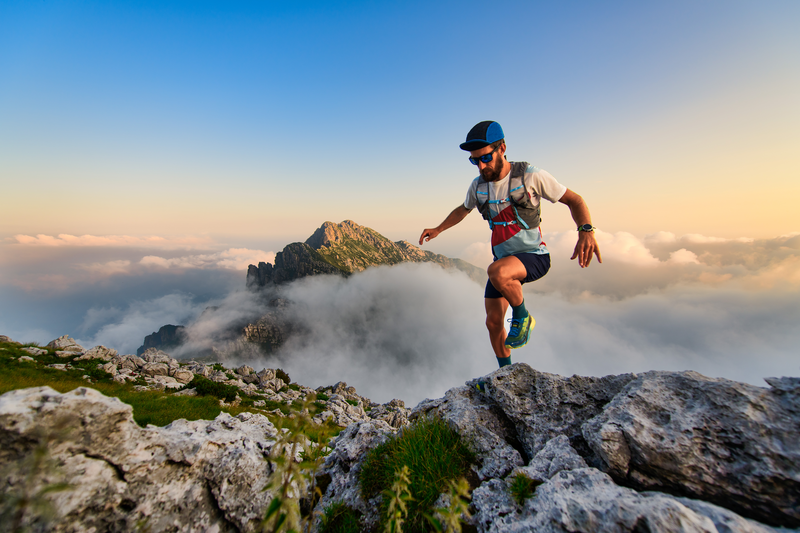
94% of researchers rate our articles as excellent or good
Learn more about the work of our research integrity team to safeguard the quality of each article we publish.
Find out more
ORIGINAL RESEARCH article
Front. Aging Neurosci. , 12 February 2021
Sec. Parkinson’s Disease and Aging-related Movement Disorders
Volume 13 - 2021 | https://doi.org/10.3389/fnagi.2021.621508
This article is part of the Research Topic Biomarkers and Pathogenesis of Alpha-Synuclein in Parkinson's Disease View all 33 articles
Introduction: Heme oxygenase-1 (HO-1) is a 32 kDa stress-response protein implicated in the pathogenesis of Parkinson’s disease (PD). Biliverdin is derived from heme through a reaction mediated by HO-1 and protects cells from oxidative stress. However, iron and carbon monoxide produced by the catabolism of HO-1 exert detrimental effects on patients with PD. The purpose of this study was to determine whether plasma HO-1 levels represent a biomarker of PD and to further explore the underlying mechanism of increased HO-1 levels by applying voxel-based morphometry (VBM).Methods: We measured plasma HO-1 levels using an enzyme-linked immunosorbent assay (ELISA) in 156 subjects, including 81 patients with early- and advanced-stage PD and 75 subjects without PD. The analyses were adjusted to control for confounders such as age, sex, and medication. We analyzed T1-weighted magnetic resonance imaging (MRI) data from 74 patients with PD using VBM to elucidate the association between altered brain volumes and HO-1 levels. Then, we compared performance on MMSE sub-items between PD patients with low and high levels of HO-1 using Mann-Whitney U tests.Results: Plasma HO-1 levels were significantly elevated in PD patients, predominantly those with early-stage PD, compared with controls (p < 0.05). The optimal cutoff value for patients with early PD was 2.245 ng/ml HO-1 [area under the curve (AUC) = 0.654]. Plasma HO-1 levels were unaffected by sex, age, and medications (p > 0.05). The right hippocampal volume was decreased in the subset of PD patients with high HO-1 levels (p < 0.05). A weak correlation was observed between right hippocampal volume and plasma HO-1 levels (r = −0.273, p = 0.018). There was no difference in total MMSE scores between the low- and high-HO-1 groups (p > 0.05), but the high-HO-1 group had higher language scores than the low-HO-1 group (p < 0.05).Conclusions: Plasma HO-1 levels may be a promising biomarker of early PD. Moreover, a high plasma concentration of the HO-1 protein is associated with a reduction in right hippocampal volume.
Parkinson’s disease (PD) is a movement disorder characterized by the loss of dopaminergic neurons in the substantia nigra and the formation of Lewy bodies induced by the aggregation of α-synuclein. Although pharmacological dopamine substitution (L-DOPA treatment) or deep brain stimulation (DBS) have been used to control the symptoms of PD and improve the quality of life up to decades after the onset of disease, these treatments are not very effective at preventing PD progression. Therefore, PD is a neurodegenerative disease that may ultimately lead to severe disability as it progresses (Poewe et al., 2017).
To date, the diagnosis of PD has been based on clinical criteria (Hughes et al., 1992). However, more than half of dopaminergic neurons are typically lost before a patient is diagnosed with PD. The long preclinical phase of PD offers the potential to administer disease-modifying treatments; therefore, an easily accessible biomarker that reflects the process of neurodegeneration is needed to diagnose the disease and predict disease progression in individual patients. Thus, a reliable biomarker would have great potential to help distinguish patients with PD from healthy persons, particularly in the early stages of the disease.
