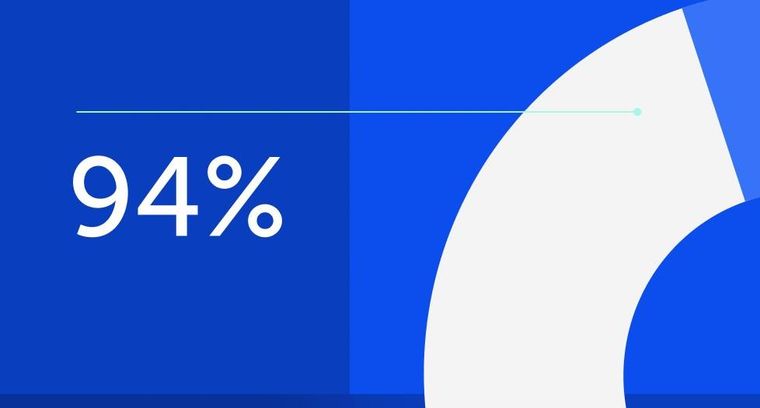
94% of researchers rate our articles as excellent or good
Learn more about the work of our research integrity team to safeguard the quality of each article we publish.
Find out more
ORIGINAL RESEARCH article
Front. Aging Neurosci., 10 January 2022
Sec. Parkinson’s Disease and Aging-related Movement Disorders
Volume 13 - 2021 | https://doi.org/10.3389/fnagi.2021.750767
This article is part of the Research TopicPrefrontal and Neuromodulatory Circuits in Cognitive Function and DysfunctionView all 5 articles
This study aimed to explore the disrupted prefrontal cortex activity specific to patients with Parkinson's disease (PD) with rapid eye movement sleep behavior disorder (RBD) compared with those without and to further examine the associations between these alterations and neuropsychological measurements. Ninety-six patients with early PD underwent both structural and functional MRI, and also neuropsychological assessments in the Parkinson's Progression Markers Initiative (PPMI) database. Of these, 46 patients who completed 1- and 3-year fMRI follow-up examinations were categorized as PD with probable RBD (PD-pRBD+) and without (PD-pRBD−). The left dorsolateral prefrontal cortex (DLPFC) seed-to-voxel functional connectivity analysis was conducted to evaluate the progressive neural alterations specific to PD-pRBD+ compared with PD-pRBD− over time. Furthermore, relationships between these alterations and neuropsychological performance were examined. Compared with patients with PD-pRBD−, patients with PD-pRBD+ initially exhibited connectivity deficits between the left DLPFC and the medial frontopolar cortex. Moreover, these patients further exhibited disrupted DLPFC connectivity in the lateral frontopolar cortex at the 3-year follow-up evaluation. Correlation analysis revealed that connectivity between the left DLPFC and frontopolar cortex was positively related to executive function in PD-pRBD+ after adjusting for nuisance variables. Progressive prefrontal cortex dysfunction associated with RBD in early PD may provide an effective subtype-specific biomarker of neurodegenerative progression, which may shed light on the neuropathological mechanisms underlying the clinical heterogeneity of this disease.
Sleep disturbances are frequently experienced in patients with Parkinson's disease (PD) (Fereshtehnejad et al., 2017; Jozwiak et al., 2017). Specifically, rapid eye movement (REM) sleep behavior disorder (RBD) affects 30–40% of PD (Sixel-Döring et al., 2016), which is associated with early neuropathological progression of this disease (Muzur et al., 2002; Vendette et al., 2007; Kim and Jeon, 2014; Pagano et al., 2018; Kim et al., 2019, 2020; Figorilli et al., 2020). However, the pathophysiology of RBD in PD is still widely unknown.
Currently, evidence has emerged linking RBD in PD to prefrontal cortex dysfunction (Lerche and Brockmann, 2018; Hanuška et al., 2019). A growing body of evidence have revealed that patients with PD having RBD have significantly impaired executive function (Fantini et al., 2018; Kamble et al., 2019), which significantly worsened during the progression of RBD symptoms in PD (Figorilli et al., 2020). In particular, a body of task functional magnetic resonance imaging (fMRI) studies have demonstrated that the dorsolateral prefrontal cortex (DLPFC) plays a cardinal role in executive function (Perlstein et al., 2001; Jia et al., 2011, 2015b; Liang et al., 2014, 2016; Panikratova et al., 2020). Furthermore, the DLPFC has been implicated in both REM-related cortical activation (Ioannides et al., 2009) and the pathophysiological basis of neurodegenerative progression in PD (Doruk et al., 2014). However, little is known about whether DLPFC activity demonstrates a specific alteration pattern in patients with PD having RBD compared with those without RBD. The neurobiological mechanisms underlying this dysfunction in early PD and the progressive alterations over time remain largely unexplored.
Accordingly, the progressive functional alterations specific to patients with PD with RBD were of particular interest in this work. The aims of this work were to explore the altered functional connectivity (FC) in the DLPFC specific to PD in patients with probable RBD (PD-pRBD+) compared with those in patients without probable RBD (PD-pRBD−) and to further explore the associations between these alterations and neuropsychological measures in these patients. On the basis of previous studies (Ioannides et al., 2009; Doruk et al., 2014; Panikratova et al., 2020), it was hypothesized that patients with PD-pRBD+ might exhibit prefrontal cortex dysfunction during the progression of this disease.
Participants were acquired from Parkinson's Progression Markers Initiative (PPMI) (http://www.ppmi-info.org/). The inclusion criteria were as follows: (1) at least two motor symptoms, (2) PD diagnosis within 2 years at baseline and an early clinical disease stage with Hoehn and Yahr stage of I–II, (3) no symptomatic treatment within 6 months of baseline, and (4) a dopamine transporter (DAT) deficit.
In this work, only individuals who underwent both structural and fMRI scanning were included. In total, there were 96 patients with PD and 22 healthy controls (HCs) at the acquisition of the first fMRI scan (i.e., baseline fMRI), 80 patients with PD at the 1-year follow-up, and 46 patients with PD at the 3-year follow-up. The study was approved by the institutional review board at each PPMI site. Written informed consent from all participants was obtained.
