- 1Shanghai Key Laboratory of Psychotic Disorders, Shanghai Mental Health Center, Shanghai Jiao Tong University School of Medicine, Shanghai, China
- 2The Clinical Hospital of Chengdu Brain Science Institute, MOE Key Lab for Neuroinformation, Center for Information in Medicine, School of Life Sciences and Technology, University of Electronic Science and Technology of China, Chengdu, China
- 3Clinical Neurocognitive Research Center, Shanghai Key Laboratory of Psychotic Disorders, Shanghai Mental Health Center, Shanghai Jiao Tong University School of Medicine, Shanghai, China
- 4Department of Geriatric Psychiatry, Shanghai Changning Mental Health Center, Shanghai, China
- 5Institute of Brain-Intelligence Technology, Zhangjiang Lab, Shanghai, China
- 6Institute of Psychology and Behavioral Science, Shanghai Jiao Tong University, Shanghai, China
- 7Center for Excellence in Brain Science and Intelligence Technology (CEBSIT), Chinese Academy of Sciences, Shanghai, China
- 8Brain Science and Technology Research Center, Shanghai Jiao Tong University, Shanghai, China
The entorhinal cortex (EC) plays an essential role in age-related cognitive decline. However, the effect of functional connectivity (FC) changes between EC and other cerebral cortices on cognitive function remains unclear. The aim of this study was to explore the modulation of two interventions (cognitive training and aerobic exercise) on EC-FC in community-dwelling older adults. In total, 94 healthy older adults aged between 65 and 75 years were assigned to either the cognitive training or aerobic exercise group to receive 24 sessions over 12 weeks, or to a control group. Resting-state functional magnetic resonance imaging was performed at both baseline and 12-month follow-up. Compared to the cognitive training group, the aerobic exercise group showed greater EC-FC in the bilateral middle temporal gyrus, right supramarginal gyrus, left angular gyrus, and right postcentral gyrus. Compared to the control group, the cognitive training group had a decreased EC-FC in the right hippocampus, right middle temporal gyrus, left angular gyrus, and right postcentral gyrus and an increased EC-FC in the bilateral pallidum, while the aerobic exercise group showed increased EC-FC between the right medial prefrontal cortex(mPFC), bilateral pallidum, and right precuneus. Baseline EC-FC in the mPFC was positively correlated with the visuospatial/constructional index score of the Repeatable Battery for the Assessment of Neuropsychological Status. In the cognitive training group, EC-FC value changes in the right hippocampus were negatively correlated with changes in the RBANS delayed memory index score, while in the aerobic exercise group, EC-FC value changes in the left angular gyrus were positively correlated with changes in the RBANS attention index score. These findings support the hypothesis that both cognitive training and aerobic exercise can modulate EC-FC in aging populations but through different neural pathways.
Introduction
Aging is accompanied with a decline in cognitive functions such as attention, processing speed, executive function, memory and reasoning (Drag and Bieliauskas, 2010). The aging of the global population has increased the prevalence of dementia, which is caused by diseases such as Alzheimer’s disease (AD), which imposes huge healthcare burdens on society and decreases the quality of life of older adults. Therefore, it is urgent to explore the pathophysiological mechanism of cognitive aging and identify effective interventions to delay the aging process. Although aging is accompanied with an alteration in the brain structure and function, increasing numbers of studies have shown that the aging brain maintains an impressive level of plasticity (Park and Reuter-Lorenz, 2009; Bherer, 2015).
Cognitive training is a promising approach to delay age-related cognitive decline (Kelly et al., 2014). Previous studies have demonstrated that cognitive training can improve memory, visual reasoning, visuospatial construction and attention in community-dwelling older adults (Mahncke et al., 2006; Cheng et al., 2012). The activity of memory-related regions, such as the frontal lobe, parietal lobe and bilateral hippocampus, is increased after cognitive training in healthy older adults and patients with mild cognitive impairment (MCI) (Belleville et al., 2011). Cognitive training increased functional connectivity (FC) between the right posterior cingulate cortex (PCC) and the left precuneus, superior parietal and temporal cortices (De Marco et al., 2016). Aerobic exercise is also reportedly beneficial for improving cognitive function, such as motor function, processing speed, and auditory and visual attention (Angevaren et al., 2008). Aerobic exercise primarily affects areas of the brain that are vulnerable to neurodegeneration, including the frontal, temporal and parietal lobes, the hippocampus/parahippocampal region, precuneus, anterior cingulate gyrus, and prefrontal cortex (PFC) (Haeger et al., 2019). Changes in body fitness, hippocampal perfusion, and hippocampal volume are positively related to changes in recognition memory and early recall for complex spatial objects (Maass et al., 2015). Aerobic fitness is also associated with an elevated executive function, which is mediated by an increase in the gray matter volume in the PFC (Weinstein et al., 2012). A 12-week moderate-intensity walking exercise can increase FC of the PCC/precuneus in individuals with MCI (Chirles et al., 2017). In summary, both cognitive training and aerobic exercise can improve cognitive function, while the intrinsic mechanism of why older adults can benefit from cognitive training and aerobic exercise remains to be determined (Owen et al., 2010; ten Brinke et al., 2015).
Located within the ventromedial portion of the temporal lobe, the human’s entorhinal cortex (EC) has long been suggested as one of the major structures significantly related to cognitive function (Coutureau and Di Scala, 2009). Previous studies found that the EC-hippocampal (HPC) circuit plays a vital role in the formation and recall of memory, preserving both spatial information and temporal information about past events (Marks et al., 2020). EC lesions disrupt a variety of functions, including attention, working memory and spatial cognition, some of which are not affected by hippocampal lesions (Oswald et al., 2001; Winters and Bussey, 2005). Such dissociations suggest that the EC may not be a pure relay station between the cortex and the hippocampus (Coutureau and Di Scala, 2009). A previous study investigating EC’s FC (termed as “EC-FC”) in patients with vascular MCI (VaMCI) revealed that VaMCI subjects showed a significantly decreased FC between the right EC and the right inferior frontal gyrus, right middle frontal gyrus, bilateral precentral gyri, right postcentral gyrus, and right superior parietal lobule. These results suggest that the EC-FC changes and their correlations with neuropsychological measures may aid in the early diagnosis of cognitive decline in the aging process (Zuo et al., 2018). EC, as a crucial cognitive function-related region, together with its associated large-scale human brain cognitive FC network, could play an important role in normative and pathologic aging and may serve as an important imaging marker for therapeutic effect on aging.
In this study, we explored the long-term effects of cognitive training and aerobic exercise on EC-FC by using seed-based FC analysis. We previously observed that the plasticity of the white mater was modified after training at the 12-month follow-up (Cao et al., 2016b). We hypothesized that prominent EC-FC changes could be found after cognitive training or aerobic exercise. In addition, cognitive training mainly improves memory, and aerobic exercise has a positive effect on attention, processing speed, and executive function (Smith et al., 2010; van Balkom et al., 2020), which might be attributed to the fact that these interventions enhance the corresponding cognition domain through different neural pathways. Therefore, we hypothesized that cognitive training and aerobic exercise modulate EC-FC through different neural circuits. Furthermore, our previous studies found a correlation between training-derived improvement in global cognition and increased FC between the central executive network after cognitive training (Cao et al., 2016a). The reduced FC between the left lateral occipital cortex and right superior temporal gyrus was associated with improved executive function after aerobic exercise (Hsu et al., 2018). Above all, we hypothesized that training-related alterations in EC-FC would be correlated with improvements in cognitive performance.
