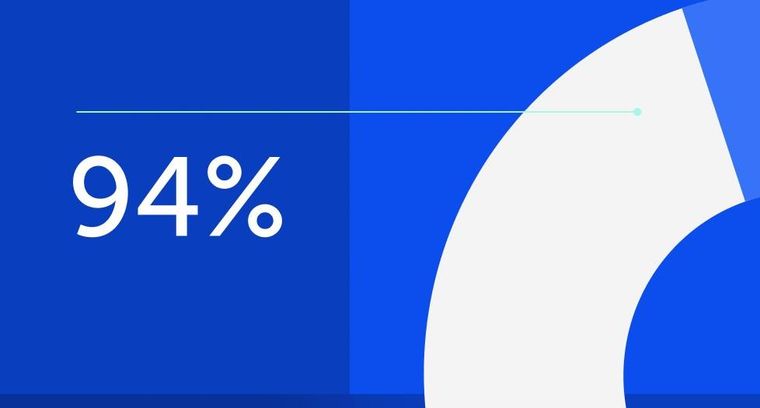
94% of researchers rate our articles as excellent or good
Learn more about the work of our research integrity team to safeguard the quality of each article we publish.
Find out more
ORIGINAL RESEARCH article
Front. Aging Neurosci., 15 April 2021
Sec. Neurocognitive Aging and Behavior
Volume 13 - 2021 | https://doi.org/10.3389/fnagi.2021.592845
Objective: There is growing evidence that testosterone may be implicated in the pathogenesis of Alzheimer’s disease (AD). We aimed to examine the relationship between plasma total testosterone levels and change in brain glucose metabolism over time among non-demented older people.
Methods: The association of plasma total testosterone levels with change in brain glucose metabolism among non-demented older people was investigated cross-sectionally and longitudinally. Given a significant difference in levels of plasma total testosterone between gender, we performed our analysis in a sex-stratified way. At baseline, 228 non-demented older people were included: 152 males and 76 females.
Results: In the cross-sectional analysis, no significant relationship between plasma total testosterone levels and brain glucose metabolism was found in males or females. In the longitudinal analysis, we found a significant association of plasma total testosterone levels with change in brain glucose metabolism over time in males, but not in females. More specifically, in males, higher levels of total testosterone in plasma at baseline were associated with slower decline in brain glucose metabolism.
Conclusion: We found that higher levels of total testosterone in plasma at baseline were associated with slower decline in brain glucose metabolism in males without dementia, indicating that testosterone may have beneficial effects on brain function.
There is growing evidence that testosterone may be implicated in the pathogenesis of Alzheimer’s disease (AD) (Holland et al., 2011). For example, males with AD and other dementias showed lower levels of total testosterone in serum compared to controls (Bowen et al., 2000; Hogervorst et al., 2001, 2003; Moffat et al., 2004; Paoletti et al., 2004). A number of cross-sectional studies have also suggested that testosterone levels are positively associated with memory performance, such as visual, working, and verbal memory (Barrett-Connor et al., 1999; Muller et al., 2005; Thilers et al., 2006; Van Strien et al., 2009). Additionally, the Baltimore Longitudinal Study of Aging (BLSA) with an average follow-up time of 19.1 years suggests that higher levels of free testosterone in serum at baseline are associated with lower risk of developing AD dementia (Moffat et al., 2004). Similarly, animal studies indicate a beneficial role of testosterone against tau phosphorylation (Papasozomenos and Shanavas, 2002) and β-amyloid (Aβ) production (Gouras et al., 2000). Further, Sundermann et al. (2020) found that lower testosterone levels in plasma were associated with higher CSF p-tau levels among APOE4 carriers. A recent meta-analysis of randomized clinical trials investigating the effects of testosterone supplementation on cognition suggested that testosterone supplementation may be a potentially preventative measure again cognitive impairment among cognitively normal older people (Tan et al., 2019). Taken together, these studies suggest that testosterone plays an important role in the pathogenesis of AD and may be neuroprotective.
