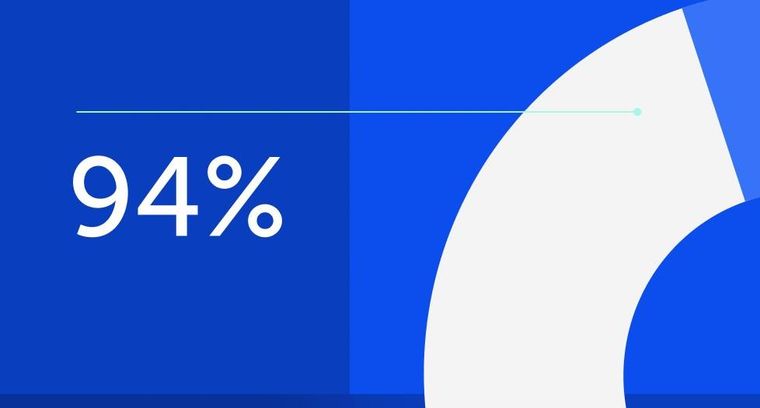
94% of researchers rate our articles as excellent or good
Learn more about the work of our research integrity team to safeguard the quality of each article we publish.
Find out more
ORIGINAL RESEARCH article
Front. Aging Neurosci., 06 October 2021
Sec. Neuroinflammation and Neuropathy
Volume 13 - 2021 | https://doi.org/10.3389/fnagi.2021.728007
This article is part of the Research TopicThe Neurovascular Unit as a Potential Biomarker and Therapeutic Target in Cerebrovascular DiseaseView all 13 articles
The mechanistic link between hypertension, diabetes and cerebral small vessel disease (CSVD) is still poorly understood. We hypothesized that hypertension and diabetes could impair cerebrovascular regulation prior to irreversibly established cerebrovascular disease. In this study, 52 hypertensive patients [54% males; age 64 ± 11 years; 58% with comorbid diabetes mellitus (DM)] without symptomatic cerebrovascular disease underwent transcranial Doppler (TCD) monitoring in the middle (MCA) and posterior (PCA) cerebral arteries, to assess vasoreactivity to carbon dioxide (VRCO2) and neurovascular coupling (NVC). 1.5T magnetic resonance imaging was also performed and white matter hyperintensity volume was automatically segmented from FLAIR sequences. TCD data from 17 healthy controls were obtained for comparison (47% males; age 60 ± 16 years). Hypertensive patients showed significant impairment of NVC in the PCA, with reduced increment in cerebral blood flow velocity during visual stimulation (22.4 ± 9.2 vs. 31.6 ± 5.7, p < 0.001), as well as disturbed NVC time-varying properties, with slower response (lower rate time: 0.00 ± 0.02 vs. 0.03 ± 6.81, p = 0.001), and reduced system oscillation (reduced natural frequency: 0.18 ± 0.08 vs. 0.22 ± 0.06, p < 0.001), when compared to controls. VRCO2 remained relatively preserved in MCA and PCA. These results were worse in hypertensive diabetic patients, with lower natural frequency (p = 0.043) than non-diabetic patients. White matter disease burden did not predict worse NVC. These findings suggest that hypertensive diabetic patients may have a precocious impairment of NVC, already occurring without symptomatic CSVD. Future research is warranted to evaluate whether NVC assessment could be useful as an early, non-invasive, surrogate marker for CSVD.
Cerebral small vessel disease (CSVD) has an enormous impact on public health worldwide (GBD 2017 Causes of Death Collaborators, 2018). It accounts for 25% of ischemic strokes and most hemorrhagic strokes and is the second leading cause for cognitive decline (Sudlow and Warlow, 1997; Iadecola et al., 2019).
Hypertension (HT) is the major vascular risk factor (VRF) for CSVD. Alongside HT, diabetes mellitus (DM) is a recognized VRF implicated in CSVD (Brundel et al., 2012; Liu et al., 2018).
While other etiologies for stroke are fairly well-studied, the pathophysiology and causality of CSVD are still poorly understood. Many of its manifestations are clinically silent until the development of clinical consequences, with stroke, cognitive decline, and gait impairment, limiting disease-specific preventive strategies (Pantoni, 2010). Also, CSVD radiological markers and clinical manifestations seem to be dissociated, for reasons not fully explained (Sorond et al., 2011; Jokumsen-Cabral et al., 2019). Biomarkers for the events predating irreversible damage could be key for better clinical management and pre-symptomatic preventive measures.
There is evidence of neurovascular dysfunction in CSVD and it may precede clinical and imaging manifestations (Wardlaw, 2010; Freeze et al., 2018; Castro et al., 2020). Very few studies have investigated neurovascular coupling (NVC) in HT, mostly using imaging modalities. Transcranial Doppler (TCD) is a non-invasive method that allows the monitoring of microvascular hemodynamic functional integrity (Claassen et al., 2016; Malojcic et al., 2017).
We aimed to study cerebrovascular regulation by TCD in hypertensive and diabetic patients without major CSVD-related impairment as a possible surrogate marker for the future development of symptomatic CSVD, to help guide therapies aimed at the cerebral microcirculation and neurovascular unit.
A cross-sectional observational study was conducted in a University Hospital. Hypertensive patients were recruited from the hospital's Hypertension Unit. Exclusion criteria were previous stroke or other significant brain pathology (dementia by clinical criteria, brain tumor, traumatic brain injury, previous cerebral infection or neurodegenerative disease), severe/unstable disease, contraindication for magnetic resonance imaging (MRI), inadequate acoustic temporal bone window, extra- or intracranial artery stenosis >50% and incapability to collaborate or to give informed consent. TCD data from healthy controls of similar age and gender were obtained from previous studies performed with the same protocol (Jokumsen-Cabral et al., 2019).
The local ethics committee approved the study protocol, which followed the tenets of the Declaration of Helsinki. Written informed consent was obtained.
