- 1Department of Neurology, The First Affiliated Hospital of Anhui Medical University, Hefei, China
- 2Anhui Province Key Laboratory of Cognition and Neuropsychiatric Disorders, Hefei, China
- 3Department of Neurology, The Second Affiliated Hospital of Anhui Medical University, Hefei, China
- 4Laboratory Center for Information Science and Technology of China, Hefei, China
- 5Collaborative Innovation Center of Neuropsychiatric Disorders and Mental Health, Hefei, China
- 6Hefei National Lab for Physical Sciences at the Microscale and the Centers for Biomedical Engineering, University of Science and Technology of China, Hefei, China
- 7The College of Mental Health and Psychological Sciences, Anhui Medical University, Hefei, China
- 8Institute of Artificial Intelligence, Hefei Comprehensive National Science Center, Hefei, China
Background: White matter hyperintensities (WMH)s is a very common neuroradiological manifestation in the elderly and is an increased risk of dementia and cognitive decline. As we all know, the thalamocortical circuit plays an important part in cognition regulation. However, the role of this circuit in WMHs and its related cognitive deficits is still unclear.
Method: Eighty WMH patients and 37 healthy controls (HCs) were enrolled in the current study. WMH patients were divided into a mild WMH group (n = 33) and moderate-severe WMH group (n = 47) according to Fazekas scores. Resting-state functional magnetic resonance imaging (rs-fMRI) data of all participants were collected for thalamocortical functional connectivity (FC) analysis. The analysis was performed in two steps. First, the whole cerebral cortex was divided into six regions of interest (ROIs), which were used as seeds to investigate the changes of FC with the thalamus. Then, the subregion of the thalamus generated in the previous step was used as the seed for FC analysis with the whole brain.
Results: In the first step of FC analysis, it was found that precentral gyrus (PrCG)-interthalamic adhesion (ITA) FC values in moderate-severe WMH group were higher than those in HC and mild WMH groups. However, when compared with the HC group, the increase of PrCG-ITA FC values in mild WMH group was not statistically significant. In the second step of FC analysis, the ITA was set as the seed, and compared with the HC group, the results showed that the FC values of the ITA-medial frontal gyrus (MFG) in mild group and moderate-severe WMH groups were significantly increased. In addition, the FC values in moderate-severe group were significantly higher than those in mild group. Finally, it was also found that FC values (PrCG-ITA and ITA-MFG) were significantly correlated with neuropsychological test results for multiple cognitive functions such as memory, execution and attention in WMH patients.
Conclusion: Abnormal thalamocortical FC was closely related with cognitive impairments in WMH patients.
Introduction
White matter hyperintensities (WMH)s is the main imaging features of cerebral small vessel disease. Specifically, they are defined as hyperintensities on a T2-weighted image in the periventricular or subcortical deep white matter, and are common in older individuals with cognitive decline (D'Arbeloff et al., 2019). Furthermore, WMHs increase risk of stroke, dementia and death (Debette and Markus, 2010; Xu et al., 2018). However, the pathogenesis of cognitive impairments of WMH remains uncertain.
Recent studies have suggested that the thalamus is involved in the regulation of cognitive function. Thalamic nuclei can be divided into two categories according to its inputs: first order and higher order (Guillery, 1995). In first order nuclei relay systems, several subregions of the thalamus receive input from surrounding sensory organs and subcortical structures and, in turn, send projections to primary sensory and motor cortex regions (Bickford et al., 2015). At the same time, in the higher-order system, several other subregions of the thalamus receive most of the inputs from the cortex directly, thereby regulating the transmission of information between the broader cortical regions related to the cognitive process (Saalmann et al., 2012; Ward, 2013). Given that the driving input to these nuclei originates from the cortex itself, and the projections of the thalamocortical are more dispersed than the first-order relay network, while higher-order nuclei also play an important role in regulating cortical activity and coordinating activities between cortical regions (Sherman, 2016). Previous studies have shown that there are significant connectivities between the thalamus and brain regions which closely related to different functions, such as the frontal, temporal, parietal and occipital lobes (Behrens et al., 2003; Johansen-Berg et al., 2005; Zhang et al., 2008, 2010). There is a study showed that patients with a higher burden of WMH showed lower default mode network functional connectivity in the thalamus. Moreover, the increase in the average dispersion of the white matter tract between thalamus and the posterior cingulate cortex is an independent risk factor for slower processing speed in patients with schizophrenia (Chen P. et al., 2019). In summary, these evidence suggests that the thalamocortical circuit may be involved in the cognitive impairments associated with WMH.
Functional magnetic resonance imaging (fMRI) is a prominent tool that helps in non-invasive examinations. Using resting-state fMRI (rs-fMRI), researchers found that the brain emitted consistent low frequency fluctuations in the range 0.01–0.08 Hz, and that these frequencies could be used to indicated intrinsic activity within the whole brain (Biswal, 2012). rs-fMRI relies on spontaneous low frequency fluctuations in the blood oxygen level dependent signal. Functional connectivity (FC) is the study of the interaction between two different brain regions. FC analysis based on regions of interest (ROI) is the most common method for investigating functionality in the brain. In general, many studies have used resting-state FC analysis to identify thalamocortical circuits in various disorders (Acharya et al., 2019; Chen X. et al., 2019; Ye et al., 2019).
In this study, we hypothesized that the thalamocortical FC may play an important role in the mechanism of cognitive impairments in WMH. We used rs-fMRI to measure thalamocortical FC in two steps: first, the cortex was segmented into six ROIs (Brown et al., 2017; Wei et al., 2020), which were regarded as a seed to locate FC changes in the thalamus. Next, the identified thalamic subregion in the first step were set as the seed to locate FC changes in the whole cortex.
Methods
Subjects
Eighty WMH patients were recruited in the Department of Neurology, the First Affiliated Hospital of Anhui Medical University (China). Based on the Fazekas scale (Kester et al., 2014), patients with WMHs were further divided into a mild (n = 33, Fazekas score 1–2) and moderate-severe group (n = 47, Fazekas score 3–6) (Morotti et al., 2020; Zhu et al., 2020). Severity of WMH was quantified using the periventricular and deep WMH Fazekas scores from 0 to 3 (Grade 0, no WMH; Grade 1, focal or punctate lesions; Grade 2, beginning of confluent lesions; and Grade 3, confluent lesions) (Fazekas et al., 1987). The UBO detector is used to extract and calculate variables in the WMH area (available for download at https://cheba.unsw.edu.au/group/neuroimaging-pipeline). It is a reliable, efficient and completely automatic segmentation process to generate WMH volumes and output the number of WMH cluster in periventricular areas, deep and lobar WMH regions, arterial areas, and even in whole brain (Jiang et al., 2018). UBO Detector is also used as a convenient tool in this study to quantitatively describe WMH volume of mild and moderate-severe group.
