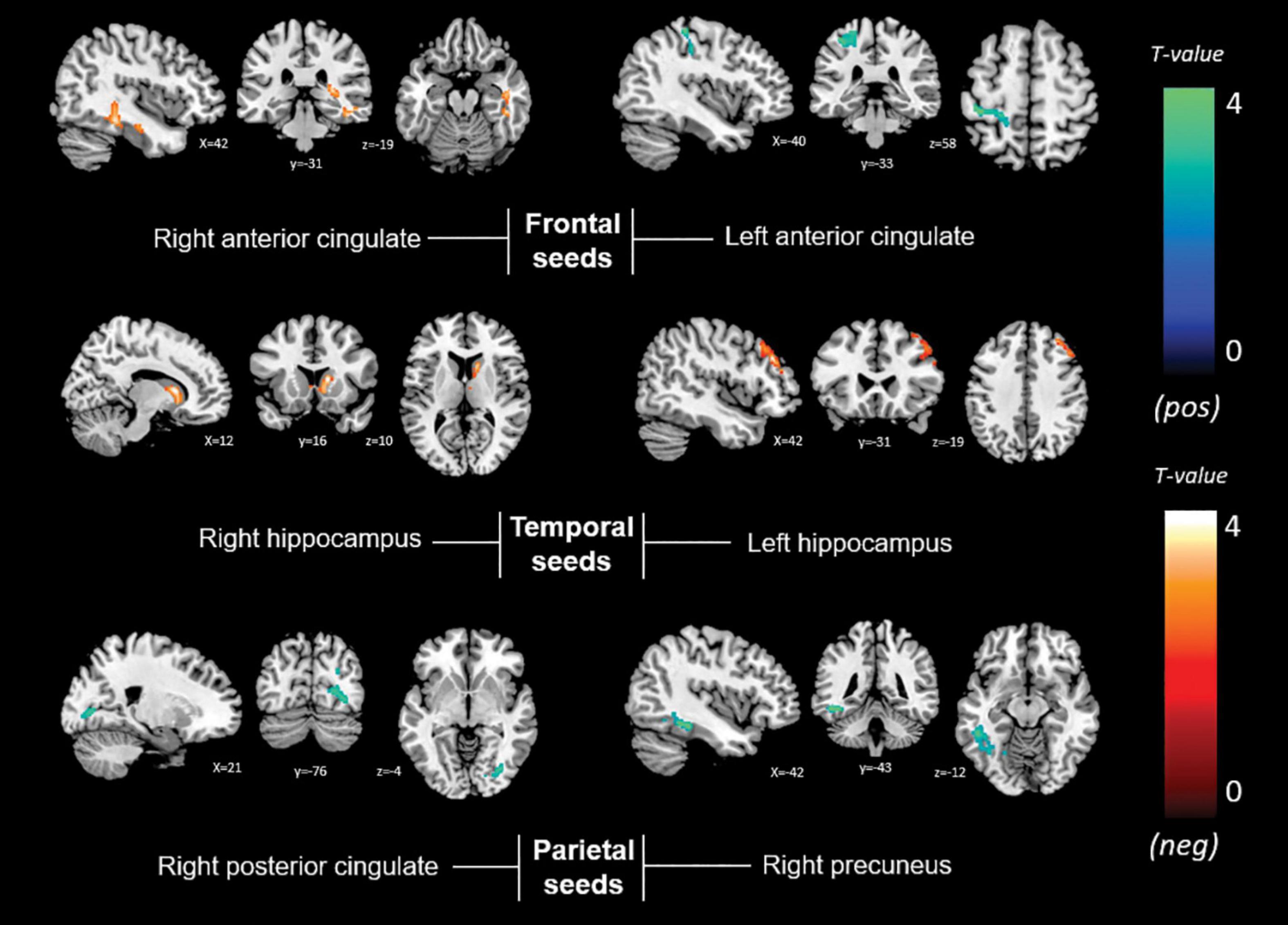
Figure 4. Positive (blue) and negative (red) correlations between brain functional connectivity of selected unilateral seed regions and memory anosognosia scores.
Non-memory anosognosia scores were negatively associated with: right lingual connectivity in the right dorsolateral prefrontal cortex (k = 590, p = 0.002); right precuneal connectivity in the right transverse temporal gyrus (BA 41) and in a caudate-thalamic area (k = 802, p = 0.001). Non-memory anosognosia scores were positively associated with: left anterior-cingulate connectivity in the right dorsolateral prefrontal cortex (k = 434, p = 0.012); left medioprefrontal connectivity in the right dorsolateral prefrontal cortex (k = 318, p = 0.048); and left hippocampal connectivity in a left caudate-insular cluster (k = 354, p = 0.031). All these findings are shown in Table 4 and Figure 5.
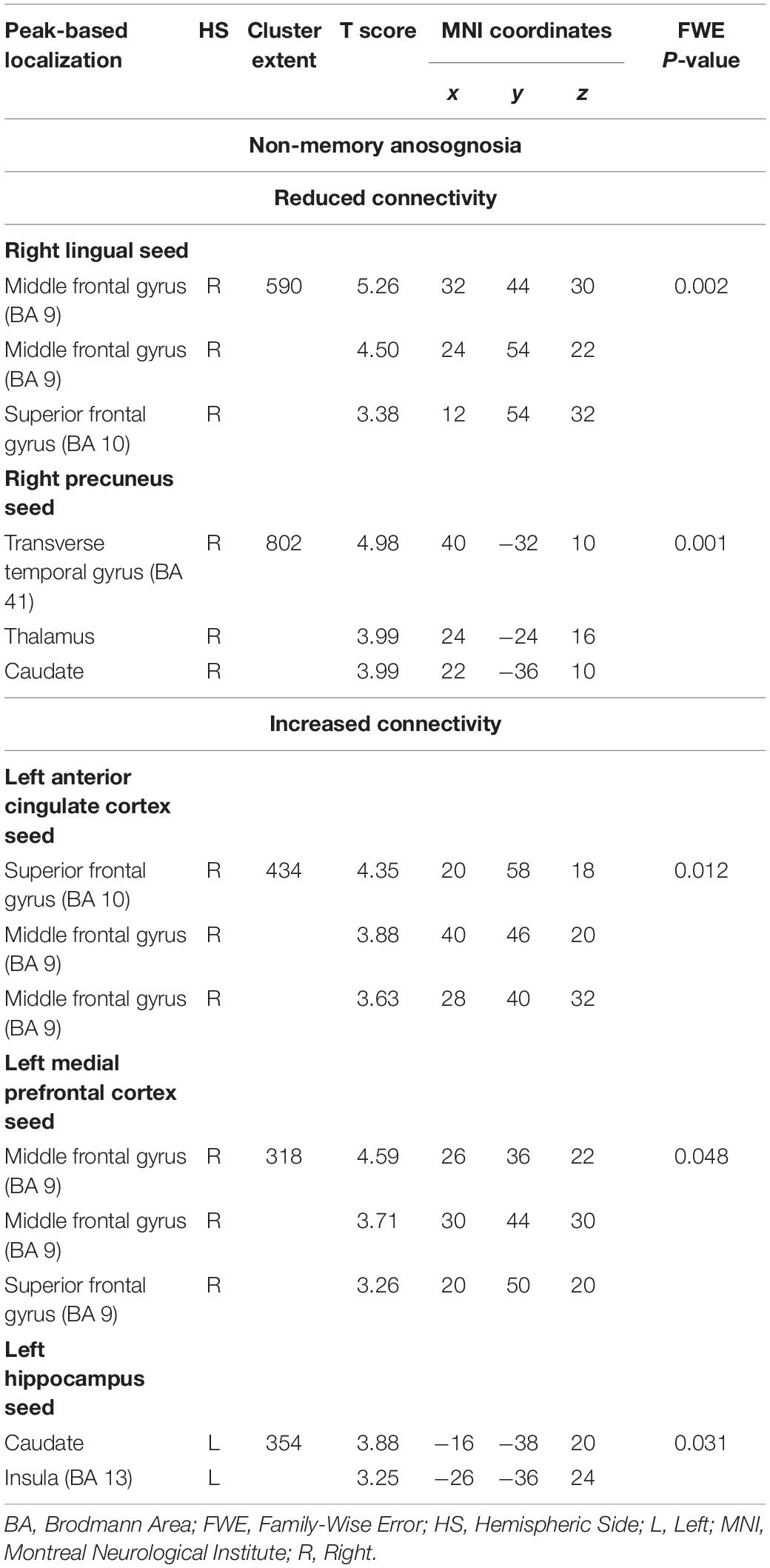
Table 4. Neural correlates of non-memory anosognosia emerging from functional-connectivity patterns obtained via seed-based analysis.
