- 1The Healthy Aging and Neuropsychology Lab, Union College, Schenectady, NY, United States
- 2Alzheimer's Disease Center, Albany Medical Center, Albany, NY, United States
- 3Sunnyview Rehabilitation Hospital, Schenectady, NY, United States
- 4Stratton VA Medical Center, Albany, NY, United States
- 5Department of Biology, Union College, Schenectady, NY, United States
- 6Biomedical Sciences Department, Oklahoma State University, Tulsa, OK, United States
- 7Department of Anatomy and Cell Biology, Oklahoma State University, Tulsa, OK, United States
- 8Department of Health & Human Physiological Sciences, Skidmore College, Saratoga Springs, NY, United States
- 9Beckman Institute, University of Illinois, Urbana-Champaign, Champaign, IL, United States
Prior research has found that cognitive benefits of physical exercise and brain health in older adults may be enhanced when mental exercise is interactive simultaneously, as in exergaming. It is unclear whether the cognitive benefit can be maximized by increasing the degree of mental challenge during exercise. This randomized clinical trial (RCT), the Aerobic and Cognitive Exercise Study (ACES) sought to replicate and extend prior findings of added cognitive benefit from exergaming to those with or at risk for mild cognitive impairment (MCI). ACES compares the effects of 6 months of an exer-tour (virtual reality bike rides) with the effects of a more effortful exer-score (pedaling through a videogame to score points). Fourteen community-dwelling older adults meeting screening criteria for MCI (sMCI) were adherent to their assigned exercise for 6 months. The primary outcome was executive function, while secondary outcomes included memory and everyday cognitive function. Exer-tour and exer-score yielded significant moderate effects on executive function (Stroop A/C; d's = 0.51 and 0.47); there was no significant interaction effect. However, after 3 months the exer-tour revealed a significant and moderate effect, while exer-score showed little impact, as did a game-only condition. Both exer-tour and exer-score conditions also resulted in significant improvements in verbal memory. Effects appear to generalize to self-reported everyday cognitive function. Pilot data, including salivary biomarkers and structural MRI, were gathered at baseline and 6 months; exercise dose was associated with increased BDNF as well as increased gray matter volume in the PFC and ACC. Improvement in memory was associated with an increase in the DLPFC. Improved executive function was associated with increased expression of exosomal miRNA-9. Interactive physical and cognitive exercise (both high and low mental challenge) yielded similarly significant cognitive benefit for adherent sMCI exercisers over 6 months. A larger RCT is needed to confirm these findings. Further innovation and clinical trial data are needed to develop accessible, yet engaging and effective interventions to combat cognitive decline for the growing MCI population.
ClinicalTrials.gov ID: NCT02237560
Introduction
Annual diagnoses of dementia, such as due to Alzheimer's disease (AD), are expected to approach 1 million in 2050, more than double the new cases diagnosed in 2000 (Alzheimer's Association, 2014). Lacking a cure for any of the many causes of this devastating decline in cognitive function and loss of independence, a rising public health outcry has helped spark initiatives like the National Alzheimer's Project Act (2011) and the Healthy Brain Initiative (Alzheimer's Association and Centers for Disease Control Prevention, 2013). Research continues to press on, seeking innovative and empirically-validated ways to prevent or ameliorate cognitive decline and impairment.
While behavioral interventions are unlikely to completely prevent or halt dementia, there is the potential for physical exercise to reduce the risk of dementia onset (Heyn et al., 2004; Hillman et al., 2008) or slow progression (Larson, 2010). It has been estimated that if the onset of dementia could be delayed a few years, the impact on the population over time could be dramatic, decreasing prevalence by 1 million cases after 10 years in the United States alone. By also delaying progression for 2 years this could reduce dementia incidence by an astonishing 18 million cases globally per year (Sano et al., 1997; Brookmeyer et al., 1998). Interventions that reach patients before they have declined into diagnosable dementia can have the greatest impact. Thus, it is especially desirable to develop interventions that target those who may be at an intermediate stage, perhaps diagnosed with or at risk for mild cognitive impairment (MCI; per DSM-IV in 1994; Jak et al., 2009) also known more recently as mild neurocognitive disorder (mNCD per DSM-V in 2013; henceforth MCI will be used for simplicity; Roberts and Knopman, 2013; Jak et al., 2016).
Neuropsychological Effects of Physical and Cognitive Exercise
Researchers have been investigating a variety of behavioral interventions, including exercise, to improve cognitive function and decrease risk of progression to dementia among individuals with MCI (e.g., Kramer et al., 1999; Colcombe and Kramer, 2003; Studenski et al., 2006; Angevaren et al., 2008; Baker et al., 2010; Geda et al., 2010; Martin et al., 2011). Physical exercise has garnered significant support in the literature for its potency in effecting cognitive benefits (e.g., Colcombe and Kramer, 2003). While the literature examining the relationship between physical exercise and cognitive benefit is not without issues (e.g., recent Cochrane Reviews; Forbes et al., 2015; Young et al., 2015, which focused on dementia and normative older adults, respectively), it is widely accepted that exercise benefits cognition, particularly executive function among symptomatic individuals, that are yet early in their cognitive decline (e.g., MCI), the very population on which this study focused (e.g., Colcombe and Kramer, 2003; Etnier and Chang, 2009; Geda et al., 2010; Gates, 2013; Hess et al., 2014; Zheng et al., 2016). Indeed, pre-clinical persons, ranging from asymptomatic older adults to those with early MCI, may experience the greatest benefit from exercise, due to the relatively preserved brain structures and functions (in contrast with more progressed dementia) that can support and supply components needed to realize neuroplasticity as triggered by exercise (e.g., Colcombe et al., 2004; Kramer and Erickson, 2007; Ahlskog et al., 2011; Teixeira et al., 2016). However, knowing that physical exercise may be good for one's body and brain may not be enough to induce regular practice of exercise. Most older adults do not meet the guideline from the American College of Sports Medicine (ACSM) which now recommends 45 min per day, 5–7 days per week, including vigorous exercise (Chodzko-Zajko et al., 2009).
In a previous randomized clinical trial (Anderson-Hanley et al., 2012), we aimed to induce a thorough dose of exercise (Vidoni et al., 2015) by assigning older adults to an exergame1. We expected that the engagement of a stimulating exergame would motivate regular exercise, therefore allowing older adults to achieve maximum cognitive benefit. Three months of pedaling a virtual-reality-enhanced stationary ergometer, displaying scenic bike tours which we referred to as a “cybercycle” (see Figure 1), was compared with a traditional stationary bike. In the end, the cybercycle condition yielded significantly greater cognitive benefit, as hypothesized. However, surprisingly, it was not due to a difference in dose; we found instead that both the cybercycle and traditional cyclists has been similarly adherent (e.g., no significant difference between groups in miles, minutes, power, heart rate, etc.).
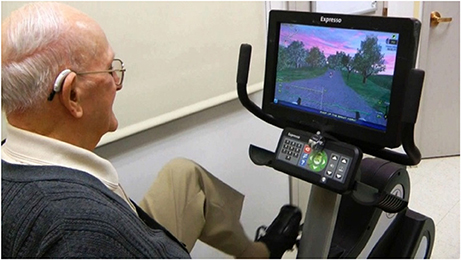
Figure 1. Stationary bike equipped with virtual reality display (aka “cybercycle” so named in our previous RCT; Anderson-Hanley et al., 2012). Former study participant demonstrating use of a cybercycle exergame; used with permission.
This unexpected finding raised the possibility that the screen display was providing benefit, beyond that of basic entertainment or motivation. We theorized the enhanced form of exercise was yielding findings much like those of the environmental enrichment literature (e.g., animals provided with greater physical and mental stimulation experience enhanced brain function; Pusic et al., 2016); thus it seems more (even two types at once) is generally better, although to a point. In humans the complexity of experience and the diversity of type, timing and compounding of “enrichment” makes this considerably challenging to study as a unique phenomenon. It seemed that for our participants, by interacting with the tour stimuli on screen (e.g., changing pedaling speed yielded a change in speed of scenery moving by), they were certainlyengaged in some additional amount of cognitive processing beyond the control condition. This layered cognitive stimulation may have combined with the physical exercise required of the exergame to create a “two-for-one” impact that resulted in the observed additional cognitive benefit for participants. Hence, we hypothesized that participants experienced better neuropsychological outcomes because of the potentially synergistic phenomena created by combining a type of “mental exercise” that was interactive with physical exercise (Anderson-Hanley et al., 2012; Frantzidis et al., 2014).
Several areas of the research literature speak to the possible validity of this hypothesized compounding or synergistic effect. As previously mentioned, there is a robust literature documenting the cognitive benefits of physical exercise. There is also a growing literature on cognitive training that purports significant effects (Willis et al., 2006; Karr et al., 2014; Toril et al., 2014; Wang et al., 2014; Huntley et al., 2015; Train the Brain Consortium, 2017). However, in our view, the claims are less convincing due to problems with reporting, limited generalizability, and other reasons that have been cited in various critiques of the literature and related “brain training” industry (e.g., Owen et al., 2010; Muijden et al., 2012; Redick et al., 2013; Boot and Kramer, 2014; Simons et al., 2016; Zokaei et al., 2016). Indeed, a Cochrane Review of 11 RCTs concluded there was “no indication of any significant benefits from cognitive training” (Bahar-Fuchs et al., 2013, p. 1). Nevertheless, considering some “mixed” results on the potential benefits of cognitive training, and as well as the animal literature on neurobiological impact of cognitive enrichments (reviewed below), there is potential for mental challenge to have its own benefit on cognition. Although perhaps it is most effectively implemented as part of a more naturalistic intervention, as when integrated with physical activity that taps additional neural mechanisms.
One way to examine this possibility further is to evaluate the body of literature that encompasses the cognitive effects of interventions with multiple components (e.g., cognitive training and physical exercise). Often these are referred to as:
• “combined” interventions, wherein the components are administered sequentially/in tandem (e.g., Barnes et al., 2013; Fiatarone et al., 2014; Law et al., 2014; Wang et al., 2014; Rahe et al., 2015; Karssemeijer et al., 2017; Lipardo et al., 2017),which can be contrasted with:
• “dual-task” interventions, wherein components are administered simultaneously, but typically are separate tasks (e.g., reciting numbers backwards while walking; Schaefer and Schumacher, 2011; Coelho et al., 2013b; Forte et al., 2013; Theill et al., 2013; Bherer, 2015; Eggenberger et al., 2015; Yokoyama et al., 2015; Desjardins-Crépeau et al., 2016), which can be further contrasted with:
• interactive interventions, as in exergaming, in which the actions in one realm affect the other (e.g., pedaling and steering controls progress in a virtual world and attainment of goals; Anderson-Hanley et al., 2012; Maillot et al., 2012; González-Palau et al., 2014; Schoene et al., 2014; Bamidis et al., 2015; Styliadis et al., 2015; Eggenberger et al., 2016; Lauenroth et al., 2016; Wang et al., 2016; Stanmore et al., 2017).
Indeed, there is a vast literature on “dual-task” experiments and interventions (Kramer et al., 1985; Hiyamizu et al., 2012; Kramer and Wong et al., 2015; Hosseini et al., 2017), which sometimes focus on the limitations of human abilities to manage two separate (non-interactive tasks) at once (e.g., walking and counting numbers backwards). Traditionally, the aim of dual-task training might focus on interference effects (Al-Yahya et al., 2011) and improving physical outcomes (e.g., decreasing falls) for impaired individuals (e.g., post-stroke), especially while managing dual tasks. More recently, studies have examined cognitive outcomes in dual tasks wherein there is more naturalistic interactivity (as in the cybercycle scenario above; Anderson-Hanley et al., 2012; as well as other interactive modalities such as dance: Foster, 2013; Kattenstroth et al., 2013; Dhami et al., 2015; Schoene et al., 2015; Burzynska et al., 2017; Marquez et al., 2017; Müller et al., 2017; Rehfeld et al., 2017). These recent studies seem to be converging on a similar cognitive-enhancing phenomenon that lends empirical support to the synergistic hypothesis outlined above and in part, elsewhere (Fissler et al., 2013). Recent reviews of the literature on the cognitive benefits of exergaming for older adults conclude the preliminary evidence is promising (Smith et al., 2010; Schoene et al., 2013; Bamidis et al., 2014; Barry et al., 2014; Chao et al., 2014; Ogawa et al., 2016; Zhu et al., 2016; Zilidou et al., 2016), but further research of the effects of these interactive interventions and the impact of specific components (e.g., passive stimulation vs. active cognitive training, aerobic vs. non-aerobic activity, etc.) is necessary.
We have previously hypothesized that the benefits of interactive physical and cognitive exercise may increase with increased mental effort and our lab published a pilot trial as a precursor to this present RCT, the Aerobic and Cognitive Exercise Study pilot (ACES-pilot; Barcelos et al., 2015). The ACES-pilot sought to investigate whether greater cognitive challenge while exergaming would yield differential outcomes in executive function and generalize to everyday functioning. Sixty-four community-dwelling older adults (mean age = 82) were randomly assigned to pedal a stationary bike, while interactively engaging on-screen with: (1) a low cognitive demand task (pedaling a steering along a virtual bike tour, referred to as exer-tour), or (2) a high cognitive demand task (pedaling and steering to chase dragons and score points in a 3D video game, referred to as exer-score). Executive function (indices from Trails, Stroop and Digit Span) was assessed before and after a single-bout and 3-month exercise intervention. A significant group × time interaction after 3 months of exergaming (Stroop; among 20 adherents). Those in the high cognitive demand group performed better than those in the low cognitive dose condition. Self-reported everyday function improved across both exercise conditions. These pilot data indicated that for older adults, cognitive benefit while exergaming increased concomitantly with higher doses of interactive mental challenge (Barcelos et al., 2015). The present study aims to replicate and extend that finding to MCI.
Neurobiological and Neuroimaging Effects of Physical and Cognitive Exercise
It is our hypothesis that interactivity can produce greater cognitive effects due to synergistic processes that compound or magnify the benefit in a non-linear way, perhaps by activation and utilization of neurobiological substrate of the mind-body interface that is evolutionarily adapted for success in naturalistic tasks (in this case, goal-directed motion through 3D space). While theory and research connecting cognitive processes to neurobiological pathways triggered by exercise in humans is expanding rapidly, there are indications of important links with various biomarkers (e.g., brain-derived neurotrophic factor [BDNF], insulin-like growth factor [IFG-1]; Cotman et al., 2007; Knaepen et al., 2010), including expression of microRNA (miRNA) in circulating exosomes (Pusic et al., 2016; Bertoldi et al., 2017), and brain structure and function (e.g., gray and white matter regions: Colcombe et al., 2006; hippocampus; Thomas et al., 2012; Erickson et al., 2015; Sexton et al., 2016). Animal models have more plainly linked certain differential, compounding or synergistic cognitive benefits with neurobiological phenomenon when both physical and mental exercise are provided (e.g., in laboratory mice exposed to enriched environments with physical and/or cognitive challenges; Greenough et al., 1999; Churchill et al., 2002; Olson et al., 2006; Galvan and Bredesen, 2007; Fabel et al., 2009; Voss et al., 2013; Jessberger and Gage, 2014). These studies and others, detail a variety of mechanisms and impacts linking mental and physical exercise to improved neuronal and brain health (e.g., via increased cerebral perfusion, neurogenesis, angiogensis, synaptogenesis; Greenough et al., 1999; Gage, 2002; Trachtenberg et al., 2002; Tsai et al., 2016; Kleemeyer et al., 2017), even detailing specific differential benefits (Black et al., 1990; Olson et al., 2006; Suo et al., 2016) such as cell proliferation with physical exercise, and cell survival with mental exercise (Van Praag et al., 2005; van Praag, 2008).
The Present Research
Since only a tiny fraction of older adults exercise at levels recommended by the ACSM and American Heart Association (AHA; Chodzko-Zajko et al., 2009), and physician recommendation appears to do little to change sedentary behaviors (Grandes et al., 2009), the novel exergame utilized in this study holds the promise of increasing compliance by virtue of the distracting components of the virtual reality environment and the interactive and challenging features of the videogame. Besides being a potentially effective way of engaging older adults in exercise, the present RCT augments prior research by exploring the neuropsychological benefits of interactive cognitive and physical training combined, rather than focusing on either alone (Colcombe and Kramer, 2003; Anguera et al., 2013), or in tandem, but lacking interactivity (Fabre et al., 2002; Oswald et al., 2006; Kraft, 2012; Suzuki et al., 2012; Hotting and Roder, 2013; Shatil, 2013; González-Palau et al., 2014; Satoh et al., 2014; Shah et al., 2014; Suo et al., 2016). By examining the neuropsychological effects some of the components separately (e.g., physical exercise and mental exercise alone), this study attempts to tease apart the unique contributions of each component to any benefit to cognition, as well as the potentially differential effect of low- vs. high-cognitive demands. The present research specifically examines the benefits of these behavioral interventions for community-dwelling older adults with or at risk for MCI and thus, attempts to extend previous findings to this more vulnerable population.
Finally, this RCT examines potential underlying mechanisms of effects of combined physical and mental exercise on cognition through the collection of neurobiological and neuroimaging data. Prior research has begun to clarify the role a number of biomarkers (primarily from serum, CNS, or saliva) that change in accord with exercise interventions and appear to be linked improvements in brain health that and/or promote improvements in cognition. For example, exercise has been shown to yield: increases in Brain-Derived Neurotrophic Factor (BDNF; Cotman et al., 2007; Coelho et al., 2013a; Leckie et al., 2014; Vaughan et al., 2014; Dinoff et al., 2016; Maass et al., 2016) and variable responses of insulin-like growth factor 1 (IGF-1; Lorens-Martín et al., 2009). Research linking traditional physical exercise and cognition in older adults has identified BDNF and IGF-1 as biomarkers linking cognitive function and healthy aging (Voss et al., 2010; Bellar et al., 2011; Erickson et al., 2012; Hotting and Roder, 2013; Phillips et al., 2014; Szuhany et al., 2014; Geerlings et al., 2015; Lara et al., 2015; Maass et al., 2016). BDNF plays an important role in neuronal growth and survival, it modulates neurotransmitters, and is involved in the cascade to promote neuronal plasticity, while IGF-1 has also been implicated as a mechanism in exercise-induced neuronal plasticity (Voss et al., 2010; Brown et al., 2013; Phillips et al., 2015; Maass et al., 2016). Cotman et al. (2007) has presented a model in which exercise induces activity of BDNF and IGF-1 in multiple pathways that facilitate: (a) learning, (b) neurogenesis, and (c) angiogenesis. Furthermore, some research has found increases in vascular endothelial growth factor with exercise (VEGF; Vital et al., 2014). While growth factors are typically said to increase with exercise, there are number of reports of decreases in inflammatory markers: C-reactive protein and Interleukin-6 (CRP and IL6; Thielen et al., 2016; Monteiro-Junior et al., 2017). Additionally, recent progressive research has also been examining the potential for circulating exosomes to serve as early markers of neuropathology, such as AD, to act as delivery vehicles for potentially reparative miRNA expressions, and even to play a positive role the neurbiological effects of exercise (e.g., Zhang et al., 2014; Kumar and Reddy, 2016; Pusic et al., 2016; Batistela et al., 2017; Bertoldi et al., 2017). Some promising research has pointed to miRNA-9 and miRNA-193 as linked to benefit in neurobiological processes such that they play a therapeutic role in dementia (e.g., Russell et al., 2013; Párrizas et al., 2015; Li et al., 2016; Riancho et al., 2017).