Methods
Participants
The present study was conducted using a subsample derived from our previous trial focusing on behavioral gains from cognitive training or aerobic exercise in healthy older adults, using the same methods of recruitment, eligibility criteria, randomization, intervention and outcome assessment. The original trial employed a prospective, block-randomized, assessor-blinded, controlled and parallel design (registration number: ChiCTR-TRC-13004788). 94 participants were recruited from 18 “Juwei” (a sub-district neighborhood administration) in the Jing’an district (central area) in Shanghai, China. Eligibility criteria were as follows: age 65–75 years; education ≥ 1 year; no difficulties with hearing, vision, or communication; no severe physical disease or psychotic disorder; and no obvious cognitive decline (a score on the Chinese version of the Mini-Mental State Examination ≥ 19). Exclusion criteria included the following: obvious cognitive decline such as AD; history or clinical evidence of neurological disease or psychiatric disorders such as brain cancer, major depressive disorder, or schizophrenia. This study was approved by the Human Research Ethics Committees of Shanghai Mental Health Center, China (approval number: 2013-40). Written informed consent was obtained from the participants after the study procedures had been explained to them.
Interventions
The eligible participants were randomly assigned to three groups: the cognitive training group, aerobic exercise group and control group. Cognitive training comprised of memory exercises (including verbal memory, episodic memory, and face-name association memory), reasoning, problem-solving/strategy, and visuospatial ability (map reading). Each session lasted 60 min. Participants in the aerobic exercise group began with a 10-min brisk walk and gradually increased their walking time by 5 min each week until a total of 45 min is accomplished. Before and after each brisk walk, participants performed a five-minute stretch exercise to warm up and cool down. Participants wore a wrist heart rate monitor (Mio Alpha, Physical Enterprises Inc.) during brisk walking to monitor heart rate changes in real time. Both intervention groups received a total quorum of 24 sessions over 12 weeks and all three groups attended lectures on healthy living monthly during the first 3 months and every 6 weeks thereafter until the 12-month follow-up.
Clinical Measurements
All measurements were conducted at baseline and 12 months after the intervention. Immediate memory (list learning and story memory), visuospatial/constructional (figure copy and line orientation), language (picture naming and semantic fluency), attention (digit span and coding), and delayed memory (list recall, list recognition, story recall and figure recall) index scores were obtained from the Repeatable Battery for the Assessment of Neuropsychological Status (RBANS; Form A and Form B), which has been verified in terms of its good reliability and validity in Chinese community-dwelling older adults (Cheng et al., 2011). According to the manual, four points should be added to the original score of semantic fluency of version B to achieve equivalence as version B is more difficult than version A (Randolf, 1998). The total scale index score of the RBANS represents global cognition. The visual reasoning test score reflected reasoning ability. The Stroop Color Word Test (SCWT) was used to measure cognitive inhibition and executive function, while the Color Trials Test (CTT) was used to measure processing speed, sequencing and mental flexibility, and visual-motor skills (Cao et al., 2016b).
Magnetic Resonance Imaging (MRI) Acquisition
Magnetic Resonance Imaging data was collected on a Siemens 3.0-Tesla Magnetom Verio scanner (Siemens Medical, Erlangen, Germany) at Shanghai Mental Health Center. Images were obtained using a standard 12-channel head coil. High-resolution T1-weighted anatomical images (repetition time [TR] = 1900 ms, echo time [TE] = 3.43 ms, flip angle = 9°, 160 transverse slices, field of view (FOV) = 240 × 240 mm2, matrix size = 256 × 256, slice thickness = 1 mm, voxel size = 0.9 × 0.9 × 1.0 mm3) were acquired using a magnetization prepared rapid gradient-echo sequence. Resting-state functional MRI (rs-fMRI) data were acquired using a single-shot, gradient-recalled echo planar imaging sequence (TR = 2000 ms, TE = 25 ms, flip angle = 90°, 32 transverse slices, FOV = 240 × 240 mm2, matrix size = 64 × 64, slice thickness = 5 mm). For each subject, a total of 155 volumes were acquired, resulting in a total scan time of 310 s. The subjects were instructed to close their eyes and asked to refrain from thinking about anything and be awake. During the scan, foam padding was used to minimize head motion and earplugs were used to reduce scanner noise for each participant. After the scan, the technicians evaluated the quality of the structural images. If any abnormalities were found in the images, the participants would undergo rescanning. All the MRI data were collected at baseline and 12 months after the interventions.
Rs-fMRI Pre-processing
Rs-fMRI data were pre-processed and analyzed using the Statistical Parametric Mapping software (SPM12)1 and the Data Processing Assistant for Resting-FMRI (DPARSF V 4.32). For the rs-fMRI data, the first five functional images were discarded to reduce the fluctuation of MRI signals during the initial stages of scanning. Then, the slice-timing, head motion correction, normalization by DARTEL and spatial smoothing (with an isotropic Gaussian kernel of a full-width-at-half-maximum (FWHM) of 8 mm) were performed. Besides, the irrelevant signals including mean white matter signal, mean cerebrospinal fluid (CSF) signal, 24 head motion parameters, and the linear trend were regressed out by using a multiple linear regression model. To reduce physiological interference, a band-pass filter (0.01 – 0.1 Hz) was applied on all functional images. Moreover, subjects with head motion exceeding 3 mm in the translation or 3° in rotation along any direction were discarded.
Seed-Based FC Analysis
The bilateral EC regions of interest (ROIs) were selected as seed regions according to a connectivity-based parcellation brain atlas (Fan et al., 2016), with the labels #115 and #116 in the brain atlas being located at the left and right EC, respectively. The blood oxygenation level-dependent rs-fMRI time series of the voxels within the bilateral EC ROIs was extracted and then averaged across the voxels, resulting in a EC seed time series. For each subject, an FC map was obtained by calculating the Pearson correlation between the seed’s time series and the other voxel’s time series within the brain. Moreover, a Fisher’s R-to-Z transformation was performed to enhance the normality of the resultant data.
Statistical Analysis
The statistical software package SPSS 17.0 (IBM Corporation, Somer, NY, United States) was used to compare demographic and neuropsychological measures. One-way analysis of variance (ANOVA), the non-parametric Kruskal-Wallis test and the Chi-squared test were performed to compare baseline characteristics between participants among the three groups. The general linear model (GLM) repeated measure analysis was used to investigate the effect on cognitive performance after the interventions. The model included the main effect for the time and group and a time × group interaction term.