For each participant, a comprehensive set of motor and non-motor symptoms was assessed. The severity and stage of the disease were assessed by the Movement Disorder Society-Unified Parkinson's Disease Rating Scale III (MDS-UPDRS III) and the Hoehn and Yahr stage. Sleep disturbance was evaluated by the Epworth sleepiness scale (ESS) and RBD screening questionnaire (RBDSQ).
All participants were administered the Montreal Cognitive Assessment (MoCA) to evaluate global cognition, the Benton Judgment of Line Orientation Score (JLO) to assess visuospatial ability, the Hopkins Verbal Learning Test-Revised (HVLT-R) to evaluate memory, the Letter–Number Sequencing (LNS), and Semantic Fluency to assess executive function/working memory, and the Symbol Digit Modalities Test (SDMT) to evaluate attention. Autonomic symptoms were assessed by the Scales for Outcomes in PD-Autonomic (SCOPA-AUT), depression was assessed by the 15-item Geriatric Depression Scale (GDS-15), and anxiety was evaluated by the State-Trait Anxiety Inventory (STAI) score. For the purpose of longitudinal assessments, the clinical and MRI data of the 46 patients with PD who completed all 3 fMRI visits (i.e., onset, 1-, and 3-year follow-up) were comprehensively analyzed. Of these, 18 patients with PD were categorized as PD-pRBD+ and 28 as PD-pRBD− according to RBDSQ with a cut-off score of 5, as suggested by the PPMI database (Mahajan et al., 2014) (Figure 1). In addition, the data of HCs were also included for comparison with the data of the patients. Clinical characteristics and neuropsychological assessments are presented in Table 1.
Figure 1. Flowchart of data inclusion. T0, fMRI data at onset; T1, the 1-year follow-up evaluation; T2, the 3-year follow-up evaluation; PD-pRBD+, Parkinson's disease (PD) with probable rapid eye movement (REM) sleep behavior disorder (pRBD); PD-pRBD−, PD without pRBD; HC, healthy control.
Table 1. Longitudinal demographic characteristics and neuropsychological assessments of PD-pRBD+ and PD-pRBD− patients over time.
High-resolution structural images were acquired by using 3D T1-weighted MPRAGE. The scan parameters were as follows: repetition time (TR) = 2,300 ms, echo time (TE) = 2.89 ms, flip angle = 9°, matrix size = 256 × 240, and 176 1 mm sagittal slices. FMRI data were obtained by using an EPI sequence that lasted 7 min (210 volumes) with the following parameters: TR = 2,400 ms, TE = 25 ms, flip angle = 80°, field of view = 240 mm × 240 mm, matrix size = 68 × 66, 40 slices, and slice thickness = 3.3 mm. Since fMRI data were not administered at first in beginning of the PPMI project, in this work, the baseline fMRI referred to first fMRI examination performed upon onset.
We performed a voxel-based morphometry (VBM) analysis using SPM12 (http://www.fil.ion.ucl.ac.uk/spm) during the visit of patients with PD to determine structural alterations between the two groups during disease progression. T1-weighted images were segmented using the unified segmentation model into gray matter (GM), white matter (WM), and cerebrospinal fluid (CSF) based on tissue probability maps in Montreal Neurological Institute (MNI) space. The spatially normalized GM maps were modulated by the Jacobian determinant of the deformation field and corrected for individual brain sizes. The modulated and normalized GM images (voxel size: 1.5 × 1.5 × 1.5 mm3) were smoothed with an 8-mm full width at half maximum (FWHM) isotropic Gaussian kernel.
Rs-fMRI data were preprocessed using SPM12, and seed-to-voxel correlation analysis was carried out by the FC (CONN) toolbox v19c (Whitfield-Gabrieli and Nieto-Castanon, 2012). The first 10 functional images were discarded to reduce the fluctuation of the MRI signal in the initial stage of scanning. The remaining 200 images of each participant were first corrected for slice timing and then realigned to eliminate the influence of head motion. Next, the realigned images were coregistered to T1 images and normalized into MNI template space using transformations from segmentation and resampled to 3 × 3 × 3 mm3. Subsequently, images were smoothed with a 6-mm FWHM isotropic Gaussian kernel.
After preprocessing, the images were then bandpass-filtered to 0.008–0.09 Hz to remove physiological noise. Artifact detection tools-based identification of outlier scans for scrubbing was performed, after which more than 90% of the valid scans remained. Further denoising steps included regression of the six motion parameters and their first-order derivatives, regression of WM and CSF signals following the implemented CompCor strategy (Behzadi et al., 2007), and linear detrending.
The seed region of the left DLPFC was defined by WFU_PickAtlas (http://fmri.wfubmc.edu/cms/software). The correlation coefficients between the seed voxels and all other brain voxels were computed to generate correlation maps. For group analyses, the correlation coefficients were converted to z-values using Fisher's r-to-z transformation to improve normality (Lowe et al., 1998).
For clinical and neuropsychological measurements, the normality of clinical data and neuropsychological measures was evaluated by a Kolmogorov–Smirnov test to choose parametric or non-parametric tests using SPSS v22 (Armonk, NY: IBM Corp). General linear models were performed on both GM maps and FC maps using a voxelwise comparison across the whole brain. An absolute GM threshold of 0.2 was used to avoid possible edge effects around the border between GM and WM. Mixed repeated measures analysis of variance was performed to compare the longitudinal changes between PD groups with visit-time (i.e., T0, T1, T2) as within-group factor and group (i.e., PD-pRBD+ and PD-pRBD−) as between-group factor. Post-hoc comparisons were further performed to determine longitudinal changes over time within group and differences between group at each visit time. FC results were reported based on an uncorrected voxelwise height threshold of p < 0.001 combined with an FWE-corrected clusterwise threshold of p < 0.05. Brain regions were localized based on Anatomy toolbox v2.2c (Eickhoff et al., 2005).
Regions with significant alterations in patients with PD-pRBD+ were further defined as regions of interest (ROIs). Aging is a risk factor in PD-pRBD+ (Dauvilliers et al., 2018). In this work, age was taken as a nuisance variable between group comparisons to control its confounding effect. Furthermore, to explore the relationship between FC values in these ROIs and performance of the patients on neuropsychological assessments, partial correlations were performed after adjusting for age, sex, education level, disease duration, and levodopa dose as nuisance variables of no interest.