Heme oxygenase-1 (HO-1) is a 32 kDa cellular stress protein of the superfamily that metabolizes heme into biliverdin, iron, and carbon monoxide in cooperation with NADPH cytochrome P450 reductase in the brain and other tissues exposed to oxidative stress. This enzyme is primarily located and functions in the endoplasmic reticulum. Biliverdin is then further oxidized to bilirubin by biliverdin reductase, which helps restore a more favorable tissue redox microenvironment (Maines, 1988; Keyse and Tyrrell, 1989). The neuroprotective effect of HO-1 has been well studied in PD. For example, failure of proteasome degradation results in the formation of misfolded proteins, which is an important mechanism of α-synuclein accumulation in PD. Meanwhile, overexpression of HO-1 has been shown to facilitate α-synuclein proteasomal degradation in M17 neuroma cells (Song et al., 2009). Heme-derived CO in glial cells plays an important role in the dopaminergic neuroprotective effect of 2’, 3’-dihydroxy-4’,6’-dimethoxychalcone (DDC) through cell-to-cell communication (Masaki et al., 2017). Simvastatin prevents oxidative stress by enhancing the expression of the antioxidant protein HO-1 in a 6-OHDA-induced PD model (Tong et al., 2018). On the other hand, the following results indicate that overactivation of HO-1 in neurons may be deleterious in PD. Overexpression of HO-1 has been demonstrated in astrocytes in postmortem PD samples (Schipper, 2004). Moreover, excessive upregulation of HO-1 promotes the accumulation of non-transferrin-bound iron deposits, mitochondrial dysfunction, macrophages, and oxidative stress (Schipper, 1999; Song et al., 2006; Zukor et al., 2009). Previous studies have reported that selective overexpression of HO-1 in astrocytes in GFAP.HMOX1 transgenic mice from 8.5 to 19 months of age lead to the behavioral, neuropathologic, and molecular biological characteristics of parkinsonism (Song et al., 2017). Based on these findings, HO-1 may be a double-edged sword, and some clinical studies have investigated this hypothesis. Significantly higher serum (Mateo et al., 2010) and salivary HO-1 levels (Song et al., 2018) have been reported in patients with PD than in normal controls, prompting the hypothesis that HO-1 levels in biological fluids represent a promising marker of PD and may be used to assess the extent of neurodegeneration.
Voxel-based morphometry (VBM) is a widely used automated neuroanatomical image analysis technique that compares the local concentrations or volumes of gray and white matter in voxels (Yousaf et al., 2018). In the past decade, VBM has been widely used in neurological research, including studies of PD (van Mierlo et al., 2015; Goto et al., 2018; Xuan et al., 2019; Wolters et al., 2020). Here, we chose to investigate potential differences in the voxels of brain regions between patients with high and low plasma HO-1 levels (using the mean value as the dividing point) by applying this structural imaging technique at an anatomical level to explore the mechanism of the differences in HO-1 levels in PD patients.
In the present study, we sought to determine by ELISA whether plasma-derived HO-1 protein concentrations are elevated in patients with idiopathic PD and to explore structural neuroimaging to elucidate the mechanism underlying the variability of HO-1 levels in the blood of these patients.
All participants were recruited from the Henan Provincial People’s Hospital. This study included 156 participants: 81 patients with PD and 75 healthy controls. After initial inclusion, seven subjects with PD and incomplete relevant imaging data were excluded. The final sample for structural MRI analyses consisted of 74 patients with PD. The inclusion criteria were as follows: (1) a diagnosis of PD was made by an experienced neurologist; and (2) PD was diagnosed according to the United Kingdom Brain Bank Clinical Diagnostic Criteria (Hughes et al., 1992). Patients with secondary parkinsonism or atypical parkinsonian syndromes (including multiple system atrophy, progressive supranuclear palsy, dementia with Lewy bodies, and cortical–basal ganglionic degeneration) and individuals with the systemic inflammatory disease were excluded from the study.
The patients with idiopathic PD were in the “on” period when they were evaluated using different scales. The severity of motor symptoms was evaluated using the Hoehn & Yahr stages (H & Y stages) and United Parkinson’s disease (PD) Rating Scale-III (UPDRSIII; Goetz et al., 2008). Cognition was assessed with the Mini-Mental State Examination (MMSE). The MMSE was divided into eight subitems: (1) orientation to time and place (10 points); (2) immediate registration (three points); (3) divided attention (five points); (4) delayed recall (three points); (5) language (four points); (6) following a three-step command (three points); (7) writing a sentence (one point); and (8) copying a figure (one point; Ringman et al., 2007). The patients were on PD medication, including carbidopa/levodopa and dopamine agonists, to relieve their symptoms; however, exposure to these medications can influence plasma HO-1 concentrations and thereby act as a confounding factor. Also, there are more than a dozen anti-PD medications, and patients with PD usually take two or more medications. We consulted the drug treatment history of the PD patients and used a previously reported formula to calculate the equivalent daily doses of L-DOPA (LEDD; Tomlinson et al., 2010).
Meanwhile, 75 sex-matched subjects without PD were recruited from the Outpatient Physical Examination Department of Henan Provincial People’s Hospital. The inclusion criteria for control subjects were as follows: (1) no history of neurological disease or psychiatric disorders; (2) no brain injury; and (3) no diabetes mellitus, hypertension, cardiovascular disease, or systemic diseases (inflammation, infection, cancer, et cetera). The inclusion and exclusion criteria were strictly enforced to minimize the potential for confounders.
We divided patients with PD into those in the early stages (H&Y stage I and H&Y stage II) and those in the advanced stages (H&Y stage III and H&Y stage IV) to investigate the relationship between plasma HO-1 levels and disease progression. This study was approved by the ethics committee of Henan Provincial People’s Hospital. All study participants and their guardians provided written informed consent. The methodology used in this study was implemented according to recognized guidelines.
Early in the morning (7:00 am) after an overnight fast, a 5-ml blood sample was collected from each participant into a tube containing the anticoagulant EDTA. The blood samples were centrifuged (2,500 g for 15 min) at 25°C within 1 h of collection. The supernatant was collected, divided into 1.5 ml centrifuge tubes, and stored at −80°C until analysis. Plasma HO-1 levels in subjects with and without PD were measured using a human HO-1 sandwich ELISA kit (Catalog Number: ADI-EKS-800; Enzo Life Sciences Inc., Farmingdale, NY, USA) according to the manufacturer’s protocol. A standard curve generated with purified HO-1 was used to calculate the HO-1 concentration of each sample from the absorbance at λ = 450 nm (Li Volti et al., 2010). The sensitivity of the HO-1 (human) ELISA kit was determined to be 0.78 ng/ml. The standard curve had a range of 0.78–25 ng/ml. The intra-assay coefficient of variation of the HO-1 (human) ELISA kit was determined to be <10%. All samples were analyzed in duplicate and processed in the first freeze-thaw cycle.