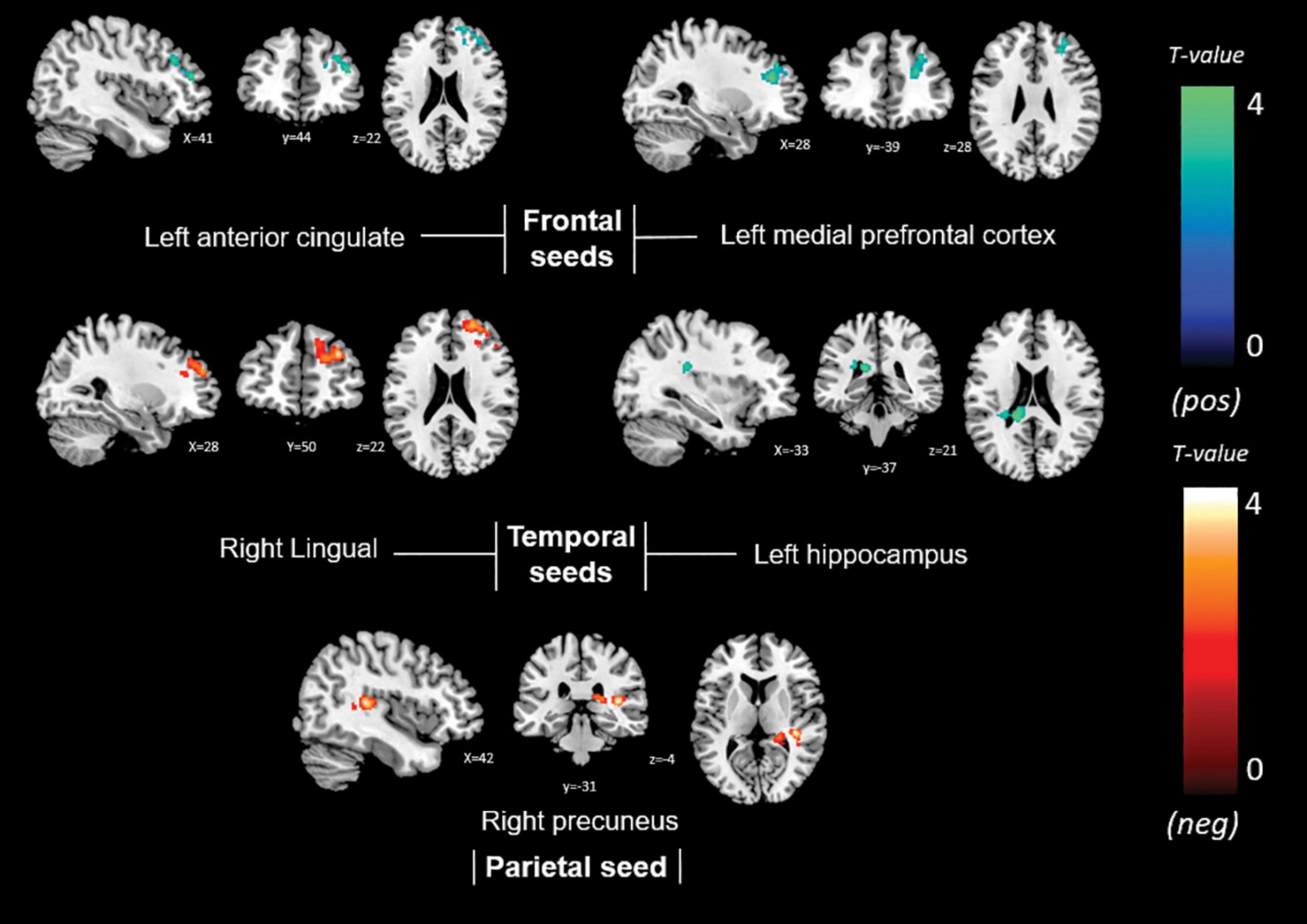
Figure 5. Positive (blue) and negative (red) correlations between brain functional connectivity of selected unilateral seed regions and non-memory anosognosia scores.
Total anosognosia scores, finally, were negatively associated with left hippocampal connectivity in the right dorsolateral prefrontal cortex (k = 457, p = 0.009), and positively associated with the left caudate nucleus (k = 330, p = 0.042). These findings are reported in Table 5 and Figure 6.
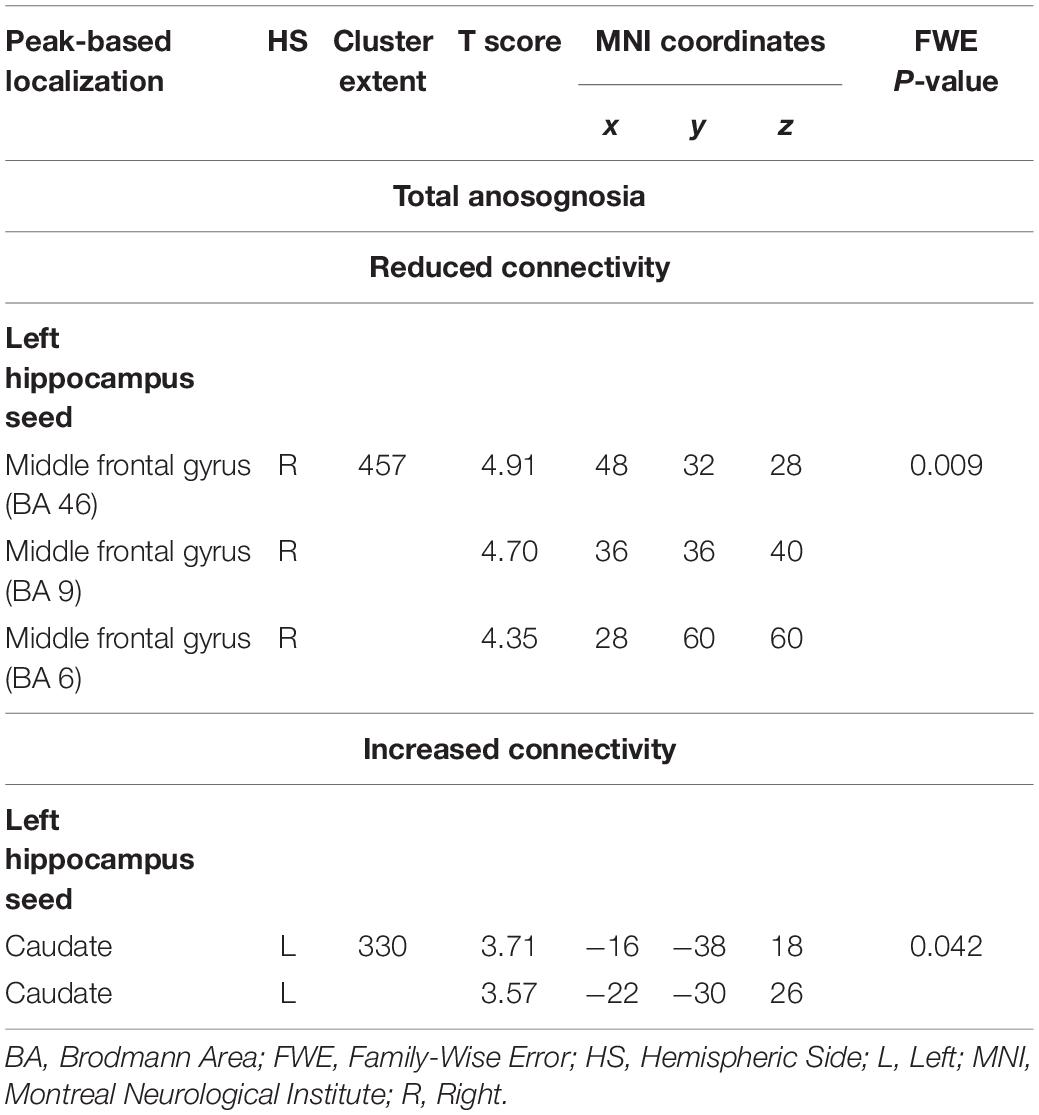
Table 5. Neural correlates of total anosognosia emerging from functional-connectivity patterns obtained via seed-based analysis.
