- 1Department of Orthopedic Surgery, the Second Affiliated Hospital, Zhejiang University School of Medicine, Hangzhou, Zhejiang, China
- 2Orthopedics Research Institute of Zhejiang University, Hangzhou, Zhejiang, China
- 3Key Laboratory of Motor System Disease Research and Precision Therapy of Zhejiang Province, Hangzhou, Zhejiang, China
- 4Clinical Research Center of Motor System Disease of Zhejiang Province, Hangzhou, China
- 5Department of Plastic Surgery, The Fourth Affiliated Hospital, Zhejiang University School of Medicine, Yiwu, Zhejiang, China
Skeletal diseases impose a considerable burden on society. The clinical and tissue-engineering therapies applied to alleviate such diseases frequently result in complications and are inadequately effective. Research has shifted from conventional therapies based on mesenchymal stem cells (MSCs) to exosomes derived from MSCs. Exosomes are natural nanocarriers of endogenous DNA, RNA, proteins, and lipids and have a low immune clearance rate and good barrier penetration and allow targeted delivery of therapeutics. MSC-derived exosomes (MSC-exosomes) have the characteristics of both MSCs and exosomes, and so they can have both immunosuppressive and tissue-regenerative effects. Despite advances in our knowledge of MSC-exosomes, their regulatory mechanisms and functionalities are unclear. Here we review the therapeutic potential of MSC-exosomes for skeletal diseases.
Introduction
The prevalences of musculoskeletal ailments are increasing with the average life expectancy (Pourakbari et al., 2019; Malekpour et al., 2022). Around 1.71 billion people globally have musculoskeletal conditions in 2019, necessitating increasingly complex treatment modalities (Cieza et al., 2021). Osteoarthritis (OA), osteoporosis (OP), intervertebral disc degeneration (IDD), fracture, bone defects, and rheumatoid arthritis (RA) are among the most prevalent skeletal diseases; these not only impose a considerable financial burden on patients but also diminish their overall wellbeing (Li et al., 2018; He et al., 2021; Ding et al., 2023; Torrecillas-Baena et al., 2023). A systematic review pointed out that the global prevalence of OP was reported to be over 23.1% in women (Salari et al., 2021). In China, about 20.6% of females above 40 years old were suffering from OP (Wang et al., 2021). For OA, the mean cost of working-age OA patients is reported to be $14,521 per year. Moreover, the estimated prevalence in adult population is up to 26% by 2040 (Lo et al., 2021). Research in tissue bioengineering has led to the development of liposomes, dendrimers, micelles, and inorganic nanoparticles, which augment the effectiveness of drugs while mitigating their systemic toxicity. However, the clinical application of these agents is hampered by their cytotoxicity and poor biodegradability. Therefore, it is important to develop novel therapeutics that can overcome the above limitations and ameliorate skeletal diseases.
Mesenchymal stem cells (MSCs) have therapeutic potential for skeletal diseases (Wang et al., 2022; Yu et al., 2022; Torrecillas-Baena et al., 2023). The multipotent characteristics of MSCs enable them to undergo self-renewal and differentiate into multiple lineages. Furthermore, they migrate toward injured areas and secrete growth factors, thereby facilitating wound healing (Gholami Farashah et al., 2022; Malekpour et al., 2022). MSC transplantation therapy (MSCT) shows considerable promise for the treatment of diverse ailments. However, the hypopermeability and low blood circulation of bone impedes the application of MSCT for skeletal diseases (Shang et al., 2021). In addition, the survival of engrafted MSCs is poor, and the effects of MSCT are characterized by the paracrine release of cytokines, and exosomes rather than the direct actions of the cells themselves (Vitha et al., 2019; Wang et al., 2022).
Exosomes, characterized as scale extracellular vesicles with diameters ranging from approximately 30–150 nm (Kubiatowicz et al., 2022), are released by various cellular entities. They transport biologically active endogenous and exogenous factors such as nucleic acids, proteins, lipids, oligonucleotides, therapeutic RNAs, and small molecules, thereby modulating physiological and pathological processes (Shang et al., 2021; Gholami Farashah et al., 2022). As natural nanocarriers, they transport endogenous factors and have a low immune-clearance rate, good barrier penetration, and allow targeted delivery of therapeutics. MSC-derived exosomes (MSC-exosomes) modulate the functionality of recipient cells by conveying information in the form of constituents of MSCs (Tan et al., 2020; Torrecillas-Baena et al., 2023). Bone marrow-derived MSCs are typically used to produce MSC-exosomes. Compared to MSCT, therapies based on MSC-exosomes have enhanced safety and more convenient storage, transportation, and administration. Consequently, the clinical potential of MSC-exosomes warrants further investigation. The isolation, engineering, cargo loading, and boosting of exosomes have been investigated (Zhang et al., 2019; Wang et al., 2023). This review primarily concentrates on the recent advancements in the utilization of MSC-exosomes for the therapeutic intervention of skeletal disorders including OP, OA, RA, fracture, and IDD. In addition, we discuss barriers to their clinical application (Figure 1).
The biogenesis and composition of exosomes
Exosome generation involves plasma membrane double invagination and the subsequent synthesis of intracellular multivesicular bodies (MVBs) that harbor intraluminal vesicles (ILVs) (Wang and Thomsen, 2021; Wang et al., 2022). The initial plasma membrane invagination gives rise to a structure that contains cell-surface and extracellular proteins, leading to the de novo creation of early sorting endosomes (ESEs), which subsequently become late-sorting endosomes (LSEs) and ultimately generate MVBs. The endoplasmic reticulum also plays a role in the synthesis and content of ESEs. MVBs can undergo fusion with lysosomes, leading to their degradation, resulting in the release of enclosed ILVs as exosomes (Vig and Fernandes, 2022). There are different up-take mechanisms, including fusion, internalization by endocytosis, phagocytosis, etc. (Figures 1, 2).
