- 1Centre of Excellence for Alzheimer’s Disease Research & Care, School of Medical and Health Sciences, Edith Cowan University, Joondalup, WA, Australia
- 2Department of Biomedical Sciences, Macquarie University, Sydney, NSW, Australia
- 3Centre for Healthy Ageing, Health Future Institute, Murdoch University, Murdoch, WA, Australia
- 4School of Molecular and Life Sciences, Faculty of Science and Engineering, Curtin University, Perth, WA, Australia
- 5Ingredients by Design Pty Ltd., Lesmurdie, WA, Australia
- 6CWEK Pty Ltd., Perth, WA, Australia
Alzheimer’s disease (AD) is characterized by the excessive deposition of extracellular amyloid-beta peptide (Aβ) and the build-up of intracellular neurofibrillary tangles containing hyperphosphorylated tau proteins. This leads to neuronal damage, cell death and consequently results in memory and learning impairments leading to dementia. Although the exact cause of AD is not yet clear, numerous studies indicate that oxidative stress, inflammation, and mitochondrial dysfunction significantly contribute to its onset and progression. There is no effective therapeutic approach to stop the progression of AD and its associated symptoms. Thus, early intervention, preferably, pre-clinically when the brain is not significantly affected, is a better option for effective treatment. Natural polyphenols (PP) target multiple AD-related pathways such as protecting the brain from Aβ and tau neurotoxicity, ameliorating oxidative damage and mitochondrial dysfunction. Among natural products, the cereal crop sorghum has some unique features. It is one of the major global grain crops but in the developed world, it is primarily used as feed for farm animals. A broad range of PP, including phenolic acids, flavonoids, and condensed tannins are present in sorghum grain including some classes such as proanthocyanidins that are rarely found in others plants. Pigmented varieties of sorghum have the highest polyphenolic content and antioxidant activity which potentially makes their consumption beneficial for human health through different pathways such as oxidative stress reduction and thus the prevention and treatment of neurodegenerative diseases. This review summarizes the potential of sorghum PP to beneficially affect the neuropathology of AD.
Introduction
Alzheimer’s disease (AD) is a progressive neurodegenerative disease characterized by different neuropathological features including excessive accumulation of Aβ peptides outside of neurons and the hyperphosphorylated form of tau protein inside neurons (Duyckaerts et al., 2009). Currently, more than 50 million people worldwide and more than 440,000 Australians are living with AD (Dementia Australia, 2018a; WHO, 2021). The number of affected people worldwide is expected to reach 152 million by 2050. According to the Australian Bureau of Statistics in 2017, AD was the first leading cause of mortality in Australian women and second leading cause of mortality in all Australians (Australian Bureau of Statistics, 2017). The estimated cost of dementia in Australia was more than $15 billion in 2018 and it will be more than $18.7 billion by 2025, and more than $36.8 billion by 2050 (The National Centre for Social and Economic Modelling NATSEM, 2016).
Despite advances in medicine and drug therapies, a disease-modifying treatment of AD is still not available. Recently, the new drug (Aduhelm) has been approved by the FDA as a modulator of amyloid plaques in the brain but its efficacy on memory and cognition is yet to be determined (Alexander et al., 2021; Canady, 2021). Current drug interventions only temporarily delay the progression of some of the cognitive symptoms of AD. Acetylcholine is a major neurotransmitter in the brain which has limited reserves in the AD brain where the enzymes cholinesterase breaks down the beneficial acetylcholine. Thus, cholinesterase inhibitors including acetylcholinesterase and butyrylcholinesterase inhibitors (AChEI and BChEI) help to attenuate the AD symptoms. The U.S. Food and Drug Administration (FDA) has approved five symptomatic drugs for the treatment of AD, including three cholinesterase inhibitors (rivastigmine, galantamine, donepezil) and memantine and memantine mixed with donepezil (Alzheimer’s Association, 2019). These treatments reduce the rate of progression of cognitive decline by increasing the neurotransmitters in the brain, but they are unable to prevent irreversible damage to neurons (Sivaraman et al., 2019). Therefore, there is a serious need to find a reliable intervention that can prevent or slow AD progression.
Natural sources of antioxidants have been identified as a promising preventive or therapeutic avenue for neuroprotection. Polyphenols (PP) are the most abundant antioxidants in the diet (Kulshreshtha and Piplani, 2016). Diets rich in PP are considered neuroprotective due to their capacity to affect several cellular pathways, that contribute to the pathogenesis of AD, will be discussed below (Malar and Devi, 2014; Lakey-Beitia et al., 2015; Omar et al., 2017).
Sorghum grain is a natural source of antioxidants with strong anti-inflammatory activities (Burdette et al., 2010; Xiong et al., 2019). The profile of PP of sorghum is unique and epidemiological evidence have demonstrated these PP may have specific health benefits such as superior chemoprotective properties and strong anti-inflammatory activity which are not provided by PP in other grains, such as rice, oats, and wheat (Awika, 2011). In addition, several PP of sorghum are thought to beneficially interfere with pathological changes in AD, such as Aβ and tau accumulation in in vitro and in vivo level (Rossi et al., 2008; Jabir et al., 2018) However, research is yet to identify the effect of sorghum PP on AD pathology.
This review will focus on the current evidence and potential mechanisms for protective effects of sorghum PP on the pathology of AD.
Alzheimer’s Disease and Associated Pathological Hallmarks
Dementia is a group of disorders characterized by progressive cognitive impairment which affects daily living activities (Roman, 2002). Dementia is considered as one of the most serious health and social concerns of the century. It has major impacts on individuals, carers, families, and societies.
Alzheimer’s disease is the most prevalent form of dementia (Puglielli et al., 2003) with the clinical symptoms of progressive memory decline and other cognitive functions, eventually leading to an inability to do daily tasks and a reliance on care (Long and Holtzman, 2019). It is pathologically characterized by the accumulation of extracellular Aβ oligomers, hyper-phosphorylation of intracellular neurofibrillary tangles (NFTs; tau protein) and neuroinflammation in the brain (Sadhukhan et al., 2018). Other primary changes of AD include increased oxidative stress, mitochondrial dysfunction, and neuroinflammation (Mecocci et al., 2018). A diagram of the generally accepted hypothesis for AD is shown in Figure 1.
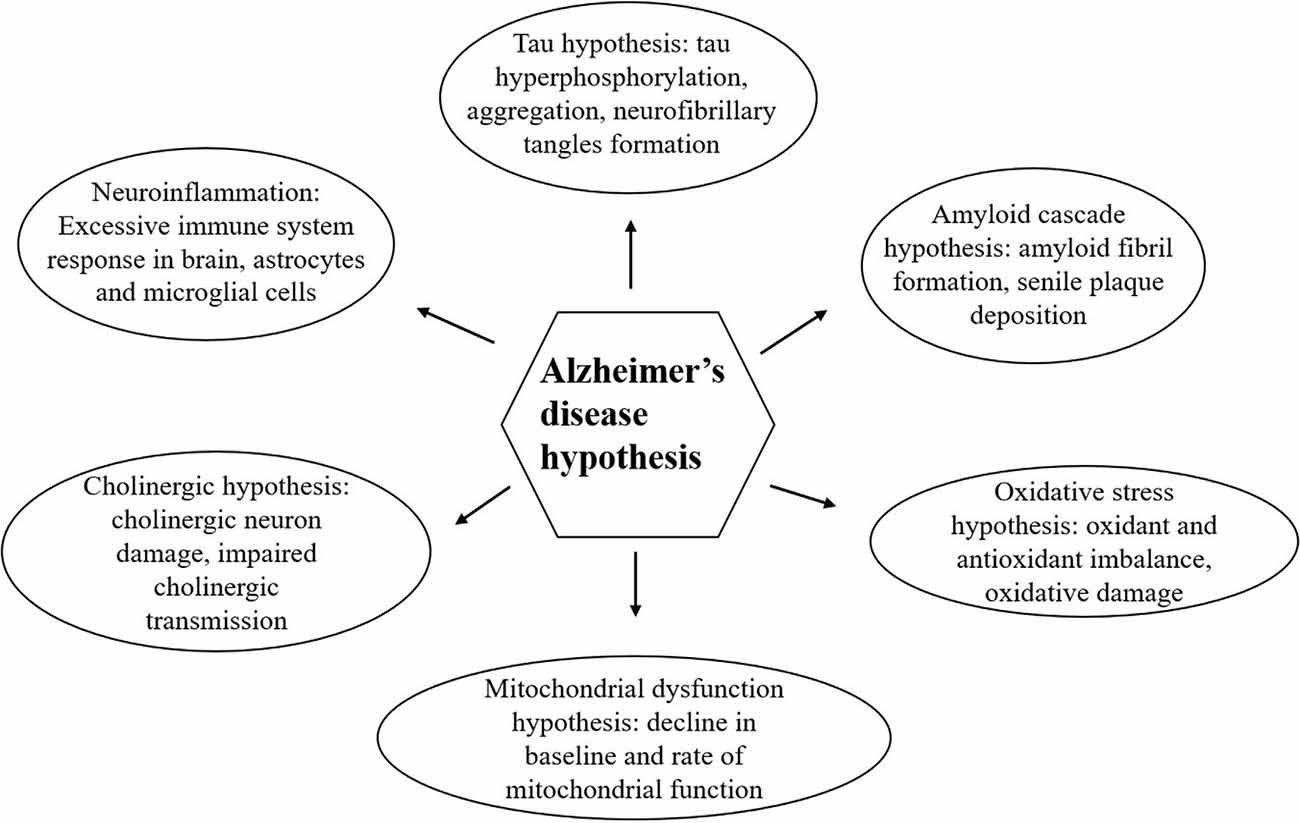
Figure 1. Alzheimer’s disease (AD) hypotheses (Swerdlow, 2018; Altinoglu and Adali, 2020). The six most common AD hypotheses include the amyloid cascade hypothesis, tau hypothesis, neuroinflammation, cholinergic hypothesis, mitochondrial dysfunction hypothesis, and oxidative stress hypothesis which are explained separately through the article. All the these mechanisms can interact with each other.
Aβ Accumulation
According to the amyloid hypothesis (Hardy and Higgins, 1992), the primary cause of AD is the accumulation and deposition of oligomeric or fibrillar Aβ peptides. The Aβ peptide consists of 38–42 amino acids that are derived from amyloid precursor protein (APP); a transmembrane protein that has two competing pathways. In the non-amyloidogenic pathway, it is cleaved by α-secretase, to produce the secretory fragment sAPPα (see Figure 2). The candidate enzymes for α-secretase which are from the a-disintegrin and metalloprotease (ADAM) family include ADAM17, ADAM9, and ADAM10 (Buxbaum et al., 1998; Lammich et al., 1999; Fahrenholz et al., 2000; Asai et al., 2003). Among these enzymes, ADAM10 is suggested as the most physiologically relevant α-secretase in neurons (Anders et al., 2001; Kuhn et al., 2010). In the amyloidogenic pathway, APP is initially cleaved by β-secretase (BACE1), then γ-secretase, resulting in the generation of Aβ peptides (Gandy et al., 1994). The non-amyloidogenic pathway is beneficial since sAPPα has neuroprotective activity (Corrigan et al., 2011). In contrast, in the amyloidogenic pathway, an over-production of Aβ and its accumulation results in cytotoxicity (Chasseigneaux and Allinquant, 2012; Paroni et al., 2019). The length of the Aβ peptide influences this toxicity, where Aβ42 (42 amino acids) is more cytotoxic than Aβ40 and Aβ43 (Fu et al., 2017). This is because Aβ42 has a higher hydrophobicity and thus higher propensity to aggregate by hydrophobic bonding into toxic oligomers compared with Aβ40 peptide (Vion et al., 2018). Several inherited and environmental factors such as APP, presenilin 1 (PSEN1), and presenilin 2 (PSEN2), gene mutations, deficit Aβ clearance, oxidative stress and mitochondrial dysfunction might be contributing factors to the over-production and accumulation of Aβ (Mao and Reddy, 2011; Hernández-Zimbrón and Rivas-Arancibia, 2015; Zuo et al., 2015; Paroni et al., 2019). Although amyloid deposition is always seen in AD patients, its pathogenic role is still unclear (Modrego and Lobo, 2019). While many questions still remain unanswered regarding the pathogenesis of AD, the amyloid hypothesis is still the most accepted theory to describe the associated neuropathological events.
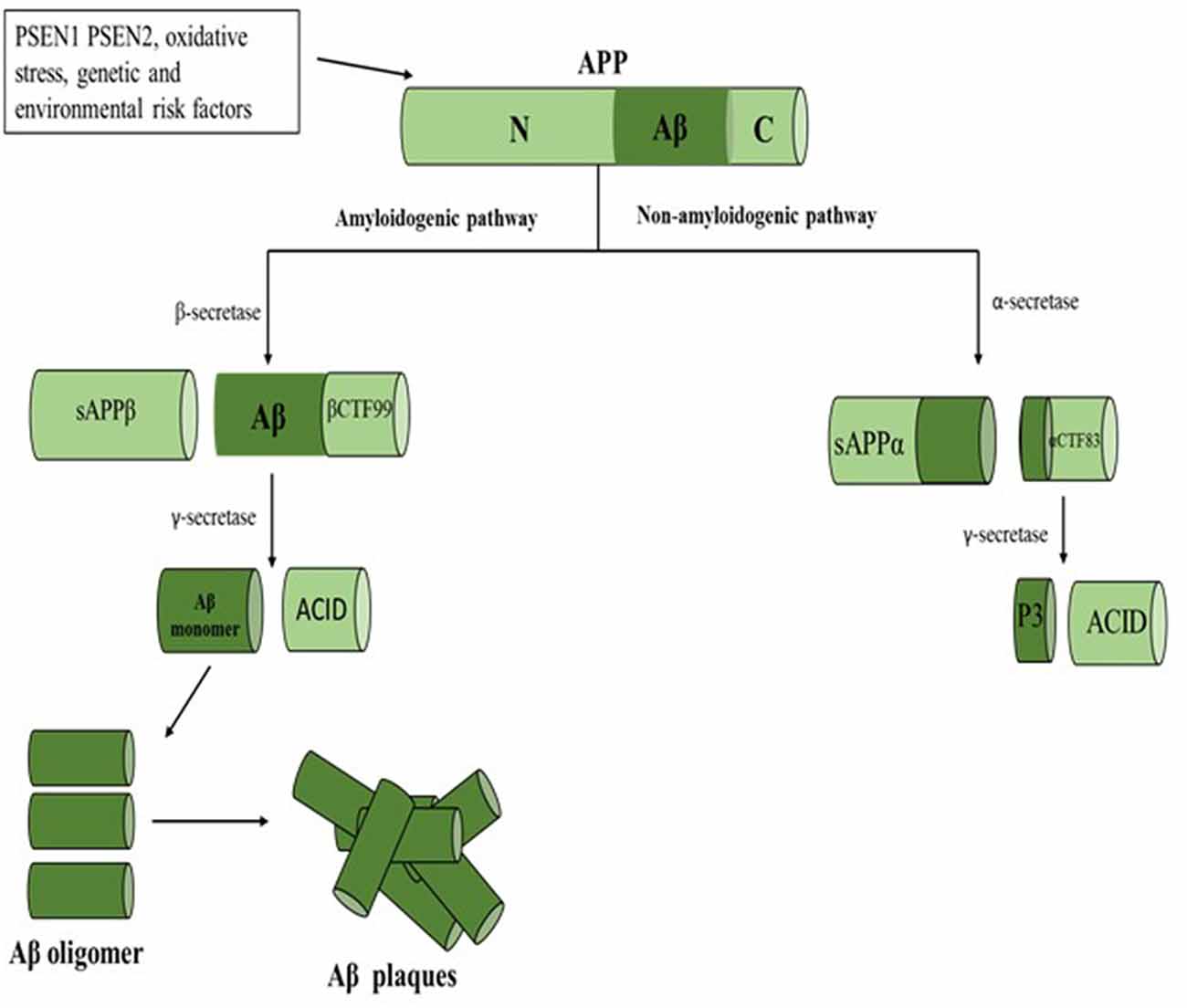
Figure 2. Schematic of Aβ hypothesis. APP is sequentially cleaved via amyloidogenic or non-amyloidogenic pathways. In the non-amyloidogenic pathway, it is cleaved by α-secretase, resulting in the production of the sAPPα. In the amyloidogenic pathway, APP is initially cleaved by β-secretase, then γ-secretase, resulting in the production of Aβ peptides. Abbreviations: APP, amyloid precursor protein; PSEN1, presenilin 1; PSEN2, presenilin 2; Aβ, amyloid beta.
Tau Proteins
Tau proteins are phosphoproteins present in all cells of the central nervous system (CNS; Lionnet et al., 2018). The main function of tau is the modulation of microtubule stability which forms the main pathway for intracellular protein trafficking (Mandelkow, 1998; Buée et al., 2000). But in AD, abnormal hyperphosphorylation of tau leads to its dysfunction, resulting in impairment of the transport system, the cytoskeleton, intracellular signaling, and mitochondrial integrity (Mandelkow, 1998; Iqbal et al., 2005). Hyperphosphorylated tau proteins dissociate from the microtubule (Figure 3) and bind with each other, forming paired helical filaments (PHFs). These accumulate, resulting in the characteristic NFTs seen in AD pathology (Gamblin et al., 2003).
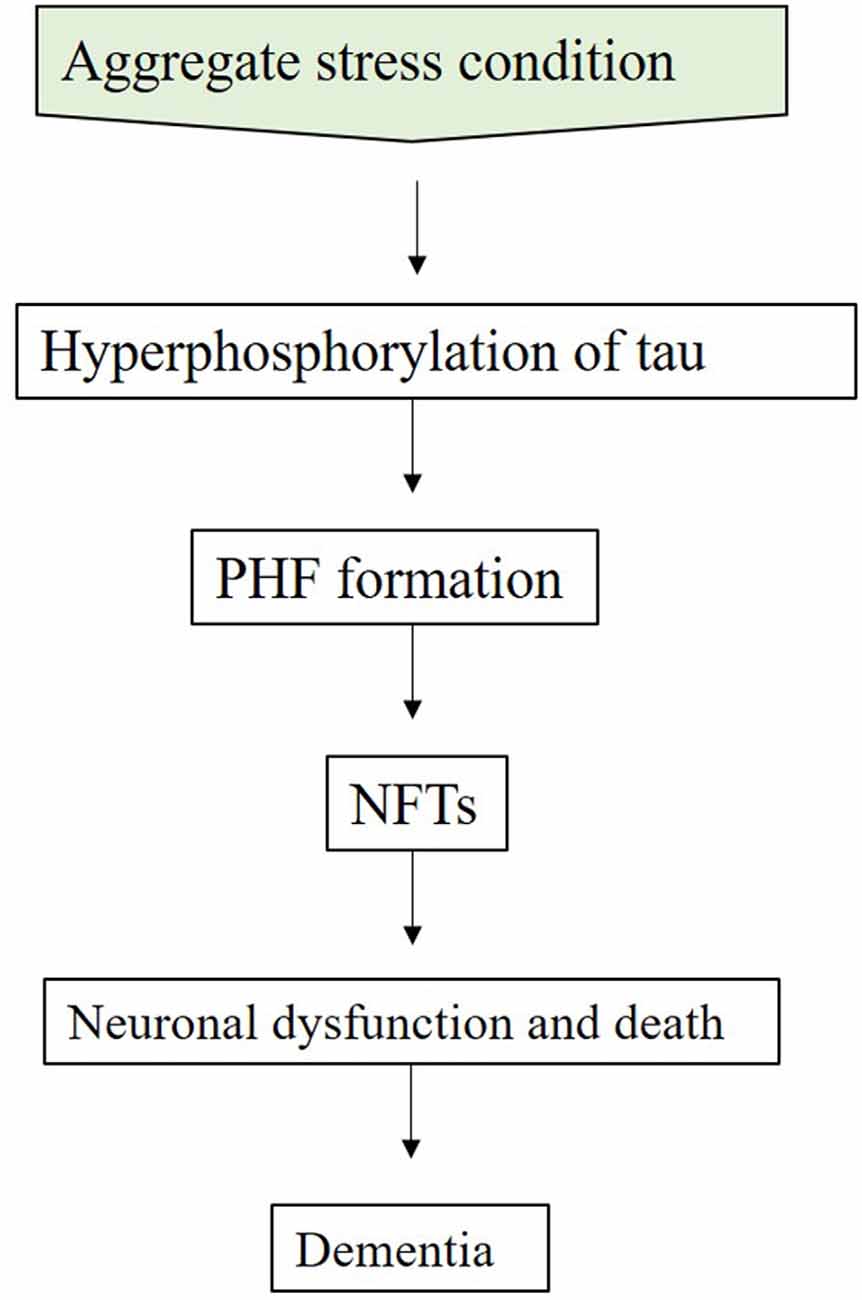
Figure 3. Schematic of tau hypothesis. Aggregate stress condition is a condition in which Aβ aggregation alters the kinase/phosphatase activity that can lead to hyperphosphorylation of tau resulting in PHF formation which consequently leads to neuronal dysfunction and dementia (Verwilst et al., 2018). Abbreviations: PHF, paired helical filament; NFT, neurofibrillary tangles.
There are other alternative hypotheses where Aβ plaques and NFTs may be formed independently and may be the products of dementia and not the cause (Hardy and Selkoe, 2002; Selkoe and Hardy, 2016). To date, there is no satisfactory hypothesis that can fully explain the exact mechanism of Aβ and tau accumulation, aggregation, and subsequent toxicity.