Despite numerous studies supporting the notion that testosterone may affect cognitive performance and the risk of developing AD, the evidence on the relationship between testosterone and brain physiology is limited. In a cross-sectional study, Moffat and Resnick (2007) found that high circulating testosterone levels were positively associated with cerebral blood flow and brain glucose metabolism, which can be used to predict cognitive and functional decline in patients with cognitive impairment and AD (Landau et al., 2011). However, cross-sectional examination of the relationship between testosterone and brain glucose metabolism prohibits us from clarifying the temporal association of testosterone with brain glucose metabolism. Additionally, no previous studies have examined the relationship between plasma total testosterone levels and change in brain glucose metabolism over time among non-demented older people. If this relationship exists, it may provide novel insights into the effect of testosterone on brain function.
In the current study, we aimed to examine the association of plasma total testosterone levels at baseline with change in brain glucose metabolism over time among non-demented older people. Further, given the difference in levels of plasma total testosterone between gender, sex-stratified models were performed in males and females separately.
Data used in the present study were downloaded from the Alzheimer’s Disease Neuroimaging Initiative (ADNI), which was launched in 20031. The primary aim of the ADNI study has been to examine whether a variety of predictors, such as cognitive assessments, neuroimaging markers, and fluid biomarkers, can be integrated to assess the progression of mild cognitive impairment (MCI) and early AD. At every ADNI center, institutional review board approved the study, and each participant provided informed consent. In the present study, we selected 228 non-demented older people, including 26 individuals with normal cognition and 202 individuals with MCI. Participants with normal cognition had a Clinical Dementia Rating (CDR) (Morris, 1993) score of 0 and a Mini-Mental State Examination (MMSE) (Folstein et al., 1975) score of 24 or higher. Individuals with MCI had an MMSE score of 24 or higher, a CDR score of 0.5, objective memory impairment as examined by the Wechsler Memory Scale Logical Memory II, and an absence of dementia.
Demographical and clinical variables were obtained from the ADNI database. A flowchart of the data selection is presented (Figure 1).
Brain glucose metabolism was examined by FDG-PET. A standard protocol was used to process images2. ADNI researchers established a “MetaROI” of brain regions based on the finding that these regions show metabolic alterations in MCI or AD subjects which are closely associated with cognitive performance (Jagust et al., 2010; Landau et al., 2011). This MetaROI was made up of five regions, including Left Angular Gyrus, Right Angular Gyrus, Bilateral Posterior Cingular, Left Inferior Temporal Gyrus, and Right Inferior Temporal Gyrus. Standardized uptake value ratios (SUVRs) were used as dependent variable in our models. SUVRs were calculated by the formula: SUVRs = the average of FDG uptake of five MetaROI/the reference region (pons and cerebellum).
Plasma total testosterone levels were measured by a multiplex-based immunoassay panel developed by Rules-Based Medicine (MyriadRBM), details of which can be found at the website3. Values are given in ng/ml. Plasma total testosterone data were log10-transformed in order to normalize the distribution before entering our analysis.
The t-test was utilized to determine the differences in continuous variables (age, education, MMSE, and plasma total testosterone levels). The chi-square test was used to compare the distribution of categorical variable (APOE4 genotype) between males and females. To examine the association of plasma total testosterone levels with change in brain glucose metabolism over time, linear mixed models were performed with FDG SUVRs as dependent variable. Given that males showed significantly higher levels of plasma total testosterone than females (mean: 0.43 vs −0.36), sex-stratified analyses were conducted. In males, levels of plasma total testosterone were categorized into two groups based on the median (0.48). In females, levels of plasma total testosterone were categorized into two groups based on the median (−0.26). Thus, plasma total testosterone was regarded as categorical variable in our models. All models included main effects of plasma total testosterone (in males: ≤0.48 vs >0.48; in females: ≤−0.26 vs >0.26), age, APOE4 genotype, education, and their interactions with time. In addition, models also included a random intercept for each subject. In our linear mixed models, a row of the long-format data frame that has a missing value was removed from the analysis. When missing values occurred for FDG SUVRs (the dependent variable) during follow-up visits, a participant was included in our linear mixed model if the participant had one or more non-missing time point.
