- 1Department of Medical Genetics, School of Medicine, Shahid Beheshti University of Medical Sciences, Tehran, Iran
- 2Department of Cell and Molecular Biology, Faculty of Life Sciences and Technology, Shahid Beheshti University, Tehran, Iran
- 3Department of Pharmacognosy, College of Pharmacy, Hawler Medical University, Erbil, Iraq
- 4Institute of Human Genetics, Jena University Hospital, Jena, Germany
- 5Skull Base Research Center, Loghman Hakim Hospital, Shahid Beheshti University Hospital, Tehra, Iran
Ischemic stroke (IS) is an acute cerebral vascular event with high mortality and morbidity. Though the precise pathophysiologic routes leading to this condition are not entirely clarified, growing evidence from animal and human experiments has exhibited the impact of non-coding RNAs in the pathogenesis of IS. Various lncRNAs namely MALAT1, linc-SLC22A2, linc-OBP2B-1, linc_luo_1172, linc-DHFRL1-4, SNHG15, linc-FAM98A-3, H19, MEG3, ANRIL, MIAT, and GAS5 are possibly involved in the pathogenesis of IS. Meanwhile, lots of miRNAs contribute in this process. Differential expression of lncRNAs and miRNAs in the sera of IS patients versus unaffected individuals has endowed these transcripts the aptitude to distinguish at risk patients. Despite conduction of comprehensive assays for evaluation of the influence of lncRNAs/miRNAs in the pathogenesis of IS, therapeutic impacts of these transcripts in IS have not been clarified. In the present paper, we review the impact of lncRNAs/miRNAs in the pathobiology of IS through assessment of evidence provided by human and animal studies.
Introduction
Ischemic stroke (IS) is an acute cerebrovascular event with high mortality and morbidity. This disorder is the third most frequent cause of mortality in Western regions of the world (Feigin et al., 2015). Current treatments for IS include thrombolysis, mechanical thromboectomy and neuroprotective therapies (Liaw and Liebeskind, 2020). Although the exact pathophysiologic routes leading to this condition are not entirely clarified, growing evidence from animal and human experiments has exhibited the impact of non-coding transcripts in the pathogenesis of IS (Zhu et al., 2019). These transcripts are highly variable in the terms of size, function, genomic location and conservation, yet in a broad classification they can be categorized based on their size to small versus long non-coding RNAs (lncRNAs). Small non-coding RNAs have some subclasses among them are microRNAs (miRNAs). Both lncRNAs and miRNAs have regulatory impacts on gene expression but via different routes. Being firstly discovered in 1993 in C. elegans (Lee et al., 1993), miRNAs comprise an ever-growing type of non-coding RNAs that target specific sequences in the 3′ untranslated regions of genes, then decreasing their expression via mRNA degradation or translation blocking (O’Brien et al., 2018). These transcripts are about 22 nucleotides in length. They can hypothetically target almost any gene in the human genome. However, the extent of miRNA response elements complementarity defines their route of action, i.e., AGO2-dependent cleavage of target transcript or RISC-associated translational suppression (Jo et al., 2015). Besides, a number of miRNAs might influence gene expression at transcriptional and post-transcriptional stages within the nucleus (O’Brien et al., 2018). However, this mode of action has not been fully discovered. The dynamic nature of miRNA-associated gene regulation potentiates them as tools for regulation of gene expression in a cell type/situation-specific mode since several events such as alternative splicing events, polyadenylation state and the presence of cell type-specific RNA binding proteins affect miRNA response elements (O’Brien et al., 2018). LncRNAs are another group of transcripts with fundamental roles in the regulation of gene transcription via several modes including acting as signal, decoy molecules, scaffolds, guide and enhancer transcripts. The chief mode action of lncRNAs is their role in the regulation of transcription in reaction to numerous stimuli through acting as molecular signals (Fang and Fullwood, 2016). Although they generally do not have open reading frame, many of them have similar characteristics with protein-coding genes among them are the presence of 5′ cap, poly A tail and alternative splicing events (Cheng et al., 2005; Derrien et al., 2012). Through participating in chromatin configuration alteration, interaction with chromatin structures, acting as competing endogenous RNAs (ceRNAs) or natural antisense lncRNAs, lncRNAs contribute in the pathogenesis of human disorders (Fang and Fullwood, 2016). Figure 1 depicts the role of a number of non-coding RNAs in the pathobiology of IS through different signaling pathways particularly PI3K/AKT and NF-κβ.
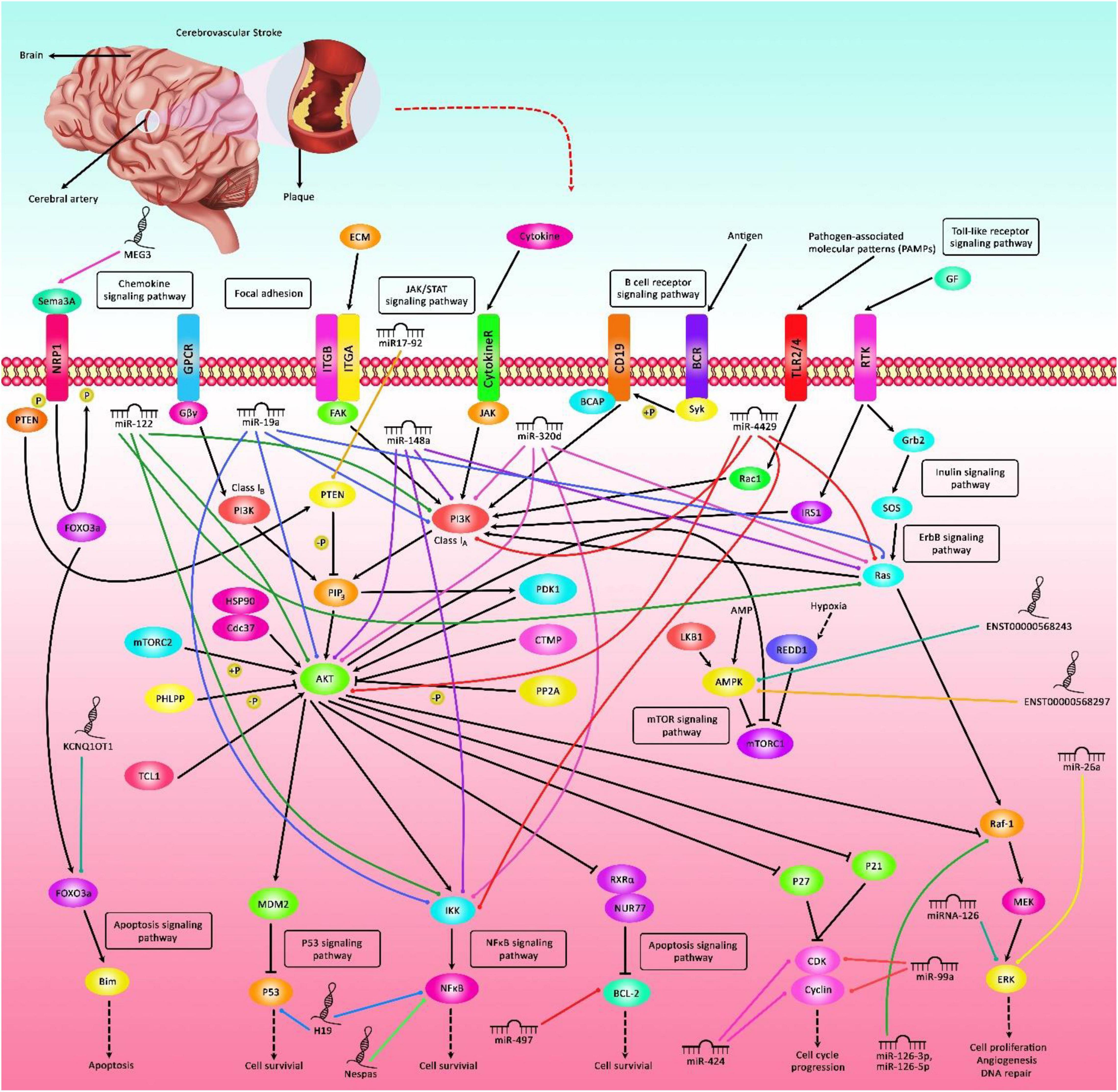
Figure 1. A schematic representation of the interaction between non-coding RNAs and various signaling cascades in ischemic stroke (IS). Differential expression of lncRNAs as well as miRNAs could have an important role in the pathogenesis of IS. Various miRNAs such as miR-19a, miR-122, miR-148a, miR-320d, and miR-4429 via targeting Akt, PI3K, Ras, and IKK could modulate expression of genes leukocytes, thus affecting the course of IS. In addition, miR-497 by regulating the expression levels of Bcl-2 and Bcl-w could induce ischemic neuronal death. Additionally, lncRNA H19 via directly targeting P53 could suppress neurogenesis following IS through p53/Notch1 axis. Furthermore, MEG3 could promote cell survival and reduce cell apoptosis via modulating the expression of Sema3A. Besides, KCNQ1OT1 through modulating the expression of FOXO3 could enhance brain injury and induce autophagy in IS.