As listed in Table 1, significantly increased disease stage, levodopa dose, and severe sleep disturbance were found in patients with PD-pRBD+ over time, as measured by Hoehn and Yahr stage (p = 0.010), levodopa equivalent daily dose (LEDD) (p = 0.002), and RBDSQ (p = 0.003), respectively. Post-hoc comparisons revealed that significantly higher Hoehn and Yahr stages (p < 0.05) and LEDD (p < 0.05) were detected at the 3-year follow-up evaluation compared with baseline in patients with PD-pRBD+. Regarding sleep behavior, significantly higher RBDSQ scores were identified at the 3-year follow-up evaluation than at both the 1-year follow-up (p < 0.05) and baseline (p < 0.05) in patients with PD-pRBD+. For patients with PD-pRBD−, a significantly higher LEDD was found over time (p < 0.001), with significantly higher LEDDs at the 1- and 3-year follow-up evaluations than at baseline (p < 0.05).
As shown in Figure 2, regarding between-group comparisons, significantly decreased general cognition measured by MoCA scores were revealed in patients with PD-pRBD+ compared with PD-pRBD−, particularly, at T0 with (26.00 ± 3.58) vs. (28.04 ± 1.95), p = 0.016, at T1 with (26.56 ± 3.18) vs. (28.18 ± 1.79), p = 0.032, and at T2 with (26.44 ± 4.12) vs. (28.39 ± 2.15), p = 0.04, respectively. In addition, in contrast to patients with PD-pRBD−, patients with PD-pRBD+ exhibited significant deficit in executive function measured by LNS scores at T0 with (10.78 ± 2.44) vs. (12.32 ± 2.14), p = 0.029; at T1 with (10.56 ± 3.01) vs. (13.04 ± 2.22), p = 0.002; and at T2 with (10.89 ± 2.59) vs. (12.61 ± 2.94), p = 0.044, respectively. Compared with patients with PD-pRBD−, the left caudate and putamen striatal binding ratios (SBRs) revealed significant decrease in patients with PD-pRBD+ at each visit (all p < 0.05). In addition, a significant decrease in the right putamen SBR was found in patients with PD-pRBD+ compared with PD-pRBD− patients at the 3-year follow-up evaluation (p < 0.05). No significant differences were detected in the remaining comparisons.
Figure 2. Significantly different neuropsychological assessments between-group comparisons. PD-pRBD+, Parkinson's disease (PD) with probable rapid eye movement (REM) sleep behavior disorder (pRBD); PD-pRBD−, PD without pRBD; T0, fMRI data at onset; T1, the 1-year follow-up evaluation; T2, the 3-year follow-up evaluation; LNS, letter-number sequencing; MoCA, Montreal cognitive assessment; ESS, Epworth sleepiness scale; RBDSQ, RBD screening questionnaire; SBR, striatal binding ratio; Lt, left; *, showed significant differences between patients with PD-pRBD+ and PD-pRBD−.
No significant crosssectional GM volume difference was detected between PD-pRBD+ and PD-pRBD− groups. Longitudinally, it was found that significantly decreased GM volume in the bilateral caudate (MNI: −9, 2, 14, F = 19.75, 365 voxels, and MNI: 11, 3, 17, F = 28.09, 466 voxels, respectively) and increased GM volume in the right thalamus (MNI: 23, −21, 6; F = 29.96, 315 voxels) were conjunctively detected in patients with PD over time (Figure 3), which was consistent with our previous findings (Jia et al., 2015a).
Figure 3. Longitudinal GM volume changes in patients with both PD-pRBD+ and PD-pRBD− over time. The results were thresholded based on a voxelwise height threshold of p < 0.05 (FWE-corrected). Mixed repeated measures analysis of variance was performed to compare the longitudinal changes between PD groups with visit-time (i.e., T0, T1, T2) as within-group factor and group (i.e., PD-pRBD+ and PD-pRBD−) as between-group factor. Post-hoc comparisons were further performed to determine longitudinal changes over time within group and differences between groups at each visit time. PD-pRBD+, Parkinson's disease (PD) with probable rapid eye movement (REM) sleep behavior disorder (pRBD); PD-pRBD−, PD without pRBD; GMV, gray matter volume; Lt, left; Rt, right. *p < 0.05; **p < 0.01; ***p < 0.001.
In this work, significantly decreased FC in the left DLPFC was detected in the right medial frontopolar area 2 (Fp2) and the bilateral inferior occipital gyri (hOc4la) in patients with PD-pRBD+ compared with patients with PD-pRBD− at baseline (Table 2, Figure 4). Furthermore, significantly decreased FC between the left DLPFC and right lateral frontopolar area (Fp1) and intraparietal sulcus (IPS) were revealed specific to patients with PD-pRBD+ compared to PD-pRBD− at the 3-year follow-up evaluation (Table 2, Figure 5). No voxels survived corrected multiple comparisons at the 1-year follow-up evaluation between groups.
Table 2. Functional connectivity alterations in the left DLPFC between patients with PD-pRBD+ and PD-pRBD− over time.
Figure 4. Decreased FC in the DLPFC in PD-pRBD+ compared to PD-pRBD− at baseline fMRI scans. (A) Decreased FC in the right Fp2; (B) Decreased FC in the bilateral hOc4la. Bar graph showed the mean extracted FC values in these regions. The results were thresholded based on an uncorrected voxelwise height threshold of p < 0.001 combined with an FWE-corrected clusterwise threshold of p < 0.05. Mixed repeated measures analysis of variance was performed to compare the longitudinal changes between PD groups with visit-time (i.e., T0, T1, T2) as within-group factor and group (i.e., PD-pRBD+ and PD-pRBD−) as between-group factor. Post-hoc comparisons were further performed to determine longitudinal changes over time within group and differences between groups at each visit time. Abbreviations: hOc41a, the anterior third of the middle and inferior lateral occipital gyri; Fp2, medial frontopolar area 2; T0, fMRI data at onset; T1, the 1-year follow-up evaluation; T2, the 3-year follow-up evaluation. PD-pRBD+, Parkinson's disease (PD) with probable rapid eye movement (REM) sleep behavior disorder (pRBD); PD-pRBD−, PD without pRBD; HC, healthy control. Lt, left; Rt, right. †showed significant differences between patients with PD-pRBD+ and PD-pRBD−; ‡showed significant differences between patients with PD-pRBD+ and HCs. *p < 0.05 with Bonferroni correction; **p < 0.005 with Bonferroni correction; ***p < 0.001 with Bonferroni correction.