All statistical work was conducted using R software (version 4.0.0) (R Core Team, 2013).
Table 1 shows the demographical and clinical information of 228 non-demented older people: 152 males and 76 females. There were no significant differences in age, education, APOE4 genotype, MMSE, or FDG SUVRs between males and females (all p > 0.05). However, males showed significantly higher levels of plasma total testosterone than females (p < 0.001). The number of participants at follow-up visits is also displayed in Table 1.
T-tests were conducted to examine the relationship between plasma total testosterone levels and FDG SUVRs. Given that males showed significantly higher levels of plasma total testosterone than females (mean: 0.43 vs −0.36, Table 1), sex-stratified analyses were conducted. In males, levels of plasma total testosterone were categorized into two groups based on the median (0.48). In females, levels of plasma total testosterone were categorized into two groups based on the median (−0.26). However, there was no statistically significant relationship between plasma total testosterone levels and FDG SUVRs in males or females (all p > 0.05, Figure 2).
Figure 2. Cross-sectional relationship between plasma testosterone and FDG SUVRs. (A) In males, there was no statistically significant relationship between plasma total testosterone levels and FDG SUVRs (p > 0.05). (B) In females, there was no statistically significant relationship between plasma total testosterone levels and FDG SUVRs (p > 0.05).
To examine the longitudinal association of plasma total testosterone levels with FDG SUVRs, linear mixed models were conducted. In males, higher plasma testosterone levels were significantly associated with slower decline in FDG SUVRs (estimate: 0.012, p < 0.001, Table 2 and Figure 3A). However, in females, we did not find a relationship between plasma total testosterone levels and change in FDG SUVRs over time (estimate: −0.0013, p = 0.7269, Table 3 and Figure 3B).
Figure 3. Longitudinal association of plasma total testosterone levels with FDG SUVRs in males and females. (A) In males, higher plasma testosterone levels were significantly associated with slower decline in FDG SUVRs. (B) In females, we did not find a relationship between plasma total testosterone levels and change in FDG SUVRs over time.
In this study, we examined the relationship between plasma total testosterone levels and brain glucose metabolism both cross-sectionally and longitudinally among non-demented older people. Given a significant difference in levels of plasma total testosterone between gender, the analysis was performed in a sex-stratified way. In the cross-sectional analysis, we did not find a significant relationship between plasma total testosterone levels and brain glucose metabolism in males or females. In the longitudinal analysis, we found a significant relationship between plasma total testosterone levels and change in brain glucose metabolism over time in males, but not in females. More specifically, in males, higher levels of total testosterone in plasma at baseline were associated with slower decline in brain glucose metabolism, indicating that testosterone may have beneficial effects on brain function.
The key finding that higher plasma total testosterone levels at baseline were associated with slower decline in brain glucose metabolism among non-demented older men is in line with other previously published findings showing that testosterone may have protective effects on brain function. For instance, compared to healthy controls, patients with dementia showed significantly lower testosterone levels in serum (Bowen et al., 2000). In addition, several longitudinal studies suggested that reduced testosterone is an independent risk factor for developing AD (Moffat et al., 2004; Chu et al., 2010), and that higher testosterone may thus protect against cognitive impairment and AD dementia. Although emerging evidence suggests a relationship between higher testosterone and better cognitive performance and lower risk of developing AD dementia, the evidence regarding the effect of testosterone on brain glucose metabolism is limited. Our study, for the first time, provides evidence that higher testosterone may slow down the process of brain glucose hypometabolism among non-demented older men.