Neuroinflammation
The neuroinflammation is a response of the innate immune system within the brain as shown by an increased level of activated microglia and astrocytes, activated complement proteins and cytokines (Heneka et al., 2015; Zhang and Jiang, 2015). In AD, Aβ plaques and NFTs exacerbate any chronic inflammatory state, resulting in the increased action of cytokines (interleukin 1, tumor necrosis factor), prostaglandins, growth factors, thromboxanes, and ROS. These, in turn, enhance the APP processing, increasing Aβ42 levels in brain (Meraz-Ríos et al., 2013). Aβ also activates proinflammatory cytokines and some pro-inflammatory enzymes, such as cyclooxygenase-2 (COX-2), inducible nitric oxide synthase (iNOS) and nuclear factor kappa B (NF-κB). This Aβ-linked inflammatory response has been claimed to lead the neuronal damage in AD (Meraz-Ríos et al., 2013; Heneka et al., 2015; Zhang and Jiang, 2015). Accumulating evidence suggests that neuroinflammation is a major contributor of AD onset and progression (Heneka et al., 2010). Long-term consumption of nonsteroidal anti-inflammatory drugs (NSAIDs) can delay the onset or progression of AD, which also supports the role of neuroinflammation in AD (Ali et al., 2019).
Oxidative Stress as Another Major Contributor to AD Pathology
A growing body of literature indicates that oxidative stress is another pathophysiological feature of the AD brain (Good et al., 1996; Agostinho et al., 2010). Oxidative stress is the imbalance between the formation and detoxification of reactive oxygen species (ROS; Adwas et al., 2019). These ROS are normally produced as by-products of oxygen metabolism which utilizes both free radicals and non-free radical oxygen intermediate species, such as hydrogen peroxide (H2O2), superoxide (), hydroxyl radical (•OH), and singlet oxygen (1O2). These ROS are known to damage many biomolecules including DNA, RNA, protein, and lipids (Pham-Huy et al., 2008). Studies suggest that oxidative stress could: (a) be a consequence of Aβ deposition; (b) induce the production of Aβ; (c) be a combination of both: (a) and (b) (Sonnen et al., 2008; Tamagno et al., 2012). ROS are produced in vivo during oxidation and are involved in the progression of various health problems including cellular aging, mutagenesis, cardiovascular problems, diabetes, and neurodegeneration (Halliwell and Gutteridge, 1999; Moskovitz et al., 2002).
A high intake of foods rich in antioxidants may be beneficial to attenuate the ROS-associated problemsbased on the result of human dietary intervention studies (Lobo et al., 2010; Liu et al., 2018).
Mitochondrial Dysfunction as One of the Suggested Contributors of AD Pathology
Mitochondria are responsible for energy homeostasis in cells. Their dysfunction may contribute to the progression of several diseases including cancer, cardiovascular diseases, diabetes and neurodegenerative diseases (de Moura et al., 2010; Wen et al., 2016). A large body of research indicates that dysfunctional mitochondria play an important role in the pathogenesis of AD (Bhatti et al., 2017).
The initial level of mitochondria functions as well as its rate of decline influences AD onset and progression (Swerdlow et al., 2014). When mitochondrial function falls below a critical threshold, abnormal tau phosphorylation processes, amyloid plaque generation, synaptic degeneration, and oxidative stress can result (Lezi and Swerdlow, 2012). Several essential mitochondrial functions such as biogenesis, fission/fusion, and bioenergetics are also associated with AD. This makes mitochondrial dysfunction an important factor to consider in AD pathogenesis and its prevention (Lezi and Swerdlow, 2012; Nicolson, 2014; Flannery and Trushina, 2019).
Risk Factors for Alzheimer’s Disease
Risk factors are the specific conditions of an individual’s lifestyle along with genetic, gender, and environmental factors that determine the likelihood of developing AD (Alzheimer’s Association, 2018). The risk factors of AD can be divided into two main groups. Modifiable risks, are those that can be reduced through specific actions such as lifestyle changes. These risks contribute to 40% of AD cases (Livingston et al., 2020). Non-modifiable risks are those that cannot be changed including parental dementia, genetic risk factors and etc. (Alzheimer’s Association, 2018).
Modifiable Risk Factors
There is compelling evidence that smoking, high blood pressure, diabetes, high cholesterol, and obesity significantly increase the risk of AD (Prince et al., 2014). Hormones (testosterone and estrogen) can have a neuroprotective effect through regulating Aβ, thus, age-related decline in these hormones can affect cognitive ability and therefore increase the risk of developing AD (Verdile et al., 2014). Traumatic brain injury (TBI) is also reported to increase AD risk (Alzheimer’s Association, 2018).
Lifestyle factors including a healthy diet; adequate physical exercise, good sleep hygiene and cognitive training have been shown to reduce the risk of developing AD (Bauer and Morley, 2018). Conversely psychological factors (e.g., depression, anxiety, and stress) and vascular risk factors contribute to an increased risk of AD. A diet with high PP and high antioxidant activity can thus be considered as an approach to assist the prevention of chronic diseases, especially AD.
Non-modifiable Factors
Age is the main risk factor for AD. As people age, the risk of AD increases exponentially, as shown in several population based studies (Corrada et al., 2010). Apolipoprotein E (APOE) ε4 allele is the major genetic risk factor, which increases the probability of developing AD (Thakur et al., 2019). The effects of APOE ε4 on cognitive ability are variable from person to person (Prince et al., 2014; O’Donoghue et al., 2018). The APOE gene is present in chromosome 19 (Dementia Australia, 2018b). In humans, there are three common alleles: ε2, 3 and 4. Each individual carries two apolipoprotein genes which can be the same type (ε2, 2; 3, 3 or 4, 4), or a combination of two types (ε2, 3; 2, 4; 3, 4; Dementia Australia, 2018b). Individuals with at least one ε4 have a 2 to 3-fold risk of AD while those with two ε4 alleles (4, 4) rarely escape the disease. Compared to the other APOE alleles, the higher risk of developing AD in ε4 alleles is associated with an earlier age of AD onset (Alzheimer’s Association, 2018). This higher risk is three fold for one copy of ε4 allele and 12 fold for two copies (Alzheimer’s Association, 2018). In contrast, APOE ε2 carriage has a neuroprotective effect relative to APOE ε3 and APOE ε4. Carrying the double-barrelled APOE ε4 combination is fortunately uncommon, affecting only about 2% of the population, whereas about 25% of people carry a single copy of APOE ε4 (Alzheimer’s Association, 2018).
Natural Products to Effectively Combat Alzheimer’s Disease
Nutraceuticals (“nutrition” + “pharmaceutical”) with diverse compositions of plant secondary metabolites may hold great potential forpreventing and treating chronic diseases such as AD. These secondary metabolites, below a toxic dosage, usually do not have the side effects seen in synthetic drugs and are more widely available through the agri-food system. Some plant secondary metabolites such as PP perform beneficial physiological acts through specific mechanisms such as targeting enzymes and receptors. Epidemiological and preclinical studies have shown the protective effect of nutraceuticals such as fatty acids and polyphenolics (PP) found in fruits, vegetables, herbs, and nuts against neurodegeneration, to improve memory and cognitive function (Cole et al., 2005; Miller et al., 2017). In light of the literature, dietary PP, one of the richest sources of antioxidant activity in the human diet have become a topic of great current interest as potential neuromodulator agents to attenuate pathological hallmarks of AD. The rationale for this is their potential protective activities such as blood-brain-barrier (BBB) penetration capacity, oxidative stress attenuation, and Aβ aggregation inhibition (Mendes et al., 2018). Taken together, these potential therapeutic effects of PP indicate great potential of this class of phytochemicals to be investigated as a protective agent for AD (Panza et al., 2018).
Polyphenols and Their Anti-Alzheimer’s Disease Potential
Polyphenols are naturally occurring compounds and secondary metabolites of plants mostly produced in response to major stress (Pandey and Rizvi, 2009; Isah, 2019). They protect plants against biotic (living beings present in an ecosystem e.g., fungi, bacteria, and protists), and abiotic (non-living components e.g., water, soil, air, sunlight, temperature, and minerals) stressors (Rauf et al., 2019) acting as antioxidants, antimicrobials, and photo-absorption molecules. Thus, they defend plants from pathogens, ultraviolet radiation damage and predators such as insect pests (Beckman, 2000). Moreover, they are involved in the structural strength of plants during growth (Pandey and Rizvi, 2009). Polyphenols have received special attention from researchers due to their antioxidant activities which enable them to scavenge free radicals formed during the pathological processes of diseases such as cancer, cardiovascular diseases, and neurodegenerative disorders (Lakey-Beitia et al., 2015). They also have anti-inflammatory activity that is important in reducing oxidative stress thus conferring potential protective effects against the neurodegenerative process (Masci et al., 2015).
Polyphenols have demonstrated that they provide their neuroprotection through antioxidant, cholinergic, Aβ, and tau aggregation pathways in vitro and in vivo (Omar et al., 2017). The PP attenuate Aβ toxicity and oxidative stress in neurons by decreasing the Aβ aggregation and increasing the scavenging of free radicals, as shown in animal and cell culture studies (Dore et al., 1999; Agostinho et al., 2010; Mathiyazahan et al., 2015; Bai et al., 2017; Hwang et al., 2017). Polyphenols donate electrons to the free radicals to neutralize them, which is important to decrease the levels of ROS within cells (Lobo et al., 2010). Additionally, there is some evidence from cellular and animal model studies that PP may inhibit the Aβ42 toxicity (Bastianetto et al., 2008; Hugel and Jackson, 2015). Decrease in the hyperphosphorylation of tau protein, the formation of NFTs, and inflammation in in vitro and in vivo studies upon addition of PP has also been demonstrated (Mendes et al., 2018).
The basic structure of PP includes two aromatic rings linked through a pyran ring (Ross and Kasum, 2002). There structures are very complex, with the two main categories of PP are flavonoids and non-flavonoid compounds (El Gharras, 2009; see Figure 4). Flavonoids contain 15 carbon atoms. They are soluble in water and characterized by two benzene rings connected through a three-carbon chain. Flavonoids are sub-divided into anthoxanthins (flavones, flavonols, flavanols, isoflavonoids, flavanones), and anthocyanins (Lakey-Beitia et al., 2015). Non-flavonoid PP are phenolic acids, stilbenes, curcuminoids, lignans, and tannins (Lakey-Beitia et al., 2015).
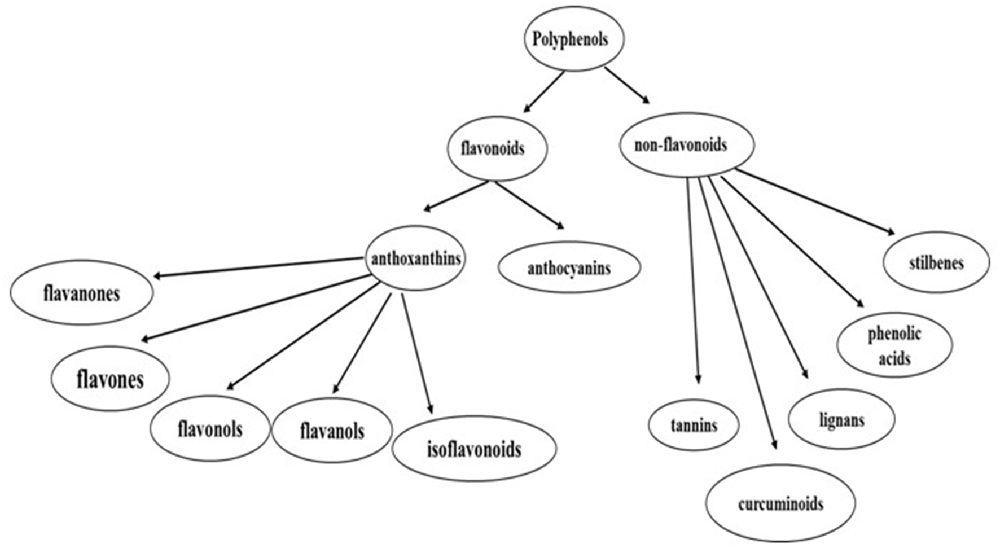
Figure 4. Polyphenols classifications. PP are divided into two main groups of flavonoids and non-flavonoids (El Gharras, 2009; Lakey-Beitia et al., 2015).
It has been hypothesized that the, the anti-amyloidogenic activity of PP is due to their physicochemical features, including the aromatic rings, molecular planarity, hydrogen bond formation, internal double bonds, and molecular weights below 500 g/mol (Lakey-Beitia et al., 2015). All these features are important for the inhibition of the amyloidogenic APP processing to reduce amyloid load, by activating α-secretase and inhibiting β- and γ-secretase (Lakey-Beitia et al., 2015).
Several in vitro and in vivo studies reported that PP-rich extracts from plants, like fruits and herbs, possess neuroprotective activities (Dai et al., 2006; Rossi et al., 2008; Loef and Walach, 2012; Hassaan et al., 2014; Dal-Pan et al., 2017; Omar et al., 2017; Polito et al., 2018). For instance, in vitro, in vivo and clinical studies showed the neuroprotective action of berry fruits through their polyphenolic contents (Vepsalainen et al., 2013; Wong et al., 2013; Subash et al., 2014). Other in vitro and in vivo studies indicate that pomegranate which is also rich in PP has the potential to attenuate AD progression by its anti-inflammatory and anti-Aβ accumulation activity (Hartman et al., 2006; Rojanathammanee et al., 2013). Moreover, extracts of other PP-rich fruits such as apple, banana, orange, grape, citrus fruit, and walnut have been also shown to inhibit Aβ neurotoxicity and oxidative stress as demonstrated by several in vitro studies (Chauhan et al., 2004; Heo et al., 2008; Toda et al., 2011; Lian et al., 2016; Braidy et al., 2017a). In one animal model study, PP-rich fruits such as Palm fruit could attenuate oxidative stress (Subash et al., 2015). Of particular interest to this current review is that several in vivo studies have reported cognition and memory enhancement activities of grapes, citrus fruit, walnut and buckwheat extracts (Wang et al., 2010; Choi et al., 2013; Lian et al., 2016; Braidy et al., 2017a; Pandareesh et al., 2018). in vitro investigation of the effect of a PP-rich extract of perennial buckwheat reported attenuation of Aβ toxicity in plasma (Liang et al., 2017). Another in vitro study using PP-rich extract of the herb Patrinina villosa Juss has shown a significant inhibitory effect on Aβ plaque aggregation (Bai et al., 2017). A cell culture study on twenty different South African medicinal PP-rich plants against AD reported the effectiveness of Xysmalobium undulatum, Cussonia paniculata, and Schotia brachypetala in decreasing the production of Aβ in comparison to other investigated extracts (Thakur et al., 2019). Moreover, based on the dietary intervention animal study of Ingale and Kasture (Ingale and Kasture, 2017), PP rich extract of purple passionflower could enhance cognitive function. Animal model studies, Capparis spinose, Caesalpinia crista, Iris germanica, and Paeonia suffruticosa could attenuate inflammation and Aβ aggregation through their polyphenolic contents, and make positive changes in cognition and memory (Costa et al., 2016; Gu et al., 2016; Borhani et al., 2017).
In vitro studies have reported that dietary drinks such as the crude juice of broccoli sprouts (Masci et al., 2015), tea (Polito et al., 2018), coffee (Ishida et al., 2018), and red wine (Dhir, 2018) are protective against Aβ-induced cytotoxicity and apoptotic cell death. They have been shown to attenuate mitochondrial dysfunction and hyperphosphorylation of tau proteins through their polyphenolic content (Lakey-Beitia et al., 2015; Sawikr et al., 2017; Polito et al., 2018).
Whole grain consumption as part of a healthy diet has been reported to be protective against several chronic diseases (Miller et al., 2000; Slavin, 2003; Aune et al., 2016). The health benefits of whole grains are in part due with their PP and the associated antioxidant activity (Slavin et al., 1997; Miller et al., 2000; Slavin, 2003; Tian et al., 2019).
Among whole grains, sorghum has some unique features that make it very attractive for neuroprotection studies. It is an inexpensive and abundant grain with a wide range of varieties, some of which are very high in PP content (including PP that are very rarely found in other plant food) and antioxidant activity. Several in vitro and in vivo studies have reported beneficial effects of sorghum PP on chronic diseases such as diabetes and cardiovascular disease, both of which are as risk factors of AD (Kim and Park, 2012; Suganyadevi et al., 2013; Stefoska-Needham et al., 2015; de Morais Cardoso et al., 2017; Moraes et al., 2018).
Sorghum
General Characteristics of Sorghum
Sorghum (Figure 5) is the fifth most-produced cereal crop in the world (Awika and Rooney, 2004). It is adaptable to grow in drought and hot climates. Thus, it is usually grown in warm semi-arid and arid areas across the globe (de Morais Cardoso et al., 2017). Sorghum grain has been mostly used as livestock feed and in the biofuel industry (de Morais Cardoso et al., 2017). Sorghum is gluten-free and low-fat while being high in protein and fiber. It has a high antioxidant and anti-inflammatory potential due to its bioactive compounds such as polyphenolics (Awika and Rooney, 2004; de Morais Cardoso et al., 2017).
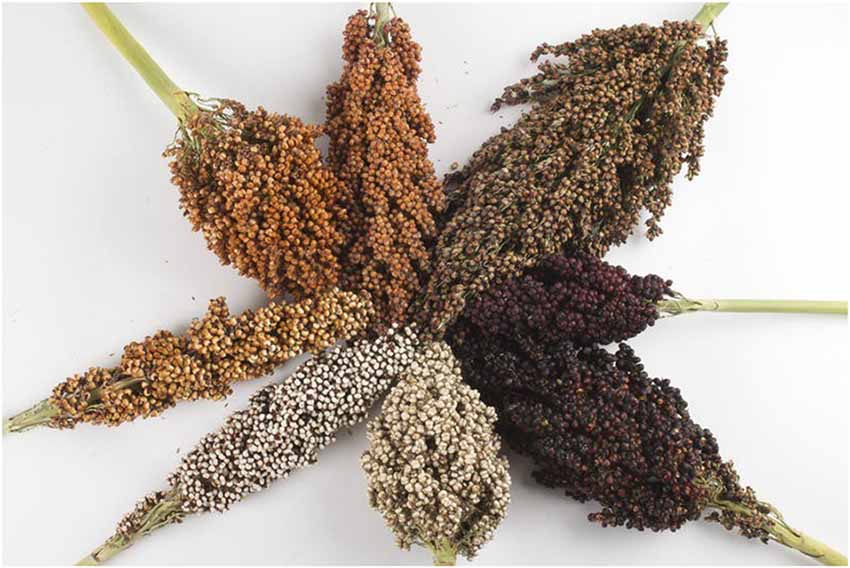
Figure 5. Different genotypes of sorghum grain: black pericarp, red pericarp, white pericarp, brown pericarp, and orange pericarp varieties. Selection of the most potent varieties of sorghum is crucial for health and medical-related purposes. Picture adopted from Barmac (2021).
Classification of Sorghum
Sorghum grain has been classified into three different groups based on extractable tannin content. Sorghum type I (low tannins extracted by 1% acidified methanol), type II (tannins extractable in 1% acidified methanol and not methanol alone), and type III (tannins extractable in both acidified methanol and methanol alone; Awika and Rooney, 2004).
Another common way of sorghum classification is based on its grain color and its total polyphenols. Sorghum has varieties with pigmented and non-pigmented precarps. White sorghum has no tannins or anthocyanins and has a very low level of total PP. Red sorghum (red pericarp) has a considerable amount of extractable PP without any tannins. Black sorghum (black pericarp) has a large amount of anthocyanins and finally, the brown sorghum (pigmented testa, different degrees of pericarp pigmentation) contains significant levels of tannins (Awika and Rooney, 2004). The concentration of flavonoid in sorghum is related to the pericarp color, pericarp thickness, and presence of testa (Taleon et al., 2012). However both environmental and genetic factors influence the phenolic level and profiles of sorghum grain (Awika and Rooney, 2004).
Sorghum PP and Health Benefits
A limited numbers of studies such as Awika et al. (Awika and Rooney, 2004; Yang et al., 2009; Awika, 2017; Girard and Awika, 2018) and Cardoso (de Morais Cardoso et al., 2017) have investigated the potential benefit of sorghum on health and disease prevention. According to their findings, sorghum should be considered as a health-beneficial grain, not just a low-value cereal grain. Sorghum has shown a positive impact on glycemic control, colonic microbiota, cholesterol attenuation, cardiovascular disease, anti-mutagenicity, and anti-inflammatory activity (Stefoska-Needham et al., 2015; de Morais Cardoso et al., 2017) which are all risk factors of AD. Below, we summarize information on the effect of sorghum on some chronic disease and their relation to AD.
Sorghum Protects Thyroid Gland Function and Combats Obesity
Sorghum is rich in manganese which is essential for thyroid hormone homeostasis. It facilitates the proper regulation of the thyroid gland promoting weight loss through regulating fat metabolism (Kangama, 2017). Moreover, sorghum contains a slow-digestible starch relative to other cereal crops which is also helpful to control obesity through slow glucose release and therefore modulation of food intake (Girard and Awika, 2018; Hasek et al., 2018). These beneficial characteristics of sorghum in controlling thyroid function (van Osch et al., 2004; Tan and Vasan, 2009; Chaker et al., 2016) and obesity (Alford et al., 2018) are considered as important risk factors of AD and thus can be very useful in its prevention.
Prevention of Cancer
The sorghum bran layer is rich in antioxidants which may reduce the risk of cancer by eliminating the possibility of free radical damage to DNA (Kangama, 2017). Sorghum extracts have been shown to have an antiproliferative effect on cancer cells (de Morais Cardoso et al., 2017). Suganyadevi et al. (2011) found that the red sorghum anthocyanin has anti-proliferative activity on a breast cancer cell line (Devi et al., 2011). Similar studies have shown the ability of sorghum extractto inhibit of cell proliferation and increase cell cycle regulator leukemia (Woo et al., 2012), breast (Park et al., 2012a), colon (Suganyadevi et al., 2011), and liver (Suganyadevi et al., 2011) cells. This characteristic is potentially beneficial to fight against diseases in which excessive free radicals play a major role including. AD (Kamath et al., 2004). The antioxidant and neuroprotective activity of the red dye extract from sorghum stem on cyclophosphamide-induced oxidative stress in rat brain is attributed its high level of phenolic and antioxidant activities (Oboh et al., 2010).
