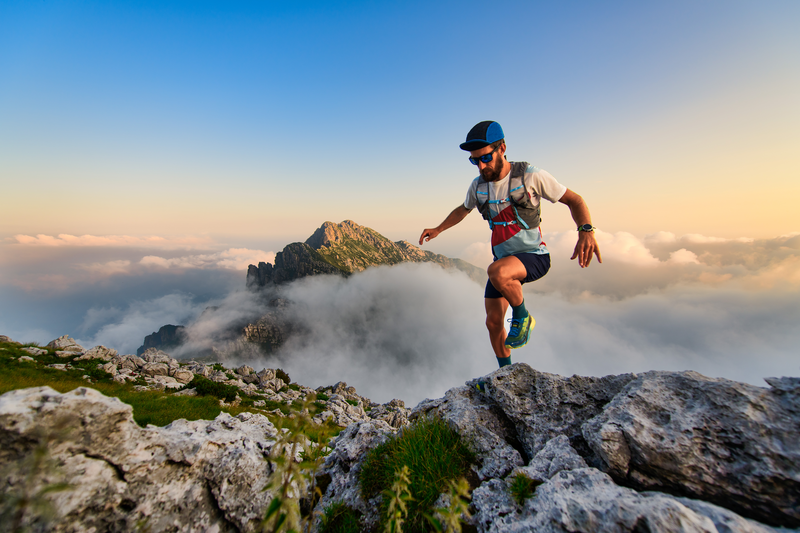
95% of researchers rate our articles as excellent or good
Learn more about the work of our research integrity team to safeguard the quality of each article we publish.
Find out more
ORIGINAL RESEARCH article
Front. Aging Neurosci. , 13 February 2019
Sec. Neuroinflammation and Neuropathy
Volume 11 - 2019 | https://doi.org/10.3389/fnagi.2019.00025
Objective: To determine the longitudinal relationship between monocyte chemotactic protein 1 (MCP-1)/CCL2 and memory function in older adults.
Methods: We examined longitudinal plasma MCP-1/CCL2 levels and a longitudinal verbal memory measure (CVLT-II 20’ recall) in a sample of 399 asymptomatic older adults (mean age = 72.1). Total visits ranged from 1 to 8, with an average time of 2.1 years between each visit, yielding 932 total observations. In order to isolate change over time, we decomposed MCP-1/CCL2 into subject-specific means and longitudinal deviations from the mean. The decomposed MCP-1/CCL2 variables were entered as predictors in linear mixed effects models, with age at baseline, sex, and education entered as covariates and recall as the longitudinal outcome. In follow-up analyses, we controlled for global cognition and APOE genotype, as well as baseline vascular risk factors. We also examined the specificity of findings by examining the longitudinal association between the MCP-1/CCL2 variables and non-memory cognitive tests.
Results: Within-subject increases in MCP-1/CCL2 levels were associated with decreases in delayed recall (t = −2.65; p = 0.01) over time. Results were independent of global cognitive function and APOE status (t = −2.30, p = 0.02), and effects remained when controlling for baseline vascular risk factors (t = −1.92, p = 0.05). No associations were noted between within-subject increases in MCP-1/CCL2 levels and other cognitive domains.
Conclusions: In an asymptomatic aging adult cohort, longitudinal increases in MCP-1/CCL2 levels were associated with longitudinal decline in memory. Results suggest that “healthy aging” is typified by early remodeling of the immune system, and that the chemokine, MCP-1/CCL2, may be associated with negative memory outcomes.
Dysregulation of immune cascades is considered to be a core component of aging and has been linked with negative pathological outcomes. The impact of the peripheral immune system on cognitive aging outcomes has been controversial, however, with higher levels of circulating inflammatory markers often being conceptualized as non-specific, downstream effects of aging with unclear significance to the central nervous system (CNS; Galasko and Montine, 2010; Galasko and Golde, 2013). Recent studies suggest, however, that the peripheral milieu actively communicates with the CNS, such that blood-borne, circulating immune markers may not only accelerate the peripheral aging process (i.e., immunosenescence; Franceschi et al., 2000; Baruch et al., 2013), but may also directly impact the CNS by impeding hippocampal neurogenesis (Villeda et al., 2011) and exacerbating cognitive decline (Cunningham et al., 2009; Westin et al., 2012).
Accumulating evidence indicates that chemokines, in particular, may play a critical role in bi-directional immune communication between the periphery and the CNS. Chemokines are chemoattractant cytokines that mediate inflammatory responses and regulate immune cell trafficking (i.e., migration of monocytes and lymphocytes). Monocyte chemotactic protein-1 (MCP-1/CCL2) was the first discovered human CC family chemokine (chr.17, q11.2; Van Coillie et al., 1999) and has been identified as a key pro-aging immune factor involved in cellular senescence (i.e., the Senescence-Associated Secretory Phenotype; Fumagalli and d’Adda di Fagagna, 2009). Animal studies suggest that blood-borne MCP-1/CCL2 levels are elevated in older mice and young heterochronic parabionts (i.e., a young mouse whose vascular system is joined with an older mouse), are linked with age-related decline in neurogenesis, and are associated with poorer memory function (Villeda et al., 2011; Villeda and Wyss-Coray, 2013); moreover, MCP-1/CCL2 knockout mice evidence increased neurogenesis in the dentate gyrus subsequent to cranial irradiation (Lee et al., 2013).
