- 1Department of Neurology, Graduate School of Medicine, Chiba University, Chiba, Japan
- 2Department of Neurology, Japanese Red Cross Narita Hospital, Chiba, Japan
- 3Department of Neurology, Chiba Rosai Hospital, Chiba, Japan
- 4Department of Neurology, JR Tokyo General Hospital, Tokyo, Japan
- 5Diagnostic Radiology and Radiation Oncology, Graduate School of Medicine, Chiba University, Chiba, Japan
- 6Department of Radiology, Chiba University Hospital, Chiba, Japan
- 7Integrative Brain Imaging Center, National Center of Neurology and Psychiatry, Tokyo, Japan
Both cognitive function and striatal dopamine function decline by normal aging. However, the relationship among these three factors remains unclear. The aim of this study was to elucidate the association among age-related changes in the striatal dopamine transporter (DAT) and cognitive function in healthy subjects. The 30 healthy volunteers were enrolled in this research, the age ranged from 41 to 82 (64.5 ± 11.5, mean ± SD). All subjects were scanned with both T1-weighted magnetic resonance imaging (MRI) and 123I-FP-CIT single-photon emission computed tomography (SPECT) images. The Wechsler Adult Intelligence Scale-Third Edition (WAIS-III) was used to evaluate cognitive function. Six spherical regions of interest (ROI) using 10 mm in diameter on the caudate nucleus, anterior putamen and posterior putamen were manually drawn on MRI image which was applied onto SPECT image. The relationship between striatal occipital ratio (SOR) values and WAIS-III subscore were analyzed by multiple regression analysis. Subscores which was significant were further analyzed by path analyses. Full intelligence quotient (IQ), verbal IQ, verbal comprehension were all positively correlated with age-adjusted striatal DAT binding (P < 0.01). Multiple regression analyses revealed that the coding digit symbol correlated with all striatal regions except for the left caudate (P < 0.04). Picture completion and right caudate, similarities and left caudate also showed a positive correlation (P < 0.04). Path analysis found that the right caudate and picture completion; the left caudate and similarities were correlated independently from age, whereas the models of coding digit symbol were not significant. These results suggest that age-based individual diversity of striatal DAT binding was associated with verbal function, and the caudate nucleus plays an important role in this association.
Introduction
In human cognitive performance peaks around age 20, and subsequently, as people age, a wide range of cognitive tasks exhibit a steady and accelerated decline (Salthouse, 2010). Cognitive functions that decline with normal aging include the speed of information processing (Verhaeghen and Salthouse, 1997), memory (Engle, 2002; Rieckmann et al., 2018), attention, and executive control (Miyake et al., 2000). Conversely, some cognitive functions, such as vocabulary and general information, remain stable throughout normal aging, at least until the seventh decade of life (Salthouse, 2010).
Dopamine plays an important role in complex cognitive functions such as working memory, cognitive flexibility, language and thought, motor planning, abstract representation, temporal analysis/sequencing, and generativity (Volkow et al., 1998; Previc, 1999; Mozley et al., 2001). The dopamine transporter (DAT) is a membrane-bound protein located on the presynaptic terminals that regulates intrasynaptic dopaminergic levels by re-uptaking dopamine; its radioligand serves as a biomarker for the presynaptic dopaminergic system (i.e., nigrostriatal and mesolimbic pathway). DAT imaging has been widely applied in both clinical and research settings. [123I]-2beta-carbometoxy-3beta-(4-iodophenyl)-N-(3-fluoropropyl) nortropane ([123I]FP-CIT; ioflupane) is a probe for single-photon emission computed tomography (SPECT), which enables us to quantify striatal DAT binding in vivo (Tatsch and Poepperl, 2013). Previous studies have shown that the presynaptic striatal dopamine function declines with normal aging (Kaasinen et al., 2015; Matsuda et al., 2018). Neurodegeneration in the nigrostriatal dopaminergic neurons occurs in Parkinson’s disease (PD), which presents as bradykinesia, rigidity, and resting tremor, accompanied by some nonmotor symptoms such as depression, autonomic dysfunction, and cognitive impairment. Dopamine has been postulated as one of the many pathological hypotheses for cognitive impairment in PD (Bosboom et al., 2004; Hirano et al., 2012; Biundo et al., 2016).
The Wechsler Adult Intelligence Scale (WAIS)-Third Edition consists of 14 subscore section tests including picture completion, vocabulary, coding digit symbol, similarities, block design, arithmetic, matrix reasoning, digit span, information, picture arrangement, comprehension, symbol search, letter-number sequencing, and object assembly (Kaufman and Lichtenberger, 2006). By calculating the subscores, one can estimate verbal comprehension (vocabulary, similarities, information, comprehension), working memory (arithmetic, digit span, letter-number sequencing), perceptual organization (picture completion, block design, matrix reasoning), processing speed (coding digit symbol, symbol search), and intelligence quotient (IQ).
The evidence of a correlational triad among normal aging, cognitive function, and striatal dopamine presynaptic function is still limited, and these relationships remain unclear. Mozley et al. scanned DAT imaging in 66 healthy subjects who were evaluated by customized cognitive tests and found that young women exhibited higher caudate DAT binding and higher language learning performance (Mozley et al., 2001). Twelve healthy subjects underwent DAT positron emission tomography and several cognitive batteries; the results showed that episodic memory and executive function were associated with striatal DAT binding and that both cognitive batteries declined with aging (Erixon-Lindroth et al., 2005). This report also demonstrated that the information subscore of the WAIS-revised was associated with inter-individual variance of striatal DAT binding. These findings should be extended by using standard cognitive tests and normalizing the data by controlling for age.
The aim of this study was to clarify the relationship between the striatal DAT function, evaluated by [123I]FP-CIT SPECT, and cognitive function measured by a standardized neuropsychological test, the WAIS-III, in healthy subjects. First, these relationships were investigated by age-normalized cognitive scores and age-adjusted striatal DAT binding. Next, the age-unadjusted striatal subregions were further explored with age-unadjusted cognitive values, followed by the evaluation of associations among age, DAT binding, and cognition, which were assessed using path analyses.
Materials and Methods
Participants
This study was approved by the ethical committee of Chiba University (#2074). All participants received explanations of the study orally and by documents and provided written informed consent. Thirty healthy Japanese subjects (21 females and 9 males) were enrolled in this study from April to December 2016; they were recruited using local advertisements. Exclusions for the subjects included: neurodevelopmental disorders; neurologic or psychiatric illnesses including Parkinsonism and rapid eye movement sleep behavior disorder and depression, magnetic resonance imaging (MRI) contraindications, and the use of medicine that influences the accumulation of [123I]FP-CIT in the brain (cocaine, mazindol, methylphenidate, and selective serotonin reuptake inhibitors). The participants received financial compensation after the study. The participants ranged in age from 41 to 82 years old [mean ± standard deviation (SD): 64.5 ± 11.5]. The educational duration ranged from 9 to 16 years (mean ± SD: 13.8 ± 2.1). Twenty-five subjects were right-handed, three subjects were left-handed, and two subjects were ambidextrous.