Managing Diabetes
Some varieties of sorghum grain possess a high amount of tannins which interact with starch and inhibits its digestion thus beneficially regulating blood glucose and insulin levels (Kangama, 2017). It has been suggested that sorghum has anti-diabetic and hypoglycemic effects through the regulation of insulin sensitivity via peroxisome proliferator-activated receptor gamma (PPAR-γ; Park et al., 2012b). Another study indicated that the hypoglycemic effect of sorghum extract is associated with hepatic gluconeogenesis not the glucose uptake of skeletal muscle (Kim and Park, 2012). Sorghum also can reduce both glucose and insulin responses (Poquette et al., 2014) and promote glucose and insulin homeostasis (Moraes et al., 2018). As diabetes could increase the risk of developing AD and declining cognitive function, anti-diabetic agents such as sorghum PP could potentially attenuate the AD pathological pathways (Arvanitakis et al., 2004; Hölscher, 2011).
Anaemia Prevention
The high level of iron and copper in sorghum helps the generation of red blood cells and improves the blood circulation and growth of cells and decreases the probability of getting anemia (Kangama, 2017). According to a population-based study, anemia is also considered as a risk factor of AD in the elderly and therefore preventing anemia would contribute to decreasing the risk of developing AD (Beard et al., 1997).
Assisting With Digestion and Cardiovascular Diseases Prevention
Sorghum assists with the proper function of the digestive system through its dietary fiber content (Kangama, 2017). This helps to control bloating, constipation, diarrhea, and excess gas. Moreover, having a high level of fiber in the diet decreases cholesterol uptake binding bile acids in the small intestine and preventing them from entering the blood-stream which is helpful for the prevention of cardiovascular which includes atherosclerosis, and stroke (Knopp et al., 1999; Kangama, 2017). Cardiovascular disease is considered an important risk factor for AD and thus its prevention will help reduce its risk (Meyer et al., 2000; Tosto et al., 2016; Tini et al., 2020).
The Anti-Alzheimer’s Disease Potential of Sorghum Polyphenolics
Some varieties of sorghum possess up to 6% (w/w dry basis) of phenolic compounds which is the highest level in any cereal grain (Su et al., 2017). Almost all classes of the phenolic compounds are present in sorghum (Awika and Rooney, 2004) including phenolic acids, flavonoids, tannins, and stilbenes (Tables 1, 2; Vanamala et al., 2017). The bran fraction of sorghum has the highest concentration of PP thus processing to remove the bran (decortication), will notably decrease the potential health benefits of the grain and therefore un-decorticated sorghum (whole grain) is recommended for consumption (Girard and Awika, 2018; Ashley et al., 2019).
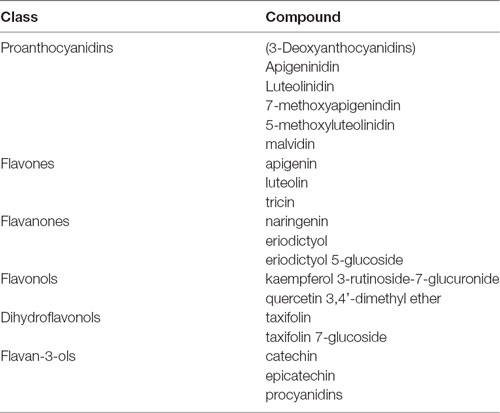
Table 1. Reported flavonoids in sorghum (Vanamala et al., 2017).
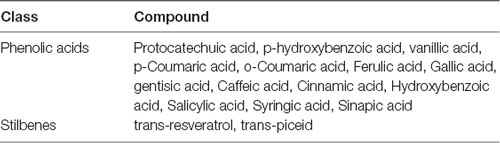
Table 2. Reported non-flavonoid in sorghum (Vanamala et al., 2017).
Based on the literature, almost all the polyphenolic compounds of the various sorghum genotypes have antioxidant activity which may be effective for the attenuation of AD pathological hallmarks (Awika and Rooney, 2004). Among all the PP of sorghum (Tables 1, 2), caffeic acid, trans-resveratrol, quercetin, catechin, cinnamic acid, cyanidin, apigenin, and kaempferol have gained the most attention for AD prevention and treatment (Rossi et al., 2008; Jabir et al., 2018). Snow et al. (2019) showed that PP exert their anti-AD properties primarily through prevention of aggregation of Aβ fibrils and tau protein NFTs. The presence of hydroxyl groups adjacent to aromatic rings may enhance the inhibition of Aβ/tau aggregation (Snow et al., 2019) by reducing the secondary folding of β-sheet structures which are characteristic of Aβ plaques and NFTs. For example, this property is found in proanthocyanidins, which are highly effective in reducing plaques and tangles in the brain as well as in improving short-term memory. Concluding from this article, sorghum PP such as epicatechin, luteolin, quercetin, etc. with adjacent hydroxyl groups can provide Aβ/tau disaggregation (Figures 6, 7).
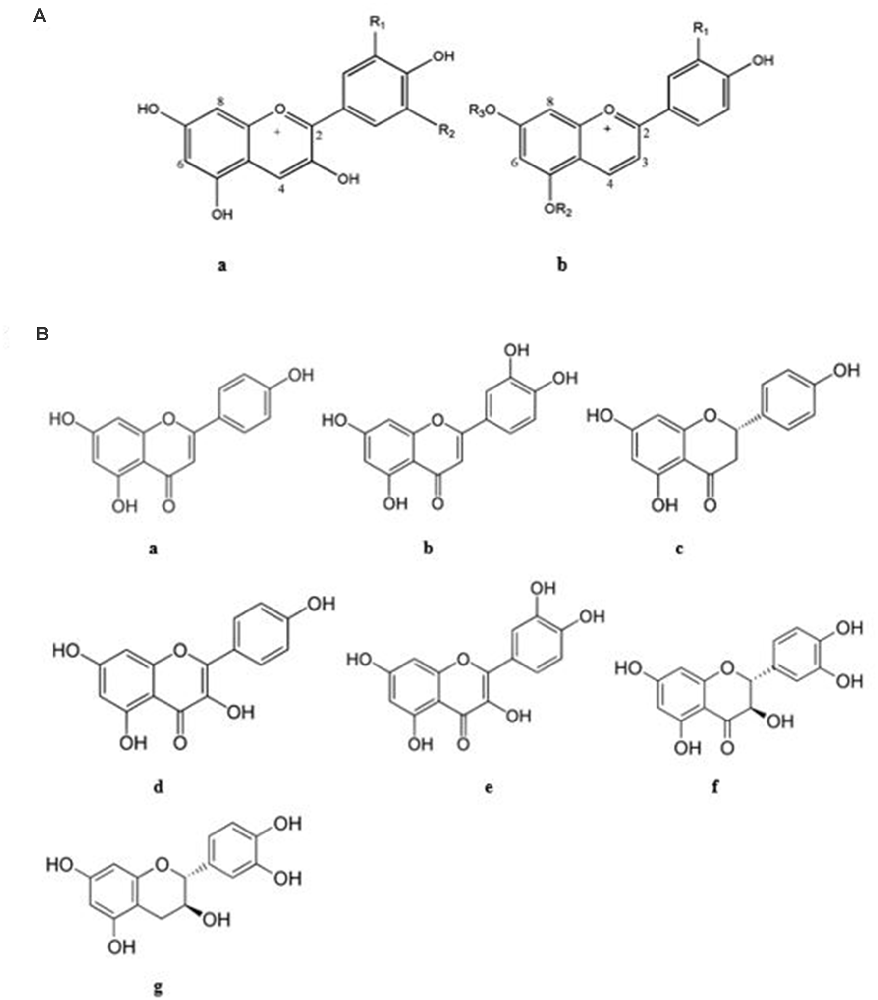
Figure 6. Chemical structure of important flavonoids of sorghum with anti-AD activities. (A) Anthocyanidins (a: R1= R2 = OCH3: malvidin, R1 = OH, R2 = H: cyanidin, R1=OCH3, R2 = H: peonidin, b: R1 = H, R2 = H, R3= H: apigeninidin, R1 = H, R2 = Glc, R3= H: apigeninidin-5-glucoside, R1 = H, R2 = H, R3=CH3: 7- methoxyapigenindin, R1 = OH, R2 = H, R3= H: luteolinidin, R1 = OH, R2 = Glc, R3= H: luteolinidin-5-glucoside, R1 = OH, R2=CH3, R3= H: 5-methoxyluteolinidin), (B) others (a: apigenin b: luteolin c: naringenin d: kaempferol e: quercetin f: taxifolin g: catechin; Awika et al., 2005; Lakey-Beitia et al., 2015; Vanamala et al., 2017; Jabir et al., 2018).
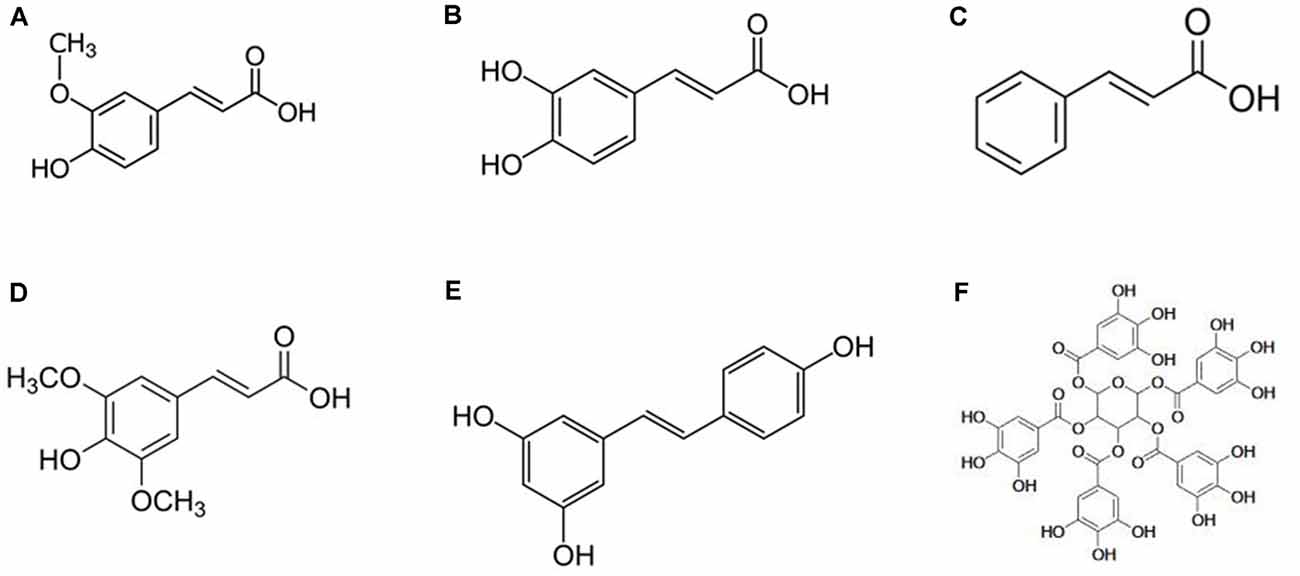
Figure 7. Chemical structure of important non-flavonoids of sorghum with anti-AD activities (Lakey-Beitia et al., 2015; Girard and Awika, 2018; Jabir et al., 2018; A: ferulic acid, B: caffeic acid, C: cinnamic acid, D: sinapic acid, E: resveratrol, and F: tannins).
Collectively the functions of sorghum PP include a combination of antioxidant, anti-amyloid, anti-tau, anti-inflammatory, AchEI and BChEI activities (Rossi et al., 2008; Omar et al., 2017; Jabir et al., 2018).
In summary, sorghum has a diverse polyphenolic profile depending on its genotype. According to in vitro and in vivo studies, several PP of sorghum have the potential to act as an anti- AD agent through different pathways such as free-radical scavenging, inhibition of Aβ and tau aggregation. The neuroprotection potential of important single PP of sorghum is illustrated in the subsequent sections.
Flavonoids
Proanthocyanidins
Pigmented sorghums are rich in anthocyanins some of which are rarely found elsewhere in plants kingdom (Su et al., 2017). High levels of different anthocyanins were detected in a red seed variety PI297139 (1,461.4 ± 98.7 mg/kg), followed by two brown varieties PI221723 and PI35038 (1,376.4 ± 33.2, 937.3 ± 29.4 mg/kg, respectively) and a yellow variety PI229838 (574.8 ± 105.4 mg/kg; Su et al., 2017).
Anthocyanins are divided into the sugar-free proanthocyanidins aglycons and the anthocyanin glycosides (Khoo et al., 2017). The most common anthocyanins found in the plant include cyanidin, delphinidin, pelargonidin, peonidin, malvidin, and petunidin (Khoo et al., 2017). Anthocyanins have several health benefits, where their intake is associated with a lower risk of several chronic diseases due to vasoprotective and anti-inflammatory activities (Lietti and Picci, 1976), antioxidant (Ali et al., 2018), anticancer, chemoprotective (Karaivanova et al., 1990), and hypoglycemic properties (Tsuda et al., 2003). In addition, anthocyanins also are beneficial in the progression/managing/controlling of cardiovascular diseases and HIV-1 (Nakaishi et al., 2000; Stintzing et al., 2002; Cooke et al., 2005; Jang et al., 2005; Julie Beattie and Duthie, 2005; Talavéra et al., 2006).
Anthocyanins are a type of PP with the ability of crossing the blood brain barrier (BBB; Belkacemi and Ramassamy, 2016). It is also reported that anthocyanins from anthocyanin-enriched bilberry and blackcurrant extracts can regulates the APP processing and spatial memory of a mouse model of AD (Vepsäläinen et al., 2013). Additionally, they could alleviate cognitive dysfunction and neuroinflammation in APP/PSEN1 transgenic mice model of AD (Li et al., 2020). According to the cell culture study of Belkacemi and Ramassamy, a mixture of anthocyanins and proanthocyanidins could beneficially affect various mechanisms involved in AD development such as through inhibition of Aβ toxicity and tau phosphorylation, prevention of oxidative stress, and amelioration of mitochondrial dysfunction (Belkacemi and Ramassamy, 2016).
The most common group of anthocyanins found in sorghum are the 3-deoxyanthocyanidins (3-DXA) and their derivatives (Hipskind et al., 1990; Figure 6A). Sorghum is the only common dietary source of the 3-DXA. The percentage of the 3-DXA in sorghum is dependent on the genotype (Awika and Rooney, 2004; Su et al., 2017). The recent animal study of Arbex et al. (2018) suggested that 3-DXA have a significant anti-inflammatory effect, thus protecting against one of the main hallmarks of AD (Arbex et al., 2018). The 3-DXAs are also reported to have antioxidant and anti-carcinogenic activities (Fratianni et al., 2007; Suganyadevi et al., 2011; Makanjuola et al., 2018). However, the data on the bioactivity of 3-DXA are very limited (Dore et al., 1999; Mathiyazahan et al., 2015). Also, there is lack of clinical trial data on the neuroprotective activity of anthocyanins and specifically 3-DXA.
Two main 3-DXAs of the sorghum are luteolinidin and apigeninidin which are orange and yellow colored, respectively. These two 3-DXAs which lack oxygen at the C-3 position are very rare in nature and also differ from the more common proanthocyanidins. The lack of oxygen at C-3 is associated with their high stability in light, heat, and change in pH (Suganyadevi et al., 2013; Figure 6A). Also, the molar absorptivity of the 3-DXA is higher compare to other proanthocyanidins except for cyanidin-3,5-diglucoside and therefore 3-DXA will be absorbed higher than other anthocyanins (Awika et al., 2004).
Flavones
Flavones are an important subgroup of flavonoids that are abundant in plants, especially herbs and cereal grains such as maize and sorghum (42–386 μg/g; Jiang et al., 2016). Common flavones include apigenin, luteolin, tangeritin, and chrysin (Singh et al., 2014; Kawser Hossain et al., 2016). Flavones have beneficial functions not only for the plant but also for human health. They possess strong antioxidant activity, which makes them potentially beneficial for the prevention and treatment of several chronic diseases including cancer, coronary heart disease, and neurodegenerative disease (Martens and Mithöfer, 2005; Singh et al., 2014).
Apigenin
Apigenin is present in several plants including sorghum with the reported concentration of 0.36–2.09 μg per gram of dry grain (Bradwell et al., 2018). This flavone from sorghum is known to be a strong antioxidant (Makanjuola et al., 2018). It has been shown to protect neurites and cell viability by enhancing the cytokine and nitric oxide release in inflammatory cells which may prevent or slow the progression of AD (Balez et al., 2016). Several studies reported the anti-inflammatory effects of apigenin in human and animal experiments (Liang et al., 1999; Rezai-Zadeh et al., 2008; Zhang et al., 2014). In one study, they induced inflammation by adding lipopolysaccharide to human and mouse macrophages then checked the effect of apigenin by PrimePCR array and through examining different mechanisms. They identified major target genes regulated by apigenin in lipopolysaccharide-mediated immune response (Zhang et al., 2014).
According to the apigenin-treated animal study of Zhao et al. (2013), in a double transgenic mouse model of AD, apigenin ameliorated the APP processing and Aβ toxicity through the regulation of BACE1 level and the reduction of Aβ deposition. They also showed apigenin beneficially reduced oxidative stress and reduced memory impairment, reduced of oxidative stress through the Morris water maze performance test (Zhao et al., 2013). It is also reported that oral administration of apigenin ameliorated the learning and memory deficits of Aβ-induced mice by attenuating oxidative damage, enhancing cholinergic neuronal transmission, and maintaining the BBB integrity in the cerebral cortex (Liu et al., 2011).
Luteolin
Luteolin is a flavone class of flavonoids found in several plants including sorghum (Lin et al., 2008). The concentration of luteolin in sorghum grain is (0.84–5.57 μg/g, dry basis) depending on the variety and environmental factors (Bradwell et al., 2018). Luteolin showed strong antioxidant and neuroinflammation activities in in vitro and in vivo studies (Paterniti et al., 2014; Kwon, 2017). Based on the animal model study of Wang et al. (2016) which was performed through Morris water maze and probe tests, luteolin (10 and 20 mg/kg) significantly attenuates spatial learning deficiencies and memory impairment. Furthermore, the animal behavioral tests study of Yu et al. (2015) found that luteolin (200 mg/kg) attenuates Aβ-induced learning and memory impairment through increasing the regulation of the cholinergic function and attenuation of oxidative stress. According to another in vivo study, luteolin could improve basal synaptic transmission and enhance the long-term potentiation (which is considered as a cellular correlate of learning and memory) through high frequency stimulation in the dental gyrus of the rat hippocampus (Xu et al., 2010). Moreover, daily oral administration of luteolin (50, 100, and 200 mg/kg) demonstrated a significant reduction of Aβ aggregation, oxidative stress, and inflammatory reaction in the hypoperfused rat brain (Fu et al., 2014).
Flavanones
Flavanones are a subgroup of flavonoids commonly available in grains and especially sorghum and some fruits such a citrus fruit (Tomás-Barberán and Clifford, 2000; Duodu and Awika, 2019). Common flavanones include hesperidin, naringenin, isosakuratenin, and eriodictyol (Das et al., 2019).
Some varieties of sorghum such as yellow sorghum possess high levels of flavanones mainly eriodictyol and naringenin; up to 1,800 μg/g depending on genotypes and environmental factors. The levels of reported flavanones in sorghum grain are much higher than in citrus fruit (400–600 μg/g) which has previously been considered as a main source of these compounds (Duodu and Awika, 2019).
According to cell culture studies, flavanones have several potential health benefits including neuroprotection potential through scavenging ROS (Lu et al., 2010), inhibiting the H2O2-induced neurotoxicity, increasing catalase activity, attenuating the intracellular free Ca2+, and decreasing the mitochondrial membrane potential (Hwang and Yen, 2008).
Naringenin
Naringenin is a compound that belongs to the flavanone group of flavonoids. It is available in several plant food including citrus fruits and sorghum (Manchope et al., 2017). Several in vivo and in vitro studies reported anti-inflammatory and antioxidant activity of naringenin (Heo et al., 2004; Manchope et al., 2017). The study of Khajevand-Khazaei et al. (2018) suggested positive effects of naringenin for the alleviation of lipopolysaccharide-induced cognitive deficits in rats; through enhancing the spatial recognition memory in Y maze, discrimination ratio in the object discrimination task, and retention in the passive avoidance test. Lipopolysaccharide and naringenin were administrated daily in a dose of 167 μg/kg and 25, 50, or 100 mg/kg, respectively (Khajevand-Khazaei et al., 2018). Naringenin is able to pass through the BBB and thus can act on the CNS. It has been shown to increase Aβ degrading enzymes through increasing M2 microglia polarization and inhibiting Aβ42 -induced M1 microglia activation in primary cultured cortical microglia (Yang et al., 2019). Two other in vivo studies also showed the ability of naringenin to improve learning and memory function through alleviation of oxidative stress and reducing apoptosis as evidenced by the Morris water maze test conducted in a rat model of AD (Ma et al., 2013; Ghofrani et al., 2015).
Flavonols
Flavonols are another subgroup of flavonoids which commonly available in onions, leeks, broccoli, blueberries and also abundant in cereal such as quinoa, barley, and sorghum (Awika, 2011; Pérez-Chabela and Hernández-Alcántara, 2018).
Common dietary flavonols include myricetin, quercetin, and kaempferol (Aherne and O’Brien, 2002). Flavonols are reported to have several health benefits including inhibiting low-density lipoprotein oxidation and thus reduced risk of atherosclerosis and general cardio protection effects (Giovinazzo and Grieco, 2019), cancer (Ali et al., 2008; Szliszka et al., 2011), and neurogenerative disease such as brain vascular atrophy, mild cognitive impairment (MCI), and AD (Patel et al., 2008).