The criteria for WMH patient enrollment were: (1) aged 50–80 years; (2) magnetic resonance suggesting the presence of white matter damage; and (3) no contraindications to MRI. Exclusion criteria included: (1) internal and external cranial artery stenosis > 50% or previous carotid surgery; (2) subcortical or cortical infarct lesions with a diameter of more than 1.5 cm; (3) craniocerebral space-occupying lesions, intracranial hemorrhage, craniocerebral trauma or history of surgery; (4) WMHs caused by metabolic and hereditary leukoencephalopathy or immune-mediated inflammatory demyelinating diseases including multiple sclerosis, optic neuromyelitis or acute diffuse encephalomyelitis; and (5) hearing and visual impairments that impeded neuropsychological testing. According to Fazekas classification (source of interpretation), the WMH group was divided into a mild WMH and moderate-severe WMH group, with 39 and 54 individuals, respectively.
Healthy control (HCs) had no history of neurological or psychiatric diseases, or drug and alcohol abuse. HCs were able to cooperate with neuropsychology background tests and magnetic resonance examination. Data requirements for rs-fMRI: excessive motion in the scanner was <2 mm in any direction and <2.0° in any angular motion.
All participant signed informed consent, and the study was approved by the Anhui Medical University Ethics Committee.
Neuropsychological Examination
A neuropsychological examination was performed on all participants the same day as the MRI examination, including mental status and global cognitive assessments.
Overall Cognition Assessment
AD-8 questionnaire was used to assess change in functional performance secondary to cognitive change (Hendry et al., 2019). The Montreal Cognitive Assessment (MoCA) was also used to assess the overall cognitive assessment of WMH patients. There is a study that had confirmed the validity, reliability and clinical applicability of the MOCA scale in patients with cerebral small vessel disease (Wong et al., 2009).
Single Cognition and Psychiatric Symptoms Assessment
Anxiety disorders were assessed using the Generalized Anxiety Disorder 7 Scale (Hinz et al., 2017). Use Patient Health Questionnaire 9 (Smarr and Keefer, 2011) to evaluate depression symptoms (Smarr and Keefer, 2011). The Chinese auditory vocabulary learning test (CAVLT) was used to evaluate the participants' verbal memory skills. The Stroop color word test (SCWT) assesses the ability of participants to inhibit cognitive interference (Stroop, 1935). The trail making test (TMT), consisting of two parts (TMT-A and TMT-B), was used as an indication of organic brain damage (Reitan, 1958), and cognitive flexibility is the TMT primary executive function. The verbal fluency test (VFT) requires participant naming as many examples of animals as possible in 60 s, it activates multiple cognitive processes (Troyer et al., 1997). Digital span (DS) was used to evaluate the ability to focus the mind, anti-interference and transient memory, reflecting the participant's attention function (Ostrosky-Solís and Lozano, 2006). The symbol digital modalities test (SDMT) is a digital replacement test which was used to determine distraction, perception speed, visual scanning speed and tracking function (Smith, 1973; Lewandowski, 1984). The Boston naming test (BNT), which one of the most widely used tests for assessing naming ability and lexical retrieval was also utilized (LaBarge et al., 1986).
MRI Data Acquisition
The MRI scan was completed at the University of Science and Technology of China (Hefei, China), and MRI images were acquired on a General Electric HD 750 w 3.0 T MRI scanner with an 8-channel head-coil (General Electric, Waukesha, WI, USA). During the scan, all subjects were told to keep relax, keep their eyes closed and stay awake. The subjects were equipped with tight but comfortable foam pads to minimize head movement, and they were equipped with earplugs to reduce the noise of the scanner. The T1-weighted imaging sequence is: slice thickness = 5 mm and total surface area = 20 layers. T2-weighted imaging sequences: layer thickness = 5 mm and total layer = 20 layers. The rs-fMRI scans were conducted with the following parameters: repetition time = 2,400 ms; echo time = 30 ms; flip angle = 90°; matrix size = 64 × 64, field of view = 192 × 192 mm2; slice thickness = 3 mm; and 46 continuous slices (one voxel = 3 × 3 × 3 mm3). Acquire T1-weighted images through a brain volume (BRAVO) sequence with 188 slices (TR = 8.16 ms; TE = 3.18 ms; flip angle = 12°; field of view = 256 × 256 mm2; slice thickness = 1 mm; and voxel size = 1 × 1 × 1 mm3).
Functional Data Preprocessing
Functional magnetic resonance data were analyzed using Data Processing and Analysis for Brain Imaging (Yan et al., 2016) and statistical parametric mapping software package was used for preprocessing (Izquierdo-Garcia et al., 2014). Initially, the first ten volumes of the scanning process are discarded to achieve stable longitudinal magnetization and adapt the participants to the noisy scanning environment, and then underwent modifications in the following order: slice timing correction; realignment; normalization by diffeomorphic anatomical registration through exponentiated lie algebra; and transfer of the functional images to the Montreal Neurological Institute space (3 × 3 × 3 mm3) based on the transformation matrix and white matter and cerebrospinal fluid signals. Twenty-four head motion parameters were removed and used as a useless covariate. The image was smoothed with the full width (the half-maximum value is 4 mm), and the higher motion time point (defined as a frame-wise displacement > 0.5), the prior time point, and the latter two time points were scrubbed (Power et al., 2012). Finally, the time points were detrended and band-pass filtered (0.01–0.08 Hz).
Cortical ROI-to-Thalamus FC Analysis
First, the cerebral cortex was divided into six brain regions, including the frontal lobe, precentral gyrus (PrCG), postcentral gyrus, temporal lobe, occipital lobe and parietal lobe (Xu et al., 2018) (Figure 1). The thalamus is spatially defined by the Harvard-Oxford atlas (http://fsl.fmrib.ox.ac.uk/fsl/fslwiki/Atlases) (Figure 1) and these ROIs were used as seeds in the analysis to identify the functional connection between the cortical ROIs and each voxel in the thalamus. Then, calculate the Pearson correlation coefficient between the average blood oxygenation level dependent (BOLD) signal of each cortical ROI and the BOLD signal of each voxel in the thalamus. Apply Fisher's z-transform to the correlation value to ensure the normality. After that, the FC values of the brain regions generated in FC analysis were extracted and the correlation with the scores of the neuropsychological examination score were investigated. Then, calculate the Pearson correlation coefficient between the average BOLD signal of each cortical ROI and the BOLD signal of each voxel in the thalamus. Apply Fisher's z-transform to the correlation value to ensure normality.