All MRI scans were acquired using a Siemens MAGNETOM Prisma 3 T MRI scanner with a 64-channel head coil. Structural images were obtained using a 3D MRI magnetization-prepared rapid gradient-echo (MPRAGE) T1-weighted sequence [matrix = 256 × 256; flip angle = 4°; field of view (FOV) = 256 × 256 mm; slice thickness = 1 mm; TR = 5,000 ms; echo time (TE) = 3.43 ms; voxel size = 1 × 1 × 1 mm].
The quality of the acquired MRI scans was visually assessed to exclude scans with severe vascular lesions, space-occupying lesions, or motion artifacts. VBM was performed with SPM81 running on MATLAB 2013b. T1 image preprocessing was implemented using VBM82 as follows (Chard et al., 2002): (1) format conversion: the DICOM images were converted to a parsable NIFTI format; (2) normalization: after image registration, all anatomical images were registered to the Montreal Neurological Institute (MNI) coordinate space with a voxel size of 1 × 1 × 1 mm3; (3) segmentation: the brain tissue was segmented into gray matter (GM), white matter, and cerebrospinal fluid; (4) smoothing: the normalized modulated images were smoothed with an 8-mm full-width at half-maximum (FWHM) Gaussian kernel.
The modulated and smoothed GM data were subjected to whole-brain analysis using a two-sample t-test. Two sets of between-group analyses were performed on the differences in GM volume using a two-sample t-test in SPM8. Age and sex were included as covariates. First, the whole-brain significance threshold was set to p < 0.05 for multiple comparisons using familywise error correction at the voxel level. Since in vivo evidence of a correlation between GM and HO-1 levels in patients with PD has never been reported, the data were also presented using a less stringent, uncorrected threshold (p < 0.001, cluster threshold = 10 voxels) to detect subtle morphological changes. Based on the results of an exploratory whole-brain analysis, we also performed ROI analyses focusing on the right hippocampus. The brain areas with identified intergroup differences served as ROIs. The XJVIEWER3 module was applied to save the ROIs as a mask, and the GM volume of the ROI was extracted using REST1.24. Finally, the results of the SPM8 analysis were visualized using MRICRON5 and Adobe Photoshop 2019 (Adobe Systems Incorporated, San Jose, CA, USA).
The normality of distributions was assessed by the Shapiro–Wilk (SW) normality test (Henderson, 2006). Normally distributed continuous data are expressed as the mean ± standard deviation (SD). Data that did not conform to the normal distribution are presented as the median (first quartile, third quartile). Pearson’s chi-square test was used to compare the distributions of categorical variables between groups. Two-group comparisons of normally distributed variables were performed using t-tests. One-way ANOVA was performed to compare variables among three or more groups. Variables that did not fit the normal distribution were assessed using the Mann-Whitney U test (two groups) or the Kruskal–Wallis (KW) rank-sum test (three or more groups).
Spearman’s rank correlation coefficient was calculated to assess whether HO-1 levels were related to medications, and the correlation between plasma HO-1 levels and ROI volumes was analyzed by calculating the Pearson correlation coefficient.
The accuracy of plasma HO-1 levels in diagnosing patients with PD was assessed by constructing a receiver operating characteristic curve. The point with the maximum Youden index on the ROC curve was considered the optimum HO-1 cutoff point to diagnose the disease.
Statistical analyses were performed using SPSS software version 25 (IBM, USA). Graphs were generated with GraphPad Prism version 8.0 (GraphPad Prism Software, Inc., San Diego, CA, USA). A value of p < 0.05 was considered to indicate a statistically significant difference.
Of the 156 study subjects, 81 (51.9%) were diagnosed with idiopathic PD, and 75 (48.1%) were non-PD controls. The clinical characteristics of patients with PD are summarized in Table 1. No intergroup differences in sex were observed (p = 0.985), but patients with PD were older than controls (p < 0.001).
There were no statistically significant differences in HO-1 levels among the three groups (early PD patients, advanced PD patients and healthy controls) in either men or women [male: 2.39 (2.11, 2.97) vs. 2.38 (2.02, 2.61) vs. 2.18 (1.70, 2.61) ng/ml, p = 0.176; female: 2.34 ± 0.60 vs. 2.10 ± 0.53 vs. 2.01 ± 0.41 ng/ml, p = 0.053; Supplementary Figure 1]. Furthermore, comparison of HO-1 levels between men and women in the early PD, the advanced PD, and control groups also showed no significant differences [early PD: 2.39 ± 0.75 vs. 2.34 ± 0.60 ng/ml, p = 0.81; advanced PD: 2.33 ± 0.58 vs. 2.10 ± 0.53 ng/ml, p = 0.22; normal controls: 2.18 (1.70, 2.61) vs. 2.07 (1.74, 2.28) ng/ml, p = 0.25; Supplementary Figures 1B–D]. We also performed the same stratified analysis with age as a confounding factor. Subjects aged ≥60 years and < 60 years were defined as elderly and young, respectively. When the levels of HO-1 were compared among the early PD, advanced PD and control groups in young and elderly subjects, the results showed no statistically significant differences [young subjects: 2.43 (1.99, 2.97) vs. 2.19 (1.62, 2.61) vs. 2.16 (1.73, 2.39) ng/ml, p = 0.15; elder subjects: 2.34 ± 0.70 vs. 2.23 ± 0.56 vs. 2.02 ± 0.49 ng/ml, p = 0.22; Supplementary Figure 1E]. Comparison of HO-1 levels between young and elderly individuals in the early PD, advanced PD, and normal control groups also showed no significant differences [early PD: 2.42 ± 0.62 vs. 2.34 ± 0.70 ng/ml, p = 0.75; advanced PD: 2.19 ± 0.60 vs. 2.23 ± 0.56 ng/ml, p = 0.84; normal controls: 2.16 (1.73, 2.39) vs. 2.09 (1.68, 2.33) ng/ml, p = 0.69; Supplementary Figures 1F–H].