In sum, exercise appears to be able to trigger or enhance the function of complex cascades of neurobiological processes that have sometimes been linked to cognitive benefits or other expressions of brain or CNS health. It is likely that a number of those processes act on neurophysiological substrate to foster improved brain health that leads to improved cognition; some of those mediating phenonmena may even be visible through structural imaging.
Structural changes in certain regions of the brain have been found to follow exercise and are often linked to concomitant changes in cognition; for example, increases in the hippocampus (Lorens-Martín et al., 2009; Erickson et al., 2011), as well as the anterior cingulate cortex, dorsal lateral prefrontal cortex, and more broadly the prefrontal cortex (ACC, DLPC, and PFC; Gordon et al., 2008; Erickson et al., 2011; Weinstein et al., 2012; Curlik and Shors, 2013; Hayes et al., 2013; Nishiguchi et al., 2015; ten Brinke et al., 2015; Jonasson et al., 2017; Li et al., 2017). While the body of research has been growing with respect to linking physical exercise and cognition via biomarkers, scant literature has yet explored how these indicators and mechanisms react in the case of combined or interactive mental and physical exercise interventions in humans, wherein there might be somewhat differential or compounding beneficial effects given a two-for-one intervention (for a promising exception, see Eggenberger et al., 2016). The present study aims to address a number of these gaps and replicate or extend other findings in the literature.
Hypotheses
This study is a partial replication and extension of the ACES-pilot (Barcelos et al., 2015) and it was hypothesized that, similar to that which was found in that prior pilot study: physical exercise interactive with effortful cognitive challenge (exer-score; Figure 2), would produce greater cognitive benefit than physical exercise that was interactive with relatively passive cognitive processing (exer-tour). We expected this previously observed phenomenon to extend to MCI specifically. The primary aim of the trial was for community-dwelling older adults with or at risk for MCI to engage their assigned intervention regularly for 6 months (longer than the ACES-pilot, which extended only to 3 months). Baseline to 6-month intervention (exer-tour vs. exer-score) were hypothesized to yield these effects:
1. executive function would increase significantly after either exer-score and exer-tour (within subjects main effect)
2. executive function would increase significantly more for exer-score (high mental challenge) than for exer-tour (low mental challenge; between subjects interaction effect)
3. effects of either exer-score or exer-tour would exceed that of game-only
a. additionally, a comparison would be made with archival/normative exercise-only data (available only for a 3-month window, this trial's midpoint)
4. salivary biomarker changes expected to be correlated with improved cognition and exercise dose included:
a. increased growth factors: BDNF, IGF-1, and VEGF
b. decreased inflammatory markers: CRP and IL-6
c. increased exosomal expression of miRNA-193 and miRNA-9
5. neuroimaging changes expected to be associated with improved cognition and exercise dose included:
a. increased gray matter volume in the ACC, DLPFC, hippocampus, and PFC.
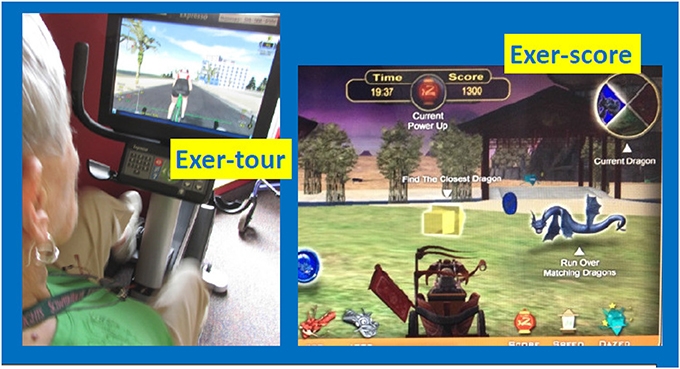
Figure 2. Exer-tour (relatively cognitively passive) vs. Exer-score (cognitively effortful). Exer-tour (pedaling controls speed on screen and progress along scenic bike paths; involves steering, but relatively passive compared to exer-score; for example, can't leave road or crash into anything or any rider which one can steer through; could cease steering without consequence other than tilted view, bike will follow curb). Exer-score (requires navigating in 360° radius to locate colored coins and matching colored dragons of varying speed/difficulty to steer through; the goal is to score points and strategy may be employed to avoid losing points by avoiding hazards, while also seeking out bonus points available via tagging specialized objects one can choose to explore). Former study participant demonstrating exer-tour condition; used with permission.
Methods
Participants
All study procedures were approved by the appropriate Institutional Review Boards (i.e., including the PI's institution and the three medical centers where the study was conducted; see section Author Notes). Older adults with a diagnosis of MCI were initially sought via referrals from neurology and neuropsychology at three medical centers in a specific region of upstate New York where participants were to exercise (using a cybercyle placed at each center's physical therapy clinic). However, given difficulties enrolling patients (especially due to travel requirements, but also due to challenges targeting patients with MCI before progression to dementia), further IRB approval was obtained to expand recruitment by locating additional cybercycles at several more locations around the region (e.g., retirement communities, YMCAs, etc.) for a total of 14 sites. Enrollment was thus expanded to include self-referred community-dwelling older adults, aiming to include especially those that might be undiagnosed (because symptoms had not affected function), but yet who might meet criteria for, or could be said to be at risk for MCI (e.g., thus those that would meet screening criteria for MCI, herein referred to as “screened as MCI” or sMCI2). Participants were recruited using fliers, newspaper ads, and information sessions. The timeline for the conduct of the study was 2014–2016. Volunteers (n = 220; see CONSORT Figure 3 for details) were screened by phone and were excluded if they had known neurologic disorders (e.g., Alzheimer's, Parkinson's, or seizures) or functional limitations that would restrict participation in cognitive testing or exercise. After reviewing the informed consent form (ICF) and answering all questions, a signed document was obtained from all participants and their surrogate, if appropriate, based on the Impaired Decision Making Capacity screen (IDMC; Karlawish, 2008). Physician approval (PCP and if applicable, cardiologist) was obtained for all participants to engage in exercise as assigned. Enrollees (n = 111) were community-dwelling older adults with a mean age of 78.1 (SD = 9.9), who were predominantly female (66%), well educated (average years of education = 16.2, SD = 2.4) Causasians (three individuals self-reported minority ethnic/racial status). Participants scored on average 23.7 (SD = 3.1) on the Montreal Cognitive Assessment (MoCA; 30-point multidimensional scale, with higher scores indicative of better global cognitive function; Nasreddine et al., 2005; Freitas et al., 2012; Julayanont et al., 2014), such that 75% of enrollees were categorized at meeting screening criteria for sMCI (“screened as MCI” based on MoCA < 26; Nasreddine et al., 2005).
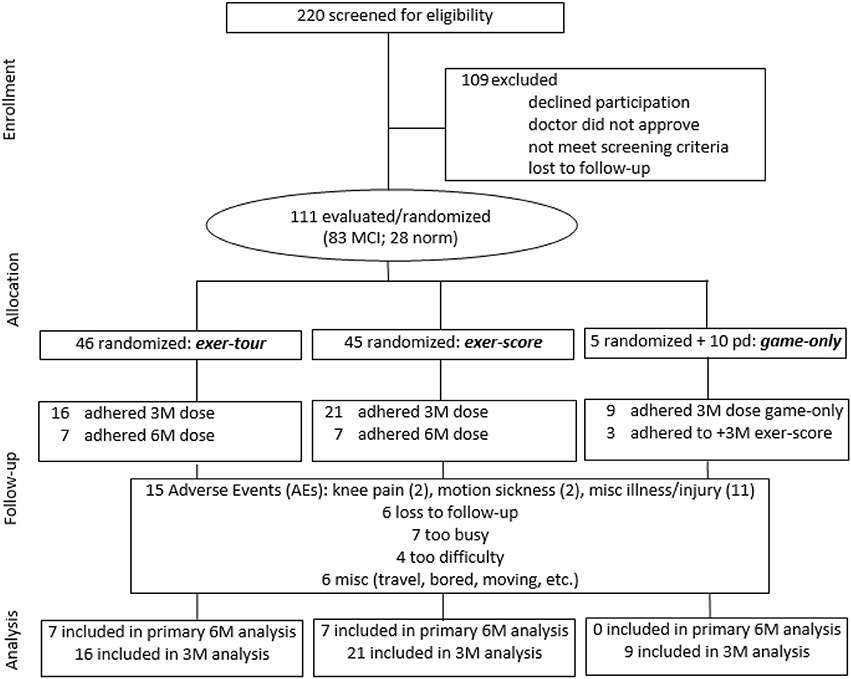
Figure 3. CONSORT flow diagram showing participant enrollment, randomization, and status in trial and analysis.
To provide an additional point of comparison of the relative effect of physical exercise alone vs. interactively combined with mental exercise, archival data was culled from our prior Cybercycle Study. In that study, 33 participants were randomly assigned to a traditional exercise or “pedal-only” condition in which participants pedaled a stationary bike (in many cases the same model as used in the present study), but without the virtual reality display (see below for between group comparisons at baseline; for additional details see Anderson-Hanley et al., 2012, 2014; Dimitrova et al., 2016).
Baseline Comparisons Between Groups
Between the three randomly assigned groups in the present study (exer-tour, exer-score, and game-only; n = 46, 45, 20, respectively), cognitive measures revealed that the groups were comparable (no significant differences) on: overall cognitive function (MoCA), rate of sMCI, and performance on individual tests of cognition including: executive function, visual spatial skill, and memory (verbal, nonverbal, list, story, immediate or delayed).
On behavioral measures, those who were in the game-only condition were somewhat less motivated to be in the study, were not as ready for exercise, and reported they were more sedentary (recall that some participants were randomly assigned to game-only, but others were recruited specifically to the condition, including with remuneration, due to a high dropout rate once randomly assigned participants learned they would not be engaging in a physical exercise component). However, self-reported cognitive engagement (both recent and lifetime), was similar across all three groups.
Baseline comparisons between the four groups, including the above three, but also a pedal-only condition (archival control group data from our prior study noted above; n = 33), revealed that all four groups were similar (no significant differences) on: the three primary outcome measures of executive function to be the focus of our study (i.e., ratio scores for Digits, Trails, and Stroop; see below for details). The four groups were also comparable in proportion of men and women and BMI, but the pedal-only condition was significantly older and had fewer years of education3 (confirming use of age and education covariates in statistical analyses).
Procedures
Participants were initially randomly assigned to one of three conditions for 6 months: (1) exer-tour: physical exercise interactive with relatively passive, low cognitive load, virtual scenic bike tour; or (2) exer-score: physical exercise interactive with a relatively effortful, high cognitive demand, videogame (see Figure 2 for sample screen shots); or (3) game-only: the same videogame operated by a joystick or keyboard (no physical exercise was required). Exercise participants pedaled a virtual reality enhanced, recumbent stationary bike (Expresso S3R, from Interactive Fitness Holdings, LLC). Individuals in the exer-tour condition pedaled through various virtual scenic bike tours while steering along the path (but without significant consequence for not steering; that is the bike would coast along the curb and one could pass through other riders fluidly). Those in the exer-score condition pedaled through a scenic landscape where the goal was to score points by collecting different colored coins and corresponding colored dragons. Throughout the videogame (either exer-score or game-only), participants could navigate through bonus items to increase their speed and score, while avoiding penalties (e.g., steering into water). The exer-tour condition was presumed to require less mental engagement than the videogame conditions, as the game called for scoring and thus, potentially effortful planning, tracking, multi-tasking, and strategizing (a validity check of this assumption was confirmed by those in the exer-score condition who reported expending significantly greater “mental effort” than those in the exer-score condition; see Table 1). Participants were provided “thank you gifts” (e.g., water- bottle, mug, etc.) at each of evaluation (baseline, 3-month, and 6-month).
Given difficulty retaining participants in the game-only condition (due to preference for active/physical exercise, boredom once started, etc.), the protocol was again adjusted, with IRB approval, to allow randomly assigned game-only participants to switch to the exer-score condition at the 3-month mid-point evaluation. Additionally, game-only participants were also recruited separately with modest periodic remuneration provided. Upon enrollment, participants were phone screened for eligibility and administered the Impaired Decision-Making Capacity structured interview (IDMC; Veterans Administration Medical Center (VAMC), 2007) and provided informed consent (co-signed by a surrogate or legally-authorized representative as applicable and/or per the IDMC). Once scheduled for an initial evaluation, participants were mailed the ICF, demographic, health and fitness history questionnaires. If they were willing, an MRI (3T) of the brain was scheduled. At the baseline evaluation, a saliva sample was collected (passive drool), and they were administered a neuropsychological test battery (specified below). Individuals were trained in the use of the equipment for their assigned condition and underwent a single bout of exercise for 20 min. Participants were asked to maintain a target heart rate level, which was calculated using the Karvonen equation (McAuley et al., 2011) and measured via steering hand-grips built into the cybercycle (displayed on screen for participant monitoring and captured by onboard computer for later data extraction and analysis). Post-testing was conducted following the single bout4 (SB) of exercise.
Following the baseline (BL) evaluation, individuals were instructed to exercise for at least 20 min at least twice a week, and to gradually increase exercise duration to 45 min and frequency to at least three to five times per week until the 3-month (3M) mid-point evaluation, and then maintain that pattern though a 6-month (6M) final evaluation. Participants were instructed to aim toward their individualized specific target heart rate (per above) throughout the intervention period. Participants were also asked to document their exercise sessions on log pages provided in a binder and used to calculate adherence statistics (e.g., frequency, heart rate average, etc.); additionally, the computer onboard the cybercycle captured similar data which was used for spot verification. A final evaluation, including repeat neuropsychological, biomarker, and neuroimaging assessments, was conducted at the end of the 6-month intervention period.
Measures
Primary Measures
Cognitive Function
Paper and pencil tests comprised the battery and alternate forms of cognitive tests were utilized at each evaluation to minimize practice/learning effects from serial testing. Cognitive testing was done at baseline (BL) before starting the intervention, after the 20-min training single-bout session, at a 3-month (3M) mid-point, and after concluding the 6-month (6M) intervention period.
Executive Function (Primary Outcome)
Three tests were selected to assess aspects of executive function, which has been shown to be a complex, yet overarching domain of cognitive function. The following three tests were chosen as they are thought to tap overlapping, yet distinct components of executive function, particularly mental flexibility (i.e., Stroop for inhibition, Trails for set shifting, and Digit Span for working memory; Wecker et al., 2000; Strauss et al., 2006). Executive function is critical cognitive function for maintaining independence in later life and thus an important focus of this study's intervention efforts (Pereira et al., 2008; Marshall et al., 2011).
Stroop (Van der Elst et al., 2006)
A short, 40-item version of Stroop was administered. Colored squares (red, green, blue) were presented in rows first (Stroop A), followed by those color words typed in black ink (Stroop B), followed by incongruent color words (Stroop C; in which participants were asked to name the color of the ink while ignoring the written word). A ratio was computed to isolate the executive function component of the task (Stroop A/C; Lansbergen et al., 2007). Among older adults, the ratio score has reasonable test-retest reliability (0.68) over 1–2 months (Ettenhofer et al., 2006) and strong test-retest reliability (0.80) over 2 weeks using the shortened version used herein (Houx et al., 2002). Higher ratios indicate better executive function.
Color trails (D'Elia et al., 1996)
Color Trails 1, requires participants to connect numbered circles in ascending order. Color Trails 2, requires individuals to connect numbered circles in consecutive order while also alternating the color of the circle (pink or yellow). Following the pattern set by Lansbergen et al. (2007) above of isolating the executive function component by dividing basic and faster processing speed by the slower interference trial, a ratio score (Trails1/2) was computed. Reliability and validity are adequate (D'Elia et al., 1996). Higher ratios represent better executive function.
Digit span (Strauss et al., 2006)
Digit Span Forward requires participants to first listen to a list of numbers and repeat them, with the string length increasing to the maximum of their ability. Digit Span Backward, requires repeating a string of numbers in reverse order. Continuing the pattern above to isolate the executive function component, the ratio of the typically smaller sum of correct interference trials on Digit Span Backward, divided by the typically greater sum of correct basic attention trials on Digit Span Forward (DigitsB/F). Good reliability and validity have been reported for Digit Span (Strauss et al., 2006). An increase in the ratio was the desired outcome.
Secondary Measures (Characterization of Sample and Possible Additional Outcomes)
Montreal Cognitive Assessment (MoCA; Nasreddine et al., 2005)
The MoCA was administered at baseline to characterize the sample as either normative aging or “screened as MCI” (sMCI; see details above). The MoCA consists of eight different subtests to assess overall cognitive impairment. Scores below 26 out of 30 were used to categorize sMCI (Nasreddine et al., 2005).
Ecological Validity (EV; Self-Reported Cognitive Function; Klusmann et al., 2010)
In an attempt to measure any generalized, clinically relevant, effects of the interventions (beyond any training to the tests that might occur when using standardized, serial testing), a participant self-report of perceived cognitive function was utilized. Participants were asked about their self-perceptions and beliefs related to their everyday functioning in regards to their cognitive functioning (e.g., memory and concentration). The Ecological Validity Questionnaire (Klusmann et al., 2010) was administered at BL, 3M and 6M. This measure consists of eleven statements that ask about participants perceptions of memory, concentration, and everyday function. Using a 5-point Likert scale (1 = absolutely wrong / bad to 5 = absolutely true / good), participants rated how much each of the statements described their behavior over the past 2 weeks. The total score can range from 11 to 55, with higher scores representing perceptions of better cognitive functioning.