Kaempferol is found in a variety of plants including sorghum (Przybylska-Balcerek et al., 2019). Epidemiological evidence suggests a positive relationship between the high intake of kaempferol and a reduced risk of developing several chronic diseases such as cancer, cardiovascular disease, and neurodegeneration (Calderon-Montano et al., 2011). Moreover, many studies have demonstrated that kaempferol has a wide range of pharmacological properties, including antioxidant (Tatsimo et al., 2012), anti-inflammatory (Devi et al., 2015), antimicrobial (Tatsimo et al., 2012), anticancer (Yoshida et al., 2008; Chen and Chen, 2013), cardioprotective (Xu et al., 2006; Choi et al., 2015), antidiabetic (Zhang and Liu, 2011; Alkhalidy et al., 2018), and neuroprotective activities (Kim et al., 2010; Calderon-Montano et al., 2011). An in vivo study by Cheng et al. (2018) reported significant anti-neuroinflammatory effects of kaempferol through high-mobility group protein 1 release and decreasing the toll-like receptor-4/myeloid differentiation factor 88 which was induced by lipopolysaccharide in the brains of mice. The effective doses of kaempferol were 50, or 100 mg/kg for 7 days (Cheng et al., 2018). Kaempferol has also been shown to have an anti-apoptotic activity in Aβ-induced neuroblastoma cell lines which can be beneficial for the prevention and treatment of AD (Kim et al., 2019). Another study in the transgenic Drosophila model of AD indicated that administration of 10, 20, 30, and 40 μM of kaempferol for 30 days could delay memory loss, reduce oxidative stress and AChE activity, and therefore is a potential therapeutic agent for AD (Beg et al., 2018).
Quercetin, a flavonoid found in various foods including sorghum, possesses strong antioxidant activity (Zhang et al., 2011). It demonstrates anti-inflammatory activities, the mechanism of which is through inhibition of the NF-κB pathway (Comalada et al., 2005), anticancer activities through a variety of mechanisms (Xing et al., 2001), attenuation of high cholesterol (Lu et al., 2010), protection form viral infections (Davis et al., 2008; Gonzalez et al., 2009), reduced risk of diabetes (Vessal et al., 2003), and cardiovascular diseases (Kleemann et al., 2011). Several of these effects can in turn potentially reduce the risk of AD (Zaplatic et al., 2019). Pre-treatment of hippocampal cell cultures with quercetin considerably attenuates Aβ-induced cytotoxicity, protein oxidation, lipid peroxidation, and apoptosis (Ansari et al., 2009). Thus, quercetin might be protective against Aβ toxicity by regulating oxidative stress (Ansari et al., 2009). Moreover, quercetin deceases ROS which is a major contributor to AD (Zaplatic et al., 2019). It also decreases extracellular Aβ, AChE level, tau toxicity, and microgliosis (Sabogal-Guaqueta et al., 2015). Additionally, quercetin improved learning and memory function in aged 3xTg-AD mice determined through the elevated plus-maze test (Orhan et al., 2007; Sabogal-Guaqueta et al., 2015).
Dihydroflavonols
Dihydroflavonols also referred to as flavanonols, are a subgroup of flavonoids available in some plants including sorghum (Gujer et al., 1986). Common dihydroflavonols include taxifolin, dihydrokaempferol, and dihydromyricetin (Espargaro et al., 2017; Sunil and Xu, 2019). They have several reported potential health benefits including antiallergic and anti-inflammatory activities (Ayoub et al., 2018). Moreover, they show strong free radical scavenging activity and protect neuronal cells from oxidative damage in vitro (Gong et al., 2009). Dihydroflavonols also demonstrated the capacity to inhibit Aβ aggregation, a main pathological hallmark of AD (Espargaro et al., 2017).
Taxifolin is a flavonoid with strong anti-inflammatory and antioxidant activities (Topal et al., 2016; Wang et al., 2018). It is available from different natural sources such as onion, milk thistle, and sorghum (Sunil and Xu, 2019). It significantly attenuated Aβ-induced cognitive impairment and neuronal cell death which was measured through novel object recognition tasks and the spatial memory in a mice model of AD (Wang et al., 2018). The in vivo study of Saito et al. (2017) showed the capacity of taxifolin in the improvement of cognitive and cerebrovascular functions which was evaluated by the water maze test and monitoring the cerebral blood flow changes in the cerebral amyloid angiopathy model of mice.
Flavan-3-ols
Flavan-3-ols are a subgroup of flavonoids available in several natural sources including teas, apples, beer, wine, and cereals like sorghum (Yao et al., 2004; Rao et al., 2018). Flavan-3-ols have a variety of health beneficial effects including reducing metabolic syndrome risk (Yang et al., 2012), antioxidant activity (Castillo et al., 2000), anti-cancer characteristic (Lei et al., 2016) and neuroprotective potential (Bastianetto et al., 2006). Common flavan-3-ols are catechin, epigallocatechin, epicatechin, epicatechin 3-gallate, epigallocatechin 3-gallate (EGCG), and theaflavin (Yao et al., 2004).
Catechins are common in tea, cocoa, berries, and sorghum. They have potent antioxidant and anti-inflammatory activities as reported by several in vitro and in vivo studies (Higdon and Frei, 2003; Abd El-Aziz et al., 2012; Zanwar et al., 2014; Stohs and Bagchi, 2015). Apart from radical scavenging activity, catechins modulate mitochondrial functions, activate survival genes, and also fight against Aβ-induced cognitive deficit and neurotoxicity through their antioxidant activity (Heo and Lee, 2005; Ban et al., 2006; Haque et al., 2008). Therefore, catechins are receiving great attention as potential neuroprotective agents (Mandel and Youdim, 2004).
Non-flavonoids
Phenolic Acids
Phenolic acids are the simplest naturally available PP (Tsao, 2010). The natural sources of phenolic acids include fruits, vegetables, and cereals, especially sorghum (Klensporf-Pawlik and Aladedunye, 2017; Ratnavathi, 2019). The phenolic acids of sorghum are mostly benzoic or cinnamic acid derivatives (Calviello et al., 2007). Phenolic acids are reported to have strong antioxidant (Sroka and Cisowski, 2003) and anti-inflammatory activities (Kang et al., 2015) as well as other health benefits including neuroprotective activities (Saibabu et al., 2015).
Cinnamic acid is an aromatic carboxylic acid (see Figure 7) with many beneficial effects. Several studies have shown the anti-microbial, antioxidant (Sova, 2012; Guzman, 2014), anti-cancer (De et al., 2011; Su et al., 2015), anti-atherogenic (Lapeyre et al., 2005), anti-tuberculosis (De et al., 2012), and anti-fungal (Tawata et al., 2014) effects of cinnamic acid. Cinnamic acid treatment in a mouse model of AD significantly reduced the Aβ plaque formation and improved the cognitive function through PPARα activation to stimulate lysosomal biogenesis. Additionally, cinnamic acid treatment improved the memory and behavioral performance in the mouse model of AD (Chandra et al., 2019). Interestingly, cinnamic acid derivatives have been also reported to act as cholinesterase inhibitors thus may have therapeutic effects on AD through this mechanism (Lan et al., 2017; Chen et al., 2018).
Ferulic acid (FA) is the most abundant phenolic acid found in sorghum and is also suggested to have strong anti-inflammatory activity (Sosulski et al., 1982; Lempereur et al., 1997; Sgarbossa et al., 2015; Ratnavathi, 2019). Due to its chemical structure (Figure 7), FA possesses a strong free radical scavenging ability (Srinivasan et al., 2007). The antioxidant effect of FA has been shown to be effective against several chronic diseases such as cancer (Rocha et al., 2012), cardiovascular (Ardiansyah et al., 2008), diabetes (Jung et al., 2007), and cellular oxidative stress (Calabrese et al., 2008). The efficacy of FA has been investigated against several neurodegenerative pathologies, particularly in AD. According to the finding, it could inhibit fibril formation (Ono et al., 2005) and protect neurons against Aβ-induced oxidative stress and neurotoxicity in vitro (Sultana et al., 2005). Moreover, the in vivo study of Yan et al. (2001) demonstrated that long-term administration of FA induces resistance to Aβ toxicity in the brain likely through its antioxidant and anti-inflammatory. These results indicate that FA at a dosage of 5.3 mg/kg/day could be beneficial for the prevention and treatment of AD (Yan et al., 2013).
Caffeic acid (CA) is a hydroxycinnamic acid derivative which is commonly found in fruits, herbs, and grains, especially sorghum. It has strong antioxidant and anti-inflammatory activities (da Cunha et al., 2004; Gülçin, 2006; Priebe et al., 2014). According to the in vivo study of Kim et al. (2015), CA was administrated to a Aβ-injected mouse model of AD at an oral dose of 50 mg/kg/day for 2 weeks. The cognitive impairment was assessed by different behavioral tests. The result demonstrated the ability of CA to enhance memory and cognitive impairment through inhibition of lipid peroxidation and NO production (Kim et al., 2015).
Sinapic acid (SA) is another phenolic acid present in sorghum that is suggested to have anti-inflammatory and neuroprotective activity (Yun et al., 2008; Zare et al., 2015). The neuroprotective examination of SA (10 mg/kg/day for 7 days) in an Aβ-induced mouse model of AD showed a strong attenuation of glial cell activation and memory impairment in a passive avoidance task. Moreover, SA attenuated neuronal cell death and cognitive dysfunction through its antioxidant and anti-inflammatory activities (Lee et al., 2012).
Stilbenes
Stilbenes are important group of non-flavonoid PP produced by plants in response to major stress, especially, fungal infection, and UV radiation (Varoni et al., 2016). Stilbenes contain two benzene rings connected by ethanol or ethylene molecule (Yu et al., 2005). Stilbenes are present in some plants such as grapes, berries, and sorghum (Yu et al., 2005; Reinisalo et al., 2015). According to the literature, they are protective against cancer, cardiovascular disease and age-related disease through their antioxidant and anti-inflammatory activities (Reinisalo et al., 2015; Sirerol et al., 2016). More than 400 stilbenes are available in nature and the most well studied ones are resveratrol, pterostilbene, piceatannol, and pinosylvin (Sirerol et al., 2016).
Resveratrol, well known as a PP from grapes, is also found in sorghum grain. It is one of the most studied stilbenes for neuroprotection and AD prevention (Dal-Pan et al., 2017). Resveratrol is classified as a non-flavonoid PP. There are two isomers of this compound in plants, trans-resveratrol and cis-resveratrol, along with their glucosides, trans-piceid, and cis-piceid (Varoni et al., 2016). Clinical trials have shown the beneficial effects of resveratrol on neurological disorders, cardiovascular disease and diabetes biomarkers (Berman et al., 2017). Currently, resveratrol is considered as a nutraceutical due to its many therapeutic effects including the regulation of caloric restriction, anti-inflammatory, and antioxidant activities (Salehi et al., 2018; Banez et al., 2020). Numerous cell culture and animal studies of resveratrol have demonstrated its anti-inflammatory, antioxidant, anti-Aβ aggregation and anti-abnormal tau phosphorylation properties (Savaskan et al., 2003; Lagouge et al., 2006; Rege et al., 2015; Wang et al., 2016; He et al., 2017). Antioxidant and anti-inflammatory activity of resveratrol could increase the clearance of Aβ, and modulate oxidative stress, neuronal energy homeostasis, and apoptosis (Bastianetto et al., 2015). Resveratrol also assists synaptic plasticity and neuroprotective kinases activities (Bastianetto et al., 2015). It is also reported to provide its neuroprotective activity through the activation of SIRT1, an enzyme that deacetylates proteins related to cellular regulation (Lagouge et al., 2006).
Tannins
Tannins are a group of non-flavonoid PP with many biological activities specifically binding to precipitate proteins and other organic molecules (Hagerman and Butler, 1989). They protect plant from predation and also help plant growth (Ferrell and Richard, 2006). They are distributed in many plants including fruits, beverages and grains such as grape, coffee, tea, wine, cacao and sorghum (Lamy et al., 2016). Brown colored sorghum varieties are known to have a high antioxidant capacity due to their higher tannin content, which is not present in all genotypes of sorghum regardless of whether they are colored or not (Awika et al., 2004). Tannins have strong antioxidant and anti-inflammatory activities (Braidy et al., 2017b). They are reported to reduce hyperphosphorylation of tau proteins in in vitro study (Yao et al., 2013). Moreover, oral administration of tannins in a transgenic mouse model of cerebral amyloidosis demonstrated an improvement in object recognition and spatial reference memory (Mori et al., 2012) and also they showed to inhibit the β-secretase activity in vitro and therefore they have significant preventative potential against AD (Mori et al., 2012). Tannins also demonstrated a significant inhibitory effect against AChE and BChE (Türkan et al., 2019). Additionally, the study of Park et al. (2019) reported strong cognitive and memory enhancing activities of tannins in a rat model via avoidance and the water maze task.
Conclusion
Currently available medication for AD is extremely limited in efficacy, therefore more studies should be conducted to discover new preventative and therapeutic agents. Recently, researchers have focused more on identifying treatments that can attenuate AD pathological hallmarks, rather than focusing on the treatments which only target the disease symptoms. Available symptomatic treatments such as AchEIs just attenuate symptoms temporarily by increasing the neurotransmitters in the brain without altering the disease progression path. For this purpose, studies on the effects of natural products such as polyphenolic antioxidants on AD pathological hallmarks are appearing in the scientific literature with increase regularity. One of the most highly concentrated food sources of antioxidant activity is sorghum grain which in colored gain varieties is due to high levels PP including 3-deoxyanthocyanidins, not found in any other common food.
To the best of our knowledge, there is no study on the effects of sorghum PP on AD pathology, therefore, the present review has illustrated the potential of sorghum PP as therapeutic agents against AD pathological hallmarks. This review has highlighted the unique chemistry and potential health beneficial properties of sorghum PP that can be leveraged to promote this under-utilized grain as a healthy food source.
As discussed throughout this review, numerous single PP have been studied and have demonstrated potential anti-AD effects in cellular and animal studies through a wide range of different mechanisms. However, a mixture of PP as found in an extract of sorghum grain could provide an additive or even synergistic multi-target therapeutic efficacy (Wang et al., 2014; Caruana et al., 2016; Andrade et al., 2019; Ayaz et al., 2019; Habtemariam, 2019).
Based on a variety of cell culture and animal model studies, sorghum PP have demonstrated several beneficial properties against some of the cellular pathways that contribute to AD pathogenesis. Among all the sorghum PP, caffeic acid, trans-resveratrol, quercetin, catechin, cinnamic acid, cyanidin, apigenin, and kaempferol have gained the most attention for their potential for AD prevention and treatment. However, the above-mentioned PP are not unique to sorghum. We hypothesise that the unique sorghum PP such as 3-DXA, and the complex mixtures of PP in sorghum grain extracts may collectively exert powerful synergistic effects on the inhibition of neurotoxic aggregation of Aβ and tau which initiate AD pathology.
Further studies to identify the specific mechanisms by which sorghum PP provide any neuroprotective activities are now necessary. One target mechanism is the antioxidant pathway in which the PP-rich extract of sorghum might reduce AD-associated oxidative stress. Both in vitro and in vivo animal model studies should be performed to gain as much evidence as possible before making recommendations for follow-on clinical trials. Moreover, anti-amyloidogenic, anti-tau/phospho tau, and anti-inflammatory mechanisms related to AD require further investigation. The new knowledge from these future studies may produce the high level of evidence require to confirm that the PP-rich extract from sorghum grain is a high efficacy preventative and therapeutic agent against AD.
Author Contributions
NR, WMADB, EH, HS, SJ, SG, and RM substantially contributed to the conception and design of the article and interpreting the relevant literature. NR (PhD candidate), wrote the first draft of the manuscript. NR, WMADB, EH, HS, SJ, and RM revised it critically for important intellectual content. All authors contributed to the article and approved the submitted version.
Funding
The authors thank Edith Cowan University for supporting this project.
Conflict of Interest
SG is the owner of the CWEK Pty Ltd., WA, Australia. SJ is the Director of Ingredients by Design Pty Ltd.
The authors declare that this study received partial funding from CWEK Pty Ltd. The funder had the following involvement in the study: Proofreading.
Publisher’s Note
All claims expressed in this article are solely those of the authors and do not necessarily represent those of their affiliated organizations, or those of the publisher, the editors and the reviewers. Any product that may be evaluated in this article, or claim that may be made by its manufacturer, is not guaranteed or endorsed by the publisher.
References
Abd El-Aziz, T. A., Mohamed, R. H., Pasha, H. F., and Abdel-Aziz, H. R. (2012). Catechin protects against oxidative stress and inflammatory-mediated cardiotoxicity in adriamycin-treated rats. Clin. Exp. Med. 12, 233–240. doi: 10.1007/s10238-011-0165-2
Adwas, A. A., Elsayed, A. S. I., Azab, A. E., and Amhimmid Quwaydir, F. (2019). Oxidative stress and antioxidant mechanisms in human body. J. Appl. Biotechnol. Bioeng. 6, 43–47. doi: 10.15406/jabb.2019.06.00173
Agostinho, P., Cunha, R. A., and Oliveira, C. (2010). Neuroinflammation, oxidative stress and the pathogenesis of Alzheimers disease. Curr. Pharm. Des. 16, 2766–2778. doi: 10.2174/138161210793176572
Aherne, S. A., and O’Brien, N. M. (2002). Dietary flavonols: chemistry, food content and metabolism. Nutrition 18, 75–81. doi: 10.1016/s0899-9007(01)00695-5
Alexander, G. C., Emerson, S., and Kesselheim, A. S. (2021). Evaluation of aducanumab for Alzheimer disease: scientific evidence and regulatory review involving efficacy, safety and futility. JAMA 325, 1717–1718. doi: 10.1001/jama.2021.3854
Alford, S., Patel, D., Perakakis, N., and Mantzoros, C. S. (2018). Obesity as a risk factor for Alzheimer’s disease: weighing the evidence. Obes. Rev. 19, 269–280. doi: 10.1111/obr.12629
Ali, H.-A., Chowdhury, A. K. A., Rahman, A. K. M., Borkowski, T., Nahar, L., and Sarker, S. D. (2008). Pachypodol, a flavonol from the leaves of Calycopteris floribunda, inhibits the growth of CaCo 2 colon cancer cell line in vitro. Phytother. Res. 22, 1684–1687. doi: 10.1002/ptr.2539
Ali, M. M., Ghouri, R. G., Ans, A. H., Akbar, A., and Toheed, A. (2019). Recommendations for anti-inflammatory treatments in Alzheimer’s Disease: a comprehensive review of the literature. Cureus 11:e4620. doi: 10.7759/cureus.4620
Ali, T., Kim, T., Rehman, S. U., Khan, M. S., Amin, F. U., Khan, M., et al. (2018). Natural dietary supplementation of anthocyanins via PI3K/Akt/Nrf2/HO-1 pathways mitigate oxidative stress, neurodegeneration and memory impairment in a mouse model of Alzheimer’s disease. Mol. Neurobiol. 55, 6076–6093. doi: 10.1007/s12035-017-0798-6
Alkhalidy, H., Moore, W., Wang, Y., Luo, J., McMillan, R. P., Zhen, W., et al. (2018). The flavonoid kaempferol ameliorates streptozotocin-induced diabetes by suppressing hepatic glucose production. Molecules 23:2338. doi: 10.3390/molecules23092338
Altinoglu, G., and Adali, T. (2020). Alzheimer’s disease targeted nano-based drug delivery systems. Curr. Drug Targets 21, 628–646. doi: 10.2174/1389450120666191118123151
Alzheimer’s Association (2018). Alzheimer’s disease fact and figures. Alzheimers Dement. 14, 367–429. doi: 10.1016/j.jalz.2018.02.001
Alzheimer’s Association (2019). Medications for Memory. Available online at: https://www.alz.org/alzheimers-dementia/treatments/medications-for-memory.
Anders, A., Gilbert, S., Garten, W., Postina, R., and Fahrenholz, F. (2001). Regulation of the α-secretase ADAM10 by its prodomain and proprotein convertases. The FASEB J. 15, 1837–1839. doi: 10.1096/fj.01-0007fje
Andrade, S., Ramalho, M. J., Loureiro, J. A., and Pereira, M. D. C. (2019). Natural compounds for Alzheimer’s disease therapy: a systematic review of preclinical and clinical studies. Int. J. Mol. Sci. 20:2313. doi: 10.3390/ijms20092313
Ansari, M. A., Abdul, H. M., Joshi, G., Opii, W. O., and Butterfield, D. A. (2009). Protective effect of quercetin in primary neurons against Abeta(1-42): relevance to Alzheimer’s disease. J. Nutr. Biochem. 20, 269–275. doi: 10.1016/j.jnutbio.2008.03.002
Arbex, P. M., Moreira, M. E. D. C., Toledo, R. C. L., de Morais Cardoso, L., Pinheiro-Sant’ana, H. M., Benjamin, L. D. A., et al. (2018). Extruded sorghum flour (Sorghum bicolor L.) modulate adiposity and inflammation in high fat diet-induced obese rats. J. Funct. Foods 42, 346–355. doi: 10.1016/j.jff.2018.01.010
Ardiansyah, Ohsaki, Y., Shirakawa, H., Koseki, T., and Komai, M. (2008). Novel effects of a single administration of ferulic acid on the regulation of blood pressure and the hepatic lipid metabolic profile in stroke-prone spontaneously hypertensive rats. J. Agric. Food Chem. 56, 2825–2830. doi: 10.1021/jf072896y
Arvanitakis, Z., Wilson, R. S., Bienias, J. L., Evans, D. A., and Bennett, D. A. (2004). Diabetes mellitus and risk of Alzheimer disease and decline in cognitive function. Arch. Neurol. 61, 661–666. doi: 10.1001/archneur.61.5.661
Asai, M., Hattori, C., Szabó, B., Sasagawa, N., Maruyama, K., Tanuma, S.-I., et al. (2003). Putative function of ADAM9, ADAM10 and ADAM17 as APP α-secretase. Biochem. Biophys. Res. Commun. 301, 231–235. doi: 10.1016/s0006-291x(02)02999-6
Ashley, D., Marasini, D., Brownmiller, C., Lee, J. A., Carbonero, F., and Lee, S. O. (2019). Impact of grain sorghum polyphenols on microbiota of normal weight and overweight/obese subjects during in vitro fecal fermentation. Nutrients 11:217. doi: 10.3390/nu11020217
Aune, D., Keum, N., Giovannucci, E., Fadnes, L. T., Boffetta, P., Greenwood, D. C., et al. (2016). Whole grain consumption and risk of cardiovascular disease, cancer and all cause and cause specific mortality: systematic review and dose-response meta-analysis of prospective studies. BMJ 353:i2716. doi: 10.1136/bmj.i2716
Awika, J. M. (2017). “Chapter 3 - sorghum: its unique nutritional and health-promoting attributes,” in Gluten-free Ancient Grains, eds John Taylor and Joseph Awika (Cambridge, England: Woodhead Publishing), 21–54.