Figure 1. Six cortical ROIs and the thalamus used in functional connectivity analysis. Occipital gyrus; Temporal gyrus; Precentral gyrus; Postcentral gyrus; Parietal gyrus; Frontal gyrus; and Thalamus.
Thalamus-to-Whole Brain FC Analysis
The clusters in the thalamus generated from thalamocortical FC analysis were then used as new ROI. FC analysis of thalamus-to-whole brain was performed among the three groups. In short, for each subject and each thalamic ROI, a correlation map is created by calculating the Pearson correlation coefficient between the average BOLD signal of the thalamic ROI and the BOLD signal of all voxels in the brain. Then, use Fisher's r-z transformation to convert these correlation graphs into Z-value graphs. Finally, the FC values were extracted and the correlation with the scores of the neuropsychological examination scores were investigated.
Statistical Analysis
Use SPSS 19.0 (SPSS, Inc., Chicago, IL, USA) to analyze the demographic information and neuropsychological background scores among three groups. All data were tested for normality. Normal distribution data is expressed as mean ± standard deviation, and non-normal distribution data is expressed as median and interquartile range. The differences among the three groups in regard to demographic, clinical, and neuropsychological data were compared using one-way analysis of variance, chi-square (χ2) tests, or the Kruska-Wallis test in cases of non-normality. Finally, Pearson's correlation analysis was used to analyze the relationship between the FC value and neuropsychological test scores of each of the participants. p < 0.05 was considered statistically significant. Multiple comparisons of these analyses were corrected using the Gaussian random field (significant at levels of voxel p < 0.001 and cluster p < 0.05).
Results
Demographic and Neuropsychological Results
There was no statistically significant difference in sex, age and education level among the three groups. Except for hypertension, there was no significant difference among the three groups in diabetes, hyperlipidemia and smoking and alcohol intake (p > 0.05). There were more hypertension patients in the mild WMH group than in the HC group (χ2 = 11.887, p = 0.001), while hypertension in the moderate-severe WMH group was significantly higher than in the HC group (χ2 = 25.076, p < 0.001). The moderate-severe WMH group performed significantly worse in MOCA, CAVLT, SDMT, TMT, and BNT tests compared with the HC group. The CAVLT scores of the moderate-severe WMH group were significantly lower than those in the mild WMH group (p < 0.05). In the TMT and BNT experiments, the mild WMH group had significantly lower scores than the HC group (p < 0.05). Demographic information and neurological background test results of the three groups are shown in Table 1.
Cortical ROI-to-Thalamus FC Results
The first FC analysis indicated that PrCG- interthalamic adhesion (ITA) FC values were different among the three groups [Figure 2: (1)]. We found that the PrCG-ITA FC values of the moderate-severe WMH group were significantly higher than those of the mild WMH and HC groups (t = 2.399, p = 0.019 and t = 4.042, p < 0.001, respectively); however, there was no statistical difference between the mild WMH and HC groups [t = 1.592, p = 0.115; Figure 2: (2)]. Pearson's correlation analysis showed that in the HC group, the PrCG-ITA FC values were positively correlated with MOCA, DS-forward and DS-backward scores (MOCA: r = 0.485, p = 0.002; DS-forward: r = 0.413, p = 0.016; DS-backward: r = 0.346, p = 0.049). In mild WMH group, the PrCG-ITA FC values were positively correlated with the STROOP-word score (r = 0.394, p = 0.035), whereas in the moderate-severe WMH group, PrCG-ITA FC values were positively correlated with CAVLT-delay recall [r = 0.338, p = 0.029; Figure 2: (3)].
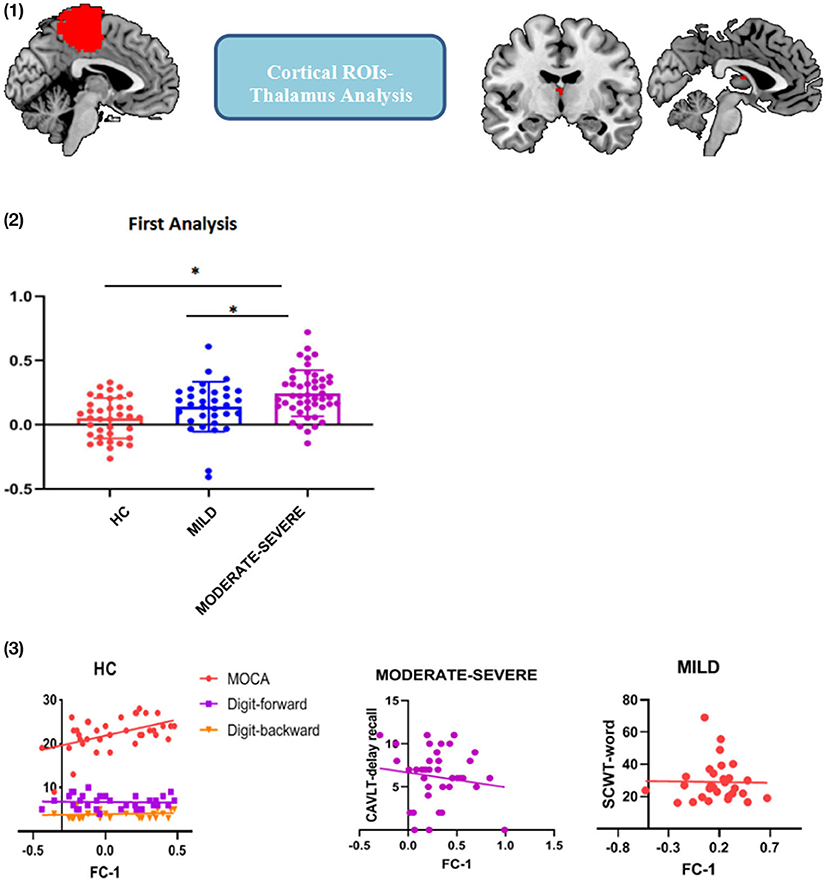
Figure 2. Functional connectivity between the precentral gyrus ROI and ITA. (1) PrCG-ITA detected in cortical ROIs-to-thalamus analysis; (2) FC strength of PrCG-ITA among the three groups; (3) Correlation between cognitive assessment scores and PrCG-ITA FC strength. FC, functional connectivity; PrCG, precentral gyrus; ITA, interthalamic adhesion; MOCA, Montreal cognitive assessment; CAVLT, Chinese auditory verbal learning test; SCWT, Stroop color and word test; WMHs, white matter hyperintensities; HC, healthy controls. *p < 0.05; error bars represent standard deviation.