Human studies of MCP-1/CCL2 levels have extended these findings, and demonstrated that baseline blood MCP-1/CCL2 levels in late life are associated with more pernicious clinical outcomes (Lee et al., 2018). Recent work from our laboratory showed that blood MCP-1/CCL2 levels have selective cognitive and neuroanatomical relevance in both typical aging as well as early stages of Alzheimer’s disease (AD), such that higher MCP-1/CCL2 levels are related to worse episodic memory function and smaller left medial temporal lobes. Moreover, study results suggested that associations between MCP-1/CCL2 and cognition were both sensitive and specific to episodic memory, as no effects were observed between the circulating chemokine and other indices of neuropsychological function (Bettcher et al., 2016). We also recently demonstrated that in the context of lower CSF levels of Aβ42 (i.e., reflecting higher brain amyloid load), higher levels of blood MCP-1/CCL2 levels were correlated with higher levels of CSF phosphorylated and total tau in asymptomatic older adults (Bettcher et al., 2018a). Collectively, these results suggest that blood-borne MCP-1/CCL2 levels may reflect early AD-related pathological changes in the CNS and may specifically relate to worse episodic memory outcomes in aging adults.
Although prior studies highlight predominantly a cross-sectional association between peripheral MCP-1/CCL2 levels and negative aging outcomes, no study to date has addressed whether increases in blood MCP-1/CCL2 levels are related to tandem declines in episodic memory over the time. Examination of peripheral MCP-1/CCL2 levels and cognitive outcomes in a longitudinal framework is important and necessary to fully explicate the strength and directionality of associations between circulating inflammation and late life decline. Moreover, delineation of these longitudinal relationships is critical to our understanding of the neurobiological role of peripheral chemokine markers on aging outcomes, and may further explicate the dynamic associations between inflammation and cognitive decline over time (Bettcher and Kramer, 2014). To address this significant gap in the literature, the overarching goal of this study is to assess the longitudinal relationship between MCP-1/CCL2 and memory function in a cohort of asymptomatic older adults. We hypothesized that increases in MCP-1/CCL2 levels would be an independent predictor of memory decline, even after accounting for mean MCP-1/CCL2 levels and global cognitive functions.
A sample of 399 clinically healthy, community dwelling older adult participants was obtained from the University of California, San Francisco (UCSF) Memory and Aging Center database based on the availability of memory testing and stored blood specimens, the latter of which was acquired within 120 days of their memory assessment. The sample cohort was assembled from several longitudinal umbrella studies on healthy aging and cognition (i.e., NIH Aging and Cognition study; Larry L. Hillblom foundation study; NIH Chronic Inflammation study), and were between the ages of 50 and 99 years. In addition to measures of episodic memory and global cognitive function, participants also underwent brief cognitive testing that included measures of processing speed, executive functions, language, and visuospatial function. These measures were included in exploratory analyses (see below) to evaluate the specificity of our findings.
In terms of inclusion/exclusion criteria of these umbrella studies, all participants were reviewed in a screening visit, which entailed an informant interview, neurological examination, and cognitive testing. Inclusion as a “neurologically healthy” participant was based on several criteria, including a Mini-Mental State Exam score of ≥26, Clinical Dementia Rating score of 0, and no subject or informant report of significant cognitive decline during the previous year. Participants were excluded if they had a major psychiatric disorder, neurological condition affecting cognition (e.g., Parkinson’s disease, epilepsy; large vessel infarct), dementia or mild cognitive impairment, substance abuse, significant systemic medical illness (e.g., cancer; renal failure), current medications likely to affect CNS functions (e.g., long-active benzodiazepines), significant sensory or motor deficits that would interfere with cognitive testing, current depression (Geriatric Depression Scale Score greater than 15 of 30; Yesavage et al., 1982) or insulin-dependent diabetes.
For the purposes of the current study, participants were included in the data analysis if they were considered healthy [i.e., no diagnosis of mild cognitive impairment (MCI), AD dementia, or any other neurodegenerative phenotype] at their baseline visit1. All participants were reviewed at a case conference with a board-certified neuropsychologist (JK) and neurologist. All longitudinal memory and blood data available for each participant were included in analyses. As noted, data were culled from numerous healthy aging studies, thus longitudinal data for each participant may include data from a single study or multiple studies over time. As such, longitudinal follow-up times for each participant varied (see “Results” section for number of follow-up visits).
Primary demographic and cognitive variables, as well as information regarding the number of observations for each participant are reported in Table 1. The study was approved by the UCSF committee on human research, and all subjects provided written, IRB-approved informed consent before participating and approved the use of their blood samples and data for the study.
Our primary outcome variable was longitudinal episodic memory and was selected due to its consistent and robust associations with early stages of AD (Kramer et al., 2003; Ewers et al., 2012; Dickerson and Wolk, 2011) as well as its sensitivity to immune dysregulation (Lin et al., 2009; Bettcher et al., 2012, 2016). Episodic memory was assessed longitudinally with a list-learning measure of verbal memory, the California Verbal Learning Test-II (Delis et al., 2000). For data reduction purposes, 20-min delayed free recall was used as the primary longitudinal variable of interest.
After collection, each blood sample was centrifuged at 2,000× g for 15 min at 4°C with the resultant plasma divided into 500 μL aliquots and stored at −80°C. All plasma assays were conducted following the manufacturer’s protocol for Human Chemokine Panel 1 V-PLEX Plus kit (Meso Scale Diagnostics, Rockville, MD, USA). We elected to use V-PLEX kits given their lot-to-lot consistency for longitudinal analyses (i.e., reducing batch effects). Each array was scanned using a MESO QuickPlex SQ 120. Manufacturer supplied software (Discover Workbench 4.0) was used to quantify the concentration of MCP-1 based on sample dilution and relative to the supplied in-assay standard curve. Nominal recovery for control levels remained between 111–120% with a coefficient of variation (CV) <10%. Standard curve CVs for participant sample detection range remained <15% with standard sample recovery at 100% (+/− 5%) across all plates.