Plasma HO-1 levels were significantly increased in PD patients compared with the control group [2.37 (1.98, 2.67) vs. 2.13 (1.73, 2.37) ng/ml; p = 0.006; Figure 1A]. Significantly higher HO-1 levels were observed in the participants with early PD than in the controls [2.43 (2.00, 2.79) vs. 2.13 (1.73, 2.37) ng/ml; p = 0.004; Figure 1B]. Although higher plasma HO-1 levels were detected in the subjects with advanced PD than in the healthy controls, this difference was not statistically significant [2.35 (1.68, 2.54) vs. 2.13 (1.73, 2.37) ng/ml; p = 0.139; Figure 1C]. In addition, differences in HO-1 concentrations based on H & Y stage were not significant [2.35 ± 0.89 vs. 2.37 ± 0.62 vs. 2.23 ± 0.58 vs. 2.15 ± 0.53 ng/ml; p = 0.787; Supplementary Figure 3].
Figure 1. Plasma HO-1 levels in PD patients and control subjects. (A) Increased plasma HO-1 levels in the PD group compared with control subjects. (B) HO-1 levels in the PD group with early-stage were highly increased in the plasma compared with the controls. (C) Plasma HO-1 levels were elevated in the advanced PD group relative to the control group, but no statistically significant. PD, Parkinson’s disease; CON, normal controls; HO-1, Heme Oxygenase-1. The central line in each box indicates the median, box edges mark the first and third quartiles, and limits of the vertical lines show ranges. *p < 0.05.
There was no significant difference in LEDD levels among H & Y stages I-IV [400 (262.50, 631.25) vs. 400.00 (200.00, 600.00) vs. 450 (287.50, 600.00) vs. 400.00 (375.00, 737.50), p = 0.917; Supplementary Figure 2A]. The median LEDD in the 81 patients with PD was 400.00 mg. However, no significant correlation was observed between plasma HO-1 levels and LEDD (r = −0.015, p = 0.892; Supplementary Figure 2B).
In order to evaluate the utility of HO-1 as a candidate biomarker of PD, an ROC curve was generated to distinguish PD patients in general from controls; the optimal cutoff value was found to be 2.255 ng/ml (sensitivity = 68%, specifity = 61.7%, AUC = 0.627, p = 0.006); the cutoff value for early-stage PD was 2.245 ng/ml (sensitivity = 66%, specifity = 66.7%, AUC = 0.654, p = 0.004). Plasma HO-1 levels did not differ between patients with advanced PD and the control group (sensitivity = 55.9%, specifity = 73.3%, AUC = 0.589, p = 0.139; Figure 2).
Figure 2. ROC curve for plasma HO-1 levels comparing PD group, early PD group, advanced PD group, and healthy control group. ROC curve, receiver operating characteristic curve; AUC, area under the curve; PD, Parkinson’s disease.
Each volumetric image section in the high-HO-1 group showed a reduced area of the right hippocampus compared to the low-HO-1 group (Figure 3A). Brain regions with different volumes between the low- and high-HO-1 groups are summarized in Table 2. As shown in Figure 3B, the volume of the ROI was significantly higher in the low-HO-1 group than in the high-HO-1 group after the extraction of volumes in different brain areas (p = 0.002). Additionally, the correlation analysis indicated a weak but significant negative correlation between the ROI volumes and plasma HO-1 levels in the PD group (r = −0.273, p = 0.018; Figure 3C). There was no significant difference in MMSE scores between the low- and high-HO-1 groups [26.00 (23.00, 28.00) vs. 26.00 (24.75, 28.00), p = 0.533; Table 3]. However, the low-HO-1 group had lower language scores than the high-HO-1 group [3.00 (3.00, 4.00) vs. 4.00 (3.00, 4.00), p = 0.018; Table 3].
Figure 3. VBM analysis of differences in brain areas between low and high levels HO-1 groups. (A) The figure shows T1-weighted imaging-based VBM analysis of the whole-brain about series of sections images. Statistically significant reduction in clusters of right hippocampal concentration in high HO-1 PD group compared with that in low HO-1 PD subjects is shown. Only clusters comprising at least 10 suprathreshold voxels were included in the analysis and are shown in the figure. To help visualization, shaded red sections were added to highlight all clusters. Color-bar indicates the level of concentration by the depth of the color. (B) Comparison of the volume of two groups with different HO-1 levels. (C) Correlation between HO-1 level and right hippocampal volume. HO-1, Heme Oxygenase-1; VBM, Voxel-Based Morphometry. *p < 0.05.
Plasma is more accessible and frequently sampled than cerebrospinal fluid, and the assessment of plasma is inexpensive compared to PET-CT imaging techniques. In this study, we used plasma HO-1 levels to distinguish patients with PD from healthy controls in a large cohort. Concentrations of the HO-1 protein were significantly increased in patients with PD, particularly those in the early stage of the disease, compared to normal controls. VBM features are not suitable as markers, but they may improve our understanding of the potential pathophysiological mechanism underlying the differences in regional brain volume. Increased levels of the HO-1 protein were associated with a reduced right hippocampal volume.