Thalamus-to-Whole Brain FC Results
In the second step, ITA was used as a seed to analyze the FC values between the thalamus and the whole cortex. Results showed that ITA-medial frontal gyrus (MFG) FC values were different among the three groups [Figure 3: (1)]. Compared with the HC group, the ITA-MFG FC values increased in the mild and moderate-severe WMH groups (t = 2.134, p = 0.036 and t = 5.197, p < 0.001, respectively). Importantly, ITA-MFG FC values in the moderate-severe WMH group increased compared with those in the mild WMH group [t = 2.480, p = 0.015; Figure 3: (2)]. Correlation analysis results suggested that ITA-MFG FC values were positively correlated with MOCA, CAVLT-learning, CAVLT-delay recall and DS-forward scores in the HC group (MOCA: r = 0.369, p = 0.025; CAVLT-learning: r = 0.375, p = 0.025; CAVLT-delay recall: r = 0.362, p = 0.042; DS-forward: r = 0.386, p = 0.027), whereas in the mild WMH group, ITA-MFG FC values were positively correlated with SCWT-word and SCWT-interference [r = 0.427, p = 0.021 and r = 0.421, p = 0.023, respectively; Figure 3: (3)].
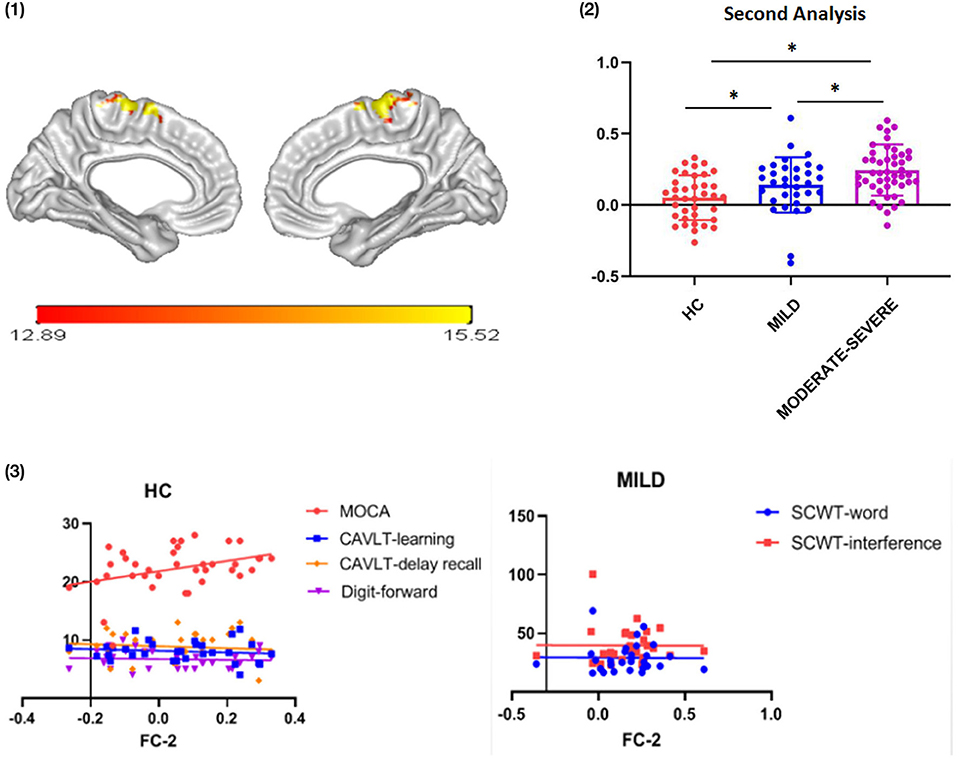
Figure 3. Functional connectivity between ITA and MFG. (1) ITA and MFG detected in thalamic subregion seed-whole brain analysis; (2) FC strength of ITA and MFG among the three groups; (3) Correlation between cognitive assessment scores and ITA-MFG FC strength. FC, functional connectivity; ITA, interthalamic adhesion; MOCA, Montreal cognitive assessment; CAVLT, Chinese auditory verbal learning test; SCWT, Stroop color and word test; WMHs, white matter hyperintensities; HC, healthy controls. *p < 0.05; error bars represent standard deviation.
Discussion
In this study, we explored the abnormalities of the thalamocortical FC in WMH patients and its relationship with cognitive function. The main results were as follows: First, PrCG-ITA FC was increased greatly in the moderate-severe WMH group, and the values of FC were significantly related to cognitive function in each group. Second, ITA-MFG FC in the moderate-severe and mild WMH groups showed varying degrees of increase. At the same time, a positive correlation between ITA-MFG FC values and cognition was also found in mild and HC groups. Overall, these results highlighted the important role of thalamocortical FC in cognitive impairment of WMH patients. We also demonstrated that WMH patients had lower cognitive scores than the normal population in terms of overall cognitive function, as well as attention, execution, memory and language. Finally, the increase of thalamocortical FC in WMH patients may be a compensatory mechanism after white matter damage.