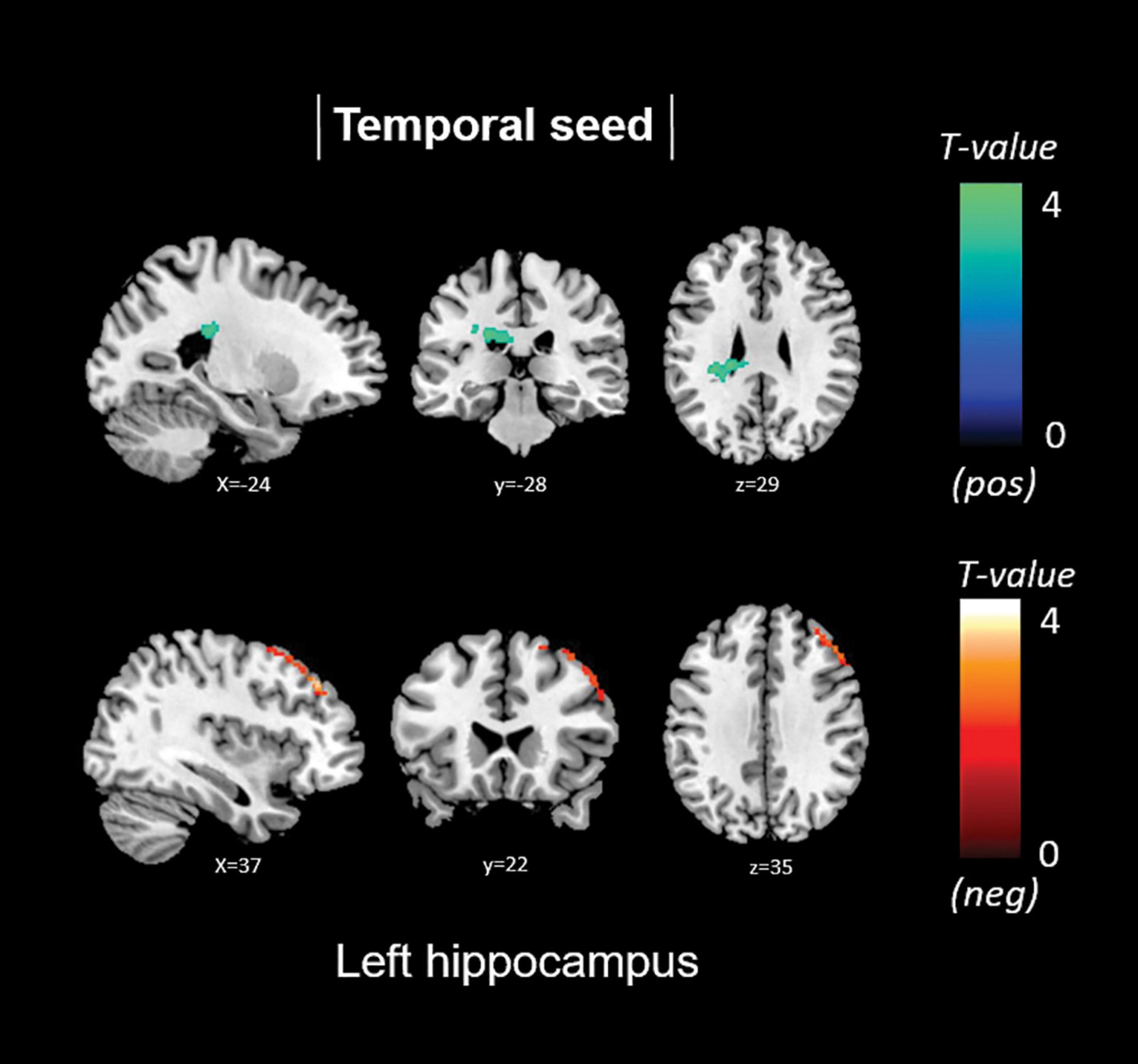
Figure 6. Positive (blue) and negative (red) correlations between brain functional connectivity of selected unilateral seed regions and total anosognosia scores.
Discussion
The present study provides evidence of a specific combination of functional brain network dynamic changes associated with multi-domain anosognosia in the early stages of AD. A better understanding of the functional substrates of domain-specific anosognosia provides insight into how disconnection of structures at the basis of the Cognitive Awareness Model translates into different mechanisms, leading to impairment of self-awareness.
Functional Connectivity Associated With Memory Anosognosia
Unawareness of memory deficits was linked to specific patterns of functional connectivity of large-scale neural networks and region-to-region pathways. Memory anosognosia scores were linked negatively to l-FPN functional connectivity within the left lingual gyrus and posterior cingulate (a DMN node). In addition, seed-based models showed a negative link with structures in the frontotemporal territory, i.e., the right fusiform gyrus (right anterior cingulate seed, aDMN associated structure), and the right dorsolateral prefrontal cortex (left hippocampal seed, DMN associated structure). It appears, therefore, that within the main human large-scale neural functional networks, there might be a possible inter-network down-regulation of FPN-DMN connectivity leading to memory anosognosia. A negative association was then found in the pathway between the right caudate and both right anterior cingulate and right hippocampal seeds. The involvement of temporal cortical structures in early AD patients presenting with higher memory anosognosia scores and functional connectivity alterations follows a pattern aligned with the pathophysiological progression of clinical AD. In this context, degeneration of the medial temporal lobe induces disruption of memory performance, but at the same time neuronal loss in these regions hampers functional interactions between crucial areas leading to reduced awareness of memory difficulties (Chavoix and Insausti, 2017). A histopathological study showed that AD patients with anosognosia had increased amyloid plaque density in the hippocampal pre-subiculum at post-mortem (Marshall et al., 2004). This finding, however, could have been partly driven by the severe pathological changes that typically affect the medial temporal lobe regions in the final stages of the disease. Pioneering studies that explored the neurofunctional substrates of memory anosognosia in AD through single-photon emission computerized tomography (Reed et al., 1993; Starkstein et al., 1995; Derouesne et al., 1999; Hanyu et al., 2008) or positron-emission tomography (Harwood et al., 2005; Jedidi et al., 2014) have highlighted right frontal cortical structures as the most consistent neural correlate. In a functional MRI study by Zamboni et al. (2013), AD patients showed decreased frontotemporal brain activation in relation to self-awareness. This evidence is aligned with our findings detecting an association with frontotemporal connectivity.
Additionally, memory anosognosia scores were also positively associated with connectivity between the right precuneus seed and the left lingual and fusiform gyrus and between the left anterior cingulate seed and left postcentral gyrus. Two recurring regions from our pattern of findings were the fusiform and lingual gyri. These structures have also been associated with anosognosia and reduced brain gray matter volumes in early AD (Guerrier et al., 2018; Valera-Bermejo et al., 2020). Functional alterations of the fusiform (Vannini et al., 2017) and lingual gyrus (Mitelpunkt et al., 2020) have been found to be associated with anosognosia in AD and might have strong links with regions within a broader network in charge of sustaining cognitive awareness. Increased frontal and parietal activity was found by Ruby et al. (2009) in a functional task-based fMRI study that showed higher activation in the prefrontal cortex of healthy controls in relation to AD patients when engaging in self-awareness tasks, while AD patients had higher activation of parietal cortices when engaging in tasks based on self-monitoring activities.
Functional Connectivity Associated With Non-memory Anosognosia
Anosognosia for executive and everyday-life deficits was positively associated with aDMN connectivity in the cerebellum. Increased connectivity in the cerebellar cortex within the aDMN could contribute to improving the cross-talk with functional networks that are involved in higher cognitive functions, as it has been shown that structures in the cerebellum provide substantial contributions to cognition, e.g., executive functions (Nowrangi et al., 2014; Xu et al., 2020). Our findings also stress the relevance of subcortical structures in contributing to the DMN in the presence of self-awareness impairment, as evidenced by the role played by the cerebellum (Buckner et al., 2011; Alves et al., 2019).