Cohen’s d, as a measure of effect sizes of the t-test for means, was calculated using G∗Power 3.1.9.3 (©Franz Faul, Edgar Erdfelder, Albert-Georg Lang, and Axel Buchner, 2006, 2009, Faul et al., 2007) and the confidence intervals of Cohen’s d were calculated by JASP Version 0.9 (JASP Team [2021]. JASP [Version 0.9] [computer software]). The net effect size (NES) was used to compare the cognitive measures at the 12-month post-test to baseline scores and control group scores. Bias-corrected NES of the intervention group was defined as: [(intervention mean at 12-month post-test - intervention mean at baseline) - (control mean at 12-month post-test - control mean at baseline)] ÷ pooled standard deviation at baseline, before applying a bias correction factor 1–3÷[4∗(nI + nc-2)-1] (Morris, 2007). Cohen defines d-values of 0.2, 0.5 and 0.8 as small, medium and large effects, respectively (Faul et al., 2007).
To evaluate the training effect on EC-FC, a voxel-wise 3 (between – subject factor: cognitive training, aerobic exercise and control groups) × 2 (within – subject factor: baseline and 12 months after the interventions) repeated analysis of covariance (ANCOVA) was performed by controlling the age, sex and years of education. The calculation of ANCOVA was limited on a union mask, which generated from the union set of the one-sample t-test results of EC-FC in each group. Then, between-groups 2 (cognitive training and control group; aerobic exercise and control group; cognitive training and aerobic exercise) × 2 (baseline and 12 months after the interventions) ANCOVA were performed in the regions with significant interaction effect of 3 × 2 ANCOVA as the post hoc test. These analyses were calculated by SPM 12, and p < 0.005 was considered statistically significant. Furthermore, the two-sample t-test was used to compare differences in EC-FC to explore the between-group differences, and paired t-test was carried out to clarify the effect of training on EC-FC for each group.
The regions with a significant interaction effect were extracted as the ROIs for the correlation analysis. Pearson correlations were conducted to evaluate the relationship between the EC-FC values in these ROIs and neuropsychological assessments at baseline after controlling for age, sex and years of education. To evaluate whether the changed EC-FC was related to the altered cognitive performance, the Pearson correlation analysis was also conducted between the changes of EC-FC in the ROIs with a significant interaction effect and the alteration of neuropsychological assessments. The neuropsychological assessments were chosen according to the NES results, the cognition outcomes which indicated a positive effect after the interventions when compared with the control group were included into the correlation analysis.
Results
Demographic and Neuropsychological Tests
A flow chart of the participant selection and inclusion procedures during the entire study is illustrated in Figure 1. Among the 539 eligible individuals who were contacted for participation from November 2013 to September 2014, 172 were included in the MRI subsample. Among them, 151 individuals subsequently completed the MRI scans; the remaining 21 were excluded due to the following: metal-related concerns, claustrophobia, history of recent scanning, conflicts of schedule, and poor health. Between baseline and a 12-month post-scan, 9 participants withdrew their consent from the study, 13 participants refused the scanning and the remaining 14 were excluded because of conflicts of schedule and history of recent scanning. Moreover, 21 participants were excluded from the statistical analyses because of abnormal MRI findings (n = 8), excessive head movements(n = 2), mixed handedness(n = 8), or lack of baseline scanning(n = 3). Overall, a total of 94 right-handed participants were used in our analysis (28 in the cognitive training group, 34 in the aerobic exercise group, and 32 in the control group). Demographic characteristics and neuropsychological scores are described in Table 1. There were no significant differences in the demographic data (age, sex, years of education) or any cognitive performance across the three groups at baseline.
Cognitive Performance at 1 Year After the Interventions
Table 2 shows the main effect of time, group and time × group interaction in terms of cognitive measures at the 12-month post-test. There were no significant time × group interactions in any cognitive performance across the three groups at 1 year after the interventions. Table 3 lists the effect size (Cohen’s d) of each group and the NES of the cognitive training and aerobic exercise groups on each cognitive measure at the 12-month post-test follow-up. The NES of cognitive training showed a small positive effect of training on the RBANS attention index score (NES = 0.343; 95% CI, 0.293∼0.387) and the RBANS delayed memory index score (NES = 0.237; 95% CI, 0.136∼0.295), while that of aerobic exercise had a small positive impact on the RBANS attention index score (NES = 0.316; 95% CI, 0.306∼0.330) and the RBANS visuospatial/constructional index score (NES = 0.239; 95% CI, 0.208∼0.271). The NES of cognitive training also showed a small to medium negative effect of training on the RBANS language index score (NES = −0.361; 95% CI, −0.374∼−0.365) and the SCWT color interfere time (NES = 0.562; 95% CI, 0.487∼−0.630), while that of aerobic exercise had a small to medium negative impact on the RBANS immediate memory index score (NES = −0.266; 95% CI, −0.322∼−0.214) and the SCWT color interfere time (NES = 0.336; 95% CI, 0.324∼−0.352).
Interaction Effect in EC-FC
The result of the interaction effect (group × time) in the mixed model analysis is shown in Figure 2. The 3 × 2 rANCOVA revealed significant interaction (p < 0.005) effects for EC-FC in some brain regions, mainly the right hippocampus, bilateral middle temporal gyrus, bilateral pallidum, right mPFC, right supramarginal gyrus, left angular gyrus and right postcentral gyrus. The post hoc test, 2 × 2 rANCOVA among the cognitive training and control group, showed significant interaction effects on the right hippocampus, bilateral middle temporal gyrus, bilateral pallidum, right supramarginal gyrus and left angular gyrus. In addition, the 2 × 2 rANCOVA among the aerobic exercise and control group showed significant interaction effects on the right hippocampus, right middle temporal gyrus, right mPFC, bilateral pallidum and right precuneus. We also performed the 2 (cognitive training and aerobic exercise) × 2 rANCOVA, and the significant interaction results were located on the bilateral middle temporal gyrus, left supramarginal gyrus, and left angular gyrus.