Cerebral small vessel disease is a collective term for many pathological types, which can be manifested as white matter hyperintensities, lacunes, microbleeds and so on. It is rarely to select patients only manifested as white matter hyperintensities, and may be combined with multiple kind of pathological changes. Therefore, we described lacunes and microbleeds in HC group, mild WMH group and moderate-severe WMH group (Table 2). The results showed that the number of lacunes in frontal lobe, basal ganglia and whole brain were more severe in the WMH group than in HC group. Besides, the lacunes of whole brain in moderate-severe group was significantly higher than that of the mild group. In terms of microbleeds, the number of moderate-severe group was significantly more than that of HC group, which also appeared in frontal lobe, basal ganglia, thalamus and whole brain (Table 2). In addition, demographic information results show that there are significantly more hypertensive patients in WMH group than in HC group. Hypertension is considered to be one of the most important factors by damaging the WMH (Pantoni et al., 1997; Xiong et al., 2011). Hypertension and white matter hyperintensities are closely linked to cognitive decline (Wolf, 2012; Peters et al., 2019) and associated with WMH progression (Verhaaren et al., 2013). Therefore, it is suggested that hypertensive patients in mild and moderate-severe WMH group are significantly higher than HC group. However, the numbers of hypertension in severe WMH group was not statistically different from that in mild group (Table 1). In addition, brain tissue is an early damage target organ for hypertension. It is probably that hyperintensities is one of the important mechanisms of cognitive impairment caused by hypertension. WMH volumes of WMH patients were also quantified by UBO detector in this study. It can be concluded that the periventricular white matter volumes of the moderate-severe WMH group were significantly greater than that of the mild WMH group. In addition, the total brain white matter volumes of the moderate-severe WMH group were significantly higher than that of the mild WMH group. But, there was no significant difference in deep white matter volumes between the two groups (Table 3).
Our findings suggested that thalamocortical circuits may be involved in the pathogenesis of WMH and are related to cognitive impairment. Several studies have implicated thalamocortical connections in wakefulness, arousal, attention and executive function. Damage to these connections has been recorded in children with cerebellar brain tumors and developmental disorders, and these connections are associated with deficits in working memory (Law et al., 2017) and decreased reading ability (Fan et al., 2014). Besides, one study mainly focused on fragile neural networks in pediatric focal epilepsy suggested that thalamocortical circuit damage is an important mechanism for impaired executive function in temporal lobe epilepsy (Law et al., 2018). In particular, another study found that in schizophrenia patients, the decrease in thalamic functional connectivity was combined with the prefrontal cortex, and the increase in thalamic functional connection was combined with the motor and somatosensory cortex (Keefe, 2014). It has also been reported that the functional connections of thalamus and sensorimotor areas were negatively related to higher cognitive functions, such as attention, vigilance, and processing speed in patients with schizophrenia (Chen P. et al., 2019). Taken together, these studies show that thalamocortical FC is inseparable from cognitive function and plays an important role in many diseases.
Recently, more and more evidence has shown that abnormalities in the thalamic cortical network may cause cognitive dysfunction in patients with WMH. One study on diffusion tensor imaging indicated that the brain structure network in WMH patients with cognitive impairment is seriously affected, and this disorder is relevant to the burden of WMH and cognitive deficits (Yang et al., 2020). It is reported that the amplitude of low-frequency fluctuations in the left parahippocampal gyrus was significantly reduced, and the amplitude of low-frequency fluctuations in the left inferior semi-lunar lobule and the right Supraorbital frontal gyrus increased of WMH patients. Besides, compared with HC group, WMH group showed an increased functional connectivity between the right insular region and right superior orbital frontal gyrus, and between the right calcarine cortex and left parahippocampal gyrus (Cheng et al., 2017). The results also indicate that abnormal thalamocortical FC is closely related with cognitive impairment in WMH patients.
ITA is an understudied neuroanatomical structure that has not been fully studied. It forms a bridge that connects the tissues of each hemisphere thalamus across the midline. However, its functional significance is still not totally understood. One group has shown that the gender difference in ITA size was related to the anatomical structure of the surrounding thalamus and neuropsychological functions, especially the attention function (Damle et al., 2017), which is also reflected in the current research. In addition, a research about fiber tractography had showed that the anterior thalamic radiation (ATR) consists of fibers between the frontal cortex and the mediodorsal thalamic nuclei (Zhou et al., 2003). The ATR is the mainly white matter bundle projection system within the brain that passes through the internal capsule, and is an important circuit component to form new memories. Impairment in executive functioning was most consistently linked to lesions in the dorsomedial nucleus (Sandson et al., 1991; Van der Werf et al., 2003), which is connected to the frontal cortex through the ATR (Biesbroek et al., 2013). In the current study, the close relationship between thalamocortical function and memory, as well as executive function, was also found.
Previous studies have shown that the PrCG, frontal gyrus and thalamus are all closely related to cognitive function. The PrCG is related to the inner sensory processing of motion and somatosensory information. Moreover, the atrophy of the PrCG may induced the decline of cognitive function in the elderly people, and led to the abnormality in mutual regulation function between the regulation of anticipatory threats and exercise avoidance support, and caused the abnormal network integration (Drabant et al., 2011; Ely et al., 2016; Di Bono et al., 2017). Through white matter fiber structure within brain, MFG is extensively connected with other cortical and subcortical areas. The PrCG is also connected to the ventral part of the MFG through the lower structure of the frontal longitudinal system, while the upper structure of the frontal longitudinal system connects the precentral gyrus with the dorsal part of the MFG (Catani et al., 2012). Importantly, the middle frontal lobe is traditionally closely related to other cognitive control processes, and its functions mainly include response selection, task switching and task set reconfiguration, prevention of interference and working memory (D'Esposito et al., 1995; Cohen et al., 2000; Dove et al., 2000; Brass and von Cramon, 2002; Chikazoe, 2010). Besides, one study hypothesized that the massive activation of brain regions in MFG was related to the combined results of Wisconsin card sorting task and task switching (Buchsbaum et al., 2005), which consistent with the results in our second FC analysis.
In summary, the above results indicated a robust thalamocortical signal transmission, in which ITA plays a role. The current study proved that the thalamocortical circuit was not only related to perception, but is also involved in the processing and transmission and integration of information, and is closely related to cognitive function. The results suggested that the abnormality of the thalamocortical circuit may be an important mechanism of cognitive impairment in patients with WMHs.
Strengths and Limitations
We considered the relatively large number of participants in the study as a major strength; however, our study also had limitations. The effect of disease burden on FC in WMHs was only based on cross-sectional data. The lacunes and microbleeds also influence the performce of cognition in WMHs patients, which is another limitation of this study. In future research, we will start from the perspective of neuroimaging to further expand the sample size of diffusion tensor imaging data, and explore whether WMH patients also have an anatomical abnormality in thalamocortical functional connectivity, and clarify its relevance to cognitive impairment.
Data Availability Statement
The raw data supporting the conclusions of this article will be made available by the authors, without undue reservation.
Ethics Statement
This study involving human subjects were reviewed and approved by the Ethics Committee of the First Affiliated Hospital of Anhui Medical University. The patients/participants provided their written informed consent to participate in this study.