Similarly, the more anosognosic, the stronger the functional connectivity pattern between left anterior brain seeds (anterior cingulate, a salience network node, and medioprefrontal cortex, a DMN node) and the right dorsolateral prefrontal cortex (a FPN node). A positive association was also found in the pattern of left hippocampal connectivity (DMN associated) with the left insular cortex (a salience network node) and left caudate. Findings in the insula show consistency with those in the literature centered on the neuroscience of self-awareness (Lou et al., 2017). One study that highlighted gray matter volumetric changes in left insular-hippocampal regions was carried out by Sánchez-Benavides et al. (2018) who found that individuals self-assessed as unaware of cognitive decline had larger gray matter insular volumes compared with individuals displaying subjective cognitive decline. Additionally, informant-related reports of cognitive changes (unrelated to self-awareness status) were associated with smaller gray matter volume in the left hippocampus. The latter study provides valuable insights about a link between insular-hippocampal alterations in psychometrically-normal older adults and abnormalities in cognitive awareness. While we found higher connectivity between the left hippocampus and insula in relation to non-memory anosognosia, Berlingeri et al. (2015) reported reduced connectivity between these same structures in dementia patients, but for memory anosognosia. These results indicate that insulo-mediotemporal regions disconnection might be pivotal for the onset of anosognosia, with the insula serving as a vicarious salience system in the presence of unawareness for executive and daily life functions, and a “mnemonic” hub relating instead to memory unawareness. Moreover, the insula has been found to be concomitantly active alongside the prefrontal cortex and anterior cingulate in tasks requiring self-referential processing, such as interoception, self-recognition, or socio-emotional awareness (Craig, 2009; Modinos et al., 2009). Higher connectivity with the executive fronto-parietal network in this structure has been found in association with higher scores on tasks involving awareness of others (tasks of social cognition/theory of mind) in patients with early stage AD (Valera-Bermejo et al., 2021). The insula, a core region of the salience network, might up-regulate its functional connectivity toward regions typically targeted by the AD etiopathological cascade to support network function, as a way to allocate additional neural resources in support of dysfunctional DMN pathways, in an attempt to minimize the loss of connectivity and, thus, sustain the circuits that are responsible for acknowledging the presence of illness. In summary, it seems that non-memory anosognosia might be modulated by the up-regulation of the executive frontoparietal and salience network in the presence of a weakened DMN, although any attempted compensatory effect is ultimately unsuccessful.
Additionally, significant negative associations were found within the map of right lingual connectivity in the right dorsolateral prefrontal cortex and within the map of right precuneal connectivity in right subcortical regions (caudate and thalamus). The findings of this study bring compelling evidence of an involvement of the right dorsolateral prefrontal cortex in modulating awareness of cognitive difficulties, with both higher connectivity observed in the left frontal lobe and lower connectivity observed in the right lingual cortex. The main nodes of the central-executive FPN are centered in the dorsolateral prefrontal cortex and posterior parietal cortex (Bressler and Menon, 2010). The dorsolateral prefrontal cortex has been associated with executive functions and working memory and a selective age-related vulnerability has been observed in the older population (MacPherson et al., 2002). Moreover, the right dorsolateral prefrontal cortex involvement in AD patients presenting with anosognosia has been evidenced in the current literature across multiple neuroimaging approaches (Reed et al., 1993; Starkstein et al., 1995; Ries et al., 2012). There is evidence that this region is part of an executive-control network that, in conjunction with the anterior cingulate cortex, coordinates behavior aimed at accomplishing life-related objectives (Cohen et al., 2000; Amanzio et al., 2011; Xu et al., 2020). In addition, the contribution of the dorsolateral prefrontal cortex to declarative memory has been established in AD (Kumar et al., 2017; Turriziani et al., 2019), as this structure is involved in working memory for manipulation and updating of conscious information that operates in close relation with a central executive system (Funahashi, 2017). Furthermore, the executive control network appears to rely on cerebro-cerebellar support in the presence of executive decline (Xu et al., 2020). Bi-directional dorsolateral prefrontal connectivity (i.e., a positive association found in contralateral frontal structures and a negative association found in ipsilateral temporal regions) might be at the basis of a role played by this region in sustaining adaptive cognitive control required by abilities such as attention (Gbadeyan et al., 2016).
Functional Connectivity Associated With Total Anosognosia
Total anosognosia scores in this early AD sample were negatively associated with l-FPN expression in the left posterior cingulate, and seed-based analyses yielded significant negative connectivity associations between the left hippocampal seed and the right dorsolateral prefrontal cortex. These findings translate into changes in inter-network communication; indeed, the posterior cingulate and hippocampal regions are intrinsically associated with the DMN while the dorsolateral prefrontal cortex supports the executive fronto-parietal network (Bressler and Menon, 2010; Alves et al., 2019). Therefore, these findings provide evidence of reduced inter-communication between the DMN and the executive fronto-parietal networks.
Positive associations were found with r-FPN expression in the left inferior lingual gyrus and adjacent inferior occipital cortex, and between the aDMN expression in the right anterior cingulate (a salience network node). Therefore, total anosognosia might be the symptomatic expression of abnormally increased up-regulation of DMN-Salience inter-network connectivity in support of cognitive shifting of reduced self-related internal abilities to external salient stimuli. In addition, seed-based analyses revealed positive significant connectivity associations between the left hippocampal seed and the left caudate nucleus. The interplay between the fronto-parietal network and the lingual gyrus is in line with a hypothesis of frontally-mediated control of disorders of awareness. The lingual gyrus is a region essential for visual perception (Yang et al., 2015), but it also plays an executive role, as shown in a study that reported activation during a divergent thinking paradigm (Zhang et al., 2016).
A comprehensive overview of the results in the present study indicates that the anterior cingulate cortex has a strong involvement in the overall clinical manifestation of multi-domain anosognosia. Structural changes are commonly seen later than functional changes in the course of AD (Ewers et al., 2011), but there is, however, evidence that variability in gray matter of the anterior cingulate cortex is related to non-memory and total anosognosia in early AD (Valera-Bermejo et al., 2020). The present rs-fMRI findings extend the involvement of this structure to anosognosia for multiple cognitive domains. Regardless of the methodological approach, the anterior cingulate has been significantly implicated in modulating levels of awareness of symptoms in early AD (Hanyu et al., 2008; Amanzio et al., 2011; Zamboni et al., 2013; Guerrier et al., 2018; Mondragón et al., 2021). These results show consistency with the premise that midline anterior brain structures are essential for self-awareness and self-referential processing (Northoff et al., 2006; Lou et al., 2017). Structure and function of the anterior cingulate cortex could play a significant role alongside the resources deployed as part of the executive supporting system. This region could serve a major role in the executive comparator system described within the Cognitive Awareness Model (Agnew and Morris, 1998), in which the presence of synaptic dysfunction in anterior midline structures could generate a mismatch in the perceived reality and lead, therefore, to an event of cognitive unawareness. On this note, executive impairment, measured through a composite neuropsychological score, was shown to be associated with hypometabolism in the anterior cingulate in patients with a PET-informed diagnosis of prodromal AD, regardless of the extent of their amyloid burden (Yoon et al., 2019). Notably, the anterior cingulate has been found to be centrally involved in the manifestation of neuropsychiatric symptoms in AD (Boublay et al., 2016), where anosognosic symptoms are also listed as part of a spectrum of behavioral disorders that affects this clinical population (Tagai et al., 2020). In this context, the anterior cingulate cortex can be considered a core region that modulates behavior through reward, motivation, and initiation, i.e., the ability to commence a task (Devinsky et al., 1995). This could result in patients presenting with self-awareness deficits and displaying higher behavioral alterations when losing aspects of their sense of self.
Subcortical Contributions to Anosognosia
Anosognosia scores were also associated with subcortical connectivity. Memory anosognosia scores were negatively associated with connectivity between the frontal and temporal cortex and the bilateral caudate and right thalamus. Non-memory anosognosia scores were also negatively associated with connectivity between the right precuneus and both right thalamus and caudate. Reduced functional connectivity between the medioprefrontal cortex and the caudate was also reported to be associated with the presence of memory anosognosia in early AD (Ries et al., 2012).
Increased connectivity was evidenced between the left hippocampus and left caudate. The latter pattern of results was also replicated when total anosognosia scores were analyzed. Subcortical contributions have been reported in dementia patients who overestimate their overall cognitive functions or during emotional control (Shany-Ur et al., 2014). This finding has been interpreted in the context of some subcortical regions being associated with the dopaminergic system that is involved in reward actions based on self-centered attention, resulting from life accomplishments (Shany-Ur et al., 2014). Dopaminergic activity has been proposed to enhance function in paralimbic structures involved in self-referential processing. Through their projections, these regions provide subcortical support to medial frontal cortical regions during conscious self-monitoring (Lou et al., 2017). Therefore, structural and functional alterations might result from adaptive, although ineffective, up-regulation of dopaminergic inputs in a systemic attempt to cope with symptoms related to unawareness.