Neuropsychological Assessment
All subjects underwent the mini-mental state examination (MMSE), frontal assessment battery (FAB), and WAIS-III. These neuropsychological tests were performed on the same day as the MRI scan. All tests were administered by an experienced clinical neuropsychologist and took from 90 to 120 min to complete. No participants reported a total MMSE score of less than 24. The WAIS-III includes 14 subtests, of which 13 were administered (the object assembly subtest was omitted since it does not contribute to the full-scale IQ; Cockcroft et al., 2015). Thirteen age-adjusted subscores were further calculated for verbal comprehension, working memory, perceptual organization and processing speed, Performance IQ (PIQ), Verbal IQ (VIQ), and the full score IQ (FIQ), which is calculated from PIQ and VIQ. The 13 age-unadjusted raw subscores and age-adjusted subscores were both used in these analyses.
Image Acquisition
MRI
All MR scans were performed using a 3.0T Discovery MR750 (GE Healthcare, Milwaukee, WI, USA). T1-weighted images were collected for the purpose of placing the region-of-interest (ROI). T1 images were obtained by sagittal 3-D fast spoiled gradient-echo (FSPGR) sequences. The following parameters were used for the T1-weighted images: repetition time, 6.4 ms; echo time, 2.6 ms; inversion time, 420 ms; flip angle, 15°; field-of-view, 240 × 240 mm; matrix, 256 × 256; slices, 178; slice thickness, 1.4 mm; overlap, 0.7 mm; and scan time, 214 s. Transaxial fluid attenuation inversion (FLAIR) imaging was also conducted for screening purposes. No intracranial abnormal structures were identified in any of the participants.
[123I]FP-CIT SPECT
A SPECT scan was conducted within three months after the MRI screening. A dose of 167 MBq [123I]FP-CIT was injected intravenously while the subjects were at rest. Image acquisition was performed 3.5 h after the injection, with Infinia + Hawkeye4 (GE Healthcare, Milwaukee, WI, USA) mounted with extended low energy general-purpose (ELEGP) collimators. Projection data were acquired for 30 min. The data were reconstructed by the ordered subset expectation maximization (OSEM) method (iteration: 5, subset: 10). Images were acquired in a 128 × 128 matrix of 2.95-mm thick axial slices under continuous rotation (8 rotations, 3 min per rotation) in 4-degree steps and were reconstructed with a Butterworth filter (cut-off frequency 0.5 cycle/cm, order 8). Attenuation was corrected using Chang’s technique (coefficient: 0.07).
Image Analysis
Automated Striatal DAT Binding Adjusted by Age
The specific binding ratios (SBRs) of the DAT-SPECT values were calculated using DaT View version 6.1 software (AZE Ltd., Kanagawa, Japan) based on Bolt’s method (Tossici-Bolt et al., 2006; Miyai et al., 2015). In brief, the ROI was automatically placed on the striatum. A reference ROI was automatically selected outside the striatal ROI in the cerebral cortex. Fully automated detection relies on a 50% iso-contour based on the non-striatum maximum uptake. This automated procedure gives a consistent ROI size in both normal and abnormal scans (Tossici-Bolt et al., 2006). The right and left striatal SBR was defined as the ratio of the concentrations of specific (striatum) to non-specific (cerebral cortex) radioligand binding. SBR was corrected by phantom experiment, following adjustment for age using 256 healthy subjects, and was called the age-adjusted specific binding ratio (SBRadj; Tossici-Bolt et al., 2006; Matsuda et al., 2018). The advantages of using the SBRadj method are that the placement of the ROI is automatic without investigator bias and age adjustment is performed using a large normal database. The drawback of this software is that subregions of the striatal ROI cannot be investigated.
Manually Placed ROI in Striatal Subdivision
To investigate DAT activities in the striatal subregions, manual ROI analyses were conducted. AquariusNET version 4.4 (TeraRecon, CA, USA) was utilized to display, fuse images, and place ROIs on 3D-T1-weighted MRI and [123I]FP-CIT SPECT images. The standard MRI position was adjusted to the anterior commissure-posterior commissure line. The SPECT images were automatically coregistered with MRI in the same space. Two continuous transverse slices including the striatum were selected for further analyses.
Circular ROIs with a diameter of 10 mm were manually placed on both sides of the caudate nucleus (CN), anterior putamen (AP), and posterior putamen (PP) on transverse T1-weighted MR images, as illustrated in Figure 1 (Scherfler et al., 2005). Using the same MR image slice, the eight circular ROIs (each 10 mm in diameter) were drawn on the occipital cortex as a reference region (Figure 1). This was performed in each of the two continuous transverse slices. Higher [123I]FP-CIT values in the same striatal region were further selected as a specific activity. The striatal DAT binding was calculated using the striatum-occipital ratio (SOR), as shown below (Árgyelán et al., 2005).
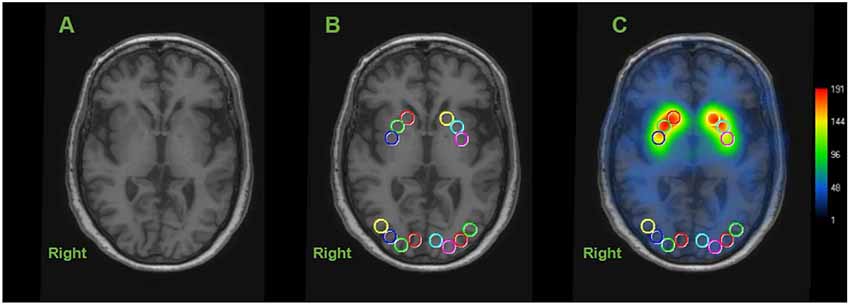
Figure 1. Representative image of regions of interest (ROIs) in dopamine transporter (DAT) image. Circular ROIs were manually placed 10 mm in diameter, in each hemisphere on the structural MRI for the caudate nucleus (CN), anterior putamen (AP) and posterior putamen (PP) and 8 on the occipital cortex as the reference region. Calculating each specific activity with DAT binding is estimated by the striatal-to-occipital ratio, defined as (striatum—occipital)/occipital counts. (A) Axial section of structural MRI (3-dimensional T1 weight image). (B) ROIs placed on an axial slice of MRI. (C) [123I]FP-CIT SPECT image overlaid onto the coregistered MRI.