Awika, J. M., Rooney, L. W., and Waniska, R. D. (2004). Properties of 3-deoxyanthocyanins from sorghum. J. Agric. Food Chem. 52, 4388–4394. doi: 10.1021/jf049653f
Awika, J. M., Rooney, L. W., and Waniska, R. D. (2005). Anthocyanins from black sorghum and their antioxidant properties. Food Chem. 90, 293–301. doi: 10.1016/j.foodchem.2004.03.058
Awika, J. M. (2011). “Sorghum flavonoids: unusual compounds with promising implications for health,” in Advances in Cereal Science: Implications to Food Processing and Health Promotion, (USA: American Chemical Society), 171–200.
Awika, J. M., and Rooney, L. W. (2004). Sorghum phytochemicals and their potential impact on human health. Phytochemistry 65, 1199–1221. doi: 10.1016/j.phytochem.2004.04.001
Ayaz, M., Ullah, F., Sadiq, A., Kim, M. O., and Ali, T. (2019). Editorial: Natural products-based drugs: potential therapeutics against Alzheimer’s disease and other neurological disorders. Front. Pharmacol. 10:1417. doi: 10.3389/fphar.2019.01417
Ayoub, I. M., Korinek, M., Hwang, T.-L., Chen, B.-H., Chang, F.-R., El-Shazly, M., et al. (2018). Probing the antiallergic and anti-inflammatory activity of biflavonoids and dihydroflavonols from dietes bicolor. J. Nat. Prod. 81, 243–253. doi: 10.1021/acs.jnatprod.7b00476
Bai, M., Yao, G.-D., Liu, S.-F., Wang, D., Liu, Q.-B., Huang, X.-X., et al. (2017). Lignans from a wild vegetable (Patrinina villosa) able to combat Alzheimer’s disease. J. Funct. Foods 28, 106–113.
Balez, R., Steiner, N., Engel, M., Muñoz, S. S., Lum, J. S., Wu, Y., et al. (2016). Neuroprotective effects of apigenin against inflammation, neuronal excitability and apoptosis in an induced pluripotent stem cell model of Alzheimer’s disease. Sci. Rep. 6:31450. doi: 10.1038/srep31450
Ban, J. Y., Jeon, S.-Y., Bae, K., Song, K.-S., and Seong, Y. H. (2006). Catechin and epicatechin from Smilacis chinae rhizome protect cultured rat cortical neurons against amyloid β protein (25-35)-induced neurotoxicity through inhibition of cytosolic calcium elevation. Life Sci. 79, 2251–2259. doi: 10.1016/j.lfs.2006.07.021
Banez, M. J., Geluz, M. I., Chandra, A., Hamdan, T., Biswas, O. S., Bryan, N. S., et al. (2020). A systemic review on the antioxidant and anti-inflammatory effects of resveratrol, curcumin and dietary nitric oxide supplementation on human cardiovascular health. Nutr. Res. 78, 11–26. doi: 10.1016/j.nutres.2020.03.002
Barmac (2021). Sorghum. Available online at: https://barmac.com.au/problem/sorghum/.
Bastianetto, S., Krantic, S., and Quirion, R. (2008). Polyphenols as potential inhibitors of amyloid aggregation and toxicity:possible significance to Alzheimers disease. Mini Rev. Med. Chem. 8, 429–435. doi: 10.2174/138955708784223512
Bastianetto, S., Menard, C., and Quirion, R. (2015). Neuroprotective action of resveratrol. Biochim. Biophys. Acta 1852, 1195–1201. doi: 10.1016/j.bbadis.2014.09.011
Bastianetto, S., Yao, Z.-X., Papadopoulos, V., and Quirion, R. (2006). Neuroprotective effects of green and black teas and their catechin gallate esters against β-amyloid-induced toxicity. Eur. J. Neurosci. 23, 55–64. doi: 10.1111/j.1460-9568.2005.04532.x
Bauer, J. M., and Morley, J. E. (2018). The relevance of healthy diets for the prevention of frailty and cognitive impairment. Curr. Opin. Clin. Nutr. Metab. Care 21, 1–3. doi: 10.1097/MCO.0000000000000438
Beard, C. M., Kokmen, E., O’Brien, P. C., Anía, B. J., and Melton, L. J. (1997). Risk of Alzheimer’s disease among elderly patients with anemia: population-based investigations in Olmsted County, Minnesota. Ann. Epidemiol. 7, 219–224. doi: 10.1016/s1047-2797(97)00015-x
Beckman, C. H. (2000). Phenolic-storing cells: keys to programmed cell death and periderm formation in wilt disease resistance and in general defence responses in plants?. Physiol. Mol. Plant Pathol. 57, 101–110. doi: 10.1006/pmpp.2000.0287
Beg, T., Jyoti, S., Naz, F., Rahul, Ali, F., Ali, S. K., et al. (2018). Protective effect of kaempferol on the transgenic Drosophila model of Alzheimer’s disease. CNS Neurol. Disord. Drug Targets 17, 421–429. doi: 10.2174/1871527317666180508123050
Belkacemi, A., and Ramassamy, C. (2016). Innovative anthocyanin/anthocyanidin formulation protects SK-N-SH cells against the amyloid-β peptide-induced toxicity: relevance to Alzheimer’s disease. Cent. Nerv. Sys. Agents Med. Chem. 16, 37–49. doi: 10.2174/1871524915666150730125532
Berman, A. Y., Motechin, R. A., Wiesenfeld, M. Y., and Holz, M. K. (2017). The therapeutic potential of resveratrol: a review of clinical trials. NPJ Precis. Oncol. 1:35. doi: 10.1038/s41698-017-0038-6
Bhatti, J. S., Bhatti, G. K., and Reddy, P. H. (2017). Mitochondrial dysfunction and oxidative stress in metabolic disorders—A step towards mitochondria based therapeutic strategies. Biochim. Biophys. Acta Mol. Basis Dis. 1863, 1066–1077. doi: 10.1016/j.bbadis.2016.11.010
Borhani, M., Sharifzadeh, M., Farzaei, M. H., Narimani, Z., Sabbaghziarani, F., Gholami, M., et al. (2017). Protective effect of iris germanica l. in beta-amyloid-induced animal model of Alzheimer’s disease. Afr. J. Tradit. Complement. Altern. Med. 14, 140–148. doi: 10.21010/ajtcam.v14i4.17
Bradwell, J., Hurd, M., Pangloli, P., McClure, A., and Dia, V. P. (2018). Storage stability of sorghum phenolic extracts’ flavones luteolin and apigenin. LWT 97, 787–793. doi: 10.1016/j.lwt.2018.08.006
Braidy, N., Behzad, S., Habtemariam, S., Ahmed, T., Daglia, M., Nabavi, S. M., et al. (2017a). Neuroprotective effects of citrus fruit-derived flavonoids, nobiletin and tangeretin in Alzheimer’s and Parkinson’s disease. CNS Neurol. Disord. Drug Targets 16, 387–397. doi: 10.2174/1871527316666170328113309
Braidy, N., Jugder, B. E., Poljak, A., Jayasena, T., Nabavi, S. M., Sachdev, P., et al. (2017b). Molecular targets of tannic acid in Alzheimer’s disease. Curr. Alzheimer Res. 14, 861–869. doi: 10.2174/1567205014666170206163158
Buée, L., Bussière, T., Buée-Scherrer, V., Delacourte, A., and Hof, P. R. (2000). Tau protein isoforms, phosphorylation and role in neurodegenerative disorders. Brain Res. Rev. 33, 95–130. doi: 10.1016/S0165-0173(00)00019-9
Burdette, A., Garner, P. L., Mayer, E. P., Hargrove, J. L., Hartle, D. K., and Greenspan, P. (2010). Anti-inflammatory activity of select sorghum (Sorghum bicolor) brans. J. Med. Food 13, 879–887. doi: 10.1089/jmf.2009.0147
Buxbaum, J. D., Liu, K. N., Luo, Y., Slack, J. L., Stocking, K. L., Peschon, J. J., et al. (1998). Evidence that tumor necrosis factor α converting enzyme is involved in regulated α-secretase cleavage of the Alzheimer amyloid protein precursor. J. Biol. Chem. 273, 27765–27767. doi: 10.1074/jbc.273.43.27765
Calabrese, V., Calafato, S., Puleo, E., Cornelius, C., Sapienza, M., Morganti, P., et al. (2008). Redox regulation of cellular stress response by ferulic acid ethyl ester in human dermal fibroblasts: role of vitagenes. Clin. Dermatol. 26, 358–363. doi: 10.1016/j.clindermatol.2008.01.005
Calderon-Montano, J. M., Burgos-Moron, E., Perez-Guerrero, C., and Lopez-Lazaro, M. (2011). A review on the dietary flavonoid kaempferol. Mini Rev. Med. Chem. 11, 298–344. doi: 10.2174/138955711795305335
Calviello, G., Serini, S., and Piccioni, E. (2007). n-3 polyunsaturated fatty acids and the prevention of colorectal cancer: molecular mechanisms involved. Curr. Med. Chem. 14, 3059–3069. doi: 10.2174/092986707782793934
Canady, V. A. (2021). FDA approves first drug therapy for Alzheimer’s in 18 years. Mental Health Weekly 31, 3–4. doi: 10.1002/mhw.32827
Caruana, M., Cauchi, R., and Vassallo, N. (2016). Putative role of red wine polyphenols against brain pathology in Alzheimer’s and Parkinson’s disease. Front. Nutr. 3:31. doi: 10.3389/fnut.2016.00031
Castillo, J., Benavente-García, O., Lorente, J., Alcaraz, M., Redondo, A., Ortuño, A., et al. (2000). Antioxidant activity and radioprotective effects against chromosomal damage induced in vivo by X-rays of flavan-3-ols (Procyanidins) from grape seeds (Vitis vinifera): comparative study versus other phenolic and organic compounds. J. Agric. Food Chem. 48, 1738–1745. doi: 10.1021/jf990665o
Chaker, L., Wolters, F. J., Bos, D., Korevaar, T. I. M., Hofman, A., van der Lugt, A., et al. (2016). Thyroid function and the risk of dementia. Neurology 87, 1688–1695. doi: 10.1212/WNL.0000000000003227
Chandra, S., Roy, A., Jana, M., and Pahan, K. (2019). Cinnamic acid activates PPARalpha to stimulate lysosomal biogenesis and lower amyloid plaque pathology in an Alzheimer’s disease mouse model. Neurobiol. Dis. 124, 379–395. doi: 10.1016/j.nbd.2018.12.007
Chasseigneaux, S., and Allinquant, B. (2012). Functions of Abeta, sAPPalpha and sAPPbeta : similarities and differences. J. Neurochem. 120, 99–108. doi: 10.1111/j.1471-4159.2011.07584.x
Chauhan, N., Wang, K. C., Wegiel, J., and Malik, M. N. (2004). Walnut extract inhibits the fibrillization of amyloid beta-protein and also defibrillizes its preformed fibrils. Curr. Alzheimer Res. 1, 183–188. doi: 10.2174/1567205043332144
Chen, A. Y., and Chen, Y. C. (2013). A review of the dietary flavonoid, kaempferol on human health and cancer chemoprevention. Food Chem. 138, 2099–2107. doi: 10.1016/j.foodchem.2012.11.139
Chen, Y., Zhu, J., Mo, J., Yang, H., Jiang, X., Lin, H., et al. (2018). Synthesis and bioevaluation of new tacrine-cinnamic acid hybrids as cholinesterase inhibitors against Alzheimer’s disease. J. Enzyme Inhib. Med. Chem. 33, 290–302. doi: 10.1080/14756366.2017.1412314
Cheng, X., Yang, Y.-L., Yang, H., Wang, Y.-H., and Du, G.-H. (2018). Kaempferol alle viates LPS-induced neuroinflammation and BBB dysfunction in mice via inhibiting HMGB1 release and down-regulating TLR4/MyD88 pathway. Int. Immunopharmacol. 56, 29–35. doi: 10.1016/j.intimp.2018.01.002
Choi, J.-H., Park, S.-E., Kim, S.-J., and Kim, S. (2015). Kaempferol inhibits thrombosis and platelet activation. Biochimie 115, 177–186. doi: 10.1016/j.biochi.2015.06.001
Choi, J. Y., Cho, E. J., Lee, H. S., Lee, J. M., Yoon, Y. H., and Lee, S. (2013). Tartary buckwheat improves cognition and memory function in an in vivo amyloid-beta-induced Alzheimer model. Food Chem. Toxicol. 53, 105–111. doi: 10.1016/j.fct.2012.11.002
Cole, G. M., Lim, G. P., Yang, F., Teter, B., Begum, A., Ma, Q., et al. (2005). Prevention of Alzheimer’s disease: Omega-3 fatty acid and phenolic anti-oxidant interventions. Neurobiol. Aging 26, 133–136. doi: 10.1016/j.neurobiolaging.2005.09.005
Comalada, M., Camuesco, D., Sierra, S., Ballester, I., Xaus, J., Gálvez, J., et al. (2005). in vivo quercitrin anti-inflammatory effect involves release of quercetin, which inhibits inflammation through down-regulation of the NF-κB pathway. Eur. J. Immunol. 35, 584–592. doi: 10.1002/eji.200425778
Cooke, D., Steward, W. P., Gescher, A. J., and Marczylo, T. (2005). Anthocyans from fruits and vegetables – does bright colour signal cancer chemopreventive activity? Eur. J. Cancer 41, 1931–1940. doi: 10.1016/j.ejca.2005.06.009
Corrada, M. M., Brookmeyer, R., Paganini-Hill, A., Berlau, D., and Kawas, C. H. (2010). Dementia incidence continues to increase with age in the oldest old: the 90+ study. Ann. Neurol. 67, 114–121. doi: 10.1002/ana.21915
Corrigan, F., Pham, C. L., Vink, R., Blumbergs, P. C., Masters, C. L., van den Heuvel, C., et al. (2011). The neuroprotective domains of the amyloid precursor protein, in traumatic brain injury, are located in the two growth factor domains. Brain Res. 1378, 137–143. doi: 10.1016/j.brainres.2010.12.077
Costa, S. L., Silva, V. D. A., dos Santos Souza, C., Santos, C. C., Paris, I., Muñoz, P., et al. (2016). Impact of plant-derived flavonoids on neurodegenerative diseases. Neurotox. Res. 30, 41–52. doi: 10.1007/s12640-016-9600-1
da Cunha, F. M., Duma, D., Assreuy, J., Buzzi, F. C., Niero, R., Campos, M. M., et al. (2004). Caffeic acid derivatives: in vitro and in vivo anti-inflammatory properties. Free Radic. Res. 38, 1241–1253. doi: 10.1080/10715760400016139
Dai, Q., Borenstein, A. R., Wu, Y., Jackson, J. C., and Larson, E. B. (2006). Fruit and vegetable juices and Alzheimer’s disease: the kame project. Am. J. Med. 119, 751–759. doi: 10.1016/j.amjmed.2006.03.045
Dal-Pan, A., Dudonne, S., Bourassa, P., Bourdoulous, M., Tremblay, C., Desjardins, Y., et al. (2017). Cognitive-enhancing effects of a polyphenols-rich extract from fruits without changes in neuropathology in an animal model of Alzheimer’s disease. J. Alzheimers Dis. 55, 115–135. doi: 10.3233/JAD-160281
Das, A. B., Goud, V. V., and Das, C. (2019). “9 - Phenolic compounds as functional ingredients in beverages,” in Value-Added Ingredients and Enrichments of Beverages, eds A. M. Grumezescu and A. M. Holban (Massachusetts, United States: Academic Press), 285–323.
Davis, J. M., Murphy, E. A., McClellan, J. L., Carmichael, M. D., and Gangemi, J. D. (2008). Quercetin reduces susceptibility to influenza infection following stressful exercise. Am. J. Physiol. Regul. Integr. Comp. Physiol. 295, R505–R509. doi: 10.1152/ajpregu.90319.2008
de Morais Cardoso, L., Pinheiro, S. S., Martino, H. S. D., and Pinheiro-Sant’Ana, H. M. (2017). Sorghum (Sorghum bicolor L.): nutrients, bioactive compounds and potential impact on human health. Crit. Rev. Food Sci. Nutr. 57, 372–390. doi: 10.1080/10408398.2014.887057
de Moura, M. B., dos Santos, L. S., and Van Houten, B. (2010). Mitochondrial dysfunction in neurodegenerative diseases and cancer. Environ. Mol. Mutagen. 51, 391–405. doi: 10.1002/em.20575
De, P., Baltas, M., and Bedos-Belval, F. (2011). Cinnamic acid derivatives as anticancer agents-a review. Curr. Med. Chem. 18, 1672–1703. doi: 10.2174/092986711795471347
De, P., De, K., Veau, D., Bedos-Belval, F., Chassaing, S., and Baltas, M. (2012). Recent advances in the development of cinnamic-like derivatives as antituberculosis agents. Expert Opin. Ther. Pat. 22, 155–168. doi: 10.1517/13543776.2012.661717
Dementia Australia. (2018a). Dementia prevalence data. Commissioned research undertaken by NATSEM, University of Canberra. p. 2018–2058. Available online at: https://www.dementia.org.au/information/statistics/prevalence-data.
Dementia Australia. (2018b). Genetics of Dementia. Available online at: https://www.dementia.org.au/information/genetics-of-dementia.
Devi, K. P., Malar, D. S., Nabavi, S. F., Sureda, A., Xiao, J., Nabavi, S. M., et al. (2015). Kaempferol and inflammation: from chemistry to medicine. Pharmacol. Res. 99, 1–10. doi: 10.1016/j.phrs.2015.05.002
Devi, P.S., Kumar, M. S., and Das, S. M. (2011). Evaluation of antiproliferative activity of red sorghum bran anthocyanin on a human breast cancer cell line (mcf-7). Int. J. Breast Cancer 2011:891481. doi: 10.4061/2011/891481
Dhir, A. (2018). “Role of the mediterranean diet in the brain and neurodegenerative disease,” in Role of the Mediterranean Diet in the Brain and Neurodegenerative Diseases, eds Tahira Farooqui and Akhlaq Farooqui (Massachusetts, United States: Elsevier, Academic Press), 484.
Dore, S., Bastianetto, S., Kar, S., and Quirion, R. (1999). Protective and rescuing abilities of IGF-I and some putative free radical scavengers against b-amyloid-inducing toxicity in neurons. Ann. N. Y. Acad. Sci. 890, 356–364. doi: 10.1111/j.1749-6632.1999.tb08015.x
Duodu, K. G., and Awika, J. M. (2019). “Chapter 8 - phytochemical-related health-promoting attributes of sorghum and millets,” in Sorghum and Millets (Second Edition), eds J. R. N. Taylor, and K. G. Duodu (Cambridge, England: AACC International Press), 225–258.
Duyckaerts, C., Delatour, B., and Potier, M.-C. (2009). Classification and basic pathology of Alzheimer disease. Acta Neuropathol. 118, 5–36. doi: 10.1007/s00401-009-0532-1
El Gharras, H. (2009). Polyphenols: food sources, properties and applications - a review. Int. J. Food Sci. Tech. 44, 2512–2518. doi: 10.1111/j.1365-2621.2009.02077.x
Espargaro, A., Ginex, T., Vadell, M. D. M., Busquets, M. A., Estelrich, J., Munoz-Torrero, D., et al. (2017). Combined in vitro cell-based/in silico screening of naturally occurring flavonoids and phenolic compounds as potential anti-Alzheimer drugs. J. Nat. Prod. 80, 278–289. doi: 10.1021/acs.jnatprod.6b00643
Fahrenholz, F., Gilbert, S., Kojro, E., Lammich, S., and Postina, R. (2000). α-secretase activity of the disintegrin metalloprotease ADAM 10: influences of domain structure. Ann. N. Y. Acad. Sci. 920, 215–222. doi: 10.1111/j.1749-6632.2000.tb06925.x
Ferrell, K. E. T., and Richard, W. (2006). Squirrels: The Animal Answer Guide. Baltimore: Johns Hopkins University Press.
Flannery, P. J., and Trushina, E. (2019). Mitochondrial dysfunction in Alzheimer’s disease and progress in mitochondria-targeted therapeutics. Curr. Behav. Neurosci. Rep. 6, 88–102. doi: 10.1007/s40473-019-00179-0
Fratianni, F., Tucci, M., Palma, M. D., Pepe, R., and Nazzaro, F. (2007). Polyphenolic composition in different parts of some cultivars of globe artichoke (Cynara cardunculus L. var. scolymus (L.) Fiori). Food Chem. 104, 1282–1286. doi: 10.1016/j.foodchem.2007.01.044
Fu, L., Sun, Y., Guo, Y., Chen, Y., Yu, B., Zhang, H., et al. (2017). Comparison of neurotoxicity of different aggregated forms of Aβ40, Aβ42 and Aβ43 in cell cultures. J. Pept. Sci. 23, 245–251. doi: 10.1002/psc.2975
Fu, X., Zhang, J., Guo, L., Xu, Y., Sun, L., Wang, S., et al. (2014). Protective role of luteolin against cognitive dysfunction induced by chronic cerebral hypoperfusion in rats. Pharmacol. Biochem. Behav. 126, 122–130. doi: 10.1016/j.pbb.2014.09.005
Gamblin, T. C., Chen, F., Zambrano, A., Abraha, A., Lagalwar, S., Guillozet, A. L., et al. (2003). Caspase cleavage of tau: linking amyloid and neurofibrillary tangles in Alzheimer’s disease. Proc. Natl. Acad. Sci. U S A 100, 10032–10037. doi: 10.1073/pnas.1630428100
Gandy, S., Caporaso, G., Buxbaum, J., Frangione, B., and Greengard, P. (1994). APP processing, Aβ-amyloidogenesis and the pathogenesis of Alzheimer’s disease. Neurobiol. Aging 15, 253–256. doi: 10.1016/0197-4580(94)90125-2
Ghofrani, S., Joghataei, M.-T., Mohseni, S., Baluchnejadmojarad, T., Bagheri, M., Khamse, S., et al. (2015). Naringenin improves learning and memory in an Alzheimer’s disease rat model: Insights into the underlying mechanisms. Eur. J. Pharmacol. 764, 195–201. doi: 10.1016/j.ejphar.2015.07.001
Giovinazzo, G., and Grieco, F. (2019). “9 - Tapping into health: wine as functional beverage,” in Alcoholic Beverages, eds A. M. Grumezescu, and A. M. Holban (Cambridge, England: Woodhead Publishing), 279–302.
