- Department of Pharmacy, The Affiliated Xuzhou Municipal Hospital of Xuzhou Medical University, Xuzhou First People’s Hospital, Xuzhou, China
Low-grade glioma (LGG) is a prevalent and lethal primary brain malignancy, with most patients succumbing to recurrence and progression. The signal transducer and activator of transcription (STAT) family has long been implicated in tumor initiation and progression. However, a comprehensive evaluation of the expression status and overall function of STAT genes in LGG remains largely unreported. In this study, we investigated the association between the expression of STAT family genes and the progression of LGG. Through a comprehensive analysis that combined bioinformatics screening and validation assays, we determined that STAT1, STAT3, and STAT5A were upregulated and contributed to the malignant progression of LGG. Notably, our findings suggest that STAT3 is a critical prognostic marker that regulates the progression of LGG. STAT3 emerged as the most significant prognostic indicator governing the advancement of LGG. Additionally, our inquiry into the STAT3-binding proteins and differentially expressed-correlated genes (DEGs) revealed that STAT3 played a pivotal role in the progression of LGG by stimulating the expression of STAT1, FOXO1, and MYC. In summary, our recent study conducted a thorough analysis of the STAT family genes and revealed that directing therapeutic interventions towards STAT3 holds potential as a viable strategy for treating patients with LGG.
Introduction
Gliomas are the most prevalent tumors in the central nervous system, exhibiting high morbidity and mortality rates (Ostrom et al., 2021; Kang et al., 2024). Low-grade glioma (LGG), classified as World Health Organization (WHO) Grade I, II, and III glioma, encompasses astrocytomas, oligodendrogliomas, and oligoastrocytomas (Xu et al., 2019). In general, LGG patients experience a longer median overall survival period compared to those with Glioblastoma (GBM, WHO grade IV) (Xu et al., 2019). Despite advances in treatment with surgery, radiotherapy, and chemotherapy, LGG remains incurable and often progresses to secondary malignant transformation (Bao et al., 2022). Therefore, the diagnosis, monitoring, and treatment of LGG patients pose significant challenges due to the diffuse infiltration of tumor cells and their resistance to radiotherapy and chemotherapy (Hayes et al., 2018).
With the concept of precision medicine, targeted therapy and immune therapy have been proposed and used in the treatment of LGG (Bao et al., 2022; Haddad et al., 2022; Lim, 2022; Lucke-Wold et al., 2024). Drugs targeting cyclin-dependent kinase 9 (CDK9), programmed cell death protein-1 (PD-1), and other proteins have been used in clinical trials to treat glioma (Lucke-Wold et al., 2024). Despite the numerous advancements in targeted therapy and immune therapy for LGG that have been developed in the past decades, the definitive improvement in clinical diagnosis and treatment remains limited and insufficient (Haddad et al., 2022; Lim, 2022; Lucke-Wold et al., 2024). The widely poor prognosis of LGG can be attributed to the great genetic heterogeneity in their clinical behavior (Wang and Mehta, 2019; Ryall et al., 2020). Although various molecular biomarkers, including isocitrate dehydrogenase 1 (IDH1), O-6-methylguanine-DNA methyltransferase (MGMT), ADP ribosylation factor like GTPase 9 (ARL9), and chromosome arms 1p/19q deletion, have been developed, the clinical impact of these biomarkers remains to be fully elucidated (Karpel-Massler et al., 2019; van der Voort et al., 2019; Mathur et al., 2020; Tan et al., 2020). Significant advancements in comprehending the molecular intricacies of LGG have not translated into substantial improvements in the early detection and prognostication of this ailment. Consequently, the development of novel and dependable biomarkers and therapeutic targets for LGG is undeniably imperative and requires immediate attention. The STAT family of proteins, consisting of seven members (STAT1, STAT2, STAT3, STAT4, STAT5a, STAT5b, and STAT6), function as transcription factors that are crucial in various physiological cellular processes, including signal transduction from the cell membrane to the nucleus, regulation of cell proliferation, differentiation, and apoptosis (Turkson and Jove, 2000; Butturini et al., 2020; Verhoeven et al., 2020).
Accumulated research has demonstrated that the abnormal expression of members of the STAT family is associated with cancer cell transformation, metastasis, survival, and resistance to drug treatment (Calò et al., 2003; Loh et al., 2019). Certain STATs, such as STAT3 and STAT5, are considered oncogenes in a variety of tumors, including hematologic and solid tumors (Lassmann et al., 2007; Benekli et al., 2009; Gu et al., 2010). Recent studies have indicated that activated STAT1 functions as a tumor suppressor through the phosphorylation of Tyr701, and the loss of STAT1 activation or expression has been observed in malignant cells (Meissl et al., 2017). Nevertheless, certain investigations have yielded inconsistent findings, indicating that patients with elevated levels of STAT1 expression in cancerous tissues experience inferior clinical outcomes relative to those with lower levels (Khodarev et al., 2010; Magkou et al., 2012; Chou et al., 2022). Khodarev et al. regards that activation of STAT1 pathway in breast tumors confers a poor prognosis for patients (Khodarev et al., 2010). Studies have demonstrated that overexpression of phosphorylated STAT1 promotes advanced progression and poorer survival in invasive breast cancer (Magkou et al., 2012). STAT1 has been shown to play an oncogenic role in Colorectal cancer (Chou et al., 2022). This implies that STAT1 may also play a role in tumorigenesis. Conversely, STAT2 is known to exert tumor-suppressive effects through its involvement in anti-apoptotic and anti-proliferative mechanisms in certain tumors (Wang et al., 2003; Du et al., 2009; Yue et al., 2015). Gamero et al. have posited that STAT2 may also play a role in the tumorigenesis of colorectal and skin cancers (Gamero et al., 2010). Their hypothesis suggests that STAT2 may activate the oncogenic STAT3 signaling pathway, thereby promoting tumor growth. A body of literature has demonstrated that elevated levels of STAT3 expression are implicated in the development and progression of various cancers, including acute myeloid leukemia, multiple myeloma, and solid tumors affecting the breast, brain, colon, esophagus, head-and-neck, kidney, liver, lung, pancreas, and prostate (Bar-Natan et al., 2012; Lee et al., 2019).
Moreover, STAT3 has been identified as a significant oncogene responsible for the advancement of glioma tumors, and its prevalent expression in high-grade glioma (HGG) is linked to unfavorable clinical outcomes (Doucette et al., 2012). In gastric cancer, the suppression of STAT4 resulted in the inhibition of cell proliferation, migration, and invasion, indicating that STAT4 could be a promising therapeutic target for the treatment of this malignancy (Zhou et al., 2014). Analogously, similar to STAT3, the malfunctioning of inhibitory signaling pathways that regulate STAT5 activation is implicated in the pathogenesis of diverse cancers (Halim et al., 2020). Recent research has revealed a strong correlation between elevated STAT6 expression and unfavorable clinical outcomes in various types of cancer, including breast, gastric, and prostate cancer (Binnemars-Postma et al., 2018; Liu et al., 2019). These findings suggest that STAT genes hold potential as both prognostic indicators and therapeutic targets for cancer treatment. Nevertheless, a comprehensive investigation into the expression patterns and overall functionality of STAT genes in LGG remains largely unexplored.
In this study, we conducted a comprehensive analysis utilizing bioinformatics and validation assays to investigate the impact of STAT family members on the malignant progression of LGG. Our findings indicate that overexpression of STAT1, STAT3, and STAT5A contribute to the progression of LGG. Conversely, STAT4 and STAT6 were found to promote glioma progression by inhibiting the antitumor immune response. However, the effects of STAT2 and STAT5B on the malignant progression of LGG were not found to be statistically significant. Among the members considered, STAT3 emerged as the most significant prognostic signature governing the progression of LGG. Furthermore, our investigation of the STAT3-binding proteins and the differentially expression-correlated genes (DEGs) revealed that STAT3 played a pivotal role in regulating the progression of LGG by upregulating the expression of STAT1, FOXO1, and Myc. In summary, our recent study conducted a comprehensive analysis of the STAT family genes and established that targeting STAT3 represents a promising therapeutic approach for patients with LGG.