Importantly, we elected to employ a hypothesis-based approach and focus on MCP-1/CCL2 for several reasons. First, recent, striking associations between higher MCP-1/CCL2 levels and reduced hippocampal neurogenesis in parabiosis animal models have been reported (Villeda et al., 2011). Second, human studies of aging suggest that MCP-1/CCL2 may be a critical chemokine involved in memory decline in AD (Westin et al., 2012). In addition, our laboratory has shown that MCP-1/CCL2 is not only sensitive to episodic memory dysfunction in aging and AD, but may also be specific to this cognitive domain (Bettcher et al., 2016). Collectively, recent data suggest that MCP-1/CCL2 levels may be a key regulator of memory outcomes in aging adults and may be dysregulated early in pathological aging trajectories. Nonetheless, in order to account for associations between chemokines and cognitive function outside of our primary hypotheses, we also examined other detectable chemokines from the Human Chemokine V-PLEX in exploratory analyses. Chemokines that were detectable for more than 80% of the sample across longitudinal time points included: Eotaxin-1, IP-10, MCP-4, MDC, TARC, and MIP-1β.
APOE single nucleotide polymorphism (SNP) genotyping was carried out by real time polymerase chain reaction (rtPCR), on an Applied Biosystems 7900HT Real Time PCR machine using the Taqman SNP Genotyping Assay for rs429358 and rs7412 with identification numbers C___3084793_20 and C____904973_10, respectively (Applied Biosystems, Foster City, CA, USA). The protocol was followed as outlined in the manufacturer’s instructions, and every assay was performed in duplicate.
Linear mixed effect models using maximum likelihood estimates with random intercepts were conducted. The predictor of interest was longitudinal MCP-1/CCL2 levels; in order to isolate change over time, we decomposed MCP-1/CCL2 into subject-specific means (i.e., between subject factor) and longitudinal deviations from the mean (i.e., within subject factor). To increase the scientific rigor of the analyses, both decomposed factors were simultaneously entered into the regression models as predictors to elucidate whether the change in MCP-1/CCL2 levels vs. average MCP-1/CCL2 levels were associated with longitudinal change in memory function. Age at baseline, sex, and education were entered as nuisance covariates in all models and 20-min delayed free recall on the CVLT-II served as the longitudinal outcome.
In secondary analyses, linear mixed effects models were repeated, but we also controlled for global cognition [Mini Mental State Examination (MMSE)] and APOE genotype. We also examined whether these longitudinal associations were independent of baseline vascular risk factors by including baseline body mass index (BMI) and baseline systolic blood pressure as nuisance covariates in our mixed effects models.
In exploratory analyses, we first evaluated whether the longitudinal associations between peripheral inflammation and memory were specific to MCP-1/CCL2 by analyzing other chemokines in separate models. Specifically, we repeated the longitudinal measurement approach used with MCP-1 (i.e., decomposing chemokine levels into subject-specific means and longitudinal deviations from the mean) with six control chemokines (i.e., Eotaxin-1, IP-10, MCP-4, MDC, TARC, and MIP-1β) in separate models. These exploratory analyses allowed us to examine whether chemokines not included in our a priori hypotheses were dynamically related to memory over time. Next, we also examined whether longitudinal changes in MCP-1/CCL2 levels were specific to longitudinal memory performance by including comparator indices of executive functions (i.e., modified trail making test, total time; stroop inhibition, total correct), processing speed (i.e., stroop color naming, total correct), language (i.e., animal fluency; total correct), and visuospatial function (i.e., VOSP number location test; total correct; Warrington and James, 1991) as separate longitudinal outcome variables. Please see prior publications for additional details on this standard battery of cognitive measures (Kramer et al., 2003).
All longitudinal analyses were conducted with SAS 9.4 using the Proc Mixed routine.
As noted in Table 1, our participant sample was comprised of 399 aging adults whose baseline ages ranged from 51 to 99 (mean = 72 years). Visits ranged from 1 to 8 with an average of 2.1 years between visits (SD = 1.2 years; minimum: 0.5 years; maximum: 7.6 years), yielding 932 total observations. Higher baseline levels of MCP-1/CCL2 were significantly associated with higher age at baseline (r = 0.24; p < 0.0001). Correlations between higher baseline MCP-1/CCL2 levels and lower baseline delayed recall were notable for small effect sizes (r = −0.10; p = 0.03).
We examined the effects of both the within-subject change in MCP-1/CCL2 levels and the average MCP-1/CCL2 levels on longitudinal memory outcomes, controlling for baseline age, sex, and education level. Results showed that within-subject increases in MCP-1/CCL2 levels (unstandardized beta = −0.011, SE = 0.004, p = 0.008) were associated with declines in delayed recall performance on memory testing over time. In contrast, when examining the effect of average MCP-1/CCL2 levels, a unit difference in mean values between participants resulted in a non-statistical difference in outcome (unstandardized beta < 0.0001, p = 0.841). As shown in Table 2, longitudinal, within-subject increases in MCP-1/CCL2 levels were associated with a 10× greater effect on longitudinal memory outcomes relative to the average MCP-1/CCL2 levels.
Table 2. Mixed effect model results: within-subject increases in monocyte chemotactic protein 1 (MCP-1) are related to longitudinal decreases in memory.
Of note, we decomposed MCP-1/CCL2 values based on subject-specific means in order to protect against subject-level confounding (Neuhaus and McCulloch, 2006); however, we also repeated the analyses using baseline MCP-1/CCL2 levels as the between subject effect, and deviation from the baseline MCP-1/CCL2 levels as the within-subject effect; no substantive changes in results were noted (baseline MCP-1/CCL2 levels: estimate = −0.0008; p = 0.88; change in MCP-1 levels: estimate = −0.010; p = 0.007).