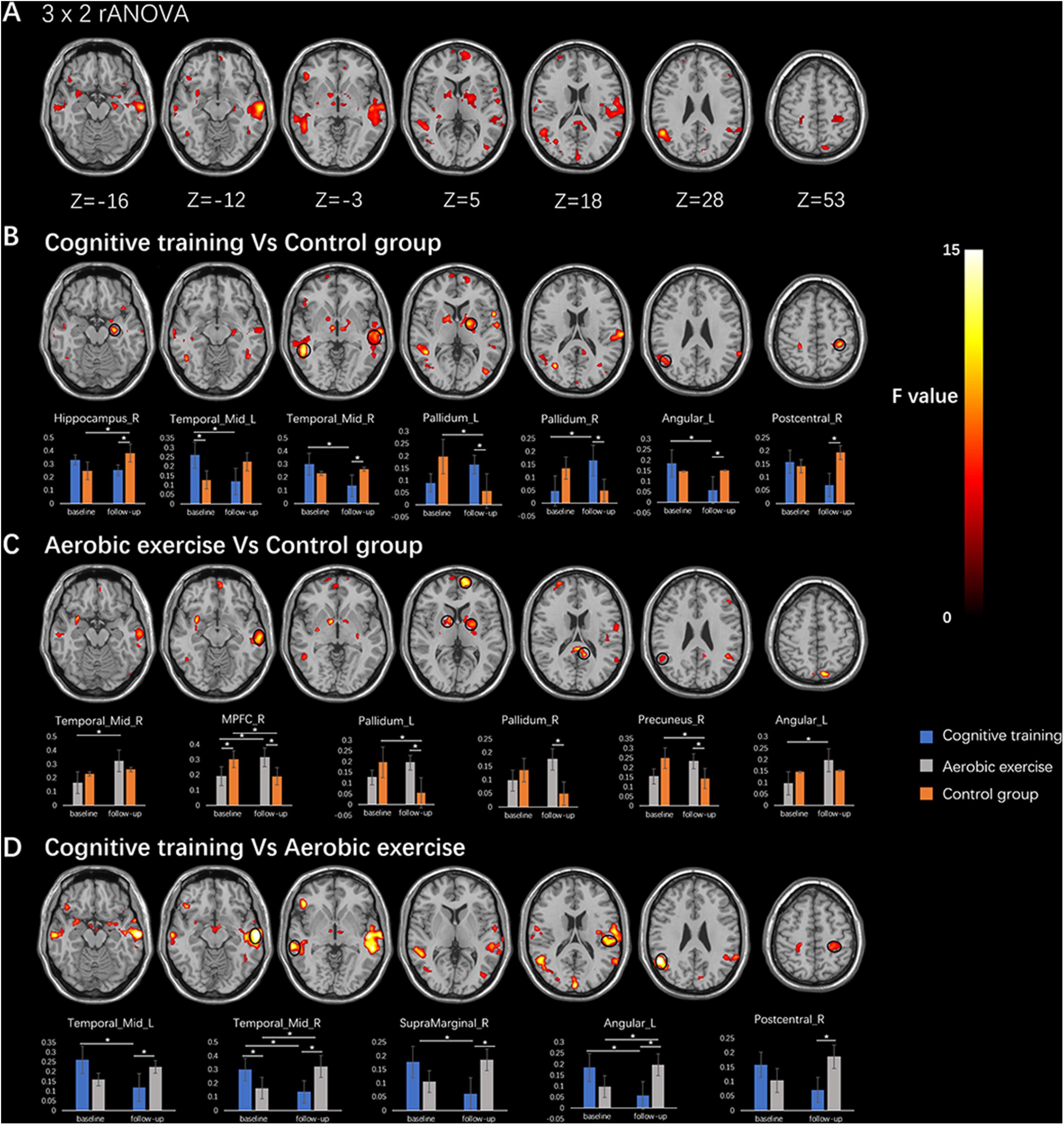
Figure 2. The results of rANCOVA in the entorhinal cortex functional connectivity (EC-FC). (A) The significant interaction effects of 3 × 2 rANCOVA were shown in the first line. (B) The significant interaction results of 2 × 2 rANCOVA among cognitive training and control group were shown in the second line, and the bar chart in the third line showed the results of the post hoc test within group/between-group. (C) The 2 × 2 rANCOVA among aerobic exercise and control group were shown in the fourth line, and the bar chart in the fifth line showed the results of the post hoc test within group/between-group. (D) The 2 × 2 rANCOVA among the cognitive training and aerobic exercise were shown in the sixth line, and the bar chart in the seventh line showed the results of the post hoc test within group/between-group. All interaction results showed p < 0.005, and * represented p < 0.05. The black circles showed the location of the ROIs.
Furthermore, the cognitive training increased the connectivity between the EC and right pallidum, and decreased the connectivity between the EC and bilateral middle temporal gyrus, left supramarginal gyrus, and left angular gyrus. The connectivity between the EC and right middle temporal gyrus, right mPFC, and left angular gyrus were increased after aerobic exercise. In the control group, the increased connectivity between EC and right hippocampus was found after 12 months, with the decreased connectivity located on the right mPFC, left pallidum, and right precuneus gyrus. Compared to the control group, the cognitive training group showed a decreased EC-FC in the right hippocampus, right middle temporal gyrus, left angular gyrus, and right postcentral gyrus, and an increased EC-FC in the bilateral pallidum; the aerobic exercise group showed an increased EC-FC in the right mPFC, bilateral pallidum, and right precuneus. Interestingly, the cognitive training group showed a lower EC-FC in the bilateral middle temporal gyrus, right supramarginal, left angular gyrus, and right postcentral gyrus than the aerobic exercise group after training, and the cognitive training group did not show an increased EC-FC compared to the aerobic exercise group.
Correlation Results
The RBANS visuospatial/constructional index score showed a positive correlation with EC-FC in the right mPFC at baseline (r = 0.24, p = 0.02, no correction), after controlling for sex and years of education.
We explored the relationships between the longitudinal EC-FC value changes and changes in the neuropsychological assessments (3 cognitive outcomes: the RBANS delayed memory index score, the RBANS attention index score, the RBANS visuospatial/constructional index score) by extracting the values of EC-FC rs-fMRI from the cluster showing significant time × group interaction (9 ROIs). As shown in Figure 3, in the cognitive training group, EC-FC value changes with the right hippocampus negatively correlated with the change in the RBANS delayed memory index score (r = −0.54, p = 0.005, corrected for multiple comparisons [FDR corrected, q<0.05]). In the aerobic exercise group, EC-FC value changes with the left angular gyrus positively correlated with the change in the RBANS attention index score (r = 0.38, p = 0.04, no correction).