In summary, taken together, the pattern of findings suggests that in the early stage of neurodegeneration there might be a rearrangement of activity within the main functional network dynamic interactions that normally support full awareness of cognitive function. Increases in activity can be interpreted as a surge in system effort that increases demands on neural resources without, however, succeeding in sustaining cognitive function. Increases in neural activity that do not result in successful performance have been reported before in MCI and early stage AD (e.g., Gardini et al., 2015) and interpreted as a progressive maladaptive reorganization of how neural resources are allocated and an early coping mechanism in response to progressive neural depletion. An alternative reading of the pattern of findings of this study would be to interpret them as a reflection of alterations in the equilibrium of activity between anticorrelated networks, in this instance the DMN and FPN, expression of their inherent functions in internal and external mediation, respectively (Fox et al., 2005). This pattern of alteration among these networks’ dynamic interactions was observed in association with all aspects of anosognosia in this study. Changes in inter-network activity expressed as increased connectivity between the DMN and the salience network, also found in this study in association with anosognosia, have been previously reported in mild probable AD dementia (Sarli et al., 2021). It might be suggested, therefore, that changes in activity in anticorrelated networks would alter the level of mutual inhibition exerted among their regions leading to a progressive loss of network integration and, in turn, to detectable cognitive and functional impairments, including alterations of awareness.
Limitations
One of the main limitations of this study, and in general of the field of anosognosia research, is the choice of instrument to measure this symptom. Although, no method to detect anosognosia is recognized as the “gold-standard”, the analysis of discrepancy scores is currently the most accepted methodological approach (de Ruijter et al., 2020). Discrepancy scoring relies on the response given by both patient and informant. Caregiver burden may inadvertently shift the perception of the patient’s abilities into an over/under-estimation. To rule out this possibility, we chose to rely on a robust instrument that has undergone methodological validation. We acknowledge, however, that there are other ways to assess anosognosia, such as the discrepancy between subjective estimate of skills and actual objective performance on a task. Lastly, we cannot completely rule out the possibility that one of the two sub-scales may have had a larger impact on the total score than the other. The lack of large-scale findings within the salience network and pDMN connectivity might be due to insufficient sample size affecting statistical power to delineate the functional neural correlates of multi-domain anosognosia. This was a major reason why we chose to rely on the supportive evidence provided by seed-based models.
Conclusion
Large-scale brain functional networks and seed-based findings showed negative fronto-temporal associations, while positive associations of parieto-temporal connectivity related with level of awareness of memory dysfunction in early AD. Conversely, awareness of symptoms within the non-memory (executive and daily-life) domain was positively linked to connectivity between the aDMN and cerebellum, between left hippocampus and left insula and between left medioprefrontal and right dorsolateral prefrontal cortex. Lastly, the total score reflective of the combined level of awareness of dysfunction in multiple cognitive and everyday-life domains showed a positive correlation with fronto-temporal pathways and with connectivity between the aDMN and the right anterior cingulate. The prefrontal cortex seems to be a critical mediator of single and multi-domain anosognosia in the early stages of AD.
Contextualizing our findings based on the organization into nodes of the central large-scale brain functional networks, an overall pattern emerged of reduced interplay between the central executive FPN and the DMN influencing awareness for memory dysfunction as well as more globally when the memory and non-memory domain scores were summed together. On the other hand, increased intercommunication of the nodes within an executive system with both the DMN and salience network was found for non-memory anosognosia (Figure 7). This could translate into a reorganization of network dynamics in an attempt of the functional system to support its awareness abilities hampered by DMN dysfunction mechanisms. The dysfunction of a central executive comparator that influences levels of awareness could promote adaptive neural changes of increased network traffic to broader brain regions such as subcortical and supporting temporo-occipital territories (fusiform-lingual gyri) to sustain awareness in the early stages of AD degeneration. This neural coping attempts, however, do not appear to exert any behavioral benefit. These early changes in network dynamics provide insights on the evolution of deficits in awareness in AD where subtle alterations can be detected even at a preclinical stage (Cacciamani et al., 2017). Multi-domain assessment of awareness, therefore, could potentially provide early signs of worse disease outcome. In the presence of anosognosia, clinicians should suspect early brain functional network breakdowns that could potentially modulate negatively the phenotypical presentation of the disease and act as a potential trigger of comorbid neuropsychiatric manifestations. Awareness of the potential implications of this symptom should lead to better cognitive and therapeutic interventions.
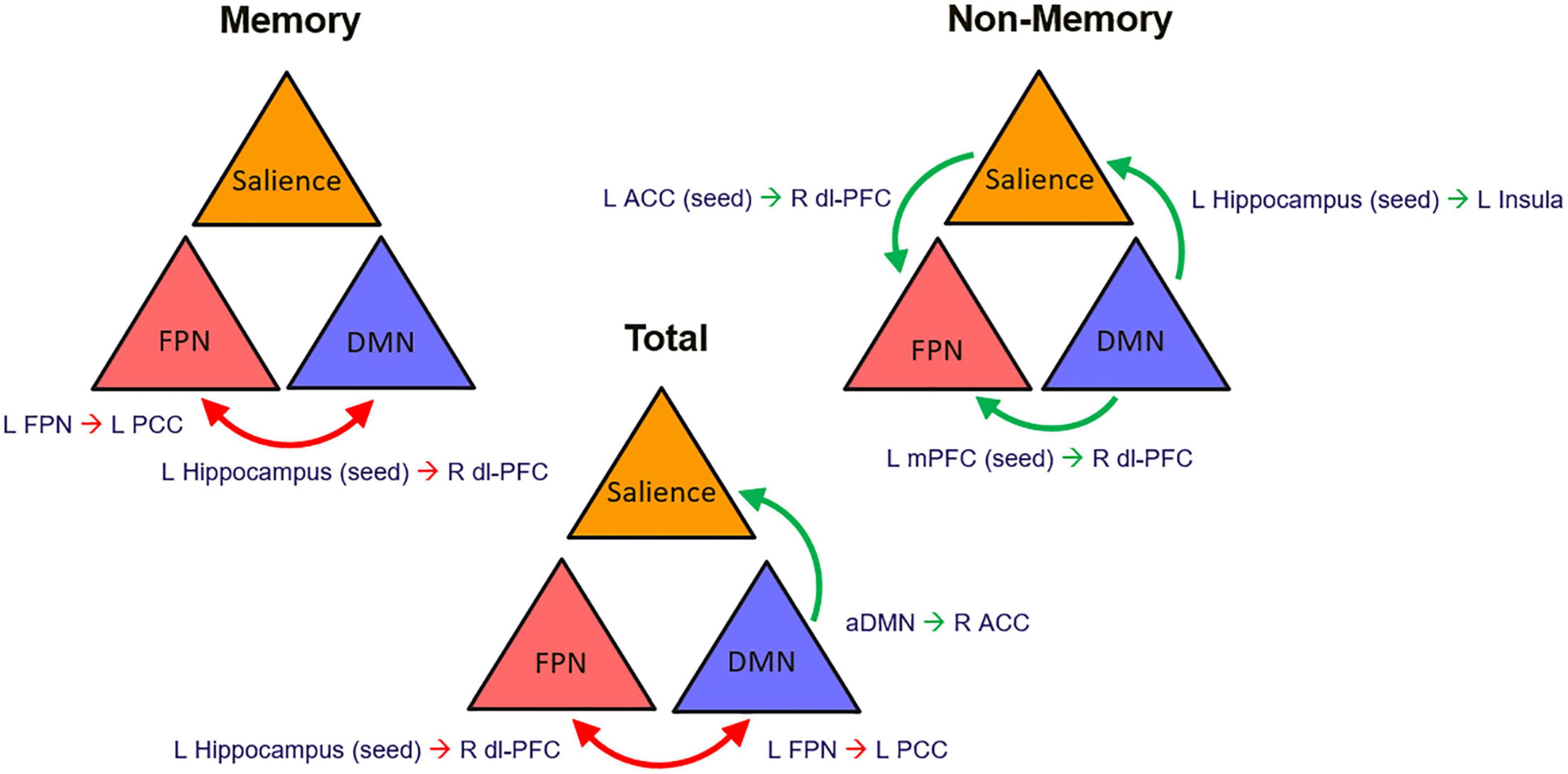
Figure 7. Graphical representation of the proposed network dynamic changes that define multi-domain anosognosia in the early stages of AD. ACC, anterior cingulate cortex; aDMN, anterior Default Mode Network; dl-PFC, dorsolateral prefrontal cortex; FPN, Fronto-Parietal Network; mPFC, medial prefrontal cortex; red arrows, decreased connectivity; green arrows, increased connectivity.
Data Availability Statement
The raw data supporting the conclusions of this article will be made available by the authors, without undue reservation.
Ethics Statement
The studies involving human participants were reviewed and approved by the Regional Ethics Committee of Yorkshire and Humber (Ref No: 12/YH/0474). The patients/participants provided their written informed consent to participate in this study.