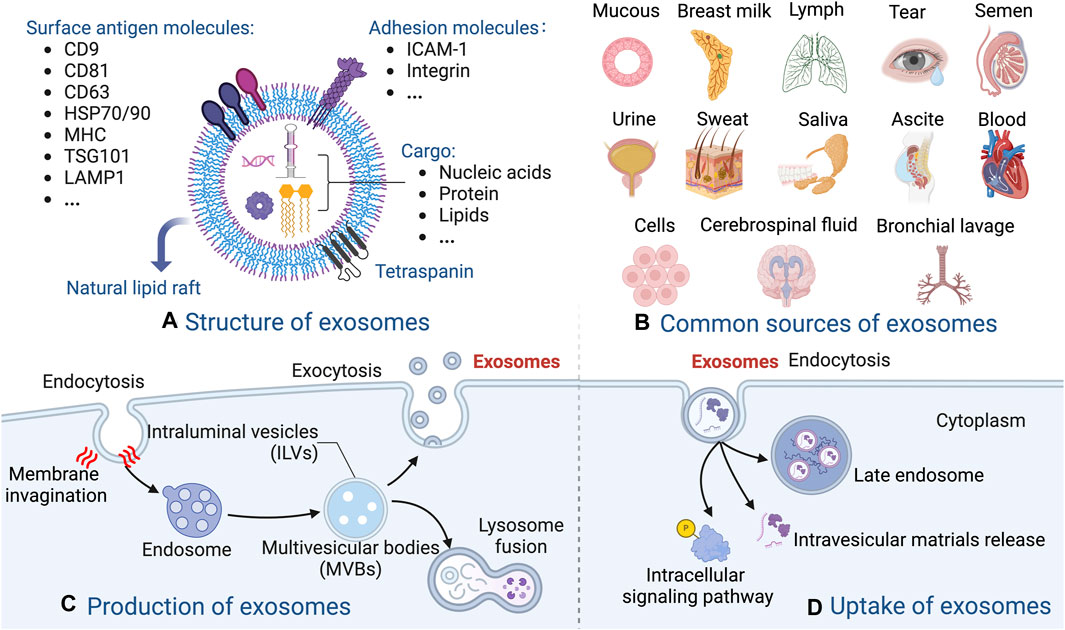
Figure 2. Overview of the biogenesis and composition of exosomes. (A) The molecular structure of exosomes. (B) The common sources of exosomes. (C) The production of exosomes. (D) Example of the uptake of exosomes.
Exosomes are present in diverse bodily fluids, including blood, saliva, amniotic fluid, hydrocephalus, and urine et al., and serve as vehicles for intercellular information exchange (Behera and Tyagi, 2018; Huang et al., 2022). The markers of exosomes include CD81, CD9, CD63, tumor susceptibility gene 101 (TSG101), heat shock protein 70/90 (HSP70/90), major histocompatibility complex (MHC), and lysosomal-associated membrane protein 1 (LAMP1) et al., which varies among different MSC types. In addition, the cargo of exosomes also shows significant differences, in terms of quantity and diversity, according to the originate cells from which they are derived (Malekpour et al., 2022; Kushioka et al., 2023). Upon binding to recipient cells, exosomes unload their cargo into these cells, thereby mediating intercellular signaling and material exchange, and ultimately modulating the functionality of the recipient cells (Zeng and Xie, 2022). Exosomes, including MSC-exosomes, markedly influence the immune response and inflammation (Huang et al., 2022). The membranes of MSC-exosomes protect their contents, and naturally occurring or artificially altered biomacromolecules on the exosomal surface facilitate the identification of target cells or tissues. Overall, the diagnostic and therapeutic potential are achieved profoundly via substantial transportation of bioactive agents within MSC-exosomes (Bellavia et al., 2018; Li et al., 2018) (Figure 2).
Therapeutic application of MSC-exosomes for skeletal diseases
MSC-exosomes in OA
OA, the most prevalent chronic disease of the joints, affects a substantial proportion of individuals ≥50 years of age. Given the aging population and the escalating rate of obesity, it is anticipated that its incidence will double over the next three decades (Bao and He, 2021; Cheng et al., 2022). OA is distinguished by the degeneration of cartilage, thickening of subchondral bone, and the development of osteophytes (Chen et al., 2023; Zou et al., 2023). Most interventions aim to manage pain, stiffness, and swelling, and arthroplasty is the only option for late-stage OA. Regrettably, despite the temporary relief provided by physical or drug therapy, restoration of joint function is challenging (Duan et al., 2020; Foo et al., 2021; Ghafouri-Fard et al., 2021). Most research on the clinical potential of MSC-exosomes has been on OA (Yuan et al., 2022; Zeng and Xie, 2022; Zhang et al., 2022; Chen et al., 2023) (Table 1; Figure 3).
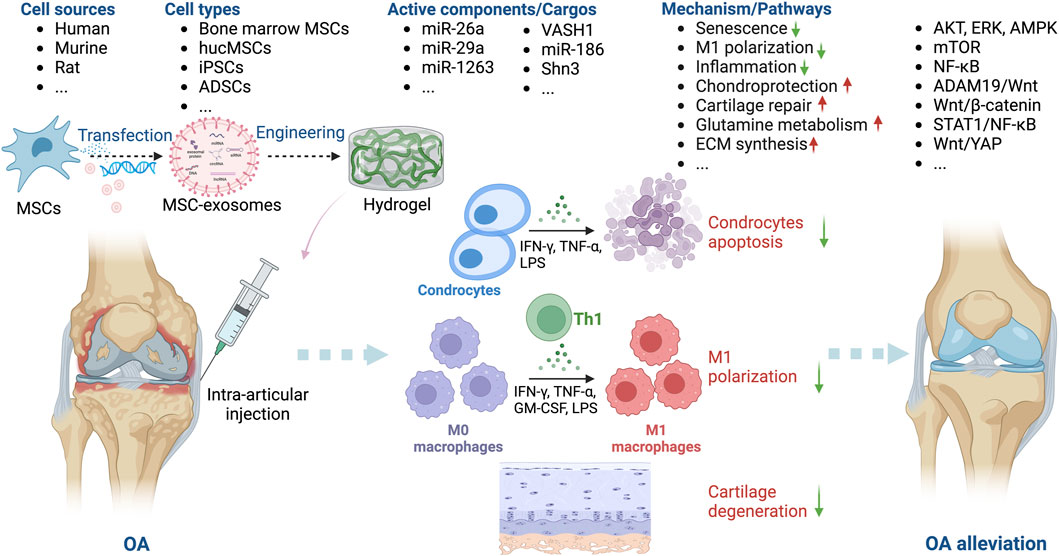
Figure 3. Overview of the therapeutic application of MSC-exosomes in OA, including the cell sources of MSC-exosomes, the active components/cargos, and the possible mechanism/pathways during the alleviation of OA using MSC-exosomes. Up-arrow (red), upregulation; down-arrow (green), downregulation.
Exosomes derived from bone marrow MSCs and those from adipose stem cells (ADSCs), infrapatellar fat pad (Wu et al., 2019), human umbilical cord (Li et al., 2022; Cao et al., 2023), synovial fluid (Xu et al., 2021), embryonic stem cells (Wang et al., 2017), and synovial membrane (Tao et al., 2017) have been used to generate exosomes for the treatment of OA. Among these sources, ADSCs and bone marrow-derived MSCs are relatively easy to obtain, which may be conducive to future therapeutical applications. Cosenza et al. showed that MSC-exosomes and MSC-derived microparticles have similar in vitro and in vivo chondroprotective effects in OA, thus reproducing the main therapeutic effects of bone marrow MSCs (Cosenza et al., 2017). MSC-exosomes protect cartilage and bone by inhibiting catabolic and inflammatory cytokines, suppressing macrophage activation, and preventing chondrocyte apoptosis (Huang et al., 2022; Kwon et al., 2022). Zhu et al. compared induced MSC (iMSC) and synovial MSC (SMSC) exosomes in mice OA model and found that the former had the greatest therapeutic effect in OA. The OARSI score of OA samples was significantly decreased after iMSC-exosomes treatment (Zhu et al., 2017).