To determine whether increases in MCP-1/CCL2 levels were related to decline in delayed memory performance, over and above global cognitive performance and APOE status, we repeated the previous analyses with APOE status (i.e., presence or absence of E4 allele) and MMSE performance (i.e., total score, time-varying covariate) as nuisance covariates. Increases in MCP-1/CCL2 levels remained an independent predictor of verbal memory decline after accounting for APOE status and MMSE changes over time (change in MCP-1/CCL2: estimate = −0.009; SE = 0.004; t = −2.30; p = 0.02).
Considering the known association between vascular risk factors and both inflammatory markers and cognitive decline, we included baseline systolic blood pressure (mean = 134.19 mmHg; SD = 16.96) and baseline BMI (mean = 25.53; SD = 3.92) as predictors in the model, in addition to covariates from the previously described analyses (Questions 1 and 2). When controlling for baseline demographics, blood pressure, BMI, as well as APOE genotype and MMSE, within-subject increases in MCP-1/CCL2 remained associated with declines in verbal memory performance, although the effect size was notably reduced (change in MCP-1/CCL2: estimate = −0.008; SE = 0.004; t = −1.92; p = 0.05).
Based on prior animal work and on our group’s previously published work, we focused on MCP-1 as the primary pro-inflammatory chemokine in analyses; however, to determine if the effects of inflammation on memory function were specific to MCP-1, we examined additional chemokines from the same V-PLEX panel in a set of separate linear models. Partially consistent with our hypotheses, within-subject increases in several comparator chemokines (i.e., Eotaxin-1, MCP-4, TARC, IP-10, and MIP-1β) were not related to longitudinal change in verbal memory (see Table 3); however, within-subject increases in MDC (estimate = −0.001; t = −2.17; p = 0.03) were significantly related to declines in verbal memory.
Table 3. Mixed effect model results: increases in comparator chemokines and their association with longitudinal memory.
Although our prior work points to a specific effect of MCP-1/CCL2 levels on episodic memory, we elected to examine whether these findings remain robust in a longitudinal sample. Consistent with our prior cross-sectional study, within-subject increases in MCP-1/CCL2 were not statistically associated with changes in processing speed (stroop color naming: estimate = 0.022; t = 1.43; p = 0.15; observations = 901), executive functions (Modified Trail Making Time: estimate = 0.026; t = 0.93; p = 0.35; observations = 903; stroop inhibition: estimate = −0.008; t = −0.59: p = 0.55; observations = 899), language (animal fluency: estimate = 0.010; t = 1.26; p = 0.21; observations = 896), or visuospatial function (VOSP number localization: estimate = 0.001; t = 0.28; p = 0.78; observations = 903).
In this retrospective, longitudinal analysis of an asymptomatic older adult cohort, we found that increases in MCP-1/CCL2 levels were associated with longitudinal decline in verbal episodic memory. Results were independent of APOE status and global cognitive function, and modest effects remained when accounting for baseline vascular risk factors. Moreover, longitudinal effects of MCP-1/CCL2 on cognition were specific to episodic memory. Results are consistent with cross-sectional studies linking blood MCP-1/CCL2 levels to worse episodic memory performance (Bettcher et al., 2016), as well as studies indicating that higher baseline MCP-1/CCL2 levels are related to decline in cognitive function over time (Lee et al., 2018). To our knowledge, however, this is the first study to extend findings to a longitudinal framework (i.e., longitudinal predictors; longitudinal outcome). Our results demonstrate that longitudinal increases in blood MCP-1/CCL2 levels are related to tandem declines in episodic memory over time in an asymptomatic aging cohort.
The noted association between increases in MCP-1/CCL2 levels and episodic memory decline highlight several important points, as well as raise questions about the role of immune dysregulation in pathological aging trajectories. First, study results suggest that longitudinal associations between MCP-1/CCL2 levels and memory performance are evident in otherwise healthy older adults. Memory scores were not in the impaired range, indicating that even within the context of “normal” memory performance, within-subject decline can be mapped on to increases in circulating MCP-1/CCL2 levels. Identifying dynamic biomarkers in plasma that are sensitive to clinically meaningful outcomes in asymptomatic adults is important for understanding both heterogeneity of aging trajectories and early disease processes. Our results point to an early and cognitively salient role for MCP-1/CCL2 levels in late life outcomes. Study results further support the possibility of a dynamic association between the peripheral immune milieu and CNS. More specifically, study findings suggest that increases in MCP-1/CCL2 levels are stronger predictors of memory decline than either average or baseline MCP-1/CCL2 levels, with change in MCP-1/CCL2 associated with a 10 × greater effect on memory outcomes than average MCP-1/CCL2 levels. From both a clinical and research perspective, this indicates that ascertaining a single time point or even averaging several time points of MCP-1/CCL2 levels may be less informative than examining the longitudinal, within-person change in levels. The temporal evolution of chemokine levels in the context of salient cognitive outcomes is critical to our understanding of how immune dysregulation might map on to—or even herald—clinical decline; while study results do not pinpoint whether immune dysregulation is an early marker of AD pathogenesis, nor do they address causality due to the non-interventional design, they do suggest that chronic immune dysregulation may presage clinical symptomology and may track with subtle memory decline in late life.