Author Contributions
JMV-B contributed to data processing and data modeling, result interpretation, and writing of the initial draft of the manuscript. MDM contributed to data collection, result interpretation, and critical revision of the manuscript. AV contributed to study conception and funding, clinical assessment and diagnosis, and revising and finalizing of the manuscript. All authors contributed to the article and approved the submitted version.
Funding
The MRI costs for the scans included in this study were covered by funding from the European Union Seventh Framework Programme (FP7/2007–2013) under grant agreement no. 601055, VPH-DARE@IT to AV. JMV-B was funded by a scholarship by the Consejo Nacional de Ciencia y Tecnología (CONACYT), Mexico.
Conflict of Interest
The authors declare that the research was conducted in the absence of any commercial or financial relationships that could be construed as a potential conflict of interest.
Publisher’s Note
All claims expressed in this article are solely those of the authors and do not necessarily represent those of their affiliated organizations, or those of the publisher, the editors and the reviewers. Any product that may be evaluated in this article, or claim that may be made by its manufacturer, is not guaranteed or endorsed by the publisher.
Footnotes
- ^ https://cordis.europa.eu/project/id/601055
- ^ http://www0.cs.ucl.ac.uk/staff/g.ridgway/vbm/get_totals.m
- ^ mialab.mrn.org/software/gift
- ^ http://marsbar.sourceforge.net/
References
Agnew, S. K., and Morris, R. G. (1998). The heterogeneity of anosognosia for memory impairment in Alzheimer’s disease: a review of the literature and a proposed model. Aging Ment. Health 2, 7–19. doi: 10.1080/13607869856876
Albert, M. S., DeKosky, S. T., Dickson, D., Dubois, B., Feldman, H. H., Fox, N. C., et al. (2011). The diagnosis of mild cognitive impairment due to Alzheimer’s disease: recommendations from the National Institute on Aging-Alzheimer’s Association workgroups on diagnostic guidelines for Alzheimer’s disease. Alzheimers Dement. 7, 270–279. doi: 10.1016/j.jalz.2011.03.008
Alves, P. N., Foulon, C., Karolis, V., Bzdok, D., Margulies, D. S., Volle, E., et al. (2019). An improved neuroanatomical model of the default-mode network reconciles previous neuroimaging and neuropathological findings. Commun. Biol. 2:370. doi: 10.1038/s42003-019-0611-3
Amanzio, M., Torta, D. M. E., Sacco, K., Cauda, F., D’Agata, F., Duca, S., et al. (2011). Unawareness of deficits in Alzheimer’s disease: role of the cingulate cortex. Brain 134, 1061–1076. doi: 10.1093/brain/awr020
Antoine, N., Bahri, M. A., Bastin, C., Collette, F., Phillips, C., Balteau, E., et al. (2019). Anosognosia and default mode subnetwork dysfunction in Alzheimer’s disease. Hum. Brain Mapp. 40, 5330–5340. doi: 10.1002/hbm.24775
Badhwar, A., Tam, A., Dansereau, C., Orban, P., Hoffstaedter, F., and Bellec, P. (2017). Resting-state network dysfunction in Alzheimer’s disease: a systematic review and meta-analysis. Alzheimers Dement. 8, 73–85. doi: 10.1016/j.dadm.2017.03.007
Berlingeri, M., Ravasio, A., Cranna, S., Basilico, S., Sberna, M., Bottini, G., et al. (2015). Unrealistic representations of “the self”: a cognitive neuroscience assessment of anosognosia for memory deficit. Conscious. Cogn. 37, 160–177. doi: 10.1016/j.concog.2015.08.010
Boublay, N., Schott, A. M., and Krolak-Salmon, P. (2016). Neuroimaging correlates of neuropsychiatric symptoms in Alzheimer’s disease: a review of 20 years of research. Eur. J. Neurol. 23, 1500–1509. doi: 10.1111/ene.13076
Bressler, S. L., and Menon, V. (2010). Large-scale brain networks in cognition: emerging methods and principles. Trends Cogn. Sci. 14, 277–290. doi: 10.1016/j.tics.2010.04.004
Brett, M., Anton, J. L., Valabrgue, R., and Poline, J.-B. (2002). Region of interest analysis using an SPM toolbox. Presented at the 8th International Conference on Functional Mapping of the Human Brain, June 2-6, 2002, Sendai, Japan. Neuroimage 13, 210–217.
Buckner, R. L., Krienen, F. M., Castellanos, A., Diaz, J. C., and Yeo, B. T. T. (2011). The organization of the human cerebellum estimated by intrinsic functional connectivity. J. Neurophysiol. 106, 2322–2345. doi: 10.1152/jn.00339.2011
Cacciamani, F., Tandetnik, C., Gagliardi, G., Bertin, H., Habert, M. O., Hampel, H., et al. (2017). Low Cognitive Awareness, but Not Complaint, is a Good Marker of Preclinical Alzheimer’s Disease. J. Alzheimers Dis. 59, 753–762. doi: 10.3233/jad-170399
Chavoix, C., and Insausti, R. (2017). Self-awareness and the medial temporal lobe in neurodegenerative diseases. Neurosci. Biobehav. Rev. 78, 1–12. doi: 10.1016/j.neubiorev.2017.04.015
Cohen, J. D., Botvinick, M., and Carter, C. S. (2000). Anterior cingulate and prefrontal cortex: who’s in control? Nat. Neurosci. 3, 421–423. doi: 10.1038/74783
Craig, A. D. (2009). How do you feel — now? The anterior insula and human awareness. Nat. Rev. Neurosci. 10, 59–70. doi: 10.1038/nrn2555
Davey, C. G., Pujol, J., and Harrison, B. J. (2016). Mapping the self in the brain’s default mode network. Neuroimage 132, 390–397. doi: 10.1016/j.neuroimage.2016.02.022
de Ruijter, N. S., Schoonbrood, A. M. G., van Twillert, B., and Hoff, E. I. (2020). Anosognosia in dementia: a review of current assessment instruments. Alzheimers Dement. 12:e12079. doi: 10.1002/dad2.12079
Derouesne, C., Thibault, S., Lagha-Pierucci, S., Baudouin-Madec, V., Ancri, D., and Lacomblez, L. (1999). Decreased awareness of cognitive deficits in patients with mild dementia of the Alzheimer type. Int. J. Geriatr. Psychiatry 14, 1019–1030. doi: 10.1002/(sici)1099-1166(199912)14:12<1019::Aid-gps61>3.0.Co;2-f
Devinsky, O., Morrell, M. J., and Vogt, B. A. (1995). Contributions of anterior cingulate cortex to behaviour. Brain 118, 279–306. doi: 10.1093/brain/118.1.279
Ewers, M., Sperling, R. A., Klunk, W. E., Weiner, M. W., and Hampel, H. (2011). Neuroimaging markers for the prediction and early diagnosis of Alzheimer’s disease dementia. Trends Neurosci. 34, 430–442. doi: 10.1016/j.tins.2011.05.005
Fox, M. D., Snyder, A. Z., Vincent, J. L., Corbetta, M., Van Essen, D. C., and Raichle, M. E. (2005). The human brain is intrinsically organized into dynamic, anticorrelated functional networks. Proc. Natl. Acad. Sci. U. S. A. 102, 9673–9678. doi: 10.1073/pnas.0504136102
Funahashi, S. (2017). Working Memory in the Prefrontal Cortex. Brain Sci. 7:49. doi: 10.3390/brainsci7050049
Gardini, S., Venneri, A., Sambataro, F., Cuetos, F., Fasano, F., Marchi, M., et al. (2015). Increased functional connectivity in the default mode network in mild cognitive impairment: a maladaptive compensatory mechanism associated with poor semantic memory performance. J. Alzheimers Dis. 45, 457–470. doi: 10.3233/JAD-142547
Gbadeyan, O., McMahon, K., Steinhauser, M., and Meinzer, M. (2016). Stimulation of Dorsolateral Prefrontal Cortex Enhances Adaptive Cognitive Control: a High-Definition Transcranial Direct Current Stimulation Study. J. Neurosci. 36, 12530–12536. doi: 10.1523/jneurosci.2450-16.2016
Greicius, M. D., Srivastava, G., Reiss, A. L., and Menon, V. (2004). Default-mode network activity distinguishes Alzheimer’s disease from healthy aging: evidence from functional MRI. Proc. Natl. Acad. Sci. U. S. A. 101, 4637–4642. doi: 10.1073/pnas.0308627101