Participant's clinical and demographic data were recorded. Vascular comorbidities were summarized into a vascular comorbidity score (VCS), including HT, DM, dyslipidemia, tobacco usage, chronic heart failure, coronary heart disease, arrhythmias, peripheral artery disease, and nephropathy. These conditions were scored as present (1 point) or absent (0 points), for a score ranging from 0 to 9 (Mossello et al., 2015). All participants underwent cervical and transcranial Doppler ultrasound (Philips iu22, The Nederlands) to exclude hemodynamically significant vessel pathology. The patients underwent routine 24-h ambulatory blood pressure monitoring (Spacelabs 90207, Redmond, Washington, USA). The mini-mental state examination (MMSE) and the Montreal cognitive assessment (MoCA) were used to screen for dementia. The patients were evaluated by an ophthalmologist, and all had normal binocular visual acuity, allowing for the TCD dynamic testing.
Evaluations were conducted in a dim-lighted, quiet room (≃22°C), in a supine position. Cerebral blood flow velocity (CBFV) was continuously recorded in the M1 segment of the right middle cerebral artery (MCA) and the P2 segment of the left posterior cerebral artery (PCA), with 2-MHz TCD probes secured with a headframe (Doppler BoxX, DWL, Singen, Germany), in order to simultaneously obtain data from both arterial territories (Azevedo et al., 2012). Continuous non-invasive arterial blood pressure (BP) was measured with the Finometer (FMS, Amsterdam, The Netherlands). Heart rate was assessed with a three-lead electrocardiogram. End-tidal carbon dioxide (EtCO2) was recorded by capnography (Respsense Nonin, Amsterdam, The Netherlands). Data was synchronized and digitally stored at 400 Hz with Powerlab (AD Instruments, Oxford, UK) for offline analysis. After resting for 20 min, the vasoreactivity to carbon dioxide (VRCO2) and NVC protocols were performed, as described below. CBFV envelopes were continuously registered and analyzed offline.
Participants were monitored through successive 2-min steps of resting, inhalation of a mixture of 5% CO2 and 95% O2 mixture (EtCO2 7–10 mmHg above baseline), resting (room air, until normocapnia) and hyperventilation (EtCO2 7–10 mmHg below baseline). VRCO2 was calculated as the slope of the relationship between EtCO2 average values plotted against those of relative CBFV achieved at the three stages, expressed as % mean CBFV per mmHg EtCO2 (Madureira et al., 2017).
NVC was assessed in the PCA territory by a visual paradigm consisting of 10 cycles, each with a 20s resting phase (eyes closed) and 40 s stimulating phase (flickering checkerboard) at 10 Hz (Rosengarten et al., 2001a). The 5s of stable measurement prior to stimulation were used as the baseline (Rosengarten et al., 2001a). All cycles were synchronized and averaged. Peak systolic data was used because it is less prone to artifacts (Rosengarten et al., 2001b). Maximal systolic CBFV change was obtained to calculate the overshoot parameter as × 100%.[12] The systolic CBFV curve was modeled into a second order linear system to describe the dynamics of NVC response in time according to the equation G(s) = , where “K” stands for gain, “Tv” for rate time, “ω” for natural frequency, and “ξ” for attenuation (Rosengarten et al., 2001a). All the parameters of the equation were determined by the least squares method. The sum of the squared residuals and the χ2 were also calculated to ensure the goodness of fit into the real measured values, as provided by the lsqnonlin function. Gain describes the relative CBFV difference between rest and steady-state level during visual stimulation; rate time indicates the initial steepness of the CBFV increase; natural frequency represents the oscillatory properties of the system; and attenuation describes dampening and tonus features, such as elastic properties of the vessel wall (Rosengarten et al., 2003). For this specific test, the MCA recordings were used as a control to detect non-specific changes in CBFV during the visual stimulation task.
Forty-six patients were eligible and agreed to undergo cerebral MRI (Siemens Aera 1.5T). Of these, data from 6 patients were excluded for lack of quality for the evaluations. White matter hyperintensity (WMH) volumes normalized by intracranial volume were derived from the T2-weighted fluid-attenuated inversion recovery sequences collected in the sagittal plane. Voxels resolution was 1 x 1 x 1, slices = 256, FOV = 256 mm, TR = 5000 ms, TE = 336 ms, TI = 1800 ms (Figure 1). Briefly, WMH masks were created using the Lesion Segmentation Algorithm (LPA, 1) from the Lesion Segmentation Toolbox for SPM12 in MATLAB R2018a. Following an initial segmentation of the FLAIR image, probability maps were binarized using AFNI (2,3, v21.0.15) command 3dcalc. Resulting segmentations were quality-checked for sufficient accuracy and volumes were calculated using Freesurfer (v7.1) command mri_segstats.
Figure 1. Automatic segmentation of WMH (A) Raw data; (B) Probability map of WMH; (C) Binarized results of WMH (values above 0 were considered WMH).
Additional signs of CSVD were evaluated by an experienced vascular Neurologist to further characterize the CSVD in the patient cohort. Enlarged perivascular spaces (PVS) were defined as small (<0.3 mm) punctate or linear (if perpendicular or longitudinal to the plane of the scan, respectively) hyperintensities on T2 images in the basal ganglia (BG) and centrum semiovale (CS) (Potter et al., 2015). The PVS burden was then stratified into three groups: <11, 11–20 and >20 (Lau et al., 2017). Lacunes were defined as rounded or ovoid lesions >3 mm and <20 mm in diameter in the BG, internal capsule, CS or brainsteam, with CSF density on T2 images (Wardlaw et al., 2013). Cerebral microbleeds were defined as round, hypodense lesions <10 mm on susceptibility weighted imaging collected in the axial plane (slice thickness 2 mm, slices = 256, FOV = 230 mm, TR = 49 ms, TE = 40 ms), according to the guidelines (Greenberg et al., 2009).