Results from our study also suggest that the relationships between longitudinal MCP-1/CCL2 levels and memory decline are independent of APOE status and global cognitive performance, with modest effects remaining after controlling for baseline vascular risk factors. We focused our analysis on episodic memory decline based on prior work indicating that MCP-1/CCL2 levels may have specific effects on medial temporal lobe structures and memory in both animal models (Villeda and Wyss-Coray, 2013) and human aging studies (Bettcher et al., 2016). Our exploratory analyses provide additional support for the specificity of MCP-1/CCL2 levels on memory performance, as the effects of within-subject increases in MCP-1/CCL2 on cognition were solely noted with the verbal memory measure in our sample. Thus, although we examined longitudinal associations with measures of processing speed, executive functions, language, and visuospatial function, no significant results were observed with other cognitive domains. Overall, our study results indicate that the strength of the association between increases in MCP-1/CCL2 levels and decreases in episodic memory are not substantively impacted by subjects’ overall cognitive status, and that longitudinal associations between MCP-1/CCL2 and cognition may be specific to memory.
It is worth noting, however, that the effect size of longitudinal MCP-1/CCL2 levels on memory were impacted by the inclusion of baseline vascular risk factors. Results remained statistically significant, but the independent association of longitudinal MCP-1/CCL2 levels on episodic memory was modest after accounting for baseline BMI and baseline systolic blood pressure. The intersection of immune biology and vascular pathways has garnered significant attention in the past decade (Yaffe et al., 2004; Hoshi et al., 2005, 2010; Fornage et al., 2008; Giunta, 2008; Hoth et al., 2008; Zhang et al., 2009; Gallacher et al., 2010; Espinola-Klein et al., 2011; Grammas et al., 2011; Grammas, 2011; Bettcher et al., 2014), but remains a poorly understood mechanism for cognitive decline in late life. Inflammation is by definition a vascular process that stimulates changes in vascular permeability and, when dysregulated, can alter endothelial metabolic pathways and induce neurotoxic effects. (Grammas, 2011) Considering that chemokines and vascular risk factors are both linked to the development of MCI and AD (Kivipelto et al., 2001; Schmidt et al., 2002; Gottesman et al., 2017), examining these factors in isolation likely represents an overly simplistic and potentially myopic approach to understanding the role of immune biology in aging outcomes. Our study suggests that whereas peripheral MCP-1/CCL2 levels may be independent contributors to episodic memory decline, disentangling their role from vascular risk factors is still an important, but understudied area of research that has yet to be resolved.
In follow-up analyses, we also examined whether the negative association between increasing inflammation and decreasing verbal memory was driven primarily by changes in MCP-1/CCL2 levels or by more diffuse alterations in chemokine levels in the periphery. The role of chemokines as potent chemotactic factors for monocytes is well documented, and MCP-1/CCL2 in particular has been strongly linked with age-related diseases, including AD (Deshmane et al., 2009; Bettcher et al., 2016, 2018a; Lee et al., 2018). Of the six comparison or “control” chemokines we examined, significant associations were only noted for increasing MDC/CCL22 levels and declining memory performance, and this association was weaker than that of MCP-1/CCL2. The role of MDC levels as potential mediators of cognitive decline has been far less researched in the field of aging and AD, thus it remains unclear if MDC levels will serve as a possible biomarker for cognitive aging. Nonetheless, while our results corroborate and extend prior reports on the role of MCP-1 in cognitive aging, they also raise important questions regarding the contribution of MDC to memory decline and the sensitivity of specific CC family chemokines to aging outcomes.
An additional consideration when interpreting the longitudinal role of peripheral MCP-1/CCL2 levels on cognitive decline is the mechanism by which the periphery might induce changes in the CNS. Increasing evidence from basic science literature points to an alteration in the milieu surrounding the epithelial monolayers of the choroid plexus (CP), which serves as a critical immune nexus between the blood and CSF. (Baruch et al., 2014). Recent reports suggest that the blood-CSF barrier undergoes functional alterations with age, which may be exacerbated in the presence of (Vargas et al., 2010) and possibly preceding (Chalbot et al., 2011) amyloid deposition. The downstream effects of these age- and pathology-related changes in the CP environment include the potential for increased communication between the periphery and CNS (Dá Mesquita et al., 2016). Although in vivo methods for assessing the immune milieu surrounding the CP have not been clearly established in humans, it may serve as a future target for understanding the mechanistic role of peripheral immune dysregulation on cognitive decline.
The current study has numerous strengths, including the longitudinal assessment of both MCP-1/CCL2 levels and verbal episodic memory measurements in healthy older adults, as well as the appraisal of APOE status and baseline vascular risk factors. In terms of methodological limitations to the study, we elected to focus on healthy older adults who enrolled in several aging studies at UCSF to maximize sample size and number of observations; as such, our participants reflected a convenience sample of community-dwelling, highly educated older adults rather than an epidemiological sample. Sampling bias and generalizability of study findings remains a critical issue in observational research; future studies should examine whether similar findings are noted using community-based sampling methods with demographically diverse populations. An additional limitation to the current study is the lack of baseline amyloid and tau biomarkers. Heterogeneity in “normal” aging trajectories is characterized in part by the presence of pathology—including vascular disease, amyloid deposition and tau pathology (Jack et al., 2013)—which was not assessed in the current study. Considering that elevations in peripheral inflammatory levels may exacerbate, and even precede, markers of AD-related pathology, it will be important to incorporate these indices to gain a better understanding of the temporal properties of immune dysregulation in late life. Finally, our statistic modeling approach included the use of the MMSE as a measure of global cognitive function; we incorporated this assessment given its availability in our dataset as well as its wide use a proxy for global cognition. In a healthy older adult sample, however, the variability in this measure is limited, and is prone to ceiling affects.
In summary, in a sample of asymptomatic older adults, longitudinal increases in MCP-1/CCL2 levels were related to declines in verbal episodic memory over time, and these results were independent of APOE status, with modest effects remaining after accounting for baseline vascular risk factors. Exploratory analyses further suggest that the longitudinal association between MCP-1/CCL2 levels and cognition may be specific to memory. Results add to a growing body of literature suggesting that “healthy aging” is typified by remodeling of the immune system, and that the blood-borne chemokine, MCP-1/CCL2, may be an early marker of innate immune dysregulation that is sensitive to negative memory outcomes.