Materials and methods
Oncomine database analysis of the expression of STAT family in pan-cancer
The Oncomine online cancer gene expression data website (Oncomine Login, www.ocommine.ORG) was utilized to analyze microarray information and a collection of bioinformatic data (Kuang et al., 2022). Specifically, the mRNA expression of STATs in clinical cancer samples was compared with samples in normal sourced from the Oncomine database. Nineteen cancer types and other cancers were used to evaluate and analyze. Amony them, 463 samples were used to analyze the differential expression of STAT1, 426 samples were used to analyze the differential expression of STAT2, 452 samples were used to analyze the differential expression of STAT3, 445 samples were used to analyze the differential expression of STAT4, 415 samples were used to analyze the differential expression of STAT5A, 462 samples were used to analyze the differential expression of STAT5B, and 448 samples were used to analyze the differential expression of STAT6. The resulting p-value was determined through the application of one way ANOVA. The statistical significance of the findings was established through the identification of a p-value of 0.05 and a folding change of 2.0.
Analysis of the expression of STAT family in LGG
Firstly, the mRNA expression levels of STAT family genes were assessed in LGG tissues and normal samples through the utilization of the Gene Expression Profiling Interactive Analysis (GEPIA) two online platform (cancer-pku.cn), which incorporated expression data from the TCGA and GTEx databases. Additionally, tumor RNA sequence data was obtained from the genome data sharing (GDC) data portal (https://portal.gdc.cancer.gov/), and the expression of STAT family genes in tumors and normal tissues was subjected to a Wilcox test using R software v4.0.3. Then, the mRNA expression data for 248 grade 1 samples, 261 grade 2 samples, and 2,642 normal samples in LGG were obtained through GDC. Subsequently, Kruskal Wallis one-way ANOVA was conducted on each gene of the STAT family using R software v4.0.3. Statistical significance was determined at p < 0.05. The representative protein immunohistochemistry (IHC) staining images for each gene of the STAT family were obtained from LGG and normal tissues in the human protein atlas (HPA) (https://www.proteinatlas.org/).
Prognosis and diagnostic analysis of STAT family genes in LGG
The present study utilized the Kaplan-Meier Plotter (http://www.kmplot.com/) to assess the impact of STAT mRNA expression on patient survival. The samples were categorized into high and low expression groups based on the median expression of each gene in LGG. The overall survival and disease-free survival were evaluated using the 95% confidence interval (CI), log rank risk ratio (HR), and p-value.
ROC curves were produced and AUC values were computed using the R package pROC for ROC analysis and ggplot2 for visualization. An AUC value ranging from 0.5 to 0.7 signifies the presence of model success, while a value between 0.7 and 0.9 indicates a strong indication of model success. A value exceeding 0.9 denotes a robust indication of model success. Statistical analysis and visualization were conducted using R version 3.6.3.
Analysis of genes differentially expressed with STAT3 in LGG
The present study employed Linked Omics to identify co-expressed genes of STAT3 in LGG. Subsequently, statistical analysis was conducted on the identified co-expressed genes, which were visualized in a heat map. Pearson’s test was utilized to assess the significant correlation of the co-expressed genes. A threshold of FDR <0.01 was deemed significant for gene expression, while a threshold of p < 0.05 was considered significant for gene correlation.
Construction of a network of protein-protein interactions
The STRING database, which provides functional protein association networks, was utilized to predict protein-protein interactions (PPI) and construct the PPI network. Through this approach, the protein-protein interaction network of the STAT3 gene was predicted, revealing two primary protein clusters that exhibit close interaction. Subsequently, 50 genes were identified as direct interactors of STAT3 and subjected to KEGG enrichment analysis, resulting in the selection of 20 genes for the generation of a network diagram.
KEGG enrichment analysis
The R software package “clusterprofiler” was utilized to conduct KEGG enrichment analysis and annotations plotting. The 50 genes that interact with STAT3 protein, as constructed by PPI network, underwent enrichment and analysis by KEGG. The gene sets exhibiting significant positive and negative correlation with PTPN2 expression, as identified by linkedomics, were intersected with the 50 genes with protein interaction, respectively. Subsequently, the two intersected gene sets were merged and subjected to KEGG enrichment analysis.
Correlation analysis between the screened key genes and STAT3
The GEPIA2 online tool (cancer-pku.cn) was utilized to examine the expression correlation between the key genes of interest in the previously intersected genes and the STAT3 gene.
Analysis of the expression and prognosis of identified pivotal genes
The present study utilized the online tool GEPIA2 GEPIA 2 (cancer-pku.cn) to examine the expression and prognostic implications of selected key genes of interest among the previously identified intersection genes.
Cell culture
The LGG cancer cell lines U118 and U87, as well as the normal astrocyte HA1800 cell line, were procured from the American Type Culture Collection (ATCC) and maintained in endotoxin-free DMEM supplemented with 10% fetal bovine serum (FBS) (Gibco). The U87 cell line was cultured in MEM with 10% FBS. To prevent potential contamination, Penicillin-Streptomycin (C0222, Beyotime) and Plasmocin prophylactic (ant-mpp, InvivoGen) were added to all media in accordance with the manufacturer’s instructions.
Transient overexpression of shNC and shSTAT3
The plasmids, pGV141-shNC and pGV141-shSTAT3, were procured and constructed from Genechem Co. Ltd. (Shanghai, China). The experimental procedure involved seeding 4 × 105 cells onto a 6-well plate and allowing them to adhere to 70% confluence. Subsequently, 200 μL of transfection complex, comprising 1 μg plasmid and 1 μL X-tremeGENE HP DNA Transfection Reagent (Roche, 06366236001), was added to each well. The expressions were validated by RT-qPCR after 24–48 h of transfection. The target sequences of shSTAT3 and shNC were listed in Supplementary Table S1.
Q-PCR analysis
The RNA extraction process involved the use of RNA-easy Isolation Reagent in accordance with the manufacturer’s instructions. The extracted RNA was then subjected to reverse transcription to cDNA using HiScript® III RT SuperMix (Vazyme, R323-01) and subsequently analyzed through qRT-PCR using ChamQ SYBR qPCR Master Mix (Low ROX premix) (Vazyme, Q331-02). The qPCR assays were conducted using an ABI 7500 Fast Real-Time PCR system (Applied Biosystem, United States) and the primers utilized are presented in Supplementary Table S2. Each sample was analyzed in triplicate in this study. The relative gene expressions were determined using the 2−ΔΔCt, with GAPDH serving as the endogenous control.
Statistical analysis
Data were analyzed using SPSS. Results were expressed as means ± SD. Differences between treatment regimens were analyzed by one way ANOVA. p < 0.05 was considered statistical significance.
Results
Transcriptional levels of STATs in patients with brain and CCNS cancers
Seven STATs have been identified in mammalian cells. In this study, we utilized Oncomine databases to compare the expression levels of STATs in cancer and normal samples (Figure 1). Our findings indicate that the mRNA expression level of STAT1 is significantly upregulated in all types of cancers, with the exception of prostate cancer. The results in TCGA also support the conclusion that STAT1 is overexpressed in various cancers (Table 1). Subsequently, we compared the mRNA expression level of STAT2 in cancer and normal samples. The results indicate that STAT2 is also overexpressed in many types of cancers. Further analysis showed that there was no significant difference in STAT2 expression in LGG compared with normal tissues (Figure 1). Different from STAT2, STAT1, STAT3, STAT5A, STAT5B, and STAT6 were found to be expressed at higher levels in Brian and CCNS cancer compared to normal samples. The expression of STAT4 was lower in Brian and CCNS cancer compared to normal samples (Figure 1). Considering the small sample data of Oncomine databases, we also analyzed the differential mRNA expression levels of STATs in TCGA. The results confirmed that the expression of STAT1, STAT3, and STAT5A were higher in LGG compared to normal samples (Table 1). However, the expression of STAT4 and STAT6 were low in LGG compared to normal samples (Table 1). Subsequently, we evaluated the relationship of STATs expression and cancer malignant progression by analyzing survival and prognosis. As shown in Table 1, the overexpression of STAT family members is correlated to the malignant progression of some cancers.
