- 1Clinical Research Center, The First Affiliated Hospital of Xi’an Jiaotong University, Xi’an, China
- 2Department of Neurology, The First Affiliated Hospital of Xi’an Jiaotong University, Xi’an, China
- 3Department of Neurology, Huxian Hospital of Traditional Chinese Medicine, Xi’an, China
Objectives: Recent studies regarding the relationships between plasma amyloid-β (Aβ) levels and cognitive performance had inconsistent results. In this study, we aimed to characterize the relationship between cognitive decline and plasma Aβ levels in a large-sample cognitively normal population.
Methods: This population-based, prospective cohort study included 1,240 participants with normal cognition. The Mini-Mental State Examination (MMSE) was used to assess cognitive function at baseline and 2 years later. Restricted cubic splines, multivariate logistic regression, and multivariate linear regression models were used to evaluate the type of relationship between cognitive decline during the 2-year follow-up period and plasma Aβ levels (Aβ40, Aβ42, and Aβ42/40).
Results: Participants with moderate Aβ40 levels had the highest risk of cognitive decline during a 2-year follow-up relative to individuals with low Aβ40 [odds ratio (OR): 0.60, 95% confidence interval (CI): 0.45–0.81, p < 0.001] or high Aβ40 (OR: 0.65, 95% CI: 0.49–0.87, p = 0.004) levels. The association between Aβ40 and cognitive decline did not depend on sex, education level, or APOE ε4 status. There was an interaction found between age (≤ 65 and > 65 years) and Aβ40 (p for interaction = 0.021). In individuals older than 65 years, there was a positive linear relationship between plasma Aβ40 and cognitive decline (OR: 1.02, 95% CI: 1.00–1.04, p = 0.027). For participants ≤ 65 years old, the lower Aβ40 and higher Aβ40 groups had a lower risk of cognitive decline than the medium Aβ40 group (OR: 0.69, 95% CI: 0.50–0.94, p = 0.02; OR: 0.63, 95% CI: 0.45–0.86, p = 0.004). None of relationship between plasma Aβ42, Aβ42/40 and cognitive decline was found during a 2-year follow-up.
Conclusion: The relationship between plasma Aβ40 and cognitive decline was not linear, but an inverted-U shape in a cognitively normal population. The underlying mechanism requires further investigation.
Introduction
Amyloid-β (Aβ) pathology has been confirmed as a pathological change in the early phase of Alzheimer ‘s disease (AD) (Sperling et al., 2011; Yaffe, 2011; Dubois et al., 2016; Jack et al., 2018; Nakamura et al., 2018; Jansen et al., 2018). Abnormal Aβ deposition in the brain increases the risk of cognitive decline and the development of dementia (Villemagne et al., 2011; van Harten et al., 2013; Jaunmuktane et al., 2015; Toledo et al., 2015; Donohue et al., 2017; Roberts et al., 2018; Greenberg et al., 2020). Accelerating our understanding of the relationship between amyloid pathology and cognitive functioning can contribute to the efficient screening of preclinical AD at an early stage, and considerably delay the progression of the disease (Jansen et al., 2018).
Amyloid pathology, visualized on positron emission tomography (PET) scans or measured in cerebrospinal fluid (CSF), has been extensively studied and has proven clinical applications for AD (Sperling et al., 2013; Vos et al., 2013; Toledo et al., 2015; Dubois et al., 2016; Olsson et al., 2016; Donohue et al., 2017). However, because these techniques are invasive or expensive, more economical and easily-acquired plasma Aβ measurements are needed. Recent studies have revealed that plasma Aβ are related to brain Aβ burden and incident dementia (Graff-Radford et al., 2007; Yaffe, 2011; Gabelle et al., 2013; Hanon et al., 2018; Hilal et al., 2018; Nakamura et al., 2018; Verberk et al., 2018; Chen et al., 2019; de Wolf et al., 2020), however, the relationships between plasma Aβ levels and cognitive performance were inconsistent. Previous studies reported that lower plasma Aβ42/40 or Aβ42 increased the risk of cognitive decline and the progression of dementia (Yaffe, 2011; Gabelle et al., 2013; Hilal et al., 2018; Verberk et al., 2018; de Wolf et al., 2020). Other studies have suggested that a positive relationship between plasma Aβ42, Aβ42/40 and cognitive impairment (Hanon et al., 2018; Wang et al., 2018; Chen et al., 2019).
In order to rapidly identify patients with cognitive decline earlier, and therefore delay the occurrence of AD, the present study aimed to determine the change in cognitive status, its relationship with plasma Aβ, and the type of association, during a 2-year follow-up in a cognitively normal population. This study also investigated whether this association was altered by sex, age, education level, or Apolipoprotein E (APOE) genotype, and estimated the relationship between plasma Aβ and cognitive disorder and cardio-cerebrovascular diseases during a 2-year follow-up.
Materials and Methods
Study Population
Participants were enrolled from a village in the suburbs of Xi’an city, located in northwestern China, between October, 2014 and November, 2015. This study was a community population cohort study in which each interviewee received a face-to-face questionnaire. Participants were asked about detailed history by neurologists, and received a nervous system examination, a systemic physical examination, blood biochemical examination aimed at determining whether there were any related neurological diseases. All patients with cerebral disease had received the CT or MRI scans. For patients who had a diagnosis of mild cognitive impairment (MCI) or dementia due to AD, we provided free MRI scan to confirm the diagnosis of AD in a local designated hospital. Inclusion criteria was that participants were (1) older than 40 years and (2) a current resident of the village and had lived there for more than 3 years. Exclusion criteria was that (1) the Mini-Mental State Examination (MMSE) was not completed; (2) there was evidence of MCI or dementia, or (3) other neurological conditions that may influence cognitive function (such as epilepsia, central nervous system infections, essential tremor, Parkinsonism, anxiety, depression, thyroid hypofunction, intracranial trauma, or surgery); (4) plasma Aβ was not measured; and (5) other baseline information was missing. This study was approved by the First Affiliated Hospital of Xi’an Jiaotong University. Written informed consent was obtained from all participants.
Cognitive Evaluation
The MMSE was conducted as global cognitive screening at enrollment. The MMSE, other neurological, physical and imaging examinations were assessed at baseline and repeated 2 years later. Cutoff values were ≤ 17 for subjects with illiteracy, ≤20 for primary school educated subjects, and ≤ 24 for those educated at the junior high school level or above (Bohm et al., 2015). After initial screening, study participants with MMSE score ≤ these cutoff values underwent the 2nd phase cognitive examinations, including the Fuld Object Memory Evaluation test, Rapid Verbal Retrieval test, Trail-Making test, Digit Span test, and Block Design test. Finally, combining all the available imaging and clinical information, the diagnosis and subtype of dementia and MCI were determined according to international diagnostic criteria for dementia (American Psychiatric Association [APA], 2000) and Petersen MCI criteria (Petersen, 2011) by a panel of senior clinicians. The diagnosis of AD was based on NINCDS-ADRDA criteria (McKhann et al., 2011).