The datasets for this study will not be made publicly available because the datasets for this manuscript are not publicly available because of HIPAA-related concerns (i.e., information that could compromise the privacy of research participants; ages over 89). Requests to access the datasets via data use agreements should be directed to Joel H. Kramer, PsyD (joel.kramer@ucsf.edu).
BB assisted in collection of the data, developed the hypotheses, conducted the statistical analysis, and wrote the manuscript draft. JN assisted with the statistical analyses and critically revised the manuscript. MW, FE, KC, RS, and AK assisted with data collection and critically revised the manuscript. RF processed all blood specimens, conducted analyses of the inflammatory markers and critically revised the manuscript. JK developed the hypotheses, collected the data, and critically revised the manuscript.
This study was supported by National Institutes of Health (NIH)-National Institute on Aging (NIA) grants K23AG042492 [principal investigator (PI): Bettcher], NIA 1R01AG032289 (PI: Kramer), R01AG048234 (PI: Kramer) and UCSF Alzheimer’s Disease Research Center (ADRC) P50 AG023501. Our work was also supported by Larry L. Hillblom Network Grant for the Prevention of Age-Associated Cognitive Decline 2014-A-004-NET (PI: Kramer). Stipend support to RS is funded by National Institute on Alcohol Abuse and Alcoholism (NIAAA) award T32AA013525.
The authors declare that the research was conducted in the absence of any commercial or financial relationships that could be construed as a potential conflict of interest.
A portion of this work was presented at the 2018 International Neuropsychological Society Conference in Washington, D.C. (Bettcher, Published Online by Cambridge University Press: 12 June 2018; Bettcher et al., 2018b).
Baruch, K., Deczkowska, A., David, E., Castellano, J. M., Miller, O., Kertser, A., et al. (2014). Aging. Aging-induced type I interferon response at the choroid plexus negatively affects brain function. Science 346, 89–93. doi: 10.1126/science.1252945
Baruch, K., Ron-Harel, N., Gal, H., Deczkowska, A., Shifrut, E., Ndifon, W., et al. (2013). CNS-specific immunity at the choroid plexus shifts toward destructive Th2 inflammation in brain aging. Proc. Natl. Acad. Sci. U S A 110, 2264–2269. doi: 10.1073/pnas.1211270110
Bettcher, B. M., Fitch, R., Wynn, M. J., Lalli, M. A., Elofson, J., Jastrzab, L., et al. (2016). MCP-1 and eotaxin-1 selectively and negatively associate with memory in MCI and Alzheimer’s disease dementia phenotypes. Alzheimers Dement. Amst. 3, 91–97. doi: 10.1016/j.dadm.2016.05.004
Bettcher, B. M., Johnson, S. C., Fitch, R., Casaletto, K. B., Heffernan, K. S., Asthana, S., et al. (2018a). Cerebrospinal fluid and plasma levels of inflammation differentially relate to CNS markers of Alzheimer’s disease pathology and neuronal damage. J. Alzheimers Dis. 62, 385–397. doi: 10.3233/jad-170602
Bettcher, B. M., Wynn, J. M., Saloner, R., Fitch, R., Karydas, A., and Kramer, J. (2018b). Final program forty sixth annual meeting international neuropsychological society. J. Int. Neuropsychol. Soc. 24:325. doi: 10.1017/s1355617718000528
Bettcher, B. M., and Kramer, J. H. (2014). Longitudinal inflammation, cognitive decline, and Alzheimer’s disease: a mini-review. Clin. Pharmacol. Ther. 96, 464–469. doi: 10.1038/clpt.2014.147
Bettcher, B. M., Watson, C. L., Walsh, C. M., Lobach, I. V., Neuhaus, J., Miller, J. W., et al. (2014). Interleukin-6, age, and corpus callosum integrity. PLoS One 9:e106521. doi: 10.1371/journal.pone.0106521
Bettcher, B. M., Wilheim, R., Rigby, T., Green, R., Miller, J. W., Racine, C. A., et al. (2012). C-reactive protein is related to memory and medial temporal brain volume in older adults. Brain Behav. Immun. 26, 103–108. doi: 10.1016/j.bbi.2011.07.240
Chalbot, S., Zetterberg, H., Blennow, K., Fladby, T., Andreasen, N., Grundke-Iqbal, I., et al. (2011). Blood-cerebrospinal fluid barrier permeability in Alzheimer’s disease. J. Alzheimers Dis. 25, 505–515. doi: 10.3233/jad-2011-101959
Cunningham, C., Campion, S., Lunnon, K., Murray, C. L., Woods, J. F., Deacon, R. M., et al. (2009). Systemic inflammation induces acute behavioral and cognitive changes and accelerates neurodegenerative disease. Biol. Psychiatry 65, 304–312. doi: 10.1016/j.biopsych.2008.07.024
Dá Mesquita, S., Ferreira, A. C., Sousa, J. C., Correia-Neves, M., Sousa, N., and Marques, F. (2016). Insights on the pathophysiology of Alzheimer’s disease: the crosstalk between amyloid pathology, neuroinflammation and the peripheral immune system. Neurosci. Biobehav. Rev. 68, 547–562. doi: 10.1016/j.neubiorev.2016.06.014
Delis, D. C., Kramer, J. H., Kaplan, E., and Ober, B. A. (2000). California Verbal Learning Test. 2nd Edn. San Antonio, TX: The Psychological Corporation.