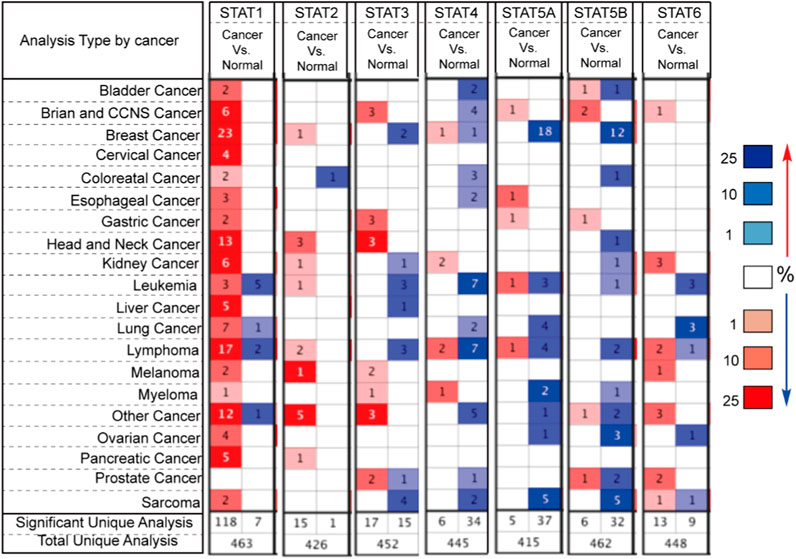
Figure 1. The Transcription Levels of STATs in Different Types of Cancers in the Oncomine database. Red and blue respectively represent cancer and normal tissues. The darker color indicates higher expression of gene. Samples from all cancers with target gene expression information used to total unique analysis. Samples from all cancers with target gene differential expression information used to significant unique analysis. The number in the square represents the number of datasets that met our screening requirements (p < 0.05).
Correlation between the mRNA levels of STATs and the clinicopathological parameters of patients with LGG
Using the Gene Expression Profiling Interactive Analysis (GEPIA) dataset and the online analytical software Xiantao Love, we conducted a comparison of mRNA expression levels of STATs factors between Brian Low-grade glioma (LGG) tissue and adjacent tissues of the TCGA project. Our findings revealed that the expression levels of STAT1, STAT3, STAT5A, and STAT6 were significantly higher in LGG tissue compared to normal tissues, while the expression level of STAT4 was lower in the former than in the latter (Figure 2A). Furthermore, we proceeded to analyze the expression of STATs with respect to tumor stage for LGG (Figure 2B). The present study revealed that the expression levels of STAT1, STAT2, STAT5A, and STAT5B were significantly elevated in LGG tissue compared to normal tissues, with higher expression levels observed in G2 stage relative to G1 stage. Additionally, the expression of STAT6 was found to be upregulated in LGG tissue at G2 stage compared to normal tissues. Conversely, the expression of STAT4 was observed to be downregulated in LGG tissue compared to normal tissues.
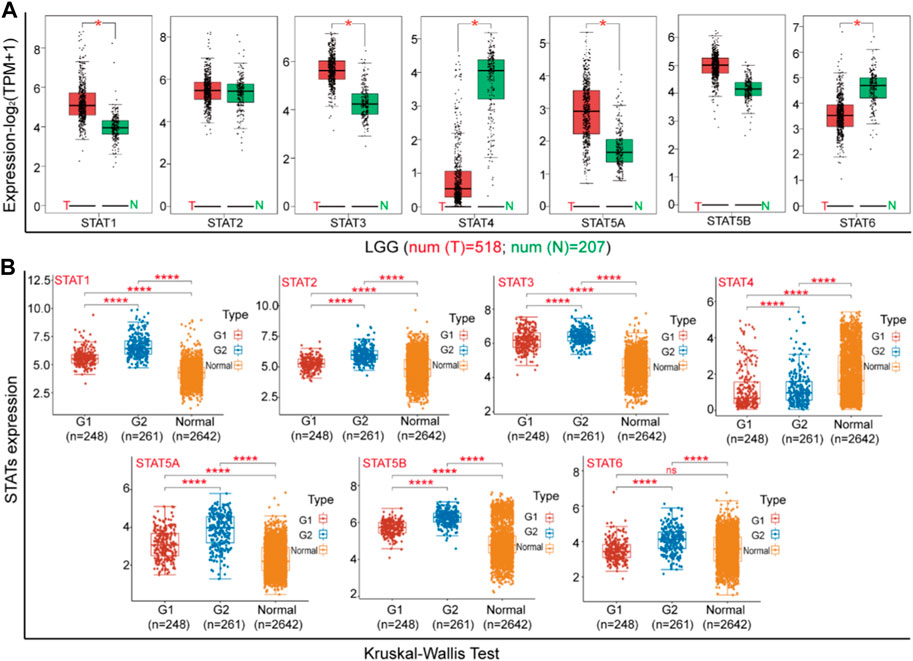
Figure 2. The expression of STATs in Brian Lower Grade Glioma (LGG). (A) The differential expression of STATs between cancerous tissues and adjacent tissues (GEPIA). (B) The expression of STATs at different stage (xiantao love).
In order to provide additional evidence for the association between STATs and the malignant advancement of LGG, an analysis was conducted to compare the expression levels of STATs in tumor tissues and normal tissues (as depicted in Figure 3). The immunohistochemical data pertaining to STATs expression in both brain glioma tissues and normal brain tissues were sourced from the human protein atlas database. Figure 3A illustrates that the expression levels of STAT1, STAT3, and STAT5A were elevated in Brian glioma tissues compared to normal Brian tissues, while the expression level of STAT4 was lower in the former than in the latter. Conversely, there were no significant differences in the expressions of STAT2 and STAT5B between Brian glioma tissues and normal Brian tissues (Figure 3A). Following this, we conducted Q-PCR to assess the expression of STATs genes in human normal astrocytes HA 1800, glioma cell lines U118 and U87 (Figure 3B). The findings indicate that the levels of STAT1, STAT3, and STAT5A were elevated in glioma cells compared to normal astrocytes, while the expression of STAT4 and STAT6 was reduced in the former relative to the latter. Conversely, there was no significant difference in the expression of STAT2 and STAT5B between these cell types.
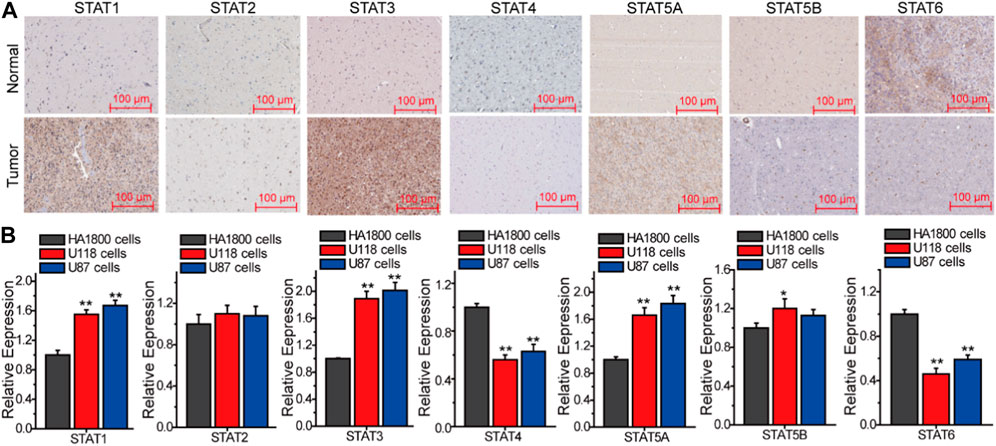
Figure 3. The expression of STATs in Gliomas. (A) The differential expression of STATs between tumor tissues and normal tissues (PROTEIN ATLAS). (B) The expression of STATs in HA 1800, U118 and U87 cells. The results from three independent experiments were statistically analyzed using one way ANOVA: *p < 0.05, **p < 0.01 compared with HA1800 cells.