It is also biologically plausible that testosterone could have some beneficial effects on brain function. First, it is possible that testosterone may reduce levels of amyloid plaques and tangles in the brain, thus contributing to higher brain glucose metabolism. For example, animal studies indicate a beneficial role of testosterone against tau phosphorylation (Papasozomenos and Shanavas, 2002) and Aβ production (Gouras et al., 2000). Second, testosterone has been reported to be beneficial to human primary neurons, and this beneficial effect is not dependent on estrogen action (Hammond et al., 2001). Third, in hippocampal CA1 neurons, androgen administration can protect against NMDA excitotoxicity and may accelerate the process of recovery after injury through enhancing fiber outgrowth (Morse et al., 1992).
This study has several limitations. First, we cannot provide a causal evidence due to the observational nature of this study. Clinical trials are required to examine whether testosterone could slow down the speed of brain glucose hypometabolism and thus protect against AD. Second, given that the ADNI study represents a convenience sample of volunteers, selection bias should be considered. Third, in ADNI, plasma levels of total testosterone were measured on the Luminex xMAP platform by Biomarkers Consortium Plasma Proteomics Project Rules-Based Medicine multiplex, but not measured by other widely used and well-validated methods, such as isotope dilution liquid chromatography tandem mass spectrometry. Therefore, our findings should be interpreted with caution, and other studies are needed to replicate our findings in the future.
In conclusion, we found that higher levels of total testosterone in plasma at baseline were associated with slower decline in brain glucose metabolism in males without dementia, indicating that testosterone may have beneficial effects on brain function.
The ADNI data presented in this article are publicly accessible. Requests to access the datasets should be directed to http://adni.loni.usc.edu/.
At every ADNI center, institutional review board approved the study (adni.loni.usc.edu). The patients/participants provided their written informed consent to participate in this study.
TY and XW designed and supervised the study. XW, ZL, QW, HL, and YG performed the research, analyzed the data, and wrote the manuscript. All authors approved the final version of manuscript.
The authors declare that the research was conducted in the absence of any commercial or financial relationships that could be construed as a potential conflict of interest.
Data collection and sharing for this project was funded by the Alzheimer’s Disease Neuroimaging Initiative (ADNI) (National Institutes of Health Grant U01 AG024904) and DOD ADNI (Department of Defense award number W81XWH-12-2-0012). ADNI was funded by the National Institute on Aging, the National Institute of Biomedical Imaging and Bioengineering, and through generous contributions from the following: AbbVie, Alzheimer’s Association; Alzheimer’s Drug Discovery Foundation; Araclon Biotech; BioClinica, Inc.; Biogen; Bristol-Myers Squibb Company; CereSpir, Inc.; Cogstate; Eisai Inc.; Elan Pharmaceuticals, Inc.; Eli Lilly and Company; EuroImmun; F. Hoffmann-La Roche Ltd. and its affiliated company Genentech, Inc.; Fujirebio; GE Healthcare; IXICO Ltd.; Janssen Alzheimer Immunotherapy Research & Development, LLC.; Johnson & Johnson Pharmaceutical Research & Development LLC.; Lumosity; Lundbeck; Merck & Co., Inc.; Meso Scale Diagnostics, LLC.; NeuroRx Research; Neurotrack Technologies; Novartis Pharmaceuticals Corporation; Pfizer Inc.; Piramal Imaging; Servier; Takeda Pharmaceutical Company; and Transition Therapeutics. The Canadian Institutes of Health Research is providing funds to support ADNI clinical sites in Canada. Private sector contributions are facilitated by the Foundation for the National Institutes of Health (www.fnih.org). The grantee organization is the Northern California Institute for Research and Education, and the study is coordinated by the Alzheimer’s Therapeutic Research Institute at the University of Southern California. ADNI data are disseminated by the Laboratory for Neuro Imaging at the University of Southern California.