Figure 5. Progressive FC decrease in the left DLPFC in PD-pRBD+ compared with PD-pRBD− at 3-year follow-up evaluation. (A) Decreased FC in the right Fp1; (B) Decreased FC in the right IPS. Bar graph showed the mean extracted FC values in these regions. Scatter plots presented the relationship between FC values and scores of LNS test. The results were thresholded based on an uncorrected voxelwise height threshold of p < 0.001 combined with an FWE-corrected clusterwise threshold of p < 0.05. Mixed repeated measures analysis of variance was performed to compare the longitudinal changes between PD groups with visit-time (i.e., T0, T1, T2) as within-group factor and group (i.e., PD-pRBD+ and PD-pRBD−) as between-group factor. Post-hoc comparisons were further performed to determine longitudinal changes over time within group and differences between groups at each visit time. Raw data for LNS and FC were adjusted for confounding variables of age, sex, education, disease duration, and levodopa dose, as demonstrated in x-axis and y-axis. Fp1, lateral frontopolar area 1; LNS, letter-number sequencing; IPS: intraparietal sulcus; T0, fMRI data at onset; T1, the 1-year follow-up evaluation; T2, the 3-year follow-up evaluation. PD-pRBD+, Parkinson's disease (PD) with probable rapid eye movement (REM) sleep behavior disorder (pRBD); PD-pRBD−, PD without pRBD; Lt, left; Rt, right. †showed significant differences between patients with PD-pRBD+ and PD-pRBD−; ‡showed significant differences between PD-pRBD+ patients and HCs. *p < 0.05 with Bonferroni correction; **p < 0.005 with Bonferroni correction; ***p < 0.001 with Bonferroni correction.
To further explore the dynamic, progressive changes, connectivity values of these regions were extracted for all patients with PD at all visits, as well as from HCs at baseline for comparison purposes. As shown in Figure 4, it was further found that extracted FC values in this altered region specific to PD-pRBD+ at baseline were also significantly decreased compared to the FC values in HCs. It was noted that patients with PD-pRBD+ exhibited significant FC recovery in the Fp2 at the 1-year follow-up evaluation and then impaired again at 3-year follow-up evaluation (Figure 4A). Similar patterns were identified in the bilateral occipital cortex (Figure 4B).
At the 3-year follow-up evaluation, in comparison with patients with PD-pRBD−, specific alterations in connectivity patterns in the right Fp1 and the IPS in PD-pRBD+ patients were also detected when compared to the FC of HCs (Figure 5). Furthermore, within the PD-pRBD+ group, patients also exhibited significantly decreased FC in the right Fp1 at the 3-year follow-up evaluation compared to that measured both at baseline and at the 1-year follow-up evaluation (Figure 5A). Correlation analysis further revealed that the connectivity between the left DLPFC and the right Fp1 positively correlated with executive function measured by the LNS test in PD-pRBD+ patients (r = 0.68, p = 0.03), whereas no significant correlation was detected in patients with PD-pRBD− (r = 0.21, p = 0.28) (Figure 5A).
Our results demonstrated that PD-pRBD+ patients exhibited specific FC dysfunction between the DLPFC and frontopolar cortex compared with the FC of patients with PD-pRBD−, which in turn was associated with a decline in executive function over time. The present findings provided new information about prefrontal cortex dysfunction specific to patients with PD with RBD during disease progression.
Indeed, RBD in patients with PD has a pervasive influence on both motor and non-motor symptoms (Pagano et al., 2018; Kim et al., 2019; Liu et al., 2020), which provides evidence for the poor prognosis for this disease. Consistently, this work found that patients with PD-pRBD+ exhibited a more rapid decline in striatal dopamine transporter binding and cognition, greater daytime sleepiness, and worse disease staging than patients with PD-pRBD− as the disease progressed.
Of note, recent studies have demonstrated the RBD increased risk of cognitive impairment (Boot et al., 2012; Postuma et al., 2019). A recent study has reported no significant differences in cognitive performance between patients with PD-pRBD+ and PD-pRBD−, whereas cognitive decline is associated with sleep efficiency (Hermann et al., 2020). In this work, we found that patients with PD-pRBD+ exhibited a more rapid decline in cognition. One possibility was that lower score at cognitive assessments tests might be caused by sleep disorders, regardless of the presence of PD. However, no significant correlation was detected between cognitive performances and sleep behaviors (RBDSQ score and ESS) in patients with PD-pRBD+, which might further eliminate this potential possibility (Table 3). Furthermore, some studies have also reported no significant differences in cognitive performances between patients with PD with and without sleep disorders (Bugalho et al., 2011; Sixel-Döring et al., 2014). The heterogeneity of patients with PD, different measurements of sleep behaviors, and different sample sizes among studies may account for these inconsistencies.
Table 3. Correlations between cognitive performance and sleep performance in patients with PD-pRBD+ by combining visit times.
Functional neuroimaging studies have demonstrated the essential role of the DLPFC in executive function (Collette and Van der Linden, 2002; Yang et al., 2009; Jia et al., 2011, 2015b; Liang et al., 2014, 2016). RBD in PD affects underlying higher-level cognitive processes, such as executive function measured by LNS tasks (Lerche and Brockmann, 2018; Hanuška et al., 2019). Accordingly, a recent study has also demonstrated decreased brain activity in the DLPFC in PD-RBD+ (Verstraeten and Cluydts, 2004; Ehgoetz Martens et al., 2020).