The advantage of using this method is that six subregions of the striatum can be evaluated, although the data are not adjusted for age.
Statistical Analysis
The normality of the variables was evaluated using Kolmogorov–Smirnov tests. Analysis 1: The relationship between SBRadj and IQ was analyzed using Pearson’s correlation analysis. To clarify which WAIS-III subscores were correlated with striatal DAT binding, multiple regression analyses (stepwise linear regression analyses) were performed using SBRadj values as the independent variable and the age-adjusted WAIS-III subscores as the dependent variables. Analysis 2: The relationship between age-unadjusted striatal DAT binding (SOR values) and raw WAIS-III subscores (i.e., age unadjusted) were analyzed by stepwise linear regression analyses. Analysis 3: To clarify the direct and indirect relationships between age, striatal DAT binding (SOR), and raw WAIS-III subscores, the significant results from analysis 2 were further investigated by path analyses that included age as a mediator. Path analysis is a form of multiple regression statistical analysis that is used to evaluate causal models by examining the relationships between a dependent variable. By using this method, one can estimate both the magnitude and significance of causal connections among variables. The direct path was defined as the relationship between striatal DAT binding (SOR) and WAIS-III subscore (c’), whereas the indirect path included the relationships between striatal DAT binding and age (a) and between age and WAIS-III subscores (b).
All statistical analyses were performed using IBM SPSS software version 22 (IBM Corporation, Armonk, NY, USA). A p-value of less than 0.05, adjusted for multiple comparisons by the false discovery rate (FDR), was considered significant. All multiple regression analyses conducted in this study showed a variance inflation factor (VIF) score of less than 1.05, excluding the possibility of collinearity between the variables.
Results
The right and left average (± SD) striatal subregion SOR values in the 30 healthy subjects were as follows: CN: 3.50 ± 0.60; AP: 3.37 ± 0.52; and PP: 2.60 ± 0.45, thus displaying an anterior-to-posterior gradient. The average SBRadj was positively correlated with the SOR of all six striatal subregions (R > 0.74, P < 0.001, Pearson’s correlation). All six striatal subdivisions (age-unadjusted SOR values) were negatively correlated with age (R < −0.41, P < 0.03).
Table 1 shows the summarized results of the WAIS-III subscores, MMSE, and FAB total scores. The following neuropsychological tests were correlated with age: coding digit symbol; block design; matrix reasoning; picture arrangement; symbol search; and FAB total score (P < 0.05, Pearson’s correlation adjusted by FDR; Table 1). The WAIS-III subscores for vocabulary, coding digit symbol, similarities, information, and comprehension were positively correlated with education (R > 0.45, P < 0.02).
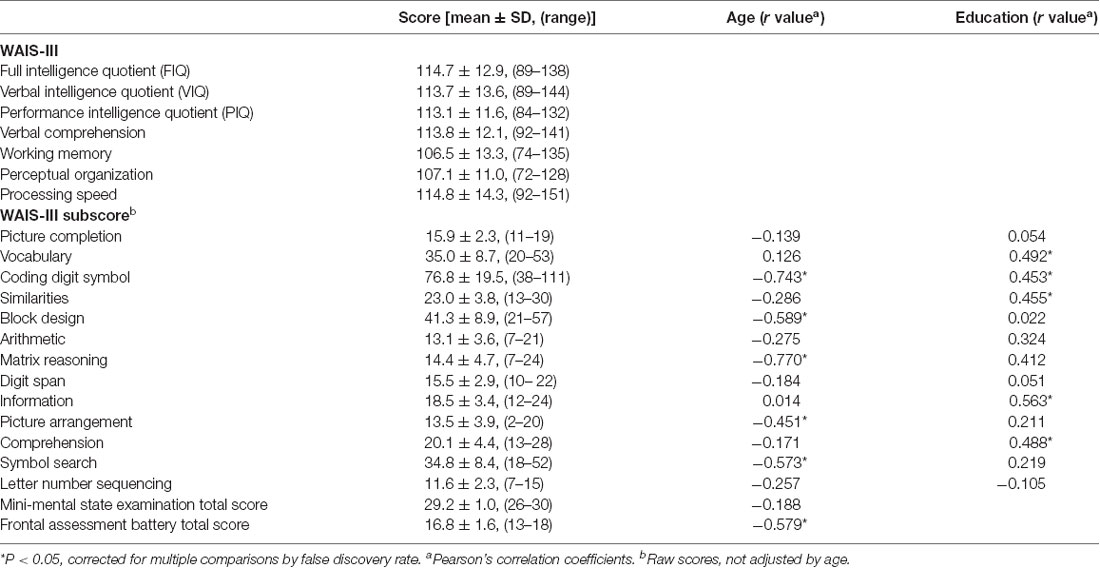
Table 1. Summary of the neuropsychological tests and correlation with age and education in 30 normal subjects.
Analysis 1: Age-Adjusted DAT Binding (SBRadj) and IQs
The FIQ, VIQ, and verbal comprehension scores were positively correlated with both right and left and average SBRadj (right: R > 0.48, P < 0.01; left: R > 0.51, P < 0.005; average: R > 0.50, P < 0.05, Pearson’s correlations; Figure 2). PIQ, working memory, perceptual organization, and processing speed were not correlated with any SBRadj values. The multiple regression analyses revealed that the right SBRadj was positively correlated with the information subscore (R = 0.528, P = 0.003) and the left SBRadj was positively correlated with the similarities subscore (R = 0.523, P = 0.003).