Normality was determined using the Shapiro–Wilk test and analysis of skewness. Data with high asymmetry was normalized using logarithmic transformation. Homogeneity of variances was tested for each analysis. Baseline characteristics were compared using the independent sample t-test and χ2-test. Mixed ANOVA or ANCOVA (for PCA) were used to compare hemodynamic data. Subgroup analyses were performed using univariate ANOVA, with Bonferroni post-hoc tests. The partial eta squared (ηp2) was used as a measure of effect size: ηp2 > 0.14 indicates a large effect, ηp2 >0.06 indicates a medium effect and ηp2 > 0.01 indicates a small effect (Cohen, 1998). Age and gender were used as covariates in the ANOVA and ANCOVA comparisons. Supplemental analysis using body mass index (BMI) and VCS as covariates (plus age and gender) were also performed. Patients were dichotomized by the median value of WMH volume to compare the results of VRCO2 and NVC in the PCA between the two WMH burden groups. Subgroups were compared using the independent sample t-test.
Values of p < 0.05 were considered significant.
Fifty-two hypertensive patients were evaluated and TCD data from 17 healthy controls were used. Baseline characteristics are reported in Table 1. Supplementary File 1 depicts the burden of PVS, microbleeds and lacunes in the patient group. Most patients had low burden of PVS in the BG (55.0%) and CS (65.0%). Nine patients (22.5%) presented lacunes and four patients (10%) presented microbleeds.
Baseline CBFV and CBFV variability (spectral power) were similar in patients and controls (Table 2). VRCO2 in either territory (PCA or MCA) was similar between groups and showed no change after adjusting for both VCS and BMI (Supplementary File 2).
Table 2. Cerebral hemodynamics, VRCO2 and NVC: patients vs. controls, controlling for age and gender.
There were no differences in VRCO2 between hypertensive non-diabetics (HT-nDM), hypertensive diabetics (HT-DM) and controls (Table 3). Those results did not change significantly after controlling for BMI or VCS (Supplementary File 3).
Table 3. Cerebral hemodynamics, VRCO2 and NVC: controls vs. HT-nDM vs. HT-DM patients, controlled for age and gender.
NVC in the PCA territory was significantly altered in HT patients, with smaller increases in CBFV during visual stimulation (p < 0.001) and disturbed NVC time-varying properties, with lower natural frequency (p < 0.001) and lower rate time (p = 0.001) (Table 2; Figure 2). These results remained similar when adjusting for BMI and VCS, although the overshoot systolic CBFV was over the limit of statistical significance when adjusting for the VCS (p = 0.065) (Supplementary File 2).
Figure 2. (A) Group-averaged evoked systolic CBFV responses during visual stimulation with the flickering checkerboard; (B) Blood pressure (BP) and heart rate (HR) during visual stimulation with the flickering checkerboard. Gray lines represent healthy controls and black lines represent HT patients (thin lines: measured responses, thick lines: modeled blood flow data).
NVC results were worse in HT-DM than HT-nDM (Table 3). For the overshoot systolic CBFV and for natural frequency, only patients with both comorbidities showed significant differences when comparing to controls. HT-DM showed lower natural frequency than non-diabetic patients (p = 0.043). When controlling for VCS, natural frequency was also worse in both HT-nDM (p = 0.020) and HT-DM (p = 0.002) when comparing to controls, with the worst results for HT-DM (HT-nDM vs. HT-DM, p=0.011) (Supplementary File 3).
Figure 3 represents the evoked systolic CBFV responses in the PCA and MCA during the visual stimulation in one HT subject, to demonstrate the individual NVC responses.
Figure 3. Evoked systolic CBFV responses in the PCA and MCA during visual stimulation with the flickering checkerboard in one HT patient. The black lines represent the PCA response (thin lines: measured responses, thick lines: modeled blood flow data) and the gray lines represent the MCA measured response, used as a control.
As shown in Table 4, VRCO2 was lower in the higher burden group in both the MCA (p = 0.004) and the PCA (p = 0.007). NVC parameters did not differ in both groups.
Our study shows that NVC was significantly impaired in hypertensive patients, with reduced CBFV increase and altered time behavior hemodynamic evoked response during visual stimulation. Moreover, NVC tended to be worse in the DM subgroup. VRCO2 remained relatively preserved. These results did not change when adjusting for other vascular risk factors. The novel finding is that the natural frequency seems to be the most sensitive parameter for discriminating abnormal NVC in these patients. Rosengarten and colleagues reported that this parameter had the lowest SD of the modeled parameters, thus having the potential for better differentiating between normal and abnormal NVC, but its capacity to identify vascular response dysfunction in disease settings needed to be studied (Rosengarten et al., 2001a). Our results seem to point toward natural frequency as a possible marker for NVC dysfunction in hypertension and diabetes.
Overall, the white matter disease (WMD) burden was low in the patient cohort. The NVC results were similar between higher and lower WMD burden groups, while higher burden patients showed worse VRCO2. It has been recently reported that reduced VRCO2 precedes the development of WMH (Sam et al., 2016), which could help explain the difference between the disease burden groups. Similar NVC with higher and lower WMH volume has been previously reported (Sorond et al., 2013). Although WMH volume predicts an increased risk of stroke and cognitive decline, the clinical expression seems to vary (Sorond et al., 2011). Moreover, silent markers of CSVD are frequently detected on the MRI of older individuals without cognitive impairment (Vernooij et al., 2009; Debette and Markus, 2010). Thus, besides macrostructural changes, other modalities reflecting microstructural integrity and function, such as TCD dynamic studies, may provide additional information to further stratify patients at risk.
Our results showed a significantly reduced PCA CBFV magnitude response and altered time behavior of reactive hyperemia during visual stimulation in HT patients, especially when diabetes was an added comorbidity. These findings indicate disturbed NVC in these patients' PCA cortical territory, independently of established WMD, as was observed by other groups (Birns et al., 2009; Purkayastha et al., 2014). The decreased CBFV during visual stimulation reflects less robust functional hyperemia, causing failure to meet the metabolic demands and neuronal damage (Iadecola et al., 2019).