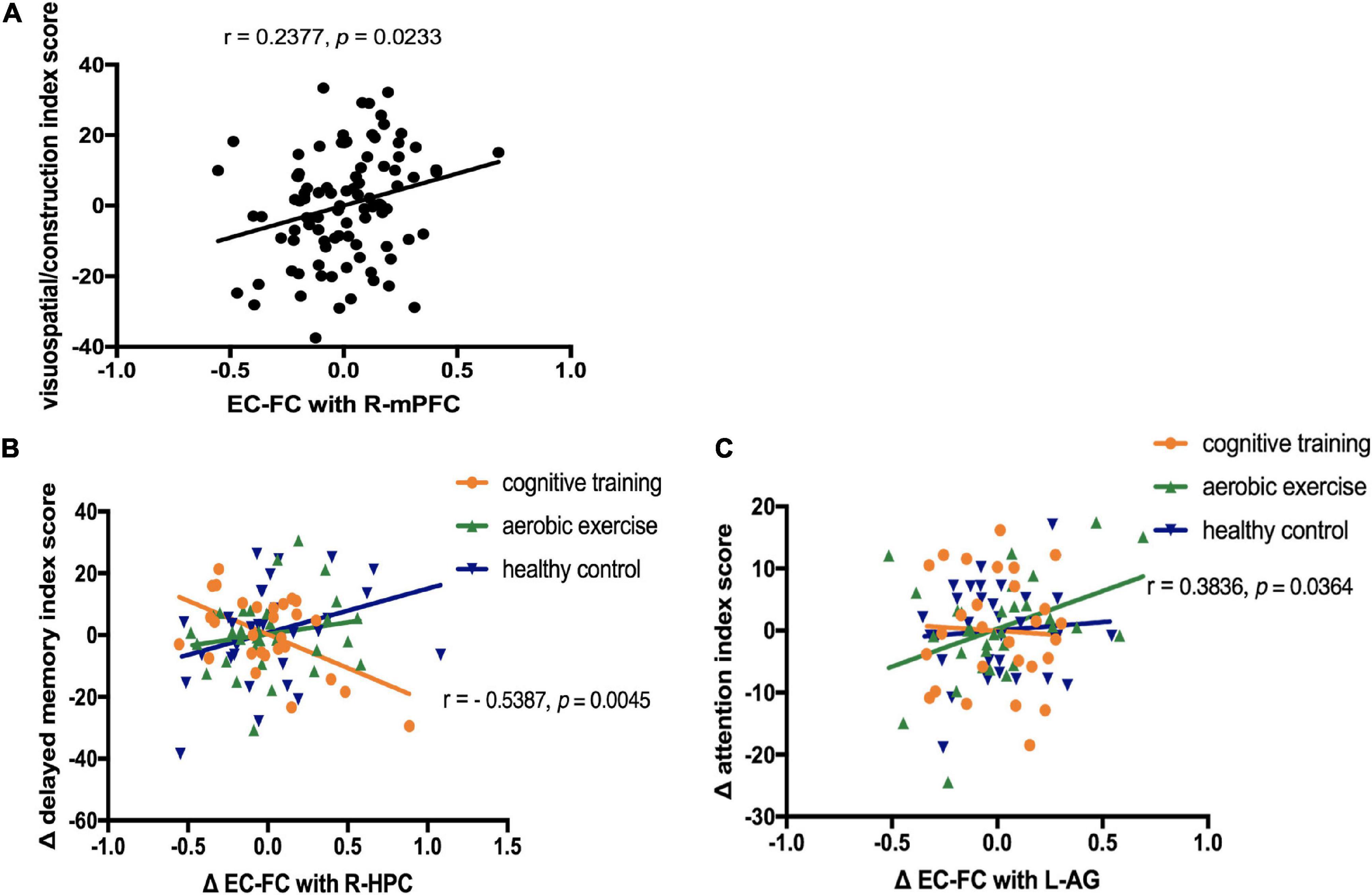
Figure 3. Correlation between the neuropsychological assessments and entorhinal cortex functional connectivity (EC-FC) values. (A) Linear correlation between the EC-FC values with the right medial prefrontal cortex(R-mPFC) and the visuospatial/constructional index score of the Repeatable Battery for the Assessment of Neuropsychological Status (RBANS) at baseline (r = 0.24, p = 0.02, no correction). (B) Linear correlation between the EC-FC value changes with the right hippocampus (R-HPC) and the change in the RBANS delayed memory index score in the cognitive training group [r = −0.54, p = 0.005, corrected for multiple comparisons (FDR, q<0.05)]. (C) Linear correlation between the EC-FC value changes with the left angular gyrus (L-AG) and the change in the RBANS attention index score in the aerobic exercise group (r = 0.38, p = 0.04, no correction).
Discussion
A series of studies focusing on the positive effect of cognitive training or aerobic exercise have shown that the community-dwelling older adults can maintain cognitive improvement for at least 1 year after the interventions (Wolinsky et al., 2006; Lautenschlager et al., 2008). Cognitive training and aerobic exercise can induce plastic changes in the neural FC in healthy older adults, and these changes may underlie the positive effects of these two interventions (Chirles et al., 2017). Our study showed that compared to the cognitive training group, the aerobic exercise group had a significant increase in the EC-FC with the bilateral middle temporal gyrus/right supramarginal gyrus/left angular gyrus/right postcentral gyrus. Compared to the control group, the cognitive training group showed a decreased EC-FC in the right hippocampus/right middle temporal gyrus/left angular gyrus/right postcentral gyrus and an increased EC-FC in the bilateral pallidum, while the aerobic exercise group showed an increased EC-FC in the right mPFC/bilateral pallidum/right precuneus. The right mPFC showed a significant positive correlation between baseline EC-FC and RBANS visuospatial/constructional index score. In the cognitive training group, the right hippocampus showed a significant negative correlation between the EC-FC value changes and the change in the RBANS delayed memory index score. In the aerobic exercise group, EC-FC value changes with the left angular gyrus positively correlated with the change in the RBANS attention index score.
The abnormality in EC has been implicated in the early stage of AD (Khan et al., 2014). Both animal and human studies have demonstrated the indispensable role of EC in object memory and spatial memory (Chao et al., 2016; Shimada et al., 2017; Butler et al., 2019; Yeung et al., 2019). We found that the significant time × group interaction effect in EC-FC was detectable even at 12 months after the intervention, which implies that both cognitive training and aerobic exercise have long-term effects on neural plasticity changes in healthy older adults. Compared to the control group, the cognitive training group showed a decreased EC-FC in the right hippocampus/right middle temporal gyrus/left angular gyrus. EC cells may demonstrate a heterogeneous pattern of activity, which may be capable of driving the activity of HPC and thus contribute to memory encoding (Marks et al., 2020). The middle temporal gyrus contributes to the controlled retrieval of conceptual knowledge, while the angular gyrus is critical for the efficient automatic retrieval of specific semantic information (Davey et al., 2015). The expectation behind cognitive training is that it may improve cognitive capacity and thus increase neural efficiency by decreasing the demands on the neural system when completing the same task (Park and Bischof, 2013). The decreased EC-FC in our findings implies that cognitive training may improve neural efficiency and reduce resource utilization; therefore, the demands on the right hippocampus, right middle temporal gyrus, and left angular gyrus could be decreased for memory encoding and semantic retrieval. In addition, we found that the aerobic exercise group showed an increased EC-FC in the right mPFC and right precuneus when compared with those of the control group. These brain areas are associated with verbal memory and attention (Leon et al., 2010; Andrews-Hanna et al., 2014). These results indicate that aerobic exercise may have an influence on cognitive domains such as memory and attention mediated by the EC. The preceding evidence indicates that cognitive training and aerobic exercise affect brain structure and function as well as cognition via distinct mechanisms (Suo et al., 2016). In the present study, we found that aerobic exercise resulted in an increased rsFC between the EC and bilateral middle temporal gyrus, right supramarginal gyrus, left angular gyrus and right postcentral gyrus compared to the cognitive training group, which is partially consistent with the neuroplasticity changes in the bilateral middle temporal gyrus, right supramarginal gyrus, and right postcentral gyrus when following long-term aerobic exercise (Voss et al., 2013; Hsu et al., 2018; Tarumi et al., 2021). Overall, our results suggest that although both cognitive training and aerobic exercise can improve cognitive performance, the two may exert their neurological effects through different neural mechanisms.