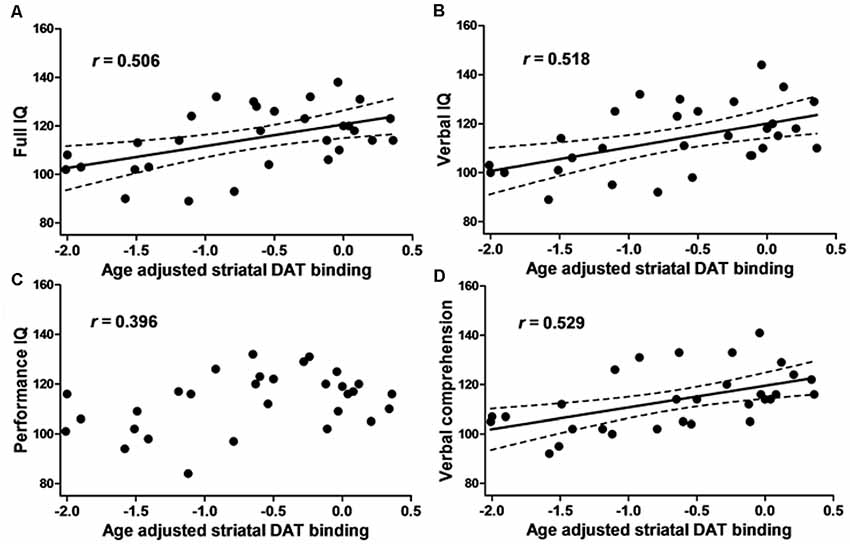
Figure 2. The relationship between the intelligence quotient (IQ) and dopamine transporter (DAT) binding. The scatter plot showing the age-adjusted intellectual score and age-adjusted right and left averaged striatal specific binding ratio (SBR) of [123I]-FP-CIT SPECT in 30 healthy subjects. (A) The full IQ, (B) Verbal IQ (VIQ) and (D) verbal comprehension were positively correlated with the striatal DAT binding. (C) Performance IQ (PIQ), working memory, perceptual organization and processing speed (see text) did not show any correlation. The dotted line represents the 95% confidence interval.
Analysis 2: Age-Unadjusted DAT Binding (SOR Value) and Age-Unadjusted WAIS-III Subscores
The multiple regression analysis identified the WAIS-III subscores associated with each SOR; these results are shown in Table 2. The right CN SOR was positively correlated with picture completion and coding digit symbol (R = 0.6, P < 0.005). The SOR value of the left CN was positively correlated with the similarities subscore (R = 0.437, P < 0.02). The SOR value of the bilateral AP and left PP were all positively correlated with the coding digit symbol subscore (R > 0.43, P < 0.02).
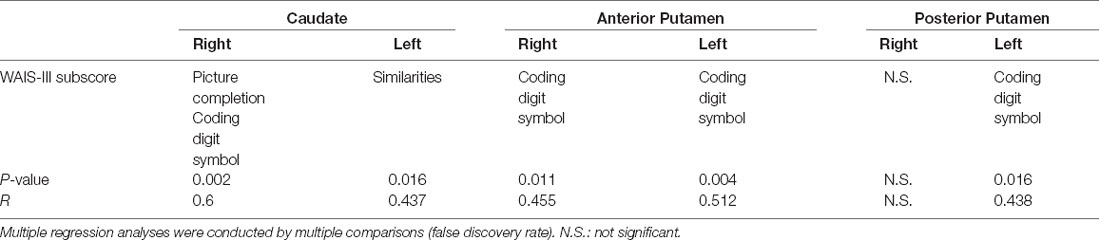
Table 2. The multiple regression analyses of Wechsler Adult Intelligence Scale—Third Edition (WAIS-III) subscores and six subregions of striatal dopamine transporter (DAT) binding.
Analysis 3: Path Analyses
Six models from the results of analysis 2 were assessed, of which two models remained significant. The right CN SOR was a significant mediator of both age (β = −7.80, P = 0.013) and the picture completion subscore (β = 1.66, P = 0.013; Figure 3A). Additionally, the left CN SOR was a significant mediator of age (β = −8.34, P = 0.007) and the similarities subscore (β = 2.36, P = 0.037; Figure 3B). The caudate DAT function is both related to normal aging and cognition (picture completion and similarities), although these two relationships are independent with each other.
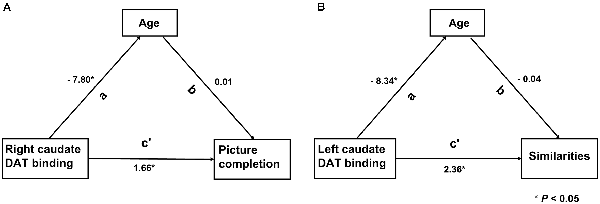
Figure 3. The relationship among the striatal DAT binding, Wechsler Adult Intelligence Scale—third edition (WAIS-III) subscores and age. Two models remarked significantly by path analysis. (A) DAT binding value of right caudate was directly associated with the WAIS-III subscores of picture completion (c’). (B) Left caudate was directly associated with the WAIS-III subscores of similarities (c’). The indirect path was significant (a path) in both models. In this model, the direct and indirect relationships between aging, striatal DAT binding and cognition, the standardized path coefficients (β) are listed as a, b and c’, where a and b are part of the indirect path and c’ is the direct path adjusted for the indirect path.
Discussion
The present study investigated the physiological relationship between presynaptic striatal dopaminergic function and cognition. The following main findings were reported: (1) The FIQ, VIQ, and verbal comprehension were positively and bilaterally correlated with age-adjusted striatal DAT binding; (2) Multiple regression analyses revealed that the right age-adjusted striatal DAT binding was positively correlated with the information subscore, whereas the left age-adjusted striatal DAT binding was positively correlated with the similarities subscore; (3) Multiple regression analyses followed by path analyses revealed that age-unadjusted caudate DAT binding was a significant mediator with normal aging and with the picture completion subscore on the right and the similarities subscore on the left. This step-by-step approach demonstrated that striatal dopaminergic function, particularly involving the left CN, is implicated in language abilities in normal subjects; this relationship was independent from normal aging.
The decline in non-verbal performance with normal aging can be explained by the decreases in processing speed and problem-solving ability (fluid intelligence); conversely, verbal intelligence remained stable across age groups and is responsible for expressing previous verbal learning and general knowledge (crystallized intelligence; Ryan et al., 2000). The present study showed that coding digit symbol, block design, matrix reasoning, and picture arrangement belong to PIQ, which is an indicator of fluid intelligence and declines with age. Conversely, subscores related to verbal comprehension (i.e., similarities, information, vocabulary), known as crystallized knowledge, did not show age-related declines. These observations are in line with previous reports. Language comprehension does not decline with age; by contrast, there is an age-related decline in language production (Shafto and Tyler, 2014). This slower access to the phonological information or selective deficits in accessing phonological representations is known as “tip of tongue” state. This state may explain the dissociation between preserved knowledge and failure of semantic retrieval or bradyphrenia. A functional neuroimaging study demonstrated that retrieval failure accompanied by tip of tongue state was associated with response in the anterior cingulate and right middle frontal regions (Maril et al., 2001). Interestingly, previous findings showed that the WAIS-III information subscore in healthy subjects was not associated with aging but was correlated with striatal DAT binding, similar to our observations (Erixon-Lindroth et al., 2005). Notably, the tasks included in VIQ are not restricted by time, which indicates that the association between striatal DAT binding and VIQ cannot be explained by motor slowness.