Association of the mRNA expression of STATs with the improved prognosis of patients with LGG
In this study, we conducted an in-depth investigation into the crucial efficacy of STATs in relation to the malignant progression of LGG. To achieve this, we utilized GEPIA tools to analyze the correlation between the mRNA levels of STATs and the survival rates of LGG patients, using data obtained from the TCGA project (Figure 4A; Table 1). Our log rank test analyses revealed that elevated levels of STAT1, STAT2, STAT3, STAT5A, and STAT6 mRNA were significantly linked to both overall survival (OS) and disease-free survival (DFS) in all LGG patients. Furthermore, an investigation was conducted to examine the impact of STATs expression on the malignant progression of LGG through the utilization of receiver operating characteristic (ROC) curves (Figure 4B). The ROC curves of STAT1, STAT4, STAT5A, and STAT5B exhibited area under the curve (AUC) values ranging from 0.7 to 0.9, indicating that the expressions of these STATs may be associated with the progression of LGG. Additionally, the AUC value of the ROC curve of STAT3 was 0.91, signifying a positive correlation between STAT3 expression and the malignant progression of LGG (Figure 4B; Table 1).
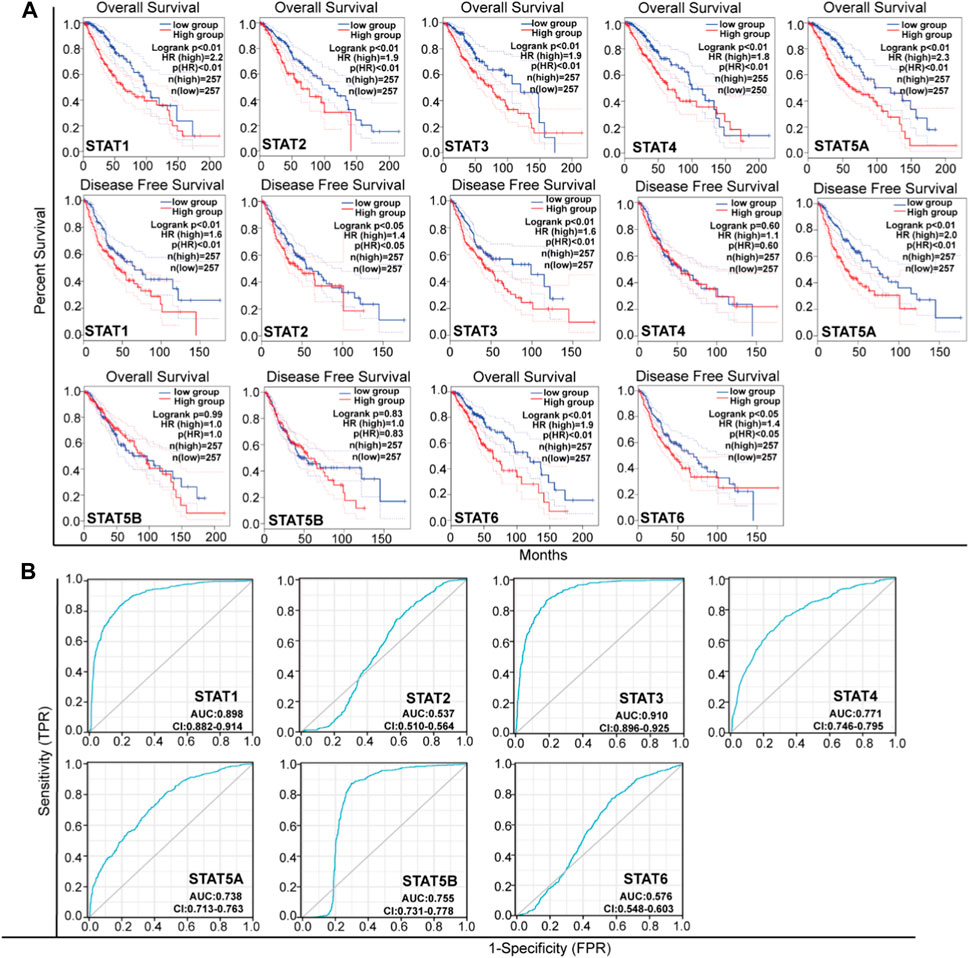
Figure 4. The Prognostic Value of mRNA level of STATs in LGG. (A) Analyze the association between patient survival and STATs expression (GEPIA). (B) ROC curves for the relationship of STATs expression and the poor prognosis of LGG. An AUC value ranging from 0.5 to 0.7 signifies the presence of model success, while a value between 0.7 and 0.9 indicates a strong indication of model success. A value exceeding 0.9 denotes a robust indication of model success.
Correlation between the expression of STAT3 and the malignant progression of LGG
The preceding analysis demonstrated a positive correlation between the expression level of STAT3 and the malignant progression of LGG. Consequently, we investigated the association between the mRNA expression of STAT3 and the hazard ratio of patients with LGG, utilizing the Xiantao love online software (Table 2). The findings indicated that the hazard ratio of patients with LGG was positively associated with the stage of LGG, the age of the patient, and the mRNA expression level of STAT3. The univariate analysis of the hazard ratio confidence interval (CI) of STAT3 was 2.41, which was significantly higher than the reference (Table 2).
The regulation of STAT3 in the malignant progression of LGG
In order to elucidate the regulatory mechanisms of STAT3 in the malignant progression of LGG, a systematic analysis was conducted on the roles of STAT3 in LGG patients utilizing the EMTome online software (Figures 5A,B). The results of the analysis revealed that 3,275 genes exhibited a negative correlation with the expression of STAT3 in LGG, while 6,680 genes exhibited a positive correlation with the expression of STAT3 in LGG, as depicted in the heat map (Figures 5A,B). Furthermore, a protein-protein interaction (PPI) network was constructed utilizing STAT3 as the central node, as illustrated in Figure 5C. In Figure 5D, it was observed that 37 genes were present in both the PPI network-related genes of STAT3 and the positively correlated genes. The Omicsmart online platform was utilized to perform KEGG pathway enrichment analysis on these 37 genes, revealing that the primary pathways associated with them were Herpesvirus infection, JAK-STAT signaling pathway, and pathways in cancer (Figure 5E). Based on the outcomes of the KEGG enrichment analysis, a subset of 15 genes that were involved in the aforementioned pathways were selected.