Our results showed a significant positive correlation between baseline EC-FC with the right mPFC and the RBANS visuospatial/constructional index score among healthy older adults. RBANS visuospatial/constructional domain include the Rey-Osterrieth Complex Figure Test(copy) and the visual judgments of line orientation, which reflects visuospatial capacities (Winstanley et al., 2003; Schaadt et al., 2016). Previous studies have demonstrated that mPFC and EC are required for spatial cognition (Leon et al., 2010; Meister and Buffalo, 2018). Our results indicate that the EC-mPFC circuit may be involved in spatial cognition by mainly influencing visuospatial capacities among community-dwelling older adults. In the cognitive training group, we found that the right hippocampus showed a significant negative correlation between the EC-FC value changes and the change of RBANS delayed memory index score. In addition, EC-FC with the right hippocampus was decreased after 12 weeks of cognitive training when compared with the control group. The RBANS delayed memory index score included delayed recall of words, story and figure and the delayed recognition of words, which involved the assessment of episodic memory. Episodic memory tends to be sensitive to age, as older adults often show a reduced recall in most episodic memory tasks (Bherer, 2015). It has been reported that patients with MCI could benefit from cognitive training in episodic memory (Li et al., 2011). The encoding and retrieval of episodic memory is believed to be directly and indirectly related to the entorhinal-hippocampus(EC-HPC) circuit (Roy et al., 2017). Taken together, our results suggest that cognitive training can improve episodic memory in healthy older adults by decreasing the rsFC between the hippocampus and EC. This finding is consistent with a previous study showing that cognitive training could bolster neural efficiency in older adults (Iordan et al., 2020), which indicates that older adults may decrease the demand for the EC-HPC circuit after cognitive training as a result of the enhanced neural processing speed. We found that the EC-FC value changes in the left angular gyrus correlated positively with the change in the RBANS attention index score in the aerobic exercise group, and the EC-FC with the left angular gyrus was increased after 12 weeks of exercise. Our findings are consistent with those of previous studies where EC was associated with spatial attention (Wilming et al., 2018). Moreover, it has been reported that angular exhibits widespread patterns of FC with the temporal cortex, the default mode network, and other regions involved in perception, attention and spatial cognition (Andrews-Hanna et al., 2014). The results indicate that aerobic exercise may improve attention by strengthening the connection between the EC and the left angular gyrus.
In addition, we also observed several negative NES in behavioral performance, such as the RBANS language index score in the cognitive training group and the RBANS immediate memory index score in the aerobic exercise group. The results may be caused by the fluctuation of the cognitive level for the individuals at baseline and 1-year follow-up.
There are several limitations in the present study. First, it was limited by the small sample size. Based on the behavioral effect size of the present study, we calculated the required sample size by G-power. The results showed that the optimal sample size is 270, which could detect significant difference. Therefore, it may lack the statistical power to detect the time × group interactions in cognitive performance among the three groups. Second, although several correlation analyses could not reach the significant level after corrected for multiple comparisons, the correlations could reflect the tendency that the changed EC-FC was related to the altered cognitive performance. To further verify the results, large sample studies combined rs-MRI with other multimodal magnetic resonance are needed in the future. Moreover, although the training and social contacts of the two intervention groups were almost equal, using the active control or the social control group would be better for controlling the psychosocial factors of the group-based interventions (Miller et al., 2012). Besides, the absence of immediate MRI scans after the 3-month interventions were completed directly led to the lack of short-term training-related MRI changes, which might have helped us to further understand the underlying neural mechanism of the interventions. Future research should focus on further investigating the short-term and long-term effects of training on cognitive gain and neuroplasticity changes. Finally, fMRI tasks such as the N-back task could be performed in older adults for better detection of the functional activity of the brain regions during the cognitive performance.
In general, our results demonstrate that cognitive training and aerobic exercise significantly modulate the resting-state FC of EC through different pathways over a 12-month follow-up period. Older adults may gain benefits in episodic memory and attention from cognitive training and aerobic exercise, and the underlying mechanism involves the EC-FC with the right hippocampus and the left angular gyrus separately. Clarifying the neural regulation mechanism of cognitive training and aerobic exercise on EC-FC may help in developing new interventions to prevent age-related diseases, such as MCI and AD.
Data Availability Statement
The raw data supporting the conclusions of this article will be made available by the authors, without undue reservation.
Ethics Statement
The studies involving human participants were reviewed and approved by the Human Research Ethics Committees of Shanghai Mental Health Center, China. The patients/participants provided their written informed consent to participate in this study. Written informed consent was obtained from the individual(s) for the publication of any potentially identifiable images or data included in this article.
Author Contributions
NG and HL analyzed the clinical and fMRI data and was responsible for writing the publication. CLi and XC designed the study and collected the data. TL and LJ collected the data. HZ and BZ analyzed the fMRI data and revised the manuscript. CLi and CLu are the guarantors of this work and took responsibility for the integrity of the data and the accuracy of the data analysis. All authors contributed to the article and approved the submitted version.
Funding
This work was supported by the Key Program of Clinical Research Center in Shanghai Mental Health Center (CRC2017ZD01); the National Key R&D Program of China (Grant nos. 2018YFC2001605 and 2020YFC2003000); Shanghai Municipal Science and Technology Major Project (No. 2018SHZDZX03) and ZJLab; and Shanghai Clinical Research Center for Mental Health (19MC1911100).
Conflict of Interest
The authors declare that the research was conducted in the absence of any commercial or financial relationships that could be construed as a potential conflict of interest.
Acknowledgments
The authors thank other members of the research group: Yan Cheng, Wei Feng, Yikang Zhu, Huiru Cui, Wei Li, and Hongyan Wang who contributed to the data collection.