HO-1 is the rate-limiting enzyme in the endoplasmic reticulum that converts the pro-oxidant heme into biliverdin, iron, and carbon monoxide in the presence of oxidative stress (Maines, 1988; Keyse and Tyrrell, 1989). HO-1 expression was observed in the neuromelanin-containing (dopaminergic) neurons of the substantia nigra compacta of postmortem brain tissues from patients with PD (Schipper et al., 1998). Considerable evidence implicates oxidative stress as a primary pathogenic mechanism underlying the death of most dopaminergic neurons in patients with PD (Blesa et al., 2015). The promoter of the HO-1 gene contains a heat shock element, and its expression is substantially upregulated in response to oxidative stress. The induction of HO-1 expression in redox tissues provides cytoprotection from oxidative damage through the production of bile pigments (bilirubin and biliverdin) with free radical-scavenging capabilities (Stocker, 1990). On the other hand, HO-1-mediated heme degradation releases free iron and CO, which exacerbates oxidative damage by producing free radicals within the mitochondrial compartment. However, little is known about the exact mechanism by which HO-1 participates in oxidative stress in patients with PD. Based on accumulating evidence, the HO-1 protein is present in peripheral body fluids, such as plasma (Bao et al., 2010; Signorelli et al., 2016; Kishimoto et al., 2018a,b; Kishimoto et al., 2020). Plasma HO-1 is leaked or secreted from epithelial and endothelial cells of different tissues that are subjected to oxidative stress. Additionally, the induction of HO-1 expression may be necessary and sufficient to provide protection after damage. Thus, plasma HO-1 levels are abnormal and elevated in patients with PD, a pathological condition, relative to the normal condition in healthy subjects.
As in previous studies (Mateo et al., 2010; Song et al., 2018), peripheral HO-1 levels were higher in patients with PD than in controls in the present study, and the difference was not affected by age, sex, or medication. Paradoxically, Schipper et al. (2000) did not observe a significant difference in plasma HO-1 levels between a small group of patients with PD and normal elderly subjects. A probable explanation for this discrepancy is the use of different methods to measure HO-1 levels in the two groups, along with the recruitment of subjects from different areas. A large cohort of patients with PD was used to investigate plasma HO-1 levels in the present study. Moreover, plasma HO-1 levels displayed moderate sensitivity and specificity in distinguishing PD patients from controls in the diagnosis of PD. In addition to the results discussed above, HO-1 concentrations were significantly increased in patients with early PD compared to controls without the disease, but there was no such difference between patients with advanced PD and healthy subjects. Our observations were consistent with those of a previous study (Song et al., 2018), where the level of HO-1 in patients with idiopathic PD peaked early and started decreasing as the disease progressed. As exemplified by a temporary elevation of particular chemicals in some patients with acute diseases, HO-1 protects against challenges or noxious stimuli resulting from oxidative damage in patients with early-stage PD. On the other hand, based on evidence from cell culture, animal model, and human neuropathology studies in the literature before this study, predominant HO-1 expression in astrocytes is considered a necessary and sufficient condition for excessive brain iron deposition, oxidative stress, mitochondrial dysfunction, and macroautophagy (Schipper et al., 2019). Hence, we hypothesized that the decreased level of HO-1 in patients with advanced-stage PD may be due to a mechanism by which the body defends against damage caused by HO-1 overexpression after the pathological features of PD have developed. Although HO-1 levels were markedly increased in the early stage of PD, plasma HO-1 levels must be measured in a larger number of patients with idiopathic PD.
The expression levels of HO-1 exhibited significant differences at various stages of PD. Therefore, we explored differences in the anatomical structures of brain regions in patients with different HO-1 levels. Interestingly, our VBM analysis showed a decrease in the volume of the right hippocampus in the group of PD patients presenting high HO-1 levels compared with the group presenting low HO-1 levels. According to Schipper et al. (1995) HO-1 was significantly overexpressed in hippocampal neurons and astrocytes in patients with AD relative to controls, as analyzed using immunoblotting, which confirmed an association between the hippocampus and HO-1 levels. Although this association was identified in patients with AD, both diseases are neurodegenerative diseases. GFAP.HMOX1 mice exhibit dysgenesis of the hippocampal dentate gyrus (Song et al., 2012). This model confirms the presence of hippocampal atrophy in mice overexpressing HO-1. Consistent with these results, we observed a reduction in hippocampal volume in patients with high peripheral plasma HO-1 concentrations. However, it is unclear why the volume reduction is limited to the right side of the hippocampus and whether the volumes of specific hippocampal subregions are decreased.
Also, we performed a simple cognitive function test (the MMSE) in PD patients with low and high HO-1 levels. The results showed that there was no significant intergroup difference in total MMSE scores. Several studies have reported that volumetric measurements of the hippocampus are the most significant predictor of AD among patients and that they may even precede the onset of clinical symptoms (Fox et al., 1996; Kaye et al., 1997; Jack et al., 1999). Additionally, hippocampal volume atrophy is a very sensitive indicator of cognitive impairment not only in people with AD but also in a broader population (Wolf et al., 2001). Taken together, the results imply that the reduction in hippocampal volume does not indicate impaired cognition. Perhaps this volume reduction occurs before the onset of cognitive impairment. The reason why the MMSE results were negative in this study may be that the clinical symptoms had not yet developed, although there were already structural changes. Regarding the specific sub-items of the MMSE, the reason for our results might be the limited specificity of the sub-items. More detailed data on cognitive function will be needed to compare the cognition of the two groups in future work. Our results also suggest that the correlation between hippocampal volume and HO-1 levels is complicated. Ultimately, plasma HO-1 is more promising than brain volumetric features as a marker of early PD, and increased plasma HO-1 is accompanied by a reduction in hippocampal volume, which can serve as a warning in the predementia stages.