Laboratory Measurement
All plasma samples were acquired between 7 and 11 a.m. Plasma Aβ40 and Aβ42 tests were measured using enzyme-linked immunosorbent assay kits (ELISA, Yuanye Co. Shanghai, China) (Wei et al., 2017; Jiang et al., 2018). Concentrations of Aβ40 and Aβ42 were conducted in duplicate and determined from standard curves using a Rayto RT-6000 analyzer (Rayto Co., Shenzhen, China) at OD 450 nm. Apolipoprotein E (APOE) was genotyped by polymerase chain reaction (PCR), followed by sequencing, as previously described (Wenham et al., 1991).
Outcome Definition
The primary outcome was cognitive decline, which was defined as the difference of MMSE between the first visit and the second visit > 0. Cognitive disorder was defined as MMSE score ≤ 17 for illiterate, ≤ 20 for primary school educated, and ≤ 24 for junior high school educated or above (Katzman et al., 1988). New cardiovascular and cerebrovascular diseases were defined as incident cardiovascular and cerebrovascular diseases during the 2-year follow-up.
Other Covariates
Lack of physical exercise was defined as exercising fewer than three times a week, fewer than 30 min a time. Smoking was defined as a current smoker. Diabetes mellitus was defined as a self-reported medical diagnosis, diabetic medication use, a fasting glucose of ≥ 7.0 mmol/l, a random plasma glucose concentration ≥ 11.1 mmol/l, or glycated hemoglobin ≥ 6.5%. Hypertension was defined as a self-reported medical diagnosis, antihypertensive medication use, a systolic blood pressure of ≥ 140 mmHg, or a diastolic blood pressure of ≥ 90 mmHg.
Statistical Analysis
Continuous variables are presented as mean ± SD, and categorical variables are presented as proportions. We determined the association between baseline information and cognitive decline using Pearson’s χ2 or Fisher’s exact test for categorical variables, and Student’s t-test or Mann–Whitney U-test for continuous variables. Differences of basic characteristics between different Aβ groups were compared using Pearson’s χ2 or Fisher’s exact test for categorical variables, and ANOVA test or Kruskal-Wallis H test for continuous variables.
Restricted cubic splines with a logistic regression model were applied to test the relationship between cognitive decline and Aβ levels, and to evaluate the type of correlation of cognitive decline with Aβ levels. Four knots defined at the 5th, 35th, 65th, and 95th percentiles of Aβ levels were prespecified (Harrell, 2001). The reference point was the mean value of Aβ levels. Aβ categories were based on the type of relationship between cognitive decline, and Aβ levels suggested in restricted cubic splines. A series of pre-planned categories of Aβ levels were tested, defined by tertiles and interquartiles. The differences in MMSE scores between baseline and 2 years later were compared with the paired t-test.
To assess the association between Aβ levels (categorical and continuous) and various outcomes, unadjusted, age- and sex-adjusted, and multivariable-adjusted logistic regression models were used. A multivariable linear regression model was used to evaluate the relationship between Aβ and 2-year MMSE score change. In multivariable models, adjustments were made for age, sex, diabetes, hypertension, smoking, lack of physical activity, APOE ε4 status, and education. In subgroup analyses, we examined the relationship between Aβ40 and cognitive decline by sex, age (≤ 65 and > 65 years), education (≥ high school and < high school), and APOE ε4 status in multivariable-adjusted logistic regression models. SPSS ver. 24.0 and R ver. 3.5.3 were used for statistical analysis. A p < 0.05 was considered significant.
Results
Characteristics of the Study Population at Baseline
The study population screening process is shown in Figure 1. There were 2,173 individuals examined from October, 2014 to November, 2015. A total of 138 participants did not complete the MMSE, 58 had MCI or dementia, 112 had neurologically-related diseases, 470 did not undergo plasma Aβ tests, 131 had missing baseline information, and 24 did not complete the follow-up MMSE. Finally, 1,240 participants were included in our study.
Baseline characteristics of the study population are listed in Table 1. Among 1240 participants, 456 experienced cognitive decline. The mean age of the study participants was 55.2 ± 9.7 years and 469 (37.8%) were men. In the group without cognitive decline, the percentage of higher education level was higher (63.3%) than that in the group with cognitive decline (55.3%, p = 0.005). MMSE score was higher (27 ± 3.2) in the group with cognitive decline than that in the population without cognitive decline(25.5 ± 3.7, p < 0.001. There were no other significant differences between two groups.
Association Between Continuous Aβ40, Aβ42, Aβ42/40 Levels and Cognitive Decline
The associations between continuous Aβ40, Aβ42, Aβ42/40 levels and cognitive decline were listed in Table 2. After multivariable adjustment, none of three biomarkers reached statistical significance (all p > 0.05).
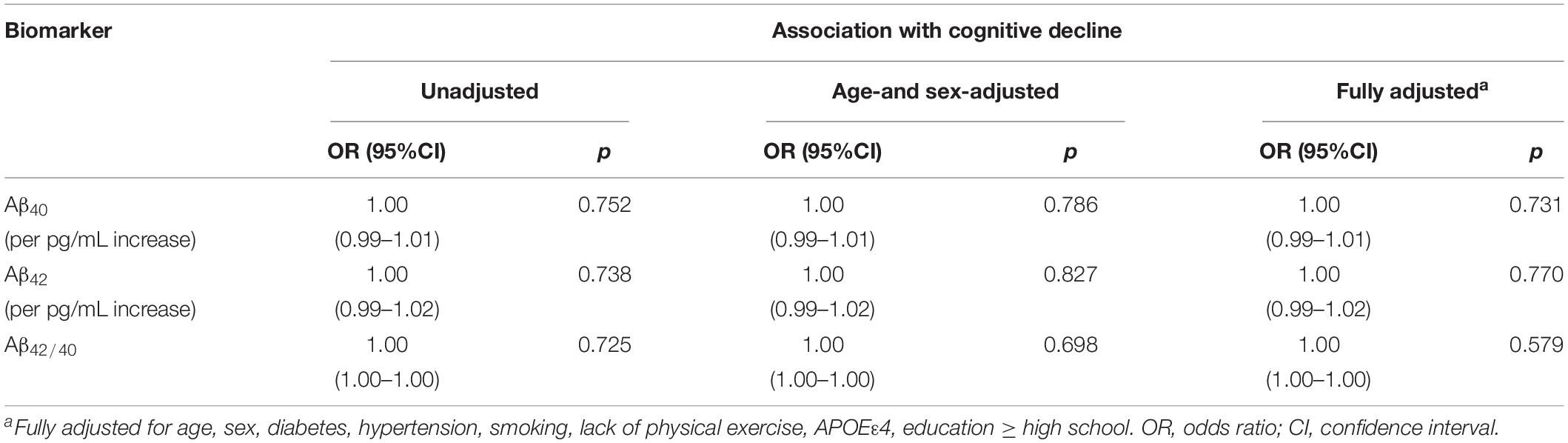
Table 2. Association of plasma levels of amyloid-β 40, amyloid-β 42, and the amyloid-β 42/amyloid-β 40 ratio with cognitive decline.