Girard, A. L., and Awika, J. M. (2018). Sorghum polyphenols and other bioactive components as functional and health promoting food ingredients. J. Cereal Sci. 84, 112–124. doi: 10.1016/j.jcs.2018.10.009
Good, P. F., Werner, P., Hsu, A., Olanow, C. W., and Perl, D. P. (1996). Evidence of neuronal oxidative damage in Alzheimer’s disease. Am. J. Pathol. 149, 21–28.
Gong, J., Huang, K., Wang, F., Yang, L., Feng, Y., Li, H., et al. (2009). Preparation of two sets of 5,6,7-trioxygenated dihydroflavonol derivatives as free radical scavengers and neuronal cell protectors to oxidative damage. Bioorg. Med. Chem. 17, 3414–3425. doi: 10.1016/j.bmc.2009.03.032
Gonzalez, O., Fontanes, V., Raychaudhuri, S., Loo, R., Loo, J., Arumugaswami, V., et al. (2009). The heat shock protein inhibitor Quercetin attenuates hepatitis C virus production. Hepatology 50, 1756–1764. doi: 10.1002/hep.23232
Gu, X., Cai, Z., Cai, M., Liu, K., Liu, D., Zhang, Q., et al. (2016). Protective effect of paeoniflorin on inflammation and apoptosis in the cerebral cortex of a transgenic mouse model of Alzheimer’s disease. Mol. Med. Rep. 13, 2247–2252. doi: 10.3892/mmr.2016.4805
Gujer, R., Magnolato, D., and Self, R. (1986). Glucosylated flavonoids and other phenolic compounds from sorghum. Phytochemistry 25, 1431–1436. doi: 10.1016/S0031-9422(00)81304-7
Gülçin, I. (2006). Antioxidant activity of caffeic acid (3,4-dihydroxycinnamic acid). Toxicology 217, 213–220. doi: 10.1016/j.tox.2005.09.011
Guzman, J. D. (2014). Natural cinnamic acids, synthetic derivatives and hybrids with antimicrobial activity. Molecules 19, 19292–19349. doi: 10.3390/molecules191219292
Habtemariam, S. (2019). Natural products in Alzheimer’s disease therapy: would old therapeutic approaches fix the broken promise of modern medicines. Molecules 24:1519. doi: 10.3390/molecules24081519
Hagerman, A. E., and Butler, L. G. (1989). Choosing appropriate methods and standards for assaying tannin. J. Chem. Ecol. 15, 1795–1810. doi: 10.1007/BF01012267
Halliwell, B., and Gutteridge, J. M. C. (1999). Free Radicals in Biology and Medicine. New York, USA: Oxford University.
Haque, A. M., Hashimoto, M., Katakura, M., Hara, Y., and Shido, O. (2008). Green tea catechins prevent cognitive deficits caused by Aβ1-40 in rats. J. Nutr. Biochem. 19, 619–626. doi: 10.1016/j.jnutbio.2007.08.008
Hardy, J., and Selkoe, D. J. (2002). The amyloid hypothesis of Alzheimer’s disease: progress and problems on the road to therapeutics. Science 297, 353–356. doi: 10.1126/science.1072994
Hardy, J. A., and Higgins, G. A. (1992). Alzheimer’s disease: the amyloid cascade hypothesis. Science 256, 184–185. doi: 10.1126/science.1566067
Hartman, R. E., Shah, A., Fagan, A. M., Schwetye, K. E., Parsadanian, M., Schulman, R. N., et al. (2006). Pomegranate juice decreases amyloid load and improves behavior in a mouse model of Alzheimer’s disease. Neurobiol. Dis. 24, 506–515. doi: 10.1016/j.nbd.2006.08.006
Hasek, L. Y., Phillips, R. J., Zhang, G., Kinzig, K. P., Kim, C. Y., Powley, T. L., et al. (2018). Dietary slowly digestible starch triggers the gut-brain axis in obese rats with accompanied reduced food intake. Mol. Nutr. Food Res. 62:1700117. doi: 10.1002/mnfr.201700117
Hassaan, Y., Handoussa, H., El-Khatib, A.H., Linscheid, M. W., El Sayed, N., and Ayoub, N. (2014). Evaluation of plant phenolic metabolites as a source of Alzheimer’s drug leads. J. Biomed Res. Int. 2014:843263. doi: 10.1155/2014/843263
He, X., Li, Z., Rizak, J. D., Wu, S., Wang, Z., He, R., et al. (2017). Resveratrol attenuates formaldehyde induced hyperphosphorylation of tau protein and cytotoxicity in N2a cells. Front. Neurosci. 10:598. doi: 10.3389/fnins.2016.00598
Heneka, M. T., O’Banion, M. K., Terwel, D., and Kummer, M. P. (2010). Neuroinflammatory processes in Alzheimer’s disease. J. Neural Trans. (Vienna) 117, 919–947. doi: 10.1007/s00702-010-0438-z
Heneka, M. T., Carson, M. J., Khoury, J. E., Landreth, G. E., Brosseron, F., Feinstein, D. L., et al. (2015). Neuroinflammation in Alzheimer’s disease. Lancet Neurol. 14, 388–405. doi: 10.1016/S1474-4422(15)70016-5
Heo, H. J., Kim, D.-O., Shin, S. C., Kim, M. J., Kim, B. G., and Shin, D.-H. (2004). Effect of antioxidant flavanone, naringenin, from citrus junos on neuroprotection. J. Agric. Food Chem. 52, 1520–1525. doi: 10.1021/jf035079g
Heo, H. J., and Lee, C. Y. (2005). Epicatechin and catechin in cocoa inhibit amyloid β protein induced apoptosis. J. Agric. Food Chem. 53, 1445–1448. doi: 10.1021/jf048989m
Heo, H. J., Choi, S. J., Choi, S.-G., Shin, D.-H., Lee, J. M., and Lee, C. Y. (2008). Effects of banana, orange and apple on oxidative stress-induced neurotoxicity in PC12 cells. J. Food Sci. 73, H28–H32. doi: 10.1111/j.1750-3841.2007.00632.x
Hernández-Zimbrón, L. F., and Rivas-Arancibia, S. (2015). Oxidative stress caused by ozone exposure induces β-amyloid 1-42 overproduction and mitochondrial accumulation by activating the amyloidogenic pathway. Neuroscience 304, 340–348. doi: 10.1186/s12879-021-06390-3
Higdon, J. V., and Frei, B. (2003). Tea catechins and polyphenols: health effects, metabolism and antioxidant functions. Crit. Rev. Food Sci. Nutr. 43, 89–143. doi: 10.1080/10408690390826464
Hipskind, J. D., Hanau, R., Leite, B., and Nicholson, R. L. (1990). Phytoalexin accumulation in sorghum: identification of an apigeninidin acyl ester. Physiol. Mol. Plant Pathol. 36, 381–396. doi: 10.1016/0885-5765(90)90067-8
Hölscher, C. (2011). Diabetes as a risk factor for Alzheimer’s disease: insulin signalling impairment in the brain as an alternative model of Alzheimer’s disease. Biochem. Soc. Trans. 39, 891–897. doi: 10.1042/BST0390891
Hugel, H. M., and Jackson, N. (2015). Polyphenols for the prevention and treatment of dementia diseases. Neural Regen. Res. 10, 1756–1758. doi: 10.4103/1673-5374.169609
Hwang, S., Lim, J. W., and Kim, H. (2017). Inhibitory effect of lycopene on amyloid-beta-induced apoptosis in neuronal cells. Nutrients 9:883. doi: 10.3390/nu9080883
Hwang, S.-L., and Yen, G.-C. (2008). Neuroprotective effects of the citrus flavanones against H2O2-induced cytotoxicity in PC12 cells. J. Agric. Food Chem. 56, 859–864. doi: 10.1021/jf072826r
Ingale, S. P., and Kasture, S. B. (2017). Protective effect of standardized extract of passiflora incarnata flower in Parkinson’s and Alzheimer’s disease. Anc. Sci. Life 36, 200–206. doi: 10.4103/asl.ASL_231_16
Iqbal, K., del C. Alonso, A., Chen, S., Chohan, M. O., El-Akkad, E., Gong, C.-X., et al. (2005). Tau pathology in Alzheimer disease and other tauopathies. Biochim. Biophys. Acta 1739, 198–210. doi: 10.1016/j.bbadis.2004.09.008
Isah, T. (2019). Stress and defense responses in plant secondary metabolites production. Biol. Res. 52:39. doi: 10.1186/s40659-019-0246-3
Ishida, K., Yamamoto, M., Misawa, K., Ota, N., and Shimotoyodome, A. (2018). Coffee polyphenols prevent cognitive dysfunction and suppress amyloid β plaques in APP/PS2 transgenic mouse. Neurosci. Res. 154, 35–44. doi: 10.1016/j.neures.2019.05.001
Jabir, N. R., Khan, F. R., and Tabrez, S. (2018). Cholinesterase targeting by polyphenols: a therapeutic approach for the treatment of Alzheimer’s disease. CNS Neurosci. Ther. 24, 753–762. doi: 10.1111/cns.12971
Jang, Y. P., Zhou, J., Nakanishi, K., and Sparrow, J. R. (2005). Anthocyanins protect against A2E photooxidation and membrane permeabilization in retinal pigment epithelial cells. Photochem. Photobiol. 81, 529–536. doi: 10.1562/2004-12-14-RA-402
Jiang, N., Doseff, A. I., and Grotewold, E. (2016). Flavones: from biosynthesis to health benefits. Plants (Basel) 5:27. doi: 10.3390/plants5020027
Julie Beattie, A. C., and Duthie, G. G. (2005). Potential health benefits of berries. Curr. Nutr. Food Sci. 1, 71–86. doi: 10.2174/1573401052953294
Jung, E. H., Kim, S. R., Hwang, I. K., and Ha, T. Y. (2007). Hypoglycemic effects of a phenolic acid fraction of rice bran and ferulic acid in C57BL/KsJ-db/db mice. J. Agric. Food Chem. 55, 9800–9804. doi: 10.1021/jf0714463
Kamath, V. G., Chandrashekar, A., and Rajini, P. S. (2004). Antiradical properties of sorghum (Sorghum bicolor L. Moench) flour extracts. J. Cereal Sci. 40, 283–288. doi: 10.1016/j.jcs.2004.08.004
Kang, J., Thakali, K. M., Jensen, G. S., and Wu, X. (2015). Phenolic acids of the two major blueberry species in the US Market and their antioxidant and anti-inflammatory activities. Plant Foods Hum. Nutr. 70, 56–62. doi: 10.1007/s11130-014-0461-6
Kangama, C. O. (2017). Importance of sorghum bicolor in african’s cultures. J. Agric. Environ. Sci. 6, 134–137. doi: 10.15640/jaes.v6n2a16
Karaivanova, M., Drenskaand, D., and Ovcharov, R. (1990). A modification of the toxic effects of platinum complexes with anthocyanins. Eksp. Med. I. Morfol. 29, 19–24.
Kawser Hossain, M., Abdal Dayem, A., Han, J., Yin, Y., Kim, K., Kumar Saha, S., et al. (2016). Molecular mechanisms of the anti-obesity and anti-diabetic properties of flavonoids. Int. J. Mol. Sci. 17:569. doi: 10.3390/ijms17040569
Khajevand-Khazaei, M.-R., Ziaee, P., Motevalizadeh, S.-A., Rohani, M., Afshin-Majd, S., Baluchnejadmojarad, T., et al. (2018). Naringenin ameliorates learning and memory impairment following systemic lipopolysaccharide challenge in the rat. Eur. J. Pharmacol. 826, 114–122. doi: 10.1016/j.ejphar.2018.03.001
Khoo, H. E., Azlan, A., Tang, S. T., and Lim, S. M. (2017). Anthocyanidins and anthocyanins: colored pigments as food, pharmaceutical ingredients and the potential health benefits. Food Nutr. Res. 61:1361779. doi: 10.1080/16546628.2017.1361779
Kim, J., and Park, Y. (2012). Anti-diabetic effect of sorghum extract on hepatic gluconeogenesis of streptozotocin-induced diabetic rats. Nutr. Metab. (Lond) 9:106. doi: 10.1186/1743-7075-9-106
Kim, J. H., Lee, S., Cho, E. J., and Kim, H. Y. (2019). Neuroprotective effects of kaempferol, quercetin and its glycosides by regulation of apoptosis. J. Korea Acad. Indus Cooperation Soc. 20, 286–293. doi: 10.5762/KAIS.2019.20.2.286
Kim, J. H., Wang, Q., Choi, J. M., Lee, S., and Cho, E. J. (2015). Protective role of caffeic acid in an A beta25–35-induced Alzheimer’s disease model. Nutr. Res. Pract. 9, 480–488. doi: 10.4162/nrp.2015.9.5.480
Kim, J. K., Choi, S. J., Cho, H. Y., Hwang, H. J., Kim, Y. J., Lim, S. T., et al. (2010). Protective effects of kaempferol (3,4’,5,7-tetrahydroxyflavone) against amyloid beta peptide (Abeta)-induced neurotoxicity in ICR mice. Biosci. Biotechnol. Biochem. 74, 397–401. doi: 10.1271/bbb.90585
Kleemann, R., Verschuren, L., Morrison, M., Zadelaar, S., van Erk, M. J., Wielinga, P. Y., et al. (2011). Anti-inflammatory, anti-proliferative and anti-atherosclerotic effects of quercetin in human in vitro and in vivo models. Atherosclerosis 218, 44–52. doi: 10.1016/j.atherosclerosis.2011.04.023
Klensporf-Pawlik, D., and Aladedunye, F. (2017). “Chapter 10 - Wild rice: nutritional and health-promoting attributes,” in Gluten-Free Ancient Grains, eds J. R. N. Taylor and J. M. Awika (Sawston, United Kingdom: Woodhead Publishing), 271–296.
Knopp, R. H., Superko, H. R., Davidson, M., Insull, W., Dujovne, C. A., Kwiterovich, P. O., et al. (1999). Long-term blood cholesterol-lowering effects of a dietary fiber supplement. Am. J. Prev. Med. 17, 18–23. doi: 10.1016/s0749-3797(99)00039-2
Kuhn, P.-H., Wang, H., Dislich, B., Colombo, A., Zeitschel, U., Ellwart, J. W., et al. (2010). ADAM10 is the physiologically relevant, constitutive α-secretase of the amyloid precursor protein in primary neurons. EMBO J. 29, 3020–3032. doi: 10.1038/emboj.2010.167
Kulshreshtha, A., and Piplani, P. (2016). Current pharmacotherapy and putative disease-modifying therapy for Alzheimer’s disease. Neurol. Sci. 37, 1403–1435. doi: 10.1007/s10072-016-2625-7
Kwon, Y. (2017). Luteolin as a potential preventive and therapeutic candidate for Alzheimer’s disease. Exp. Gerontol. 95, 39–43. doi: 10.1016/j.exger.2017.05.014
Lagouge, M., Argmann, C., Gerhart-Hines, Z., Meziane, H., Lerin, C., Daussin, F., et al. (2006). Resveratrol improves mitochondrial function and protects against metabolic disease by activating SIRT1 and PGC-1alpha. Cell 127, 1109–1122. doi: 10.1016/j.cell.2006.11.013
Lakey-Beitia, J., Berrocal, R., Rao, K. S., and Durant, A. A. (2015). Polyphenols as therapeutic molecules in Alzheimer’s disease through modulating amyloid pathways. Mol. Neurobiol. 51, 466–479. doi: 10.1007/s12035-014-8722-9
Lammich, S., Kojro, E., Postina, R., Gilbert, S., Pfeiffer, R., Jasionowski, M., et al. (1999). Constitutive and regulated alpha-secretase cleavage of Alzheimer’s amyloid precursor protein by a disintegrin metalloprotease. Proc. Natl. Acad. Sci. U S A 96, 3922–3927. doi: 10.1073/pnas.96.7.3922
Lamy, E., Pinheiro, C., Rodrigues, L., Capela-Silva, F., Lopes, O. S., Moreira, P., et al. (2016). “Determinants of tannin-rich food and beverage consumption: oral perception vs. psychosocial aspects,” in Tannins: Biochemistry, Food Sources and Nutritional Properties, (New York, NY: Nova Publishers), 29–58.
Lan, J. S., Hou, J. W., Liu, Y., Ding, Y., Zhang, Y., Li, L., et al. (2017). Design, synthesis and evaluation of novel cinnamic acid derivatives bearing N-benzyl pyridinium moiety as multifunctional cholinesterase inhibitors for Alzheimer’s disease. J. Enzyme Inhib. Med. Chem. 32, 776–788. doi: 10.1080/14756366.2016.1256883
Lapeyre, C., Delomenede, M., Bedos-Belval, F., Duran, H., Negre-Salvayre, A., and Baltas, M. (2005). Design, synthesis and evaluation of pharmacological properties of cinnamic derivatives as antiatherogenic agents. J. Med. Chem. 48, 8115–8124. doi: 10.1021/jm050454c
Lee, H. E., Kim, D. H., Park, S. J., Kim, J. M., Lee, Y. W., Jung, J. M., et al. (2012). Neuroprotective effect of sinapic acid in a mouse model of amyloid beta(1–42) protein-induced Alzheimer’s disease. Pharmacol. Biochem. Behav. 103, 260–266. doi: 10.1016/j.pbb.2012.08.015
Lei, L., Yang, Y., He, H., Chen, E., Du, L., Dong, J., et al. (2016). Flavan-3-ols consumption and cancer risk: a meta-analysis of epidemiologic studies. Oncotarget 7, 73573–73592. doi: 10.18632/oncotarget.12017
Lempereur, I., Rouau, X., and Abecassis, J. (1997). Genetic and agronomic variation in arabinoxylan and ferulic acid contents of durum wheat (Triticum durum L.) grain and its milling fractions. J. Cereal Sci. 25, 103–110. doi: 10.1006/jcrs.1996.0090
Lezi, E., and Swerdlow, R. H. (2012). Mitochondria in neurodegeneration. Adv. Exp. Med. Biol. 942, 269–286. doi: 10.1007/978-94-007-2869-1_12
Li, J., Zhao, R., Jiang, Y., Xu, Y., Zhao, H., Lyu, X., et al. (2020). Bilberry anthocyanin improves the neuroinflammation and cognitive disfunctions in APP/PSEN1 mice via the CD33/TREM2/TYROBP signaling pathway in microglia. Food Funct. 11, 1572–1584. doi: 10.1039/c9fo02103e
Lian, Q., Nie, Y., Zhang, X., Tan, B., Cao, H., Chen, W., et al. (2016). Effects of grape seed proanthocyanidin on Alzheimer’s disease in vitro and in vivo. Exp. Ther. Med. 12, 1681–1692. doi: 10.3892/etm.2016.3530
Liang, C., Yuan, J. P., Ding, T., Yan, L., Ling, L., Zhou, X. F., et al. (2017). Neuroprotective effect of fagopyrum dibotrys extract against Alzheimer’s disease. Evid. Based Complement. Alternat. Med. 2017:3294586. doi: 10.1155/2017/3294586
Liang, Y. C., Huang, Y. T., Tsai, S. H., Lin-Shiau, S. Y., Chen, C. F., and Lin, J. K. (1999). Suppression of inducible cyclooxygenase and inducible nitric oxide synthase by apigenin and related flavonoids in mouse macrophages. Carcinogenesis 20, 1945–1952. doi: 10.1093/carcin/20.10.1945
Lietti, A. C. A., and Picci, M. (1976). Studies on vaccinium myrtillus anthocyanosides. I. vasoprotective and anti-inflammatory activity. Arzneimittelforschung 26, 829–832.
Lin, Y., Shi, R., Wang, X., and Shen, H.-M. (2008). Luteolin, a flavonoid with potential for cancer prevention and therapy. Curr. Cancer Drug Targets 8, 634–646. doi: 10.2174/156800908786241050
Lionnet, A., Wade, M. A., Corbille, A. G., Prigent, A., Paillusson, S., Tasselli, M., et al. (2018). Characterisation of tau in the human and rodent enteric nervous system under physiological conditions and in tauopathy. Acta Neuropathol. Commun. 6:65. doi: 10.1186/s40478-018-0568-3
Liu, R., Zhang, T., Yang, H., Lan, X., Ying, J., and Du, G. (2011). The flavonoid apigenin protects brain neurovascular coupling against amyloid-beta(2)(5)(-)(3)(5)-induced toxicity in mice. J. Alzheimers Dis. 24, 85–100. doi: 10.3233/JAD-2010-101593
Liu, Z., Ren, Z., Zhang, J., Chuang, C.-C., Kandaswamy, E., Zhou, T., et al. (2018). Role of ROS and nutritional antioxidants in human diseases. Front. Physiol. 9:477. doi: 10.3389/fphys.2018.00477
Livingston, G., Huntley, J., Sommerlad, A., Ames, D., Ballard, C., Banerjee, S., et al. (2020). Dementia prevention, intervention and care: 2020 report of the Lancet commission. Lancet 396, 413–446. doi: 10.1016/S0140-6736(20)30367-6
Lobo, V., Patil, A., Phatak, A., and Chandra, N. (2010). Free radicals, antioxidants and functional foods: impact on human health. Pharmacogn. Rev. 4, 118–126. doi: 10.4103/0973-7847.70902
Loef, M., and Walach, H. (2012). Fruit, vegetables and prevention of cognitive decline or dementia: a systematic review of cohort studies. J. Nutr. Health Aging 16, 626–630. doi: 10.1093/nutrit/nuab057
Long, J. M., and Holtzman, D. M. (2019). Alzheimer disease: an update on pathobiology and treatment strategies. Cell 179, 312–339. doi: 10.1016/j.cell.2019.09.001
Lu, J., Wu, D.-M., Zheng, Y.-L., Hu, B., Zhang, Z.-F., Shan, Q., et al. (2010). Quercetin activates AMP-activated protein kinase by reducing PP2C expression protecting old mouse brain against high cholesterol-induced neurotoxicity. J. Pathol. 222, 199–212. doi: 10.1002/path.2754
Lu, Y.-H., Su, M.-Y., Huang, H.-Y., Lin, L., and Yuan, C.-G. (2010). Protective effects of the citrus flavanones to PC12 cells against cytotoxicity induced by hydrogen peroxide. Neurosci. Lett. 484, 6–11. doi: 10.1016/j.neulet.2010.07.078
Ma, J., Yang, W.-Q., Zha, H., and Yu, H.-R. (2013). [Effect of naringenin on learning and memory ability on model rats with Alzheimer disease]. Zhong Yao Cai. 36, 271–276.