Intra-articular injection is typically used to introduce MSC-exosomes into patients with OA (Tao et al., 2017; Huang et al., 2022; Kwon et al., 2022; Li et al., 2022; Cao et al., 2023). MSC-exosomes ameliorate OA by inhibiting inflammation, alleviating senescence, and protecting chondrocytes via the signal transducers and activators of transcription 1 (STAT1), protein kinase B (AKT), extracellular signal-regulated kinase (ERK), AMP-activated protein kinase (AMPK), mammalian target of rapamycin (mTOR), nuclear factor-kappa B (NF-κB), ADAM metallopeptidase domain 19 (ADAM19)/Wnt, and Wnt/yes-associated protein (YAP) signaling pathways (Song et al., 2021; Rosini et al., 2023; Wang et al., 2023). Zhang et al. reported that bone marrow-derived MSC-exosomes delay OA progression by modulating macrophage polarization (Zhang et al., 2020). Liao et al. showed that low-intensity pulsed ultrasound (LIPUS) strengthens the effect of bone marrow MSC-exosomes on cartilage regeneration in OA by strengthening the inhibition of NF-κB pathway-mediated inflammation and enhancing cartilage matrix synthesis (Liao et al., 2021). In addition, stimulation with a 75 Hz pulsed electromagnetic field promotes the ADSC-exosome–mediated suppression of inflammation and protection of cartilage (Xu et al., 2022).
Several OA studies have combined bioengineering approaches, typically biomaterials, with MSC-exosomes. Zeng et al. constructed a mussel-inspired multifunctional hydrogel system for codelivery of MSC-exosomes and icariin. MSC-exosomes enhanced the uptake of icariin by chondrocytes by at least twofold, thereby promoting cartilage regeneration in a papain-induced OA model (Zeng et al., 2023). Cao et al. engineered human umbilical cord MSC (hucMSC)-exosomes with a two-phase microgel targeting chondrocytes; the hucMSC-exosomes rejuvenated OA chondrocytes (Cao et al., 2023). Others have reported similar findings (Zhang et al., 2022; Pang et al., 2023), suggesting that combinations of novel biomaterials and MSC-exosomes have potential as cell-free therapeutics for OA.
MSC-exosomes in OP
Under normal physiological conditions, the equilibrium between bone resorption and bone formation preserves the integrity and quality of bone tissue. This delicate balance is disturbed in a number of bone disorders. OP, one of the most prevalent skeletal disorders worldwide, disproportionately affects the elderly, particularly women (Tan et al., 2020; Xie et al., 2020). Hormonal, nutritional, behavioral, and genetic factors may contribute to its development and progression, although aging and deficiencies in estrogen are the primary causes. Perturbations in bone metabolism, such as an imbalance in the activities of osteoclasts (OCs) and osteoblasts (OBs), are also implicated in its pathogenesis. During recovery from OP, OBs secrete osteoids to facilitate bone regeneration (Li et al., 2018; Yang et al., 2022). Currently available treatments (including anti-resorptive and anabolic drugs) control OP by promoting bone formation, impeding adipocyte development, or inhibiting OC differentiation. However, the potential adverse effects, which include fever, nausea, bone pain, and cancer, are nonnegligible (Duan and Guan, 2021; Li et al., 2022; Huo et al., 2023).
MSCs can differentiate into OBs and produce extracellular matrix (ECM), thereby promoting bone formation. Their ability to sustain bone homeostasis declines with aging, menopause, and ovariectomy (OVX), resulting in the accumulation of bone mineral adipocytes, ultimately leading to OP (Behera and Tyagi, 2018; Zeng and Xie, 2022; Ding et al., 2023). OVX-induced OP (Luo et al., 2019; Yahao and Xinjia, 2021; Cui et al., 2022; Qi et al., 2023), senile OP (SOP) (Lu et al., 2020), disuse OP (DOP) (Yang et al., 2020), glucocorticoid-induced OP (GIOP) (Yao et al., 2023), and diabetic OP (Zhang et al., 2021) are the models typically used to assess the therapeutic effect of MSC-exosomes in OP (Table 2). Bone marrow-derived MSCs are usually used to generate exosomes (Luo et al., 2019; Lu et al., 2020; Li et al., 2021; Qi et al., 2023), as are hucMSCs (Yang et al., 2020), ADSCs (Yao et al., 2023), and induced pluripotent stem cells (iPSCs) (Cui et al., 2022). The effects of MSC-exosomes in OP is mediated by enhancement of osteogenesis and angiogenesis, possibly via the vasohibin 1 (VASH1) (Lu et al., 2020), Mob1/Hippo (Yang et al., 2020), NOD-like receptor thermal protein domain associated protein 3 (NLRP3) (Zhang et al., 2021), schnurri-3 (Shn3)/Slit guidance ligand 3 (SLIT3) (Cui et al., 2022), and nuclear factor erythroid 2-related factor 2 (Nrf2)/heme oxygenase-1 (HO1) (Yao et al., 2023) signaling pathways (Figure 4). Zuo et al. reported that bone marrow-derived MSC-exosomes increased the β-catenin expression of recipient bone marrow-derived MSCs and restored the adipogenesis–osteogenesis balance, thereby alleviating radiation-induced bone loss (Zuo et al., 2019). ADSC exosomes alleviate streptozotocin (STZ)-induced diabetic OP by suppressing the NLRP3 inflammasome activation (Zhang et al., 2021).