Verbal Memory (Alzheimer's Disease Assessment Scale; ADAS Word List—Immediate and Delayed Recall; Harrison et al., 2007; Podhorna et al., 2016).
Participants are shown a list of 11 words on cards and they recall as many as they are able immediately and also after a delay interval. The number of errors/omissions comprises the score, so lower scores are better.
Get-up-and-go Test (Podsiadlo and Richardson, 1991)
Participants rise from sitting, walk 10 feet, turn around and return to sitting position. The time it takes to complete the task is the score. Lower scores are better.
Other Measures Include
The Physical Activity Readiness Questionnaire (PAR-Q; 0–7, higher scores indicates barriers to readiness; NASM) and Self-reported Physical Activity (SRPA); mental and physical effort thermometers; Exercise Induced Feeling Inventory (EIFI); the Confusion and Vigor subscales of the Brunel Mood Scale (Terry et al., 2003); experience of Flow (Payne et al., 2011), and other neuropsychological measures (e.g., Rey-O, NAART, etc.) not expected to be affected by the intervention, but included for separate use in full characterization of enrollees for later cross-sectional analyses. Due to the focus of this report on a priori hypotheses, and that the small adherent sample available for final analysis and circumscribes statistical power, some of these measures will be reported separately in cross-sectional or other limited analysis.
Exploratory Measures
The following biomarker and neuroimaging data were collected from some willing and able participants, who comprised a small subset of the enrolled participants. Due to the small and incomplete sample, we report these as pilot data to examine comparisons that were planned a priori and provide tentative findings, which may be useful in directing future research.
Biomarkers
Saliva samples (passive drool per Salimetrics protocol) were collected at: BL, SB, 3M, and 6M from willing and able participants. Samples were centrifuged, with a portion reserved for protein analyses and a portion further processed for exosome analyses. Exosomes were isolated from whole saliva via differential ultracentrifugation as described in previously published protocols (Thery et al., 2006; Gallo and Alevizos, 2013; Witwer et al., 2013). Briefly, whole saliva was subjected to differential centrifugation steps at 300 × g, 1,500 × g, 17,000 × g, and 160,000 × g. The exosome pellet was suspended in 1X PBS. All samples were stored at −80°C until analyses were conducted.
Protein Assays
Frozen samples were shipped overnight on dry ice for analysis (to RayBiotech, Inc., Norcross, GA). Protein concentrations of BDNF, CRP, IGF-1, IL-6, and VGEF were obtained via ELISAs (e.g., Mandel et al., 2011). Each patient sample was run in duplicate using 100 ml of plasma diluted by a factor of 2. A commercial multiplexed sandwich ELSIA-based array was used (Quantibody custom array, RayBiotech Inc., Norcross, GA, USA). All of the samples were tested using a panel of cytokines per above. The antibody array is a glass-chip-based multiplexed sandwich ELISA system designed to determine the concentrations all cytokines simultaneously. One standard glass slide was spotted with 16 wells of identical biomarker antibody arrays. Each antibody, together with the positive and negative control, was arrayed in quadruplicate. The samples and standards were added to the wells of the chip array and incubated for 3 h at 4 uC. This was followed by three to four washing steps and the addition of primary antibody and HRP-conjugated streptavidin to the wells. The signals (Cy3 wavelengths: 555 nm excitation, 655 nm emission) were scanned and extracted with an Innopsys laser scanner (Innopsys, Carbonne France), and quantified using Quantibody Analyzer software (Ray Biotech Inc). Each signal was identified by its spot location. The scanner software calculated background signals automatically. Concentration levels, expressed in picograms per milliliter (pg/ml), were calculated against a standard curve set for each biomarker from the positive and negative controls.
Exosome Analyses
Frozen samples were shipped overnight on dry ice for analysis (to VY at Oklahoma State University). The exosome/PBS solution was lyophilized for 9 h and RNA extraction was performed using Trizol™ reagent (Life Technologies®, catalog # 15596026.PPS) following the manufacturer's protocol. A fixed volume of 1,000 ng RNA was reverse-transcribed using the High Capacity cDNA Reverse Transcription Kit (Appled Biosystems, catalog #: 4368814). PCR forward and reverse primers for the human miRNA-193 (forward: CTTTTGGAGGCTGTGGTCTCAGAATC; reverse: CCAGTTGGATAAAACATAAACTCATCTCGCC) and miRNA-9 (forward: AGGCGGGGTTGGTTGTTATCTTTG; reverse: CTAGCTTTATGAAGACTCCACACCACTCATAC) were used for real-time quantitative RT-PCR (qRT-PCR) amplification. miRNA-193 and miRNA-9 expression was normalized against the U6 housekeeping miRNA (forward: GTGCCTGCTTCGGCAGC; reverse: TATGGAACGCTTCACGAATTTGCGTG).
The real-time qRT-PCR was performed using SYBR® Select Master Mix (ThermoFisher, cat #: 4472919). 100 ng of cDNA was loaded per well and amplified for 45 cycles using Opticon® 2 real-time PCR instrument (Biorad, model #: CFB-3220) operated by Opticon Monitor 3 software. All samples and references were run in triplicate and each well contained 20 μL total volume. Raw florescence readings were directly imported into, and baseline corrected with, LinregPCR software package (Version 2016.1). Linear regression was performed on the baseline-corrected data to calculate efficiency using a common window-of-linearity for each primer pair (Ruijter et al., 2009). Between-session variations were estimated using a maximum likelihood approach (Ruijter et al., 2006) and inter-plate variability was minimized using factor correction calculated using Factor qPCR software (Version 2016.0). Taking into account the reaction efficiency of each primer set, we used REST 2009 software package (Version 2.0.13, QIAGEN, Valencia, CA, USA) to calculate the relative RNA expression ratios in samples by a Pair Wise Fixed Reallocation Randomization model with 8000 iterations and a combination of randomization and bootstrapping techniques (Pfaffl et al., 2002).
Neuroimaging
Brain imaging T1-weighted 3D MP-RAGE axial images were acquired with a Siemens MAGNETOM Verio 3T MRI scanner running syngo MR B19 software, using an 32-channel bodycoil using the following parameters: 0.5 × 0.5 × 1.3 mm3 voxel size; 256 × 256 matrix; 25 cm field of view; flip angle = 8°; echo time = 2.9 ms; repetition time = 1,640 ms; inversion time = 828 ms. Gray matter (GM) volume was analyzed using FreeSurfer software v. 5.3.05 (Fischl et al., 2002), in a Linux environment, as described in detail previously (Destrieux et al., 2010). Briefly, cortical reconstruction and volumetric segmentation require the initial motion correction and averaging of T1 images, signal intensity normalization, the removal of non-brain tissue, automated Talairach transformation and segmentation of gray and white matter6, and the parcellation of the cortex into well-described units, using gyral and sulcal landmarks for automatic differentiation. Each segmented brain volume was then inspected visually for processing errors which can occur during automation, and these were corrected using manual edits and re-processed. The Longitudinal Stream pipeline, available in Freesurfer v.5.3.0 (see website7), was used to create an unbiased within-subject template for the two timepoints in the present study (Reuter et al., 2012). Moreover, we expect good reliability and a minimal risk of bias-introduction with the automated pipeline, given that the within-subject scans took place in the same scanner using the same parameters and our focus was on cortical ROIs, which show somewhat better scan-rescan reliability than subcortical structures (e.g., Mills and Tamnes, 2014).
Given the literature cited above and a prioi hypotheses, the regions-of-interest (ROIs) included: hippocampus, dorsolateral prefrontal cortex (DLPFC), prefrontal cortex (PFC) and anterior cingulate cortex (ACC)8. Freesurfer does not directly extract these ROIs as they are not singular regions of the brain (see discussion above as to why they are structurally and functionally significant in the present context). As such, we defined our ROIs by combining the following Freesurfer labels: DLPFC = frontal middle gyrus and sulcus from the Destrieux et al. (2010) atlas; ACC = rostral and caudal anterior cingulate cortices from the Desikan et al. (2006) atlas; PFC = ACC + medial orbitofrontal and transverse frontopolar regions (Domenech and Koechlin, 2015). The DLPFC ROI that was chosen is one of the most conservative and non-controversial groupings, although some others have included more medial and/or caudal regions in their work (Vijayakumar et al., 2014; Jonasson et al., 2017). The volumes extracted are a measure of total gray matter only (white matter was parcellated separately and excluded). For the final analysis, GM volumes of each region were extracted and considered as a ratio to total intracranial volume to account for variations in brain size. Given the small sample size, volumetric ratios were used in parametric partial correlations with cognitive outcomes (controlling for age and sex).
Statistical Analyses
Data were analyzed using SPSS version 23 for Windows (IBM Corporation). The primary goal of the RCT was to examine, for those with sMCI, the possible differential effects (via neuropsychological, neurobiological, and neuroimaging measures) of long-term use of different combinations of physical and cognitive exercise (exer-tour vs. exer-score). Analyses focused on those sMCI participants that adhered to the protocol (approximating the minimum prescribed dose by averaging 3 × /wk for 6 months, allowing for 2 weeks of vacation, illness, or equipment failure, in each of the 3-month evaluation windows). Given the small sample of completers, there was concern about insufficient power since a priori power analysis suggested a larger sample was indicated (Faul et al., 2007). Additionally, small samples often violate assumptions of standard parametric tests. However, the data for the primary outcome variables was found to be normally distributed (Shapiro-Wilk tests for StroopA/C, Trails1/2, and DigF/B were 0.52, 0.71, 0.16, respectively; Normal Q-Q plots were also reviewed which appeared to be normally distributed, with data following a linear pattern) and error variances were consistent with parametric assumptions (Levine's tests were not significant). Furthermore, given the importance of covariates (more readily implemented in parametric tests), along with the possibility that effects could be magnified in the sMCI sample, and following guidance in the literature on statistical strength of ANCOVA above nonparametric approach, especially in clinical trials (wherein a focus on change from baseline facilitates a trend toward normality; Vickers, 2005), it was decided to proceed with standard parametric analyses. Repeated measure ANOVAs, controlling for age and education were used to examine group (exer-tour vs. exer-score) × time (BL vs. 3M and 6M) interaction effects on the primary outcome measures of executive function. Covariates entered into the repeated measures analyses included: age and education, due to links to outcomes noted in the theoretical and empirical literature (Hannay and Lezak, 2004; Lam et al., 2013). Paired t-tests were used to evaluate within group change from baseline to the 3-month mid-point, and baseline to conclusion of the 6-month intervention.
Partial correlations, controlling for age and sex (based on prior literature demonstrating links to secondary outcomes; e.g., BNDF decreases with age: Lommatzsch et al., 2005; brain region variability with sex: Ruigrok et al., 2014), were conducted to explore the relationship between cognitive change and possible corresponding change in biomarkers (proteins and exosomes) and neuroimaging regions of interest, per above hypotheses. Further correlations were conducted to explore relationships between baseline memory and exercise dose as represented by ride frequency.
Results
Effect On Primary Outcome: Executive Function
Full (6-Month) Intervention (Exer-Tour vs. Exer-Score)
Fourteen participants who met criteria for sMCI (MoCA < 26) were adherent to the protocol approximating the minimum assigned weekly average of 3 × /week over 6 months. Half had been randomized to exer-tour and half to exer-score. After 6 months of interactive physical and cognitive exercise, the repeated measures ANCOVA omnibus test of executive function measures revealed a non-significant group x time interaction effect, F(3, 7) = 0.85, p = 0.51, ηp2 = 0.27. Univariate tests for StroopA/C, Trails1/2, and DigB/F were also not significant (p = 0.82, 0.11, 0.99, respectively). Paired t-tests were conducted to examine the change within groups over 6 months and StroopA/C improved significantly in both the exer-tour, t(1, 5) = −2.6, p = 0.049, and exer-score conditions; t(1, 6) = −5.5; p = 0.001 (Figure 4); Trails1/2 and DigB/F did not change significantly in either group (Table 2). Paired t-tests of the change from baseline to the mid-point (3M) evaluation revealed no significant differences between the exer-tour and exer-score conditions at that point among sMCI participants (n = 14) that were adherent through 6 months (Table 2). sMCI participants who had been assigned to either exer-tour or exer-score and were not adherent over 6 months (n = 54) did not differ significantly from adherent sMCI participants (n = 14) on any baseline variables (including demographics, cognitive, physiological and functional variables; Table 1).
Mid-point (3-Month) Intervention (Exer-Tour vs. Exer-Score vs. Game-Only vs. Pedal-Only)
Given the high level of attrition from the game-only condition, as noted above, a mid-course adjustment was made to enroll game-only participants into a 3M-only intervention window. Doing so provided some comparative data regarding the possible relative effect of the mental exercise component separate from physical exercise (videogame practice only). Additionally, we were able to extract comparative 3M control data from our prior Cybercycle Study regarding the effect of a pedal-only condition for older adults. These 3M analyses utilized all older adults (sMCI + normative9) that were adherent through 3M in their assigned condition (thus the n's for exer-tour, exer-score, game-only, and pedal-only were: 15, 19, 8, 31, respectively). Paired t-tests were conducted and revealed significant gains on StroopA/C by 3M for exer-tour and pedal-only, with statistically significant moderate effects (d = 0.49 and 0.35, respectively; Figure 5), while exer-score and game-only yielded non-significant small effects (d = 0.14 and 0.13, respectively). TrailsA/B and DigF/B did not change significantly. The 6M effect sizes for the two conditions that progressed to 6M (exer-score and exer-tour) are also included in Figure 4 and reveal that both the exer-tour and exer-score conditions yielded statistically significant moderate effects by 6M (d = 0.52 and 0.47, respectively); thus, the exer-score group seems to “catch up” to the exer-tour from 3 to 6M.
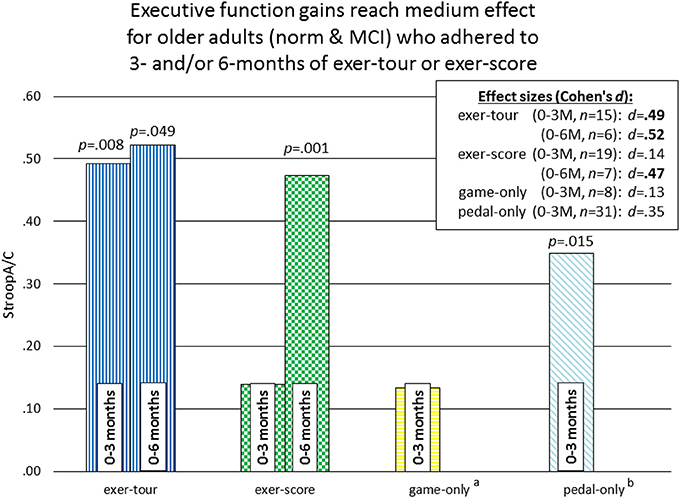
Figure 5. Comparison of effect sizes of components of interactive physical and cognitive exercise across four intervention conditions for 0–3M adherents. p-values represent significant change over time within-group (paired) t-tests. athe game-only condition was initially randomly assigned, but subsequently recruited separately due to challenges with enrollment and retention (see section Methods for further details). bthe pedal-only comparison data is archival/perviously reported from our lab and was obtained during the Cybercycle Study (Anderson-Hanley et al., 2012), which examined only a 3-month interval and was collected from older adults in the same region, from many of the same retirement communities, and with the same measures of executive function; this data is included here as a point of comparaitve reference, illustrating the magnitude of effect from physical exercise alone.
Effect on Secondary Outcomes
Full (6-Month) Intervention (Exer-Tour vs. Exer-Score)
There were significant group x time interactions among the 14 sMCI adherents for: (1) immediate verbal memory (exer-tour improved significantly more than exer-score; p = 0.018), (2) self-report of everyday cognitive function (as assessed on the Ecological Validity Questionnaire), with exer-tour reporting significantly more improvement while exer-score reported decline; p = 0.046), and (3) physical ability (exer-tour increased significantly more than exer-score; p = 0.001; see Table 3).
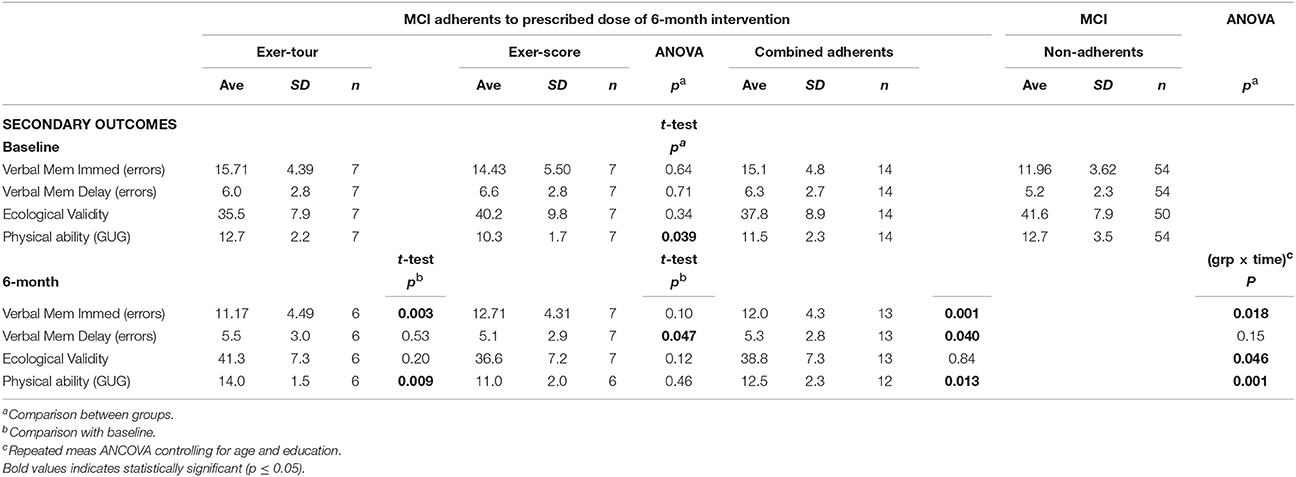
Table 3. Exer-tour vs. exer-score for secondary outcomes: memory, everyday function (self-reported cognition and physical ability (0–6M).