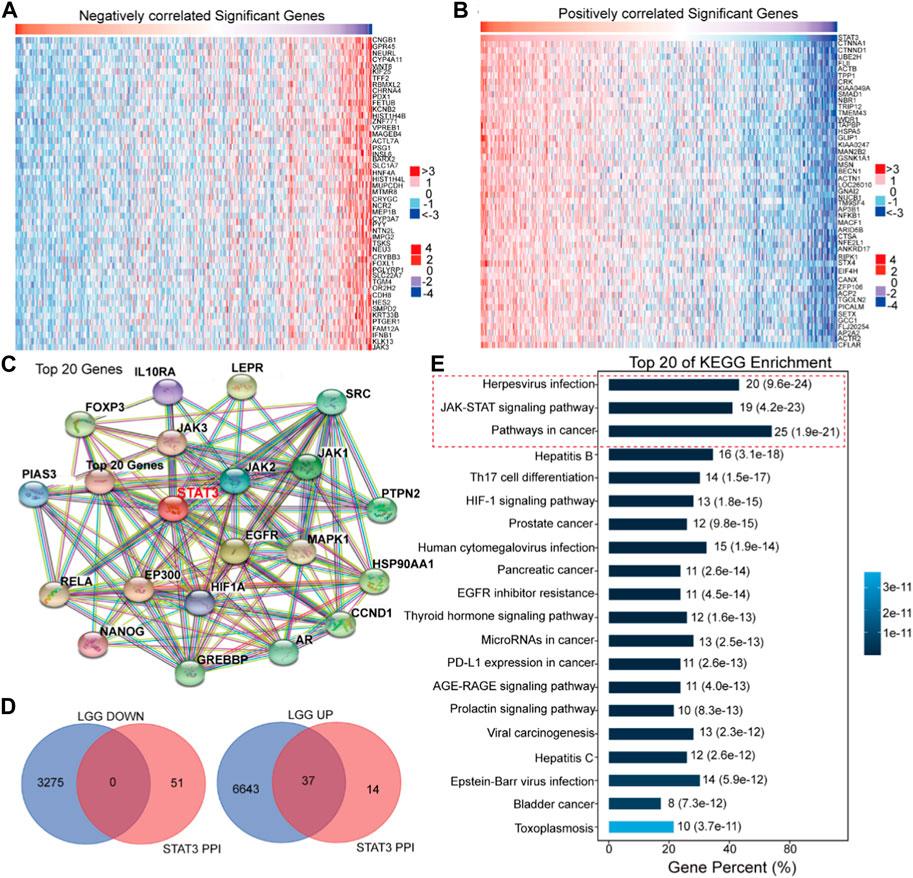
Figure 5. Bioinformatics analysis of the regulation of STAT3 in the malignant progression of LGG. (A) The negatively correlated significant genes of STAT3 in LGG (EMTome). (B) The positively correlated significant genes of STAT3 in LGG (EMTome). (C) The PPI analysis of STAT3 (STRING). (D) Online prediction the common genes in STAT3 PPI network and STAT3 correlated genes (BioVenn). (E) KEGG enrichment of the common genes (Omicshare).
In order to elucidate the correlation between STAT3 and a chosen set of 15 genes, we conducted an analysis of their respective expression patterns with respect to one another. Following correlation analysis, a positive correlation was observed between the expression of STAT3 and that of Janus kinase 2 (JAK2), AKT1, protein tyrosine phosphatase non-receptor type 2 (PTPN2), STAT1, Janus kinase 2 (JAK1), mitogen-activated protein kinase 1 (MAPK1), JUN, vascular endothelial growth factor A (VEGFA), forkhead box O1 (FOXO1), heat shock protein 90 alpha family class A member 1 (HSP90AA1), epidermal growth factor receptor (EGFR), cyclin dependent kinase inhibitor 1A (CDKN1A), cyclin D1 (CCND1), and Myc (Figure 6).
The regulation of STAT3 in LGG progression involves the participation of STAT1, FOXO1, and MYC
In order to identify the genes most likely to be involved in the regulation of STAT3 in LGG malignant progression, an analysis was conducted on the differential expressions of 14 genes in LGG tissues and normal adjacent tissues (as depicted in Figure 7A). The mRNA expressions of STAT1, CDKN1A, EGFR, FOXO1, CCND1, MAPK1, Myc, and JAK1 were found to be higher in LGG tissues than in normal adjacent tissues. Subsequently, the correlation between the mRNA levels of these eight genes and the survival of patients with LGG was analyzed (as shown in Figure 7A). The results of the log rank test analyses indicate a significant association between increased levels of STAT1, FOXO1, and Myc and the overall survival (OS) and disease-free survival (DFS) of LGG, as depicted in Figures 7B,C.
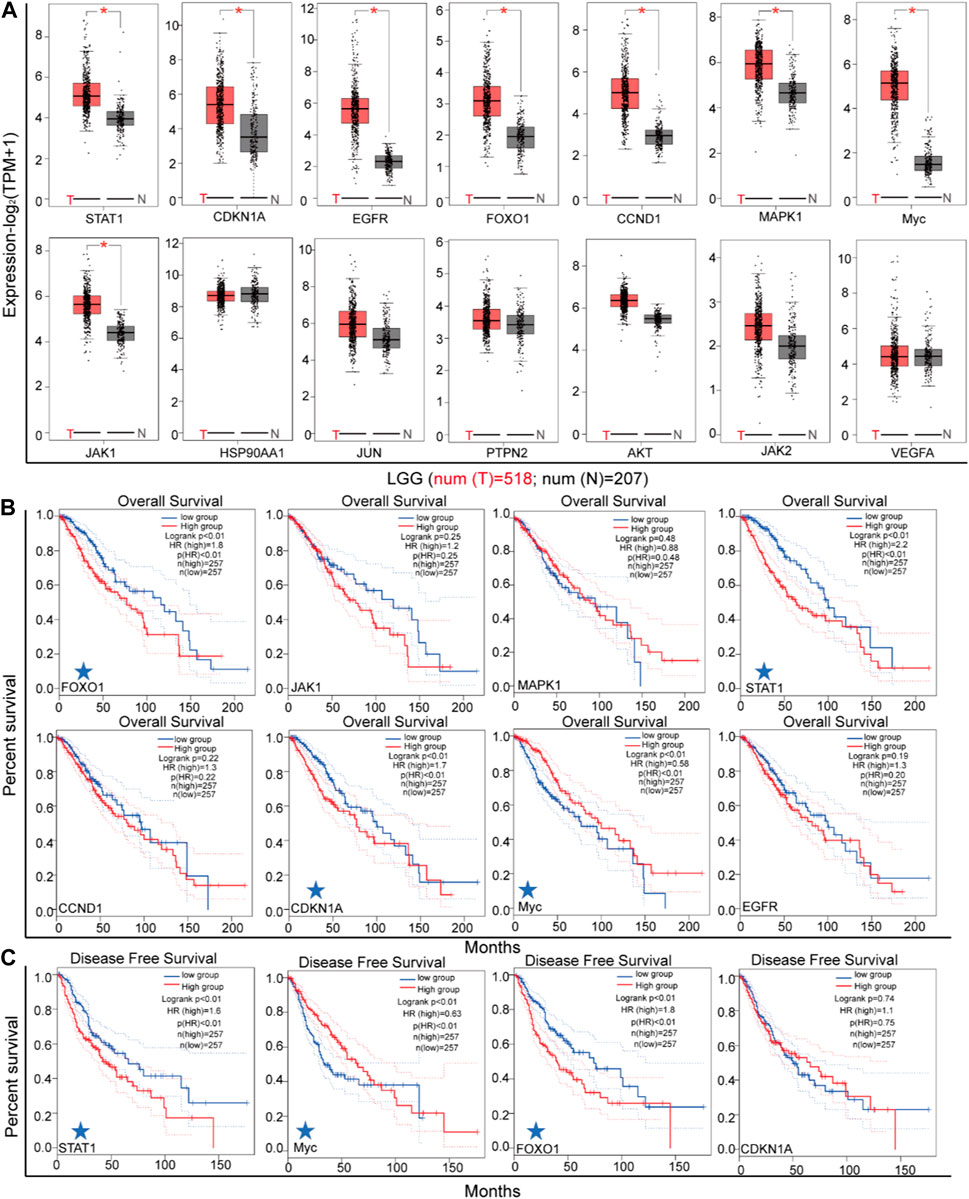
Figure 7. The expression of key genes in LGG. (A) The differential expression of key genes between LGG tissues and adjacent tissues (GEPIA). (B) The association between patient overall survival and genes expression (GEPIA). (C) The association between disease free survival and genes expression (GEPIA).
In order to further validate the involvement of STAT1, FOXO1, and Myc as key genes in the STAT3-regulated malignant progression of LGG, we conducted Q-PCR analysis to assess the expression of these genes under different conditions, as illustrated in Figure 8. Our findings demonstrate a significant increase in the expression of STAT1, FOXO1, and Myc in glioma cells U118 and U87, as compared to normal astrocytes HA 1800 (Figure 8A). The inhibition of STAT3 expression in U118 and U87 glioma cells resulted in a significant reduction in the expressions of STAT1, FOXO1, and MYC (Figures 8B,C). These findings indicate that STAT1, FOXO1, and Myc are genes that play a role in the STAT3-regulated signaling pathway.