Deshmane, S. L., Kremlev, S., Amini, S., and Sawaya, B. E. (2009). Monocyte chemoattractant protein-1 (MCP-1): an overview. J. Interferon Cytokine Res. 29, 313–326. doi: 10.1089/jir.2008.0027
Dickerson, B. C., Wolk, D. A., and Alzheimer’s Disease Neuroimaging Initiative. (2011). Dysexecutive versus amnesic phenotypes of very mild Alzheimer’s disease are associated with distinct clinical, genetic and cortical thinning characteristics. J. Neurol. Neurosurg. Psychiatry 82, 45–51. doi: 10.1136/jnnp.2009.199505
Espinola-Klein, C., Gori, T., Blankenberg, S., and Munzel, T. (2011). Inflammatory markers and cardiovascular risk in the metabolic syndrome. Front. Biosci. 16, 1663–1674. doi: 10.2741/3812
Ewers, M., Walsh, C., Trojanowski, J. Q., Shaw, L. M., Petersen, R. C., Jack, C. R. Jr., et al. (2012). Prediction of conversion from mild cognitive impairment to Alzheimer’s disease dementia based upon biomarkers and neuropsychological test performance. Neurobiol. Aging 33, 1203–1214. doi: 10.1016/j.neurobiolaging.2010.10.019
Fornage, M., Chiang, Y. A., O’Meara, E. S., Psaty, B. M., Reiner, A. P., Siscovick, D. S., et al. (2008). Biomarkers of inflammation and MRI-defined small vessel disease of the brain: the cardiovascular health study. Stroke 39, 1952–1959. doi: 10.1161/strokeaha.107.508135
Franceschi, C., Bonafe, M., Valensin, S., Olivieri, F., De Luca, M., Ottaviani, E., et al. (2000). Inflamm-aging. An evolutionary perspective on immunosenescence. Ann. N Y Acad. Sci. 908, 244–254. doi: 10.1111/j.1749-6632.2000.tb06651.x
Fumagalli, M., and d’Adda di Fagagna, F. (2009). SASPense and DDRama in cancer and ageing. Nat. Cell Biol. 11, 921–923. doi: 10.1038/ncb0809-921
Galasko, D., and Golde, T. E. (2013). Biomarkers for Alzheimer’s disease in plasma, serum and blood—conceptual and practical problems. Alzheimers Res. Ther. 5:10. doi: 10.1186/alzrt164
Galasko, D., and Montine, T. J. (2010). Biomarkers of oxidative damage and inflammation in Alzheimer’s disease. Biomark. Med. 4, 27–36. doi: 10.2217/bmm.09.89
Gallacher, J., Bayer, A., Lowe, G., Fish, M., Pickering, J., Pedro, S., et al. (2010). Is sticky blood bad for the brain?: hemostatic and inflammatory systems and dementia in the caerphilly prospective study. Arterioscler. Thromb. Vasc. Biol. 30, 599–604. doi: 10.1161/atvbaha.109.197368
Giunta, S. (2008). Exploring the complex relations between inflammation and aging (inflamm-aging): anti-inflamm-aging remodelling of inflamm- aging, from robustness to frailty. Inflamm. Res. 57, 558–563. doi: 10.1007/s00011-008-7243-2
Gottesman, R. F., Schneider, A. L., Zhou, Y., Coresh, J., Green, E., Gupta, N., et al. (2017). Association between midlife vascular risk factors and estimated brain amyloid deposition. JAMA 317, 1443–1450. doi: 10.1001/jama.2017.3090
Grammas, P. (2011). Neurovascular dysfunction, inflammation and endothelial activation: implications for the pathogenesis of Alzheimer’s disease. J. Neuroinflammation 8:26. doi: 10.1186/1742-2094-8-26
Grammas, P., Sanchez, A., Tripathy, D., Luo, E., and Martinez, J. (2011). Vascular signaling abnormalities in Alzheimer disease. Cleve. Clin. J. Med. 78, S50–S53. doi: 10.3949/ccjm.78.s1.09
Hoshi, T., Kitagawa, K., Yamagami, H., Furukado, S., Hougaku, H., and Hori, M. (2005). Relations of serum high-sensitivity C-reactive protein and interleukin-6 levels with silent brain infarction. Stroke 36, 768–772. doi: 10.1161/01.str.0000158915.28329.51
Hoshi, T., Yamagami, H., Furukado, S., Miwa, K., Tanaka, M., Sakaguchi, M., et al. (2010). Serum inflammatory proteins and frontal lobe dysfunction in patients with cardiovascular risk factors. Eur. J. Neurol. 17, 1134–1140. doi: 10.1111/j.1468-1331.2010.02990.x
Hoth, K. F., Haley, A. P., Gunstad, J., Paul, R. H., Poppas, A., Jefferson, A. L., et al. (2008). Elevated C-reactive protein is related to cognitive decline in older adults with cardiovascular disease. J. Am. Geriatr. Soc. 56, 1898–1903. doi: 10.1111/j.1532-5415.2008.01930.x
Jack, C. R. Jr., Knopman, D. S., Jagust, W. J., Petersen, R. C., Weiner, M. W., Aisen, P. S., et al. (2013). Tracking pathophysiological processes in Alzheimer’s disease: an updated hypothetical model of dynamic biomarkers. Lancet Neurol. 12, 207–216. doi: 10.1016/s1474-4422(12)70291-0
Kivipelto, M., Helkala, E. L., Laakso, M. P., Hänninen, T., Hallikainen, M., Alhainen, K., et al. (2001). Midlife vascular risk factors and Alzheimer’s disease in later life: longitudinal, population based study. BMJ 322, 1447–1451. doi: 10.1136/bmj.322.7300.1447
Kramer, J. H., Jurik, J., Sha, S. J., Rankin, K. P., Rosen, H. J., Johnson, J. K., et al. (2003). Distinctive neuropsychological patterns in frontotemporal dementia, semantic dementia, and Alzheimer disease. Cogn. Behav. Neurol. 16, 211–218. doi: 10.1097/00146965-200312000-00002