The associations between Aβ levels and cognitive decline using restricted cubic splines are shown in Figure 2. Aβ40 showed a non-linear relationship (p overall = 0.040, p non-linear = 0.017) with cognitive decline. Aβ42 and Aβ42/40 didn’t show any relationship with cognitive decline (p overall > 0.05; p non-linear > 0.05).
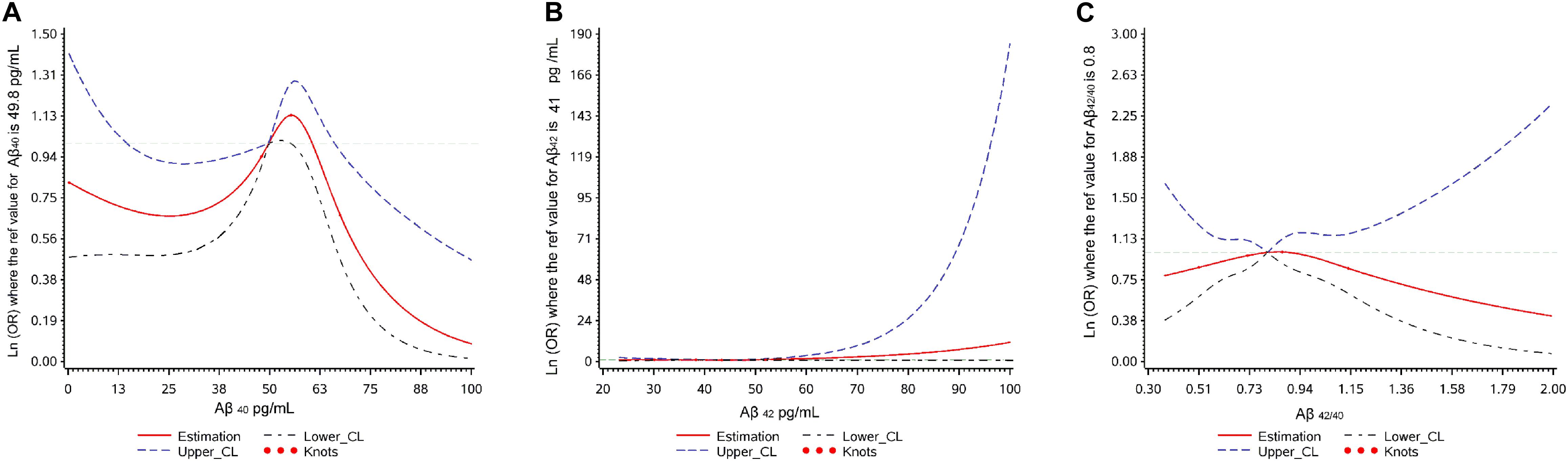
Figure 2. Plot of dose-response relationship between plasma Aβ levels and cognitive decline during two-year follow-up.
Non-linear Relationship Between Plasma Aβ40 Level and Cognitive Decline
Because of the non-linear relationship between plasma Aβ40 and cognitive decline, and the inverted U shape found in the restricted cubic spline, we transformed continuous Aβ40 into two kinds of categorical variables: three tertiles defined by the 33rd percentiles and 66th percentiles, and 3-class classified variables defined by the 25th and 75th percentiles. In multivariate logistic regression analyses evaluating the relationship between cognitive decline and categorical Aβ40, 3-classified Aβ40 had a better goodness of fit (χ2 = 32.20, p < 0.001) than Aβ40 defined by the 33rd and 66th percentiles (χ2 = 15.44, p = 0.117). Therefore, 3-classified Aβ40, defined by the 25th and 75th percentiles, was used in the present study.
Two-year changes in MMSE score in the three Aβ40 groups are shown in Figure 3. Paired comparisons of MMSE scores between baseline and 2 years later in the medium and high Aβ40 groups were significant (p < 0.001, Figure 3A). When plasma was 45 pg/mL ≤ Aβ40 < 58.4 pg/mL, the change in MMSE scores between the 2 visits was more evident than in the other 2 groups (Figure 3B). The changes in MMSE scores in the low, medium, and high Aβ40 groups were 0.09 ± 1.53, 0.65 ± 1.89, and 0.39 ± 1.78 pg/mL respectively. Baseline characteristics of the study population, stratified by low (< 45 pg/mL), medium (45 pg/mL ≤ Aβ40 < 58.4 pg/mL), and high Aβ40 (≥58.4 pg/mL) are listed in Supplementary Table S1.
The relationship between plasma Aβ40 and various outcomes during follow-up using multivariable logistic regression models are listed in Table 3. In unadjusted analyses, compared with participants with Aβ40 between 45 and 58.4 pg/mL, participants in the low Aβ40 group and high Aβ40 group had lower risks of cognitive decline (OR: 0.61, 95% CI: 0.46–0.82, p < 0.001; OR: 0.67, 95% CI: 0.50–0.89, p = 0.006). After adjustments for age and sex, the results were the same as before. In the fully adjusted model, participants in the low Aβ40 (< 45 pg/mL) and high Aβ40 groups (≥58.4 pg/mL) still had a lower risk of cognitive decline, compared with the medium Aβ40 group (OR: 0.60, 95% CI: 0.45–0.81, p < 0.001; OR: 0.65, 95% CI: 0.49–0.87, p = 0.004). In addition, participants in the low Aβ40 group had a lower risk of a new cognitive disorder (OR: 0.29, 95% CI: 0.12–0.70, p = 0.006) than participants in the medium Aβ40 group, while participants with high Aβ40 levels did not show this association. There were no significant associations found in other outcomes. The relationships of categorical Aβ40 defined by the 33th percentiles and 66th percentiles, and cognitive decline are listed in Supplementary Table S2.