Human Studies
Long Non-coding RNAs and Ischemic Stroke
Assessment of expression of lncRNAs has been the focus of numerous studies conducted in human subjects. For instance, a high throughput study has been performed on blood specimens of patients with IS and controls who have been matched with cases in terms of vascular risk factors. The study has revealed differential expression of approximately 300 lncRNAs between IS group and male controls, while 97 lncRNAs have been differentially expressed between IS group and female controls. Notably, some of differentially expressed lncRNAs have been shown to reside in genomic regions formerly recognized as IS risk loci namely lipoprotein, lipoprotein(a)-like 2, ABO blood group, prostaglandin 12 synthase, and α-adducins (Dykstra-Aiello et al., 2016). Another study has reported distinct lncRNAs signatures in peripheral blood mononuclear cells (PBMCs) among patients with IS, transient ischemic attack (TIA) and healthy subjects. Notably, expressions of linc-DHFRL1-4, SNHG15, and linc-FAM98A-3 have been substantially increased in IS patients versus healthy controls and TIA patients. Expression of linc-FAM98A-3 has been returned to normal level by day 7, whereas SNHG15 levels have been continued to be high during the follow-up period, demonstrating the capability of lncRNAs to observe IS dynamics (Deng et al., 2018). Another microarray-based assay has reported up-regulation of 560 and down-regulation of 690 lncRNAs in IS patients versus controls among them have been lncRNAs ENST00000568297, ENST00000568243, and NR_046084. Dysregulated lncRNAs have been predicted to partake in IS pathology by modulating central miRNAs, mRNAs, or IS-associated pathways (Guo et al., 2018). Assessment of lncRNA signature at two time points after IS has revealed differential expression of 3,009 and 2,034 lncRNAs 24 h and 7 days after IS, respectively. These results have shown the impact of IS on lncRNA signature at both the acute and subacute phases. Notably, expression of lncRNAs in the processing and presentation processes of antigens have been increased at 24 h and returned to basal amounts on day 7 following IS. Besides, expressions of inflammatory mediator regulation of TRP channels and GABAergic synapses have been decreased on day 7 following IS (Zhu et al., 2018). Levels of H19 in the circulation of patients with IS have been positively correlated with the National Institute of Health Stroke Scale Scores of the patients in in three time points following stroke attack. Mechanistically, H19 silencing could reduce expression of neurogenesis related proteins. In addition, H19 precludes the development of neurogenesis after IS via p53/Notch1 pathway (Wang et al., 2019a). Another experiment has reported association between H19 and Acute Stroke Treatment (TOAST) subclasses of atherosclerotic patients. Forced over-expression of H19 has enhanced ACP5 expression, increased cell proliferation and blocked cell apoptosis. Up-regulation of H19 has increased the plaque size in the animal model, thus H19 participates in the atherosclerotic processes and surges the risk of IS through increasing ACP5 levels (Huang et al., 2019). RMST is another up-regulated lncRNA in the plasma specimens of IS patients (Hou and Cheng, 2018). A previous study in Chinese Han population has shown over-expression of ANRIL in IS patients parallel with down-regulation of CDKN2A. The rs2383207 and rs1333049 SNPs have been associated with risk of IS in male subjects (Yang et al., 2018). Another study has reported higher levels of ANRIL in patients with the atrial fibrillation (AF) and ischemic stroke compared with AF patients without IS. Serum levels of ANRIL have been correlated with the NIHSS and the mRS scores (Zeng and Jin, 2020). Table 1 gives a summary of human studies reporting elevation of lncRNAs in IS.
Contrary to two mentioned studies in the previous section, Feng et al. have demonstrated decreased levels of ANRIL in plasma specimens of patients with acute IS patients versus controls (Feng et al., 2019). ZFAS1 is another down-regulated lncRNA in IS patients. Moreover, expression of ZFAS1 in patients with large-artery atherosclerosis (LAA) stroke has been lower compared with those with non-LAA stroke and controls. In addition, ZFAS1 expression has been lower in the small vessel occlusion group compared with cardioembolism (Wang et al., 2019b). FLJ23867, H3F3AP6, TNPO1P1 are also among lncRNAs which are down-regulated in IS PBMCs compared with control PBMCs (Zhu et al., 2019). An lncRNA profiling using RNA-seq method and subsequent KEGG pathway and gene ontology (GO) enrichment assays have shown down-regulation of RPS6KA2-AS1 and lnc-CALM1-7 in exosomes retrieved from sera of patients with acute minor IS (Xu et al., 2020). Table 2 gives a summary of human studies that displayed under-expression of lncRNAs in IS.