Lee, S. W., Haditsch, U., Cord, B. J., Guzman, R., Kim, S. J., Boettcher, C., et al. (2013). Absence of CCL2 is sufficient to restore hippocampal neurogenesis following cranial irradiation. Brain Behav. Immun. 30, 33–44. doi: 10.1016/j.bbi.2012.09.010
Lee, W. J., Liao, Y. C., Wang, Y. F., Lin, I. F., Wang, S. J., and Fuh, J. L. (2018). Plasma MCP-1 and cognitive decline in patients with Alzheimer’s disease and mild cognitive impairment: a two-year follow-up study. Sci. Rep. 8:1280. doi: 10.1038/s41598-018-19807-y
Lin, H. B., Yang, X. M., Li, T. J., Cheng, Y. F., Zhang, H. T., and Xu, J. P. (2009). Memory deficits and neurochemical changes induced by C-reactive protein in rats: implication in Alzheimer’s disease. Psychopharmacology 204, 705–714. doi: 10.1007/s00213-009-1499-2
Neuhaus, J., and McCulloch, C. E. (2006). Separating between- and within-cluster covariate effects by using conditional and partitioning methods. J. R. Stat. Soc. B 68, 859–872. doi: 10.1111/j.1467-9868.2006.00570.x
Schmidt, R., Schmidt, H., Curb, J. D., Masaki, K., White, L. R., and Launer, L. J. (2002). Early inflammation and dementia: a 25-year follow-up of the honolulu-asia aging study. Ann. Neurol. 52, 168–174. doi: 10.1002/ana.10265
Van Coillie, E., Van Damme, J., and Opdenakker, G. (1999). The MCP/eotaxin subfamily of CC chemokines. Cytokine Growth Factor Rev. 10, 61–86. doi: 10.1016/s1359-6101(99)00005-2
Vargas, T., Ugalde, C., Spuch, C., Antequera, D., Morán, M. J., Martín, M. A., et al. (2010). Aβ accumulation in choroid plexus is associated with mitochondrial-induced apoptosis. Neurobiol. Aging 31, 1569–1581. doi: 10.1016/j.neurobiolaging.2008.08.017
Villeda, S. A., Luo, J., Mosher, K. I., Zou, B., Britschgi, M., Bieri, G., et al. (2011). The ageing systemic milieu negatively regulates neurogenesis and cognitive function. Nature 477, 90–94. doi: 10.1038/nature10357
Villeda, S. A., and Wyss-Coray, T. (2013). The circulatory systemic environment as a modulator of neurogenesis and brain aging. Autoimmun. Rev. 12, 674–677. doi: 10.1016/j.autrev.2012.10.014
Warrington, E. K., and James, M. (1991). The Visual Object and Space Perception Battery. Bury St. Edmonds, Suffolk, England: Thames Valley Test Company.
Westin, K., Buchhave, P., Nielsen, H., Minthon, L., Janciauskiene, S., and Hansson, O. (2012). CCL2 is associated with a faster rate of cognitive decline during early stages of Alzheimer’s disease. PLoS One 7:e30525. doi: 10.1371/journal.pone.0030525
Yaffe, K., Kanaya, A., Lindquist, K., Simonsick, E. M., Harris, T., Shorr, R. I., et al. (2004). The metabolic syndrome, inflammation and risk of cognitive decline. JAMA 292, 2237–2242. doi: 10.1001/jama.292.18.2237
Yesavage, J. A., Brink, T. L., Rose, T. L., Lum, O., Huang, V., Adey, M., et al. (1982). Development and validation of a geriatric depression screening scale: a preliminary report. J. Psychiatr. Res. 17, 37–49. doi: 10.1016/0022-3956(82)90033-4
Keywords: chemokines, inflammation, CCL2, memory, longitudinal, cognitive aging
Citation: Bettcher BM, Neuhaus J, Wynn MJ, Elahi FM, Casaletto KB, Saloner R, Fitch R, Karydas A and Kramer JH (2019) Increases in a Pro-inflammatory Chemokine, MCP-1, Are Related to Decreases in Memory Over Time. Front. Aging Neurosci. 11:25. doi: 10.3389/fnagi.2019.00025
Received: 26 November 2018; Accepted: 29 January 2019;
Published: 13 February 2019.
Edited by:
Daniel Ortuño-Sahagún, Universidad de Guadalajara, MexicoReviewed by:
Hirokazu Ohtaki, Showa University, JapanCopyright © 2019 Bettcher, Neuhaus, Wynn, Elahi, Casaletto, Saloner, Fitch, Karydas and Kramer. This is an open-access article distributed under the terms of the Creative Commons Attribution License (CC BY). The use, distribution or reproduction in other forums is permitted, provided the original author(s) and the copyright owner(s) are credited and that the original publication in this journal is cited, in accordance with accepted academic practice. No use, distribution or reproduction is permitted which does not comply with these terms.
*Correspondence: Brianne M. Bettcher, YnJpYW5uZS5iZXR0Y2hlckB1Y2RlbnZlci5lZHU=
Disclaimer: All claims expressed in this article are solely those of the authors and do not necessarily represent those of their affiliated organizations, or those of the publisher, the editors and the reviewers. Any product that may be evaluated in this article or claim that may be made by its manufacturer is not guaranteed or endorsed by the publisher.
Research integrity at Frontiers
Learn more about the work of our research integrity team to safeguard the quality of each article we publish.