Barrett-Connor, E., Goodman-Gruen, D., and Patay, B. (1999). Endogenous sex hormones and cognitive function in older men. J. Clin. Endocrinol. Metab. 84, 3681–3685. doi: 10.1210/jc.84.10.3681
Bowen, R. L., Isley, J. P., and Atkinson, R. L. (2000). An association of elevated serum gonadotropin concentrations and Alzheimer disease? J. Neuroendocrinol. 12, 351–354. doi: 10.1046/j.1365-2826.2000.00461.x
Chu, L. W., Tam, S., Wong, R. L., Yik, P. Y., Song, Y., Cheung, B. M., et al. (2010). Bioavailable testosterone predicts a lower risk of Alzheimer’s disease in older men. J. Alzheimers Dis. 21, 1335–1345. doi: 10.3233/jad-2010-100027
Folstein, M. F., Folstein, S. E., and Mchugh, P. R. (1975). “Mini-mental state”. A practical method for grading the cognitive state of patients for the clinician. J. Psychiatr. Res. 12, 189–198.
Gouras, G. K., Xu, H., Gross, R. S., Greenfield, J. P., Hai, B., Wang, R., et al. (2000). Testosterone reduces neuronal secretion of Alzheimer’s beta-amyloid peptides. Proc. Natl. Acad. Sci. U.S.A. 97, 1202–1205. doi: 10.1073/pnas.97.3.1202
Hammond, J., Le, Q., Goodyer, C., Gelfand, M., Trifiro, M., and Leblanc, A. (2001). Testosterone-mediated neuroprotection through the androgen receptor in human primary neurons. J. Neurochem. 77, 1319–1326. doi: 10.1046/j.1471-4159.2001.00345.x
Hogervorst, E., Combrinck, M., and Smith, A. D. (2003). Testosterone and gonadotropin levels in men with dementia. Neuro Endocrinol. Lett. 24, 203–208.
Hogervorst, E., Williams, J., Budge, M., Barnetson, L., Combrinck, M., and Smith, A. D. (2001). Serum total testosterone is lower in men with Alzheimer’s disease. Neuro Endocrinol. Lett. 22, 163–168.
Holland, J., Bandelow, S., and Hogervorst, E. (2011). Testosterone levels and cognition in elderly men: a review. Maturitas 69, 322–337. doi: 10.1016/j.maturitas.2011.05.012
Jagust, W. J., Bandy, D., Chen, K., Foster, N. L., Landau, S. M., Mathis, C. A., et al. (2010). The Alzheimer’s disease neuroimaging initiative positron emission tomography core. Alzheimers. Dement. 6, 221–229.
Landau, S. M., Harvey, D., Madison, C. M., Koeppe, R. A., Reiman, E. M., Foster, N. L., et al. (2011). Associations between cognitive, functional, and FDG-PET measures of decline in AD and MCI. Neurobiol. Aging 32, 1207–1218. doi: 10.1016/j.neurobiolaging.2009.07.002
Moffat, S. D., and Resnick, S. M. (2007). Long-term measures of free testosterone predict regional cerebral blood flow patterns in elderly men. Neurobiol. Aging 28, 914–920. doi: 10.1016/j.neurobiolaging.2006.04.001
Moffat, S. D., Zonderman, A. B., Metter, E. J., Kawas, C., Blackman, M. R., Harman, S. M., et al. (2004). Free testosterone and risk for Alzheimer disease in older men. Neurology 62, 188–193. doi: 10.1212/wnl.62.2.188
Morris, J. C. (1993). The Clinical Dementia Rating (CDR): current version and scoring rules. Neurology 43, 2412–2414. doi: 10.1212/wnl.43.11.2412-a
Morse, J. K., Dekosky, S. T., and Scheff, S. W. (1992). Neurotrophic effects of steroids on lesion-induced growth in the hippocampus. II. Hormone replacement. Exp. Neurol. 118, 47–52. doi: 10.1016/0014-4886(92)90021-h
Muller, M., Aleman, A., Grobbee, D. E., De Haan, E. H., and Van Der Schouw, Y. T. (2005). Endogenous sex hormone levels and cognitive function in aging men: is there an optimal level? Neurology 64, 866–871. doi: 10.1212/01.wnl.0000153072.54068.e3
Paoletti, A. M., Congia, S., Lello, S., Tedde, D., Orr, M., Pistis, M., et al. (2004). Low androgenization index in elderly women and elderly men with Alzheimer’s disease. Neurology 62, 301–303. doi: 10.1212/01.wnl.0000094199.60829.f5
Papasozomenos, S., and Shanavas, A. (2002). Testosterone prevents the heat shock-induced overactivation of glycogen synthase kinase-3 beta but not of cyclin-dependent kinase 5 and c-Jun NH2-terminal kinase and concomitantly abolishes hyperphosphorylation of tau: implications for Alzheimer’s disease. Proc. Natl. Acad. Sci. U.S.A. 99, 1140–1145. doi: 10.1073/pnas.032646799
R Core Team (2013). R: A Language and Environment for Statistical Computing. Vienna: R Foundation for Statistical Computing.