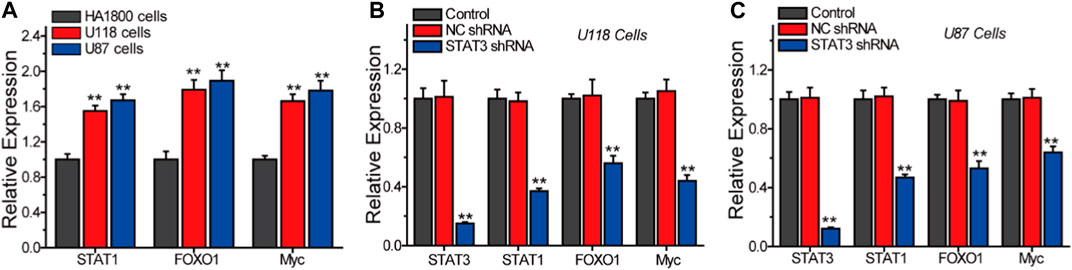
Figure 8. Q-PCR analyze the relative expression of STAT3, STAT1, FOXO1 and Myc in different cells. (A) The expression of STAT1, FOXO1 and Myc in HA 1800, U118 and U87 cells. The effects of STAT3 on the expression of STAT1, FOXO1 and Myc in U118 cells (B) and U87 cells (C).
Discussion
LGGs, being the most prevalent brain tumors in children, are currently classified by the World Health Organisation as grades I and II (Ryall et al., 2020). The diagnosis and treatment of patients with LGGs pose a challenge due to the heterogeneity in their clinical behavior (Wang and Mehta, 2019; Ryall et al., 2020). Presently, the primary strategies for glioma treatment include surgery, chemotherapy, and/or radiotherapy. Nevertheless, the adverse effects and drug resistance associated with chemotherapy and radiotherapy often lead to treatment failures (Wang and Mehta, 2019). Hence, the identification of biomarkers and therapeutic targets for gliomas is of paramount importance. The dysregulation of STAT family members has been documented in numerous cancers, indicating their involvement in cancer progression by integrating signals from diverse signaling pathways (Loh et al., 2019; Verhoeven et al., 2020). Therefore, investigating the association between STAT expression and the malignant progression of gliomas is imperative to identify potential targets for diagnosis and treatment. In this study, we examine the correlation between STAT expression and the progression of LGG.
Each member of the STAT family performs distinct functions in signal transduction and facilitates cellular responses to various cytokines (Loh et al., 2019). Our research has revealed that STAT1 is frequently upregulated in numerous cancers and is positively correlated with the malignant progression of LGG (Yang et al., 2020). While the majority of evidence suggests that STAT1 functions as a tumor suppressor in cancer cells, some studies have indicated that under specific conditions, STAT1 may exert tumor promoter effects (Yang et al., 2020). Hence, it is plausible that STAT1 may act as a tumor promoter in gliomas. Conversely, the mRNA expression of STAT2 does not appear to be associated with the malignant progression of LGG. The constitutive activation of STAT3 is essential for the carcinogenesis of head and neck cancer, and there is evidence to suggest that STAT3 is involved in the transition from LGG to high grade glioma (Doucette et al., 2012; Lee et al., 2019). Our research indicates that STAT3 is overexpressed in LGG and contributes to the malignant progression of gliomas. Analogous to STAT3, dysregulated STAT5 signaling has been linked to elevated cell proliferation, survival, and metastasis in various cancers (Halim et al., 2020). STAT5 comprises two subtypes, namely, STAT5A and STAT5B (Zhang and Liu, 2017). Our investigation revealed that the expression and biological activity of STAT5A and STAT5B differed. Specifically, STAT5A was found to be upregulated in LGG tissues and exhibited a positive correlation with the malignant advancement of gliomas. The correlation between STAT5B and the progression of LGG was found to be insignificant. Our study has emphasized the predominant role of STAT5A in gliomas. The expression and function of STAT4 and STAT6 in gliomas is a topic of interest. Upon analysis, it was determined that the mRNA expression of STAT4 and STAT6 was comparatively lower in glioma tissues as opposed to adjacent tissues. However, the results of survival analysis indicate that the overexpression of STAT4 and STAT6 promote the malignant progression of LGG. STAT4 is known to localize to the cytoplasm and bind to the membrane following phosphorylation. The activation of STAT4 is deemed critical for the promotion of cellular-mediated immune response through multiple signaling pathways (Maurer et al., 2019). STAT6 has been linked to tumorigenesis, immunosuppression, proliferation, metastasis, and unfavorable prognosis (Huang et al., 2020). As pro-cancer factors, although the expression levels of STAT4 and STAT6 are relative low, they may play an important role in the malignant transformation of LGG and the occurrence of drug resistance. As we know, LGG often progresses to secondary malignant transformation (Lucke-Wold et al., 2024). In this malignant transformation, the expression levels of STAT4 and STAT6 may be elevated, which further promotes this malignant transformation. Meanwhile, LGG easily develops resistance to radiotherapy, chemotherapy, and immunotherapy (Hayes et al., 2018). The expression levels of STAT4 and STAT6 may also increase to promote the process of LGG developing drug resistance. Therefore, it is worth paying attention to evaluate the expression levels of STAT4 and STAT6 during the malignant transformation of LGG. Among the STAT family members, STAT3 exhibited the strongest effect on the malignancy of gliomas, and thus, was selected for further analysis.
Upon analysis, it was determined that 6,680 genes exhibited a positive correlation with the expression of STAT3 in LGG. Among these genes, 37 were found to be involved in the STAT3 PPI network. Through systematic analysis, it was discovered that STAT1, FOXO1, and MYC were pivotal factors in the regulation of STAT3 during the progression of LGG. The expression levels of STAT1, FOXO1, and MYC were observed to be higher in gliomas compared to normal astrocytes and exhibited a positive correlation with the expression of STAT3. The prevailing consensus is that the transcription factor FOXO1 impedes cancer progression through the activation of cell apoptosis and inhibition of cell metastasis (Kim et al., 2018). Some studies have shown that FOXO1 plays a pivotal role as a tumor suppressor in lung cancer (Ebrahimnezhad et al., 2023). However, many researches suggest that FOXO1 promotes the malignant progression of leukemia (Liu et al., 2019). Tomiyasu et al also regard that FOXO1 supports the malignant proliferation of breast cancer cells that and coloreatal cancer cells by promoting p53 degradation (Tomiyasu et al., 2024). Hence, the biological role of FOXO1 in cancer progression is contingent upon the tumor type (Kim et al., 2018; Liu et al., 2019; Ebrahimnezhad et al., 2023; Tomiyasu et al., 2024). Specifically, the mRNA expression level of FOXO1 exhibited a positive correlation with the progression of LGG, and the repression of FOXO1 was induced by the silencing of STAT3. The induction of FOXO1 by STAT3 has been found to promote the malignant progression of LGG. The deregulation of the oncogene MYC is a common occurrence in many cancers and is often associated with poor prognosis and unfavorable survival (Chen et al., 2018). Our research has revealed that MYC is overexpressed in LGG tissues and that repression of STAT3 leads to a decrease in Myc expression. These findings suggest that STAT3 plays a crucial role in the malignant progression of LGG by regulating the expression of STAT1, FOXO1, and MYC.
Conclusion
In brief, the present study examined the impact of STAT family members on the malignant advancement of LGG. Our findings indicate that STAT1, STAT3, and STAT5A were upregulated and facilitated the progression of LGG. Conversely, STAT4 and STAT6 were found to promote glioma progression by inhibiting the antitumor immune response. The effects of STAT2 and STAT5B on the malignant progression of LGG were deemed insignificant. Among these members, STAT3 was identified as the most significant factor in regulating the progression of LGG by facilitating the expression of STAT1, FOXO1, and MYC. STAT3, STAT1, FOXO1, and MYC, play a crucial role in driving the malignant progression of LGG. Therefore, targeting STAT3 represents a promising therapeutic strategy for patients afflicted with gliomas.