Author Contributions
CC: collect and analyze data and write articles. XW, SC, ZW, and WP: collect data and statistical results. JZ: provide ideas and collect data. JY and BQ: measurement services. YT: provide ideas and recruit participants. QW and KW: guiding experimental ideas and assisting in writing articles. All authors contributed to the article and approved the submitted version.
Funding
This study was supported by grants from the National Key Research and Development Program of China (2016YFC1300604), the National Natural Science Foundation of China-Major Project (82090034), and the National Nature Science Foundation of China (31970979).
Conflict of Interest
The authors declare that the research was conducted in the absence of any commercial or financial relationships that could be construed as a potential conflict of interest.
Acknowledgments
The authors would like to thank Information Science Laboratory Center of USTC for the measurement services and the great effort of each participant.
References
Acharya, A., Liang, X., Tian, W., Jiang, C., Han, Y., and Yi, L. (2019). White matter hyperintensities relate to basal ganglia functional connectivity and memory performance in aMCI and SVMCI. Front. Neurosci. 13:1204. doi: 10.3389/fnins.2019.01204
Behrens, T. E., Johansen-Berg, H., Woolrich, M. W., Smith, S. M., Wheeler-Kingshott, C. A. M., Boulby, P. A., et al. (2003). Non-invasive mapping of connections between human thalamus and cortex using diffusion imaging. Nat. Neurosci. 6, 750–757. doi: 10.1038/nn1075
Bickford, M. E., Zhou, N., Krahe, T. E., Govindaiah, G., and Guido, W. (2015). Retinal and tectal “Driver-Like” inputs converge in the shell of the mouse dorsal lateral geniculate nucleus. J. Neurosci. 35, 10523–10534. doi: 10.1523/JNEUROSCI.3375-14.2015
Biesbroek, J. M., Kuijf, H. J., van der Graaf, Y., Vincken, K. L., Postma, A., Mali, W. P. T. M., et al. (2013). Association between subcortical vascular lesion location and cognition: a voxel-based and tract-based lesion-symptom mapping study. The SMART-MR study. PLoS ONE 8:e60541. doi: 10.1371/journal.pone.0060541
Biswal, B. B. (2012). Resting state fMRI: a personal history. Neuroimage 62, 938–944. doi: 10.1016/j.neuroimage.2012.01.090
Brass, M., and von Cramon, D. Y. (2002). The role of the frontal cortex in task preparation. Cereb. Cortex 12, 908–914. doi: 10.1093/cercor/12.9.908
Brown, E. C., Clark, D. L., Hassel, S., MacQueen, G., and Ramasubbu, R. (2017). Thalamocortical connectivity in major depressive disorder. J. Affect. Disord. 217, 125–131. doi: 10.1016/j.jad.2017.04.004
Buchsbaum, B. R., Greer, S., Chang, W. L., and Berman, K. F. (2005). Meta-analysis of neuroimaging studies of the Wisconsin card-sorting task and component processes. Hum. Brain Mapp. 25, 35–45. doi: 10.1002/hbm.20128
Catani, M., Dell'acqua, F., Vergani, F., Malik, F., Hodge, H., Roy, P., et al. (2012). Short frontal lobe connections of the human brain. Cortex 48, 273–291. doi: 10.1016/j.cortex.2011.12.001
Chen, P., Ye, E., Jin, X., Zhu, Y., and Wang, L. (2019). Association between Thalamocortical functional connectivity abnormalities and cognitive deficits in Schizophrenia. Sci. Rep. 9:2952. doi: 10.1038/s41598-019-39367-z
Chen, X., Huang, L., Ye, Q., Yang, D., Qin, R., Luo, C., et al. (2019). Disrupted functional and structural connectivity within default mode network contribute to WMH-related cognitive impairment. Neuroimage Clin. 24:102088. doi: 10.1016/j.nicl.2019.102088
Cheng, R., Qi, H., Liu, Y., Zhao, S., Li, C., Liu, C., et al. (2017). Abnormal amplitude of low-frequency fluctuations and functional connectivity of resting-state functional magnetic resonance imaging in patients with leukoaraiosis. Brain Behav. 7:e00714. doi: 10.1002/brb3.714
Chikazoe, J. (2010). Localizing performance of go/no-go tasks to prefrontal cortical subregions. Curr. Opin. Psychiatry 23, 267–272. doi: 10.1097/YCO.0b013e3283387a9f
Cohen, J. D., Botvinick, M., and Carter, C. S. (2000). Anterior cingulate and prefrontal cortex: who's in control? Nat. Neurosci. 3, 421–423. doi: 10.1038/74783
Damle, N. R., Ikuta, T., John, M., Peters, B. D., DeRosse, P., Malhotra, A. K., et al. (2017). Relationship among interthalamic adhesion size, thalamic anatomy and neuropsychological functions in healthy volunteers. Brain Struct. Funct. 222, 2183–2192. doi: 10.1007/s00429-016-1334-6
D'Arbeloff, T., Elliott, M. L., Knodt, A. R., Melzer, T. R., Keenan, R., Ireland, D., et al. (2019). White matter hyperintensities are common in midlife and already associated with cognitive decline. Brain Commun. 1:fcz041. doi: 10.1093/braincomms/fcz041
Debette, S., and Markus, H. S. (2010). The clinical importance of white matter hyperintensities on brain magnetic resonance imaging: systematic review and meta-analysis. Br. Med. J. 341:c3666. doi: 10.1136/bmj.c3666
D'Esposito, M., Detre, J. A., Alsop, D. C., Shin, R. K., Atlas, S., and Grossman, M. (1995). The neural basis of the central executive system of working memory. Nature 378, 279–281. doi: 10.1038/378279a0
Di Bono, M. G., Priftis, K., and Umilta, C. (2017). Bridging the gap between brain activity and cognition: beyond the different tales of fMRI data analysis. Front. Neurosci. 11:31. doi: 10.3389/fnins.2017.00031
Dove, A., Pollmann, S., Schubert, T., Wiggins, C. J., and von Cramon, D. Y. (2000). Prefrontal cortex activation in task switching: an event-related fMRI study. Brain Res. Cogn. Brain Res. 9, 103–109. doi: 10.1016/S0926-6410(99)00029-4
Drabant, E. M., Kuo, J. R., Ramel, W., Blechert, J., Edge, M. D., and Cooper, J. R. (2011). Experiential, autonomic, and neural responses during threat anticipation vary as a function of threat intensity and neuroticism. Neuroimage 55, 401–410. doi: 10.1016/j.neuroimage.2010.11.040
Ely, B. A., Xu, J., Goodman, W. K., Lapidus, K. A., Gabbay, V., and Stern, E. R. (2016). Resting-state functional connectivity of the human habenula in healthy individuals: associations with subclinical depression. Hum. Brain Mapp. 37, 2369–2384. doi: 10.1002/hbm.23179
Fan, Q., Davis, N., Anderson, A. W., and Cutting, L. E. (2014). Thalamo-cortical connectivity: what can diffusion tractography tell us about reading difficulties in children? Brain Connect. 4, 428–439. doi: 10.1089/brain.2013.0203
Fazekas, F., Chawluk, J. B., Hurtig, H. I., and Zimmerman, R. A. (1987). MR signal abnormalities at 1.5 T in Alzheimer's dementia and normal aging. AJR Am. J. Roentgenol. 149, 351–356. doi: 10.2214/ajr.149.2.351
Guillery, R. W. (1995). Anatomical evidence concerning the role of the thalamus in corticocortical communication: a brief review. J. Anat. 187 (Pt 3), 583–592.