In normal human aging, functional imaging studies of striatal DAT binding demonstrate a progressive decline of DAT expression. Previous [123I]FP-CIT imaging studies have reported linear normal aging effects in striatal binding with declines of 3.6%–8.1% per decade (Kazumata et al., 1998; Eusebio et al., 2012; Varrone et al., 2013; Kaasinen et al., 2015; Matsuda et al., 2018). This age-dependent decline in striatal DAT binding has been reported in humans and in primates and in rodent brain histochemical studies (Allard and Marcusson, 1989; De Keyser et al., 1990; Emborg et al., 1998; Hebert et al., 1999). A multicenter trial was conducted to generate an [123I]FP-CIT SPECT database of healthy controls from different SPECT cameras, in which the present subjects were part of the study (Matsuda et al., 2018). A significant effect of age was found, with a DAT binding (SBR) decline rate of 6.3% per decade. In this study, the age-unadjusted bilateral striatal SBR reported a 5.7% (CN: 7.2%, anterior 7.3%, PP: 6.2%) decline per decade, which is comparable to the values of previous reports. We have replicated the previous small-size DAT image research showing that the information subscore was related to inter-individual DAT differences (Erixon-Lindroth et al., 2005). Notably, most of the WAIS-III subscores increased in heterogeneity with aging (Ardila, 2007). This suggests that individual residuals from the age-adjusted mean may be particularly meaningful for investigating cognitive function in the process of aging. The advantage of the present study is that both cognitive function and striatal DAT binding were controlled by age, namely IQ and SBRadj, respectively. We propose that individual verbal ability can be explained by caudate DAT binding expressed as a deviation from the age-adjusted mean. Verbal ability is variable among subjects, and females tend to be better than males, which was first reported in a previous DAT imaging study in healthy subjects (Mozley et al., 2001). Interestingly, young women have higher striatal DAT binding than men, which is in line with our results representing the verbal aspect of striatal dopaminergic function (Matsuda et al., 2018). However, to our knowledge, most studies, including ours, are cross-sectional, and there are no longitudinal studies of DAT imaging in normal subjects. Therefore, it is difficult to reach a definitive conclusion, but we can deduce from these studies that individual striatal DAT slowly declines with normal aging, which is experienced as a decline in language function in each individual. Nonetheless, these observations lead us to the notion that language ability is associated with individual diversity of caudate dopaminergic function.
Verbal working memory is predominantly localized in the left hemisphere, whereas the spatial system localizes in the right hemisphere (Smith and Jonides, 1997). The similarity subscore, included in verbal comprehension, was related to the left-side caudate DAT function.
A meta-analysis of functional neuroimaging in healthy subjects demonstrated that the CN is coactivated with the mesial and inferior frontal gyrus, ipsilateral dorsolateral prefrontal cortex, and thalamus (Postuma and Dagher, 2006). The connectivity between the prefrontal cortex and the head of the CN is in line with the classical striato-pallido-thalamo-cortical circuits hypothesis (Alexander et al., 1990) and with evidence from a primate study (Yeterian and Pandya, 1991). Interestingly, a previous volumetric study performed in young adults showed that caudate volume was positively correlated with FIQ and VIQ but less so with PIQ; this agrees with the present results, which further supports that dopaminergic function is crucial to the association between IQ and the CN (Grazioplene et al., 2015). Likewise, the findings from this study demonstrated the relationship between cognitive function and DAT binding in the CN but not in the putamen. Neuroimaging studies that use whole-brain functional neuroimaging, semantic priming, and the neuronal adaptation technique have shown that people who can communicate in multiple languages activate the CN regardless of the type of language, which indicates that the CN is a center for language control (Crinion et al., 2006). During motor sequence acquisition in healthy subjects, perfusion images have shown activation of the bilateral caudate nuclei, the left PP, the right dentate nucleus, the ventral prefrontal cortex, the left inferior parietal lobule, and the inferior and superior temporal gyri (Carbon et al., 2003) This learning network was related to caudate DAT binding in healthy subjects (Carbon et al., 2004). The CN may play a role in prediction-error learning, which may contribute to verbal ability.
An autopsy study has found that the cellular loss of the substantia nigra subregion was prominent in the caudal subregion in normal aging (Fearnley and Lees, 1991). PD is characterized by the progressive loss of striatonigral dopaminergic neurons, which sheds light on the role of dopaminergic function in cognition. A postmortem study of patients with PD who did not show overt cognitive impairment demonstrated that the dopaminergic nerve terminals are most affected in the posterior portion of the putamen (2% of normal controls), in contrast with the relatively preserved head of the CN (19% of healthy controls; Kish et al., 1988). The ventral tier of the substantia nigra was more affected in the PD group, and this subregion projects to the dorsal putamen, which is responsible for motor function. In the same line, in non-demented PD, dopamine is predominantly depleted in the putamen with relative sparing of the CN (Kish et al., 1988). PD subjects with dementia demonstrated diminished dopaminergic function in widespread striatum, notably in the CN, compared with those without dementia (Ito et al., 2002). Accordingly, a PD-related cognitive covariance pattern demonstrated an association with dopaminergic function in the CN, which was not evident with putaminal DAT binding (Niethammer et al., 2013). Previous neuroimaging studies have repeatedly shown the pivotal role of caudate dopaminergic function and cognition in PD (Jokinen et al., 2009; Polito et al., 2012). Dopaminergic function in the CN of PD appears to be associated with verbal memory and verbal fluency and attention-demanding executive function (Marié et al., 1999; Rinne et al., 2000; Jokinen et al., 2009; Polito et al., 2012; Siepel et al., 2016). Interestingly, dopaminergic dysfunction in PD was particularly related to prefrontal cortices when investigated with glucose metabolism images (Carbon et al., 2004; Polito et al., 2012). The role of dopamine in the cognitive impairment of PD has been postulated to affect executive, memory, and visuospatial function (Niethammer et al., 2013) and language function (Mentis et al., 2002; Huang et al., 2007). Although dysfunction in the striato-frontal dopaminergic circuit is not the only pathophysiology responsible for cognitive symptoms in PD, it has a significant impact on executive and language function. These cognitive symptoms in PD subjects may potentially be modulated by dopaminergic medications.