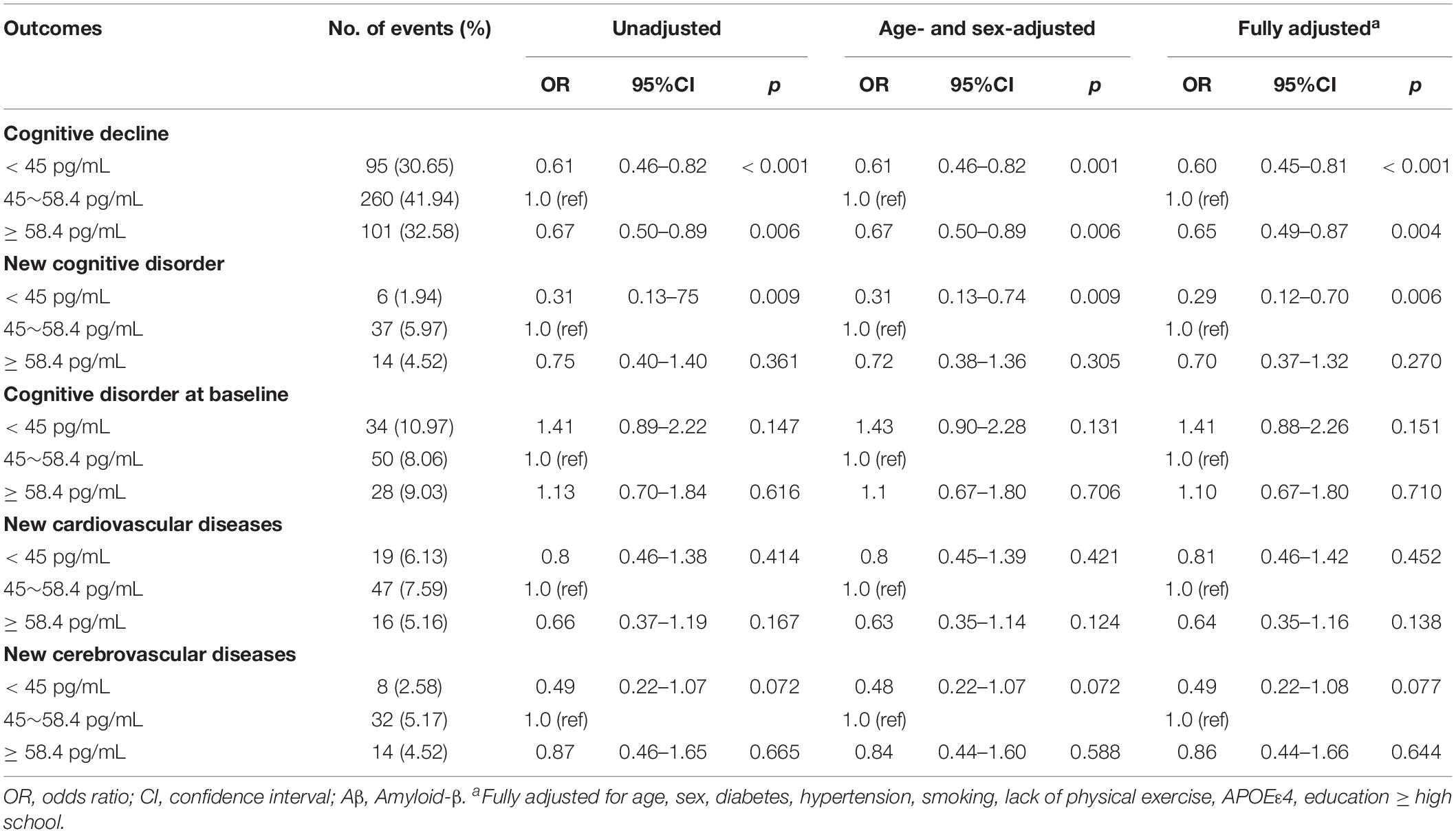
Table 3. Results of multivariable logistic regression models examining the relationship between plasma Aβ40 and various outcomes during follow-up.
The relationship between plasma Aβ40 and 2-year change in MMSE score using a multivariate linear regression model is shown in Figure 4. An inverted U-shaped association between Aβ40 and MMSE score change was still apparent. Compared with participants with medium Aβ40, those with low Aβ40 and high Aβ40 had a lower risk of MMSE score change (β: −0.57, 95% CI: −0.81 to −0.33, p < 0.001; β: −0.29, 95% CI: −0.53 to −0.05, p = 0.020).