Hendry, K., Green, C., McShane, R., Noel-Storr, A. H., Stott, D. J., Anwer, S., et al. (2019). AD-8 for detection of dementia across a variety of healthcare settings. Cochrane Database Syst. Rev. 3:CD011121. doi: 10.1002/14651858.CD011121.pub2
Hinz, A., Klein, A. M., Brahler, E., Glaesmer, H., Luck, T., and Riedel-Heller, S. (2017). Psychometric evaluation of the generalized anxiety disorder screener GAD-7, based on a large German general population sample. J. Affect. Disord. 210, 338–344. doi: 10.1016/j.jad.2016.12.012
Izquierdo-Garcia, D., Hansen, A. E., Forster, S., Benoit, D., Schachoff, S., Furst, S., et al. (2014). An SPM8-based approach for attenuation correction combining segmentation and nonrigid template formation: application to simultaneous PET/MR brain imaging. J. Nucl. Med. 55, 1825–1830. doi: 10.2967/jnumed.113.136341
Jiang, J., Jiang, J., Liu, T., Zhu, W., Koncz, R., Liu, H., et al. (2018). UBO detector - a cluster-based, fully automated pipeline for extracting white matter hyperintensities. Neuroimage. 174, 539–549. doi: 10.1016/j.neuroimage.2018.03.050
Johansen-Berg, H., Behrens, T. E. J., Sillery, E., Ciccarelli, O., Thompson, A. J., Smith, S. M., et al. (2005). Functional-anatomical validation and individual variation of diffusion tractography-based segmentation of the human thalamus. Cereb. Cortex 15, 31–39. doi: 10.1093/cercor/bhh105
Keefe, R. S. E. (2014). The longitudinal course of cognitive impairment in schizophrenia: an examination of data from premorbid through posttreatment phases of illness. J. Clin. Psychiatry 752, 8–13. doi: 10.4088/JCP.13065su1.02
Kester, M. I., Goos, J. D. C., Teunissen, C. E., Benedictus, M. R., Bouwman, F. H., Wattjes, M. P., et al. (2014). Associations between cerebral small-vessel disease and Alzheimer disease pathology as measured by cerebrospinal fluid biomarkers. JAMA Neurol. 71, 855–862. doi: 10.1001/jamaneurol.2014.754
LaBarge, E., Edwards, D., and Knesevich, J. W. (1986). Performance of normal elderly on the Boston Naming test. Brain Lang. 27, 380–384. doi: 10.1016/0093-934X(86)90026-X
Law, N., Smith, M. L., Greenberg, M., Bouffet, E., Taylor, M. D., Laughlin, S., et al. (2017). Executive function in paediatric medulloblastoma: the role of cerebrocerebellar connections. J. Neuropsychol. 11, 174–200. doi: 10.1111/jnp.12082
Law, N., Smith, M. L., and Widjaja, E. (2018). Thalamocortical connections and executive function in pediatric temporal and frontal lobe epilepsy. AJNR Am. J. Neuroradiol. 39, 1523–1529. doi: 10.3174/ajnr.A5691
Lewandowski, L. J. (1984). The symbol digit modalities test: a screening instrument for brain-damaged children. Percept. Mot. Skills 59, 615–618. doi: 10.2466/pms.1984.59.2.615
Morotti, A., Shoamanesh, A., Oliveira-Filho, J., Schlunk, F., Romero, J. M., Jessel, M., et al. (2020). White matter hyperintensities and blood pressure lowering in acute intracerebral hemorrhage: a secondary analysis of the ATACH-2 trial. Neurocrit. Care 32, 180–186. doi: 10.1007/s12028-019-00761-0
Ostrosky-Solís, F., and Lozano, A. (2006). Digit span: effect of education and culture. Int. J. Psychol. 41, 333–341. doi: 10.1080/00207590500345724
Pantoni, L., and Garcia, J. H. (1997). Pathogenesis of leukoaraiosis: a review. Stroke 28, 652–659. doi: 10.1161/01.STR.28.3.652
Peters, R., Peters, R., Booth, A., Rockwood, K., Peters, J., D'Este, C., et al. (2019). Combining modifiable risk factors and risk of dementia: a systematic review and meta-analysis. BMJ Open 9:e022846. doi: 10.1136/bmjopen-2018-022846
Power, J. D., Barnes, K. A., Snyder, A. Z., Schlaggar, B. L., and Petersen, S. E. (2012). Spurious but systematic correlations in functional connectivity MRI networks arise from subject motion. Neuroimage 59, 2142–2154. doi: 10.1016/j.neuroimage.2011.10.018
Reitan, R. M. (1958). Validity of the Trail Making Test as an indicator of organic brain damage. Percept Motor Skills 8, 271–276. doi: 10.2466/pms.1958.8.3.271
Saalmann, Y. B., Pinsk, M. A., Wang, L., Li, X., and Kastner, S. (2012). The pulvinar regulates information transmission between cortical areas based on attention demands. Science 337, 753–756. doi: 10.1126/science.1223082
Sandson, T. A., Daffner, K. R., Carvalho, P. A., and Mesulam, M. M. (1991). Frontal lobe dysfunction following infarction of the left-sided medial thalamus. Arch. Neurol. 48, 1300–1303. doi: 10.1001/archneur.1991.00530240106031
Sherman, S. M. (2016). Thalamus plays a central role in ongoing cortical functioning. Nat. Neurosci. 19, 533–541. doi: 10.1038/nn.4269
Smarr, K. L., and Keefer, A. L. (2011). Measures of depression and depressive symptoms: beck depression INVENTORY-II (BDI-II), Center for Epidemiologic Studies Depression Scale (CES-D), Geriatric Depression Scale (GDS), Hospital Anxiety and Depression Scale (HADS), and Patient Health Questionnaire-9 (PHQ-9). Arthritis Care Res. 63, S454–S466. doi: 10.1002/acr.20556
Stroop, J. R. (1935). Studies of Interference in Serial Verbal Reactions. Nashville, TN: George Peabody College for Teachers, p. 19.