Data availability statement
The datasets presented in this study can be found in online repositories. The names of the repository/repositories and accession number(s) can be found below: https://doi.org/10.6084/m9.figshare.26027179.v1.
Ethics statement
Ethical approval was not required for the studies on humans in accordance with the local legislation and institutional requirements because only commercially available established cell lines were used. Ethical approval was not required for the studies on animals in accordance with the local legislation and institutional requirements because only commercially available established cell lines were used.
Author contributions
CL: Writing–review and editing, Writing–original draft, Supervision, Project administration, Funding acquisition. YL: Writing–original draft, Data curation. FJ: Writing–original draft, Formal Analysis. SZ: Writing–original draft, Formal Analysis. HJ: Writing–review and editing, Writing–original draft, Funding acquisition.
Funding
The author(s) declare that financial support was received for the research, authorship, and/or publication of this article. This study was supported by Project Foundation of the Xuzhou Municipal Health Commission (XWYXKY202209), Science and Technology Plane Project Foundation of Xuzhou (KC22145).
Conflict of interest
The authors declare that the research was conducted in the absence of any commercial or financial relationships that could be construed as a potential conflict of interest.
Publisher’s note
All claims expressed in this article are solely those of the authors and do not necessarily represent those of their affiliated organizations, or those of the publisher, the editors and the reviewers. Any product that may be evaluated in this article, or claim that may be made by its manufacturer, is not guaranteed or endorsed by the publisher.
Supplementary material
The Supplementary Material for this article can be found online at: https://www.frontiersin.org/articles/10.3389/fmolb.2024.1419072/full#supplementary-material
References
Bao, J.-H., Lu, W.-C., Duan, H., Ye, Y.-Q., Li, J.-B., Liao, W.-T., et al. (2022). Identification of a novel cuproptosis-related gene signature and integrative analyses in patients with lower-grade gliomas. Front. Immunol. 13, 933973. doi:10.3389/fimmu.2022.933973
Bar-Natan, M., Nelson, E. A., Xiang, M., and Frank, D. A. (2012). STAT signaling in the pathogenesis and treatment of myeloid malignancies. JAKSTAT 1 (2), 55–64. doi:10.4161/jkst.20006
Benekli, M., Baumann, H., and Wetzler, M. (2009). Targeting signal transducer and activator of transcription signaling pathway in leukemias. J. Clin. Oncol. 27 (26), 4422–4432. doi:10.1200/JCO.2008.21.3264
Binnemars-Postma, K., Bansal, R., Storm, G., and Prakash, J. (2018). Targeting the Stat6 pathway in tumor-associated macrophages reduces tumor growth and metastatic niche formation in breast cancer. FASEB J. 32 (2), 969–978. doi:10.1096/fj.201700629R
Butturini, E., Carcereri de Prati, A., and Mariotto, S. (2020). Redox regulation of STAT1 and STAT3 signaling. Int. J. Mol. Sci. 21 (19), 7034–7462. doi:10.3390/ijms21197034
Calò, V., Migliavacca, M., Bazan, V., Macaluso, M., Buscemi, M., Gebbia, N., et al. (2003). STAT proteins: from normal control of cellular events to tumorigenesis. J. Cell Physiol. 197 (2), 157–168. doi:10.1002/jcp.10364
Chen, H., Liu, H., and Qing, G. (2018). Targeting oncogenic Myc as a strategy for cancer treatment. Signal Transduct. Target Ther. 3, 5. doi:10.1038/s41392-018-0008-7
Chou, P.-H., Luo, C.-K., Wali, N., Lin, W.-Y., Ng, S.-K., Wang, C.-H., et al. (2022). A chemical probe inhibitor targeting STAT1 restricts cancer stem cell traits and angiogenesis in colorectal cancer. J. Biomed. Sci. 29 (1), 20. doi:10.1186/s12929-022-00803-4
Doucette, T. A., Kong, L. Y., Yang, Y., Ferguson, S. D., Yang, J., Wei, J., et al. (2012). Signal transducer and activator of transcription 3 promotes angiogenesis and drives malignant progression in glioma. Neuro Oncol. 14 (9), 1136–1145. doi:10.1093/neuonc/nos139
Du, Z., Fan, M., Kim, J. G., Eckerle, D., Lothstein, L., Wei, L., et al. (2009). Interferon-resistant Daudi cell line with a Stat2 defect is resistant to apoptosis induced by chemotherapeutic agents. J. Biol. Chem. 284 (41), 27808–27815. doi:10.1074/jbc.M109.028324
Ebrahimnezhad, M., Natami, M., Bakhtiari, G. H., Tabnak, P., Ebrahimnezhad, N., Yousefi, B., et al. (2023). FOXO1, a tiny protein with intricate interactions: promising therapeutic candidate in lung cancer. Biomed. Pharmacother. 169, 115900. doi:10.1016/j.biopha.2023.115900
Gamero, A. M., Young, M. R., Mentor-Marcel, R., Bobe, G., Scarzello, A. J., Wise, J., et al. (2010). STAT2 contributes to promotion of colorectal and skin carcinogenesis. Cancer Prev. Res. (Phila) 3 (4), 495–504. doi:10.1158/1940-6207.CAPR-09-0105
Gu, L., Vogiatzi, P., Puhr, M., Dagvadorj, A., Lutz, J., Ryder, A., et al. (2010). Stat5 promotes metastatic behavior of human prostate cancer cells in vitro and in vivo. Endocr. Relat. Cancer 17 (2), 481–493. doi:10.1677/ERC-09-0328
Haddad, A. F., Young, J. S., Oh, J. Y., Okada, H., and Aghi, M. K. (2022). The immunology of low-grade gliomas. Neurosurg. Focus 52 (2), E2. doi:10.3171/2021.11.FOCUS21587
Halim, C. E., Deng, S., Ong, M. S., and Yap, C. T. (2020). Involvement of STAT5 in oncogenesis. Biomedicines 8 (9), 316. doi:10.3390/biomedicines8090316
Hayes, J., Yu, Y., Jalbert, L. E., Mazor, T., Jones, L. E., Wood, M. D., et al. (2018). Genomic analysis of the origins and evolution of multicentric diffuse lower-grade gliomas. Neuro Oncol. 20 (5), 632–641. doi:10.1093/neuonc/nox205
Huang, H., Zheng, Y., Li, L., Shi, W., Zhang, R., Liu, H., et al. (2020). The roles of post-translational modifications and coactivators of STAT6 signaling in tumor growth and progression. Future Med. Chem. 12 (21), 1945–1960. doi:10.4155/fmc-2020-0224
Kang, Q.-M., Wang, J., Chen, S.-M., Song, S.-R., and Yu, S.-C. (2024). Glioma-associated mesenchymal stem cells. Brain 147 (3), 755–765. doi:10.1093/brain/awad360
Karpel-Massler, G., Nguyen, T. T. T., Shang, E., and Siegelin, M. D. (2019). Novel IDH1-targeted glioma therapies. CNS Drugs 33 (12), 1155–1166. doi:10.1007/s40263-019-00684-6
Khodarev, N., Ahmad, R., Rajabi, H., Pitroda, S., Kufe, T., McClary, C., et al. (2010). Cooperativity of the MUC1 oncoprotein and STAT1 pathway in poor prognosis human breast cancer. Oncogene 29 (6), 920–929. doi:10.1038/onc.2009.391
Kim, C. G., Lee, H., Gupta, N., Ramachandran, S., Kaushik, I., Srivastava, S., et al. (2018). Role of Forkhead Box Class O proteins in cancer progression and metastasis. Semin. Cancer Biol. 50, 142–151. doi:10.1016/j.semcancer.2017.07.007