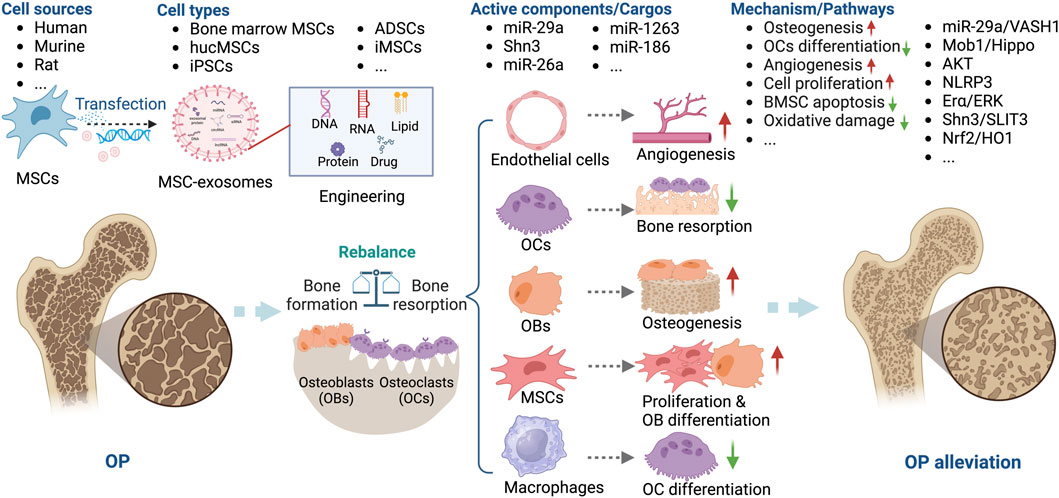
Figure 4. Overview of the therapeutic application of MSC-exosomes in OP, including the cell sources of MSC-exosomes, the active components/cargos, and the possible mechanism/pathways during the alleviation of OP using MSC-exosomes. Up-arrow (red), upregulation; down-arrow (green), downregulation.
Several miRNAs, including miR-26a, miR-29a, miR-1263, and miR-186, are implicated in the pathogenesis of OP (Lai et al., 2022). MSC-exosomes have been used to deliver miRNAs or anti-miRNAs for the treatment of OP. Bioengineering methods such as the use of aptamers and OB differentiation preconditioning can be used to amplify the effects of MSC-exosomes in OP. Luo et al. (Luo et al., 2019) showed that bone marrow MSC-exosomes administered intravenously did not ameliorate OVX-induced OP in a mouse model. They conjugated a bone marrow MSC-specific aptamer to the surface of bone marrow-derived MSC-exosomes to target bone marrow, which enhanced bone regeneration in OVX mice. Similarly, Cui et al. functionalized iPSC exosomes by modifying a bone-targeting peptide. The exosomes were loaded with the Shn3 siRNA and showed therapeutic potential for OP by enhancing bone and vessel formation and inhibiting OCs (Cui et al., 2022). Ge et al. compared the functions of hucMSC-exosomes from normal culture and those produced via osteogenic differentiation preconditioning. Interestingly, exosomes produced in an hucMSC/OB coculture system showed greater promotion of osteogenesis, confirming the importance of preconditioning for the effectiveness of MSC-exosomes in OP (Yahao and Xinjia, 2021). Consequently, MSC-exosomes have potential as a novel therapeutic strategy for OP.
MSC-exosomes in RA
RA is a chronic autoimmune disease that affects up to 2.5% of the population in each country, causing cartilage destruction and bone erosion (You et al., 2021). Current treatments focus on suppressing inflammation, but side effects like bone loss and long-term toxicities remain a challenge (Xu et al., 2022). Defective immune regulation leads to autoreactive T and B lymphocytes activation and differentiation, leading to the produce of autoreactive antibodies, activation of inflammatory responses and cartilage degeneration (Cosenza et al., 2017; Chang et al., 2022; Heydari et al., 2023). MSC-exosomes have not only anti-inflammatory but also immunomodulatory effects, which is why they help mitigate joint destruction (Heydari et al., 2023; Zhao et al., 2023).
Collagen-induced arthritis (CIA) (Chen et al., 2018; You et al., 2021) and Freund’s adjuvant-induced arthritis (FAIA) (Chang and Kan, 2021) in DBA/1J mice, C57BL/6 mice, and rats are frequently used animal models of RA. Dermal microvascular endothelial cells (DMECs) (Zhang et al., 2021), fibroblast-like synoviocytes (FLSs) (Ma et al., 2022), and lymphocytes (Tian et al., 2022) have been used to evaluate the role of MSC-exosomes in RA. MSC-exosomes have been shown to modulate the pathogenesis of RA (You et al., 2021; Ma et al., 2022; Rui et al., 2023). You et al. modified the surface of ADSC exosomes to reprogram macrophages. After intravenous injection (i.v.), the engineered ADSC exosomes accumulated in diseased joints and modulated the synovial microenvironment, thereby having a marked anti-inflammatory effect in RA (You et al., 2021). Rui et al. synthesized silk fibroin hydrogel encapsulated with olfactory ecto-MSCs (OEMSCs)-derived exosomes; their implantation altered T follicular helper cell polarization by regulating programmed cell death ligand 1 (PD-L1), thereby alleviating synovial inflammation and joint destruction (Rui et al., 2023) (Table 3).
The potential mechanisms underlying the therapeutic effects of MSC-exosomes in RA include modification of macrophage heterogeneity and autoimmunity. These effects are mediated by regulation of the matrix metalloproteinase 14 (MMP14)/vascular endothelial growth factor (VEGF) (Chen et al., 2018), interleukin 17 receptor A (IL-17RA)/NF-κB activator 1 (Act1)/TNF receptor associated factor 6 (TRAF6)/NF-κB (Tian et al., 2022), protein inhibitor of activated STAT3 (PIAS3)/STAT3/VEGF (Zhang et al., 2021), and PD-L1/phosphoinositide 3-kinase (PI3K)/AKT (Rui et al., 2023) signaling pathways. In addition, miR-150-5p (Chen et al., 2018), circFBXW7 (Chang and Kan, 2021), fibrinogen-like protein 1 (FGL1) (Xu et al., 2022), miR-146a, and miR-155 (Tavasolian et al., 2020) modulate the therapeutic effect of MSC-exosomes in RA. Huang et al. showed that miR-140-3p alleviates the inflammatory response of RA synovial fibroblasts (Huang et al., 2022). Given their immunoregulatory, chondroprotective, and regenerative activities, these factors likely contribute to the protective effects of MSC-exosomes in RA (Figure 5).