Biomarkers10
Biomarker samples were sought from all participants, but not all were willing or able to provide saliva samples (data from completers was available from a maximum of n = 16 for analysis).11 Target analytes included: BDNF, CRP, IGF-1, IL-6, and VEGF. Due to low concentrations of IGF-1 in the samples collected, the assay results were below the limit of detection on too many samples and this assay was excluded from analyses. Partial correlations (controlling for age and sex; Table 4) were conducted to examine the change from baseline to 6M in biomarkers related to change in the primary outcome measure, executive function (StroopA/C) which, per above, changed significantly with the intervention. Similarly, change in biomarkers were correlated with a secondary outcome that was impacted significantly by both interventions, was verbal memory, and we chose to focus on delayed verbal memory specifically (ADAS errors), since one of the more salient cognitive functions to impact for MCI patients. Finally, the exercise dose, based on average number of rides, was examined for association with the biomarkers change to evaluated the possibility of a dose effect. Exercise dose (rides) was found to correlate significantly with an increase in BDNF (r = 0.50, p = 0.04). Delayed recall errors showed a trend toward a moderate inverse correlation with increased VEGF such that better memory performance tended to coincide with increasing VEGF (r = −0.42, p = 0.08). Exosome analyses revealed a significant positive correlation between miRNA-9 expression with improved StroopA/C performance (r = 0.98, p = 0.002).
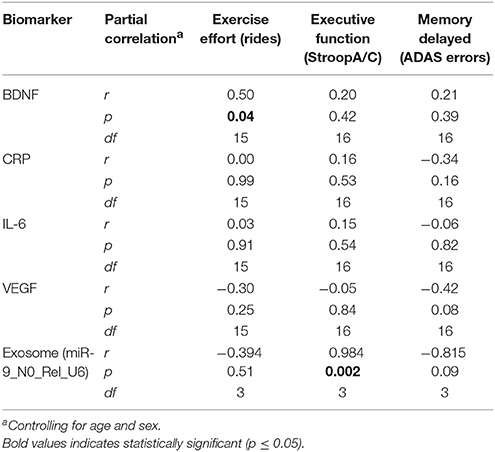
Table 4. Neurobiological change (0–6M) correlates with exercise dose/effort and change in cognitive function for participants in exer-tour and exer-score: Exploratory pilot data.
Neuroimaging7
Neuroimaging (3T structural MRI) was grant-funded and sought from all participants, but not all were willing or able to undergo imaging (data from completers was available from a maximum of n = 8 for analysis). Partial correlations, just as described above, are reported in Table 5. Greater exercise dose (number of rides over 6M) was positively correlated with increasing PFC (r = 0.80, p = 0.003) and ACC (right; r = 0.70, p = 0.05; Figure 6). Verbal memory (delayed recall) errors were inversely related to increasing DLPFC, such that improvement in memory corresponded to increasing volume (r = −0.80, p = 0.01; Figure 6).
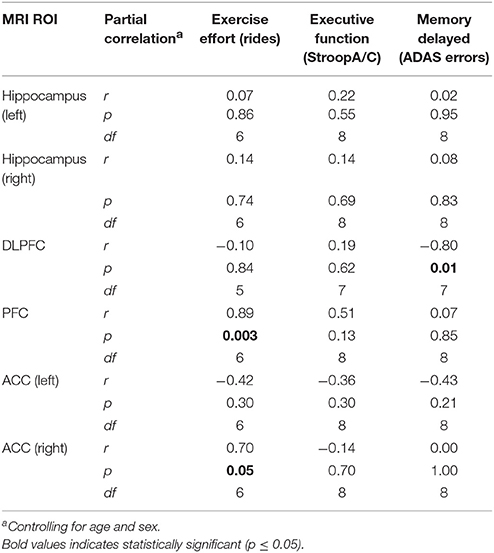
Table 5. Neuroimaging change (0–6M) correlations with exercise dose/effort and changes in cognitive function (0–6M) for participants in exer-tour and exer-score: Exploratory pilot data.
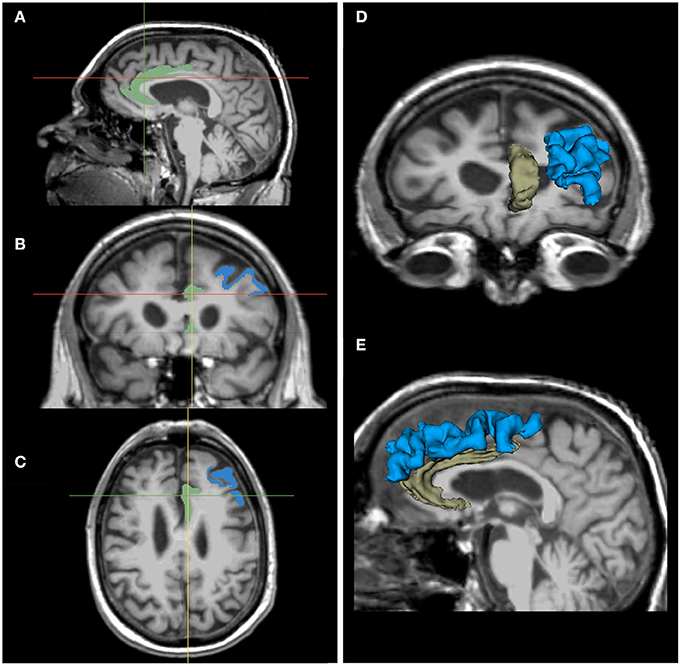
Figure 6. Neuroimaging correlates with exercise and cognition13: MRI of ACC and DLPFC. Notes: Illustration of regions-of-interest (ROI): ACC (increased with exercise dose) and DLPFC (increased with improvement in verbal memory); shown here in one individual and in one hemisphere for ease of presentation. Images of ACC (green) and DLPFC (blue) in 3 sagittal (A), coronal (B), and axial (C) images aligned by crosshairs. ROI 3D model reconstructions give a sense of their structure in 3D space, as seen from the anterior (D) and left (E) sides.
Discussion
This RCT was an attempt to replicate and extend findings of prior research, which found greater cognitive benefit for older adults engaged in interactive mental and physical exercise (i.e., while exergaming using a cybercycle; Anderson-Hanley et al., 2012) and furthermore, given pilot data which suggested greater mental challenge may increase cognitive benefit of exergaming (Barcelos et al., 2015). This RCT examined whether similar effects would be found among community-dwelling older adults with or at risk for MCI (screened as MCI; sMCI). We hypothesized that a more mentally challenging exergame would yield greater benefit to executive function than a more passive exergame. Community dwelling older adults, with a focus on those with sMCI, were randomly assigned to: exer-tour (pedaling along a virtual bike path), exer-score (pedaling to score points by navigating an interactive videogame), or a game-only condition (the latter having to be adapted due to issues with participant adherence, resulting in a shorter and remunerated, quasi-experimental arm).
Fourteen older adults with sMCI were adherent to the minimum assigned dose (average 3 × /wk of exercise) for 6 months. Both conditions were found to yield significant improvement on one of three measures of executive function (Stroop A/C), producing similar moderate effect sizes at 6 months (d = 0.52 and 0.47, respectively). There was no interaction effect; however, from informal examination of the graph of the data (Figure 4), it appears that the exer-score condition did not produce incremental gains at the mid-point (3M), while the exer-tour condition did appear to yield benefit sooner, although by 6 months the exer-score and exer-tour gains were similar. It is hypothesized that since the exer-score condition does require significant mental effort to play, it may have taken longer for exer-score participants (with sMCI) to master the interactive pedaling and game-play. Thus, there may have been delay in triggering the synergistic effects of the interactive exercise that would lead to a neurobiological cascade of events ultimately linked to any cognitive benefit. Furthermore, it may be different brain networks are activated by various aspects of the interactive physical and mental tasks in each condition, thus resulting in differential enhancement of network functions; for example, the effect on Stroop herein is consistent with activation of the fronto-parietal network used in inhibition and switching and the fact that the volume of the ACC as shown on MRI imaging was enlarged proportionally to exercise dose further lends support to the impact on that specific network (Grandjean et al., 2012).
The differential relative impact of the two forms of interactive physical and cognitive exercise, exer-tour and exer-score, was apparent when comparing mid-point (3M) moderate effect with small effect (d = 0.52 and 0.14, respectively), while the game-only condition showed little impact (i.e., of cognitive training; d = 0.13). By contrast, the pedal-only condition by 3M was more consistent with the literature on the benefits of physical exercise alone for cognition as it yielded a significant within-group improvement (Colcombe and Kramer, 2003), although it was a more modest effect (d = 0.35) than the exer-tour, which is also consistent with past research in which the cybercycle effect exceeded exercise alone (Anderson-Hanley et al., 2012). It may be that the measures of executive function that were chosen were not as sensitive to the particular impact of each of the conditions as they could be; while Stroop A/C revealed an effect, Trails 2/1 and Digit Span B/F did not, perhaps because the components of executive function were not particularly enhanced (e.g., task switching and working memory, respectively). Future research, could strategically select measures that might better detect change in selective attention or inhibitory control which arguably might be more affected by repeated use of the exer-score condition in which one is chasing dragons while scanning the horizon for additional opportunities and avoiding pitfalls, and so forth (e.g., Flanker or Go/no-Go tasks might have greater sensitivity to detect for specific and relevant changes in executive function; Diamond, 2013).
Analysis of secondary outcomes from the present study yielded promising indications that this type of exergaming activity can yield cognitive gains in other realms of cognition12 (e.g., verbal memory) among individuals with sMCI (with exer-tour outperforming exer-score). Moreover, these effects seem to generalize beyond standardized tests of cognition, to self-reported perceptions of everyday cognitive function and also vigor13 (again, with exer-tour outperforming exer-score). The latter finding may benefit from further probing, perhaps via focused exit interview questions regarding possible reasons for this curious finding. Perhaps the regular practice of a mentally challenging videogame led participants in that condition to subjectively feel less capable (even though by 6M the gains exer-score made in executive function matched that of exer-tour). It is also likely that there would be individual variability in response to the gaming scenarios (e.g., prior research has found that one's trait competitiveness can interact with benefit from presumably facilitative features of an exergame, such as the presence or absence of an avatar (Snyder et al., 2012). Indeed in conduct of this trial, anecdotally, it was noted that some individuals were keenly enthused by and motivated to keep track of their score, while others were lackadaisical in their approach to pedaling around for exercise but without much care for scoring. Indeed, research shows the salience to the individual of the storyboard of a videogame, as well as other features may affect enjoyment or persistence, and can facilitate or limit use (Wang and Goh, 2017); all relevant issues to consider when designing and planning a long-term clinical trial with a goal of ensuring a thorough dose, via consistent adherence.
These findings of significant effects with both types of exergaming: exer-tour (low mental challenge) and exer-score (high mental challenge) on executive function and memory, mirrors one of the few similar studies, by Eggenberger et al. (2015), who reported similar trends when comparing an interactive dance exergame, with dual-task walking (non-interactive), and physical exercise alone. It is interesting to note that effect sizes were larger in our study and thus reached statistical significance, while the trial above had a much larger sample, but the effects were smaller leading to marginal results, despite having exergaming conditions that were in many ways similar. Perhaps this was due to the fact that the sample herein was sMCI, specifically, while the Eggenberger study utilized normative older adults. It seems likely that general, MCI participants have already begun some cognitive decline and so they may have more room to grow, so to speak, or at least more distance from the ceiling on cognitive tests to reveal change when attempting to attenuate the anticipated downward slope of cognitive decline, and thus the effect may be more readily apparent. Future research could further evaluate the possibilities by enrolling and comparing effects of both normative and MCI participants, with care taken to avoid possible ceiling effects on baseline assessments.
Finally, possible mechanisms linking exercise to enhanced cognition via presumed improved brain health were explored with promising findings from pilot data sampled from willing participants. Biomarker changes (e.g., BDNF and exosomes) were found to correspond to improvements in cognition and dose of exercise, each consistent with prior research (e.g., BDNF has been found to promote the differentiation of new neurons and synapses, and therefore, has been proposed to be a mediator of adult neuroplasticity; Huang and Reichardt, 2001; Leßmann and Brigadski, 2009; Flöel et al., 2010; Park and Poo, 2013; Edelmann et al., 2014). Furthermore, neuroimaging revealed promising gains in key ROIs (e.g., DLPFC and ACC) that corresponded with improvements in cognition and dose of exercise, again consistent with reports in the literature (Burdette et al., 2010; Weinstein et al., 2012; Chapman et al., 2013, 2016; Hayes et al., 2013; Ehlers et al., 2017; Li et al., 2017). While we retain a cautious stance for interpreting these findings given the limitations already noted, we are heartened that changes found are largely consistent with changes in gray matter volume that have been noted in other studies of learning and physical training. Some of which have reported statistically significant changes as early as 1–2 weeks (e.g., Ceccarelli et al., 2009; Zatorre et al., 2012) and specifically regarding training-induced changes in older adults before the 6-month time point (e.g., Boyke et al., 2008).
Strengths of this RCT include a study design crafted to clarify whether cognitive benefit could be maximized with a more challenging videogame component integrated with the physical exercise. In contrast to the findings of the ACES-pilot study (Barcelos et al., 2015), which found that after just three months of exer-score resulted in greater cognitive benefit than the exer-tour. The present findings suggest that for a more impaired MCI population the benefit of exer-tour shows up by three months, but it may be necessary for participants to stick with the interactive exercise through a longer window, six months, in order to master the challenges and reap the benefits of the enhanced exercise experience. Additionally, this study design began to tease apart the impact the of component parts of exergaming (e.g., physical exercise only vs. mental exercise only), as well as explore possible neurobiological mechanisms and neuroimaging markers that tentatively shed light and nudge future research regarding the ways in which the body and mind might interact for neuroprotection or amelioration of cognitive decline. A particular strength of this study is the use of alternate forms and additional serial testing (via single bout and 3M evaluation) that preceded the final 6-month evaluation such that practice or learning effects should have largely “washed out” or likely plateaued by that point (Beglinger et al., 2005), thus greater confidence can be placed in the results as representing a genuine effect.
A significant limitation of this study is the difficulty participants experienced in engaging a new behavioral approach to preventing or curbing cognitive decline. Attrition far exceeded our expectations based on past pilots and standard rates noted in the literature. The fact that this study focused on persons with sMCI, who face more than the usual challenges in starting a new exercise regimen, was likely a major contributing factor. Additionally, the requirement set out initially by grant reviewers and medical center IRBs that persons with MCI be considered a vulnerable population and must exercise in a limited environment with professional oversight (i.e., physical therapy clinics) proved daunting as participants were unwilling or unable to arrange transport 3–7 × /wk to engage in regular exercise. Obtaining approval to make the equipment more accessible within retirement communities and neighborhood wellness centers did help somewhat, but nevertheless, retention remained a significant issue. The resultant small sample does diminish statistical power, although despite this, effects were sizeable enough to detect. Additionally, a small sample limits our ability to ask nuanced questions of the dataset which the study design would have facilitated if a larger sample had been obtained. For example, it was an a priori goal to compare known subtypes of MCI (i.e., amnestic vs. not) to see what, if any, impact subtype of MCI might have had on participants' abilities to learn and engage the more challenging exer-score videogame. This will have to wait for a future trial with a larger sample, perhaps by recruiting, as was necessary herein, those yet undiagnosed, but going beyond a screening tool, a future study could prospectively build in the mechanisms for a complete clinical workup of MCI as well. Nevertheless, much was learned regarding the needs of MCI patients and families in the conduct of such a trial, and will enhance future trial designs and interventions (see below). It will remain challenging however, to balance ideals about statistical power with the reality and importance of continuing to conduct research with clinical samples.
Future research is needed to address limitations of this study and improve retention and adherence to these types of behavioral interventions which have promising findings despite the variety of hindrances. For example, to try to address the challenges of enrolling and retaining MCI participants, our lab has been developing a more strategic and tailored in-home approach to facilitate adoption of this type of long-term behavioral intervention. We have developed a portable, adaptive, tablet-based neuro-exergame14, Memory Lane™, specifically designed to target executive function by way of integrating specific mental challenges presented via a relatively simple, in-home interactive Physical and Cognitive Exercise System (iPACES™15). While still only available for research purposes, the design had limited bulkiness of equipment and used budget components such that a large clinical trial will remain feasible (for example, an under-table pedaler paired with a budget tablet). Preliminary results from single bout use of our prototype iPACES™ have been promising (Anderson-Hanley et al., 2017), and we hope these various studies will encourage others to identify, design, and implement novel behavioral interventions to combat and ameliorate cognitive decline. Indeed there are a number of other open protocols currently recruiting to innovative behavioral interventions (Legault et al., 2011; Lee et al., 2016); for example, the Aerobic Exercise and Cognitive Training Trial (ACT) out of the University of Minnesota is enrolling patients with MCI to better understand the benefit of combined physical and mental activities (NCT03313895), and the Multidomain Alzheimer Prevention Trial (MAPT) combines physical and cognitive exercise, while also layering a nutritional component that may be able to further maximize any cognitive benefit derived (Vellas et al., 2014). Some research teams have been pushing the envelope on the possibilities given advancing technologies and have even explored web-connected exergaming to facilitate participation (Bamidis et al., 2011; Konstantinidis et al., 2017). The availability of such innovative interventions through data-yielding clinical trials is heartening to many individuals and families already facing the challenges of cognitive decline.
Given the encroaching dementia epidemic, it behooves researchers and society at large to continue to press for innovative and effective solutions that aim to maximize cognitive benefits. In this way we can hope to make meaningful contributions to the important cause of slowing or preventing the onset of cognitive decline. It is likely that a multi-factorial approach will yield the greatest impact, but there is much work to be done to clarify which components and intensities (e.g., of physical and mental exercise, nutritional and/or social supports, etc.), to meld and leverage for the best and most meaningful impact on brain health so as to ensure sustained cognitive functions in later life. Furthermore, it seems likely that there is a great deal to learn about factors that predict response to any intervention, including physical and mental exercise. It may be that certain genetic factors, such as ApoE status may moderate the body's ability to spark a given neurobiological cascade and thus reap the benefits of a given intervention such as interactive physical and cognitive exercise (Raichlen and Alexander, 2014). The more we learn about these underlying mechanisms and the nuanced effects exposed by analyzing larger samples, the more effective we can be in designing and tailoring interventions (Morrison-Bogorad et al., 2007; Lieberman, 2009; Larson, 2010; Read and Shortell, 2011; Gerling and Mandryk, 2014; Barha et al., 2016) to do the most good for the greatest number of people as fast as we can, especially now that so many are faced with sliding into the long suffering.