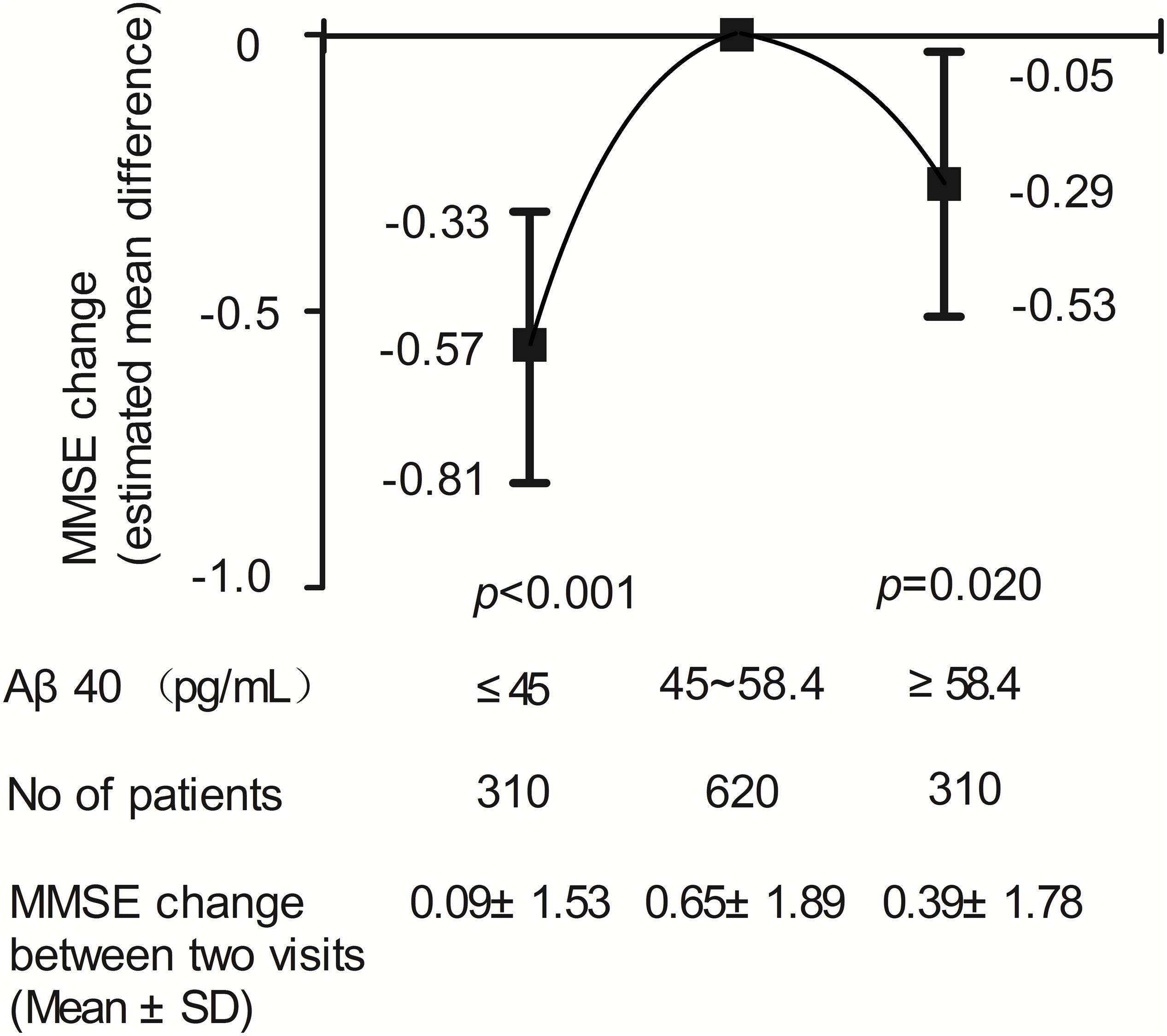
Figure 4. Results of multivariable linear regression analysis evaluating the relation between plasma Aβ40 and cognitive decline during two-year follow-up.
Subgroup Analyses of the Relationship Between Plasma Aβ40 and Cognitive Decline
Subgroup analyses of the relationship between plasma Aβ40 and cognitive decline are listed in Table 4. The association between Aβ40 and cognitive decline did not depend on sex, education level, or APOE ε4 status. There was an interaction found between age (≤ 65 and > 65 years) and Aβ40 (p for interaction = 0.021). For participants ≤ 65 years old, the lower Aβ40 and higher Aβ40 groups had a lower risk of cognitive decline than the medium Aβ40 group (OR: 0.69, 95% CI: 0.50–0.94, p = 0.02; OR: 0.63, 95% CI: 0.45–0.86, p = 0.004). In participants aged > 65 years, lower Aβ40 levels correlated with cognitive decline (OR: 0.29, 95% CI: 0.14–0.63, p = 0.002) compared with the medium Aβ40 group. Continuous Aβ40 level was associated with the increased risk of cognitive decline (OR: 1.02, 95%CI: 1.00–1.04, p = 0.027).
Discussion
In this large, prospective, population-based cohort study, we found an inverted U-shaped relationship between plasma Aβ40 and cognitive decline during a 2-year follow-up in individuals with normal cognition. People with medium plasma Aβ40 concentrations (45–58.4 pg/mL) had the highest risk of cognitive decline than persons with plasma Aβ40 < 45 pg/mL and ≥ 58.4 pg/mL.
Previous studies have shown evidence of an association between plasma Aβ levels and the progression of AD, although most studies focused on plasma Aβ42 and plasma Aβ42/40 ratio (Schupf et al.; Graff-Radford et al., 2007; Yaffe, 2011; Gabelle et al., 2013; de Rojas et al., 2018; Hanon et al., 2018; Hilal et al., 2018; Verberk et al., 2018; Chen et al., 2019). Hanon et al. (2018) did not find a significant linear association between Aβ40 and MMSE score in their prospective cohort study, which included 1,040 participants. Several prospective cohort studies found that plasma Aβ42 or Aβ42/40 ratio was associated with an increased risk of developing AD, while no associations of this kind were found for plasma Aβ40 (Graff-Radford et al., 2007; Schupf et al., 2008; Yaffe, 2011; Gabelle et al., 2013; de Rojas et al., 2018; Hilal et al., 2018; Verberk et al., 2018). Chen et al. reported a linear relationship between plasma Aβ42 and MMSE score, regardless of plasma Aβ40 (Chen et al., 2019). This is the first study to report a non-linear relationship between plasma Aβ40 and cognitive decline. The reason may be attributed to different study population and different follow-up time. Most of previous studies observed marked cognitive decline (clinical progression to MCI) or the incidence of dementia for a long follow-up time in old and high-risk dementia populations (Yaffe, 2011; Gabelle et al., 2013; Verberk et al., 2018; de Wolf et al., 2020). In our study, we just observed 2-year MMSE change in a cognitively normal population with middle and old age. Two prior studies ever reported that higher plasma Aβ40 level was correlated with a decreased hippocampal volume (Kaffashian et al., 2015; Hanon et al., 2018). There was also evidence to reveal that Aβ levels were linked with brain atrophy only at an initial stage before cognitive impairment. The relationship between plasma Aβ levels and brain structural variation or cognitive outcomes during earlier stage of AD progression or normal cognitive aging may be different with the association at later stages (Hanon et al., 2018). Therefore, the association between plasma Aβ40 and cognitive decline may be earlier detected than the relationship between plasma Aβ42 and cognition progression due to a shorter observation period (2-year follow-up) and younger population in our study. It is why we only find the correlation of cognitive decline with plasma Aβ40, not plasma Aβ42.
The precise mechanisms underlying the relationship between plasma Aβ40 and cognitive decline remain unclear. There may be both of neurodegenerative and cerebrovascular pathologies promoting cognitive impairment in the study participants (Greenberg et al., 2020). Aβ40 was reported to be associated with the development of vascular dementia as well as AD (Hansson et al., 2012). Compared with moderate plasma Aβ40 group, higher plasma Aβ40 level may indicate more Aβ efflux from the brain to the peripheral blood and fewer deposition in brain. Therefore, the cognitive decline in the high plasma Aβ40 group may be slower. Besides, although the concentration of Aβ40 measured in peripheral blood was not high in the moderate Aβ40 group, the deposit of Aβ40 in the wall of vessels may be relatively high, which was involved with the development of vascular dementia. Compared with moderate Aβ40 group, low concentration of plasma Aβ40 may suggest increased degradation of Aβ40 by peripheral tissues, such as liver and kidney and decreased production of Aβ40 from brain and peripheral tissues (Kaffashian et al., 2015). It could reflect favorable condition of blood vessels, liver and kidney, which was significant to maintain normal cognitive function.