Sundermann, E. E., Panizzon, M. S., Chen, X., Andrews, M., Galasko, D., and Banks, S. J. (2020). Sex differences in Alzheimer’s-related Tau biomarkers and a mediating effect of testosterone. Biol. Sex Differ. 11:33.
Tan, S., Sohrabi, H. R., Weinborn, M., Tegg, M., Bucks, R. S., Taddei, K., et al. (2019). Effects of testosterone supplementation on separate cognitive domains in cognitively healthy older men: a meta-analysis of current randomized clinical trials. Am. J. Geriatr. Psychiatry 27, 1232–1246. doi: 10.1016/j.jagp.2019.05.008
Thilers, P. P., Macdonald, S. W., and Herlitz, A. (2006). The association between endogenous free testosterone and cognitive performance: a population-based study in 35 to 90 year-old men and women. Psychoneuroendocrinology 31, 565–576. doi: 10.1016/j.psyneuen.2005.12.005
Keywords: testosterone, Alzheimer’s disease, brain glucose metabolism, FDG, longitudinal study
Citation: Wang X, Lv Z, Wu Q, Liu H, Gu Y and Ye T (2021) Lower Plasma Total Testosterone Levels Were Associated With Steeper Decline in Brain Glucose Metabolism in Non-demented Older Men. Front. Aging Neurosci. 13:592845. doi: 10.3389/fnagi.2021.592845
Received: 08 August 2020; Accepted: 17 March 2021;
Published: 15 April 2021.
Edited by:
Christian J. Pike, University of Southern California, Los Angeles, United StatesReviewed by:
Amy Christensen, University of Southern California, Los Angeles, United StatesCopyright © 2021 Wang, Lv, Wu, Liu, Gu and Ye. This is an open-access article distributed under the terms of the Creative Commons Attribution License (CC BY). The use, distribution or reproduction in other forums is permitted, provided the original author(s) and the copyright owner(s) are credited and that the original publication in this journal is cited, in accordance with accepted academic practice. No use, distribution or reproduction is permitted which does not comply with these terms.
*Correspondence: Teng Ye, MTU4NTg4MDYyMTJAMTYzLmNvbQ==
†These authors have contributed equally to this work
‡Data used in preparation of this article were obtained from the Alzheimer’s Disease Neuroimaging Initiative (ADNI) database (adni.loni.usc.edu). As such, the investigators within the ADNI contributed to the design and implementation of ADNI and/or provided data but did not participate in analysis or writing of this report. A complete listing of ADNI investigators can be found at: http://adni.loni.usc.edu/wp-content/uploads/how_to_apply/ADNI_Acknowledgement_List.pdf
Disclaimer: All claims expressed in this article are solely those of the authors and do not necessarily represent those of their affiliated organizations, or those of the publisher, the editors and the reviewers. Any product that may be evaluated in this article or claim that may be made by its manufacturer is not guaranteed or endorsed by the publisher.
Research integrity at Frontiers
Learn more about the work of our research integrity team to safeguard the quality of each article we publish.