Ethics Statement
This study was carried out in accordance with the recommendations of the Institutional Review Board (AMC, SV, VA, and Union College) with written informed consent from all subjects. All subjects gave written informed consent in accordance with the Declaration of Helsinki. The protocol was approved by the IRB.
Author Notes
Thank you to:
• Our participants and site administrators from: Albany Medical Center, Stratton VA Medical Center, Sunnyview Rehabilitation Hospital, Beverwyck, CDPHP Wellness Center, Coburg Village, Glen Eddy, Hawthorne Ridge, Jewish Community Center of Niskayuna, Kimgsway Community, Prestwick Chase, Schaffer Heights, and the YMCA of Saratoga Springs.
• Research assistants: Chris Avanessian, Joel Gideon, Julie Warren, and the NY6 2016 Summer Research Team: Elizabeth Altman, Zachariah Arnold, Amelia Denney, Casey Terzian, Jennifer Vu, and Emily West.
• Colleagues who provided advice and assistance at various stages of this project: Drs. Adam Brickman, Amanda Damjanovic, Michael Hogan, Michael McCann, and Jeffrey Woods.
• Dr. Gaetano Pastena and the clinical team at Community Care Niskayuna and Latham who assisted in collection of 3T MRI imaging.
• Ross Stensrud of Interactive Fitness Holdings for technical assistance with data retrieval from the Expresso bike platforms utilized in this study.
Earlier versions of these results were presented at the annual meetings of: the Society for Neuroscience Chapter, the International Neuropsychological Society, and the American Academy for Clinical Neuropsychology.
Author Contributions
CA-H, NB, MM, VY, DH wrote pieces of the manuscript; CA-H, NB, EZ, RG, MD contributed to study design, implementation and interpretation; BC contributed to biomarker data analysis and interpretation; KM contributed to exosome study design, data analysis and interpretation; DH contributed to MRI data analysis and interpretation; PA contributed to study design, exercise science factors, implementation and interpretation; AK contributed to study design, interpretation.
Funding
This research was funded by a grant from the NIA (1R15AG042109-01A1) with additional support provided by Union College via Faculty and Student Research Grants.
Conflict of Interest Statement
The authors declare that the research was conducted in the absence of any commercial or financial relationships that could be construed as a potential conflict of interest.
The reviewer DM and handling Editor declared their shared affiliation.
Footnotes
1. ^ Exergaming is a subset of health games, sometimes referred to as active games, that combine physical exercise with interactive gaming features, often through a virtual reality-type platform (Van Schaik et al., 2008; Read and Shortell, 2011; Stanmore et al., 2017).
2. ^ Screened as MCI (sMCI) based on a MoCA cut-off score for MCI of < 26 (Nasreddine et al., 2005). This screening approach was utilized after initial planned attempts to enroll clinically diagnosed patients with MCI (e.g., recruited from neurologists, neuropsychologists, and geriatricians, yielded patients that had often already progressed in their decline toward dementia). Indeed, by the time some patients had been referred for clinical evaluations, received a formal diagnosis and subsequently were referred to our study, their disease had progressed such that they no longer fit our target population of MCI. As a result, attempts to enroll such referrals led to exclusion as the patient sometimes would be unable to understand or participate in the research and/or complex intervention procedures.
3. ^ This difference is surmised to be likely due to differences in recruitment as the prior, Cybercycle Study, only included participants exercising at their residential facility, whereas the present study included those that could travel to exercise equipment, often at locations across town.
4. ^ single-bout results are not reported herein in part due to space constraints, but also given the focus of the RCT on long-term intervention results which necessarily analyzes a different/smaller sample of those adherent for 6-months; furthermore the single-bout was not a part of a priori hypotheses in the study design, but rather included to accompany the training session and provide a repeat testing experience/familiarization with tests before the follow-up evaluations (3 M and 6 M) which would partly help “wash out”/reach sooner the plateau of practice/learning effects and thus more plainly isolate intervention effects at the follow-up evaluations; finally, the single-bout assessment provides a consistent pattern with our prior RCTs (the Cybercycle Study and the ACES-pilot) in which similarly a second round of testing before the long-term outcome for similar reasons, as such this increases comparability of our data across trials and time-points (both in terms of amount of familiarization with testing and matching of alternate forms at each time-point).
5. ^ http://surfer.nmr.mgh.harvard.edu/
6. ^ total gray matter volumes were the focus of this study (white matter not considered herein)
7. ^ https://surfer.nmr.mgh.harvard.edu/fswiki/LongitudinalProcessing
8. ^ While the PFC and ACC overlap, they are reported separately here, in an attempt to replicate prior reports in the literature which identified change after exercise in each ROI.
9. ^ The 3M analyses were not limited to sMCI, because added comparison of the fourth group at 3M (the pedal-only condition from the archival dataset; Anderson-Hanley et al., 2012), could not be sorted by the same sMCI criteria used in the present study (MoCA scores were not available for pedal-only participants). Thus, the decision was made to utilize all adherents (normative + sMCI) at 3M from the four groups to retain comparability within the 3M comparisons.
10. ^ Results for bioassay and neuroimaging data analyses are to be interpreted tentatively as exploratory pilot results, given the small sample sizes even with collapsing across conditions.
11. ^ Additional cognitive domains that might be sensible to assess in a follow-up study, might include visuospatial skills, given the integration and regular use of visuospatial tracking in the conditions with virtual reality screens.
12. ^ The two mood subscales (vigor and confusion from the BMS) were probed retrospectively on suggestion of an anonymous reviewer and the ex-tour reported a significantly greater sense of vigor at 6M, than exer-score.
13. ^ As noted above, both ACC and PFC were analyzed in order to replicate prior research which reported each ROI correlated with outcomes (e.g., exercise dose or cognition), but because of the overlap of these ROIs only one is shown here for clarity.
14. ^ Neuro-exergaming (Anderson-Hanley et al., 2016, 2017), is an adaptation of existing terms and used herein to indicate the integration of neurogaming with exergaming for the express purpose of benefiting brain health and fostering improved cognitive function
15. ^ iPACES™ 2013-present, patent pending No. 62/140,991; BSK Ref. No.: 416P1700A
References
Ahlskog, J. E., Geda, Y. E., Graff-Radford, N. R., and Petersen, R. C. (2011). Physical exercise as a preventive or disease-modifying treatment of dementia and brain aging. Mayo Clin. Proc. 86, 876–884. doi: 10.4065/mcp.2011.0252
Alzheimer's Association (2014). Alzheimer's Associationreport: Alzheimer's disease facts and figures. Alzheimer's Demen. 10, 47–92. Available online at: https://www.cdc.gov/aging/pdf/2013-healthy-brain-initiative.pdf
Alzheimer's Association and Centers for Disease Control and Prevention (2013). The Healthy Brain Initiative: The Public Health Road Map for State and National Partnerships, 2013–2018: Chicago, IL: Alzheimer's Association.
Al-Yahya, E., Dawes, H., Smith, L., Dennis, A., Howells, K., and Cockburn, J. (2011). Cognitive motor interference while walking: a systematic review and meta-analysis. Neurosci. Biobehav. Rev. 35, 715–728. doi: 10.1016/j.neubiorev.2010.08.008
Anderson-Hanley, C., Barcelos, B., Maloney, M., Michel, M., Striegnitz, S., and Saulnier, T. (2016). “Neuropsychological effects of an Interactive Physical & Cognitive Exercise System (iPACES) for older adults: pilot comparison of in-home neuro-exergame versus neuro-game,” in Presentation at the Annual Meeting of the International Neuropsychological Society (Boston, MA).
Anderson-Hanley, C., Arciero, P. J., Barcelos, N., Nimon, J., Rocha, T., Thurin, M., et al. (2014). Executive function and self-regulated exergaming adherence among older adults. Front. Hum. Neurosci. 8:989. doi: 10.3389/fnhum.2014.00989
Anderson-Hanley, C., Arciero, P. J., Brickman, A., Nimon, J., Okuma, N., Westen, S., et al. (2012). Exergaming improves older adult cognition: a cluster randomized clinical trial. Am. J. Prev. Med. 42, 109–119. doi: 10.1016/j.amepre.2011.10.016
Anderson-Hanley, C., Maloney, M., Barcelos, N., Striegnitz, K., and Kramer, A. F. (2017). Neuropsychological benefits of neuro-exergaming for older adults: a pilot study of an interactive physical and cognitive exercise system (iPACES™). J. Aging Phys. Activity 25, 73–83. doi: 10.1123/japa.2015-0261
Angevaren, M., Aufdemkampe, G., Verhaar, H. J., Aleman, A., and Vanhees, L. (2008). Physical activity and enhanced fitness to improve cognitive function in older people without known cognitive impairment. Cochrane Database Syst. Rev. 3:CD005381. doi: 10.1002/14651858.CD005381.pub3
Anguera, J. A., Boccanfuso, J., Rintoul, J. L., Al-Hashimi, O., Faraji, F., and Gazzaley, A. (2013). Video game training enhances cognitive control in older adults. Nature 501, 97–101. doi: 10.1038/nature12486
Bahar-Fuchs, A., Clare, L., and Woods, B. (2013). Cognitive training and cognitive rehabilitation for persons with mild to moderate dementia of the Alzheimer's or vascular type: a review. Alzheimer's Res. Ther. 5:35. doi: 10.1186/alzrt189
Baker, L. D., Frank, L. L., Foster-Schubert, K., Green, P. S., Wilkinson, C. W., McTiernan, A., et al. (2010). Effects of aerobic exercise on mild cognitive impairment: a controlled trial. Arch. Neurol. 67, 71–79. doi: 10.1001/archneurol.2009.307
Bamidis, P. D., Fissler, P., Papageorgiou, S. G., Zilidou, V., Konstantinidis, E. I., Billis, A. S., et al. (2015). Gains in cognition through combined cognitive and physical training: the role of training dosage and severity of neurocognitive disorder. Front. Aging Neurosci. 7:152. doi: 10.3389/fnagi.2015.00152
Bamidis, P. D., Konstantinidis, E. I., Billis, A., Frantzidis, C., Tsolaki, M., Hlauschek, W., et al. (2011). “A Web services-based exergaming platform for senior citizens: the Long Lasting Memories project approach to e-health care,” in Conference Proceedings:…Annual International Conference Of The IEEE Engineering In Medicine And Biology Society. IEEE Engineering In Medicine And Biology Society. Annual Conference, 20112505-2509 (Boston, MA).
Bamidis, P. D., Vivas, A. B., Styliadis, C., Frantzidis, C., Klados, M., Schlee, W., et al. (2014). A review of physical and cognitive interventions in aging. Neurosci. Biobehav. Rev. 44, 206–220. doi: 10.1016/j.neubiorev.2014.03.019
Barcelos, N., Shah, N., Cohen, K., Hogan, M. J., Mulkerrin, E., Arciero, P. J., et al. (2015). Aerobic and cognitive exercise (ACE) pilot study for older adults: executive function improves with cognitive challenge while exergaming. J. Int. Neuropsychol. Soc. 21, 768–779. doi: 10.1017/S1355617715001083
Barha, C. K., Galea, L. A., Nagamatsu, L. S., Erickson, K. I., and Liu-Ambrose, T. (2016). Personalising exercise recommendations for brain health: considerations and future directions. Brit. J. Sports Med. 51, 636–639. doi: 10.1136/bjsports-2016-096710
Barnes, D. E., Santos-Modesitt, W., Poelke, G., Kramer, A. F., Castro, C., Middleton, L. E., et al. (2013). The mental activity and exercise (MAX) trial. A randomized controlled trial to enhance cognitive function in older adults. JAMA 173, 797–804. doi: 10.1001/jamainternmed.2013.189
Barry, G., Galna, B., and Rochester, L. (2014). The role of exergaming in Parkinson's disease rehabilitation: a systematic review of the evidence. J. Neuroeng. Rehabil. 11:33. doi: 10.1186/1743-0003-11-33
Batistela, M. S., Josviak, N. D., Sulzbach, C. D., and de Souza, R. R. (2017). An overview of circulating cell-free microRNAs as putative biomarkers in Alzheimer's and Parkinson's Diseases. Int. J. Neurosci. 127, 547–558. doi: 10.1080/00207454.2016.1209754
Beglinger, L. J., Gaydos, B., Tangphao-Daniels, O., Duff, K., Kareken, D. A., Crawford, J., et al. (2005). Practice effects and the use of alternate forms in serial neuropsychological testing. Arch. Clin. Neuropsychol. 20, 517–529. doi: 10.1016/j.acn.2004.12.003
Bellar, D., Glickman, E. L., Juvancic-Heltzel, J., and Gunstad, J. (2011). Serum insulin like growth factor-1 is associated with working memory, executive function, and selective attention in a sample of healthy, fit older adults. Neuroscience 178, 133–137. doi: 10.1016/j.neuroscience.2010.12.023
Bertoldi, K., Cechinel, L. R., Schallenberger, B., Corssac, G. B., Davies, S., Guerreiro, I. K., et al. (2017). Circulating extracellular vesicles in the aging process: impact of aerobic exercise. Mol. Cell. Biochem. 440, 115–125. doi: 10.1007/s11010-017-3160-4
Bherer, L. (2015). Cognitive plasticity in older adults: effects of cognitive training and physical exercise. Ann. N. Y. Acad. Sci. 1337, 1–6. doi: 10.1111/nyas.12682
Black, J. E., Isaacs, K. R., Anderson, B. J., Alcantara, A. A., and Greenough, W. T. (1990). Learning causes synaptogenesis, whereas motor activity causes angiogenesis, in cerebellar cortex of adult rats. Proc. Natl. Acad. Sci. U.S.A. 87, 5568–5572. doi: 10.1073/pnas.87.14.5568
Boot, W. R., and Kramer, A. F. (2014). The brain games conundrum: does cognitive training really sharpen the mind? Cerebrum 2014, 1–15.
Boyke, J., Driemeyer, J., Gaser, C., Büchel, C., and May, A. (2008). Training-induced brain structure changes in the elderly. J. Neurosci. 28, 7031–7035. doi: 10.1523/JNEUROSCI.0742-08.2008
Brookmeyer, R., Gray, S., and Kawas, C. (1998). Projections of Alzheimer's disease in the United States and the public health impact of delaying disease onset. Amer. J. Public Health 88, 1337–1342. doi: 10.2105/AJPH.88.9.1337
Brown, B. M., Peiffer, J. J., and Martins, R. N. (2013). Multiple effects of physical activity on molecular and cognitive signs of brain aging: can exercise slow neurodegeneration and delay Alzheimer's disease? Mol. Psychiatry 18, 864–874. doi: 10.1038/mp.2012.162
Burdette, J. H., Laurienti, P. J., Espeland, M. A., Morgan, A., Telesford, Q., Vechlekar, C. D., et al. (2010). Using network science to evaluate exercise-associated brain changes in older adults. Front. Aging Neurosci. 2:23. doi: 10.3389/fnagi.2010.00023
Burzynska, A. Z., Jiao, Y., Knecht, A. M., Fanning, J., Awick, E. A., Chen, T., et al. (2017). White matter integrity declined over 6-months, but dance intervention improved integrity of the fornix of older adults. Front. Aging Neurosci. 9:59. doi: 10.3389/fnagi.2017.00059
Ceccarelli, A., Rocca, M. A., Pagani, E., Falini, A., Comi, G., and Filippi, M. (2009). Cognitive learning is associated with gray matter changes in healthy human individuals: a tensor-based morphometry study. Neuroimage 48, 585–589. doi: 10.1016/j.neuroimage.2009.07.009
Chao, Y., Scherer, Y., and Montgomery, C. (2014). Effects of using nintendo wii exergames in older adults: a review of the literature. J. Aging Health 27, 379–402. doi: 10.1177/0898264314551171
Chapman, S. B., Aslan, S., Spence, J. S., DeFina, L. F., Keebler, M. W., Didehbani, N., et al. (2013). Shorter term aerobic exercise improves brain, cognition, and cardiovascular fitness in aging. Front. Aging Neurosci. 5:75. doi: 10.3389/fnagi.2013.00075
Chapman, S. B., Aslan, S., Spence, J. S., Keebler, M. W., DeFina, L. F., Didehbani, N., et al. (2016). Distinct brain and behavioral benefits from cognitive vs. physical training: a randomized trial in aging adults. Front. Hum. Neurosci. 10:338. doi: 10.3389/fnhum.2016.00338
Chodzko-Zajko, W. J., Proctor, D. N., Fiatarone Singh, M. A., Minson, C. T., Nigg, C. R., Salem, G. J., et al. (2009). American College of sports medicine position stand. exercise and physical activity for older adults. Med. Sci. Sports Exerc. 41, 1510–1530. doi: 10.1249/MSS.0b013e3181a0c95c
Churchill, J. D., Galvez, R., Colcombe, S., Swain, R. A., Kramer, A. F., and Greenough, W. T. (2002). Exercise, experience and the aging brain. Neurobiol. Aging 23, 941–955. doi: 10.1016/S0197-4580(02)00028-3
Coelho, F. G., Andrade, L. P., Pedroso, R. V., Santos-Galduroz, R. F., Gobbi, S., Costa, J. L., et al. (2013b). Multimodal exercise intervention improves frontal cognitive functions and gait in Alzheimer's disease: a controlled trial. Geriatr. Gerontol. Int. 13, 198–203. doi: 10.1111/j.1447-0594.2012.00887.x
Coelho, F. G., Gobbi, S., Andreatto, C. A. A., Corazza, D. I., Pedroso, R. V., and Santos-Galduróz, R. F. (2013a). Physical exercise modulates peripheral levels of brain-derived neurotrophic factor (BDNF): a systematic review of experimental studies in the elderly. Arch. Gerontol. Geriatr. 56, 10–15. doi: 10.1016/j.archger.2012.06.003
Colcombe, S. J., Erickson, K. I., Scalf, P. E., Kim, J. S., Prakash, R., McAuley, E., et al. (2006). Aerobic exercise training increases brain volume in aging humans. J. Gerontol. 61, 1166–1170. doi: 10.1093/gerona/61.11.1166
Colcombe, S., and Kramer, A. F. (2003). Fitness effects on the cognitive function of older adults: a meta-analytic study. Psychol. Sci. 14, 125–130. doi: 10.1111/1467-9280.t01-1-01430
Colcombe, S. J., Kramer, A. F., Erickson, K. I., Scalf, P., McAuley, E., Cohen, N. J., et al. (2004). Cardiovascular fitness, cortical plasticity, and aging. Proc. Natl. Acad. Sci. U.S.A. 101, 3316–3321. doi: 10.1073/pnas.0400266101
Cotman, C. W., Berchtold, N. C., and Christie, L.-A. (2007). Exercise builds brain health: key roles of growth factor cascades and inflammation. Trends Neurosci. 30, 464–472. doi: 10.1016/j.tins.2007.06.011
Curlik, D., and Shors, T. (2013). Training your brain: do mental and physical (MAP) training enhance cognition through the process of neurogenesis in the hippocampus? Neuropharmacology 64, 506–514. doi: 10.1016/j.neuropharm.2012.07.027
D'Elia, L. G., Satz, P., Uchiyama, C. L., and White, T. (1996). Color Trails Test Manual. Odessa, FL: Psychological Assessment Resources.