The characteristics of Aβ40 is different with Aβ42. Aβ40 is more soluble than Aβ42, which can diffuse along perivascular drainage pathways to deposit in the walls of vessels, while Aβ42 tends to retained in the brain parenchyma and initiate insoluble plaque nucleation (Greenberg et al., 2020). In addition, Aβ40 is produced or degraded by peripheral tissues as well as Aβ40 produced and deleted in the brain (Kaffashian et al., 2015). Plasma Aβ40 may be not a direct surrogate marker for cerebral Aβ40, which can’t reflect Aβ40 and Aβ42 metabolism in the brain (Sonnen et al., 2008). Accordingly, the effect of Aβ40 on the physiologic process of dementia may be more complicated than Aβ42. Because this study is a community-based cohort study, which failed to measure the liver and kidney function comprehensively, nor did it assess the deposition of Aβ in the brain parenchyma and vascular wall. The mechanisms of plasma Aβ40 affecting cognitive performance still needs further studies.
Of note, we found that the inverted U-shaped relationship between plasma Aβ40 and cognitive decline was more marked for young people (40–65 years old), while there seems to be a positive linear relationship between plasma Aβ40 and cognitive decline in individuals older than 65 years. This result agrees with other studies that suggested that amyloid-cognition association varied by age (Rowe et al., 2010; Rentz et al., 2011; Sperling et al., 2013; Petersen et al., 2016; Jansen et al., 2018). Jansen et al. (2018) found that the relationship between higher amyloid deposition in the brain and low memory scores was more evident in individuals older than 70 years old. Coincidentally, another recent study found no association between continuous PET-Aβ levels and memory scores in younger people (50–69 years old) (Mielke et al., 2016). In older population (> 65 years old), our results supported the previous studies (Hansson et al., 2012; Kaffashian et al., 2015; Hanon et al., 2018). For younger individuals (40–65 years old), our studies suggested that conventional evaluation methods (continuous or dichotomized Aβ40) were not suitable for assessing the relationship between plasma Aβ40 and cognitive decline.
There are still limitations that need to be considered. First, only the total MMSE score was evaluated for every participant in this study; sub-items of MMSE and other cognitive scores should be included to confirm cognitive decline. Second, ELISA is not the most sensitive technique for assessing plasma Aβ levels. Recent studies have employed single-molecule array or hybrid mass spectrometry techniques which may be more accurate approaches than ELISA (Nakamura et al., 2018; Vergallo et al., 2019). Nevertheless, ELISA is suitable for epidemiological studies with large sample size because of simple operation and cheapness. Third, the correlation of plasma Aβ40 with CSF Aβ40 and brain Aβ40, and the mechanism of transportation and clearance of Aβ40, were not studied. It would be useful to explore the dynamic transportation mechanism of Aβ40 between the brain and peripheral blood in future studies, in order to verify the present results.
Conclusion
This population-based, prospective cohort study showed that the relationship between plasma Aβ40 and cognitive decline is not linear, but an inverted-U shape, in a cognitively normal population. Medium concentration of plasma Aβ40 is associated with a greater risk of cognitive decline, compared with low and high levels of plasma Aβ40, primarily in younger persons (≤65 years old). The underlying mechanism needs to be further elucidated.
Data Availability Statement
The raw data supporting the conclusions of this article will be made available by the authors, without undue reservation, to any qualified researcher.
Ethics Statement
The studies involving human participants were reviewed and approved by the ethics committee for medical research at the First Affiliated Hospital of Xi’an Jiaotong University. The patients/participants provided their written informed consent to participate in this study.
Author Contributions
FG and QQ: conception and design of the study. SS, CC, LD, LG, SW, JW, and QQ acquisition of the data. FG, SS, and CC: analysis of data. FG, JW, KH, MD, and QQ interpretation of data. FG: wrote the first manuscript draft. SS, CC, LD, LG, SW, JW, KH, MD, JW, and QQ: revised the manuscript for intellectual content. All authors contributed to the article and approved the submitted version.
Funding
This work was supported by the Nature Science Foundation of China (No. 81771168), the Key Research & Development Programs of Shaanxi Province (No. 2018ZDXM-SF-052), and the Clinical Research Award of the First Affiliated Hospital of Xi’an Jiaotong University, China (No. XJTU1AF-CRF-2018-008).
Conflict of Interest
The authors declare that the research was conducted in the absence of any commercial or financial relationships that could be construed as a potential conflict of interest.
Acknowledgments
We are thankful for the cooperation of all participants in our study.
Supplementary Material
The Supplementary Material for this article can be found online at: https://www.frontiersin.org/articles/10.3389/fnagi.2020.557005/full#supplementary-material
References
American Psychiatric Association [APA], (2000). Diagnostic and Statistical Manual of Mental Disorders, 4th Edn. Washington, DC: American Psychiatric Association.