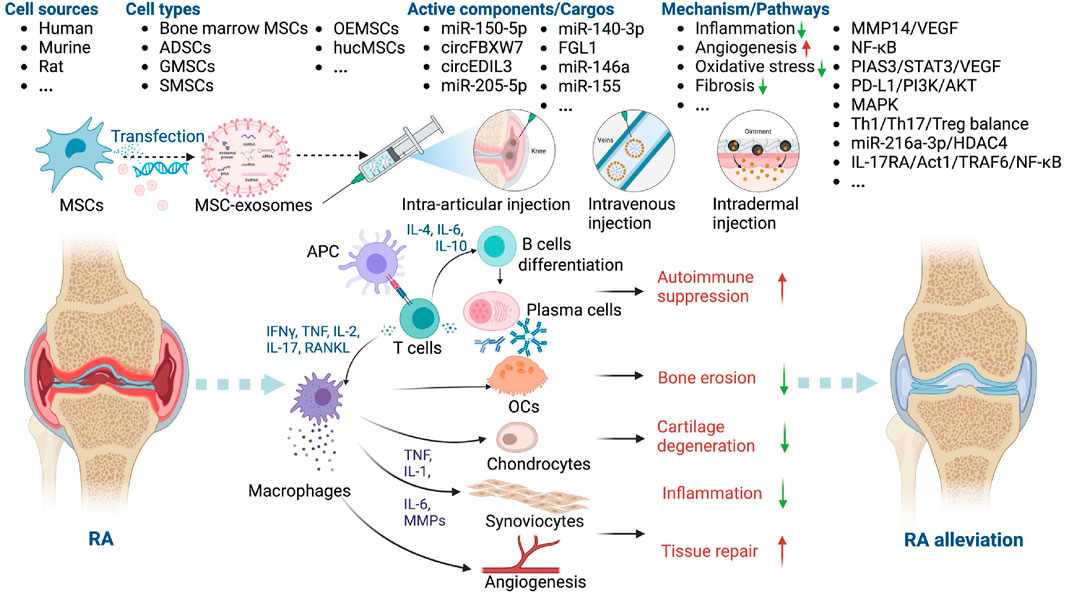
Figure 5. Overview of the therapeutic application of MSC-exosomes in RA, including the cell sources of MSC-exosomes, the active components/cargos, and the possible mechanism/pathways during the alleviation of RA using MSC-exosomes. Up-arrow (red), upregulation; down-arrow (green), downregulation.
MSC-exosomes in fracture and bone-defect healing
Fractures and bone defects are common musculoskeletal issues, and approximately 5%–10% of patients experience delayed union or nonunion as a result of inadequate bone regeneration (He et al., 2021; Smolinska et al., 2023). Bone regeneration involves various cell types, including OBs, OCs, endothelial cells, chondrocytes, and MSCs. Bone regeneration can be mediated by intramembranous ossification or endochondral ossification. MSC-derived OBs directly contribute to the calcification of bone via intramembranous osteogenesis, whereas endochondral ossification is an intricate process regulated by different cells, including chondrocytes. MSCs promote bone regeneration, an effect mediated by several key factors, including exosomes (Liu et al., 2020; Huang et al., 2022; Smolinska et al., 2023). Indeed, MSC-exosomes promote the repair of fractures (Table 4) and bone defects (Table 5).
Angiogenesis and ossification are prerequisites for bone healing, and MSC-exosomes augment OB differentiation and mineral deposition, thus facilitating angiogenesis, via the bone morphogenetic protein 2 (BMP-2)/Smad1/Runx2, hypoxia-inducible factor α (HIF-1α)/VEGF (Zhang et al., 2020), LDL receptor related protein 4 (LRP4)/Wnt/β-catenin (Yu et al., 2021), W domain-containing E3 ubiquitin protein ligase 1 (WWP1)/Smad ubiquitin regulatory factor 2 (Smurf2)/KLF transcription factor 5 (KLF5)/β-catenin (Huang et al., 2021) signaling pathways. Moreover, the cytokines, miRNAs, and lncRNAs such as miR-136-5p (Yu et al., 2021), lncTUG1 (Li et al., 2023), and monocyte chemoattractant protein-1 (MCP-1) (Furuta et al., 2016) delivered by MSC-exosomes promote the healing of fractures and bone defects (Figure 6).
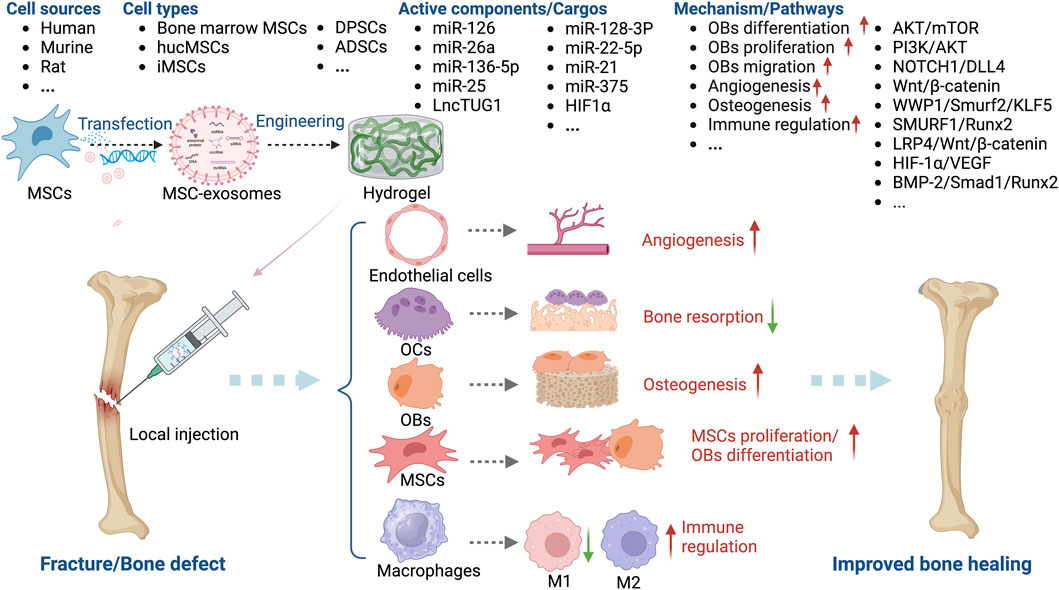
Figure 6. Overview of the therapeutic application of MSC-exosomes in fracture/bone defect, including the cell sources of MSC-exosomes, the active components/cargos, and the possible mechanism/pathways during the alleviation of fracture/bone defect using MSC-exosomes. Up-arrow (red), upregulation; down-arrow (green), downregulation.
Furuta et al. investigated MSC-exosomes in a CD9−/− mouse femur fracture model and found that bone union was significantly accelerated by MSC paracrine signaling (Furuta et al., 2016). Liu et al. showed that exosomes from hypoxia-preconditioned hucMSCs have a greater effect on fracture healing than those from normoxia-preconditioned hucMSCs, emphasizing the importance of mimicking normal physiological conditions (Liu et al., 2020). Unlike OP, OA, and RA, MSC-exosomes for the healing of fractures and bone defects are typically administered in a hydrogel-based local implant or by local injection. Ma et al. combined small intestinal submucosa hydrogels with bone marrow-derived MSC-exosomes and fusion peptides to enhance the osteogenesis-promoting role of exosomes in a calvarial defect model (Ma et al., 2022). Chen et al. incorporated miR-375-carrying ADSC exosomes in a hydrogel to achieve the slow and controlled release of miR-375, which had a marked bone-healing effect in a rat model of a calvarial defect (Chen et al., 2019).