Desikan, R. S., Ségonne, F., Fischl, B., Quinn, B. T., Dickerson, B. C., Blacker, D., et al. (2006). An automated labeling system for subdividing the human cerebral cortex on MRI scans into gyral based regions of interest. Neuroimage 31, 968–980. doi: 10.1016/j.neuroimage.2006.01.021
Desjardins-Crépeau, L., Berryman, N., Fraser, S. A., Vu, T. T. M., Kergoat, M.-J., Li, K. Z., et al. (2016). Effects of combined physical and cognitive training on fitness and neuropsychological outcomes in healthy older adults. Clin. Interv. Aging 11:1287. doi: 10.2147/CIA.S115711
Destrieux, C., Fischl, B., Dale, A., and Halgren, E. (2010). Automatic parcellation of human cortical gyri and sulci using standard anatomical nomenclature. Neuroimage 53, 1–15. doi: 10.1016/j.neuroimage.2010.06.010
Dhami, P., Moreno, S., and DeSouza, J. X. (2015). New framework for rehabilitation—Fusion of cognitive and physical rehabilitation: the hope for dancing. Front. Psychol. 5:1478. doi: 10.3389/fpsyg.2014.01478
Diamond, A. (2013). Executive functions. Annu. Rev. Psychol. 64, 135–168. doi: 10.1146/annurev-psych-113011-143750
Dimitrova, J., Hogan, M., Khader, P., O'Hora, D., Kilmartin, L., Walsh, J. C., et al. (2016). Comparing the effects of an acute bout of physical exercise with an acute bout of interactive mental and physical exercise on electrophysiology and executive functioning in younger and older adults. Aging Clin. Exp. Res. 29, 959–967. doi: 10.1007/s40520-016-0683-6
Dinoff, A., Herrmann, N., Swardfager, W., Liu, C. S., Sherman, C., Chan, S., et al. (2016). The effect of exercise training on resting concentrations of peripheral brain-derived neurotrophic factor (BDNF): a meta-analysis. PLoS ONE 11:e0163037. doi: 10.1371/journal.pone.0163037
Domenech, P., and Koechlin, E. (2015). Executive control and decision-making in the prefrontal cortex. Curr. Opin. Behav. Sci. 1, 101–106. doi: 10.1016/j.cobeha.2014.10.007
Edelmann, E., Lessmann, V., and Brigadski, T. (2014). Pre- and postsynaptic twists in BDNF secretion and action in synaptic plasticity. Neuropharmacology 76, 610–627. doi: 10.1016/j.neuropharm.2013.05.043
Eggenberger, P., Schumacher, V., Angst, M., Theill, N., and de Bruin, E. D. (2015). Does multicomponent physical exercise with simultaneous cognitive training boost cognitive performance in older adults? A 6-month randomized controlled trial with a 1-year follow-up. Clin. Interv. Aging 10, 1335–1349. doi: 10.2147/CIA.S87732
Eggenberger, P., Wolf, M., Schumann, M., and de Bruin, E. D. (2016). Exergame and balance training modulate prefrontal brain activity during walking and enhance executive function in older adults. Front. Aging Neurosci. 8:66. doi: 10.3389/fnagi.2016.00066
Ehlers, D. K., Daugherty, A. M., Burzynska, A. Z., Fanning, J., Awick, E. A., Chaddock-Heyman, L., et al. (2017). Regional brain volumes moderate, but do not mediate, the effects of group-based exercise training on reductions in loneliness in older adults. Front. Aging Neurosci. 9:110. doi: 10.3389/fnagi.2017.00110
Erickson, K. I., Hillman, C., and Kramer, A. F. (2015). Physical activity, brain, and cognition. Curr. Opin. Behav. Sci. 4, 27–32. doi: 10.1016/j.cobeha.2015.01.005
Erickson, K. I., Miller, D. L., and Roecklein, K. A. (2012). The aging hippocampus: interactions between exercise, depression, and BDNF. Neuroscientist 18, 82–97. doi: 10.1177/1073858410397054
Erickson, K. I., Voss, M. W., Prakash, R. S., Basak, C., Szabo, A., Chaddock, L., et al. (2011). Exercise training increases size of hippocampus and improves memory. Proc. Natl. Acad. Sci. U.S.A. 108, 3017–3022. doi: 10.1073/pnas.1015950108
Etnier, J. L., and Chang, Y. K. (2009). The effect of physical activity on executive function: a brief commentary on definitions, measurement issues, and the current state of the literature. J. Sport Exerc. Psychol. 31, 469–483. doi: 10.1123/jsep.31.4.469
Ettenhofer, M. L., Hambrick, D. Z., and Abeles, N. (2006). Reliability and stability of executive functioning in older adults. Neuropsychology 20, 607–613. doi: 10.1037/0894-4105.20.5.607
Fabel, K., Wolf, S., Ehninger, D., Babu, H., Galicia, P., and Kempermann, G. (2009). Additive effects of physical exercise and environmental enrichment on adult hippocampal neurogenesis in mice. Front. Neurosci. 3:50. doi: 10.3389/neuro.22.002.2009
Fabre, C., Chamari, K., Mucci, P., Masse-Biron, J., and Prefaut, C. (2002). Improvement of cognitive function by mental and/or individualized aerobic training in healthy elderly subjects. Int. J. Sports Med. 23, 415–421. doi: 10.1055/s-2002-33735
Faul, F., Erdfelder, E., Lang, A.-G., and Buchner, A. (2007). G+Power 3: a flexible statistical power analysis program for the social, behavioral, and biomedical sciences. Behav. Res. Methods 39, 175–191. doi: 10.3758/BF03193146
Fiatarone, S. M. A., Gates, N., Saigal, N., Wilson, G. C., Meiklejohn, J., Brodaty, H., et al. (2014). The Study of Mental and Resistance Training (SMART) study-resistance training and/or cognitive training in mild cognitive impairment: a randomized, double-blind, double-sham controlled trial. J. Am. Med. Dir. Assoc. 15, 873–880. doi: 10.1016/j.jamda.2014.09.010
Fischl, B., Salat, D. H., Busa, E., Albert, M., Dieterich, M., Haselgrove, C., et al. (2002). Whole brain segmentation: automated labeling of neuroanatomical structures in the human brain. Neuron 33, 341–355. doi: 10.1016/S0896-6273(02)00569-X
Fissler, P., Kuster, O., Schlee, W., and Kolassa, I.-T. (2013). Novelty interventions to enhance broad cognitive abilities and prevent dementia: synergistic approaches for the facilitation of positive plastic change. Progress. Brain Res. 207, 403–434. doi: 10.1016/B978-0-444-63327-9.00017-5
Flöel, A., Ruscheweyh, R., Krüger, K., Willemer, C., Winter, B., Völker, K., et al. (2010). Physical activity and memory functions: are neurotrophins and cerebral gray matter volume the missing link? Neuroimage 49, 2756–2763. doi: 10.1016/j.neuroimage.2009.10.043
Forbes, D., Forbes, S. C., Blake, C. M., Thiessen, E. J., and Forbes, S. (2015). Exercise programs for people with dementia. Cochrane Database Syst. Rev. CD006489. doi: 10.1002/14651858.CD006489.pub4
Forte, R., Boreham, C. G., Leite, J. C., De Vito, G., Brennan, L., Gibney, E. R., et al. (2013). Enhancing cognitive functioning in the elderly: multicomponent vs resistance training. Clin. Interv. Aging 8, 9–27. doi: 10.2147/CIA.S36514
Foster, P. P. (2013). How does dancing promote brain reconditioning in the elderly? Front. Aging Neurosci. 5:4. doi: 10.3389/fnagi.2013.00004
Frantzidis, C. A., Ladas, A. I., Vivas, A. B., Tsolaki, M., and Bamidis, P. D. (2014). Cognitive and physical training for the elderly: evaluating outcome efficacy by means of neurophysiological synchronization. Int. J. Psychophysiol. 93, 1–11. doi: 10.1016/j.ijpsycho.2014.01.007
Freitas, S., Simões, M. R., Marôco, J., Alves, L., and Santana, I. (2012). Construct validity of the Montreal Cognitive Assessment (MoCA). J. Int. Neuropsychol. Soc. 18, 242–250. doi: 10.1017/S1355617711001573
Gage, F. H. (2002). Neurogenesis in the adult brain. J. Neurosci. 22, 612–613. doi: 10.1523/JNEUROSCI.22-03-00612.2002
Gallo, A., and Alevizos, I. (2013). Isolation of circulating microRNA in saliva. Methods Mol. Biol. 1024, 183–190. doi: 10.1007/978-1-62703-453-1_14
Galvan, V., and Bredesen, D. E. (2007). Neurogenesis in the adult brain: implications for Alzheimer's disease. CNS Neurol. Disord. Drug Targets 6, 303–310.
Gates, N. (2013). The effect of exercise training on cognitive function in older adults with mild cognitive impairment: a meta-analysis of randomized controlled trials. Amer. J. Geriatr. Psychiatry 21, 1086–1098. doi: 10.1016/j.jagp.2013.02.018
Geda, Y. E., Roberts, R. O., Knopman, D. S., Christianson, T. J., Pankratz, V. S., Ivnik, R. J., et al. (2010). Physical exercise, aging, and mild cognitive impairment. Arch. Neurol. 67, 80–86. doi: 10.1001/archneurol.2009.297
Geerlings, M. I., Sigurdsson, S., Eiriksdottir, G., Garcia, M. E., Harris, T. B., Gudnason, V., et al. (2015). Salivary cortisol, brain volumes, and cognition in community-dwelling elderly without dementia. Neurology 85, 976–983. doi: 10.1212/WNL.0000000000001931
Gerling, K., and Mandryk, R. (2014). Custom-designed motion-based games for older adults: a review of literature in human-computer interaction. Gerontechnology 12, 68–80. doi: 10.4017/gt.2013.12.2.001.00
González-Palau, F., Franco, M., Bamidis, P., Losada, R., Parra, E., Papageorgiou, S. G., et al. (2014). The effects of a computer-based cognitive and physical training program in a healthy and mildly cognitive impaired aging sample. Aging Ment. Health 18, 838–846. doi: 10.1080/13607863.2014.899972
Gordon, B. A., Rykhlevskaia, E. I., Brumback, C. R., Lee, Y., Elavsky, S., Konopack, J. F., et al. (2008). Neuroanatomical correlates of aging, cardiopulmonary fitness level, and education. Psychophysiology 45, 825–838. doi: 10.1111/j.1469-8986.2008.00676.x
Grandes, G., Sanchez, A., Sanchez-Pinilla, R. O., Torcal, J., Montoya, I., Lizarraga, K., et al. (2009). Effectiveness of physical activity advice and prescription by physicians in routine primary care: a cluster randomized trial. Arch. Intern. Med. 169, 694–701. doi: 10.1001/archinternmed.2009.23
Grandjean, J., D'Ostilio, K., Phillips, C., Balteau, E., Degueldre, C., Luxen, A., et al. (2012). Modulation of brain activity during a Stroop inhibitory task by the kind of cognitive control required. PLoS ONE 7:e41513. doi: 10.1371/journal.pone.0041513
Greenough, W. T., Cohen, N. J., and Juraska, J. M. (1999). New neurons in old brains: learning to survive? Nat. Neurosci. 2, 203–205. doi: 10.1038/6300
Hannay, J. H., and Lezak, M. D. (2004). “The neuropsychological examination: interpretation,” in Neuropsychological Assessment, 4th Edn. eds M. D. Lezak, D. B. Howieson, and D. W. Loring (New York, NY: Oxford University Press), 133–156.