The present study has several limitations. First, few subjects were assessed. The small number of subjects may hamper the possibility of identifying significant correlations between striatal DAT binding and cognitive function. For example, the WAIS-III information subscore was positively correlated with SBRadj (P < 0.005) but not to achieve the subregion of striatal SOR (P > 0.3). In the future, increasing the sample size and diversity of cognitive tests may improve the correlation between striatal DAT binding and cognitive function. Second, not all the subjects were right-handed. However, 73% of left-handed people have left-hemisphere dominancy, so this will not strongly affect the results (Knecht et al., 2000). Third, this study had a cross-sectional design, which is insufficient to reach conclusions with regard to aging. Some of the differences noted between younger and older adults may be due to generational differences, such as differences in access to education, differences in life experiences, differences in childhood nutrition, et cetera. Fourth, gender influenced the distribution of dopamine in PD and normal subjects in a previous study (Kaasinen et al., 2015). In this study, although the male-to-female ratio was shifted (70% women), there was no gender difference in either striatal DAT binding or cognitive function (data not shown). Fifth, we assumed that the association between cognition and DAT binding was linear; however, it could be non-linear, such as an inverted U-shape, which is known to be present in the dopaminergic function, and this issue should be addressed in future studies (Cools et al., 2001). Sixth, we cannot exclude the possibility that the age-related decline of striatal dopamine was influenced by volume los by normal aging. Seventh, [123I]FP-CIT binds both to DAT and a little portion to serotonin transporter (Ziebell et al., 2010). Therefore, the current result may have subtle influence from the serotonin.
In conclusion, verbal function is influenced by striatal dopaminergic function, particularly by the caudate nucleus, whereas declines in non-verbal cognitive function can be explained by normal aging. This result sheds light on the association between individual deviations of striatal dopamine and language abilities in humans.
Data Availability Statement
The raw data supporting the conclusions of this article will be made available by the authors, without undue reservation, to any qualified researcher.
Ethics Statement
The studies involving human participants were reviewed and approved by the ethical committee of Chiba University (#2074). The patients/participants provided their written informed consent to participate in this study.
Author Contributions
HM and SK organized the research project. SH, SF, and YN are responsible for the subjects’ recruitment and execution. SH and HL executed the research project and statistical analysis. HL wrote the first draft of the manuscript. KK, AI, and HT were responsible for the subject’s characterization and sample collection. TU, TH, and TI were responsible for scanning the subjects and supported the image technology. All authors contributed to and have approved the final manuscript.
Funding
The current study was funded by the Nihon-medi Physics, Co. Ltd., Japan. The funder was not involved in the study design, collection, analysis, interpretation of data, the writing of this article or the decision to submit it for publication.
Conflict of Interest
The authors declare that the research was conducted in the absence of any commercial or financial relationships that could be construed as a potential conflict of interest.
Acknowledgments
We would like to thank Mr. Keisuke Shimizu, Medical Center for Dementia, Chiba University Hospital, Chiba, Japan, for performing the neuropsychological tests.
Abbreviations
DAT, dopamine transporter; FAB, frontal assessment battery; IQ, intelligence quotient; MMSE, mini-mental state examination; ROI, region of interest; SBR, specific binding ratio; SPECT, single-photon emission computed tomography; SOR, striatum-occipital ratio; WAIS-III, Wechsler Adult Intelligence Scale-Third Edition.
References
Alexander, G. E., Crutcher, M. D., and DeLong, M. R. (1990). Basal ganglia-thalamocortical circuits: parallel substrates for motor, oculomotor, “prefrontal” and “limbic” functions. Prog. Brain Res. 85, 119–146. doi: 10.1016/s0079-6123(08)62678-3
Allard, P., and Marcusson, J. O. (1989). Age-correlated loss of dopamine uptake sites labeled with [3H]GBR-12935 in human putamen. Neurobiol. Aging 10, 661–664. doi: 10.1016/0197-4580(89)90001-8
Ardila, A. (2007). Normal aging increases cognitive heterogeneity: analysis of dispersion in WAIS-III scores across age. Arch. Clin. Neuropsychol. 22, 1003–1011. doi: 10.1016/j.acn.2007.08.004
Árgyelán, M., Szabó, Z., Kanyó, B., Tanács, A., Kovács, Z., Janka, Z., et al. (2005). Dopamine transporter availability in medication free and in bupropion treated depression: a 99mTc-TRODAT-1 SPECT study. J. Affect. Disord. 89, 115–123. doi: 10.1016/j.jad.2005.08.016
Biundo, R., Weis, L., and Antonini, A. (2016). Cognitive decline in Parkinson’s disease: the complex picture. NPJ Parkinsons Dis. 2:16018. doi: 10.1038/npjparkd.2016.18
Bosboom, J. L. W., Stoffers, D., and Wolters, E. C. (2004). Cognitive dysfunction and dementia in Parkinson’s disease. J. Neural Transm. 111, 1303–1315. doi: 10.1007/s00702-004-0168-1
Carbon, M., Ghilardi, M. F., Feigin, A., Fukuda, M., Silvestri, G., Mentis, M. J., et al. (2003). Learning networks in health and Parkinson’s disease: reproducibility and treatment effects. Hum. Brain Mapp. 19, 197–211. doi: 10.1002/hbm.10115
Carbon, M., Ma, Y. L., Barnes, A., Dhawan, V., Chaly, T., Ghilardi, M. F., et al. (2004). Caudate nucleus: influence of dopaminergic input on sequence learning and brain activation in Parkinsonism. NeuroImage 21, 1497–1507. doi: 10.1016/j.neuroimage.2003.12.014
Cockcroft, K., Alloway, T., Copello, E., and Milligan, R. (2015). A cross-cultural comparison between South African and British students on the Wechsler Adult Intelligence Scales Third Edition (WAIS-III). Front. Psychol. 6:297. doi: 10.3389/fpsyg.2015.00297
Cools, R., Barker, R. A., Sahakian, B. J., and Robbins, T. W. (2001). Enhanced or impaired cognitive function in Parkinson’s disease as a function of dopaminergic medication and task demands. Cereb. Cortex 11, 1136–1143. doi: 10.1093/cercor/11.12.1136
Crinion, J., Turner, R., Grogan, A., Hanakawa, T., Noppeney, U., Devlin, J. T., et al. (2006). Language control in the bilingual brain. Science 312, 1537–1540. doi: 10.1126/science.1127761
De Keyser, J., Ebinger, G., and Vauquelin, G. (1990). Age-related changes in the human nigrostriatal dopaminergic system. Ann. Neurol. 27, 157–161. doi: 10.1002/ana.410270210
Emborg, M. E., Ma, S. Y., Mufson, E. J., Levey, A. I., Taylor, M. D., Brown, W. D., et al. (1998). Age-related declines in nigral neuronal function correlate with motor impairments in rhesus monkeys. J. Comp. Neurol. 401, 253–265. doi: 10.1002/(sici)1096-9861(19981116)401:2<53::aid-cne7>3.0.co;2-x
Engle, R. W. (2002). Working memory capacity as executive attention. Curr. Dir. Psychol. Sci. 11, 19–23. doi: 10.1111/1467-8721.00160
Erixon-Lindroth, N., Farde, L., Wahlin, T. B. R., Sovago, J., Halldin, C., and Bäckman, L. (2005). The role of the striatal dopamine transporter in cognitive aging. Psychiatry Res. 138, 1–12. doi: 10.1016/j.pscychresns.2004.09.005
Eusebio, A., Azulay, J. P., Ceccaldi, M., Girard, N., Mundler, O., and Guedj, E. (2012). Voxel-based analysis of whole-brain effects of age and gender on dopamine transporter SPECT imaging in healthy subjects. Eur. J. Nucl. Med. Mol. Imaging 39, 1778–1783. doi: 10.1007/s00259-012-2207-8
Fearnley, J. M., and Lees, A. J. (1991). Ageing and Parkinson’s disease: substantia nigra regional selectivity. Brain 114, 2283–2301. doi: 10.1093/brain/114.5.2283
Grazioplene, R. G., Ryman, S., Gray, J. R., Rustichini, A., Jung, R. E., and DeYoung, C. G. (2015). Subcortical intelligence: caudate volume predicts IQ in healthy adults. Hum. Brain Mapp. 36, 1407–1416. doi: 10.1002/hbm.22710
Hebert, M. A., Larson, G. A., Zahniser, N. R., and Gerhardt, G. A. (1999). Age-related reductions in [3H]WIN 35,428 binding to the dopamine transporter in nigrostriatal and mesolimbic brain regions of the fischer 344 rat. J. Pharmacol. Exp. Ther. 288, 1334–1339.