MSC-exosomes in IDD
The intervertebral disc (IVD), including the nucleus pulposus (NP) and annulus fibrosus (AF), is an important load-bearing component of the spinal column. The accumulation of advanced glycation end products (AGEs) causes endoplasmic reticulum (ER) stress in the IVD. Subsequently, IDD is initiated by the apoptosis of NP cells (NPCs) and increased pro-inflammatory cytokine production and disruption of the ECM (Widjaja et al., 2022; Xia et al., 2022). IDD is the main reason for low back pain in over 90% of people over 50 (Krut et al., 2021). Surgical therapy and pain relief medication are the main treatments, but their effectiveness is uncertain (Xiao et al., 2022).
MSCs can enhance the viability of disc cells and thus impede IDD progression, typically by modulating the levels of MMP12 and HSP47 (Leung et al., 2014). MSC-exosomes modulate the inflammatory response of NPCs, suppressing their apoptosis and upregulating ECM synthesis (Bhujel et al., 2022; Distefano et al., 2022; Hu et al., 2023) (Table 6).
The key factors for MSC-exosome treatment of IDD are miR-410 (Zhang et al., 2020), miR-31-5p (Xie et al., 2020), miR-302c (Yu et al., 2023), and the lncRNA colon adenocarcinoma hypermethylated (CAHM) (Li et al., 2022). Treatment with MSC-exosomes decreases the levels of markers of ECM degradation, such as IL-1β, cyclooxygenase (COX)-2, MMP13, and iNOS (Liang et al., 2021; Lu et al., 2021). MSC-exosomes inhibit AGE-induced ER stress in NPCs by modulating AKT and ERK signaling (Liao et al., 2019). The NLRP3 (Zhang et al., 2020; Yu et al., 2023) and mTOR/autophagy pathways are also implicated in the effects of MSC-exosomes in IDD (Liang et al., 2019). Xie et al. showed that MSC-exosomes protect against IDD in a rat model by inhibiting oxidative stress, an effect reversed in part by miR-31-5p knockdown (Xie et al., 2020). Li et al. demonstrated that MSC-exosomes inhibit M1 macrophage polarization, NPC apoptosis, ECM degradation, and IDD progression by delivering the lncRNA CAHM, an effect reversed in part by siCAHM (Li et al., 2022). Moreover, Guan et al. (Guan et al., 2023) reported that MSC-exosomes can modulate macrophage polarization and NPC senescence, thereby suppressing the apoptosis of NPCs and mitigating IDD (Figure 7).
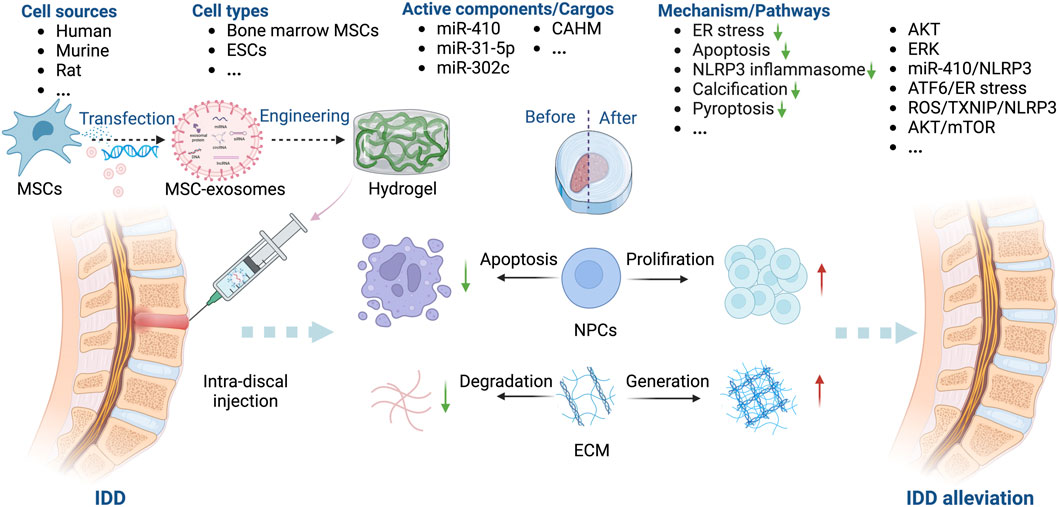
Figure 7. Overview of the therapeutic application of MSC-exosomes in IDD, including the cell sources of MSC-exosomes, the active components/cargos, and the possible mechanism/pathways during the alleviation of IDD using MSC-exosomes. ECM: extracellular matrix. Up-arrow (red), upregulation; down-arrow (green), downregulation.
Obstacles to the use of MSC-exosomes-based therapeutics in skeletal diseases
Research has focused on the therapeutic potential of MSCs and exosomes for skeletal diseases. Besides exhibiting similar therapeutic effects, MSC-exosomes could overcome the safety and ethical concerns associated with MSCs injection. In addition, exosomes have less-stringent storage requirements than MSCs. Their efficacy and ability to be targeted to bone marrow, cartilage, and macrophages can be enhanced by bioengineering and preconditioning. These characteristics make exosomes good candidates for the treatment of skeletal diseases.
Currently, the existing clinical trials of MSC-exosomes–based therapies are mainly focused on cardiovascular disease, liver cirrhosis, psoriasis, macular holes, dry eye disease, diabetes mellitus, pneumonia, sepsis, wound healing and cancer (data from http://clinicaltrials.gov). For example, Dehghani et al. reported that no post-interventional adverse effects were observed following intraparenchymal implantation of MSC-exosomes in five ischemic stroke patients (Dehghani et al., 2022). There are also several ongoing clinical trials investigating the role of MSC-exosomes in skeletal diseases. Matas et al. are conducting an interventional clinical trial to compare the safety and efficacy of MSC-exosomes injection in patients with mild to moderate symptomatic OA (Phase I, No. NCT05060107, 2021-10-05∼2023-10-05). In another clinical trial, researchers are comparing injection of SF-MSC-exosomes with SF-MSCs in degenerative meniscal injury (Phase II, No. NCT05261360, 2022-03∼2025-03). Also, autogenous MSC culture medium that containing exosomes are being studied in a clinical trial to enhance the osteogenesis of bone grafting (Phase I/II, No. NCT04998058, 2023-12-15∼2024-12-30).
However, whether MSC-exosomes can replace MSCs, and whether stem cells and exosomes in combination have synergistic therapeutic effects, is unclear. In addition, the long-term hazards of MSC-exosome therapy are unknown, necessitating evaluation of their effect on the immune system and the bone microenvironment. Moreover, compared with the existing MSCs-related therapies, the standardized methods of isolation, characterization and purification of MSC-exosomes need to be improved in terms of their reliability, cost, yield, and reproducibility. The ability of exosomes to deliver therapeutics for skeletal diseases also merits further investigation.
Conclusion and future directions
Exosomes have considerable therapeutic potential for skeletal diseases but are at an early stage of development. The functionality, appropriate dosage, distribution, and clearance of exosomes need to be investigated to ensure their safety and efficacy. Their clinical application is impeded by a variety of challenges; therefore, further research is needed.