Harrison, J., Minassian, S. L., Jenkins, L., Black, R. S., Koller, M., and Grundman, M. (2007). A neuropsychological test battery for use in Alzheimer disease clinical trials. Arch. Neurol. 64, 1323–1329. doi: 10.1001/archneur.64.9.1323
Hayes, S. M., Hayes, J. P., Cadden, M., and Verfaellie, M. (2013). A review of cardiorespiratory fitness-related neuroplasticity in the aging brain. Front. Aging Neurosci. 5:31. doi: 10.3389/fnagi.2013.00031
Hess, N. C. L., Dieberg, G., McFarlane, J. R., and Smart, N. A. (2014). The effect of exercise intervention on cognitive performance in persons at risk for, or with, dementia: a systematic review and meta-analysis. Healthy Aging Res. 3:3. doi: 10.12715/har.2014.3.3
Heyn, P., Abreu, B. C., and Ottenbacher, K. J. (2004). The effects of exercise training on elderly persons with cognitive impairment and dementia: a meta-analysis. Arch. Phys. Med. Rehabil. 85, 1694–1704. doi: 10.1016/j.apmr.2004.03.019
Hillman, C., Erickson, K., and Kramer, A. (2008). Be smart, exercise your heart: exercise effects on brain and cognition. Nat. Rev. Neurosci. 9, 58–65. doi: 10.1038/nrn2298
Hiyamizu, M., Morioka, S., Shomoto, K., and Shimada, T. (2012). Effects of dual task balance training on dual task performance in elderly people: a randomized controlled trial. Clin. Rehabil. 26, 58–67. doi: 10.1177/0269215510394222
Hosseini, S. H., Bruno, J. L., Baker, J. M., Gundran, A., Harbott, L. K., Gerdes, J. C., et al. (2017). Neural, physiological, and behavioral correlates of visuomotor cognitive load. Sci. Rep. 7:8866. doi: 10.1038/s41598-017-07897-z
Hotting, K., and Roder, B. (2013). Effects of physical exercise on neuroplasticity and cognition. Neurosci. Biobehav. Rev. 37, 2243–2257. doi: 10.1016/j.neubiorev.2013.04.005
Houx, P. J., Shepherd, J., Blauw, G.-J., Murphy, M. B., Ford, I., Bollen, E. L., et al. (2002). Testing cognitive function in elderly populations: The PROSPER study. J. Neurol. Neurosurg. Psychiatr. 73, 385–389. doi: 10.1136/jnnp.73.4.385
Huang, E. J., and Reichardt, L. F. (2001). Neurotrophins: roles in neuronal development and function. Annu. Rev. Neurosci. 24, 677–736. doi: 10.1146/annurev.neuro.24.1.677
Huntley, J. D., Gould, R. L., Liu, K., Smith, M., and Howard, R. J. (2015). Do cognitive interventions improve general cognition in dementia? A meta-analysis and meta-regression. BMJ Open 5:e005247. doi: 10.1136/bmjopen-2014-005247
Jak, A. J., Bondi, M. W., Delano-Wood, L., Wierenga, C., Corey-Bloom, J., Salmon, D. P., et al. (2009). Quantification of five neuropsychological approaches to defining mild cognitive impairment. Am. J. Geriatr. Psychiatry 17, 368–375. doi: 10.1097/JGP.0b013e31819431d5
Jak, A. J., Preis, S. R., Beiser, A. S., Seshadri, S., Wolf, P. A., Bondi, M. W., et al. (2016). Neuropsychological criteria for mild cognitive impairment and dementia risk in the Framingham Heart Study. J. Int. Neuropsychol. Soc. 22, 937–943. doi: 10.1017/S1355617716000199
Jessberger, S., and Gage, F. H. (2014). Adult neurogenesis: bridging the gap between mice and humans. Trends Cell Biol. 24, 558–563. doi: 10.1016/j.tcb.2014.07.003
Jonasson, L. S., Nyberg, L., Kramer, A. F., Lundquist, A., Riklund, K., and Boraxbekk, C. J. (2017). Aerobic exercise intervention, cognitive performance, and brain structure: Results from the Physical Influences on Brain in Aging (PHIBRA) Study. Front. Aging Neurosci. 8:336. doi: 10.3389/fnagi.2016.00336
Julayanont, P., Brousseau, M., Chertkow, H., Phillips, N., and Nasreddine, Z. S. (2014). Montreal Cognitive Assessment Memory Index Score (MoCA-MIS) as a predictor of conversion from mild cognitive impairment to Alzheimer's disease. J. Am. Geriatr. Soc. 62, 679–684. doi: 10.1111/jgs.12742
Karlawish, J. (2008). Measuring decision-making capacity in cognitively impaired individuals. Neurosignals 16, 91–98. doi: 10.1159/000109763
Karr, J. E., Areshenkoff, C. N., Rast, P., and Garcia-Barrera, M. A. (2014). An empirical comparison of the therapeutic benefits of physical exercise and cognitive training on the executive functions of older adults: a meta-analysis of controlled trials. Neuropsychology 28, 829–845. doi: 10.1037/neu0000101
Karssemeijer, E. A., Aaronson, J. A., Bossers, W. J., Smits, T., Olde Rikkert, M. M., and Kessels, R. C. (2017). Positive effects of combined cognitive and physical exercise training on cognitive function in older adults with mild cognitive impairment or dementia: a meta-analysis. Ageing Res. Rev. 40, 75–83. doi: 10.1016/j.arr.2017.09.003
Kattenstroth, J., Kalisch, T., Holt, S., Tegenthoff, M., and Dinse, H. R. (2013). Six months of dance intervention enhances postural, sensorimotor, and cognitive performance in elderly without affecting cardio-respiratory functions. Front. Aging Neurosci. 5:5. doi: 10.3389/fnagi.2013.00005
Kleemeyer, M. M., Polk, T. A., Schaefer, S., Bodammer, N. C., Brechtel, L., and Lindenberger, U. (2017). Exercise-induced fitness changes correlate with changes in neural specificity in older adults. Front. Hum. Neurosci. 11:123. doi: 10.3389/fnhum.2017.00123
Klusmann, V., Evers, A., Schwarzer, R., Schlattmann, P., Reischies, F. M., Heuser, I., et al. (2010). Complex mental and physical activity in older women and cognitive performance: a 6-month randomized controlled trial. J. Gerontol. 65, 680–688. doi: 10.1093/gerona/glq053
Knaepen, K., Goekint, M., Heyman, E. M., and Meeusen, R. (2010). Neuroplasticity – exercise-induced response of peripheral brain-derived neurotrophic factor: a systematic review of experimental studies in human subjects. Sports Med. 40, 765–801. doi: 10.2165/11534530-000000000-00000
Konstantinidis, E. I., Bamparopoulos, G., and Bamidis, P. D. (2017). Moving real exergaming engines on the web: the webfitforall case study in an active and healthy ageing living lab environment. IEEE J. Biomed. Health Inform. 21, 859–866. doi: 10.1109/JBHI.2016.2559787
Kraft, E. (2012). Cognitive function, physical activity, and aging: possible biological links and implications for multimodal interventions. Aging Neuropsychol. C 19, 248–263. doi: 10.1080/13825585.2011.645010
Kramer, A. F., Hahn, S., Cohen, N. J., Banich, M. T., McAuley, E., Harrison, C. R., et al. (1999). Ageing, fitness and neurocognitive function. Nature 400, 418–419. doi: 10.1038/22682
Kramer, A., and Erickson, K. (2007). Capitalizing on cortical plasticity: Influence of physical activity on cognition and brain function. Trends Cogn. Sci. 11, 342–348. doi: 10.1016/j.tics.2007.06.009
Kramer, A., Wickens, C., and Donchin, E. (1985). Processing of stimulus properties: evidence for dual-task integrality. J. Exp. Psychol. 11, 393–408. doi: 10.1037/0096-1523.11.4.393
Kumar, S., and Reddy, P. H. (2016). Are circulating microRNAs peripheral biomarkers for Alzheimer's disease? Biochim. Biophys. Acta 1862, 1617–1627. doi: 10.1016/j.bbadis.2016.06.001
Lam, M., Eng, G. K., Rapisarda, A., Subramanium, M., Kraus, M., Keefe, R. E., et al. (2013). Formulation of the age–education index: measuring age and education effects in neuropsychological performance. Psychol. Assess. 25, 61–70. doi: 10.1037/a0030548
Lansbergen, M. M., Kenemans, J. L., and van Engeland, H. (2007). Stroop interference and attention-deficit/hyperactivity disorder: a review and meta-analysis. Neuropsychology 21, 251–262. doi: 10.1037/0894-4105.21.2.251
Lara, J., Cooper, R., Nissan, J., Ginty, A. T., Khaw, K.-T., Deary, I. J., et al. (2015). A proposed panel of biomarkers of healthy ageing. BMC Med. 13:222. doi: 10.1186/s12916-015-0470-9
Larson, E. (2010). Prospects for delaying the rising tide of worldwide, late-life dementias. Int. Psychogeriatr. 22, 1196–1202. doi: 10.1017/S1041610210001080
Lauenroth, A., Ioannidis, A. E., and Teichmann, B. (2016). Influence of combined physical and cognitive training on cognition: a systematic review. BMC Geriatr. 16:141. doi: 10.1186/s12877-016-0315-1
Law, L. L., Barnett, F., Yau, M. K., and Gray, M. A. (2014). Effects of combined cognitive and exercise interventions on cognition in older adults with and without cognitive impairment: a systematic review. Ageing Res. Rev. 15, 61–75. doi: 10.1016/j.arr.2014.02.008
Leckie, R. L., Oberlin, L. E., Voss, M. W., Prakash, R. S., Szabo-Reed, A., Chaddock-Heyman, L., et al. (2014). BDNF mediates improvements in executive function following a 1-year exercise intervention. Front. Hum. Neurosci. 8:985. doi: 10.3389/fnhum.2014.00985
Lee, Y. Y., Wu, C. Y., Teng, C. H., Hsu, W. C., Chang, K. C., and Chen, P. (2016). Evolving methods to combine cognitive and physical training for individuals with mild cognitive impairment: study protocol for a randomized controlled study. Trials 17:526. doi: 10.1186/s13063-016-1650-4
Legault, C., Jennings, J. M., Katula, J. A., Dagenbach, D., Gaussoin, S. A., Sink, K. M., et al. (2011). Designing clinical trials for assessing the effects of cognitive training and physical activity interventions on cognitive outcomes: The Seniors Health and Activity Research Program Pilot (SHARP-P) study, a randomized controlled trial. BMC Geriatr. 11:27. doi: 10.1186/1471-2318-11-27
Leßmann, V., and Brigadski, T. (2009). Mechanisms, locations, and kinetics of synaptic BDNF secretion: an update. Neurosci. Res. 65, 11–22. doi: 10.1016/j.neures.2009.06.004
Li, M., Huang, M., Li, S., Tao, J., Zheng, G., and Chen, L. (2017). The effects of aerobic exercise on the structure and function of DMN-related brain regions: a systematic review. Int. J. Neurosci. 127, 634–649. doi: 10.1080/00207454.2016.1212855
Li, S., Yan, Y., Jiao, Y., Gao, Z., Xia, Y., Kong, L., et al. (2016). Neuroprotective effect of osthole on neuron synapses in an alzheimer's disease cell model via upregulation of MicroRNA-9. J. Mol. Neurosci. 60, 71–81. doi: 10.1007/s12031-016-0793-9
Lieberman, D. A. (2009). “Designing serious games for learning and health in informal and formal settings,” in Serious Games: Mechanisms and Effects, eds U. Ritterfeld, M. Cody, and P. Vorderer (New York, NY: Routledge), 117–130.
Lipardo, D. S., Aseron, A. C., Kwan, M. M., and Tsang, W. W. (2017). Effect of exercise and cognitive training on falls and fall-related factors in older adults with mild cognitive impairment: a systematic review. Arch. Phys. Med. Rehabil. 98, 2079–2096. doi: 10.1016/j.apmr.2017.04.021
Lommatzsch, M., Zingler, D., Schuhbaeck, K., Schloetcke, K., Zingler, C., Schuff-Werner, P., et al. (2005). The impact of age, weight and gender on BDNF levels in human platelets and plasma. Neurobiol. Aging 26, 115–123. doi: 10.1016/j.neurobiolaging.2004.03.002
Lorens-Martín, M., Torres-Alemán, I., and Trejo, J. L. (2009). Reviews: mechanisms mediating brain plasticity: IGF-1 and adult hippocampal neurogenesis. Neuroscientist 15, 134–148. doi: 10.1177/1073858408331371
Maass, A., Duzel, S., Brigads, T., Goerke, M., Becke, A., Sobieray, U., et al. (2016). Relationships of peripheral IGF-1, VEGF and BDNF levels to exercise-related changes in memory, hippocampal perfusion and volumes in older adults. Neuroimage 131, 142–154. doi: 10.1016/j.neuroimage.2015.10.084
Maillot, P., Perrot, A., and Hartley, A. (2012). Effects of interactive physical-activity video- game training on physical and cognitive function in older adults. Psychol. Aging 3, 589–600. doi: 10.1037/a0026268
Mandel, A., Ozdener, H., and Utermohlen, V. (2011). Brain-derived neurotrophic factor in human saliva: ELISA optimization and biological correlates. J. Immunoassay Immunochem. 32, 18–30. doi: 10.1080/15321819.2011.538625
Marquez, D. X., Wilson, R., Aguiñaga, S., Vásquez, P., Fogg, L., Yang, Z., et al. (2017). Regular latin dancing and health education may improve cognition of late middle-aged and older latinos. J. Aging Phys. Act. 25, 482–489. doi: 10.1123/japa.2016-0049
Marshall, G. A., Rentz, D. M., Frey, M. T., Locascio, J. J., Johnson, K. A., and Sperling, R. A. (2011). Executive function and instrumental activities of daily living in mild cognitive impairment and Alzheimer's disease. Alzheimer's Dementia 7, 300–308. doi: 10.1016/j.jalz.2010.04.005
Martin, M., Clare, L., Altgassen, A. M., Cameron, M. H., and Zehnder, F. (2011). Cognition-based interventions for healthy older people and people with mild cognitive impairment. Cochrane Database Syst. Rev. 1:CD006220. doi: 10.1002/14651858.CD006220.pub2
McAuley, E., Mullen, S. P., Szabo, A. N., White, S. M., Wojcicki, T. R., Mailey, E. L., et al. (2011). Self-regulatory processes and exercise adherence in older adults: Executive function and self-efficacy effects. Am. J. Prev. Med. 41, 284–290. doi: 10.1016/j.amepre.2011.04.014
Mills, K. L., and Tamnes, C. K. (2014). Methods and considerations for longitudinal structural brain imaging analysis across development. Dev. Cogn. Neurosci. 9, 172–190. doi: 10.1016/j.dcn.2014.04.004
Monteiro-Junior, R. S., de Tarso Maciel-Pinheiro, P., da Matta Mello Portugal, E., da Silva Figueiredo, L. F., Terra, R., Carneiro, L. F., et al. (2017). Effect of exercise on inflammatory profile of older persons: systematic review and meta-analyses. J. Phys. Act. Health 15, 64–71. doi: 10.1123/jpah.2016-0735
Morrison-Bogorad, M., Cahan, V., and Wagster, M. (2007). Brain health interventions: the need for further research. Alzheimers Dem. 3, S80–S85. doi: 10.1016/j.jalz.2007.01.015
Muijden, J. V., Band, G. P. H., and Hommel, B. (2012). Online games training aging brains: limited transfer to cognitive control functions. Front. Hum. Neurosci. 6:221. doi: 10.3389/fnhum.2012.00221
Müller, P., Rehfeld, K., Schmicker, M., Hökelmann, A., Dordevic, M., Lessmann, V., et al. (2017). Evolution of neuroplasticity in response to physical activity in old age: the case for dancing. Front. Aging Neurosci. 9:56. doi: 10.3389/fnagi.2017.00056
Nasreddine, Z. S., Phillips, N. A., Bédirian, V., Charbonneau, S., Whitehead, V., Collin, I., et al. (2005). The montreal cognitive assessment, MoCA: a brief screening tool for mild cognitive impairment. J. Am. Geriatr. Soc. 53, 695–699. doi: 10.1111/j.1532-5415.2005.53221.x
National Alzheimer's Project Act (2011). National Alzheimer's Project Actof 2011. Public Law. No. 111–375.
Nishiguchi, S., Yamada, M., Tanigawa, T., Sekiyama, K., Kawagoe, T., Suzuki, M., et al. (2015). A 12-week physical and cognitive exercise program can improve cognitive function and neural efficiency in community-dwelling older adults: a randomized controlled trial. J. Am. Geriatr. Soc. 63, 1355–1363. doi: 10.1111/jgs.13481
Ogawa, E. F., You, T., and Leveille, S. G. (2016). Potential benefits of exergaming for cognition and dual-task function in older adults: a systematic review. J. Aging Phys. Activ. 24:332336. doi: 10.1123/japa.2014-0267
Olson, A. K., Eadie, B. D., Ernst, C., and Christie, B. R. (2006). Environmental enrichment and voluntary exercise massively increase neurogenesis in the adult hippocampus via dissociable pathways. Hippocampus 16, 250–260. doi: 10.1002/hipo.20157
Oswald, W. D., Gunzelmann, T., Rupprecht, R., and Hagen, B. (2006). Differential effects of single versus combined cognitive and physical training with older adults: the SimA study in a 5- year perspective. Eur. J. Aging 3, 179–192. doi: 10.1007/s10433-006-0035-z
Owen, A., Hampshire, A., Grahn, J. A., Stenton, R., Dajani, S., Burns, A. S., et al. (2010). Putting brain training to the test. Nature 465, 775–778. doi: 10.1038/nature09042
Park, H., and Poo, M. (2013). Neurotrophin regulation of neural circuit development and function. Nat. Rev. Neurosci. 14, 7–23. doi: 10.1038/nrn3379
Párrizas, M., Brugnara, L., Esteban, Y., González-Franquesa, A., Canivell, S., Murillo, S., et al. (2015). Circulating miR-192 and miR-193b are markers of prediabetes and are modulated by an exercise intervention. J. Clin. Endocrinol. Metab. 100, E407–E415. doi: 10.1210/jc.2014-2574
Payne, B. R., Jackson, J. J., Noh, S. R., and Stine-Morrow, E. L. (2011). In the zone: Flow state and cognition in older adults. Psychol. Aging 26, 738–743. doi: 10.1037/a0022359
Pereira, F. S., Yassuda, M. S., Oliveira, A. M., and Forlenza, O. V. (2008). Executive dysfunction correlates with impaired functional status in older adults with varying degrees of cognitive impairment. Int. Psychogeriatr. 20, 1104–1115. doi: 10.1017/S1041610208007631
Pfaffl, M. W., Horgan, G. W., and Dempfle, L. (2002). Relative expression software tool (REST (c)) for group-wise comparison and statistical analysis of relative expression results in real-time PCR. Nucleic Acids Res. 30:e36. doi: 10.1093/nar/30.9.e36
Phillips, C., Baktir, M. A., Das, D., Lin, B., and Salehi, A. (2015). The link between physical activity and cognitive dysfunction in Alzheimer disease. Phys. Ther. 95, 1046–1060. doi: 10.2522/ptj.20140212
Phillips, C., Baktir, M. A., Srivatsan, M., and Salehi, A. (2014). Neuroprotective effects of physical activity on the brain: a closer look at trophic factor signaling. Front. Cell. Neurosci. 8:170. doi: 10.3389/fncel.2014.00170
Podhorna, J., Krahnke, T., Shear, M., and Harrison, J. E. (2016). Alzheimer's Disease Assessment Scale-Cognitive subscale variants in mild cognitive impairment and mild Alzheimer's disease: change over time and the effect of enrichment strategies. Alzheimer's Res. Ther. 8:8. doi: 10.1186/s13195-016-0170-5
Podsiadlo, D., and Richardson, S. (1991). The timed “Up & Go”: a test of basic functional mobility for frail elderly persons. J. Am. Geriatr. Soc. 39, 142–148. doi: 10.1111/j.1532-5415.1991.tb01616.x
Pusic, K. M., Pusic, A. D., and Kraig, R. P. (2016). Environmental enrichment stimulates immune cell secretion of exosomes that promote CNS myelination and may regulate inflammation. Cell. Mol. Neurobiol. 36, 313–325. doi: 10.1007/s10571-015-0269-4
Rahe, J., Becker, J., Fink, G. R., Kessler, J., Kukolja, J., Rahn, A., et al. (2015). Cognitive training with and without additional physical activity in healthy older adults: cognitive effects, neurobiological mechanisms, and prediction of training success. Front. Aging Neurosci. 7:187. doi: 10.3389/fnagi.2015.00187
Raichlen, D. A., and Alexander, G. E. (2014). Exercise, APOE genotype, and the evolution of the human lifespan. Trends Neurosci. 37, 247–255. doi: 10.1016/j.tins.2014.03.001
Read, J. L., and Shortell, S. M. (2011). Interactive games to promote behavior change in prevention and treatment. JAMA 305, 1704–1705. doi: 10.1001/jama.2011.408
Redick, T. S., Shipstead, Z., Harrison, T. L., Hicks, K. L., Fried, D. E., Hambrick, D. Z., et al. (2013). No evidence of intelligence improvement after working memory training: a randomized, placebo-controlled study. J. Exp. Psychol. Gen. 142, 359–379. doi: 10.1037/a0029082
Rehfeld, K., Müller, P., Aye, N., Schmicker, M., Dordevic, M., Kaufmann, J., et al. (2017). Dancing or fitness sport? The effects of two training programs on hippocampal plasticity and balance abilities in healthy seniors. Front. Hum. Neurosci. 11:305. doi: 10.3389/fnhum.2017.00305
Reuter, M., Schmansky, N. J., Rosas, H. D., and Fischl, B. (2012). Within-subject template estimation for unbiased longitudinal image analysis. Neuroimage 62, 1402–1418. doi: 10.1016/j.neuroimage.2012.02.084
Riancho, J., Vázquez-Higuera, J. L., Pozueta, A., Lage, C., Kazimierczak, M., Bravo, M., et al. (2017). MicroRNA profile in patients with Alzheimer's disease: analysis of miR-9-5p and miR-598 in raw and exosome enriched cerebrospinal fluid samples. J. Alzheimer's Dis. 57, 483–491. doi: 10.3233/JAD-161179
Roberts, R., and Knopman, D. S. (2013). Classification and epidemiology of MCI. Clin. Geriatr. Med. 29, 753–772. doi: 10.1016/j.cger.2013.07.003
Ruigrok, A. V., Salimi-Khorshidi, G., Lai, M., Baron-Cohen, S., Lombardo, M. V., Tait, R. J., et al. (2014). A meta-analysis of sex differences in human brain structure. Neurosci. Biobehav. Rev. 39, 34–50. doi: 10.1016/j.neubiorev.2013.12.004
Ruijter, J. M., Thygesen, H. H., Schoneveld, O. J., Das, A T., Berkhout, B., and Lamers, W. H. (2006). Factor correction as a tool to eliminate between-session variation in replicate experiments: application to molecular biology and retrovirology. Retrovirology 3:2. doi: 10.1186/1742-4690-3-2
Ruijter, J. M., Ramakers, C., Hoogaars, W. M., Karlen, Y., Bakker, O., van den Hoff, M. J., et al. (2009). Amplification efficiency: linking baseline and bias in the analysis of quantitative PCR data. Nucleic Acids Res. 37:e45. doi: 10.1093/nar/gkp045
Russell, A. P., Lamon, S., Boon, H., Wada, S., Güller, I., Brown, E. L., et al. (2013). Regulation of miRNAs in human skeletal muscle following acute endurance exercise and short-term endurance training. J. Physiol. 591, 4637–4653. doi: 10.1113/jphysiol.2013.255695
Sano, M., Ernesto, C., Thomas, R. G., Klauber, M. R., Schafer, K., Grundman, M., et al. (1997). A controlled trial of selegiline, alpha-tocopherol, or both as treatment for Alzheimer's disease. The Alzheimer's Disease Cooperative Study. N. Engl. J. Med. 336, 1216–1222. doi: 10.1056/NEJM199704243361704
Satoh, M., Ogawa, J., Tokita, T., Nakaguchi, N., Nakao, K., Kida, H., et al. (2014). The effects of physical exercise with music on cognitive function of elderly people: Mihama-Kiho Project. PLoS ONE 9:e95230. doi: 10.1371/journal.pone.0095230
Schaefer, S., and Schumacher, V. (2011). The interplay between cognitive and motor functioning in healthy older adults: findings from dual-task studies and suggestions for intervention. Gerontology 57, 239–246. doi: 10.1159/000322197
Schoene, D., Lord, S. R., Delbaere, K., Severino, C., Davies, T. A., and Smith, S. T. (2013). A randomized controlled pilot study of home-based step training in older people using videogame technology. PLoS ONE 8:e57734. doi: 10.1371/journal.pone.0057734
Schoene, D., Valenzuela, T., Lord, S. R., and de Bruin, E. D. (2014). The effect of interactive cognitive-motor training in reducing fall risk in older people: a systematic review. BMC Geriatr. 14:107. doi: 10.1186/1471-2318-14-107
Schoene, D., Valenzuela, T., Toson, B., Delbaere, K., Severino, C., Garcia, J., et al. (2015). Interactive cognitive-motor step training improves cognitive risk factors of falling in older adults - a randomized controlled trial. PLoS ONE 10:e0145161. doi: 10.1371/journal.pone.0145161
Sexton, C. E., Betts, J. F., Demnitz, N., Dawes, H., Ebmeier, K. P., and Johansen-Berg, H. (2016). A systematic review of MRI studies examining the relationship between physical fitness and activity and the white matter of the ageing brain. Neuroimage 131, 81–90. doi: 10.1016/j.neuroimage.2015.09.071
Shah, T., Verdile, G., Sohrabi, H., Campbell, A., Putland, E., Cheetham, C., et al. (2014). A combination of physical activity and computerized brain training improves verbal memory and increases cerebral glucose metabolism in the elderly. Transl. Psychiatry 4:e487. doi: 10.1038/tp.2014.122
Shatil, E. (2013). Does combined cognitive training and physical activity training enhance cognitive abilities more than either alone? A four-condition randomized controlled trial among healthy older adults. Front. Aging Neurosci. 5:8. doi: 10.3389/fnagi.2013.00008
Simons, D. J., Boot, W. R., Charness, N., Gathercole, S. E., Chabris, C. F., Hambrick, D. Z., et al. (2016). Do ‘brain-training' programs work? Psychol. Sci. Public Interest 17, 103–186. doi: 10.1177/1529100616661983
Smith, P. J., Blumenthal, J. A., Hoffman, B. M., Cooper, H., Strauman, T. A., et al. (2010). Aerobic exercise and neurocognitive performance: a meta-analytic review of randomized control trials. Psychosom. Med. 72, 239–252. doi: 10.1097/PSY.0b013e3181d14633
Snyder, A., Anderson-Hanley, C., and Arciero, P. J. (2012). Virtual and live social facilitation while exergaming: competitiveness moderates effort. J. Sport Exerc. Psychol. 34, 252–259. doi: 10.1123/jsep.34.2.252
Stanmore, E., Stubbs, B., Vancampfort, D., de Bruin, E. D., and Firth, J. (2017). The effect of active video games on cognitive functioning in clinical and non-clinical populations: a meta-analysis of randomized controlled trials. Neurosci. Biobehav. Rev. 78, 34–43. doi: 10.1016/j.neubiorev.2017.04.011
Strauss, E., Sherman, E. M. S., and Spreen, O. (2006). A Compendium of Neuropsychological Tests, 3rd Edn. New York, NY: Oxford University Press.