Supplementary Material
The Supplementary Material for this article can be found online at: https://www.frontiersin.org/articles/10.3389/fnagi.2021.655245/full#supplementary-material
Footnotes
References
Andrews-Hanna, J. R., Smallwood, J., and Spreng, R. N. (2014). The default network and self-generated thought: component processes, dynamic control, and clinical relevance. Ann. N. Y. Acad. Sci. 1316, 29–52. doi: 10.3389/fnana.2017.00030
Angevaren, M., Aufdemkampe, G., Verhaar, H. J., Aleman, A., and Vanhees, L. (2008). Physical activity and enhanced fitness to improve cognitive function in older people without known cognitive impairment. Cochrane Database Syst. Rev. 3:Cd005381. doi: 10.1002/14651858.CD005381.pub3
Belleville, S., Clément, F., Mellah, S., Gilbert, B., Fontaine, F., and Gauthier, S. (2011). Training-related brain plasticity in subjects at risk of developing Alzheimer’s disease. Brain 134, 1623–1634. doi: 10.1093/brain/awr037
Bherer, L. (2015). Cognitive plasticity in older adults: effects of cognitive training and physical exercise. Ann. N. Y. Acad. Sci. 1337, 1–6. doi: 10.1111/nyas.12682
Butler, W. N., Hardcastle, K., and Giocomo, L. M. (2019). Remembered reward locations restructure entorhinal spatial maps. Science 363, 1447–1452. doi: 10.1126/science.aav5297
Cao, W., Cao, X., Hou, C., Li, T., Cheng, Y., Jiang, L., et al. (2016a). Effects of cognitive training on resting-state functional connectivity of default mode, salience, and central executive networks. Front. Aging Neurosci. 8:70. doi: 10.3389/fnagi.2016.00070
Cao, X., Yao, Y., Li, T., Cheng, Y., Feng, W., Shen, Y., et al. (2016b). The impact of cognitive training on cerebral white matter in community-dwelling elderly: one-year prospective longitudinal diffusion tensor imaging study. Sci. Rep. 6:33212. doi: 10.1038/srep33212
Chao, O. Y., Huston, J. P., Li, J. S., Wang, A. L., and de Souza Silva, M. A. (2016). The medial prefrontal cortex-lateral entorhinal cortex circuit is essential for episodic-like memory and associative object-recognition. Hippocampus 26, 633–645. doi: 10.1002/hipo.22547
Cheng, Y., Wu, W., Feng, W., Wang, J., Chen, Y., Shen, Y., et al. (2012). The effects of multi-domain versus single-domain cognitive training in non-demented older people: a randomized controlled trial. BMC Med. 10:30. doi: 10.1186/1741-7015-10-30
Cheng, Y., Wu, W., Wang, J., Feng, W., Wu, X., and Li, C. (2011). Reliability and validity of the repeatable battery for the assessment of neuropsychological status in community-dwelling elderly. Arch. Med. Sci. 7, 850–857. doi: 10.5114/aoms.2011.25561
Chirles, T. J., Reiter, K., Weiss, L. R., Alfini, A. J., Nielson, K. A., and Smith, J. C. (2017). Exercise training and functional connectivity changes in mild cognitive impairment and healthy elders. J. Alzheimers Dis. 57, 845–856. doi: 10.3233/jad-161151
Coutureau, E., and Di Scala, G. (2009). Entorhinal cortex and cognition. Prog. Neuro. Psychopharmacol. Biol. Psychiatry 33, 753–761. doi: 10.1016/j.pnpbp.2009.03.038
Davey, J., Cornelissen, P. L., Thompson, H. E., Sonkusare, S., Hallam, G., Smallwood, J., et al. (2015). Automatic and controlled semantic retrieval: TMS reveals distinct contributions of posterior middle temporal gyrus and angular gyrus. J. Neurosci. 35, 15230–15239. doi: 10.1523/jneurosci.4705-14.2015
De Marco, M., Meneghello, F., Duzzi, D., Rigon, J., Pilosio, C., and Venneri, A. (2016). Cognitive stimulation of the default-mode network modulates functional connectivity in healthy aging. Brain Res. Bull. 121, 26–41. doi: 10.1016/j.brainresbull.2015.12.001
Drag, L. L., and Bieliauskas, L. A. (2010). Contemporary review 2009: cognitive aging. J. Geriatr. Psychiatry Neurol. 23, 75–93. doi: 10.1177/0891988709358590
Fan, L., Li, H., Zhuo, J., Zhang, Y., Wang, J., Chen, L., et al. (2016). The human brainnetome atlas: a new brain atlas based on connectional architecture. Cereb. Cortex 26, 3508–3526. doi: 10.1093/cercor/bhw157
Faul, F., Erdfelder, E., Lang, A. G., and Buchner, A. (2007). G∗Power 3: a flexible statistical power analysis program for the social, behavioral, and biomedical sciences. Behav. Res. Methods 39, 175–191. doi: 10.3758/bf03193146
Haeger, A., Costa, A. S., Schulz, J. B., and Reetz, K. (2019). Cerebral changes improved by physical activity during cognitive decline: a systematic review on MRI studies. Neuroimage Clin. 23:101933. doi: 10.1016/j.nicl.2019.101933
Hsu, C. L., Best, J. R., Davis, J. C., Nagamatsu, L. S., Wang, S., Boyd, L. A., et al. (2018). Aerobic exercise promotes executive functions and impacts functional neural activity among older adults with vascular cognitive impairment. Br. J. Sports Med. 52, 184–191. doi: 10.1136/bjsports-2016-096846
Iordan, A. D., Cooke, K. A., Moored, K. D., Katz, B., Buschkuehl, M., Jaeggi, S. M., et al. (2020). Neural correlates of working memory training: evidence for plasticity in older adults. Neuroimage 217:116887. doi: 10.1016/j.neuroimage.2020.116887
Kelly, M. E., Loughrey, D., Lawlor, B. A., Robertson, I. H., Walsh, C., and Brennan, S. (2014). The impact of cognitive training and mental stimulation on cognitive and everyday functioning of healthy older adults: a systematic review and meta-analysis. Ageing Res. Rev. 15, 28–43. doi: 10.1016/j.arr.2014.02.004
Khan, U. A., Liu, L., Provenzano, F. A., Berman, D. E., Profaci, C. P., Sloan, R., et al. (2014). Molecular drivers and cortical spread of lateral entorhinal cortex dysfunction in preclinical Alzheimer’s disease. Nat. Neurosci. 17, 304–311. doi: 10.1038/nn.3606
Lautenschlager, N. T., Cox, K. L., Flicker, L., Foster, J. K., van Bockxmeer, F. M., Xiao, J., et al. (2008). Effect of physical activity on cognitive function in older adults at risk for Alzheimer disease: a randomized trial. JAMA 300, 1027–1037. doi: 10.1001/jama.300.9.1027
Leon, W. C., Bruno, M. A., Allard, S., Nader, K., and Cuello, A. C. (2010). Engagement of the PFC in consolidation and recall of recent spatial memory. Learn. Mem. 17, 297–305. doi: 10.1101/lm.1804410
Li, H., Li, J., Li, N., Li, B., Wang, P., and Zhou, T. (2011). Cognitive intervention for persons with mild cognitive impairment: A meta-analysis. Ageing Res. Rev. 10, 285–296. doi: 10.1016/j.arr.2010.11.003
Maass, A., Düzel, S., Goerke, M., Becke, A., Sobieray, U., Neumann, K., et al. (2015). Vascular hippocampal plasticity after aerobic exercise in older adults. Mol. Psychiatry 20, 585–593. doi: 10.1038/mp.2014.114
Mahncke, H. W., Connor, B. B., Appelman, J., Ahsanuddin, O. N., Hardy, J. L., Wood, R. A., et al. (2006). Memory enhancement in healthy older adults using a brain plasticity-based training program: a randomized, controlled study. Proc. Natl. Acad. Sci. U S A 103, 12523–12528. doi: 10.1073/pnas.0605194103
Marks, W. D., Yamamoto, N., and Kitamura, T. (2020). Complementary roles of differential medial entorhinal cortex inputs to the hippocampus for the formation and integration of temporal and contextual memory (Systems Neuroscience). Eur. J. Neurosci. doi: 10.1111/ejn.14737 ∗∗onlineprint,