ANRIL low-expression has been determined as a marker of better recurrence-free survival in of AF patients with IS. Based on the outcomes of Cox regression model, serum levels of ANRIL, NIH Stroke Scale (NIHSS) score, infarct volume, and smoking have been the risk factors for AF with IS (Zeng and Jin, 2020). Another study has reported that down-regulation of ANRIL in acute IS can differentiate these patients from healthy subjects with area under curve (AUC) of 0.759. Besides, expression levels of this lncRNA has been negatively correlated with NIHSS score and high-sensitivity C-reactive protein (hs-CRP), TNF-α and IL-6 concentrations, while being positively correlated with IL-10 concentrations (Feng et al., 2019). Down-regulation of ZFAS1 could predict risk of LAA strokes. Based on the results of receiver operating characteristic curve, ZFAS1 has 89.39% sensitivity in distinguishing LAA stroke patients from controls (Wang et al., 2019b). Another biomarker discovery study in PBMCs of IS patients has demonstrated AUC values of 0.73, 0.74, and 0.69 for ENST00000568297, ENST00000568243 and NR_046084, respectively (Deng et al., 2018). Moreover, MIAT levels in IS patients have been remarkably increased in correlation with NIHSS scores, mRS, hs-CRP and infarct size. Based on the results of ROC (receiver operating characteristic) curves, MIAT has been suggested as a possible marker for distinguishing IS patients from the healthy subjects with AUC value of 0.842. Moreover, patients with over-expression of MIAT had a comparatively poor prognosis. Multivariate analysis has shown the potential of MIAT as an independent prognostic biomarker of functional outcome and mortality of IS (Zhu et al., 2018). Table 3 gives a brief review of investigations that reported diagnostic/prognostic role of lncRNAs in IS.
MicroRNAs and Stroke
Expression of miR-205-5p has been surged in the serum specimens of CIS patients and human brain microvascular endothelial cells under oxygen glucose deprivation/re-oxygenation. Besides, this condition has interfered with the tube formation of human brain microvascular endothelial cells. miR-205-5p knock-down has enhanced proliferation and angiogenic capacity of endothelial cells to resist oxygen glucose deprivation/re-oxygenation injury (Gao et al., 2020). The relationship between upper limb recovery after IS and miRNA signature has been assessed by another group. Authors have discovered lower levels of miR-371-3p, miR-524, miR-520g, miR-1255A, miR-453, and miR-583, while upper levels of miR-941, miR-449b, and miR-581 in good recover group compared with poor recovery group. These miRNAs have been shown to congregate on pathways related with axon guidance, developmental processes and carcinogenesis (Edwardson et al., 2018b). Expression of let-7e-5p has also been shown to be elevated in IS patients compared with control subjects. Over-expression of let-7e-5p has been associated with elevated probability of IS. This miRNA has been suggested to influence expression of four genes enriched in the MAPK pathway including CASP3 and NLK (Huang et al., 2016). miRNA levels might also distinguish IS patients from those with hemorrhagic stroke (HS). Leung et al. have demonstrated higher median plasma levels of miR-124-3p in acute phase of HS patients compared with similar phase of IS, while miR-16 had the opposite trend. Both miRNAs have been suggested as diagnostic markers for discrimination of HS from IS (Leung et al., 2014). A high throughput miRNA profiling in IS has reported differential expression of 115 miRNAs between IS cases and healthy controls. These transcripts have been linked with axon guidance, glioma, MAPK, mTOR and Erb-B signaling pathways. miR-32-3p, miR-106-5p, and miR-532-5p have been the first ranked ones (Li et al., 2015). Table 4 provides the summary of researches which reported elevation of miRNAs in IS.
Blood amounts of miR-30a and miR-126 have been substantially decreased in all assessed patients with IS until 24 weeks. Circulating let-7b has been decreased in patients with LAA compared with healthy subjects, while circulating let-7b have been higher in patients with other kinds of IS until 24 weeks. Notably, aberrant miRNAs levels have been resolved 48 weeks after IS onset in all patients. Authors have suggested that miR-30a might affect IS through modulation of RhoB and beclin-1. Moreover, miR-126 and let-7 can contribute in this process through modulation of VCAM-1 and inflammatory responses, respectively (Long et al., 2013). Another investigation has demonstrated that miRNA signature reveal not only the chronological development of IS but also the specific reasons for development of IS. Authors have suggested a 32-miRNA panel that can distinguish stroke etiologies during the acute phase. Moreover, miR-125b-2∗, miR-27a∗, miR-422a, miR-488, and miR-627 have been constantly dysregulated in acute stroke independent of age or severity or underlying metabolic background (Sepramaniam et al., 2014). Table 5 provides outcome of human studies reporting down-regulation of miRNAs in IS.
Diagnostic and prognostic role of miRNAs have been appraised in IS. Elevated serum amounts of miR-15a, miR-16, and miR-17-5p in acute IS patients could be used as diagnostic markers. Based on the multivariate logistic regression analysis, serum miR-17-5p levels could discriminate the presence of acute IS. miR-15a, miR-16, and miR-17-5p had AUC values of 0.698, 0.82, and 0.784, respectively. Combination of three miRNAs increased the AUC value to 0.845 (Wu et al., 2015). ROC curve analysis has revealed AUC values of 0.91, 0.91, 0.92, and 0.93 for plasma miR-30a levels, at 24 h, 1, 4, and 24 weeks, respectively. These values have been 0.93, 0.92, 0.92, and 0.91 for miR-126 at these time points, respectively. Taken together, miR-30a, miR-126 and let-7b can be suitable biomarkers for IS (Long et al., 2013). Expression levels of miR-145 and miR-210 have been remarkably elevated in IS patients with robust AUC values of 0.90 and 1.0, respectively. Yet, dysregulation of miR-145 and miR-210 has not been exclusive for the acute phase as they have been also up-regulated in recovery phase (Sepramaniam et al., 2014). Table 6 provides summary of studies reporting diagnostic/prognostic role of miRNAs in IS.
Animal Studies
Investigations in animal models of IS have provided valuable data about the mechanisms of involvement of lncRNAs/miRNAs in IS and possible application of targeted therapies against these transcripts. For instance, expression of RMST has been elevated in primary hippocampal neurons exposed with oxygen-glucose deprivation and in animal models of IS induced by middle cerebral artery occlusion (MCAO). RMST silencing has amended brain injury in the mentioned animal model and attenuated hippocampal neuron defects (Hou and Cheng, 2018). H19 is another up-regulated lncRNA in animal models of IS whose silencing has diminished the size of brain tissue damage following middle cerebral artery obstruction and reperfusion and ameliorated the neurological defects. Mechanistically, H19 silencing could reduce expression of neurogenesis related proteins. Taken together, H19 precludes the development of neurogenesis after IS via p53/Notch1 pathway (Wang et al., 2019a). A throughput miRNA sequencing in infarcted brain regions after regional cerebral ischemia has shown up-regulation of 20 miRNAs while down-regulation of 17 miRNAs in the infarct area among them have been miR-211-5p, miR-183-5p, miR-182, and miR-96-5p which have been functionally related with some important pathways in the neurons (Duan et al., 2019). Table 7 summarizes the data regarding the roles of up-regulated non-coding RNAs in the pathogenesis of IS as revealed by animal studies.
Meg3 is a down-regulated lncRNA after IS. Up-regulation of Meg3 has inhibited functional recovery and diminished capillary mass after IS. On the other hand, its silencing has amended brain lesions and enhanced angiogenesis after IS. Meg3 exerts these functions through inhibiting notch pathway (Liu et al., 2017). Expression of lncRNA-1810034E14Rik has also been down-regulated in LPS-exposed or oxygen-glucose deprivation-induced microglial cells. Up-regulation of 1810034E14Rik has reduced the infarct volume, ameliorated brain injury in MCAO model and decreased production of inflammatory cytokines both in the animal model and in microglial cells. Besides, 1810034E14Rik up-regulation could block the induction of microglial cells and suppress p65 phosphorylation of p65 (Qu et al., 2019). The above-mentioned examples indicate that down-regulation of lncRNAs in IS might be a compensative mechanism for amelioration of neuron damage or can be directly participate in the pathogenic mechanisms during IS. Table 8 summarizes the data regarding the roles of down-regulated non-coding RNAs in the pathogenesis of IS as revealed by animal studies.