Troyer, A.K., Moscovitch, M., and Winocur, G. (1997). Clustering and switching as two components of verbal fluency: evidence from younger and older healthy adults. Neuropsychology 11, 138–146. doi: 10.1037/0894-4105.11.1.138
Van der Werf, Y. D., Scheltens, P., Lindeboom, J., Witter, M. P., Uylings, H. B. M., and Jolles, J. (2003). Deficits of memory, executive functioning and attention following infarction in the thalamus; a study of 22 cases with localised lesions. Neuropsychologia 41, 1330–1344. doi: 10.1016/S0028-3932(03)00059-9
Verhaaren, B. F., Vernooij, M. W., de Boer, R., Hofman, A., Niessen, W. J., van der Lugt, A., et al. (2013). High blood pressure and cerebral white matter lesion progression in the general population. Hypertension 61, 1354–1359. doi: 10.1161/HYPERTENSIONAHA.111.00430
Ward, L. M. (2013). The thalamus: gateway to the mind. Wiley Interdiscip. Rev. Cogn. Sci. 4, 609–622. doi: 10.1002/wcs.1256
Wei, Q., Bai, T., Brown, E. C., Xie, W., Chen, Y., Ji, G., et al. (2020). Thalamocortical connectivity in electroconvulsive therapy for major depressive disorder. J. Affect. Disord. 264, 163–171. doi: 10.1016/j.jad.2019.11.120
Wolf, P. A. (2012). Contributions of the Framingham heart study to stroke and dementia epidemiologic research at 60 years. Arch. Neurol. 69, 567–571. doi: 10.1001/archneurol.2011.977
Wong, A., Xiong, Y. Y., Kwan, P. W. L., Chan, A. Y. Y., Lam, W. W. M., Wang, K., et al. (2009). The validity, reliability and clinical utility of the Hong Kong Montreal Cognitive Assessment (HK-MoCA) in patients with cerebral small vessel disease. Dement. Geriatr. Cogn. Disord. 28, 81–87. doi: 10.1159/000232589
Xiong, Y., Yang, J., Wong, A., Wong, C. H. K., Chan, S. S. W., Li, H. H. S., et al. (2011). Operational definitions improve reliability of the age-related white matter changes scale. Eur. J. Neurol. 18, 744–749. doi: 10.1111/j.1468-1331.2010.03272.x
Xu, X., Gao, Y., Liu, R., Qian, L., Chen, Y., Wang, X., et al. (2018). Progression of white matter hyperintensities contributes to lacunar infarction. Aging Dis. 9, 444–452. doi: 10.14336/AD.2017.0808
Yan, C., Wang, X.-D., Zuo, X.-N., and Zang, Y.-F. (2016). DPABI: data processing & analysis for (Resting-State) brain imaging. Neuroinformatics 14, 339–351. doi: 10.1007/s12021-016-9299-4
Yang, D., Huang, L., Luo, C., Li, M., Qin, R., Ma, J., et al. (2020). Impaired structural network properties caused by white matter hyperintensity related to cognitive decline. Front. Neurol. 11, 250–250. doi: 10.3389/fneur.2020.00250
Ye, Q., Chen, X., Qin, R., Huang, L., Yang, D., Liu, R., et al. (2019). Enhanced regional homogeneity and functional connectivity in subjects with white matter hyperintensities and cognitive impairment. Front. Neurosci. 13, 695. doi: 10.3389/fnins.2019.00695
Zhang, D., Snyder, A. Z., Fox, M. D., Sansbury, M. W., Shimony, J. S., and Raichle, M. E. (2008). Intrinsic functional relations between human cerebral cortex and thalamus. J. Neurophysiol. 100, 1740–1748. doi: 10.1152/jn.90463.2008
Zhang, D., Snyder, A. Z., Shimony, J. S., Fox, M. D., and Raichle, M. E. (2010). Noninvasive functional and structural connectivity mapping of the human thalamocortical system. Cereb. Cortex 20, 1187–1194. doi: 10.1093/cercor/bhp182
Zhou, S. Y., Suzuki, M., Hagino, H., Takahashi, T., Kawasaki, Y., Nohara, S., et al. (2003). Decreased volume and increased asymmetry of the anterior limb of the internal capsule in patients with schizophrenia. Biol. Psychiatry. 54, 427–436. doi: 10.1016/S0006-3223(03)00007-6
Keywords: cognition, thalamocortical, functional connectivity, resting-state, white matter hyperintensities
Citation: Chen C, Wang X, Cao S, Zhang J, Wang Z, Pan W, Yang J, Tian Y, Qiu B, Wei Q and Wang K (2021) Thalamocortical Functional Connectivity in Patients With White Matter Hyperintensities. Front. Aging Neurosci. 13:632237. doi: 10.3389/fnagi.2021.632237
Received: 22 November 2020; Accepted: 23 February 2021;
Published: 18 March 2021.
Edited by:
Sabina Capellari, University of Bologna, ItalyCopyright © 2021 Chen, Wang, Cao, Zhang, Wang, Pan, Yang, Tian, Qiu, Wei and Wang. This is an open-access article distributed under the terms of the Creative Commons Attribution License (CC BY). The use, distribution or reproduction in other forums is permitted, provided the original author(s) and the copyright owner(s) are credited and that the original publication in this journal is cited, in accordance with accepted academic practice. No use, distribution or reproduction is permitted which does not comply with these terms.
*Correspondence: Kai Wang, d2FuZ2thaTE5NjQmI3gwMDA0MDsxMjYuY29t; Qiang Wei, d2VpcWlhbmcxOTg5MDkxNCYjeDAwMDQwOzEyNi5jb20=