Author contributions
XY: Funding acquisition, Supervision, Validation, Writing–original draft, Writing–review and editing. SZ: Data curation, Visualization, Writing–original draft. JL: Data curation, Methodology, Software, Visualization, Writing–original draft. XC: Data curation, Investigation, Software, Writing–review and editing. TZ: Writing–review and editing. RH: Writing–review and editing, Supervision. CY: Conceptualization, Methodology, Visualization, Writing–review and editing, Funding acquisition. JX: Conceptualization, Data curation, Funding acquisition, Project administration, Resources, Supervision, Validation, Visualization, Writing–review and editing.
Funding
The author(s) declare that financial support was received for the research, authorship, and/or publication of this article. This study was supported by the National Natural Science Foundation of China (No. 32371412, 32071349, 81701820, and 82102597), Zhejiang Provincial Natural Science Foundation of China (LY23H060009 and LY24C100001), and Zhejiang Province Medical and Health Science and Technology Plan Project (2024KY1040).
Acknowledgments
All the figures in this study were created with BioRender.com.
Conflict of interest
The authors declare that the research was conducted in the absence of any commercial or financial relationships that could be construed as a potential conflict of interest.
Publisher’s note
All claims expressed in this article are solely those of the authors and do not necessarily represent those of their affiliated organizations, or those of the publisher, the editors and the reviewers. Any product that may be evaluated in this article, or claim that may be made by its manufacturer, is not guaranteed or endorsed by the publisher.
References
Bao, C., and He, C. (2021). The role and therapeutic potential of MSC-derived exosomes in osteoarthritis. Arch. Biochem. Biophys. 710, 109002. doi:10.1016/j.abb.2021.109002
Behera, J., and Tyagi, N. (2018). Exosomes: mediators of bone diseases, protection, and therapeutics potential. Oncoscience 5, 181–195. doi:10.18632/oncoscience.421
Bellavia, D., Raimondi, L., Costa, V., De Luca, A., Carina, V., Maglio, M., et al. (2018). Engineered exosomes: a new promise for the management of musculoskeletal diseases. Biochim. Biophys. Acta Gen. Subj. 1862, 1893–1901. doi:10.1016/j.bbagen.2018.06.003
Bhujel, B., Shin, H. E., Choi, D. J., and Han, I. (2022). Mesenchymal stem cell-derived exosomes and intervertebral disc regeneration: review. Int. J. Mol. Sci. 23, 7306. doi:10.3390/ijms23137306
Cao, H., Chen, M., Cui, X., Liu, Y., Liu, Y., Deng, S., et al. (2023). Cell-free osteoarthritis treatment with sustained-release of chondrocyte-targeting exosomes from umbilical cord-derived mesenchymal stem cells to rejuvenate aging chondrocytes. ACS Nano 17, 13358–13376. doi:10.1021/acsnano.3c01612
Chang, L., and Kan, L. (2021). Mesenchymal stem cell-originated exosomal circular RNA circFBXW7 attenuates cell proliferation, migration and inflammation of fibroblast-like synoviocytes by targeting miR-216a-3p/HDAC4 in rheumatoid arthritis. J. Inflamm. Res. 14, 6157–6171. doi:10.2147/jir.S336099
Chang, T. H., Wu, C. S., Chiou, S. H., Chang, C. H., and Liao, H. J. (2022). Adipose-derived stem cell exosomes as a novel anti-inflammatory agent and the current therapeutic targets for rheumatoid arthritis. Biomedicines 10, 1725. doi:10.3390/biomedicines10071725
Chen, A., Chen, Y., Rong, X., You, X., Wu, D., Zhou, X., et al. (2023). The application of exosomes in the early diagnosis and treatment of osteoarthritis. Front. Pharmacol. 14, 1154135. doi:10.3389/fphar.2023.1154135
Chen, S., Tang, Y., Liu, Y., Zhang, P., Lv, L., Zhang, X., et al. (2019). Exosomes derived from miR-375-overexpressing human adipose mesenchymal stem cells promote bone regeneration. Cell Prolif. 52, e12669. doi:10.1111/cpr.12669
Chen, Z., Wang, H., Xia, Y., Yan, F., and Lu, Y. (2018). Therapeutic potential of mesenchymal cell-derived miRNA-150-5p-expressing exosomes in rheumatoid arthritis mediated by the modulation of MMP14 and VEGF. J. Immunol. 201, 2472–2482. doi:10.4049/jimmunol.1800304
Cheng, J., Sun, Y., Ma, Y., Ao, Y., Hu, X., and Meng, Q. (2022). Engineering of MSC-derived exosomes: a promising cell-free therapy for osteoarthritis. Membr. (Basel) 12, 739. doi:10.3390/membranes12080739
Cieza, A., Causey, K., Kamenov, K., Hanson, S. W., Chatterji, S., and Vos, T. (2021). Global estimates of the need for rehabilitation based on the global burden of disease study 2019: a systematic analysis for the global burden of disease study 2019. Lancet 396, 2006–2017. doi:10.1016/S0140-6736(20)32340-0
Cosenza, S., Ruiz, M., Maumus, M., Jorgensen, C., and Noël, D. (2017). Pathogenic or therapeutic extracellular vesicles in rheumatic diseases: role of mesenchymal stem cell-derived vesicles. Int. J. Mol. Sci. 18, 889. doi:10.3390/ijms18040889
Cosenza, S., Ruiz, M., Toupet, K., Jorgensen, C., and Noël, D. (2017). Mesenchymal stem cells derived exosomes and microparticles protect cartilage and bone from degradation in osteoarthritis. Sci. Rep. 7, 16214. doi:10.1038/s41598-017-15376-8
Cosenza, S., Toupet, K., Maumus, M., Luz-Crawford, P., Blanc-Brude, O., Jorgensen, C., et al. (2018). Mesenchymal stem cells-derived exosomes are more immunosuppressive than microparticles in inflammatory arthritis. Theranostics 8, 1399–1410. doi:10.7150/thno.21072
Cui, Y., Guo, Y., Kong, L., Shi, J., Liu, P., Li, R., et al. (2022). A bone-targeted engineered exosome platform delivering siRNA to treat osteoporosis. Bioact. Mater 10, 207–221. doi:10.1016/j.bioactmat.2021.09.015