Discussion
A wealth of information about the role of non-coding RNAs in the development of IS has been obtained from combination of RNA-sequencing assays and bioinformatics assays such as GO, KEGG pathway enrichment assays and network analyses. These kinds of studies not only exhibited dysregulation of these transcripts, but also provided mechanistical insights about their route of actions. Generally, non-coding RNAs might participate in the pathophysiology of IS through different routes. As a number of differentially expressed lncRNAs between IS patients and healthy controls map to genomic loci near IS-associated genes, regulation of gene expression through cis-acting modes is a possible route. Another possible mechanism of contribution of lncRNAs in the pathology of IS is their ceRNA role. MEG3/miR-424-5p, KCNQ1OT1/miR-200a and MALAT1/miR-205-5p are few examples of interplay between lncRNAs and miRNAs in the context of IS.
Aberrant expression of non-coding RNAs in IS patients might be due to the presence of a number of genomic variants within the coding genes as demonstrated for ANRIL lncRNA. This lncRNA is among the mostly assessed lncRNAs in IS. However, the results of all studies are not consistent in this regard. Such inconsistency might be due to phase of sampling during the course of IS or the presence of other confounding parameters. The presence of lncRNAs in the serum specimens and exosomes extracted from these specimens facilitates diagnosis of IS and its clinical variants using this noninvasive route of sampling.
MicroRNAs contribute in the pathogenesis of IS through modulation of genes implicated in the atherosclerosis or inflammatory responses. Exosomal miRNAs might affect communication between several types of cells including endothelial and smooth muscle cells. IS-related circulating miRNAs might hypothetically exert similar functions. Yet, this hypothesis should be judged in upcoming studies. Peripheral expression of miRNAs can be used to differentiate IS patients from healthy subjects or IS patients from other related conditions such as HS. Moreover, their signature might predict recovery from IS-related clinical signs.
The observed sex-biased pattern of differentially expression of lncRNAs (Dykstra-Aiello et al., 2016) might determine different pathogenic processes for the evolution of IS among men and women which should be further examined. Moreover, a number of investigations have displayed specific lncRNA signatures at certain time points following IS, demonstrating the specific roles of lncRNAs in each step of pathogenic processes following IS.
In spite of conduction of various functional studies to unravel the role of non-coding RNAs in IS, therapeutic application of these transcripts have not been clarified. Therefore, future investigation should appraise the possibility of using these transcripts as therapeutic targets in IS. Another limitation of most of mentioned studies is their relatively small sample sizes and lack of simultaneous appraisal of exposures and outcomes in cross-sectional studies. Application of non-coding RNAs as therapeutic targets for IS has faced some challenges in terms of safe delivery of the drug to specific targets, avoidance of off-target effects and determination of best time for intervention. This filed is still in its infancy.
Author Contributions
SG-F wrote the manuscript and revised it. MT designed the study and supervised it. NA, ZS-F, and BH collected the data and designed the figures and the tables. All authors approved the manuscript.
Conflict of Interest
The authors declare that the research was conducted in the absence of any commercial or financial relationships that could be construed as a potential conflict of interest.
The reviewer RE declared a shared affiliation with several of the authors, SG-F, ZS-F, and NA, to the handling editor at the time of the review.
Publisher’s Note
All claims expressed in this article are solely those of the authors and do not necessarily represent those of their affiliated organizations, or those of the publisher, the editors and the reviewers. Any product that may be evaluated in this article, or claim that may be made by its manufacturer, is not guaranteed or endorsed by the publisher.
Abbreviations
AF, atrial fibrillation; AUC, area under curve; ceRNAs, competing endogenous RNAs; GO, gene ontology; HS, hemorrhagic stroke; hs-CRP, high-sensitivity C-reactive protein; IS, Ischemic stroke; LAA, large-artery atherosclerosis; lncRNAs, long non-coding RNAs; MCAO, middle cerebral artery occlusion; miRNAs, microRNAs; NIHSS, NIH Stroke Scale; PBMCs, peripheral blood mononuclear cells; ROC, receiver operating characteristic; TIA, transient ischemic attack.
References
Bam, M., Yang, X., Sen, S., Zumbrun, E. E., Dennis, L., Zhang, J., et al. (2018). Characterization of dysregulated miRNA in Peripheral blood mononuclear cells from ischemic stroke patients. Mol. Neurobiol. 55, 1419–1429. doi: 10.1007/s12035-016-0347-8
Bonauer, A., Carmona, G., Iwasaki, M., Mione, M., Koyanagi, M., Fischer, A., et al. (2009). MicroRNA-92a controls angiogenesis and functional recovery of ischemic tissues in mice. Science 324, 1710–1713. doi: 10.1126/science.1174381
Caballero-Garrido, E., Pena-Philippides, J. C., Lordkipanidze, T., Bragin, D., Yang, Y., Erhardt, E. B., et al. (2015). In vivo inhibition of miR-155 promotes recovery after experimental mouse stroke. J. Neurosci. 35, 12446–12464. doi: 10.1523/jneurosci.1641-15.2015