The reduced overshoot systolic CBFV in the patient group was not accompanied by a reduction in the modeled parameter gain. This could reflect lack of statistical power to detect the differences between the groups, since the mean gain was lower in the patient group. In addition, it has been demonstrated that the overshoot of systolic CBFV is significantly influenced by not only gain, but also rate time and attenuation (Rosengarten et al., 2001a). Hence, the parameter gain and the overshoot systolic CBFV may not be perfectly matched.
These results are in line with studies on genetically determined CSVD. CADASIL patients demonstrated less robust functional hyperemia in the PCA during visual stimulation, with changes in time dynamics, very similar to our findings (Jokumsen-Cabral et al., 2019). Comparable NVC dysfunction in the posterior circulation was shown in Fabry patients (Azevedo et al., 2012; Castro et al., 2020). Both diseases are characterized by abnormal material deposition in the vessel walls (Baudrimont et al., 1993; Rombach et al., 2010). Interestingly, amyloid deposition also seems to cause NVC impairment (Iadecola and Davisson, 2008; Brickman et al., 2015), and HT appears to have a role in promoting amyloid deposition, thus working synergistically to worsen CSVD and cognitive decline (Iadecola and Davisson, 2008).
Chronic HT leads to structural (mal)adaptations in the cerebral circulation, with remodeling of the cerebral arteries and arterioles. This remodeling involves smooth muscle cell hypertrophy and hyperplasia, increased deposition of extracellular matrix components and degenerated smooth muscle (lipohyalinosis) and fibrinoid necrosis, leading to arterial stiffening and loss of elasticity (Iadecola and Gottesman, 2019). These changes in the proximal resistance arteries cause substantial burden on the vulnerable downstream microcirculation, promoting pressure-induced oxidative stress to the endothelial cells and neuroinflammation (Ungvari et al., 2021). In experimental models, HT results in impairment of endothelium mediated neurovascular coupling responses, in part resulting from this oxidative stress and neuroinflammation (Iadecola and Gottesman, 2019; Ungvari et al., 2021). Hence, the structural changes induced by HT play an important role in the loss of functional integrity of the neurovascular unit. Natural frequency is assumed to represent the tonus and the speed of the system (Rosengarten et al., 2003), which would be altered by the increased rigidity of the vessels and endothelial dysfunction, and our results show that this parameter was the most sensitive in differentiating patients from controls.
Less effective NVC in the MCA territory has been associated with significant cognition, balance, and walking velocity changes in the elderly (Sorond et al., 2011; Purkayastha et al., 2014). Despite the presence and burden of WMD, normal NVC was associated with preserved walking speed, while slower walking is one of the earliest manifestations of CSVD. Further prospective work could use NVC for predicting symptomatic CSVD. Curiously, cocoa and deferoxamine have been demonstrated to reverse some of these changes suggesting the possibility for pharmacological modulation of the neurovascular function (Sorond et al., 2013, 2015).
Comorbid diabetes associated with increased cerebrovascular dysfunction. NVC was worse in this subgroup of hypertensive patients, particularly in its oscillatory properties (natural frequency), in accordance to previous studies in early type 1 DM (Rosengarten et al., 2002). This might signify higher rigidity of the small arteries due to the accumulation of advanced glycated by-products. In fact, type 2 DM patients have particularly high incidence of lacunar stroke (van Harten et al., 2006; Brundel et al., 2014). Diabetes induced chronic vascular changes include not only macrovascular disorders, such as cardiovascular and cerebrovascular large vessel disease, but also microvascular disorders, with nephropathy, retinopathy and neuropathy (Chawla et al., 2016). Furthermore, studies have implicated DM as a risk factor for cognitive impairment, which may be related to CSVD. However, the mechanism by which cognitive decline occurs and whether it can be explained by dysfunction of the neurovascular unit remains to be elucidated (Mogi and Horiuchi, 2011). The sympathetic nervous system seems to attenuate the cerebrovascular response to hypercapnia, suggesting a direct effect on the cerebral vasculature (Jordan et al., 2000). NVC is also affected by autonomic dysfunction (Azevedo et al., 2011). Thus, differences in DM vs. nDM hypertensive patients could be related to DM associated dysautonomia in CSVD.
Overall, our study further supports cerebrovascular dynamics dysfunction as a major player in explaining the relationship between increased VRF burden and CSVD manifestations, independently of macroscopic white matter lesions.
We acknowledge several methodological limitations. Due to the cross-section design, our study cannot provide evidence to support cerebrovascular dysfunction as an early predictor of CSVD. However, these patients had well-controlled HT, based on average ABPM values, with no clinical manifestations of cerebrovascular disease. All the patients were referred to the Hypertension Unit due to severe or difficult to manage HT, and we do not know the duration of the untreated disease, which could impact the degree of microvascular dysfunction.