Hirano, S., Shinotoh, H., and Eidelberg, D. (2012). Functional brain imaging of cognitive dysfunction in Parkinson’s disease. J. Neurol. Neurosurg. Psychiatry 83, 963–969. doi: 10.1136/jnnp-2011-301818
Huang, C. R., Mattis, P., Tang, C. K., Perrine, K., Carbon, M., and Eidelberg, D. (2007). Metabolic brain networks associated with cognitive function in Parkinson’s disease. NeuroImage 34, 714–723. doi: 10.1016/j.neuroimage.2006.09.003
Ito, K., Nagano-Saito, A., Kato, T., Arahata, Y., Nakamura, A., Kawasumi, Y., et al. (2002). Striatal and extrastriatal dysfunction in Parkinson’s disease with dementia: a 6–[18F]fluoro-L-dopa PET study. Brain 125, 1358–1365. doi: 10.1093/brain/awf134
Jokinen, H., Kalska, H., Ylikoski, R., Madureira, S., Verdelho, A., van der Flier, W. M., et al. (2009). Longitudinal cognitive decline in subcortical ischemic vascular disease—the LADIS study. Cerebrovasc. Dis. 27, 384–391. doi: 10.1159/000207442
Kaasinen, V., Joutsa, J., Noponen, T., Johansson, J., and Seppanen, M. (2015). Effects of aging and gender on striatal and extrastriatal [123I]FP-CIT binding in Parkinson’s disease. Neurobiol. Aging 36, 1757–1763. doi: 10.1016/j.neurobiolaging.2015.01.016
Kaufman, A. S., and Lichtenberger, E. O. (2006). Assessing Adolescent and Adult Intelligence. 3rd Edn. Hoboken, NJ: Wiley.
Kazumata, K., Dhawan, V., Chaly, T., Antonini, A., Margouleff, C., Belakhlef, A., et al. (1998). Dopamine transporter imaging with fluorine-18-FPCIT and PET. J. Nucl. Med. 39, 1521–1530.
Kish, S. J., Shannak, K., and Hornykiewicz, O. (1988). Uneven pattern of dopamine loss in the striatum of patients with idiopathic Parkinson’s disease. Pathophysiologic and clinical implications. N. Engl. J. Med. 318, 876–880. doi: 10.1056/nejm198804073181402
Knecht, S., Dräger, B., Deppe, M., Bobe, L., Lohmann, H., Flöel, A., et al. (2000). Handedness and hemispheric language dominance in healthy humans. Brain 123, 2512–2518. doi: 10.1093/brain/123.12.2512
Marié, R. M., Barré, L., Dupuy, B., Viader, F., Defer, G., and Baron, J. C. (1999). Relationships between striatal dopamine denervation and frontal executive tests in Parkinson’s disease. Neurosci. Lett. 260, 77–80. doi: 10.1016/s0304-3940(98)00928-8
Maril, A., Wagner, A. D., and Schacter, D. L. (2001). On the tip of the tongue: an event-related fMRI study of semantic retrieval failure and cognitive conflict. Neuron 31, 653–660. doi: 10.1016/s0896-6273(01)00396-8
Matsuda, H., Murata, M., Mukai, Y., Sako, K., Ono, H., Toyama, H., et al. (2018). Japanese multicenter database of healthy controls for [123I]FP-CIT SPECT. Eur. J. Nucl. Med. Mol. Imaging 45, 1405–1416. doi: 10.1007/s00259-018-3976-5
Mentis, M. J., McIntosh, A. R., Perrine, K., Dhawan, V., Berlin, B., Feigin, A., et al. (2002). Relationships among the metabolic patterns that correlate with mnemonic, visuospatial, and mood symptoms in Parkinson’s disease. Am. J. Psychiatry 159, 746–754. doi: 10.1176/appi.ajp.159.5.746
Miyai, M., Yamamoto, Y., Uchibe, T., Yada, N., Haramoto, M., Katsube, T., et al. (2015). [Comparison of quantitative value of dopamine transporter scintigraphy calculated from different analytical software]. Nihon Hoshasen Gijutsu Gakkai Zasshi 71, 1209–1214. doi: 10.6009/jjrt.2015_jsrt_71.12.1209
Miyake, A., Friedman, N. P., Emerson, M. J., Witzki, A. H., Howerter, A., and Wager, T. D. (2000). The unity and diversity of executive functions and their contributions to complex “frontal lobe” tasks: a latent variable analysis. Cogn. Psychol. 41, 49–100. doi: 10.1006/cogp.1999.0734
Mozley, L. H., Gur, R. C., Mozley, P. D., and Gur, R. E. (2001). Striatal dopamine transporters and cognitive functioning in healthy men and women. Am. J. Psychiatry 158, 1492–1499. doi: 10.1176/appi.ajp.158.9.1492
Niethammer, M., Tang, C. C., Ma, Y. L., Mattis, P. J., Ko, J. H., Dhawan, V., et al. (2013). Parkinson’s disease cognitive network correlates with caudate dopamine. NeuroImage 78, 204–209. doi: 10.1016/j.neuroimage.2013.03.070
Polito, C., Berti, V., Ramat, S., Vanzi, E., De Cristofaro, M. T., Pellicano, G., et al. (2012). Interaction of caudate dopamine depletion and brain metabolic changes with cognitive dysfunction in early Parkinson’s disease. Neurobiol. Aging 33, 206.e29–206.e39. doi: 10.1016/j.neurobiolaging.2010.09.004
Postuma, R. B., and Dagher, A. (2006). Basal ganglia functional connectivity based on a meta-analysis of 126 positron emission tomography and functional magnetic resonance imaging publications. Cereb. Cortex 16, 1508–1521. doi: 10.1093/cercor/bhj088
Previc, F. H. (1999). Dopamine and the origins of human intelligence. Brain Cogn. 41, 299–350. doi: 10.1006/brcg.1999.1129