Meister, M. L. R., and Buffalo, E. A. (2018). Neurons in primate entorhinal cortex represent gaze position in multiple spatial reference frames. J. Neurosci. 38, 2430–2441. doi: 10.1523/jneurosci.2432-17.2018
Miller, D. I., Taler, V., Davidson, P. S., and Messier, C. (2012). Measuring the impact of exercise on cognitive aging: methodological issues. Neurobiol. Aging 33:622. doi: 10.1016/j.neurobiolaging.2011.02.020
Morris, S. B. (2007). Estimating effect sizes from the pretest-posttest-control group designs. Organ. Res. Methods 11, 364–386. doi: 10.1177/1094428106291059
Oswald, C. J., Yee, B. K., Rawlins, J. N., Bannerman, D. B., Good, M., and Honey, R. C. (2001). Involvement of the entorhinal cortex in a process of attentional modulation: evidence from a novel variant of an IDS/EDS procedure. Behav. Neurosci. 115, 841–849. doi: 10.1037//0735-7044.115.4.841
Owen, A. M., Hampshire, A., Grahn, J. A., Stenton, R., Dajani, S., Burns, A. S., et al. (2010). Putting brain training to the test. Nature 465, 775–778. doi: 10.1038/nature09042
Park, D. C., and Bischof, G. N. (2013). The aging mind: neuroplasticity in response to cognitive training. Dialogues Clin. Neurosci. 15, 109–119. doi: 10.31887/DCNS.2013.15.1/dpark
Park, D. C., and Reuter-Lorenz, P. (2009). The adaptive brain: aging and neurocognitive scaffolding. Annu. Rev. Psychol. 60, 173–196. doi: 10.1146/annurev.psych.59.103006.093656
Roy, D. S., Kitamura, T., Okuyama, T., Ogawa, S. K., Sun, C., Obata, Y., et al. (2017). Distinct neural circuits for the formation and retrieval of episodic memories. Cell 170, 1000–1012. doi: 10.1016/j.cell.2017.07.013
Schaadt, A. K., Reinhart, S., Keller, I., Hildebrandt, H., Kerkhoff, G., and Utz, K. S. (2016). The effect of rotating random dot motion on visuospatial line orientation in patients with right-sided stroke. Neuropsychologia 92, 167–173. doi: 10.1016/j.neuropsychologia.2016.05.030
Shimada, H., Ishii, K., Makizako, H., Ishiwata, K., Oda, K., and Suzukawa, M. (2017). Effects of exercise on brain activity during walking in older adults: a randomized controlled trial. J. Neuroeng. Rehabil. 14:50. doi: 10.1186/s12984-017-0263-9
Smith, P. J., Blumenthal, J. A., Hoffman, B. M., Cooper, H., Strauman, T. A., Welsh-Bohmer, K., et al. (2010). Aerobic exercise and neurocognitive performance: a meta-analytic review of randomized controlled trials. Psychosom. Med. 72, 239–252. doi: 10.1097/PSY.0b013e3181d14633
Suo, C., Singh, M. F., Gates, N., Wen, W., Sachdev, P., Brodaty, H., et al. (2016). Therapeutically relevant structural and functional mechanisms triggered by physical and cognitive exercise. Mol. Psychiatry 21, 1633–1642. doi: 10.1038/mp.2016.19
Tarumi, T., Tomoto, T., Repshas, J., Wang, C., Hynan, L. S., Cullum, C. M., et al. (2021). Midlife aerobic exercise and brain structural integrity: associations with age and cardiorespiratory fitness. Neuroimage 225, 117512. doi: 10.1016/j.neuroimage.2020.117512
ten Brinke, L. F., Bolandzadeh, N., Nagamatsu, L. S., Hsu, C. L., Davis, J. C., Miran-Khan, K., et al. (2015). Aerobic exercise increases hippocampal volume in older women with probable mild cognitive impairment: a 6-month randomised controlled trial. Br. J. Sports Med. 49, 248–254. doi: 10.1136/bjsports-2013-093184
van Balkom, T. D., van den Heuvel, O. A., Berendse, H. W., van der Werf, Y. D., and Vriend, C. (2020). The effects of cognitive training on brain network activity and connectivity in aging and neurodegenerative diseases: a systematic review. Neuropsychol. Rev. 30, 267–286. doi: 10.1007/s11065-020-09440-w
Voss, M. W., Erickson, K. I., Prakash, R. S., Chaddock, L., Kim, J. S., Alves, H., et al. (2013). Neurobiological markers of exercise-related brain plasticity in older adults. Brain Behav. Immun. 28, 90–99. doi: 10.1016/j.bbi.2012.10.021
Weinstein, A. M., Voss, M. W., Prakash, R. S., Chaddock, L., Szabo, A., White, S. M., et al. (2012). The association between aerobic fitness and executive function is mediated by prefrontal cortex volume. Brain Behav. Immun. 26, 811–819. doi: 10.1016/j.bbi.2011.11.008
Wilming, N. A.-O., König, P. A.-O., König, S. A.-O., and Buffalo, E. A.-O. (2018). Entorhinal cortex receptive fields are modulated by spatial attention, even without movement. Elife 7:e31745. doi: 10.7554/eLife.31745
Winstanley, C. A., Chudasama, Y., Dalley, J. W., Theobald, D. E., Glennon, J. C., and Robbins, T. W. (2003). Intra-prefrontal 8-OH-DPAT and M100907 improve visuospatial attention and decrease impulsivity on the five-choice serial reaction time task in rats. Psychopharmacology 167, 304–314. doi: 10.1007/s00213-003-1398-x
Winters, B. D., and Bussey, T. J. (2005). Removal of cholinergic input to perirhinal cortex disrupts object recognition but not spatial working memory in the rat. Eur. J. Neurosci. 21, 2263–2270. doi: 10.1111/j.1460-9568.2005.04055.x
Wolinsky, F. D., Unverzagt, F. W., Smith, D. M., Jones, R., Stoddard, A., and Tennstedt, S. L. (2006). The ACTIVE cognitive training trial and health-related quality of life: protection that lasts for 5 years. J. Gerontol. A Biol. Sci. Med. Sci. 61, 1324–1329. doi: 10.1093/gerona/61.12.1324
Yeung, L. K., Olsen, R. K., Hong, B., Mihajlovic, V., D’Angelo, M. C., Kacollja, A., et al. (2019). Object-in-place memory predicted by anterolateral entorhinal cortex and parahippocampal cortex volume in older adults. J. Cogn. Neurosci. 31, 711–729. doi: 10.1162/jocn_a_01385
Keywords: cognitive training, aerobic exercise, entorhinal cortex, resting state functional connectivity, older adults
Citation: Gu N, Li H, Cao X, Li T, Jiang L, Zhang H, Zhao B, Luo C and Li C (2021) Different Modulatory Effects of Cognitive Training and Aerobic Exercise on Resting State Functional Connectivity of Entorhinal Cortex in Community-Dwelling Older Adults. Front. Aging Neurosci. 13:655245. doi: 10.3389/fnagi.2021.655245
Received: 18 January 2021; Accepted: 03 May 2021;
Published: 31 May 2021.
Edited by:
Alessandro Martorana, University of Rome Tor Vergata, ItalyReviewed by:
Simona Gabriella Di Santo, Santa Lucia Foundation, Scientific Institute for Research, Hospitalization and Healthcare, ItalyDaniele Corbo, University of Brescia, Italy
Copyright © 2021 Gu, Li, Cao, Li, Jiang, Zhang, Zhao, Luo and Li. This is an open-access article distributed under the terms of the Creative Commons Attribution License (CC BY). The use, distribution or reproduction in other forums is permitted, provided the original author(s) and the copyright owner(s) are credited and that the original publication in this journal is cited, in accordance with accepted academic practice. No use, distribution or reproduction is permitted which does not comply with these terms.
*Correspondence: Chunbo Li, licb@smhc.org.cn; Cheng Luo, @uestc.edu.cn
†These authors have contributed equally to this work and share first authorship