Importantly, the frontopolar cortex is involved in the most abstract level of executive control for information integration to coordinate dorsolateral functions, subsequently optimizing task performance (Burgess et al., 2000; Fletcher and Henson, 2001; Rogers et al., 2004). In this work, disrupted connectivity between the DLPFC frontopolar cortex was detected specifically in patients with PD-pRBD+ compared with PD-pRBD−. More specifically, altered connectivity in the Fp2 exhibited an inverse U-shape during the disease progression in patients with PD-pRBD+. Actually, increased brain activity in frontal cortex in PD-pRBD+ has been reported (Li et al., 2017), possibly due to the upregulated frontal dopamine level to compensate the low dopamine levels in the striatum (Cools et al., 2010). In line with this view, in this work, significant decrease of striatal binding ratios has been identified in PD-pRBD+. On this basis, the increased brain activity in prefrontal cortex might reflect compensation for basal ganglia dysfunction at 1-year follow-up in patients with PD-pRBD+, whereas, the substantial increase of the LEDD and decrease of striatal dopamine at 3-year follow-up in patients with PD-pRBD+ might suggest the loss of compensation during disease progression (Vijayraghavan et al., 2007; Williams-Gray et al., 2007). Of note, alteration in the medial part of frontal pole (Fp2) precedes the lateral area (Fp1) that was observed only at the 3-year follow-up assessment, whereas in the PD-pRBD− group, there is no significant connectivity alteration between the DLPFC and frontopolar cortex over time. Furthermore, correlation analysis suggested that the underlying impaired FC may be responsible for the deficits in executive functions in this disease. Alternatively, it has been argued that dysconnectivity in the frontopolar cortex may be not due to sleep disturbances but to dopaminergic denervation during disease progression, as suggested previously (Kim et al., 2020). However, this possibility was eliminated to some extent, as the results remained after adjusting for the levodopa dose and striatal binding ratios.
Besides, we found that patients with PD-pRBD+ exhibited significantly disrupted connectivity between the left DLPFC and right IPS at the 3-year follow-up evaluation. In particular, the IPS plays an important role in executive function such as mental representation and spatial processing (Husain and Nachev, 2007; Gillebert et al., 2011). Note that the frontal–intraparietal network is involved in the development of visuo-spatial working memory (Klingberg, 2006; Jia et al., 2015b); thus the activation of IPS is often associated with simultaneous activity in the dorsolateral frontal lobe (Corbetta and Shulman, 2002; Husain and Rorden, 2003). Moreover, sleep deprivation study has reported that fronto–parietal activation decreases in response to a working memory task (Lythe et al., 2012), which supports the disrupted connectivity between the left DLPFC and right IPS in patients with PD-pRBD+. Meanwhile, an increasing number of studies have shown that patients with PD have a high risk of visual hallucination (Stephenson et al., 2010) and patients with RBD are also characterized by the presence of impairment in visuospatial ability (Plomhause et al., 2014). Recent data suggest that dorsal pathway into the parietal cortex and prefrontal cortex involved with visually guided movement and attention control (Gilbert and Li, 2013) is in line with the disrupted connectivity between the left DLPFC and the bilateral occipital cortex in patients with PD-pRBD+ at baseline.
In addition, it is noteworthy that thalamostriatal abnormalities in patients with PD have been reported in our recent studies, both structurally (Jia et al., 2015a) and functionally (Jia et al., 2018, 2020). Consistently, GMV alterations associated with patients with PD over time were also identified in the caudate and thalamus. However, no significant regional brain volume differences were identified between the two PD groups in line with a previous study (García-Lorenzo et al., 2013). On this basis, the present findings of disrupted prefrontal cortex functional connectivity were less likely confounded by the structural changes in PD.
Although our study was strengthened by longitudinal data, several methodological limitations in this work should be acknowledged. The sample size of 46 patients with PD (i.e., 18 PD-pRBD+ and 28 PD-pRBD−) in this work approximately meets the requirement of 20 samples, as recommended previously for reliability of results based on fMRI (Thirion et al., 2007). In addition, the current results were reported with a strict threshold corrected for multiple comparisons as recommended by Woo et al. (2014) to reduce false positives, and we are reasonably confident about the observed significant effects. However, no voxel survived corrected multiple comparisons at the 1-year follow-up evaluation between groups, suggesting that alterations with small effect size requires a relatively large sample size. Meanwhile, although the RBDSQ has been validated to screen for RBD in patients with PD, the lack of polysomnography may limit the extension of the current findings. Furthermore, interaction analysis including HCs would help clarify more specific changes associated with RBD in patients with PD to rule out the effect of normal aging over time. Thus, further studies are required to carry out more objective measures of RBD and include longitudinal fMRI data of HCs.
In conclusion, this longitudinal work explored the neurobiological mechanisms associated with sleep disturbances in patients with PD during disease progression. Our results suggest that progressive prefrontal cortex dysfunction in early PD with RBD might be an effective subtype-specific biomarker of the neurodegenerative progression, which further advances the present understanding of cortical dysfunction and sheds light on the neuropathological mechanisms and new therapeutic strategy associated with RBD in this disease.
Data used in the preparation of this article were obtained from PPMI database (www.ppmi-info.org/data). The original contributions presented in the study are included in the article/supplementary material, further inquiries can be directed to the corresponding authors.
The study was approved by the institutional review board at each PPMI site. The patients/participants provided their written informed consent to participate in this study. Written informed consent was obtained from the individual(s) for the publication of any potentially identifiable images or data included in this article.
XJ, JW, and QY contributed to design the study. XJ, WF, ZW, YLiu, YLi, and HaL contributed to acquire and analyze the data. XJ, WF, ZW, YLi, HuL, TM, JW, and QY contributed to interpret the findings and draft the manuscript. XJ, TM, JW, and QY contributed to revise the manuscript. All authors contributed to the article and approved the submitted version.
This work was supported by grants from the National Natural Science Foundation of China (62076169), Beijing Hospitals Authority Youth Program (QML20200304), and Basic research foundation of Shenzhen Science and Technology Stable Support Plan (GXWD20201230155427003-20200822115709001).
The authors declare that the research was conducted in the absence of any commercial or financial relationships that could be construed as a potential conflict of interest.
All claims expressed in this article are solely those of the authors and do not necessarily represent those of their affiliated organizations, or those of the publisher, the editors and the reviewers. Any product that may be evaluated in this article, or claim that may be made by its manufacturer, is not guaranteed or endorsed by the publisher.