The main strength of this study is that it is one of very few to measure peripheral plasma HO-1 levels in PD patients and control subjects in a sample size that allows statistical comparisons. Moreover, the anatomy of the brain was altered by HO-1 levels, advancing the current understanding of the underlying pathophysiological mechanism of HO-1. However, the study has some limitations. First, abnormal HO-1 levels have been observed in diseases of other organs, such as hypertension, diabetes, and cardiovascular disease (Bao et al., 2010; Signorelli et al., 2016; Kishimoto et al., 2018a,b, 2020). Although healthy controls with these complications were excluded from the study, these cofounders were still present in patients with PD. Second, plasma HO-1 concentrations in patients with atypical Parkinsonism, such as multiple system atrophy (MSA) and progressive supranuclear palsy (PSP), need to be measured in the future to determine the specificity of HO-1 as a marker of idiopathic PD. Third, larger sample size is warranted to analyze the role of HO-1 levels in predicting the progression of PD. In contrast to cross-sectional studies, a more accurate and appropriate approach would be to assess HO-1 levels and measure changes in the volumes of different brain structures in follow-up studies.
Based on our findings, plasma HO-1 levels are a promising biomarker for diagnosing early PD and may assist clinicians in achieving unexpectedly good treatment outcomes by intervening in the early stage of the disease. Moreover, we observed a correlation between high HO-1 levels and GM volume in the hippocampus. Given the significance of reduced hippocampal volume, high HO-1 levels accompanied by a reduction in hippocampal volume in early PD may be a warning sign of cognitive impairment. Also, inhibiting HO-1 overexpression in response to nociceptive stimuli at a critical time in the development of PD might be an effective method for managing neurodegeneration.
The raw data supporting the conclusions of this article will be made available by the authors, without undue reservation.
The studies involving human participants were reviewed and approved by Medical Ethics Committee of Henan Provincial People’s Hospital. The patients/participants provided their written informed consent to participate in this study.
WS, JM, and JZ completed the research design. WS performed the experiments. ZW, XS, and ML conducted the literature search and data collection. SH, SYH, ZZ, and DL performed the statistical analysis and interpretation of data. WS, JM, and JZ were responsible for writing the manuscript. All authors contributed to the article and approved the submitted version.
This study was supported by Henan Province Science and Technology Development Plan (192102310085) and Henan Province Medical Science and Technology Research Program (201701018).
The authors declare that the research was conducted in the absence of any commercial or financial relationships that could be construed as a potential conflict of interest.
The Supplementary Material for this article can be found online at: https://www.frontiersin.org/articles/10.3389/fnagi.2021.621508/full#supplementary-material.
Supplementary Figure S1 | HO-1 levels were analyzed for all participants and stratified by age-group and sex-group. PD, Parkinson’s disease; HO-1, Heme Oxygenase-1. *p < 0.05.
Supplementary Figure S2 | The effects of medication. (A) The box plot between H and Y stages and LEDD. (B) Spearman correlation analysis for the relationship between LEDD and HO-1. LEDD, The equivalent daily dose of L-DOPA; H and Y stages, Hoehn and Yahr stages; HO-1, Heme Oxygenase-1. *p < 0.05.
Supplementary Figure S3 | Distribution of plasma HO-1 concentrations by H and Y stages. There were no significant differences in the distribution of plasma HO-1 concentrations by H and Y stages in PD patients (p > 0.05). H and Y stages, Hoehn and Yahr stages; HO-1, Heme Oxygenase-1. *p < 0.05.
Bao, W., Song, F., Li, X., Rong, S., Yang, W., Zhang, M., et al. (2010). Plasma heme oxygenase-1 concentration is elevated in individuals with type 2 diabetes mellitus. PLoS One 5:e12371. doi: 10.1371/journal.pone.0012371
Blesa, J., Trigo-Damas, I., Quiroga-Varela, A., and Jackson-Lewis, V. R. (2015). Oxidative stress and Parkinson’s disease. Front Neuroanat. 9:91. doi: 10.3389/fnana.2015.00091
Chard, D. T., Parker, G. J. M., Griffin, C. M. B., Thompson, A. J., and Miller, D. H. (2002). The reproducibility and sensitivity of brain tissue volume measurements derived from an SPM-based segmentation methodology. J. Magn. Reson. Imaging 15, 259–267. doi: 10.1002/jmri.10064
Fox, N. C., Warrington, E. K., Freeborough, P. A., Hartikainen, P., Kennedy, A. M., Stevens, J. M., et al. (1996). Presymptomatic hippocampal atrophy in Alzheimer’s disease. a longitudinal MRI study. Brain 119, 2001–2007. doi: 10.1093/brain/119.6.2001