Although the patient group's sample size is relatively large for hemodynamic physiology studies, this is an exploratory study and must be validated in larger, multicenter cohorts. Also, the control group is relatively small, not exactly age- and gender-matched, and the differences in comorbidities between the two groups can be potential confounders to the observed differences. Besides HT and DM, already discussed, other vascular comorbidities have been associated with the development of CSVD. Dyslipidemia plays an important role in the development of large vessel disease and stroke, but its role in CSVD is still controversial (Tsai et al., 2018). However, animal studies have demonstrated cerebral autoregulation impairment with hyperlipidemia and a relationship between hyperlipidemia and the development of CSVD (Ayata et al., 2013; Kraft et al., 2017). Obesity has been demonstrated to have an impact in the development of CSVD (Yamashiro et al., 2014), and it has been shown to affect cerebral vasoreactivity (Selim et al., 2008). Smoking appears to worsen the effects of hypertension in the cerebral microvasculature (Hara et al., 2019), and there is impaired neurovascular coupling in the PCA of young chronic smokers (Olah et al., 2008). Chronic heart failure can affect cerebral autoregulation, reduce cerebral blood flow (CBF) and has been shown to affect NVC in the PCA (Aires et al., 2020; Ovsenik et al., 2021). The prevalence of CSVD is higher in patients with coronary artery disease (Berry et al., 2019), and it has been identified as an independent risk factor for vascular dementia (Gorelick et al., 2011). It was recently demonstrated that aTrial fibrillation reduces cerebral autoregulation and impairs neurovascular coupling responses to visual stimulation (Junejo et al., 2020). There is evidence that peripheral artery disease is associated with white matter disease and the development of vascular dementia (Bots et al., 1993; Gorelick et al., 2011). In patients with ischemic stroke, impaired renal function correlated with worse dCA and associated with an increased risk of hemorrhagic transformation (Castro et al., 2018). However, in addition to adjusting for age and gender, adjusting for the vascular comorbidities did not modify the results significantly.
Hypertension affects cerebral small vessels heterogeneously, and MRI seems the logical choice for its superior spatial resolution. However, MRI VRCO2 protocols are more prone to failure (Moreton et al., 2018), expensive and not as standardized as TCD (Malojcic et al., 2017). Moreover, TCD offers extraordinary time resolution (~5 ms) for studying the time behavior of CBFV activation in downstream cortical microvasculature. There are also inherent limitations for continuous blood flow monitoring by TCD, namely, providing a measure of blood flow velocity and not flow. However, the former is an adequate surrogate for the latter as long as the insonated artery diameter remains constant. Since data were acquired in a supine position and relied on spontaneous measurements, changes in artery diameter are not anticipated for NVC. Nevertheless, it has been demonstrated that with increasing partial pressure of arterial CO2 there is noticeable increase in vessel diameter, which could lead to underestimation of cerebral blood flow in the VRCO2 protocol (Coverdale et al., 2014).
We did not report the presence of cortical microinfarcts (CMI) in this study, despite having been found in cohorts of hypertensive patients (although not consistently) and despite their importance in cognitive decline (van Veluw et al., 2017). We did not detect CMI upon visual inspection of the MRI scans. However, the protocols for detecting CMI are mostly validated in 3T MRI scanners (Coverdale et al., 2014), and our 1.5T scan protocol is underpowered for their detection.
In conclusion, neurovascular coupling, and more specifically the modeled parameter natural frequency, seems to be particularly affected in hypertension and diabetes and could be useful as an early biomarker for microvascular dysfunction, future irreversible vascular damage, and cognitive decline. Additionally, our study supports TCD dynamic tests as useful tools for better understating microvascular damage associated with these diseases, but future research is warranted to confirm these results.
The raw data supporting the conclusions of this article may be made available by the authors upon request, without undue reservation.
The studies involving human participants were reviewed and approved by Comissão de Ética da U. L. S. Matosinhos, Hospital de Pedro Hispano, EPE, Unidade Local de Saúde de Matosinhos, Portugal. The patients/participants provided their written informed consent to participate in this study.
AM: conception and design, data collection, literature search, drafting the manuscript, and critical revision of the manuscript. PC: conception and design, data collection, critical revision of the manuscript, and supervision. GP, CF, and JP: data collection and critical revision of the manuscript. FS, AM, and JH: critical revision of the manuscript. EA: conception and design, critical revision of the manuscript, and supervision. All authors contributed to the article and approved the submitted version.
This study received financial support from Associação para o Estudo das Doenças Neurovasculares for performing MRI studies.
The authors declare that the research was conducted in the absence of any commercial or financial relationships that could be construed as a potential conflict of interest.
All claims expressed in this article are solely those of the authors and do not necessarily represent those of their affiliated organizations, or those of the publisher, the editors and the reviewers. Any product that may be evaluated in this article, or claim that may be made by its manufacturer, is not guaranteed or endorsed by the publisher.
We would like to acknowledge the support received from Associação para o Estudo das Doenças Neurovasculares and we wish to thank the participants who volunteered to be part of this study.