Rieckmann, A., Johnson, K. A., Sperling, R. A., Buckner, R. L., and Hedden, T. (2018). Dedifferentiation of caudate functional connectivity and striatal dopamine transporter density predict memory change in normal aging. Proc. Natl. Acad. Sci. U S A 115, 10160–10165. doi: 10.1073/pnas.1804641115
Rinne, J. O., Portin, R., Ruottinen, H., Nurmi, E., Bergman, J., Haaparanta, M., et al. (2000). Cognitive impairment and the brain dopaminergic system in Parkinson disease: [F-18]fluorodopa positron emission tomographic study. Arch. Neurol. 57, 470–475. doi: 10.1001/archneur.57.4.470
Ryan, J. J., Sattler, J. M., and Lopez, S. J. (2000). Age effects on wechsler adult intelligence scale-III subtests. Arch. Clin. Neuropsychol. 15, 311–317. doi: 10.1016/s0887-6177(99)00019-0
Salthouse, T. A. (2010). Selective review of cognitive aging. J. Int. Neuropsychol. Soc. 16, 754–760. doi: 10.1017/S1355617710000706
Scherfler, C., Seppi, K., Donnemiller, E., Goebel, G., Brenneis, C., Virgolini, I., et al. (2005). Voxel-wise analysis of [I-123]β-CIT SPECT differentiates the Parkinson variant of multiple system atrophy from idiopathic Parkinson’s disease. Brain 128, 1605–1612. doi: 10.1093/brain/awh485
Shafto, M. A., and Tyler, L. K. (2014). Language in the aging brain: the network dynamics of cognitive decline and preservation. Science 346, 583–587. doi: 10.1126/science.1254404
Siepel, F. J., Dalen, I., Gruner, R., Booij, J., Bronnick, K. S., Buter, T. C., et al. (2016). Loss of dopamine transporter binding and clinical symptoms in dementia with lewy bodies. Mov. Disord. 31, 118–125. doi: 10.1002/mds.26327
Smith, E. E., and Jonides, J. (1997). Working memory: a view from neuroimaging. Cogn. Psychol. 33, 5–42. doi: 10.1006/cogp.1997.0658
Tatsch, K., and Poepperl, G. (2013). Nigrostriatal dopamine terminal imaging with dopamine transporter SPECT: an update. J. Nucl. Med. 54, 1331–1338. doi: 10.2967/jnumed.112.105379
Tossici-Bolt, L., Hoffmann, S. M., Kemp, P. M., Mehta, R. L., and Fleming, J. S. (2006). Quantification of [123I]FP-CIT SPECT brain images: an accurate technique for measurement of the specific binding ratio. Eur. J. Nucl. Med. Mol. Imaging 33, 1491–1499. doi: 10.1007/s00259-006-0155-x
Varrone, A., Dickson, J. C., Tossici-Bolt, L., Sera, T., Asenbaum, S., Booij, J., et al. (2013). European multicentre database of healthy controls for [I-123]FP-CIT SPECT (ENC-DAT): age-related effects, gender differences and evaluation of different methods of analysis. Eur. J. Nucl. Med. Mol. Imaging 40, 213–227. doi: 10.1007/s00259-012-2276-8
Verhaeghen, P., and Salthouse, T. A. (1997). Meta-analyses of age-cognition relations in adulthood: estimates of linear and nonlinear age effects and structural models. Psychol. Bull. 122, 231–249. doi: 10.1037/0033-2909.122.3.231
Volkow, N. D., Wang, G. J., Fowler, J. S., Ding, Y. S., Gur, R. C., Gatley, J., et al. (1998). Parallel loss of presynaptic and postsynaptic dopamine markers in normal aging. Ann. Neurol. 44, 143–147. doi: 10.1002/ana.410440125
Yeterian, E. H., and Pandya, D. N. (1991). Prefrontostriatal connections in relation to cortical architectonic organization in rhesus-monkeys. J. Comp. Neurol. 312, 43–67. doi: 10.1002/cne.903120105
Ziebell, M., Holm-Hansen, S., Thomsen, G., Wagner, A., Jensen, P., Pinborg, L. H., et al. (2010). Serotonin transporters in dopamine transporter imaging: A head-to-head comparison of dopamine transporter SPECT radioligands 123I-FP-CIT and 123I-PE2I. J. Nucl. Med. 51, 1885–1891. doi: 10.2967/jnumed.110.078337
Keywords: dopamine transporter, SPECT, cognitive function, aging, Wechsler Adult Intelligence Scale, verbal function
Citation: Li H, Hirano S, Furukawa S, Nakano Y, Kojima K, Ishikawa A, Tai H, Horikoshi T, Iimori T, Uno T, Matsuda H and Kuwabara S (2020) The Relationship Between the Striatal Dopaminergic Neuronal and Cognitive Function With Aging. Front. Aging Neurosci. 12:41. doi: 10.3389/fnagi.2020.00041
Received: 12 November 2019; Accepted: 06 February 2020;
Published: 28 February 2020.
Edited by:
Dominic James Hare, The University of Melbourne, AustraliaReviewed by:
Emmanuel Brouillet, Commissariat à l’Energie Atomique et aux Energies Alternatives (CEA), FranceJulien Rossignol, Central Michigan University, United States
Copyright © 2020 Li, Hirano, Furukawa, Nakano, Kojima, Ishikawa, Tai, Horikoshi, Iimori, Uno, Matsuda and Kuwabara. This is an open-access article distributed under the terms of the Creative Commons Attribution License (CC BY). The use, distribution or reproduction in other forums is permitted, provided the original author(s) and the copyright owner(s) are credited and that the original publication in this journal is cited, in accordance with accepted academic practice. No use, distribution or reproduction is permitted which does not comply with these terms.
*Correspondence: Shigeki Hirano, c19oaXJhbm9AY2hpYmEtdS5qcA==