Chang, L., Zhang, W., Shi, S., Peng, Y., Wang, D., Zhang, L., et al. (2020). microRNA-195 attenuates neuronal apoptosis in rats with ischemic stroke through inhibiting KLF5-mediated activation of the JNK signaling pathway. Mol. Med. 26:31. doi: 10.1186/s10020-020-00150-w
Chen, F., Zhang, L., Wang, E., Zhang, C., and Li, X. (2018a). LncRNA GAS5 regulates ischemic stroke as a competing endogenous RNA for miR-137 to regulate the Notch1 signaling pathway. Biochem. Biophys. Res. Commun. 496, 184–190. doi: 10.1016/j.bbrc.2018.01.022
Chen, Y., Song, Y., Huang, J., Qu, M., Zhang, Y., Geng, J., et al. (2017). Increased circulating exosomal miRNA-223 is associated with acute ischemic stroke. Front. Neurol. 8:57. doi: 10.3389/fneur.2017.00057
Chen, Z., Wang, K., Huang, J., Zheng, G., Lv, Y., Luo, N., et al. (2018b). Upregulated serum MiR-146b serves as a biomarker for acute ischemic stroke. Cell. Physiol. Biochem. 45, 397–405. doi: 10.1159/000486916
Cheng, J., Kapranov, P., Drenkow, J., Dike, S., Brubaker, S., Patel, S., et al. (2005). Transcriptional maps of 10 human chromosomes at 5-nucleotide resolution. Science 308, 1149–1154. doi: 10.1126/science.1108625
Cuomo, O., Cepparulo, P., Anzilotti, S., Serani, A., Sirabella, R., Brancaccio, P., et al. (2019). Anti-miR-223-5p ameliorates ischemic damage and improves neurological function by preventing NCKX2 downregulation after ischemia in rats. Mol. Therapy Nucleic Acids 18, 1063–1071. doi: 10.1016/j.omtn.2019.10.022
Deng, Q.-W., Li, S., Wang, H., Sun, H.-L., Zuo, L., Gu, Z.-T., et al. (2018). Differential long noncoding RNA expressions in peripheral blood mononuclear cells for detection of acute ischemic stroke. Clin. Sci. 132, 1597–1614. doi: 10.1042/cs20180411
Deng, W., Fan, C., Zhao, Y., Mao, Y., Li, J., Zhang, Y., et al. (2020a)). MicroRNA-130a regulates neurological deficit and angiogenesis in rats with ischaemic stroke by targeting XIAP. J. Cell Mol. Med. 24, 10987–11000. doi: 10.1111/jcmm.15732
Deng, Y., Chen, D., Gao, F., Lv, H., Zhang, G., Sun, X., et al. (2020b). Silencing of long non-coding RNA GAS5 suppresses neuron cell apoptosis and nerve injury in ischemic stroke through inhibiting DNMT3B-Dependent MAP4K4 methylation. Trans. Stroke Res. 11, 950–966. doi: 10.1007/s12975-019-00770-3
Deng, Y., Chen, D., Wang, L., Gao, F., Jin, B., Lv, H., et al. (2019). Silencing of long noncoding RNA nespas aggravates microglial cell death and neuroinflammation in ischemic stroke. Stroke 50, 1850–1858. doi: 10.1161/STROKEAHA.118.023376
Derrien, T., Johnson, R., Bussotti, G., Tanzer, A., Djebali, S., Tilgner, H., et al. (2012). The GENCODE v7 catalog of human long noncoding RNAs: analysis of their gene structure, evolution, and expression. Genome Res. 22, 1775–1789. doi: 10.1101/gr.132159.111
Dong, H., Cui, B., and Hao, X. (2019). MicroRNA-22 alleviates inflammation in ischemic stroke via p38 MAPK pathways. Mol. Med. Rep. 20, 735–744. doi: 10.3892/mmr.2019.10269
Duan, X., Gan, J., Peng, D. Y., Bao, Q., Xiao, L., Wei, L., et al. (2019). Identification and functional analysis of microRNAs in rats following focal cerebral ischemia injury. Mol. Med. Rep. 19, 4175–4184. doi: 10.3892/mmr.2019.10073
Dykstra-Aiello, C., Jickling, G. C., Ander, B. P., Shroff, N., Zhan, X., Liu, D., et al. (2016). Altered expression of long noncoding RNAs in blood after ischemic stroke and proximity to putative stroke risk loci. Stroke 47, 2896–2903. doi: 10.1161/STROKEAHA.116.013869
Edwardson, M. A., Zhong, X., Cheema, A., and Dromerick, A. (2018a). Plasma microRNA markers of upper limb recovery following human stroke. J. Clin. Trans. Sci. 2:45. doi: 10.1017/cts.2018.176
Edwardson, M. A., Zhong, X., Fiandaca, M. S., Federoff, H. J., Cheema, A. K., and Dromerick, A. W. (2018b). Plasma microRNA markers of upper limb recovery following human stroke. Sci. Rep. 8:12558.
Fang, Y., and Fullwood, M. J. (2016). Roles, functions, and mechanisms of long non-coding RNAs in cancer. Genom. Proteom. Bioinform. 14, 42–54. doi: 10.1016/j.gpb.2015.09.006
Feigin, V. L., Krishnamurthi, R. V., Parmar, P., Norrving, B., Mensah, G. A., Bennett, D. A., et al. (2015). Update on the global burden of ischemic and hemorrhagic stroke in 1990-2013: the GBD 2013 study. Neuroepidemiology 45, 161–176. doi: 10.1159/000441085
Feng, L., Guo, J., and Ai, F. (2019). Circulating long noncoding RNA ANRIL downregulation correlates with increased risk, higher disease severity and elevated pro-inflammatory cytokines in patients with acute ischemic stroke. J. Clin. Lab. Anal. 33:e22629. doi: 10.1002/jcla.22629
Feng, M., Zhu, X., and Zhuo, C. (2021). H19/miR-130a-3p/DAPK1 axis regulates the pathophysiology of neonatal hypoxic-ischemia encephalopathy. Neurosci. Res. 163, 52–62. doi: 10.1016/j.neures.2020.03.005
Gan, C., Wang, C., and Tan, K. (2012). Circulatory microRNA-145 expression is increased in cerebral ischemia. Genet. Mol. Res. 11, 147–152. doi: 10.4238/2012.January.27.1
Gao, C., Zhang, C.-C., Yang, H.-X., and Hao, Y.-N. (2020). MALAT1 protected the angiogenesis function of human brain microvascular endothelial cells (HBMECs) under oxygen glucose deprivation/re-oxygenation (OGD/R) challenge by interacting with miR-205-5p/VEGFA pathway. Neuroscience 435, 135–145. doi: 10.1016/j.neuroscience.2020.03.027
Geng, W., Tang, H., Luo, S., Lv, Y., Liang, D., Kang, X., et al. (2019). Exosomes from miRNA-126-modified ADSCs promotes functional recovery after stroke in rats by improving neurogenesis and suppressing microglia activation. Am. J. Transl. Res. 11, 780–792.