The Supplementary Material for this article can be found online at: https://www.frontiersin.org/articles/10.3389/fnagi.2021.728007/full#supplementary-material
Aires, A., Andrade, A., Azevedo, E., Gomes, F., Araujo, J. P., and Castro, P. (2020). Neurovascular coupling impairment in heart failure with reduction ejection fraction. Brain Sci. 10:714. doi: 10.3390/brainsci10100714
Ayata, C., Shin, H. K., Dilekoz, E., Atochin, D. N., Kashiwagi, S., Eikermann-Haerter, K., et al. (2013). Hyperlipidemia disrupts cerebrovascular reflexes and worsens ischemic perfusion defect. J. Cereb. Blood Flow Metab. 33, 954–962. doi: 10.1038/jcbfm.2013.38
Azevedo, E., Castro, P., Santos, R., Freitas, J., Coelho, T., Rosengarten, B., et al. (2011). Autonomic dysfunction affects cerebral neurovascular coupling. Clin. Auton. Res. 21, 395–403. doi: 10.1007/s10286-011-0129-3
Azevedo, E., Mendes, A., Seixas, D., Santos, R., Castro, P., Ayres-Basto, M., et al. (2012). Functional transcranial doppler: presymptomatic changes in Fabry disease. Eur. Neurol. 67, 331–337. doi: 10.1159/000337906
Baudrimont, M., Dubas, F., Joutel, A., Tournier-Lasserve, E., and Bousser, M. G. (1993). Autosomal dominant leukoencephalopathy and subcortical ischemic stroke. A clinicopathological study. Stroke 24, 122–125. doi: 10.1161/01.STR.24.1.122
Berry, C., Sidik, N., Pereira, A. C., Ford, T. J., Touyz, R. M., Kaski, J. C., et al. (2019). Small-vessel disease in the heart and brain: current knowledge, unmet therapeutic need, and future directions. J. Am. Heart Assoc. 8:e011104. doi: 10.1161/JAHA.118.011104
Birns, J., Jarosz, J., Markus, H. S., and Kalra, L. (2009). Cerebrovascular reactivity and dynamic autoregulation in ischaemic subcortical white matter disease. J. Neurol. Neurosurg. Psychiatry 80, 1093–1098. doi: 10.1136/jnnp.2009.174607
Bots, M. L., van Swieten, J. C., Breteler, M. M., de Jong, P. T., van Gijn, J., Hofman, A., et al. (1993). Cerebral white matter lesions and atherosclerosis in the rotterdam study. Lancet 341, 1232–1237. doi: 10.1016/0140-6736(93)91144-B
Brickman, A. M., Guzman, V. A., Gonzalez-Castellon, M., Razlighi, Q., Gu, Y., Narkhede, A., et al. (2015). Cerebral autoregulation, beta amyloid, and white matter hyperintensities are interrelated. Neurosci. Lett. 592, 54–58. doi: 10.1016/j.neulet.2015.03.005
Brundel, M., Kappelle, L. J., and Biessels, G. J. (2014). Brain imaging in type 2 diabetes. Eur. Neuropsychopharmacol. 24, 1967–1981. doi: 10.1016/j.euroneuro.2014.01.023
Brundel, M., van den Berg, E., Reijmer, Y. D., de Bresser, J., Kappelle, L. J., Biessels, G. J., et al. (2012). Cerebral haemodynamics, cognition and brain volumes in patients with type 2 diabetes. J. Diabetes Complications 26, 205–209. doi: 10.1016/j.jdiacomp.2012.03.021
Castro, P., Azevedo, E., Rocha, I., Sorond, F., and Serrador, J. M. (2018). Chronic kidney disease and poor outcomes in ischemic stroke: is impaired cerebral autoregulation the missing link? BMC Neurol. 18:21. doi: 10.1186/s12883-018-1025-4
Castro, P., Gutierres, M., Pereira, G., Ferreira, S., Oliveira, J. P., and Azevedo, E. (2020). Evaluation of cerebral microvascular regulatory mechanisms with transcranial doppler in fabry disease. Brain Sci 10:528. doi: 10.3390/brainsci10080528
Chawla, A., Chawla, R., and Jaggi, S. (2016). Microvasular and macrovascular complications in diabetes mellitus: distinct or continuum? Indian J. Endocrinol. Metab. 20, 546–551. doi: 10.4103/2230-8210.183480
Claassen, J. A., Meel-van den Abeelen, A. S., Simpson, D. M., Panerai, R. B., and International Cerebral Autoregulation Research, N. (2016). Transfer function analysis of dynamic cerebral autoregulation: a white paper from the International cerebral autoregulation research network. J. Cereb. Blood Flow Metab. 36, 665–680. doi: 10.1177/0271678X15626425
Cohen, J. (1998). Statistical Power Analysis for the Behavioral Sciences, 2nd Edn. Hillsdale, NJ: Erlbaum.
Coverdale, N. S., Gati, J. S., Opalevych, O., Perrotta, A., and Shoemaker, J. K. (2014). Cerebral blood flow velocity underestimates cerebral blood flow during modest hypercapnia and hypocapnia. J. Appl. Physiol. 117, 1090–1096. doi: 10.1152/japplphysiol.00285.2014
Debette, S., and Markus, H. S. (2010). The clinical importance of white matter hyperintensities on brain magnetic resonance imaging: systematic review and meta-analysis. BMJ 341:c3666. doi: 10.1136/bmj.c3666
Freeze, W. M., Jacobs, H. I. L., Schreuder, F., van Oostenbrugge, R. J., Backes, W. H., Verhey, F. R., et al. (2018). Blood-brain barrier dysfunction in small vessel disease related intracerebral hemorrhage. Front. Neurol. 9:926. doi: 10.3389/fneur.2018.00926
GBD 2017 Causes of Death Collaborators (2018). Global, regional, and national age-sex-specific mortality for 282 causes of death in 195 countries and territories, 1980-2017: a systematic analysis for the Global Burden of Disease Study 2017. Lancet 392, 1736–1788. doi: 10.1016/S0140-6736(18)32203-7
Gorelick, P. B., Scuteri, A., Black, S. E., Decarli, C., Greenberg, S. M., Iadecola, C., et al. (2011). Vascular contributions to cognitive impairment and dementia: a statement for healthcare professionals from the american heart association/american stroke association. Stroke 42, 2672–2713. doi: 10.1161/STR.0b013e3182299496