Grieder, M., Wang, D. J. J., Dierks, T., Wahlund, L. O., and Jann, K. (2018). Default Mode Network Complexity and Cognitive Decline in Mild Alzheimer’s Disease. Front. Neurosci. 12:770. doi: 10.3389/fnins.2018.00770
Guerrier, L., Le Men, J., Gane, A., Planton, M., Salabert, A. S., Payoux, P., et al. (2018). Involvement of the Cingulate Cortex in Anosognosia: a Multimodal Neuroimaging Study in Alzheimer’s Disease Patients. J. Alzheimers Dis. 65, 443–453. doi: 10.3233/jad-180324
Hanseeuw, B. J., Scott, M. R., Sikkes, S. A. M., Properzi, M., Gatchel, J. R., Salmon, E., et al. (2020). Evolution of anosognosia in alzheimer’s disease and its relationship to amyloid. Ann. Neurol. 87, 267–280. doi: 10.1002/ana.25649
Hanyu, H., Sato, T., Akai, T., Shimizu, S., Hirao, K., Kanetaka, H., et al. (2008). Neuroanatomical correlates of unawareness of memory deficits in early Alzheimer’s disease. Dement. Geriatric Cogn. Disord. 25, 347–353. doi: 10.1159/000119594
Harwood, D. G., Sultzer, D. L., Feil, D., Monserratt, L., Freedman, E., and Mandelkern, M. A. (2005). Frontal Lobe Hypometabolism and Impaired Insight in Alzheimer Disease. Am. J. Geriatric Psychiatry 13, 934–941. doi: 10.1097/00019442-200511000-00003
Jedidi, H., Feyers, D., Collette, F., Bahri, M. A., Jaspar, M., d’Argembeau, A., et al. (2014). Dorsomedial prefrontal metabolism and unawareness of current characteristics of personality traits in Alzheimer’s disease. Soc. Cogn. Affect. Neurosci. 9, 1458–1463. doi: 10.1093/scan/nst132
Cardoso, M. J., Leung, K., Modat, M., Keihaninejad, S., Cash, D., Barnes, J., et al. (2013). STEPS: similarity and Truth Estimation for Propagated Segmentations and its application to hippocampal segmentation and brain parcelation. Med. Image Anal. 17, 671–684. doi: 10.1016/j.media.2013.02.006
Kumar, S., Zomorrodi, R., Ghazala, Z., Goodman, M. S., Blumberger, D. M., Cheam, A., et al. (2017). Extent of Dorsolateral Prefrontal Cortex Plasticity and Its Association With Working Memory in Patients With Alzheimer Disease. JAMA Psychiatry 74, 1266–1274. doi: 10.1001/jamapsychiatry.2017.3292
Lacerda, I. B., Santos, R. L., Belfort, T., Neto, J. P. S., and Dourado, M. C. N. (2021). Domains of awareness in Alzheimer’s disease: the influence of executive function. Int. J. Geriatric Psychiatry 36, 926–934. doi: 10.1002/gps.5495
Lancaster, J. L., Woldorff, M. G., Parsons, L. M., Liotti, M., Freitas, C. S., Rainey, L., et al. (2000). Automated Talairach atlas labels for functional brain mapping. Hum. Brain Mapp. 10, 120–131.
Leicht, H., Berwig, M., and Gertz, H. J. (2010). Anosognosia in Alzheimer’s disease: the role of impairment levels in assessment of insight across domains. J. Int. Neuropsychol. Soc. 16, 463–473. doi: 10.1017/s1355617710000056
Lou, H. C., Changeux, J. P., and Rosenstand, A. (2017). Towards a cognitive neuroscience of self-awareness. Neurosci. Biobehav. Rev. 83, 765–773. doi: 10.1016/j.neubiorev.2016.04.004
MacPherson, S. E., Phillips, L. H., and Della Sala, S. (2002). Age, executive function, and social decision making: a dorsolateral prefrontal theory of cognitive aging. Psychol. Aging 17, 598–609.
Maldjian, J. A., Laurienti, P. J., Kraft, R. A., and Burdette, J. H. (2003). An automated method for neuroanatomic and cytoarchitectonic atlas-based interrogation of fMRI data sets. Neuroimage 19, 1233–1239.
Marshall, G. A., Kaufer, D. I., Lopez, O. L., Rao, G. R., Hamilton, R. L., and DeKosky, S. T. (2004). Right prosubiculum amyloid plaque density correlates with anosognosia in Alzheimer’s disease. J. Neurol. Neurosurg. Psychiatry 75, 1396–1400. doi: 10.1136/jnnp.2003.030007
McKhann, G. M., Knopman, D. S., Chertkow, H., Hyman, B. T., Jack, C. R., Kawas, C. H., et al. (2011). The diagnosis of dementia due to Alzheimer’s disease: recommendations from the National Institute on Aging-Alzheimer’s Association workgroups on diagnostic guidelines for Alzheimer’s disease. Alzheimers Dement. 7, 263–269. doi: 10.1016/j.jalz.2011.03.005
Migliorelli, R. T. A., Sabe, L., Petracca, G., Petracchi, M., Leiguarda, R., and Starkstein, S. E. (1995). Anosognosia in Alzheimer’s disease: a study of associated factors. J. Neuropsychiatry Clin. Neurosci. 7, 338–344. doi: 10.1176/jnp.7.3.338
Mitelpunkt, A., Galili, T., Kozlovski, T., Bregman, N., Shachar, N., Markus-Kalish, M., et al. (2020). Novel Alzheimer’s disease subtypes identified using a data and knowledge driven strategy. Sci. Rep. 10, 1327–1327. doi: 10.1038/s41598-020-57785-2
Modinos, G., Ormel, J., and Aleman, A. (2009). Activation of anterior insula during self-reflection. PLoS One 4:e4618. doi: 10.1371/journal.pone.0004618
Mondragon, J. D., Maurits, N. M., and De Deyn, P. P. (2019). Functional Neural Correlates of Anosognosia in Mild Cognitive Impairment and Alzheimer’s Disease: a Systematic Review. Neuropsychol. Rev. 29, 139–165. doi: 10.1007/s11065-019-09410-x
Mondragón, J. D., Maurits, N. M., and De Deyn, P. P. (2021). Functional connectivity differences in Alzheimer’s disease and amnestic mild cognitive impairment associated with AT(N) classification and anosognosia. Neurobiol. Aging 101, 22–39. doi: 10.1016/j.neurobiolaging.2020.12.021
Northoff, G., Heinzel, A., de Greck, M., Bermpohl, F., Dobrowolny, H., and Panksepp, J. (2006). Self-referential processing in our brain–a meta-analysis of imaging studies on the self. Neuroimage 31, 440–457. doi: 10.1016/j.neuroimage.2005.12.002
Nowrangi, M. A., Lyketsos, C., Rao, V., and Munro, C. A. (2014). Systematic review of neuroimaging correlates of executive functioning: converging evidence from different clinical populations. J. Neuropsychiatry Clin. Neurosci. 26, 114–125. doi: 10.1176/appi.neuropsych.12070176
Perrotin, A., Desgranges, B., Landeau, B., Mezenge, F., La Joie, R., Egret, S., et al. (2015). Anosognosia in Alzheimer disease: disconnection between memory and self-related brain networks. Ann. Neurol. 78, 477–486. doi: 10.1002/ana.24462
Postema, M. C., De Marco, M., Colato, E., and Venneri, A. (2019). A study of within-subject reliability of the brain’s default-mode network. Magnet. Reson. Mater. Phys. Biol. Med. 32, 391–405. doi: 10.1007/s10334-018-00732-0
Raichle, M. E. (2015). The Brain’s Default Mode Network. Annu. Rev. Neurosci. 38, 433–447. doi: 10.1146/annurev-neuro-071013-014030
Reed, B. R., Jagust, W. J., and Coulter, L. (1993). Anosognosia in Alzheimer’s disease: relationships to depression, cognitive function, and cerebral perfusion. J. Clin. Exp. Neuropsychol. 15, 231–244. doi: 10.1080/01688639308402560
Ries, M. L., Jabbar, B. M., Schmitz, T. W., Trivedi, M. A., Gleason, C. E., Carlsson, C. M., et al. (2007). Anosognosia in mild cognitive impairment: relationship to activation of cortical midline structures involved in self-appraisal. J. Int. Neuropsychol. Soc. 13, 450–461. doi: 10.1017/s1355617707070488
Ries, M. L., McLaren, D. G., Bendlin, B. B., Guofanxu, Rowley, H. A., Birn, R., et al. (2012). Medial prefrontal functional connectivity–relation to memory self-appraisal accuracy in older adults with and without memory disorders. Neuropsychologia 50, 603–611. doi: 10.1016/j.neuropsychologia.2011.12.014