Studenski, S., Carlson, M. C., Fillit, H., Greenough, W. T., Kramer, A., and Rebok, G. W. (2006). From bedside to bench: does mental and physical activity promote cognitive vitality in late life? Sci. Aging Knowledge Environ. 10:pe21. doi: 10.1126/sageke.2006.10.pe21
Styliadis, C., Kartsidis, P., Paraskevopoulos, E., Ioannides, A. A., and Bamidis, P. D. (2015). Neuroplastic effects of combined computerized physical and cognitive training in elderly individuals at risk for dementia: an eLORETA controlled study on resting states. Neural Plast. 2015, 172–192. doi: 10.1155/2015/172192
Suo, C., Singh, M. F., Gates, N., Wen, W., Sachdev, P., Brodaty, H., et al. (2016). Therapeutically relevant structural and functional mechanisms triggered by physical and cognitive exercise. Mol. Psychiatry 21, 1633–1642. doi: 10.1038/mp.2016.19
Suzuki, T., Shimada, H., Makizako, H., Doi, T., Yoshida, D., Tsutsumimoto, K., et al. (2012). Effects of multicomponent exercise on cognitive function in older adults with amnestic mild cognitive impairment: a randomized controlled trial. BMC Neurol. 12:128. doi: 10.1186/1471-2377-12-128
Szuhany, K. L., Bugatti, M., and Otto, M. W. (2014). A meta-analytic review of the effects of exercise on brain-derived neurotrophic factor. J. Psychiatr. Res. 60, 56–64. doi: 10.1016/j.jpsychires.2014.10.003
Teixeira, C. L., Rezende, T. J., Weiler, M., Nogueira, M. H., Campos, B. M., Pegoraro, L. F., et al. (2016). Relation between aerobic fitness and brain structures in amnestic mild cognitive impairment elderly. Age 38:51. doi: 10.1007/s11357-016-9912-3
ten Brinke, L. F., Bolandzadeh, N., Nagamatsu, L. S., Hsu, C. L., Davis, J. C., Miran-Khan, K., et al. (2015). Aerobic exercise increases hippocampal volume in older women with probable mild cognitive impairment: a 6-month randomised controlled trial. Br. J. Sports Med. 49, 248–254. doi: 10.1136/bjsports-2013-093184
Terry, P. C., Lane, A. M., and Fogarty, G. J. (2003). Construct validity of the Profile of Mood States-Adolescents for use with adults. Psychol. Sport Exerc. 4, 125–139. doi: 10.1016/S1469-0292(01)00035-8
Theill, N., Schumacher, V., Adelsberger, R., Martin, M., and Jäncke, L. (2013). Effects of simultaneously performed cognitive and physical training in older adults. BMC Neurosci. 14:103. doi: 10.1186/1471-2202-14-103
Thery, C., Amigorena, S., Raposo, G., and Clayton, A. (2006). Isolation and characterization of exosomes from cell culture supernatants and biological fluids. Curr. Protoc. Cell. Biol. Chapter 3:Unit 3 22. doi: 10.1002/0471143030.cb0322s30
Thielen, J., Kärgel, C., Müller, B. W., Rasche, I., Genius, J., Bus, B., et al. (2016). Aerobic activity in the healthy elderly is associated with larger plasticity in memory related brain structures and lower systemic inflammation. Front. Aging Neurosci. 8:319. doi: 10.3389/fnagi.2016.00319
Thomas, A. G., Dennis, A., Bandettini, P. A., and Johansen-Berg, H. (2012). The effects of aerobic activity on brain structure. Front. Psychol. 3:86. doi: 10.3389/fpsyg.2012.00086
Toril, P., Reales, J. M., and Ballesteros, S. (2014). Video game training enhances cognition of older adults: a meta-analytic study. Psychol. Aging 29, 706–716. doi: 10.1037/a0037507
Trachtenberg, J. T., Chen, B. E., Knott, G. W., Feng, G., Sanes, J. R., Welker, E., et al. (2002). Longterm in vivo imaging of experience-dependent synaptic plasticity in adult cortex. Nature 420, 788–794. doi: 10.1038/nature01273
Train the Brain Consortium (2017). Randomized trial on the effects of a combined physical/cognitive training in aged MCI subjects: the Train the Brain study. Sci. Rep. 7:39471. doi: 10.1038/srep39471
Tsai, S., Chen, P., Calkins, M. J., Wu, S., and Kuo, Y. (2016). Exercise counteracts aging-related memory impairment: a potential role for the astrocytic metabolic shuttle. Front. Aging Neurosci. 8:57. doi: 10.3389/fnagi.2016.00057
Van der Elst, W., Van Boxtel, M. J., Van Breukelen, G. P., and Jolles, J. (2006). The stroop color-word test: influence of age, sex, and education; and normative data for a large sample across the adult age range. Assessment 13, 62–79. doi: 10.1177/1073191105283427
van Praag, H. (2008). Neurogenesis and exercise: past and future directions. Neuromol. Med. 10, 128–140. doi: 10.1007/s12017-008-8028-z
Van Praag, H., Shubert, T., Zhao, C., and Gage, F. H. (2005). Exercise enhances learning and hippocampal neurogenesis in aged mice. J. Neurosci. 25, 8680–8685. doi: 10.1523/JNEUROSCI.1731-05.2005
Van Schaik, P., Blake, J., Pernet, F., Spears, I., and Fencott, C. (2008). Virtual augmented exercise gaming for older adults. CyberPsychol. Behav. 11, 103–106. doi: 10.1089/cpb.2007.9925
Vaughan, S., Wallis, M., Polit, D., Steele, M., Shum, D., and Morris, N. (2014). The effects of multimodal exercise on cognitive and physical functioning and brain-derived neurotrophic factor in older women: a randomised controlled trial. Age Ageing 43, 623–629. doi: 10.1093/ageing/afu010
Vellas, B., Carrie, I., Gillette-Guyonnet, S., Touchon, J., Dantoine, T., Dartigues, J. F., et al. (2014). MAPT Study: a multidomain approach for preventing Alzheimer's disease: design and baseline data. J. Prevent. Alzheimer's Dis. 1, 13–22.
Veterans Administration Medical Center (VAMC). (2007). Impaired Decision Making Capacity Screening Assessment Form. Unpublished document. Syracuse, NY: VAMC. Available online at: https://www.visn2.va.gov/VISN2/research/syr/docs/IDMC_Assesment_Form.doc
Vickers, A. J. (2005). Parametric versus non-parametric statistics in the analysis of randomized trials with non-normally distributed data. BMC Med. Res. Methodol. 5:35. doi: 10.1186/1471-2288-5-35
Vidoni, E. D., Johnson, D. K., Morris, J. K., Van Sciver, A., Greer, C. S., Billinger, S. A., et al. (2015). Dose-response of aerobic exercise on cognition: a community-based, pilot randomized controlled trial. PLoS ONE 10:e0131647. doi: 10.1371/journal.pone.0131647
Vijayakumar, N., Whittle, S., Yücel, M., Dennison, M., Simmons, J., and Allen, N. B. (2014). Thinning of the lateral prefrontal cortex during adolescence predicts emotion regulation in females. Soc. Cogn. Affect. Neurosci. 9, 1845–1854. doi: 10.1093/scan/nst183
Vital, T. M., Stein, A. M., de Melo Coelho, F. G., Arantes, F. J., Teodorov, E., and Santos-Galduróz, R. F. (2014). Physical exercise and vascular endothelial growth factor (VEGF) in elderly: a systematic review. Arch. Gerontol. Geriatr. 59, 234–239. doi: 10.1016/j.archger.2014.04.011
Voss, M. W., Prakash, R. S., Erickson, K. I., Basak, C., Chaddock, L., Kim, J. S., et al. (2010). Plasticity of brain networks in a randomized intervention trial of exercise training in older adults. Front. Aging Neurosci. 2:32. doi: 10.3389/fnagi.2010.00032
Voss, M., Vivar, C., Kramer, A. F., and van Praag, H. (2013). Bridging animal and human models of exercise-induced brain plasticity. Trends Cogn. Sci. 17, 525–544. doi: 10.1016/j.tics.2013.08.001
Wang, C., Yu, J., Wang, H., Tan, C., Meng, X., and Tan, L. (2014). Non-pharmacological interventions for patients with mild cognitive impairment: a meta-analysis of randomized controlled trials of cognition-based and exercise interventions. J. Alzheimer's Dis. 42, 663–678. doi: 10.3233/JAD-140660
Wang, P., Liu, H., Zhu, X., Meng, T., Li, H., and Zuo, X. (2016). Action video game training for healthy adults: a meta-analytic study. Front. Psychol. 7:907. doi: 10.3389/fpsyg.2016.00907
Wang, X., and Goh, D. H. (2017). Video game acceptance: a meta-analysis of the extended technology acceptance model. Cyberpsychol. Behav. Soc. Netw. 20, 662–671. doi: 10.1089/cyber.2017.0086
Wecker, N. S., Kramer, J. H., Wisniewski, A., Delis, D. C., and Kaplan, E. (2000). Age effects on executive ability. Neuropsychology 14, 409–414. doi: 10.1037/0894-4105.14.3.409
Weinstein, A. M., Voss, M. W., Prakash, R. S., Chaddock, L., Szabo, A., White, S. M., et al. (2012). The association between aerobic fitness and executive function is mediated by prefrontal cortex volume. Brain Behav. Immun. 26, 811–819. doi: 10.1016/j.bbi.2011.11.008
Willis, S. L., Tennstedt, S. L., Marsiske, M., Ball, K., Elias, J., Koepke, K. M., et al. (2006). Long-term effects of cognitive training on everyday functional outcomes in older adults. JAMA 296, 2805–2814. doi: 10.1001/jama.296.23.2805
Witwer, K. W., Buzás, E. I., Bemis, L. T., Bora, A., Lässer, C., Lötvall, J., et al. (2013). Standardization of sample collection, isolation and analysis methods in extracellular vesicle research. J. Extracell. Vesicles 2. doi: 10.3402/jev.v2i0.20360
Wong, C. N., Chaddock-Heyman, L., Voss, M. W., Burzynska, A. Z., Basak, C., Erickson, K. I., et al. (2015). Brain activation during dual-task processing is associated with cardiorespiratory fitness and performance in older adults. Front. Aging Neurosci. 7:154. doi: 10.3389/fnagi.2015.00154
Yokoyama, H., Okazaki, K., Imai, D., Yamashina, Y., Takeda, R., Naghavi, N., et al. (2015). The effect of cognitive-motor dual-task training on cognitive function and plasma amyloid beta peptide 42/40 ratio in healthy elderly persons: a randomized controlled trial. BMC Geriatr. 15:60. doi: 10.1186/s12877-015-0058-4
Young, J., Angevaren, M., Rusted, J., and Tabet, N. (2015). Aerobic exercise to improve cognitive function in older people without known cognitive impairment. Cochrane Database Syst. Rev. CD005381. doi: 10.1002/14651858.CD005381.pub4
Zatorre, R. J., Douglas Fields, R., and Johansen-Berg, H. (2012). Plasticity in gray and white: Neuroimaging changes in brain structure during learning. Nat. Neurosci. 15, 528–536. doi: 10.1038/nn.3045
Zhang, R., Zhang, Q., Niu, J., Lu, K., Xie, B., Cui, D., et al. (2014). Screening of microRNAs associated with Alzheimer's disease using oxidative stress cell model and different strains of senescence accelerated mice. J. Neurol. Sci. 338, 57–64. doi: 10.1016/j.jns.2013.12.017
Zheng, G., Xia, R., Zhou, W., Tao, J., and Chen, L. (2016). Aerobic exercise ameliorates cognitive function in older adults with mild cognitive impairment: a systematic review and meta-analysis of randomised controlled trials. Brit. J. Sports Med. doi: 10.1136/bjsports-2015-095699. [Epub ahead of print].
Zhu, X., Yin, S., Lang, M., He, R., and Li, J. (2016). The more the better? A meta-analysis on effects of combined cognitive and physical intervention on cognition in healthy older adults. Ageing Res. Rev. 31, 67–79. doi: 10.1016/j.arr.2016.07.003
Zilidou, V. I., Konstantinidis, E. I., Romanopoulou, E. D., Karagianni, M., Kartsidis, P., and Bamidis, P. D. (2016). “Investigating the effectiveness of physical training through exergames: focus on balance and aerobic protocols,” in International Conference on, Technology and Innovation in Sports, Health and Wellbeing (TISHW) (Vila Real: IEEE), 1–6.
Keywords: cognitive, exercise, aging, MCI, dementia, neuropsychological, exergame, Alzheimer's disease
Citation: Anderson-Hanley C, Barcelos NM, Zimmerman EA, Gillen RW, Dunnam M, Cohen BD, Yerokhin V, Miller KE, Hayes DJ, Arciero PJ, Maloney M and Kramer AF (2018) The Aerobic and Cognitive Exercise Study (ACES) for Community-Dwelling Older Adults With or At-Risk for Mild Cognitive Impairment (MCI): Neuropsychological, Neurobiological and Neuroimaging Outcomes of a Randomized Clinical Trial. Front. Aging Neurosci. 10:76. doi: 10.3389/fnagi.2018.00076
Received: 08 September 2017; Accepted: 07 March 2018;
Published: 04 May 2018.
Edited by:
Christos Frantzidis, Aristotle University of Thessaloniki, GreeceReviewed by:
Despina Moraitou, Aristotle University of Thessaloniki, GreeceAristea Kyriaki Ladas, University of Sheffield, United Kingdom
Copyright © 2018 Anderson-Hanley, Barcelos, Zimmerman, Gillen, Dunnam, Cohen, Yerokhin, Miller, Hayes, Arciero, Maloney and Kramer. This is an open-access article distributed under the terms of the Creative Commons Attribution License (CC BY). The use, distribution or reproduction in other forums is permitted, provided the original author(s) and the copyright owner are credited and that the original publication in this journal is cited, in accordance with accepted academic practice. No use, distribution or reproduction is permitted which does not comply with these terms.
*Correspondence: Cay Anderson-Hanley, YW5kZXJzb2NAdW5pb24uZWR1