Kuang, W., Wang, X., Ding, J., Li, J., Ji, M., Chen, W., et al. (2022). PTPN2, A key predictor of prognosis for pancreatic adenocarcinoma, significantly regulates cell cycles, apoptosis, and metastasis. Front. Immunol. 13, 805311. doi:10.3389/fimmu.2022.805311
Lassmann, S., Schuster, I., Walch, A., Göbel, H., Jütting, U., Makowiec, F., et al. (2007). STAT3 mRNA and protein expression in colorectal cancer: effects on STAT3-inducible targets linked to cell survival and proliferation. J. Clin. Pathol. 60 (2), 173–179. doi:10.1136/jcp.2005.035113
Lee, H., Jeong, A. J., and Ye, S. K. (2019). Highlighted STAT3 as a potential drug target for cancer therapy. BMB Rep. 52 (7), 415–423. doi:10.5483/BMBRep.2019.52.7.152
Lim, Y. J. (2022). Medical treatment of pediatric low-grade glioma. Brain tumor Res. Treat. 10 (4), 221–225. doi:10.14791/btrt.2022.0039
Liu, H., Ni, Z., Shi, L., Ma, L., and Zhao, J. (2019). MiR-486-5p inhibits the proliferation of leukemia cells and induces apoptosis through targeting FOXO1. Mol. Cell Probes 44, 37–43. doi:10.1016/j.mcp.2019.02.001
Loh, C. Y., Arya, A., Naema, A. F., Wong, W. F., Sethi, G., and Looi, C. Y. (2019). Signal transducer and activator of transcription (STATs) proteins in cancer and inflammation: functions and therapeutic implication. Front. Oncol. 9, 48. doi:10.3389/fonc.2019.00048
Lucke-Wold, B., Rangwala, B. S., Shafique, M. A., Siddiq, M. A., Mustafa, M. S., Danish, F., et al. (2024). Focus on current and emerging treatment options for glioma: a comprehensive review. World J. Clin. Oncol. 15 (4), 482–495. doi:10.5306/wjco.v15.i4.482
Magkou, C., Giannopoulou, I., Theohari, I., Fytou, A., Rafailidis, P., Nomikos, A., et al. (2012). Prognostic significance of phosphorylated STAT-1 expression in premenopausal and postmenopausal patients with invasive breast cancer. Histopathology 60 (7), 1125–1132. doi:10.1111/j.1365-2559.2011.04143.x
Mathur, R., Zhang, Y., Grimmer, M. R., Hong, C., Zhang, M., Bollam, S., et al. (2020). MGMT promoter methylation level in newly diagnosed low-grade glioma is a predictor of hypermutation at recurrence. Neuro Oncol. 22 (11), 1580–1590. doi:10.1093/neuonc/noaa059
Maurer, B., Kollmann, S., Pickem, J., Hoelbl-Kovacic, A., and Sexl, V. (2019). STAT5A and STAT5B-twins with different personalities in hematopoiesis and leukemia. Cancers (Basel) 11 (11), 1726. doi:10.3390/cancers11111726
Meissl, K., Macho-Maschler, S., Müller, M., and Strobl, B. (2017). The good and the bad faces of STAT1 in solid tumours. Cytokine 89, 12–20. doi:10.1016/j.cyto.2015.11.011
Ostrom, Q. T., Cioffi, G., Waite, K., Kruchko, C., and Barnholtz-Sloan, J. S. (2021). CBTRUS statistical report: primary brain and other central nervous system tumors diagnosed in the United States in 2014–2018. Neuro Oncol. 23 (12), iii1–iii105. doi:10.1093/neuonc/noab200
Ryall, S., Tabori, U., and Hawkins, C. (2020). Pediatric low-grade glioma in the era of molecular diagnostics. Acta Neuropathol. Commun. 8 (1), 30. doi:10.1186/s40478-020-00902-z
Tan, Y., Zhang, S., Xiao, Q., Wang, J., Zhao, K., Liu, W., et al. (2020). Prognostic significance of ARL9 and its methylation in low-grade glioma. Genomics 112 (6), 4808–4816. doi:10.1016/j.ygeno.2020.08.035
Tomiyasu, H., Habara, M., Hanaki, S., Sato, Y., Miki, Y., and Shimada, M. (2024). FOXO1 promotes cancer cell growth through MDM2-mediated p53 degradation. J. Biol. Chem. 300 (4), 107209. doi:10.1016/j.jbc.2024.107209
Turkson, J., and Jove, R. (2000). STAT proteins: novel molecular targets for cancer drug discovery. Oncogene 19 (56), 6613–6626. doi:10.1038/sj.onc.1204086
van der Voort, S. R., Incekara, F., Wijnenga, M. M. J., Kapas, G., Gardeniers, M., Schouten, J. W., et al. (2019). Predicting the 1p/19q codeletion status of presumed low-grade glioma with an externally validated machine learning algorithm. Clin. Cancer Res. 25 (24), 7455–7462. doi:10.1158/1078-0432.CCR-19-1127
Verhoeven, Y., Tilborghs, S., Jacobs, J., De Waele, J., Quatannens, D., Deben, C., et al. (2020). The potential and controversy of targeting STAT family members in cancer. Semin. Cancer Biol. 60, 41–56. doi:10.1016/j.semcancer.2019.10.002
Wang, J., Pham-Mitchell, N., Schindler, C., and Campbell, I. L. (2003). Dysregulated Sonic hedgehog signaling and medulloblastoma consequent to IFN-alpha-stimulated STAT2-independent production of IFN-gamma in the brain. J. Clin. Invest. 112 (4), 535–543. doi:10.1172/JCI18637
Wang, T. J. C., and Mehta, M. P. (2019). Low-grade glioma radiotherapy treatment and trials. Neurosurg. Clin. N. Am. 30 (1), 111–118. doi:10.1016/j.nec.2018.08.008
Xu, Y., Geng, R., Yuan, F., Sun, Q., Liu, B., and Chen, Q. (2019). Identification of differentially expressed key genes between glioblastoma and low-grade glioma by bioinformatics analysis. Peer J. 7, e6560. doi:10.7717/peerj.6560
Yang, C., Mai, H., Peng, J., Zhou, B., Hou, J., and Jiang, D. (2020). STAT4: an immunoregulator contributing to diverse human diseases. Int. J. Biol. Sci. 16 (9), 1575–1585. doi:10.7150/ijbs.41852
Yue, C., Xu, J., Tan Estioko, M. D., Kotredes, K. P., Lopez-Otalora, Y., Hilliard, B. A., et al. (2015). Host STAT2/type I interferon axis controls tumor growth. Int. J. Cancer 136 (1), 117–126. doi:10.1002/ijc.29004
Keywords: LGG, STAT3, FoxO1, STAT1, prognosis
Citation: Li Y, Jiang F, Zhu S, Jia H and Li C (2024) STAT3 drives the malignant progression of low-grade gliomas through modulating the expression of STAT1, FOXO1, and MYC. Front. Mol. Biosci. 11:1419072. doi: 10.3389/fmolb.2024.1419072
Received: 17 April 2024; Accepted: 06 May 2024;
Published: 14 June 2024.
Edited by:
Lei Huang, University of Massachusetts Medical School, United StatesReviewed by:
Yue Liu, The University of Texas at Austin, United StatesHaocai Chang, South China Normal University, China
Jing Wang, West Virginia University, United States
Copyright © 2024 Li, Jiang, Zhu, Jia and Li. This is an open-access article distributed under the terms of the Creative Commons Attribution License (CC BY). The use, distribution or reproduction in other forums is permitted, provided the original author(s) and the copyright owner(s) are credited and that the original publication in this journal is cited, in accordance with accepted academic practice. No use, distribution or reproduction is permitted which does not comply with these terms.
*Correspondence: Hongwei Jia, MTUxNjIxNDMwOTNAMTYzLmNvbQ==; Changwei Li, MTU4NjIyNzg5MDlAMTYzLmNvbQ==
†These authors have contributed equally to this work