Guang, Y., Zhendong, L., Lu, W., Xin, C., Xiaoxiong, W., Qi, D., et al. (2019). MicroRNA-195 protection against focal cerebral ischemia by targeting CX3CR1. J. Neurosurg. JNS 131, 1445–1454. doi: 10.3171/2018.5.JNS173061
Guo, D., Ma, J., Yan, L., Li, T., Li, Z., Han, X., et al. (2017). Down-Regulation of lncrna MALAT1 attenuates neuronal cell death through suppressing beclin1-dependent autophagy by regulating Mir-30a in cerebral ischemic stroke. Cell. Physiol. Biochem. 43, 182–194. doi: 10.1159/000480337
Guo, X., Yang, J., Liang, B., Shen, T., Yan, Y., Huang, S., et al. (2018). Identification of novel LncRNA biomarkers and construction of LncRNA-Related networks in han chinese patients with ischemic stroke. Cell. Physiol. Biochem. 50, 2157–2175. doi: 10.1159/000495058
Hou, X.-X., and Cheng, H. (2018). Long non-coding RNA RMST silencing protects against middle cerebral artery occlusion (MCAO)-induced ischemic stroke. Biochem. Biophys. Res. Commun. 495, 2602–2608. doi: 10.1016/j.bbrc.2017.12.087
Huang, S., Lv, Z., Guo, Y., Li, L., Zhang, Y., Zhou, L., et al. (2016). Identification of blood Let-7e-5p as a biomarker for ischemic stroke. PLoS One 11:e0163951. doi: 10.1371/journal.pone.0163951
Huang, Y., Wang, L., Mao, Y., and Nan, G. (2019). Long noncoding RNA-H19 contributes to atherosclerosis and induces ischemic stroke via the upregulation of acid phosphatase 5. Front. Neurol. 10:32. doi: 10.3389/fneur.2019.00032
Ji, Q., Ji, Y., Peng, J., Zhou, X., Chen, X., Zhao, H., et al. (2016). Increased brain-specific MiR-9 and MiR-124 in the serum exosomes of acute ischemic stroke patients. PLoS One 11:e0163645. doi: 10.1371/journal.pone.0163645
Jia, J., Cui, Y., Tan, Z., Ma, W., and Jiang, Y. (2020). MicroRNA-579-3p exerts neuroprotective effects against ischemic stroke via anti-inflammation and anti-apoptosis. Neuropsychiatric Dis. Treatment 16, 1229–1238. doi: 10.2147/ndt.s240698
Jia, L., Hao, F., Wang, W., and Qu, Y. (2015). Circulating miR-145 is associated with plasma high-sensitivity C-reactive protein in acute ischemic stroke patients. Cell Biochem. Funct. 33, 314–319. doi: 10.1002/cbf.3116
Jickling, G. C., Ander, B. P., Zhan, X., Noblett, D., Stamova, B., and Liu, D. (2014). microRNA expression in peripheral blood cells following acute ischemic stroke and their predicted gene targets. PLoS One 9:e99283. doi: 10.1371/journal.pone.0099283
Jin, F., and Xing, J. (2017). Circulating pro-angiogenic and anti-angiogenic microRNA expressions in patients with acute ischemic stroke and their association with disease severity. Neurol. Sci. 38, 2015–2023. doi: 10.1007/s10072-017-3071-x
Jo, M. H., Shin, S., Jung, S.-R., Kim, E., Song, J.-J., and Hohng, S. (2015). Human Argonaute 2 has diverse reaction pathways on target RNAs. Mol. Cell 59, 117–124. doi: 10.1016/j.molcel.2015.04.027
Kim, T., Mehta, S. L., Morris-Blanco, K. C., Chokkalla, A. K., Chelluboina, B., Lopez, M., et al. (2018). The microRNA miR-7a-5p ameliorates ischemic brain damage by repressing α-synuclein. Sci. Signal. 11:eaat4285. doi: 10.1126/scisignal.aat4285
Kolosowska, N., Gotkiewicz, M., Dhungana, H., Giudice, L., Giugno, R., Box, D., et al. (2020). Intracerebral overexpression of miR-669c is protective in mouse ischemic stroke model by targeting MyD88 and inducing alternative microglial/macrophage activation. J. Neuroinflamm. 17:194. doi: 10.1186/s12974-020-01870-w
Kotb, H. G., Ibrahim, A. H., Mohamed, E. F., Ali, O. M., Hassanein, N., Badawy, D., et al. (2019). The expression of microRNA 146a in patients with ischemic stroke: an observational study. Int. J. Gen. Med. 12, 273–278. doi: 10.2147/IJGM.S213535
Lee, R. C., Feinbaum, R. L., and Ambros, V. (1993). The C. elegans heterochronic gene lin-4 encodes small RNAs with antisense complementarity to lin-14. Cell 75, 843–854. doi: 10.1016/0092-8674(93)90529-y
Leung, L. Y., Chan, C. P., Leung, Y. K., Jiang, H. L., Abrigo, J. M., Wang de, F., et al. (2014). Comparison of miR-124-3p and miR-16 for early diagnosis of hemorrhagic and ischemic stroke. Clin. Chim. Acta 433, 139–144. doi: 10.1016/j.cca.2014.03.007
Li, L., Dong, L., Zhao, J., He, W., Chu, B., Zhang, J., et al. (2020). Circulating miRNA-3552 as a potential biomarker for ischemic stroke in rats. BioMed. Res. Int. 2020:4501393. doi: 10.1155/2020/4501393
Li, P., Teng, F., Gao, F., Zhang, M., Wu, J., and Zhang, C. (2015). Identification of circulating MicroRNAs as potential biomarkers for detecting acute ischemic stroke. Cell Mol. Neurobiol. 35, 433–447.
Liang, J., Wang, Q., Li, J.-Q., Guo, T., and Yu, D. (2020). Long non-coding RNA MEG3 promotes cerebral ischemia-reperfusion injury through increasing pyroptosis by targeting miR-485/AIM2 axis. Exp. Neurol. 325:113139. doi: 10.1016/j.expneurol.2019.113139
Liang, Y., Xu, J., Wang, Y., Tang, J. Y., Yang, S. L., Xiang, H. G., et al. (2018a). Inhibition of MiRNA-125b decreases cerebral ischemia/reperfusion injury by targeting CK2α/NADPH oxidase signaling. Cell. Physiol. Biochem. 45, 1818–1826. doi: 10.1159/000487873
Liang, Z., Chi, Y. J., Lin, G. Q., Luo, S. H., Jiang, Q. Y., and Chen, Y. K. (2018b). MiRNA-26a promotes angiogenesis in a rat model of cerebral infarction via PI3K/AKT and MAPK/ERK pathway. Eur. Rev. Med. Pharmacol. Sci. 22, 3485–3492. doi: 10.26355/eurrev_201806_15175
Liaw, N., and Liebeskind, D. (2020). Emerging therapies in acute ischemic stroke. F1000Res 9:F1000 Faculty Rev-546.
Liu, J., Li, Q., Zhang, K.-S., Hu, B., Niu, X., Zhou, S.-M., et al. (2017). Downregulation of the long non-coding RNA Meg3 promotes angiogenesis after ischemic brain injury by activating notch signaling. Mol. Neurobiol. 54, 8179–8190. doi: 10.1007/s12035-016-0270-z
Liu, X. S., Chopp, M., Wang, X. L., Zhang, L., Hozeska-Solgot, A., Tang, T., et al. (2013). MicroRNA-17-92 cluster mediates the proliferation and survival of neural progenitor cells after stroke. J. Biol. Chem. 288, 12478–12488. doi: 10.1074/jbc.M112.449025
Liu, X. S., Fan, B., Wang, X., Chopp, M., and Zhang, Z. G. (2019). “Differential long noncoding RNA and messenger RNA expression profiling and functional network analysis in stroke-induced neurogenesis,” in Proceedings of the 2019 IEEE International Conference on Bioinformatics and Biomedicine (BIBM), (Piscataway, NJ: IEEE).
Liu, Y., Zhang, J., Han, R., Liu, H., Sun, D., and Liu, X. (2015). Downregulation of serum brain specific microRNA is associated with inflammation and infarct volume in acute ischemic stroke. J. Clin. Neurosci. 22, 291–295. doi: 10.1016/j.jocn.2014.05.042
Liu da, Z., Jickling, G. C., Ander, B. P., Hull, H., Zhan, X., Cox, C., et al. (2016). Elevating microRNA-122 in blood improves outcomes after temporary middle cerebral artery occlusion in rats. J. Cereb. Blood Flow Metab. 36, 1374–1383. doi: 10.1177/0271678X15610786
Long, G., Wang, F., Li, H., Yin, Z., Sandip, C., Lou, Y., et al. (2013). Circulating miR-30a, miR-126 and let-7b as biomarker for ischemic stroke in humans. BMC Neurol. 13:178. doi: 10.1186/1471-2377-13-178
O’Brien, J., Hayder, H., Zayed, Y., and Peng, C. (2018). Overview of microRNA biogenesis, mechanisms of actions, and circulation. Front. Endocrin. 9:402. doi: 10.3389/fendo.2018.00402
Peng, G., Yuan, Y., Wu, S., He, F., Hu, Y., and Luo, B. (2015). MicroRNA let-7e is a potential circulating biomarker of acute stage ischemic stroke. Trans. Stroke Res. 6, 437–445. doi: 10.1007/s12975-015-0422-x
Qu, M., Pan, J., Wang, L., Zhou, P., Song, Y., Wang, S., et al. (2019). MicroRNA-126 regulates angiogenesis and neurogenesis in a mouse model of focal cerebral ischemia. Mol. Therapy Nucleic Acids 16, 15–25. doi: 10.1016/j.omtn.2019.02.002
Salinas, J., Lin, H., Aparico, H. J., Huan, T., Liu, C., Rong, J., et al. (2019). Whole blood microRNA expression associated with stroke: results from the framingham heart study. PLoS One 14:e0219261. doi: 10.1371/journal.pone.0219261
Sepramaniam, S., Tan, J.-R., Tan, K.-S., DeSilva, D. A., Tavintharan, S., Woon, F.-P., et al. (2014). Circulating microRNAs as biomarkers of acute stroke. Int. J. Mol. Sci. 15, 1418–1432.