Makanjuola, S. B. L., Ogundaini, A. O., Ajonuma, L. C., and Dosunmu, A. (2018). Apigenin and apigeninidin isolates from the Sorghum bicolor leaf targets inflammation via cyclo-oxygenase-2 and prostaglandin-E2 blockade. Int. J. Rheum Dis. 21, 1487–1495. doi: 10.1111/1756-185X.13355
Malar, D. S., and Devi, K. P. (2014). Dietary polyphenols for treatment of Alzheimer’s disease–future research and development. Curr. Pharm. Biotechnol. 15, 330–342. doi: 10.2174/1389201015666140813122703
Manchope, M. F., Casagrande, R., and Verri, W. A., Jr. (2017). Naringenin: an analgesic and anti-inflammatory citrus flavanone. Oncotarget 8, 3766–3767. doi: 10.18632/oncotarget.14084
Mandel, S., and Youdim, M. B. H. (2004). Catechin polyphenols: neurodegeneration and neuroprotection in neurodegenerative diseases. Free Radic. Biol. Med. 37, 304–317. doi: 10.1016/j.freeradbiomed.2004.04.012
Mandelkow, E. (1998). Tau in Alzheimer’s disease. Trends Cell Biol. 8, 425–427. doi: 10.1016/s0962-8924(98)01368-3
Mao, P., and Reddy, P. H. (2011). Aging and amyloid beta-induced oxidative DNA damage and mitochondrial dysfunction in Alzheimer’s disease: Implications for early intervention and therapeutics. Biochim. Biophys. Acta 1812, 1359–1370. doi: 10.1016/j.bbadis.2011.08.005
Martens, S., and Mithöfer, A. (2005). Flavones and flavone synthases. Phytochemistry 66, 2399–2407. doi: 10.1016/j.phytochem.2005.07.013
Masci, A., Mattioli, R., Costantino, P., Baima, S., Morelli, G., Punzi, P., et al. (2015). Neuroprotective effect of Brassica oleracea sprouts crude juice in a cellular model of Alzheimer’s disease. Oxid. Med. Cell Longev. 2015:781938. doi: 10.1155/2015/781938
Mathiyazahan, D. B., Justin Thenmozhi, A., and Manivasagam, T. (2015). Protective effect of black tea extract against aluminium chloride-induced Alzheimer’s disease in rats: a behavioural, biochemical and molecular approach. J. Func. Foods 16, 423–435. doi: 10.1016/j.jff.2015.05.001
Mecocci, P., Boccardi, V., Cecchetti, R., Bastiani, P., Scamosci, M., Ruggiero, C., et al. (2018). A long journey into aging, brain aging and Alzheimer’s disease following the oxidative stress tracks. J. Alzheimers Dis. 62, 1319–1335. doi: 10.3233/JAD-170732
Mendes, D., Oliveira, M. M., Moreira, P. I., Coutinho, J., Nunes, F. M., Pereira, D. M., et al. (2018). Beneficial effects of white wine polyphenols-enriched diet on Alzheimer’s disease-like pathology. J. Nutr. Biochem. 55, 165–177. doi: 10.1016/j.jnutbio.2018.02.001
Meraz-Ríos, M. A., Toral-Rios, D., Franco-Bocanegra, D., Villeda-Hernández, J., and Campos-Peña, V. (2013). Inflammatory process in Alzheimer’s Disease. Front. Integr. Neurosci. 7:59. doi: 10.1097/JCN.0000000000000616
Meyer, J. S., Rauch, G. M., Rauch, R. A., Haque, A., and Crawford, K. (2000). Cardiovascular and other risk factors for Alzheimer’s disease and vascular dementia. Ann. N Y Acad. Sci. 903, 411–423. doi: 10.1111/j.1749-6632.2000.tb06393.x
Miller, H. E., Rigelhof, F., Marquart, L., Prakash, A., and Kanter, M. (2000). Antioxidant content of whole grain breakfast cereals, fruits and vegetables. J. Am. Coll. Nutr. 19, 312S–319S. doi: 10.1080/07315724.2000.10718966
Miller, M. G., Thangthaeng, N., Poulose, S. M., and Shukitt-Hale, B. (2017). Role of fruits, nuts and vegetables in maintaining cognitive health. Exp. Gerontol. 94, 24–28. doi: 10.1016/j.exger.2016.12.014
Modrego, P., and Lobo, A. (2019). A good marker does not mean a good target for clinical trials in Alzheimer’s disease: the amyloid hypothesis questioned. Neurodegener. Dis. Manag. 9, 119–121. doi: 10.2217/nmt-2019-0006
Moraes, É. A., Marineli, R. D. S., Lenquiste, S. A., Queiroz, V. A. V., Camargo, R. L., Borck, P. C., et al. (2018). Whole sorghum flour improves glucose tolerance, insulin resistance and preserved pancreatic islets function in obesity diet-induced rats. J. Funct. Foods 45, 530–540. doi: 10.1016/j.jff.2017.03.047
Mori, T., Rezai-Zadeh, K., Koyama, N., Arendash, G. W., Yamaguchi, H., Kakuda, N., et al. (2012). Tannic acid is a natural beta-secretase inhibitor that prevents cognitive impairment and mitigates Alzheimer-like pathology in transgenic mice. J. Biol. Chem. 287, 6912–6927. doi: 10.1074/jbc.M111.294025
Moskovitz, J., Yim, M. B., and Chock, P. B. (2002). Free radicals and disease. Arch. Biochem. Biophys. 397, 354–359. doi: 10.1006/abbi.2001.2692
Nakaishi, H., Matsumoto, H., Tominaga, S., and Hirayama, M. (2000). Effects of black current anthocyanoside intake on dark adaptation and VDT work-induced transient refractive alteration in healthy humans. Altern Med. Rev. 5, 553–562.
Nicolson, G. L. (2014). Mitochondrial dysfunction and chronic disease: treatment with natural supplements. Altern. Ther. Health Med. 20, 18–25.
Oboh, G., Akomolafe, T. L., and Adetuyi, A. O. (2010). Inhibition of cyclophosphamide-induced oxidative stress in brain by dietary inclusion of red dye extracts from sorghum (Sorghum bicolor) stem. J. Med. Food 13, 1075–1080. doi: 10.1089/jmf.2009.0226
O’Donoghue, M. C., Murphy, S. E., Zamboni, G., Nobre, A. C., and Mackay, C. E. (2018). APOE genotype and cognition in healthy individuals at risk of Alzheimer’s disease: a review. Cortex 104, 103–123. doi: 10.1016/j.cortex.2018.03.025
Omar, S. H., Scott, C. J., Hamlin, A. S., and Obied, H. K. (2017). The protective role of plant biophenols in mechanisms of Alzheimer’s disease. J. Nutr. Biochem. 47, 1–20. doi: 10.1016/j.jnutbio.2017.02.016
Ono, K., Hirohata, M., and Yamada, M. (2005). Ferulic acid destabilizes preformed beta-amyloid fibrils in vitro. Biochem. Biophys. Res. Commun. 336, 444–449. doi: 10.1016/j.bbrc.2005.08.148
Orhan, I., Kartal, M., Tosun, F., and ener, B. (2007). Screening of various phenolic acids and flavonoid derivatives for their anticholinesterase potential. Z. Naturforsch. C J. Biosci. 62, 829–832. doi: 10.1515/znc-2007-11-1210
Pandareesh, M. D., Chauhan, V., and Chauhan, A. (2018). Walnut supplementation in the diet reduces oxidative damage and improves antioxidant status in transgenic mouse model of Alzheimer’s disease. J. Alzheimers Dis. 64, 1295–1305. doi: 10.3233/JAD-180361
Pandey, K. B., and Rizvi, S. I. (2009). Plant polyphenols as dietary antioxidants in human health and disease. Oxid. Med. Cell Longev. 2, 270–278. doi: 10.4161/oxim.2.5.9498
Panza, F., Lozupone, M., Solfrizzi, V., Custodero, C., Valiani, V., D’Introno, A., et al. (2018). “Chapter 9 - Contribution of mediterranean diet in the prevention of Alzheimer’s disease,” in Role of the Mediterranean Diet in the Brain and Neurodegenerative Diseases, eds Tahira Farooqui and Akhlaq Farooqui (Massachusetts, United States: Academic Press), 139–155.
Park, J. H., Lee, C. D., Lee, J. K., Kim, C. J., and Lee, J. Y. (2019). Cognitive and memory enhancing activities of tannins on scopolamine-induced learning and memory impairment in rats. BioCell 43.
Park, J. H., Darvin, P., Lim, E. J., Joung, Y. H., Hong, D. Y., Park, E. U., et al. (2012a). Hwanggeumchal sorghum induces cell cycle arrest and suppresses tumor growth and metastasis through Jak2/STAT pathways in breast cancer xenografts. PLoS One 7:e40531. doi: 10.1371/journal.pone.0040531
Park, J. H., Lee, S. H., Chung, I. M., and Park, Y. (2012b). Sorghum extract exerts an anti-diabetic effect by improving insulin sensitivity via PPAR-gamma in mice fed a high-fat diet. Nutr. Res. Pract. 6, 322–327. doi: 10.4162/nrp.2012.6.4.322
Paroni, G., Bisceglia, P., and Seripa, D. (2019). Understanding the amyloid hypothesis in Alzheimer’s disease. J. Alzheimer’s Dis. 68, 493–510. doi: 10.3233/JAD-180802
Patel, A. K., Rogers, J. T., and Huang, X. (2008). Flavanols, mild cognitive impairment and Alzheimer’s dementia. Int. J. Clin. Exp. Med. 1, 181–191.
Paterniti, I., Cordaro, M., Campolo, M., Siracusa, R., Cornelius, C., Navarra, M., et al. (2014). Neuroprotection by association of palmitoylethanolamide with luteolin in experimental Alzheimer’s disease models: the control of neuroinflammation. CNS Neurol. Disord. Drug Targets 13, 1530–1541. doi: 10.2174/1871527313666140806124322
Pérez-Chabela, M. L., and Hernández-Alcántara, A. M. (2018). “Chapter 8 - Agroindustrial coproducts as sources of novel functional ingredients,” in Food Processing for Increased Quality and Consumption, eds A. M. Grumezescu and A. M. Holban (New York: Academic Press), 219–250.
Pham-Huy, L. A., He, H., and Pham-Huy, C. (2008). Free radicals antioxidants in disease and health. Int. J. Biomed. Sci. 4, 89–96.
Polito, C.A., Cai, Z. Y., Shi, Y. L., Li, X. M., Yang, R., Shi, M., et al. (2018). Association of tea consumption with risk of Alzheimer’s disease and anti-beta-amyloid effects of tea. Nutrients 10:655. doi: 10.3390/nu10050655
Poquette, N. M., Gu, X., and Lee, S. O. (2014). Grain sorghum muffin reduces glucose and insulin responses in men. Food Funct. 5, 894–899. doi: 10.1039/c3fo60432b
Priebe, W., Fokt, I., Szymanski, S., Madden, T., Myers, J., and Conrad, C. (2014). Orally bioavailable caffeic acid related anticancer drugs. University of Texas System United States. 8779151 - 11695547 - USPTO. Available online at: https://www.mysciencework.com/patent/show/orally-bioavailable-caffeic-acid-related-anticancer-drugs-US8779151B2.
Prince, M., Albanese, E., Guerchet, M., and Prina, M. (2014). World Alzheimer report 2014. Dementia and Risk Reduction.
Przybylska-Balcerek, A., Frankowski, J., and Stuper-Szablewska, K. (2019). Bioactive compounds in sorghum. Eur. Food Res. Technol. 245, 1075–1080. doi: 10.1007/s00217-018-3207-0
Puglielli, L., Tanzi, R. E., and Kovacs, D. M. (2003). Alzheimer’s disease: the cholesterol connection. Nat. Neurosci. 6, 345–351. doi: 10.1038/nn0403-345
Rao, S., Santhakumar, A. B., Chinkwo, K. A., Wu, G., Johnson, S. K., and Blanchard, C. L. (2018). Characterization of phenolic compounds and antioxidant activity in sorghum grains. J. Cereal Sci. 84, 103–111. doi: 10.1016/j.jcs.2018.07.013
Ratnavathi, C. V. (2019). “Chapter 12 - Grain Structure, Quality and Nutrition,” in Breeding Sorghum for Diverse End Uses, ed C. Aruna (Sawston, United Kingdom: Woodhead Publishing), 193–207.
Rauf, A., Imran, M., Abu-Izneid, T., Iahtisham-Ul-Haq, Patel, S., Pan, X., et al. (2019). Proanthocyanidins: a comprehensive review. Biomed. Pharmacother. 116:108999. doi: 10.1016/j.biopha.2019.108999
Rege, S. D., Geetha, T., Broderick, T. L., and Babu, J. R. (2015). Resveratrol protects β amyloid-induced oxidative damage and memory associated proteins in H19–7 hippocampal neuronal cells. Curr. Alzheimer Res. 12, 147–156. doi: 10.2174/1567205012666150204130009
Reinisalo, M., Kårlund, A., Koskela, A., Kaarniranta, K., and Karjalainen, R. O. (2015). Polyphenol stilbenes: molecular mechanisms of defence against oxidative stress and aging-related diseases. Oxid. Med. Cell Longev. 2015:340520. doi: 10.1155/2015/340520
Rezai-Zadeh, K., Ehrhart, J., Bai, Y., Sanberg, P. R., Bickford, P., Tan, J., et al. (2008). Apigenin and luteolin modulate microglial activation via inhibition of STAT1-induced CD40 expression. J. Neuroinflammation 5:41. doi: 10.1186/1742-2094-5-41
Rocha, L. D., Monteiro, M. C., and Teodoro, A. J. (2012). Anticancer properties of hydroxycinnamic acids—a review. Cancer Clin. Oncol. 1. doi: 10.5539/cco.v1n2p109
Rojanathammanee, L., Puig, K. L., and Combs, C. K. (2013). Pomegranate polyphenols and extract inhibit nuclear factor of activated T-cell activity and microglial activation in vitro and in a transgenic mouse model of Alzheimer disease. J. Nutr. 143, 597–605. doi: 10.3945/jn.112.169516
Roman, G. C. (2002). Defining dementia: clinical criteria for the diagnosis of vascular dementia. Acta Neurol. Scand. Suppl. 106, 6–9. doi: 10.1034/j.1600-0404.106.s178.2.x
Ross, J. A., and Kasum, C. M. (2002). Dietary flavonoids: bioavailability, metabolic effects and safety. Annu. Rev. Nutr. 22, 19–34. doi: 10.1146/annurev.nutr.22.111401.144957
Rossi, L., Mazzitelli, S., Arciello, M., Capo, C. R., and Rotilio, G. (2008). Benefits from dietary polyphenols for brain aging and Alzheimer’s disease. Neurochem. Res. 33, 2390–2400. doi: 10.1007/s11064-008-9696-7
Sabogal-Guaqueta, A. M., Munoz-Manco, J. I., Ramirez-Pineda, J. R., Lamprea-Rodriguez, M., Osorio, E., and Cardona-Gomez, G. P. (2015). The flavonoid quercetin ameliorates Alzheimer’s disease pathology and protects cognitive and emotional function in aged triple transgenic Alzheimer’s disease model mice. Neuropharmacology 93, 134–145. doi: 10.1016/j.neuropharm.2015.01.027
Sadhukhan, P., Saha, S., Dutta, S., Mahalanobish, S., and Sil, P. C. (2018). Nutraceuticals: an emerging therapeutic approach against the pathogenesis of Alzheimer’s disease. Pharmacol. Res. 129, 100–114. doi: 10.1016/j.phrs.2017.11.028
Saibabu, V., Fatima, Z., Khan, L. A., and Hameed, S. (2015). Therapeutic potential of dietary phenolic acids. Adv. Pharmacol. Sci. 2015:823539. doi: 10.1155/2015/823539
Saito, S., Yamamoto, Y., Maki, T., Hattori, Y., Ito, H., Mizuno, K., et al. (2017). Taxifolin inhibits amyloid-β oligomer formation and fully restores vascular integrity and memory in cerebral amyloid angiopathy. Acta Neuropathol. Commun. 5:26. doi: 10.1186/s40478-017-0429-5
Salehi, B., Mishra, A. P., Nigam, M., Sener, B., Kilic, M., Sharifi-Rad, M., et al. (2018). Resveratrol: a double-edged sword in health benefits. Biomedicines 6:91. doi: 10.3390/biomedicines6030091
Savaskan, E., Olivieri, G., Meier, F., Seifritz, E., Wirz-Justice, A., and Müller-Spahn, F. (2003). Red wine ingredient resveratrol protects from β-amyloid neurotoxicity. Gerontology 49, 380–383. doi: 10.1159/000073766
Sawikr, Y., Yarla, N. S., Peluso, I., Kamal, M. A., Aliev, G., and Bishayee, A. (2017). Neuroinflammation in Alzheimer’s disease: the preventive and therapeutic potential of polyphenolic nutraceuticals. Adv. Protein Chem. Struct. Biol. 108, 33–57. doi: 10.1016/bs.apcsb.2017.02.001
Selkoe, D. J., and Hardy, J. (2016). The amyloid hypothesis of Alzheimer’s disease at 25 years. EMBO Mol. Med. 8, 595–608. doi: 10.15252/emmm.201606210
Sgarbossa, A., Giacomazza, D., and Di Carlo, M. (2015). Ferulic acid: a hope for Alzheimer’s disease therapy from plants. Nutrients 7, 5764–5782. doi: 10.3390/nu7075246
Singh, M., Kaur, M., and Silakari, O. (2014). Flavones: an important scaffold for medicinal chemistry. Eur. J. Med. Chem. 84, 206–239. doi: 10.1016/j.ejmech.2014.07.013
Sirerol, J. A., Rodríguez, M. S., Asensi, M. A., Estrela, J. M., and Ortega, A. L. (2016). Role of natural stilbenes in the prevention of cancer. Oxid. Med. Cell Longev. 2016:3128951. doi: 10.1155/2016/3128951
Sivaraman, D., Anbu, N., Kabilan, N., Pitchiah Kumar, M., Shanmugapriya, P., Christian, G. J., et al. (2019). Review on current treatment strategy in Alzheimer’s disease and role of Herbs in treating neurological disorders. Int. J. Trans. Res. Ind. Med. 1, 33–43.