Goetz, C. G., Tilley, B. C., Shaftman, S. R., Stebbins, G. T., Fahn, S., Martinez-Martin, P., et al. (2008). Movement disorder society-sponsored revision of the unified Parkinson’s disease rating scale (MDS-UPDRS): scale presentation and clinimetric testing results. Mov. Disord. 23, 2129–2170. doi: 10.1002/mds.22340
Goto, M., Kamagata, K., Hatano, T., Hattori, N., Abe, O., Aoki, S., et al. (2018). Depressive symptoms in Parkinson’s disease are related to decreased left hippocampal volume: correlation with the 15-item shortened version of the geriatric depression scale. Acta. Radiol. 59, 341–345. doi: 10.1177/0284185117719100
Henderson, A. R. (2006). Testing experimental data for univariate normality. Clin. Chim. Acta 366, 112–129. doi: 10.1016/j.cca.2005.11.007
Hughes, A. J., Daniel, S. E., Kilford, L., and Lees, A. J. (1992). Accuracy of clinical diagnosis of idiopathic Parkinson’s disease: a clinico-pathological study of 100 cases. J. Neurol. Neurosurg. Psychiatry 55, 181–184. doi: 10.1136/jnnp.55.3.181
Jack, C. R. Jr., Petersen, R. C., Xu, Y. C., O’Brien, P. C., Smith, G. E., Ivnik, R. J., et al. (1999). Prediction of AD with MRI-based hippocampal volume in mild cognitive impairment. Neurology 52, 1397–1403. doi: 10.1212/wnl.52.7.1397
Kaye, J. A., Swihart, T., Howieson, D., Dame, A., Moore, M. M., Karnos, T., et al. (1997). Volume loss of the hippocampus and temporal lobe in healthy elderly persons destined to develop dementia. Neurology 48, 1297–1304. doi: 10.1212/wnl.48.5.1297
Keyse, S. M., and Tyrrell, R. M. (1989). Heme oxygenase is the major 32-kDa stress protein induced in human skin fibroblasts by UVA radiation, hydrogen peroxide, and sodium arsenite. Proc. Natl. Acad. Sci. U S A 86, 99–103. doi: 10.1073/pnas.86.1.99
Kishimoto, Y., Ibe, S., Saita, E., Sasaki, K., Niki, H., Miura, K., et al. (2018a). Plasma heme oxygenase-1 levels in patients with coronary and peripheral artery diseases. Dis. Markers 2018:6138124. doi: 10.1155/2018/6138124
Kishimoto, Y., Sasaki, K., Saita, E., Niki, H., Ohmori, R., Kondo, K., et al. (2018b). Plasma heme oxygenase-1 levels and carotid atherosclerosis. Stroke 49, 2230–2232. doi: 10.1161/STROKEAHA.118.022256
Kishimoto, Y., Niki, H., Saita, E., Ibe, S., Umei, T., Miura, K., et al. (2020). Blood levels of heme oxygenase-1 versus bilirubin in patients with coronary artery disease. Clin. Chim. Acta. 504, 30–35. doi: 10.1016/j.cca.2020.01.030
Li Volti, G., Galvano, F., Frigiola, A., Guccione, S., Di Giacomo, C., Forte, S., et al. (2010). Potential immunoregulatory role of heme oxygenase-1 in human milk: a combined biochemical and molecular modeling approach. J. Nutr. Biochem. 21, 865–871. doi: 10.1016/j.jnutbio.2009.06.011
Maines, M. D. (1988). Heme oxygenase: function, multiplicity, regulatory mechanisms, and clinical applications. FASEB J. 2, 2557–2568.
Masaki, Y., Izumi, Y., Matsumura, A., Akaike, A., and Kume, T. (2017). Protective effect of Nrf2-ARE activator isolated from green perilla leaves on dopaminergic neuronal loss in a Parkinson’s disease model. Eur. J. Pharmacol. 798, 26–34. doi: 10.1016/j.ejphar.2017.02.005
Mateo, I., Infante, J., Sánchez-Juan, P., García-Gorostiaga, I., Rodríguez-Rodríguez, E., Vázquez-Higuera, J. L., et al. (2010). Serum heme oxygenase-1 levels are increased in Parkinson’s disease but not in Alzheimer’s disease. Acta. Neurol. Scand. 121, 136–138. doi: 10.1111/j.1600-0404.2009.01261.x
Poewe, W., Seppi, K., Tanner, C. M., Halliday, G. M., Brundin, P., Volkmann, J., et al. (2017). Parkinson disease. Nat. Rev. Dis. Primers 3:17013. doi: 10.1038/nrdp.2017.13
Ringman, J. M., Rodriguez, Y., Diaz-Olavarrieta, C., Chavez, M., Thompson, M., Fairbanks, L., et al. (2007). Performance on MMSE sub-items and education level in presenilin-1 mutation carriers without dementia. Int. Psychogeriatr. 19, 323–332. doi: 10.1017/S1041610206003772
Schipper, H. M. (1999). Glial HO-1 expression, iron deposition and oxidative stress in neurodegenerative diseases. Neurotox. Res. 1, 57–70. doi: 10.1007/BF03033339
Schipper, H. M. (2004). Heme oxygenase-1: transducer of pathological brain iron sequestration under oxidative stress. Ann. N. Y. Acad. Sci. 1012, 84–93. doi: 10.1196/annals.1306.007
Schipper, H. M., Chertkow, H., Mehindate, K., Frankel, D., Melmed, C., and Bergman, H. (2000). Evaluation of heme oxygenase-1 as a systemic biological marker of sporadic AD. Neurology 54, 1297–1304. doi: 10.1212/wnl.54.6.1297
Schipper, H. M., Cisse, S., and Stopa, E. G. (1995). Expression of heme oxygenase-1 in the senescent and Alzheimer-diseased brain. Ann. Neurol. 37, 758–768. doi: 10.1002/ana.410370609
Schipper, H. M., Liberman, A., and Stopa, E. G. (1998). Neural heme oxygenase-1 expression in idiopathic Parkinson’s disease. Exp. Neurol. 150, 60–68. doi: 10.1006/exnr.1997.6752
Schipper, H. M., Song, W., Tavitian, A., and Cressatti, M. (2019). The sinister face of heme oxygenase-1 in brain aging and disease. Prog. Neurobiol. 172, 40–70. doi: 10.1016/j.pneurobio.2018.06.008