Shi, F.-P., Wang, X.-H., Zhang, H.-X., Shang, M.-M., Liu, X.-X., Sun, H.-M., et al. (2018). MiR-103 regulates the angiogenesis of ischemic stroke rats by targeting vascular endothelial growth factor (VEGF). Iran. J. Basic Med. Sci. 21, 318–324. doi: 10.22038/IJBMS.2018.27267.6657
Silva, C. I., Novais, P. C., Rodrigues, A. R., Carvalho, C. A. M., Colli, B. O., and Carlotti, C. G. Jr., et al. (2017). Expression of NMDA receptor and microRNA-219 in rats submitted to cerebral ischemia associated with alcoholism. Arquivos de Neuro-Psiquiatria 75, 30–35. doi: 10.1590/0004-282X20160188
Sonoda, T., Matsuzaki, J., Yamamoto, Y., Sakurai, T., Aoki, Y., Takizawa, S., et al. (2019). Serum MicroRNA-Based risk prediction for stroke. Stroke 50, 1510–1518. doi: 10.1161/STROKEAHA.118.023648
Sørensen, S. S., Nygaard, A.-B., Carlsen, A. L., Heegaard, N. H. H., Bak, M., and Christensen, T. (2017). Elevation of brain-enriched miRNAs in cerebrospinal fluid of patients with acute ischemic stroke. Biomarker Res. 5:24. doi: 10.1186/s40364-017-0104-9
Spinetti, G., Fortunato, O., Caporali, A., Shantikumar, S., Marchetti, M., Meloni, M., et al. (2013). MicroRNA-15a and microRNA-16 impair human circulating proangiogenic cell functions and are increased in the proangiogenic cells and serum of patients with critical limb ischemia. Circ. Res. 112, 335–346. doi: 10.1161/CIRCRESAHA.111.300418
Suofu, Y., Wang, X., He, Y., Li, F., Zhang, Y., Carlisle, D. L., et al. (2020). Mir-155 knockout protects against ischemia/reperfusion-induced brain injury and hemorrhagic transformation. NeuroReport 31, 235–239. doi: 10.1097/WNR.0000000000001382
Tao, Z., Zhao, H., Wang, R., Liu, P., Yan, F., Zhang, C., et al. (2015). Neuroprotective effect of microRNA-99a against focal cerebral ischemia-reperfusion injury in mice. J. Neurol. Sci. 355, 113–119. doi: 10.1016/j.jns.2015.05.036
Tian, C., Li, Z., Yang, Z., Huang, Q., Liu, J., and Hong, B. (2016). Plasma MicroRNA-16 is a biomarker for diagnosis, stratification, and prognosis of hyperacute cerebral infarction. PLoS One 11:e0166688. doi: 10.1371/journal.pone.0166688
Tiedt, S., Prestel, M., Malik, R., Schieferdecker, N., Duering, M., Kautzky, V., et al. (2017). RNA-Seq identifies circulating miR-125a-5p, miR-125b-5p, and miR-143-3p as potential biomarkers for acute ischemic stroke. Circ. Res. 121, 970–980. doi: 10.1161/CIRCRESAHA.117.311572
van Kralingen, J. C., McFall, A., Ord, E. N. J., Coyle, T. F., Bissett, M., McClure, J. D., et al. (2019). Altered extracellular vesicle microRNA expression in ischemic stroke and small vessel disease. Trans. Stroke Res. 10, 495–508. doi: 10.1007/s12975-018-0682-3
Vijayan, M., Kumar, S., Yin, X., Zafer, D., Chanana, V., Cengiz, P., et al. (2018). Identification of novel circulatory microRNA signatures linked to patients with ischemic stroke. Hum. Mol. Genet. 27, 2318–2329. doi: 10.1093/hmg/ddy136
Wang, H., Zheng, X., Jin, J., Zheng, L., Guan, T., Huo, Y., et al. (2020a). LncRNA MALAT1 silencing protects against cerebral ischemia-reperfusion injury through miR-145 to regulate AQP4. J. Biomed. Sci. 27:40. doi: 10.1186/s12929-020-00635-0
Wang, J., Cao, B., Zhao, H., Gao, Y., Luo, Y., Chen, Y., et al. (2019a). Long noncoding RNA H19 prevents neurogenesis in ischemic stroke through p53/Notch1 pathway. Brain Res. Bull. 150, 111–117. doi: 10.1016/j.brainresbull.2019.05.009
Wang, J., Ruan, J., Zhu, M., Yang, J., Du, S., Xu, P., et al. (2019b). Predictive value of long noncoding RNA ZFAS1 in patients with ischemic stroke. Clin. Exp. Hypertens 41, 615–621. doi: 10.1080/10641963.2018.1529774
Wang, J., Zhao, H., Fan, Z., Li, G., Ma, Q., Tao, Z., et al. (2017). Long noncoding RNA H19 promotes neuroinflammation in ischemic stroke by driving histone deacetylase 1-Dependent M1 microglial polarization. Stroke 48, 2211–2221. doi: 10.1161/STROKEAHA.117.017387
Wang, W., Sun, G., Zhang, L., Shi, L., and Zeng, Y. (2014). Circulating microRNAs as novel potential biomarkers for early diagnosis of acute stroke in humans. J. Stroke Cereb. Dis. 23, 2607–2613. doi: 10.1016/j.jstrokecerebrovasdis.2014.06.002
Wang, X., Chen, S., Ni, J., Cheng, J., Jia, J., and Zhen, X. (2018). miRNA-3473b contributes to neuroinflammation following cerebral ischemia. Cell Death Dis. 9:11. doi: 10.1038/s41419-017-0014-7
Wang, Y., Luo, Y., Yao, Y., Ji, Y., Feng, L., Du, F., et al. (2020b). Silencing the lncRNA Maclpil in pro-inflammatory macrophages attenuates acute experimental ischemic stroke via LCP1 in mice. J. Cereb. Blood Flow Metab. 40, 747–759. doi: 10.1177/0271678X19836118
Wu, J., Du, K., and Lu, X. (2015). Elevated expressions of serum miR-15a, miR-16, and miR-17-5p are associated with acute ischemic stroke. Int. J. Clin. Exp. Med. 8, 21071–21079.