Slavin, J. (2003). Why whole grains are protective: biological mechanisms. Proc. Nutr. Soc. 62, 129–134. doi: 10.1079/PNS2002221
Slavin, J., Jacobs, D., and Marquart, L. (1997). Whole-grain consumption and chronic disease: protective mechanisms. Nutr. Cancer 27, 14–21. doi: 10.1080/01635589709514495
Snow, A. D., Castillo, G. M., Nguyen, B. P., Choi, P. Y., Cummings, J. A., Cam, J., et al. (2019). The Amazon rain forest plant Uncaria tomentosa (cat’s claw) and its specific proanthocyanidin constituents are potent inhibitors and reducers of both brain plaques and tangles. Sci. Rep. 9:561. doi: 10.1038/s41598-019-38645-0
Sonnen, J. A., Breitner, J. C., Lovell, M. A., Markesbery, W. R., Quinn, J. F., and Montine, T. J. (2008). Free radical-mediated damage to brain in Alzheimer’s disease and its transgenic mouse models. Free Radic. Biol. Med. 45, 219–230. doi: 10.1016/j.freeradbiomed.2008.04.022
Sosulski, F., Krygier, K., and Hogge, L. (1982). Free, esterified and insoluble-bound phenolic acids. 3. Composition of phenolic acids in cereal and potato flours. J. Agric. Food Chem. 30, 337–340. doi: 10.1021/jf00110a030
Sova, M. (2012). Antioxidant and antimicrobial activities of cinnamic acid derivatives. Mini Rev. Med. Chem. 12, 749–767. doi: 10.2174/138955712801264792
Srinivasan, M., Sudheer, A. R., and Menon, V. P. (2007). Ferulic Acid: therapeutic potential through its antioxidant property. J. Clin. Biochem. Nutr. 40, 92–100. doi: 10.3164/jcbn.40.92
Sroka, Z., and Cisowski, W. (2003). Hydrogen peroxide scavenging, antioxidant and anti-radical activity of some phenolic acids. Food Chem. Toxicol. 41, 753–758. doi: 10.1016/s0278-6915(02)00329-0
Stefoska-Needham, A., Beck, E. J., Johnson, S. K., and Tapsell, L. C. (2015). Sorghum: an underutilized cereal whole grain with the potential to assist in the prevention of chronic disease. Food Rev. Int. 31, 401–437. doi: 10.1080/87559129.2015.1022832
Stintzing, F. C., Stintzing, A. S., Carle, R., Frei, B., and Wrolstad, R. E. (2002). Color and antioxidant properties of cyanidin-based anthocyanin pigments. J. Agric. Food Chem. 50, 6172–6181. doi: 10.1021/jf0204811
Stohs, S. J., and Bagchi, D. (2015). Antioxidant, anti-inflammatory and chemoprotective properties of acacia catechu heartwood extracts. Phytother. Res. 29, 818–824. doi: 10.1002/ptr.5335
Su, P., Shi, Y., Wang, J., Shen, X., and Zhang, J. (2015). Anticancer agents derived from natural cinnamic acids. Anticancer Agents Med. Chem. 15, 980–987. doi: 10.2174/1871520615666150130111120
Su, X., Rhodes, D., Xu, J., Chen, X., Davis, H., Wang, D., et al. (2017). Phenotypic diversity of anthocyanins in sorghum accessions with various pericarp pigments. J. Nutr. Food Sci. 7:1000610. doi: 10.4172/2155-9600.1000610
Subash, S., Essa, M. M., Al-Asmi, A., Al-Adawi, S., Vaishnav, R., and Guillemin, G. J. (2015). Effect of dietary supplementation of dates in Alzheimer’s disease APPsw/2576 transgenic mice on oxidative stress and antioxidant status. Nutr. Neurosci. 18, 281–288. doi: 10.1179/1476830514Y.0000000134
Subash, S., Essa, M. M., Al-Adawi, S., Memon, M. A., Manivasagam, T., and Akbar, M. (2014). Neuroprotective effects of berry fruits on neurodegenerative diseases. Neural Regen. Res. 9, 1557–1566. doi: 10.4103/1673-5374.139483
Suganyadevi, P., Saravanakumar, K. M., and Mohandas, S. (2011). Identification of 3- deoxyanthocyanins from red sorghum (Sorghum bicolor) bran and its biological properties. Afr. J. Pure Appl. Chem. 5, 181–193. doi: 10.1016/j.lfs.2013.01.006
Suganyadevi, P., Saravanakumar, K. M., and Mohandas, S. (2013). The antiproliferative activity of 3-deoxyanthocyanins extracted from red sorghum (Sorghum bicolor) bran through P(53)-dependent and Bcl-2 gene expression in breast cancer cell line. Life Sci. 92, 379–382. doi: 10.1016/j.lfs.2013.01.006
Sultana, R., Ravagna, A., Mohmmad-Abdul, H., Calabrese, V., and Butterfield, D. A. (2005). Ferulic acid ethyl ester protects neurons against amyloid beta- peptide (1–42)-induced oxidative stress and neurotoxicity: relationship to antioxidant activity. J. Neurochem. 92, 749–758. doi: 10.1111/j.1471-4159.2004.02899.x
Sunil, C., and Xu, B. (2019). An insight into the health-promoting effects of taxifolin (dihydroquercetin). Phytochemistry 166:112066.
Swerdlow, R. H., Burns, J. M., and Khan, S. M. (2014). The Alzheimer’s disease mitochondrial cascade hypothesis: progress and perspectives. Biochim. Biophys. Acta 1842, 1219–1231. doi: 10.1016/j.bbadis.2013.09.010
Swerdlow, R. H. (2018). Mitochondria and mitochondrial cascades in Alzheimer’s disease. J. Alzheimer’s Dis. 62, 1403–1416. doi: 10.3233/JAD-170585
Szliszka, E., Helewski, K. J., Mizgala, E., and Krol, W. (2011). The dietary flavonol fisetin enhances the apoptosis-inducing potential of TRAIL in prostate cancer cells. Int. J. Oncol. 39, 771–779. doi: 10.3892/ijo.2011.1116
Talavéra, S., Felgines, C., Texier, O., Besson, C., Mazur, A., Lamaison, J.-L., et al. (2006). Bioavailability of a bilberry anthocyanin extract and its impact on plasma antioxidant capacity in rats. J. Sci. Food Agric. 86, 90–97. doi: 10.1186/s13568-020-01008-4
Taleon, V., Dykes, L., Rooney, W. L., and Rooney, L. W. (2012). Effect of genotype and environment on flavonoid concentration and profile of black sorghum grains. J. Cereal Sci. 56, 470–475. doi: 10.1016/j.jcs.2012.05.001
Tamagno, E., Guglielmotto, M., Monteleone, D., and Tabaton, M. (2012). Amyloid-β production: major link between oxidative stress and BACE1. Neurotox. Res. 22, 208–219. doi: 10.1007/s12640-011-9283-6
Tan, Z. S., and Vasan, R. S. (2009). Thyroid function and Alzheimer’s disease. J. Alzheimers Dis. 16, 503–507. doi: 10.3233/JAD-2009-0991
Tatsimo, S. J. N., Tamokou, J. D. D., Havyarimana, L., Csupor, D., Forgo, P., Hohmann, J., et al. (2012). Antimicrobial and antioxidant activity of kaempferol rhamnoside derivatives from Bryophyllum pinnatum. BMC Res. Notes 5:158. doi: 10.1186/1756-0500-5-158
Tawata, S., Taira, S., Kobamoto, N., Zhu, J., Ishihara, M., and Toyama, S. (2014). Synthesis and antifungal activity of cinnamic acid esters. Biosci. Biotechnol. Biochem. 60, 909–910. doi: 10.1271/bbb.60.909
Thakur, A., Chun, Y. S., October, N., Yang, H. O., and Maharaj, V. (2019). Potential of south african medicinal plants targeting the reduction of Abeta42 protein as a treatment of Alzheimer’s disease. J. Ethnopharmacol. 231, 363–373. doi: 10.1016/j.jep.2018.11.034
The National Centre for Social and Economic Modelling NATSEM (2016). Economic cost of dementia in Australia. p. 2016–2056.
Tian, S., Sun, Y., Chen, Z., Yang, Y., and Wang, Y. (2019). Functional properties of polyphenols in grains and effects of physicochemical processing on polyphenols. J. Food Qual. 2019:8. doi: 10.1155/2019/2793973
Tini, G., Scagliola, R., Monacelli, F., La Malfa, G., Porto, I., Brunelli, C., et al. (2020). Alzheimer’s disease and cardiovascular disease: a particular association. Cardiol. Res. Pract. 2020:2617970. doi: 10.1155/2020/2617970
Toda, T., Sunagawa, T., Kanda, T., Tagashira, M., Shirasawa, T., and Shimizu, T. (2011). Apple procyanidins suppress amyloid beta-protein aggregation. Biochem. Res. Int. 2011:784698. doi: 10.1155/2011/784698
Tomás-Barberán, F. A., and Clifford, M. N. (2000). Flavanones, chalcones and dihydrochalcones - nature, occurrence and dietary burden. J. Sci. Food Agric. 80, 1073–1080. doi: 10.1002/(sici)1097-0010(20000515)80:7<1073::aid-jsfa568>3.0.co;2-b
Topal, F., Nar, M., Gocer, H., Kalin, P., Kocyigit, U. M., Gülçin, I., et al. (2016). Antioxidant activity of taxifolin: an activity-structure relationship. J. Enzyme Inhib. Med. Chem. 31, 674–683. doi: 10.3109/14756366.2015.1057723
Tosto, G., Bird, T. D., Bennett, D. A., Boeve, B. F., Brickman, A. M., Cruchaga, C., et al. (2016). The role of cardiovascular risk factors and stroke in familial Alzheimer disease. JAMA Neurol. 73, 1231–1237. doi: 10.1001/jamaneurol.2016.2539
Tsao, R. (2010). Chemistry and biochemistry of dietary polyphenols. Nutrients 2, 1231–1246. doi: 10.3390/nu2121231
Tsuda, T., Horio, F., Uchida, K., Aoki, H., and Osawa, T. (2003). Dietary cyanidin 3-O-β-D-glucoside-rich purple corn color prevents obesity and ameliorates hyperglycemia in mice. J. Nutr. 133, 2125–2130. doi: 10.1093/jn/133.7.2125
Türkan, F., Taslimi, P., and Saltan, F. Z. (2019). Tannic acid as a natural antioxidant compound: discovery of a potent metabolic enzyme inhibitor for a new therapeutic approach in diabetes and Alzheimer’s disease. J. Biochem. Mol. Toxicol. 33:e22340. doi: 10.1002/jbt.22340
van Osch, L. A. D. M., Hogervorst, E., Combrinck, M., and Smith, A. D. (2004). Low thyroid-stimulating hormone as an independent risk factor for Alzheimer disease. Neurology 62, 1967–1971. doi: 10.1212/01.wnl.0000128134.84230.9f
Vanamala, J. K. P., Massey, A. R., Pinnamaneni, S. R., Reddivari, L., and Reardon, K. F. (2017). Grain and sweet sorghum (Sorghum bicolor L. Moench) serves as a novel source of bioactive compounds for human health. Crit. Rev. Food Sci. Nutr. 2017, 1–15. doi: 10.1080/10408398.2017.1344186
Varoni, E. M., Lo Faro, A. F., Sharifi-Rad, J., and Iriti, M. (2016). Anticancer molecular mechanisms of resveratrol. Front. Nutr. 3:8. doi: 10.3389/fnut.2016.00008
Vepsäläinen, S., Koivisto, H., Pekkarinen, E., Mäkinen, P., Dobson, G., McDougall, G. J., et al. (2013). Anthocyanin-enriched bilberry and blackcurrant extracts modulate amyloid precursor protein processing and alleviate behavioral abnormalities in the APP/PS1 mouse model of Alzheimer’s disease. J. Nutr. Biochem. 24, 360–370. doi: 10.1016/j.jnutbio.2012.07.006
Vepsalainen, S., Koivisto, H., Pekkarinen, E., Makinen, P., Dobson, G., McDougall, G. J., et al. (2013). Anthocyanin-enriched bilberry and blackcurrant extracts modulate amyloid precursor protein processing and alleviate behavioral abnormalities in the APP/PS1 mouse model of Alzheimer’s disease. J. Nutr. Biochem. 24, 360–370. doi: 10.1016/j.jnutbio.2012.07.006
Verdile, G., Laws, S. M., Henley, D., Ames, D., Bush, A. I., Ellis, K. A., et al. (2014). Associations between gonadotropins, testosterone and beta amyloid in men at risk of Alzheimer’s disease. Mol. Psychiatry 19, 69–75. doi: 10.1038/mp.2012.147
Verwilst, P., Kim, H. S., Kim, S., Kang, C., and Kim, J. S. (2018). Shedding light on tau protein aggregation: the progress in developing highly selective fluorophores. Chem. Soc. Rev. 47, 2249–2265. doi: 10.1039/c7cs00706j
Vessal, M., Hemmati, M., and Vasei, M. (2003). Antidiabetic effects of quercetin in streptozocin-induced diabetic rats. Comp. Biochem. Physiol. C Toxicol. Pharmacol. 135, 357–364. doi: 10.1016/s1532-0456(03)00140-6
Vion, E., Page, G., Bourdeaud, E., Paccalin, M., Guillard, J., and Rioux Bilan, A. (2018). Trans epsilon-viniferin is an amyloid-beta disaggregating and anti-inflammatory drug in a mouse primary cellular model of Alzheimer’s disease. Mol. Cell Neurosci. 88, 1–6. doi: 10.1016/j.mcn.2017.12.003
Wang, G., Chen, L., Pan, X., Chen, J., Wang, L., Wang, W., et al. (2016). The effect of resveratrol on beta amyloid-induced memory impairment involves inhibition of phosphodiesterase-4 related signaling. Oncotarget 7, 17380–17392. doi: 10.18632/oncotarget.8041
Wang, H., Wang, H., Cheng, H., and Che, Z. (2016). Ameliorating effect of luteolin on memory impairment in an Alzheimer’s disease model. Mol. Med. Rep. 13, 4215–4220. doi: 10.3892/mmr.2016.5052
Wang, J., Bi, W., Cheng, A., Freire, D., Vempati, P., Zhao, W., et al. (2014). Targeting multiple pathogenic mechanisms with polyphenols for the treatment of Alzheimer’s disease-experimental approach and therapeutic implications. Front. Aging Neurosci. 6:42. doi: 10.3389/fnagi.2014.00042
Wang, J., Santa-Maria, I., Ho, L., Ksiezak-Reding, H., Ono, K., Teplow, D. B., et al. (2010). Grape derived polyphenols attenuate tau neuropathology in a mouse model of Alzheimer’s disease. J. Alzheimers Dis. 22, 653–661. doi: 10.3233/JAD-2010-101074
Wang, Y., Wang, Q., Bao, X., Ding, Y., Shentu, J., Cui, W., et al. (2018). Taxifolin prevents β-amyloid-induced impairments of synaptic formation and deficits of memory via the inhibition of cytosolic phospholipase A2/prostaglandin E2 content. Metab. Brain Dis. 33, 1069–1079.
Wen, R., Banik, B., Pathak, R. K., Kumar, A., Kolishetti, N., and Dhar, S. (2016). Nanotechnology inspired tools for mitochondrial dysfunction related diseases. Adv. Drug Deliv. Rev. 99, 52–69. doi: 10.1016/j.addr.2015.12.024
WHO (2021). Dementia. Available online at: https://www.who.int/news-room/fact-sheets/detail/dementia.
Wong, D. Y., Musgrave, I. F., Harvey, B. S., and Smid, S. D. (2013). Acai (Euterpe oleraceae Mart.) berry extract exerts neuroprotective effects against beta-amyloid exposure in vitro. Neurosci. Lett. 556, 221–226. doi: 10.1016/j.neulet.2013.10.027
Woo, H. J., Oh, I. T., Lee, J. Y., Jun, D. Y., Seu, M. C., Woo, K. S., et al. (2012). Apigeninidin induces apoptosis through activation of Bak and Bax and subsequent mediation of mitochondrial damage in human promyelocytic leukemia HL-60 cells. Process Biochem. 47, 1861–1871. doi: 10.1016/j.procbio.2012.06.012
Xing, N., Chen, Y., Mitchell, S. H., and Young, C. Y. F. (2001). Quercetin inhibits the expression and function of the androgen receptor in LNCaP prostate cancer cells. Carcinogenesis 22, 409–414. doi: 10.1093/carcin/22.3.409
Xiong, Y., Zhang, P., Warner, R. D., and Fang, Z. (2019). Sorghum grain: from genotype, nutrition and phenolic profile to its health benefits and food applications. Compr. Rev. Food Sci. Food Saf. 18, 2025–2046. doi: 10.1111/1541-4337.12506
Xu, B., Li, X. X., He, G. R., Hu, J. J., Mu, X., Tian, S., et al. (2010). Luteolin promotes long-term potentiation and improves cognitive functions in chronic cerebral hypoperfused rats. Eur. J. Pharmacol. 627, 99–105. doi: 10.1016/j.ejphar.2009.10.038
Xu, Y.C., Yeung, D. K. Y., Man, R. Y. K., and Leung, S. W. S. (2006). Kaempferol enhances endothelium-independent and dependent relaxation in the porcine coronary artery. Mol. Cell. Biochem. 287:61. doi: 10.1007/s11010-005-9061-y
Yan, J. J., Cho, J. Y., Kim, H. S., Kim, K. L., Jung, J. S., Huh, S. O., et al. (2001). Protection against beta-amyloid peptide toxicity in vivo with long-term administration of ferulic acid. Br. J. Pharmacol. 133, 89–96. doi: 10.1038/sj.bjp.0704047
Yan, J.-J., Jung, J.-S., Kim, T.-K., Hasan, M. A., Hong, C.-W., Nam, J.-S., et al. (2013). Protective effects of ferulic acid in amyloid precursor protein plus presenilin-1 transgenic mouse model of Alzheimer disease. Biol. Pharm. Bull. 2013, 140–143. doi: 10.1248/bpb.b12-00798
Yang, L., Browning, J. D., and Awika, J. M. (2009). Sorghum 3-deoxyanthocyanins possess strong phase II enzyme inducer activity and cancer cell growth inhibition properties. J. Agric. Food Chem. 57, 1797–1804. doi: 10.1021/jf8035066
Yang, Y. J., Kim, Y. J., Yang, Y. K., Kim, J. Y., and Kwon, O. (2012). Dietary flavan-3-ols intake and metabolic syndrome risk in Korean adults. Nutr. Res. Pract. 6, 68–77. doi: 10.4162/nrp.2012.6.1.68
Yang, Z., Kuboyama, T., and Tohda, C. (2019). Naringenin promotes microglial M2 polarization and Aβ degradation enzyme expression. Phytother Res. 33, 1114–1121. doi: 10.1002/ptr.6305
Yao, J., Gao, X., Sun, W., Yao, T., Shi, S., and Ji, L. (2013). Molecular hairpin: a possible model for inhibition of tau aggregation by tannic acid. Biochemistry 52, 1893–1902. doi: 10.1021/bi400240c
Yao, L. H., Jiang, Y. M., Shi, J., Tomás-Barberán, F. A., Datta, N., Singanusong, R., et al. (2004). Flavonoids in food and their health benefits. Plant Foods Hum. Nutr. 59, 113–122. doi: 10.1007/s11130-004-0049-7
Yoshida, T., Konishi, M., Horinaka, M., Yasuda, T., Goda, A. E., Taniguchi, H., et al. (2008). Kaempferol sensitizes colon cancer cells to TRAIL-induced apoptosis. Biochem. Biophys. Res. Commun. 375, 129–133. doi: 10.1016/j.bbrc.2008.07.131
Yu, C. K., Springob, K., Schmidt, J., Nicholson, R. L., Chu, I. K., and Yip, W. K., et al. (2005). stilbene synthase gene (SbSTS1) is involved in host and nonhost defense responses in sorghum. Plant Physiol. 138, 393–401. doi: 10.1104/pp.105.059337
Yu, T.-X., Zhang, P., Guan, Y., Wang, M., and Zhen, M.-Q. (2015). Protective effects of luteolin against cognitive impairment induced by infusion of Aβ peptide in rats. Int. J. Clin. Exp. Pathol. 8, 6740–6747.
Yun, K. J., Koh, D. J., Kim, S. H., Park, S. J., Ryu, J. H., Kim, D. G., et al. (2008). Anti-inflammatory effects of sinapic acid through the suppression of inducible nitric oxide synthase, cyclooxygase-2 and proinflammatory cytokines expressions via nuclear factor-kappaB inactivation. J. Agric. Food Chem. 56, 10265–10272. doi: 10.1021/jf802095g
Zanwar, A. A., Badole, S. L., Shende, P. S., Hegde, M. V., and Bodhankar, S. L. (2014). “Chapter 21 - Antioxidant role of catechin in health and disease,” in Polyphenols in Human Health and Disease, eds R. R. Watson V. R. Preedy and S. Zibadi (San Diego: Academic Press), 267–271.
Zaplatic, E., Bule, M., Shah, S. Z. A., Uddin, M. S., and Niaz, K. (2019). Molecular mechanisms underlying protective role of quercetin in attenuating Alzheimer’s disease. Life Sci. 224, 109–119. doi: 10.1016/j.lfs.2019.03.055
Zare, K., Eidi, A., Roghani, M., and Rohani, A. H. (2015). The neuroprotective potential of sinapic acid in the 6-hydroxydopamine-induced hemi-parkinsonian rat. Metab. Brain Dis. 30, 205–213. doi: 10.1007/s11011-014-9604-6
Zhang, F., and Jiang, L. (2015). Neuroinflammation in Alzheimer’s disease. Neuropsychiatr. Dis. Treat. 11, 243–256. doi: 10.2147/NDT.S75546
Zhang, M., Swarts, S. G., Yin, L., Liu, C., Tian, Y., Cao, Y., et al. (2011). Antioxidant properties of quercetin. Adv. Exp. Med. Biol. 701, 283–289. doi: 10.1007/978-1-4419-7756-4_38
Zhang, X., Wang, G., Gurley, E. C., and Zhou, H. (2014). Flavonoid apigenin inhibits lipopolysaccharide-induced inflammatory response through multiple mechanisms in macrophages. PLoS One 9:e107072. doi: 10.1371/journal.pone.0107072
Zhang, Y., and Liu, D. (2011). Flavonol kaempferol improves chronic hyperglycemia-impaired pancreatic beta-cell viability and insulin secretory function. Eur. J. Pharmacol. 670, 325–332. doi: 10.1016/j.ejphar.2011.08.011
Zhao, L., Wang, J. L., Liu, R., Li, X. X., Li, J. F., and Zhang, L. (2013). Neuroprotective, anti-amyloidogenic and neurotrophic effects of apigenin in an Alzheimer’s disease mouse model. Molecules 18, 9949–9965. doi: 10.3390/molecules18089949
Keywords: Alzheimer’s disease, sorghum, polyphenols, antioxidant, amyloid-beta, tau, mitochondrial dysfunction, flavonoids
Citation: Rezaee N, Fernando WMADB, Hone E, Sohrabi HR, Johnson SK, Gunzburg S and Martins RN (2021) Potential of Sorghum Polyphenols to Prevent and Treat Alzheimer’s Disease: A Review Article. Front. Aging Neurosci. 13:729949. doi: 10.3389/fnagi.2021.729949
Received: 24 June 2021; Accepted: 24 August 2021;
Published: 06 October 2021.
Edited by:
Alan David Snow, Cognitive Clarity Inc., United StatesReviewed by:
Nobuyuki Kimura, National Center for Geriatrics and Gerontology (NCGG), JapanPatrizia Giannoni, University of Nîmes, France
Copyright © 2021 Rezaee, Fernando, Hone, Sohrabi, Johnson, Gunzburg and Martins. This is an open-access article distributed under the terms of the Creative Commons Attribution License (CC BY). The use, distribution or reproduction in other forums is permitted, provided the original author(s) and the copyright owner(s) are credited and that the original publication in this journal is cited, in accordance with accepted academic practice. No use, distribution or reproduction is permitted which does not comply with these terms.
*Correspondence: W.M.A.D. Binosha Fernando, dy5mZXJuYW5kb0BlY3UuZWR1LmF1