For up-to-date information on the study, visit (www.ppmi-info.org). PPMI—a public–private partnership—is funded by the Michael J Fox Foundation for Parkinson's Research and funding partners, including Abbvie, Avid Radiopharmaceuticals, Biogen, Bristol-Myers Squibb, Covance, GE Healthcare, Genetech, GlaxosmithKline, Lilly, Lundbeck, Merck, Meso Scale Discovery, Pfizer, Piramal, Roche, Servier, and UCB.
Behzadi, Y., Restom, K., Liau, J., and Liu, T. T. (2007). A component based noise correction method (CompCor) for BOLD and perfusion based fMRI. Neuroimage 37, 90–101. doi: 10.1016/j.neuroimage.2007.04.042
Boot, B. P., Boeve, B. F., Roberts, R. O., Ferman, T. J., Geda, Y. E., Pankratz, V. S., et al. (2012). Probable rapid eye movement sleep behavior disorder increases risk for mild cognitive impairment and Parkinson disease: a population-based study. Ann. Neurol. 71, 49–56. doi: 10.1002/ana.22655
Bugalho, P., da Silva, J. A., and Neto, B. (2011). Clinical features associated with REM sleep behavior disorder symptoms in the early stages of Parkinson's disease. J. Neurol. 258, 50–55. doi: 10.1007/s00415-010-5679-0
Burgess, P. W., Veitch, E., de Lacy Costello, A., and Shallice, T. (2000). The cognitive and neuroanatomical correlates of multitasking. Neuropsychologia 38, 848–863. doi: 10.1016/S0028-3932(99)00134-7
Collette, F., and Van der Linden, M. (2002). Brain imaging of the central executive component of working memory. Neurosci. Biobehav. Rev. 26, 105–125. doi: 10.1016/S0149-7634(01)00063-X
Cools, R., Miyakawa, A., Sheridan, M., and D'Esposito, M. (2010). Enhanced frontal function in Parkinson's disease. Brain 133, 225–233. doi: 10.1093/brain/awp301
Corbetta, M., and Shulman, G. L. (2002). Control of goal-directed and stimulus-driven attention in the brain. Nat. Rev. Neurosci. 3, 201–215. doi: 10.1038/nrn755
Dauvilliers, Y., Schenck, C. H., Postuma, R. B., Iranzo, A., Luppi, P. H., Plazzi, G., et al. (2018). REM sleep behaviour disorder. Nat. Rev. Dis. Primers 4:19. doi: 10.1038/s41572-018-0016-5
Doruk, D., Gray, Z., Bravo, G. L., Pascual-Leone, A., and Fregni, F. (2014). Effects of tDCS on executive function in Parkinson's disease. Neurosci. Lett. 582, 27–31. doi: 10.1016/j.neulet.2014.08.043
Ehgoetz Martens, K. A., Matar, E., Shine, J. M., Phillips, J. R., Georgiades, M. J., Grunstein, R. R., et al. (2020). The neural signature of impaired dual-tasking in idiopathic rapid eye movement sleep behavior disorder patients. Mov. Disord. 35, 1596–1606. doi: 10.1002/mds.28114
Eickhoff, S. B., Stephan, K. E., Mohlberg, H., Grefkes, C., Fink, G. R., Amunts, K., et al. (2005). A new SPM toolbox for combining probabilistic cytoarchitectonic maps and functional imaging data. Neuroimage 25, 1325–1335. doi: 10.1016/j.neuroimage.2004.12.034
Fantini, M. L., Figorilli, M., Arnulf, I., Zibetti, M., Pereira, B., Beudin, P., et al. (2018). Sleep and REM sleep behaviour disorder in Parkinson's disease with impulse control disorder. J. Neurol. Neurosurg. Psychiatry 89, 305–310. doi: 10.1136/jnnp-2017-316576
Fereshtehnejad, S. M., Zeighami, Y., Dagher, A., and Postuma, R. B. (2017). Clinical criteria for subtyping Parkinson's disease: biomarkers and longitudinal progression. Brain 140, 1959–1976. doi: 10.1093/brain/awx118
Figorilli, M., Marques, A. R., Vidal, T., Delaby, L., Meloni, M., Pereira, B., et al. (2020). Does REM sleep behavior disorder change in the progression of Parkinson's disease? Sleep Med. 68, 190–198. doi: 10.1016/j.sleep.2019.12.013
Fletcher, P. C., and Henson, R. N. (2001). Frontal lobes and human memory: insights from functional neuroimaging. Brain 124, 849–881. doi: 10.1093/brain/124.5.849
García-Lorenzo, D., Longo-Dos Santos, C., Ewenczyk, C., Leu-Semenescu, S., Gallea, C., Quattrocchi, G., et al. (2013). The coeruleus/subcoeruleus complex in rapid eye movement sleep behaviour disorders in Parkinson's disease. Brain 136, 2120–2129. doi: 10.1093/brain/awt152
Gilbert, C. D., and Li, W. (2013). Top-down influences on visual processing. Nat. Rev. Neurosci. 14, 350–363. doi: 10.1038/nrn3476
Gillebert, C. R., Mantini, D., Thijs, V., Sunaert, S., Dupont, P., and Vandenberghe, R. (2011). Lesion evidence for the critical role of the intraparietal sulcus in spatial attention. Brain 134, 1694–1709. doi: 10.1093/brain/awr085
Hanuška, J., Rusz, J., Bezdicek, O., Ulmanov,á, O., Bonnet, C., Dušek, P., et al. (2019). Eye movements in idiopathic rapid eye movement sleep behaviour disorder: high antisaccade error rate reflects prefrontal cortex dysfunction. J. Sleep Res. 28:e12742. doi: 10.1111/jsr.12742
Hermann, W., Schmitz-Peiffer, H., Kasper, E., Fauser, M., Franke, C., Wienecke, M., et al. (2020). Sleep disturbances and sleep disordered breathing impair cognitive performance in Parkinson's disease. Front. Neurosci. 14:689. doi: 10.3389/fnins.2020.00689
Husain, M., and Nachev, P. (2007). Space and the parietal cortex. Trends Cogn. Sci. 11, 30–36. doi: 10.1016/j.tics.2006.10.011
Husain, M., and Rorden, C. (2003). Non-spatially lateralized mechanisms in hemispatial neglect. Nat. Rev. Neurosci. 4, 26–36. doi: 10.1038/nrn1005