Greenberg, S. M., Vernooij, M. W., Cordonnier, C., Viswanathan, A., Al-Shahi Salman, R., Warach, S., et al. (2009). Cerebral microbleeds: a guide to detection and interpretation. Lancet Neurol. 8, 165–174. doi: 10.1016/S1474-4422(09)70013-4
Hara, M., Yakushiji, Y., Suzuyama, K., Nishihara, M., Eriguchi, M., Noguchi, T., et al. (2019). Synergistic effect of hypertension and smoking on the total small vessel disease score in healthy individuals: the Kashima scan study. Hypertens. Res. 42, 1738–1744. doi: 10.1038/s41440-019-0282-y
Iadecola, C., and Davisson, R. L. (2008). Hypertension and cerebrovascular dysfunction. Cell Metab. 7, 476–484. doi: 10.1016/j.cmet.2008.03.010
Iadecola, C., Duering, M., Hachinski, V., Joutel, A., Pendlebury, S. T., Schneider, J. A., et al. (2019). Vascular cognitive impairment and dementia: JACC scientific expert panel. J. Am. Coll. Cardiol. 73, 3326–3344. doi: 10.1016/j.jacc.2019.04.034
Iadecola, C., and Gottesman, R. F. (2019). Neurovascular and cognitive dysfunction in hypertension. Circ. Res. 124, 1025–1044. doi: 10.1161/CIRCRESAHA.118.313260
Jokumsen-Cabral, A., Aires, A., Ferreira, S., Azevedo, E., and Castro, P. (2019). Primary involvement of neurovascular coupling in cerebral autosomal-dominant arteriopathy with subcortical infarcts and leukoencephalopathy. J. Neurol. 266, 1782–1788. doi: 10.1007/s00415-019-09331-y
Jordan, J., Shannon, J. R., Diedrich, A., Black, B., Costa, F., Robertson, D., et al. (2000). Interaction of carbon dioxide and sympathetic nervous system activity in the regulation of cerebral perfusion in humans. Hypertension 36, 383–388. doi: 10.1161/01.HYP.36.3.383
Junejo, R. T., Braz, I. D., Lucas, S. J., van Lieshout, J. J., Phillips, A. A., Lip, G. Y., et al. (2020). Neurovascular coupling and cerebral autoregulation in atrial fibrillation. J. Cereb. Blood Flow Metab. 40, 1647–1657. doi: 10.1177/0271678X19870770
Kraft, P., Schuhmann, M. K., Garz, C., Jandke, S., Urlaub, D., Mencl, S., et al. (2017). Hypercholesterolemia induced cerebral small vessel disease. PLoS ONE 12:e0182822. doi: 10.1371/journal.pone.0182822
Lau, K. K., Li, L., Schulz, U., Simoni, M., Chan, K. H., Ho, S. L., et al. (2017). Total small vessel disease score and risk of recurrent stroke: validation in 2 large cohorts. Neurology 88, 2260–2267. doi: 10.1212/WNL.0000000000004042
Liu, J., Rutten-Jacobs, L., Liu, M., Markus, H. S., and Traylor, M. (2018). Causal impact of type 2 diabetes mellitus on cerebral small vessel disease: a mendelian randomization analysis. Stroke 49, 1325–1331. doi: 10.1161/STROKEAHA.117.020536
Madureira, J., Castro, P., and Azevedo, E. (2017). Demographic and systemic hemodynamic influences in mechanisms of cerebrovascular regulation in healthy adults. J. Stroke Cerebrovasc. Dis. 26, 500–508. doi: 10.1016/j.jstrokecerebrovasdis.2016.12.003
Malojcic, B., Giannakopoulos, P., Sorond, F. A., Azevedo, E., Diomedi, M., Oblak, J. P., et al. (2017). Ultrasound and dynamic functional imaging in vascular cognitive impairment and Alzheimer's disease. BMC Med. 15:27. doi: 10.1186/s12916-017-0799-3
Mogi, M., and Horiuchi, M. (2011). Neurovascular coupling in cognitive impairment associated with diabetes mellitus. Circ. J. 75, 1042–1048. doi: 10.1253/circj.CJ-11-0121
Moreton, F. C., Cullen, B., Delles, C., Santosh, C., Gonzalez, R. L., Dani, K., et al. (2018). Vasoreactivity in CADASIL: comparison to structural MRI and neuropsychology. J. Cereb. Blood Flow Metab. 38, 1085–1095. doi: 10.1177/0271678X17710375
Mossello, E., Pieraccioli, M., Nesti, N., Bulgaresi, M., Lorenzi, C., Caleri, V., et al. (2015). Effects of low blood pressure in cognitively impaired elderly patients treated with antihypertensive drugs. JAMA Intern. Med. 175, 578–585. doi: 10.1001/jamainternmed.2014.8164
Olah, L., Raiter, Y., Candale, C., Molnar, S., Rosengarten, B., Bornstein, N. M., et al. (2008). Visually evoked cerebral vasomotor response in smoking and nonsmoking young adults, investigated by functional transcranial doppler. Nicotine Tob. Res. 10, 353–358. doi: 10.1080/14622200701825874
Ovsenik, A., Podbregar, M., and Fabjan, A. (2021). Cerebral blood flow impairment and cognitive decline in heart failure. Brain Behav. 11:e02176. doi: 10.1002/brb3.2176
Pantoni, L. (2010). Cerebral small vessel disease: from pathogenesis and clinical characteristics to therapeutic challenges. Lancet Neurol. 9, 689–701. doi: 10.1016/S1474-4422(10)70104-6
Potter, G. M., Chappell, F. M., Morris, Z., and Wardlaw, J. M. (2015). Cerebral perivascular spaces visible on magnetic resonance imaging: development of a qualitative rating scale and its observer reliability. Cerebrovasc. Dis. 39, 224–231. doi: 10.1159/000375153
Purkayastha, S., Fadar, O., Mehregan, A., Salat, D. H., Moscufo, N., Meier, D. S., et al. (2014). Impaired cerebrovascular hemodynamics are associated with cerebral white matter damage. J. Cereb. Blood Flow Metab. 34, 228–234. doi: 10.1038/jcbfm.2013.180
Rombach, S. M., Twickler, T. B., Aerts, J. M., Linthorst, G. E., Wijburg, F. A., and Hollak, C. E. (2010). Vasculopathy in patients with Fabry disease: current controversies and research directions. Mol. Genet. Metab. 99, 99–108. doi: 10.1016/j.ymgme.2009.10.004
Rosengarten, B., Aldinger, C., Kaufmann, A., and Kaps, M. (2001b). Comparison of visually evoked peak systolic and end diastolic blood flow velocity using a control system approach. Ultrasound Med. Biol. 27, 1499–1503. doi: 10.1016/S0301-5629(01)00464-1