Ruby, P., Collette, F., D’Argembeau, A., Péters, F., Degueldre, C., Balteau, E., et al. (2009). Perspective taking to assess self-personality: what’s modified in Alzheimer’s disease? Neurobiol. Aging 30, 1637–1651. doi: 10.1016/j.neurobiolaging.2007.12.014
Sánchez-Benavides, G., Grau-Rivera, O., Suárez-Calvet, M., Minguillon, C., Cacciaglia, R., Gramunt, N., et al. (2018). Brain and cognitive correlates of subjective cognitive decline-plus features in a population-based cohort. Alzheimers Res. Ther. 10, 123–123. doi: 10.1186/s13195-018-0449-9
Sarli, G., De Marco, M., Hallikainen, M., Soininen, H., Bruno, G., and Venneri, A. (2021). Regional Strength of Large-Scale Functional Brain Networks is Associated with Regional Volumes in Older Adults and in Alzheimer’s Disease. Brain Connect. 11, 201–212. doi: 10.1089/brain.2020.0899
Shany-Ur, T., Lin, N., Rosen, H. J., Sollberger, M., Miller, B. L., and Rankin, K. P. (2014). Self-awareness in neurodegenerative disease relies on neural structures mediating reward-driven attention. Brain 137, 2368–2381. doi: 10.1093/brain/awu161
Sorg, C., Riedl, V., Mühlau, M., Calhoun, V. D., Eichele, T., Läer, L., et al. (2007). Selective changes of resting-state networks in individuals at risk for Alzheimer’s disease. Proc. Natl. Acad. Sci. U. S. A 104, 18760–18765. doi: 10.1073/pnas.0708803104
Starkstein, S. E. (2014). Anosognosia in Alzheimer’s disease: diagnosis, frequency, mechanism and clinical correlates. Cortex 61, 64–73. doi: 10.1016/j.cortex.2014.07.019
Starkstein, S. E., Vázquez, S., Migliorelli, R., Tesón, A., Sabe, L., and Leiguarda, R. (1995). A single-photon emission computed tomographic study of anosognosia in alzheimerand#39;s disease. Arch. Neurol. 52, 415–420. doi: 10.1001/archneur.1995.00540280105024
Stewart, G., McGeown, W. J., Shanks, M. F., and Venneri, A. (2010). Anosognosia for memory impairment in Alzheimer’s disease. Acta Neuropsychiatr. 22, 180–187. doi: 10.1111/j.1601-5215.2010.00463.x
Tagai, K., Nagata, T., Shinagawa, S., and Shigeta, M. (2020). Anosognosia in patients with Alzheimer’s disease: current perspectives. Psychogeriatrics 20, 345–352. doi: 10.1111/psyg.12507
Therriault, J., Ng, K. P., Pascoal, T. A., Mathotaarachchi, S., Kang, M. S., Struyfs, H., et al. (2018). Anosognosia predicts default mode network hypometabolism and clinical progression to dementia. Neurology 90, e932–e939. doi: 10.1212/wnl.0000000000005120
Turriziani, P., Smirni, D., Mangano, G. R., Zappalà, G., Giustiniani, A., Cipolotti, L., et al. (2019). Low-Frequency Repetitive Transcranial Magnetic Stimulation of the Right Dorsolateral Prefrontal Cortex Enhances Recognition Memory in Alzheimer’s Disease. J. Alzheimers Dis. 72, 613–622. doi: 10.3233/jad-190888
Tzourio-Mazoyer, N., Landeau, B., Papathanassiou, D., Crivello, F., Etard, O., Delcroix, N., et al. (2002). Automated anatomical labeling of activations in SPM using a macroscopic anatomical parcellation of the MNI MRI single-subject brain. Neuroimage 15, 273–289. doi: 10.1006/nimg.2001.0978
Valera-Bermejo, J. M., De Marco, M., Mitolo, M., Cerami, C., Dodich, A., and Venneri, A. (2021). Large-Scale Functional Networks, Cognition and Brain Structures Supporting Social Cognition and Theory of Mind Performance in Prodromal to Mild Alzheimer’s Disease. Front. Aging Neurosci. 13:766703. doi: 10.3389/fnagi.2021.766703
Valera-Bermejo, J. M., De Marco, M., Mitolo, M., McGeown, W. J., and Venneri, A. (2020). Neuroanatomical and cognitive correlates of domain-specific anosognosia in early Alzheimer’s disease. Cortex 129, 236–246. doi: 10.1016/j.cortex.2020.04.026
Vannini, P., Hanseeuw, B., Munro, C. E., Amariglio, R. E., Marshall, G. A., Rentz, D. M., et al. (2017). Anosognosia for memory deficits in mild cognitive impairment: insight into the neural mechanism using functional and molecular imaging. Neuroimage Clin. 15, 408–414. doi: 10.1016/j.nicl.2017.05.020
Vemuri, P., Jones, D. T., and Jack, C. R. Jr. (2012). Resting state functional MRI in Alzheimer’s Disease. Alzheimers Res. Ther. 4:2. doi: 10.1186/alzrt100
Voss, H. U., Zevin, J. D., and McCandliss, B. D. (2006). Functional MR Imaging at 3.0 T versus 1.5 T: a Practical Review. Neuroimag. Clin. North Am. 16, 285–297. doi: 10.1016/j.nic.2006.02.008
Wang, Y., and Li, T.-Q. (2015). Dimensionality of ICA in resting-state fMRI investigated by feature optimized classification of independent components with SVM. Front. Hum. Neurosci. 9:259–259. doi: 10.3389/fnhum.2015.00259
Whitwell, J. L. (2009). Voxel-Based Morphometry: an Automated Technique for Assessing Structural Changes in the Brain. J. Neurosci. 29:9661.
Xu, W., Chen, S., Xue, C., Hu, G., Ma, W., Qi, W., et al. (2020). Functional MRI-Specific Alterations in Executive Control Network in Mild Cognitive Impairment: an ALE Meta-Analysis. Front. Aging Neurosci. 12:578863. doi: 10.3389/fnagi.2020.578863
Yang, Y.-L., Deng, H.-X., Xing, G.-Y., Xia, X.-L., and Li, H.-F. (2015). Brain functional network connectivity based on a visual task: visual information processing-related brain regions are significantly activated in the task state. Neural Regener. Res. 10, 298–307. doi: 10.4103/1673-5374.152386
Yoon, H. J., Kim, S. G., Kim, S. H., Choo, I. L. H., Park, S. H., and Seo, E. H. (2019). Distinct Neural Correlates of Executive Function by Amyloid Positivity and Associations with Clinical Progression in Mild Cognitive Impairment. Yonsei Med. J. 60, 935–943. doi: 10.3349/ymj.2019.60.10.935
Zamboni, G., Drazich, E., McCulloch, E., Filippini, N., Mackay, C. E., Jenkinson, M., et al. (2013). Neuroanatomy of impaired self-awareness in Alzheimer’s disease and mild cognitive impairment. Cortex 49, 668–678. doi: 10.1016/j.cortex.2012.04.011
Zamboni, G., and Wilcock, G. (2011). Lack of awareness of symptoms in people with dementia: the structural and functional basis. Int. J. Geriatric Psychiatry 26, 783–792. doi: 10.1002/gps.2620
Keywords: anosognosia, large-scale networks, mild cognitive impairment, functional MRI, unawareness, resting-state
Citation: Valera-Bermejo JM, De Marco M and Venneri A (2022) Altered Interplay Among Large-Scale Brain Functional Networks Modulates Multi-Domain Anosognosia in Early Alzheimer’s Disease. Front. Aging Neurosci. 13:781465. doi: 10.3389/fnagi.2021.781465
Received: 22 September 2021; Accepted: 29 December 2021;
Published: 03 February 2022.
Edited by:
Howard Rosen, University of California, San Francisco, United StatesReviewed by:
Oriol Turró-Garriga, Institute of Biomedical Research of Girona, SpainChristine Bastin, University of Liège, Belgium
Copyright © 2022 Valera-Bermejo, De Marco and Venneri. This is an open-access article distributed under the terms of the Creative Commons Attribution License (CC BY). The use, distribution or reproduction in other forums is permitted, provided the original author(s) and the copyright owner(s) are credited and that the original publication in this journal is cited, in accordance with accepted academic practice. No use, distribution or reproduction is permitted which does not comply with these terms.
*Correspondence: Annalena Venneri, annalena.venneri@brunel.ac.uk