Ioannides, A. A., Kostopoulos, G. K., Liu, L., and Fenwick, P. B. (2009). MEG identifies dorsal medial brain activations during sleep. Neuroimage 44, 455–468. doi: 10.1016/j.neuroimage.2008.09.030
Jia, X., Li, Y., Li, K., Liang, P., and Fu, X. (2018). Precuneus dysfunction in Parkinson's disease with mild cognitive impairment. Front. Aging Neurosci. 10:427. doi: 10.3389/fnagi.2018.00427
Jia, X., Liang, P., Li, Y., Shi, L., Wang, D., and Li, K. (2015a). Longitudinal study of gray matter changes in Parkinson disease. AJNR Am. J. Neuroradiol. 36, 2219–2226. doi: 10.3174/ajnr.A4447
Jia, X., Liang, P., Lu, J., Yang, Y., Zhong, N., and Li, K. (2011). Common and dissociable neural correlates associated with component processes of inductive reasoning. Neuroimage 56, 2292–2299. doi: 10.1016/j.neuroimage.2011.03.020
Jia, X., Liang, P., Shi, L., Wang, D., and Li, K. (2015b). Prefrontal and parietal activity is modulated by the rule complexity of inductive reasoning and can be predicted by a cognitive model. Neuropsychologia 66, 67–74. doi: 10.1016/j.neuropsychologia.2014.10.015
Jia, X., Pan, Z., Chen, H., Wang, Z., Li, K., Wang, X., et al. (2020). Differential functional dysconnectivity of caudate nucleus subdivisions in Parkinson's disease. Aging 12, 16183–16194. doi: 10.18632/aging.103628
Jozwiak, N., Postuma, R. B., Montplaisir, J., Latreille, V., Panisset, M., Chouinard, S., et al. (2017). REM sleep behavior disorder and cognitive impairment in Parkinson's disease. Sleep 40:zsx101. doi: 10.1093/sleep/zsx101
Kamble, N., Yadav, R., Lenka, A., Kumar, K., Nagaraju, B. C., and Pal, P. K. (2019). Impaired sleep quality and cognition in patients of Parkinson's disease with REM sleep behavior disorder: a comparative study. Sleep Med. 62, 1–5. doi: 10.1016/j.sleep.2019.04.001
Kim, R., Yoo, D., Im, J. H., Kim, H. J., and Jeon, B. (2019). REM sleep behavior disorder predicts functional dependency in early Parkinson's disease. Parkinsonism Relat. Disord. 66, 138–142. doi: 10.1016/j.parkreldis.2019.07.025
Kim, Y. E., and Jeon, B. S. (2014). Clinical implication of REM sleep behavior disorder in Parkinson's disease. J. Parkinsons. Dis. 4, 237–244. doi: 10.3233/JPD-130293
Kim, Y. E., Kim, Y. J., Hwang, H. S., and Ma, H. I. (2020). REM sleep behavior disorder in early Parkinson's disease predicts the rapid dopaminergic denervation. Parkinsonism Relat. Disord. 80, 120–126. doi: 10.1016/j.parkreldis.2020.09.032
Klingberg, T. (2006). Development of a superior frontal-intraparietal network for visuo-spatial working memory. Neuropsychologia 44, 2171–2177. doi: 10.1016/j.neuropsychologia.2005.11.019
Lerche, S., and Brockmann, K. (2018). REM sleep behaviour disorder (RBD): risk for Parkinsonism and executive dysfunction in elderly. Oncotarget 9, 36732–36733. doi: 10.18632/oncotarget.26417
Li, D., Huang, P., Zang, Y., Lou, Y., Cen, Z., Gu, Q., et al. (2017). Abnormal baseline brain activity in Parkinson's disease with and without REM sleep behavior disorder: a resting-state functional MRI study. J. Magn. Reson. Imaging 46, 697–703. doi: 10.1002/jmri.25571
Liang, P., Jia, X., Taatgen, N. A., Borst, J. P., and Li, K. (2016). Activity in the fronto-parietal network indicates numerical inductive reasoning beyond calculation: an fMRI study combined with a cognitive model. Sci. Rep. 6:25976. doi: 10.1038/srep25976
Liang, P., Jia, X., Taatgen, N. A., Zhong, N., and Li, K. (2014). Different strategies in solving series completion inductive reasoning problems: an fMRI and computational study. Int. J. Psychophysiol. 93, 253–260. doi: 10.1016/j.ijpsycho.2014.05.006
Liu, R., Umbach, D. M., Tröster, A. I., Huang, X., and Chen, H. (2020). Non-motor symptoms and striatal dopamine transporter binding in early Parkinson's disease. Parkinsonism Relat. Disord. 72, 23–30. doi: 10.1016/j.parkreldis.2020.02.001
Lowe, M. J., Mock, B. J., and Sorenson, J. A. (1998). Functional connectivity in single and multislice echoplanar imaging using resting-state fluctuations. Neuroimage 7, 119–132. doi: 10.1006/nimg.1997.0315
Lythe, K. E., Williams, S. C., Anderson, C., Libri, V., and Mehta, M. A. (2012). Frontal and parietal activity after sleep deprivation is dependent on task difficulty and can be predicted by the fMRI response after normal sleep. Behav. Brain Res. 233, 62–70. doi: 10.1016/j.bbr.2012.04.050
Mahajan, A., Rosenthal, L. S., Gamaldo, C., Salas, R. E., Pontone, G. M., McCoy, A., et al. (2014). REM sleep behavior and motor findings in Parkinson's disease: a cross-sectional analysis. Tremor. Other Hyperkinet. Mov. 4:245. doi: 10.5334/tohm.184
Muzur, A., Pace-Schott, E. F., and Hobson, J. A. (2002). The prefrontal cortex in sleep. Trends Cogn. Sci. 6, 475–481. doi: 10.1016/S1364-6613(02)01992-7
Pagano, G., De Micco, R., Yousaf, T., Wilson, H., Chandra, A., and Politis, M. (2018). REM behavior disorder predicts motor progression and cognitive decline in Parkinson disease. Neurology 91, e894–e905. doi: 10.1212/WNL.0000000000006134
Panikratova, Y. R., Vlasova, R. M., Akhutina, T. V., Korneev, A. A., Sinitsyn, V. E., and Pechenkova, E. V. (2020). Functional connectivity of the dorsolateral prefrontal cortex contributes to different components of executive functions. Int. J. Psychophysiol. 151, 70–79. doi: 10.1016/j.ijpsycho.2020.02.013