Bohm, M., Schumacher, H., Leong, D., Mancia, G., Unger, T., Schmieder, R., et al. (2015). Systolic blood pressure variation and mean heart rate is associated with cognitive dysfunction in patients with high cardiovascular risk. Hypertension 65, 651–661. doi: 10.1161/Hypertensionaha.114.04568
Chen, T. B., Lee, Y. J., Lin, S. Y., Chen, J. P., Hu, C. J., Wang, P. N., et al. (2019). Plasma Abeta42 and total tau predict cognitive decline in amnestic mild cognitive impairment. Sci. Rep. 9:13984. doi: 10.1038/s41598-019-50315-9
de Rojas, I., Romero, J., Rodriguez-Gomez, O., Pesini, P., Sanabria, A., Perez-Cordon, A., et al. (2018). Correlations between plasma and PET beta-amyloid levels in individuals with subjective cognitive decline: the fundacio ACE healthy brain initiative (FACEHBI). Alzheimers Res. Ther. 10:119. doi: 10.1186/s13195-018-0444-1
de Wolf, F., Ghanbari, M., Licher, S., McRae-McKee, K., Gras, L., Weverling, G. J., et al. (2020). Plasma tau, neurofilament light chain and amyloid-beta levels and risk of dementia; a population-based cohort study. Brain 143, 1220–1232. doi: 10.1093/brain/awaa054
Donohue, M. C., Sperling, R. A., Petersen, R., Sun, C. K., Weiner, M. W., Aisen, P. S., et al. (2017). Association between elevated brain amyloid and subsequent cognitive decline among cognitively normal persons. JAMA 317, 2305–2316. doi: 10.1001/jama.2017.6669
Dubois, B., Hampel, H., Feldman, H. H., Scheltens, P., Aisen, P., Andrieu, S., et al. (2016). Preclinical Alzheimer’s disease: definition, natural history, and diagnostic criteria. Alzheimers Dement. 12, 292–323. doi: 10.1016/j.jalz.2016.02.002
Gabelle, A., Richard, F., Gutierrez, L. A., Schraen, S., Delva, F., Rouaud, O., et al. (2013). Plasma amyloid-beta levels and prognosis in incident dementia cases of the 3-City Study. J. Alzheimers Dis. 33, 381–391. doi: 10.3233/JAD-2012-121147
Graff-Radford, N. R., Crook, J. E., Lucas, J., Boeve, B. F., Knopman, D. S., Ivnik, R. J., et al. (2007). Association of low plasma Abeta42/Abeta40 ratios with increased imminent risk for mild cognitive impairment and Alzheimer disease. Arch. Neurol. 64, 354–362. doi: 10.1001/archneur.64.3.354
Greenberg, S. M., Bacskai, B. J., Hernandez-Guillamon, M., Pruzin, J., Sperling, R., and van Veluw, S. J. (2020). Cerebral amyloid angiopathy and Alzheimer disease - one peptide, two pathways. Nat. Rev. Neurol. 16, 30–42. doi: 10.1038/s41582-019-0281-2
Hanon, O., Vidal, J. S., Lehmann, S., Bombois, S., Allinquant, B., Treluyer, J. M., et al. (2018). Plasma amyloid levels within the Alzheimer’s process and correlations with central biomarkers. Alzheimers Dement. 14, 858–868. doi: 10.1016/j.jalz.2018.01.004
Hansson, O., Stomrud, E., Vanmechelen, E., Ostling, S., Gustafson, D. R., Zetterberg, H., et al. (2012). Evaluation of plasma a beta as predictor of Alzheimer’s disease in older individuals without dementia: a population-based study. J. Alzheimers Dis. 28, 231–238. doi: 10.3233/Jad-2011-111418
Harrell, F. E. (2001). Regression Modeling Strategies: With Applications to Linear Models, Logistic Regression, and Survival Analysis. New York, NY: Springer.
Hilal, S., Wolters, F. J., Verbeek, M. M., Vanderstichele, H., Ikram, M. K., Stoops, E., et al. (2018). Plasma amyloid-beta levels, cerebral atrophy and risk of dementia: a population-based study. Alzheimers Res. Ther. 10:63. doi: 10.1186/s13195-018-0395-6
Jack, C. R. Jr., Bennett, D. A., Blennow, K., Carrillo, M. C., Dunn, B., Haeberlein, S. B., et al. (2018). NIA-AA research framework: toward a biological definition of Alzheimer’s disease. Alzheimers Dement. 14, 535–562. doi: 10.1016/j.jalz.2018.02.018
Jansen, W. J., Ossenkoppele, R., Tijms, B. M., Fagan, A. M., Hansson, O., Klunk, W. E., et al. (2018). Association of cerebral amyloid-beta aggregation with cognitive functioning in persons without dementia. JAMA Psychiatry 75, 84–95. doi: 10.1001/jamapsychiatry.2017.3391
Jaunmuktane, Z., Mead, S., Ellis, M., Wadsworth, J. D., Nicoll, A. J., Kenny, J., et al. (2015). Evidence for human transmission of amyloid-beta pathology and cerebral amyloid angiopathy. Nature 525, 247–250. doi: 10.1038/nature15369
Jiang, Y., Shang, S. H., Li, P., Chen, C., Dang, L., Wang, J. J., et al. (2018). Pulse pressure is associated with plasma amyloid-beta transport dysfunction. J. Hypertens. 36, 569–579. doi: 10.1097/Hjh.0000000000001565
Kaffashian, S., Tzourio, C., Soumare, A., Dufouil, C., Mazoyer, B., Schraen-Maschke, S., et al. (2015). Association of plasma beta-amyloid with MRI markers of structural brain aging the 3-city dijon study. Neurobiol. Aging 36, 2663–2670. doi: 10.1016/j.neurobiolaging.2015.03.016
Katzman, R., Zhang, M. Y., Ouangyaqu, Y. Q., Wang, Z. Y., Liu, W. T., Yu, E., et al. (1988). A Chinese version of the mini-mental state examination - impact of illiteracy in a shanghai dementia survey. J. Clin. Epidemiol. 41, 971–978. doi: 10.1016/0895-4356(88)90034-0
McKhann, G. M., Knopman, D. S., Chertkow, H., Hyman, B. T., Jack, C. R., Kawas, C. H., et al. (2011). The diagnosis of dementia due to Alzheimer’s disease: recommendations from the national institute on aging-Alzheimer’s association workgroups on diagnostic guidelines for Alzheimer’s disease. Alzheimers Dement. 7, 263–269. doi: 10.1016/j.jalz.2011.03.005
Mielke, M. M., Machulda, M. M., Hagen, C. E., Christianson, T. J., Roberts, R. O., Knopman, D. S., et al. (2016). Influence of amyloid and APOE on cognitive performance in a late middle-aged cohort. Alzheimers Dement. 12, 281–291. doi: 10.1016/j.jalz.2015.09.010
Nakamura, A., Kaneko, N., Villemagne, V. L., Kato, T., Doecke, J., Dore, V., et al. (2018). High performance plasma amyloid-beta biomarkers for Alzheimer’s disease. Nature 554, 249–254. doi: 10.1038/nature25456
Olsson, B., Lautner, R., Andreasson, U., Ohrfelt, A., Portelius, E., Bjerke, M., et al. (2016). CSF and blood biomarkers for the diagnosis of Alzheimer’s disease: a systematic review and meta-analysis. Lancet Neurol. 15, 673–684. doi: 10.1016/s1474-4422(16)00070-3