Wu, J., Fan, C. L., Ma, L. J., Liu, T., Wang, C., Song, J. X., et al. (2017). Distinctive expression signatures of serum microRNAs in ischaemic stroke and transient ischaemic attack patients. Thrombosis Haemostasis 117, 992–1001. doi: 10.1160/TH16-08-0606
Xiang, Y., Zhang, Y., Xia, Y., Zhao, H., Liu, A., and Chen, Y. (2020). LncRNA MEG3 targeting miR-424-5p via MAPK signaling pathway mediates neuronal apoptosis in ischemic stroke. Aging 12, 3156–3174. doi: 10.18632/aging.102790
Xiao, Z. H., Wang, L., Gan, P., He, J., Yan, B. C., and Ding, L. D. (2020). Dynamic changes in miR-126 expression in the hippocampus and penumbra following experimental transient global and focal cerebral ischemia–reperfusion. Neurochem. Res. 45, 1107–1119. doi: 10.1007/s11064-020-02986-4
Xu, X., Zhuang, C., and Chen, L. (2020). Exosomal long non-coding RNA expression from serum of patients with acute minor stroke. Neuropsychiatric Dis. Treatment 16:153. doi: 10.2147/NDT.S230332
Yang, J., Gu, L., Guo, X., Huang, J., Chen, Z., Huang, G., et al. (2018). LncRNA ANRIL expression and ANRIL gene polymorphisms contribute to the risk of ischemic stroke in the chinese han population. Cell Mol. Neurobiol. 38, 1253–1269. doi: 10.1007/s10571-018-0593-6
Yao, P., Li, Y.-L., Chen, Y., Shen, W., Wu, K.-Y., and Xu, W.-H. (2020). Overexpression of long non-coding RNA Rian attenuates cell apoptosis from cerebral ischemia-reperfusion injury via Rian/miR-144-3p/GATA3 signaling. Gene 737:144411. doi: 10.1016/j.gene.2020.144411
Yin, K.-J., Deng, Z., Huang, H., Hamblin, M., Xie, C., Zhang, J., et al. (2010). miR-497 regulates neuronal death in mouse brain after transient focal cerebral ischemia. Neurobiol. Dis. 38, 17–26. doi: 10.1016/j.nbd.2009.12.021
You, D., and You, H. (2019). Repression of long non-coding RNA MEG3 restores nerve growth and alleviates neurological impairment after cerebral ischemia-reperfusion injury in a rat model. Biomed. Pharmacotherapy 111, 1447–1457. doi: 10.1016/j.biopha.2018.12.067
Zeng, W., and Jin, J. (2020). The correlation of serum long non-coding RNA ANRIL with risk factors, functional outcome, and prognosis in atrial fibrillation patients with ischemic stroke. J. Clin. Lab. Anal. 34:e23352. doi: 10.1002/jcla.23352
Zhan, R., Xu, K., Pan, J., Xu, Q., Xu, S., and Shen, J. (2017). Long noncoding RNA MEG3 mediated angiogenesis after cerebral infarction through regulating p53/NOX4 axis. Biochem. Biophys. Res. Commun. 490, 700–706. doi: 10.1016/j.bbrc.2017.06.104
Zhang, B., Wang, D., Ji, T.-F., Shi, L., and Yu, J.-L. (2017a). Overexpression of lncRNA ANRIL up-regulates VEGF expression and promotes angiogenesis of diabetes mellitus combined with cerebral infarction by activating NF-κB signaling pathway in a rat model. Oncotarget 8, 17347–17359. doi: 10.18632/oncotarget.14468
Zhang, X., Tang, X., Liu, K., Hamblin, M. H., and Yin, K.-J. (2017b). Long noncoding RNA Malat1 regulates cerebrovascular pathologies in ischemic stroke. J. Neurosci. 37, 1797–1806. doi: 10.1523/jneurosci.3389-16.2017
Zhanin, I. S., Gusar, V. A., Timofeeva, A. T., Pinelis, V. G., and Asanov, A. Y. (2018). MicroRNA expression profile in patients in the early stages of ischemic stroke. Neurol. Neuropsychiatry Psychosomat. 10, 72–78. doi: 10.1016/j.jpsychires.2019.05.018
Zhao, H., Wang, J., Gao, L., Wang, R., Liu, X., Gao, Z., et al. (2013). MiRNA-424 protects against permanent focal cerebral ischemia injury in mice involving suppressing microglia activation. Stroke 44, 1706–1713. doi: 10.1161/STROKEAHA.111.000504
Zheng, L., Cheng, W., Wang, X., Yang, Z., Zhou, X., and Pan, C. (2017). Overexpression of MicroRNA-145 ameliorates astrocyte injury by targeting aquaporin 4 in cerebral ischemic stroke. BioMed. Res. Int. 2017:9530951. doi: 10.1155/2017/9530951
Zheng, Z., Liu, S., Wang, C., and Han, X. (2018). A functional polymorphism rs145204276 in the promoter of long noncoding RNA GAS5 is associated with an increased risk of ischemic stroke. J. Stroke Cereb. Dis. 27, 3535–3541. doi: 10.1016/j.jstrokecerebrovasdis.2018.08.016
Zhu, M., Li, N., Luo, P., Jing, W., Wen, X., Liang, C., et al. (2018). Peripheral blood leukocyte expression of lncRNA MIAT and its diagnostic and prognostic value in ischemic stroke. J. Stroke Cereb. Dis. 27, 326–337. doi: 10.1016/j.jstrokecerebrovasdis.2017.09.009
Zhu, W., Tian, L., Yue, X., Liu, J., Fu, Y., and Yan, Y. (2019). LncRNA expression profiling of ischemic stroke during the transition from the acute to subacute stage. Front. Neurol. 10:36. doi: 10.3389/fneur.2019.00036
Keywords: lncRNA, miRNA, stroke, expression, biomarker
Citation: Ghafouri-Fard S, Shirvani-Farsani Z, Hussen BM, Taheri M and Arefian N (2021) Emerging Impact of Non-coding RNAs in the Pathology of Stroke. Front. Aging Neurosci. 13:780489. doi: 10.3389/fnagi.2021.780489
Received: 21 September 2021; Accepted: 28 October 2021;
Published: 19 November 2021.
Edited by:
Li Li, Capital Medical University, ChinaReviewed by:
Laurent Metzinger, University of Picardie Jules Verne, FranceAtefe Abak, Tabriz University of Medical Sciences, Iran
Ilgiz Fanilevich Gareev, First Affiliated Hospital of Harbin Medical University, China
Hazha Hidayat, Salahaddin University, Iraq
Reyhane Eghtedarian, Shahid Beheshti University, Iran
Copyright © 2021 Ghafouri-Fard, Shirvani-Farsani, Hussen, Taheri and Arefian. This is an open-access article distributed under the terms of the Creative Commons Attribution License (CC BY). The use, distribution or reproduction in other forums is permitted, provided the original author(s) and the copyright owner(s) are credited and that the original publication in this journal is cited, in accordance with accepted academic practice. No use, distribution or reproduction is permitted which does not comply with these terms.
*Correspondence: Mohammad Taheri, Mohammad_823@yahoo.com; Noormohammad Arefian, narefian@yahoo.com