Perlstein, W. M., Carter, C. S., Noll, D. C., and Cohen, J. D. (2001). Relation of prefrontal cortex dysfunction to working memory and symptoms in schizophrenia. Am. J. Psychiatry 158, 1105–1113. doi: 10.1176/appi.ajp.158.7.1105
Plomhause, L., Dujardin, K., Boucart, M., Herlin, V., Defebvre, L., Derambure, P., et al. (2014). Impaired visual perception in rapid eye movement sleep behavior disorder. Neuropsychology 28, 388–393. doi: 10.1037/neu0000006
Postuma, R. B., Iranzo, A., Hu, M., Högl, B., Boeve, B. F., Manni, R., et al. (2019). Risk and predictors of dementia and parkinsonism in idiopathic REM sleep behaviour disorder: a multicentre study. Brain 142, 744–759. doi: 10.1093/brain/awz030
Rogers, M. A., Kasai, K., Koji, M., Fukuda, R., Iwanami, A., Nakagome, K., et al. (2004). Executive and prefrontal dysfunction in unipolar depression: a review of neuropsychological and imaging evidence. Neurosci. Res. 50, 1–11. doi: 10.1016/j.neures.2004.05.003
Sixel-Döring, F., Trautmann, E., Mollenhauer, B., and Trenkwalder, C. (2014). Rapid eye movement sleep behavioral events: a new marker for neurodegeneration in early Parkinson disease? Sleep 37, 431–438. doi: 10.5665/sleep.3468
Sixel-Döring, F., Zimmermann, J., Wegener, A., Mollenhauer, B., and Trenkwalder, C. (2016). The evolution of REM sleep behavior disorder in early Parkinson disease. Sleep 39, 1737–1742. doi: 10.5665/sleep.6102
Stephenson, R., Houghton, D., Sundarararjan, S., Doty, R. L., Stern, M., Xie, S. X., et al. (2010). Odor identification deficits are associated with increased risk of neuropsychiatric complications in patients with Parkinson's disease. Mov. Disord. 25, 2099–2104. doi: 10.1002/mds.23234
Thirion, B., Pinel, P., Mériaux, S., Roche, A., Dehaene, S., and Poline, J. B. (2007). Analysis of a large fMRI cohort: Statistical and methodological issues for group analyses. Neuroimage 35, 105–120. doi: 10.1016/j.neuroimage.2006.11.054
Vendette, M., Gagnon, J. F., Décary, A., Massicotte-Marquez, J., Postuma, R. B., Doyon, J., et al. (2007). REM sleep behavior disorder predicts cognitive impairment in Parkinson disease without dementia. Neurology 69, 1843–1849. doi: 10.1212/01.wnl.0000278114.14096.74
Verstraeten, E., and Cluydts, R. (2004). Executive control of attention in sleep apnea patients: theoretical concepts and methodological considerations. Sleep Med. Rev. 8, 257–267. doi: 10.1016/j.smrv.2004.01.001
Vijayraghavan, S., Wang, M., Birnbaum, S. G., Williams, G. V., and Arnsten, A. F. (2007). Inverted-U dopamine D1 receptor actions on prefrontal neurons engaged in working memory. Nat. Neurosci. 10, 376–384. doi: 10.1038/nn1846
Whitfield-Gabrieli, S., and Nieto-Castanon, A. (2012). Conn: a functional connectivity toolbox for correlated and anticorrelated brain networks. Brain Connect. 2, 125–141. doi: 10.1089/brain.2012.0073
Williams-Gray, C. H., Hampshire, A., Robbins, T. W., Owen, A. M., and Barker, R. A. (2007). Catechol O-methyltransferase Val158Met genotype influences frontoparietal activity during planning in patients with Parkinson's disease. J. Neurosci. 27, 4832–4838. doi: 10.1523/JNEUROSCI.0774-07.2007
Woo, C. W., Krishnan, A., and Wager, T. D. (2014). Cluster-extent based thresholding in fMRI analyses: pitfalls and recommendations. Neuroimage 91, 412–441 doi: 10.1016/j.neuroimage.2013.12.058
Keywords: frontopolar cortex, dorsolateral prefrontal cortex, RBD, functional connectivity, Parkinson's disease
Citation: Jia X, Fan W, Wang Z, Liu Y, Li Y, Li H, Li H, Ma T, Wang J and Yang Q (2022) Progressive Prefrontal Cortex Dysfunction in Parkinson's Disease With Probable REM Sleep Behavior Disorder: A 3-Year Longitudinal Study. Front. Aging Neurosci. 13:750767. doi: 10.3389/fnagi.2021.750767
Received: 31 July 2021; Accepted: 19 November 2021;
Published: 10 January 2022.
Edited by:
Rubem C. A. Guedes, Federal University of Pernambuco, BrazilReviewed by:
Hui Lu, George Washington University, United StatesCopyright © 2022 Jia, Fan, Wang, Liu, Li, Li, Li, Ma, Wang and Yang. This is an open-access article distributed under the terms of the Creative Commons Attribution License (CC BY). The use, distribution or reproduction in other forums is permitted, provided the original author(s) and the copyright owner(s) are credited and that the original publication in this journal is cited, in accordance with accepted academic practice. No use, distribution or reproduction is permitted which does not comply with these terms.
*Correspondence: Jing Wang, a3ljNjYzNkAxMjYuY29t; Qi Yang, eWFuZ3lhbmdxaXFpQGdtYWlsLmNvbQ==
†These authors have contributed equally to this work and share first authorship
Disclaimer: All claims expressed in this article are solely those of the authors and do not necessarily represent those of their affiliated organizations, or those of the publisher, the editors and the reviewers. Any product that may be evaluated in this article or claim that may be made by its manufacturer is not guaranteed or endorsed by the publisher.
Research integrity at Frontiers
Learn more about the work of our research integrity team to safeguard the quality of each article we publish.