Signorelli, S. S., Volsi, G. L., Fiore, V., Mangiafico, M., Barbagallo, I., Parenti, R., et al. (2016). Plasma heme oxygenase-1 is decreased in peripheral artery disease patients. Mol. Med. Rep. 14, 3459–3463. doi: 10.3892/mmr.2016.5644
Song, W., Cressatti, M., Zukor, H., Liberman, A., Galindez, C., and Schipper, H. M. (2017). Parkinsonian features in aging GFAP.HMOX1 transgenic mice overexpressing human HO-1 in the astroglial compartment. Neurobiol. Aging 58, 163–179. doi: 10.1016/j.neurobiolaging.2017.06.017
Song, W., Kothari, V., Velly, A. M., Cressatti, M., Liberman, A., Gornitsky, M., et al. (2018). Evaluation of salivary heme oxygenase-1 as a potential biomarker of early Parkinson’s disease. Mov. Disord. 33, 583–591. doi: 10.1002/mds.27328
Song, W., Patel, A., Qureshi, H. Y., Han, D., Schipper, H. M., and Paudel, H. K. (2009). The parkinson disease-associated a30p mutation stabilizes alpha-synuclein against proteasomal degradation triggered by heme oxygenase-1 over-expression in human neuroblastoma cells. J. Neurochem. 110, 719–733. doi: 10.1111/j.1471-4159.2009.06165.x
Song, W., Su, H., Song, S., Paudel, H. K., and Schipper, H. M. (2006). Over-expression of heme oxygenase-1 promotes oxidative mitochondrial damage in rat astroglia. J. Cell Physiol. 206, 655–663. doi: 10.1002/jcp.20509
Song, W., Zukor, H., Lin, S. H., Hascalovici, J., Liberman, A., Tavitian, A., et al. (2012). Schizophrenia-like features in transgenic mice overexpressing human HO-1 in the astrocytic compartment. J. Neurosci. 32, 10841–10853. doi: 10.1523/JNEUROSCI.6469-11.2012
Stocker, R. (1990). Induction of heme oxygenase as a defence against oxidative stress. Free Radic. Res. Commun. 9, 101–112. doi: 10.3109/10715769009148577
Tomlinson, C. L., Stowe, R., Patel, S., Rick, C., Gray, R., and Clarke, C. E. (2010). Systematic review of levodopa dose equivalency reporting in Parkinson’s disease. Mov. Disord. 25, 2649–2653. doi: 10.1002/mds.23429
Tong, H., Zhang, X., Meng, X., Lu, L., Mai, D., and Qu, S. (2018). Simvastatin inhibits activation of NADPH oxidase/p38 MAPK pathway and enhances expression of antioxidant protein in parkinson disease models. Front Mol. Neurosci. 11:165. doi: 10.3389/fnmol.2018.00165
van Mierlo, T. J., Chung, C., Foncke, E. M., Berendse, H. W., and van den Heuvel, O. A. (2015). Depressive symptoms in Parkinson’s disease are related to decreased hippocampus and amygdala volume. Mov. Disord. 30, 245–252. doi: 10.1002/mds.26112
Wolf, H., Grunwald, M., Kruggel, F., Riedel-Heller, S. G., Angerhöfer, S., Hojjatoleslami, A., et al. (2001). Hippocampal volume discriminates between normal cognition; questionable and mild dementia in the elderly. Neurobiol. Aging 22, 177–186. doi: 10.1016/s0197-4580(00)00238-4
Wolters, A. F., Moonen, A. J. H., Lopes, R., Leentjens, A. F. G., Duits, A. A., Defebvre, L., et al. (2020). Grey matter abnormalities are associated only with severe cognitive decline in early stages of Parkinson’s disease. Cortex 123, 1–11. doi: 10.1016/j.cortex.2019.09.015
Xuan, M., Guan, X., Huang, P., Shen, Z., Gu, Q., Yu, X., et al. (2019). Different patterns of gray matter density in early- and middle-late-onset Parkinson’s disease: a voxel-based morphometry study. Brain Imag. Behav. 13, 172–179. doi: 10.1007/s11682-017-9745-4
Yousaf, T., Dervenoulas, G., and Politis, M. (2018). Advances in MRI methodology. Int. Rev. Neurobiol. 141, 31–76. doi: 10.1016/bs.irn.2018.08.008
Keywords: biomarker, heme oxygenase-1, Parkinson’s disease, plasma, voxel-based morphometry
Citation: Sun W, Zheng J, Ma J, Wang Z, Shi X, Li M, Huang S, Hu S, Zhao Z and Li D (2021) Increased Plasma Heme Oxygenase-1 Levels in Patients With Early-Stage Parkinson’s Disease. Front. Aging Neurosci. 13:621508. doi: 10.3389/fnagi.2021.621508
Received: 26 October 2020; Accepted: 22 January 2021;
Published: 12 February 2021.
Edited by:
Shaomin Li, Brigham and Women’s Hospital and Harvard Medical School, United StatesReviewed by:
Marisa Cressatti, Jewish General Hospital, CanadaCopyright © 2021 Sun, Zheng, Ma, Wang, Shi, Li, Huang, Hu, Zhao and Li. This is an open-access article distributed under the terms of the Creative Commons Attribution License (CC BY). The use, distribution or reproduction in other forums is permitted, provided the original author(s) and the copyright owner(s) are credited and that the original publication in this journal is cited, in accordance with accepted academic practice. No use, distribution or reproduction is permitted which does not comply with these terms.
*Correspondence: Jianjun Ma, bWFqajExMjRAMTYzLmNvbQ==; Jinhua Zheng, NzMxNzYzNzk1QHFxLmNvbQ==
Disclaimer: All claims expressed in this article are solely those of the authors and do not necessarily represent those of their affiliated organizations, or those of the publisher, the editors and the reviewers. Any product that may be evaluated in this article or claim that may be made by its manufacturer is not guaranteed or endorsed by the publisher.
Research integrity at Frontiers
Learn more about the work of our research integrity team to safeguard the quality of each article we publish.