Rosengarten, B., Budden, C., Osthaus, S., and Kaps, M. (2003). Effect of heart rate on regulative features of the cortical activity-flow coupling. Cerebrovasc. Dis. 16, 47–52. doi: 10.1159/000070115
Rosengarten, B., Dost, A., Kaufmann, A., Gortner, L., and Kaps, M. (2002). Impaired cerebrovascular reactivity in type 1 diabetic children. Diabetes Care 25, 408–410. doi: 10.2337/diacare.25.2.408-a
Rosengarten, B., Huwendiek, O., and Kaps, M. (2001a). Neurovascular coupling in terms of a control system: validation of a second-order linear system model. Ultrasound Med. Biol. 27, 631–635. doi: 10.1016/S0301-5629(01)00355-6
Sam, K., Crawley, A. P., Conklin, J., Poublanc, J., Sobczyk, O., Mandell, D. M., et al. (2016). Development of white matter hyperintensity is preceded by reduced cerebrovascular reactivity. Ann. Neurol. 80, 277–285. doi: 10.1002/ana.24712
Selim, M., Jones, R., Novak, P., Zhao, P., and Novak, V. (2008). The effects of body mass index on cerebral blood flow velocity. Clin. Auton. Res. 18, 331–338. doi: 10.1007/s10286-008-0490-z
Sorond, F. A., Hurwitz, S., Salat, D. H., Greve, D. N., and Fisher, N. D. (2013). Neurovascular coupling, cerebral white matter integrity, and response to cocoa in older people. Neurology 81, 904–909. doi: 10.1212/WNL.0b013e3182a351aa
Sorond, F. A., Kiely, D. K., Galica, A., Moscufo, N., Serrador, J. M., Iloputaife, I., et al. (2011). Neurovascular coupling is impaired in slow walkers: the MOBILIZE Boston study. Ann. Neurol. 70, 213–220. doi: 10.1002/ana.22433
Sorond, F. A., Tan, C. O., LaRose, S., Monk, A. D., Fichorova, R., Ryan, S., et al. (2015). Deferoxamine, cerebrovascular hemodynamics, and vascular aging: potential role for hypoxia-inducible transcription factor-1-regulated pathways. Stroke 46, 2576–2583. doi: 10.1161/STROKEAHA.115.009906
Sudlow, C. L., and Warlow, C. P. (1997). Comparable studies of the incidence of stroke and its pathological types: results from an international collaboration. International Stroke Incidence Collaboration. Stroke 28, 491–499. doi: 10.1161/01.STR.28.3.491
Tsai, H. H., Kim, J. S., Jouvent, E., and Gurol, M. E. (2018). Updates on prevention of hemorrhagic and lacunar strokes. J. Stroke 20, 167–179. doi: 10.5853/jos.2018.00787
Ungvari, Z., Toth, P., Tarantini, S., Prodan, C. I., Sorond, F., Merkely, B., et al. (2021). Hypertension-induced cognitive impairment: from pathophysiology to public health. Nat. Rev. Nephrol. 1–16. doi: 10.1038/s41581-021-00430-6
van Harten, B., de Leeuw, F. E., Weinstein, H. C., Scheltens, P., and Biessels, G. J. (2006). Brain imaging in patients with diabetes: a systematic review. Diabetes Care 29, 2539–2548. doi: 10.2337/dc06-1637
van Veluw, S. J., Shih, A. Y., Smith, E. E., Chen, C., Schneider, J. A., Wardlaw, J. M., et al. (2017). Detection, risk factors, and functional consequences of cerebral microinfarcts. Lancet Neurol. 16, 730–740. doi: 10.1016/S1474-4422(17)30196-5
Vernooij, M. W., Ikram, M. A., Vrooman, H. A., Wielopolski, P. A., Krestin, G. P., Hofman, A., et al. (2009). White matter microstructural integrity and cognitive function in a general elderly population. Arch. Gen. Psychiatry 66, 545–553. doi: 10.1001/archgenpsychiatry.2009.5
Wardlaw, J. M. (2010). Blood-brain barrier and cerebral small vessel disease. J. Neurol. Sci. 299, 66–71. doi: 10.1016/j.jns.2010.08.042
Wardlaw, J. M., Smith, E. E., Biessels, G. J., Cordonnier, C., Fazekas, F., Frayne, R., et al. (2013). Neuroimaging standards for research into small vessel disease and its contribution to ageing and neurodegeneration. Lancet Neurol. 12, 822–838. doi: 10.1016/S1474-4422(13)70124-8
Keywords: hypertension, diabetes mellitus, neurovascular coupling (NVC), transcranial doppler (TCD), cerebral small vessel disease
Citation: Monteiro A, Castro P, Pereira G, Ferreira C, Sorond F, Milstead A, Higgins JP, Polónia J and Azevedo E (2021) Neurovascular Coupling Is Impaired in Hypertensive and Diabetic Subjects Without Symptomatic Cerebrovascular Disease. Front. Aging Neurosci. 13:728007. doi: 10.3389/fnagi.2021.728007
Received: 20 June 2021; Accepted: 31 August 2021;
Published: 06 October 2021.
Edited by:
Anusha Mishra, Oregon Health and Science University, United StatesReviewed by:
Eszter Farkas, University of Szeged, HungaryCopyright © 2021 Monteiro, Castro, Pereira, Ferreira, Sorond, Milstead, Higgins, Polónia and Azevedo. This is an open-access article distributed under the terms of the Creative Commons Attribution License (CC BY). The use, distribution or reproduction in other forums is permitted, provided the original author(s) and the copyright owner(s) are credited and that the original publication in this journal is cited, in accordance with accepted academic practice. No use, distribution or reproduction is permitted which does not comply with these terms.
*Correspondence: Ana Monteiro, dXAyMDEzMDg2ODlAdXAucHQ=
Disclaimer: All claims expressed in this article are solely those of the authors and do not necessarily represent those of their affiliated organizations, or those of the publisher, the editors and the reviewers. Any product that may be evaluated in this article or claim that may be made by its manufacturer is not guaranteed or endorsed by the publisher.
Research integrity at Frontiers
Learn more about the work of our research integrity team to safeguard the quality of each article we publish.