Petersen, R. C. (2011). Clinical practice. Mild cognitive impairment. N. Engl. J. Med. 364, 2227–2234. doi: 10.1056/NEJMcp0910237
Petersen, R. C., Wiste, H. J., Weigand, S. D., Rocca, W. A., Roberts, R. O., Mielke, M. M., et al. (2016). Association of elevated amyloid levels with cognition and biomarkers in cognitively normal people from the community. JAMA Neurol. 73, 85–92. doi: 10.1001/jamaneurol.2015.3098
Rentz, D. M., Amariglio, R. E., Becker, J. A., Frey, M., Olson, L. E., Frishe, K., et al. (2011). Face-name associative memory performance is related to amyloid burden in normal elderly. Neuropsychologia 49, 2776–2783. doi: 10.1016/j.neuropsychologia.2011.06.006
Roberts, R. O., Aakre, J. A., Kremers, W. K., Vassilaki, M., Knopman, D. S., Mielke, M. M., et al. (2018). Prevalence and outcomes of amyloid positivity among persons without dementia in a longitudinal, population-based setting. JAMA Neurol. 75, 970–979. doi: 10.1001/jamaneurol.2018.0629
Rowe, C. C., Ellis, K. A., Rimajova, M., Bourgeat, P., Pike, K. E., Jones, G., et al. (2010). Amyloid imaging results from the Australian imaging, biomarkers and lifestyle (AIBL) study of aging. Neurobiol. Aging 31, 1275–1283. doi: 10.1016/j.neurobiolaging.2010.04.007
Schupf, N., Tang, M. F., Fukuyama, H., Manly, J., Andrews, H., Mehta, P., et al. (2008). Peripheral Abeta subspecies as risk biomarkers of Alzheimer’s disease. Proc. Natl. Acad. Sci. U.S.A. 16, 14052–14057. doi: 10.1073/pnas.0805902105
Sonnen, J. A., Montine, K. S., Quinn, J. F., Kaye, J. A., Breitner, J. C. S., and Montine, T. J. (2008). Biomarkers for cognitive impairment and dementia in elderly people. Lancet Neurol. 7, 704–714. doi: 10.1016/S1474-4422(08)70162-5
Sperling, R. A., Aisen, P. S., Beckett, L. A., Bennett, D. A., Craft, S., Fagan, A. M., et al. (2011). Toward defining the preclinical stages of Alzheimer’s disease: recommendations from the National Institute on Aging-Alzheimer’s Association workgroups on diagnostic guidelines for Alzheimer’s disease. Alzheimers Dement. 7, 280–292. doi: 10.1016/j.jalz.2011.03.003
Sperling, R. A., Johnson, K. A., Doraiswamy, P. M., Reiman, E. M., Fleisher, A. S., Sabbagh, M. N., et al. (2013). Amyloid deposition detected with florbetapir F 18 (F-18-AV-45) is related to lower episodic memory performance in clinically normal older individuals. Neurobiol. Aging 34, 822–831. doi: 10.1016/j.neurobiolaging.2012.06.014
Toledo, J. B., Zetterberg, H., van Harten, A. C., Glodzik, L., Martinez-Lage, P., Bocchio-Chiavetto, L., et al. (2015). Alzheimer’s disease cerebrospinal fluid biomarker in cognitively normal subjects. Brain 138, 2701–2715. doi: 10.1093/brain/awv199
van Harten, A. C., Smits, L. L., Teunissen, C. E., Visser, P. J., Koene, T., Blankenstein, M. A., et al. (2013). Preclinical AD predicts decline in memory and executive functions in subjective complaints. Neurology 81, 1409–1416. doi: 10.1212/WNL.0b013e3182a8418b
Verberk, I. M. W., Slot, R. E., Verfaillie, S. C. J., Heijst, H., Prins, N. D., van Berckel, B. N. M., et al. (2018). Plasma amyloid as prescreener for the earliest Alzheimer pathological changes. Ann. Neurol. 84, 648–658. doi: 10.1002/ana.25334
Vergallo, A., Mégret, L., Lista, S., Cavedo, E., Zetterberg, H., and Blennow, K. (2019). Plasma amyloid β 40/42 ratio predicts cerebral amyloidosis in cognitively normal individuals at risk for Alzheimer’s disease. Alzheimers Dement. 15, 764–775. doi: 10.1016/j.jalz.2019.03.009
Villemagne, V. L., Pike, K. E., Chetelat, G., Ellis, K. A., Mulligan, R. S., Bourgeat, P., et al. (2011). Longitudinal assessment of Abeta and cognition in aging and Alzheimer disease. Ann. Neurol. 69, 181–192. doi: 10.1002/ana.22248
Vos, S. J. B., Xiong, C. J., Visser, P. J., Jasielec, M. S., Hassenstab, J., Grant, E. A., et al. (2013). Preclinical Alzheimer’s disease and its outcome: a longitudinal cohort study. Lancet Neurol. 12, 957–965. doi: 10.1016/S1474-4422(13)70194-7
Wang, J., Qiao, F., Shang, S., Li, P., Chen, C., Dang, L., et al. (2018). Elevation of Plasma amyloid-beta level is more significant in early stage of cognitive impairment: a population-based cross-sectional study. J. Alzheimers Dis. 64, 61–69. doi: 10.3233/JAD-180140
Wei, M., Zhao, B. Y., Huo, K., Deng, Y. N., Shang, S. H., Liu, J., et al. (2017). Sleep Deprivation induced plasma amyloid-beta transport disturbance in healthy young adults. J. Alzheimers Dis. 57, 899–906. doi: 10.3233/Jad-161213
Wenham, P. R., Price, W. H., and Blundell, G. (1991). Apolipoprotein-E genotyping by one-stage pcr. Lancet 337, 1158–1159. doi: 10.1016/0140-6736(91)92823-K
Keywords: Alzheimer’s disease, plasma amyloid-β, cognitive decline, cognitively normal population, age
Citation: Gao F, Shang S, Chen C, Dang L, Gao L, Wei S, Wang J, Huo K, Deng M, Wang J and Qu Q (2020) Non-linear Relationship Between Plasma Amyloid-β 40 Level and Cognitive Decline in a Cognitively Normal Population. Front. Aging Neurosci. 12:557005. doi: 10.3389/fnagi.2020.557005
Received: 29 April 2020; Accepted: 19 August 2020;
Published: 11 September 2020.
Edited by:
Jiehui Jiang, Shanghai University, ChinaReviewed by:
Nobuyuki Kimura, National Center for Geriatrics and Gerontology (NCGG), JapanKewei Chen, Banner Alzheimer’s Institute, United States
Copyright © 2020 Gao, Shang, Chen, Dang, Gao, Wei, Wang, Huo, Deng, Wang and Qu. This is an open-access article distributed under the terms of the Creative Commons Attribution License (CC BY). The use, distribution or reproduction in other forums is permitted, provided the original author(s) and the copyright owner(s) are credited and that the original publication in this journal is cited, in accordance with accepted academic practice. No use, distribution or reproduction is permitted which does not comply with these terms.
*Correspondence: Qiumin Qu, cXVxaXVtaW5AMTI2LmNvbQ==