Dehghani, L., Khojasteh, A., Soleimani, M., Oraee-Yazdani, S., Keshel, S. H., Saadatnia, M., et al. (2022). Safety of intraparenchymal injection of allogenic placenta mesenchymal stem cells derived exosome in patients undergoing decompressive craniectomy following malignant middle cerebral artery infarct, A pilot randomized clinical trial. Int. J. Prev. Med. 13, 7. doi:10.4103/ijpvm.ijpvm_441_21
Ding, X., Lv, S., Guo, Z., Gong, X., Wang, C., Zhang, X., et al. (2023). Potential therapeutic options for premature ovarian insufficiency: experimental and clinical evidence. Reprod. Sci. 30, 3428–3442. doi:10.1007/s43032-023-01300-1
DiStefano, T. J., Vaso, K., Danias, G., Chionuma, H. N., Weiser, J. R., and Iatridis, J. C. (2022). Extracellular vesicles as an emerging treatment option for intervertebral disc degeneration: therapeutic potential, translational pathways, and regulatory considerations. Adv. Healthc. Mater 11, e2100596. doi:10.1002/adhm.202100596
Duan, K., and Guan, J. (2021). Research progress of exosomes in treatment of osteoporosis. Zhongguo Xiu Fu Chong Jian Wai Ke Za Zhi 35, 1642–1649. doi:10.7507/1002-1892.202105106
Duan, L., Liang, Y., Xu, X., Xiao, Y., and Wang, D. (2020). Recent progress on the role of miR-140 in cartilage matrix remodelling and its implications for osteoarthritis treatment. Arthritis Res. Ther. 22, 194. doi:10.1186/s13075-020-02290-0
Foo, J. B., Looi, Q. H., How, C. W., Lee, S. H., Al-Masawa, M. E., Chong, P. P., et al. (2021). Mesenchymal stem cell-derived exosomes and MicroRNAs in cartilage regeneration: biogenesis, efficacy, miRNA enrichment and delivery. Pharm. (Basel) 14, 1093. doi:10.3390/ph14111093
Fu, Y., Li, J., Zhang, Z., Ren, F., Wang, Y., Jia, H., et al. (2022). Umbilical cord mesenchymal stem cell-derived exosomes alleviate collagen-induced arthritis by balancing the population of Th17 and regulatory T cells. FEBS Lett. 596, 2668–2677. doi:10.1002/1873-3468.14460
Furuta, T., Miyaki, S., Ishitobi, H., Ogura, T., Kato, Y., Kamei, N., et al. (2016). Mesenchymal stem cell-derived exosomes promote fracture healing in a mouse model. Stem Cells Transl. Med. 5, 1620–1630. doi:10.5966/sctm.2015-0285
Ghafouri-Fard, S., Niazi, V., Hussen, B. M., Omrani, M. D., Taheri, M., and Basiri, A. (2021). The emerging role of exosomes in the treatment of human disorders with a special focus on mesenchymal stem cells-derived exosomes. Front. Cell Dev. Biol. 9, 653296. doi:10.3389/fcell.2021.653296
Gholami Farashah, M. S., Javadi, M., Mohammadi, A., Soleimani Rad, J., Shakouri, S. K., and Roshangar, L. (2022). Bone marrow mesenchymal stem cell's exosomes as key nanoparticles in osteogenesis and bone regeneration: specific capacity based on cell type. Mol. Biol. Rep. 49, 12203–12218. doi:10.1007/s11033-022-07807-1
Guan, M., Liu, C., Zheng, Q., Chu, G., Wang, H., Jin, J., et al. (2023). Exosome-laden injectable self-healing hydrogel based on quaternized chitosan and oxidized starch attenuates disc degeneration by suppressing nucleus pulposus senescence. Int. J. Biol. Macromol. 232, 123479. doi:10.1016/j.ijbiomac.2023.123479
He, X. Y., Yu, H. M., Lin, S., and Li, Y. Z. (2021). Advances in the application of mesenchymal stem cells, exosomes, biomimetic materials, and 3D printing in osteoporosis treatment. Cell Mol. Biol. Lett. 26, 47. doi:10.1186/s11658-021-00291-8
Heydari, R., Koohi, F., Rasouli, M., Rezaei, K., Abbasgholinejad, E., Bekeschus, S., et al. (2023). Exosomes as rheumatoid arthritis diagnostic biomarkers and therapeutic agents. Vaccines (Basel) 11, 687. doi:10.3390/vaccines11030687
Hu, Y. C., Zhang, X. B., Lin, M. Q., Zhou, H. Y., Cong, M. X., Chen, X. Y., et al. (2023). Nanoscale treatment of intervertebral disc degeneration: mesenchymal stem cell exosome transplantation. Curr. Stem Cell Res. Ther. 18, 163–173. doi:10.2174/1574888x17666220422093103
Huang, X., Lan, Y., Shen, J., Chen, Z., and Xie, Z. (2022). Extracellular vesicles in bone homeostasis: emerging mediators of osteoimmune interactions and promising therapeutic targets. Int. J. Biol. Sci. 18, 4088–4100. doi:10.7150/ijbs.69816
Huang, Y., Chen, L., Chen, D., Fan, P., and Yu, H. (2022). Exosomal microRNA-140-3p from human umbilical cord mesenchymal stem cells attenuates joint injury of rats with rheumatoid arthritis by silencing SGK1. Mol. Med. 28, 36. doi:10.1186/s10020-022-00451-2
Huang, Y., Xu, Y., Feng, S., He, P., Sheng, B., and Ni, J. (2021). miR-19b enhances osteogenic differentiation of mesenchymal stem cells and promotes fracture healing through the WWP1/Smurf2-mediated KLF5/β-catenin signaling pathway. Exp. Mol. Med. 53, 973–985. doi:10.1038/s12276-021-00631-w
Huo, K. L., Yang, T. Y., Zhang, W. W., and Shao, J. (2023). Mesenchymal stem/stromal cells-derived exosomes for osteoporosis treatment. World J. Stem Cells 15, 83–89. doi:10.4252/wjsc.v15.i3.83
Jiang, K., Jiang, T., Chen, Y., and Mao, X. (2021). Mesenchymal stem cell-derived exosomes modulate chondrocyte glutamine metabolism to alleviate osteoarthritis progression. Mediat. Inflamm. 2021, 2979124. doi:10.1155/2021/2979124
Jin, Y., Xu, M., Zhu, H., Dong, C., Ji, J., Liu, Y., et al. (2021). Therapeutic effects of bone marrow mesenchymal stem cells-derived exosomes on osteoarthritis. J. Cell Mol. Med. 25, 9281–9294. doi:10.1111/jcmm.16860
Kang, Y., Xu, C., Meng, L., Dong, X., Qi, M., and Jiang, D. (2022). Exosome-functionalized magnesium-organic framework-based scaffolds with osteogenic, angiogenic and anti-inflammatory properties for accelerated bone regeneration. Bioact. Mater 18, 26–41. doi:10.1016/j.bioactmat.2022.02.012
Kong, R., Zhang, J., Ji, L., Yu, Y., Gao, J., and Zhao, D. (2023). Synovial mesenchymal stem cell-derived exosomal microRNA-320c facilitates cartilage damage repair by targeting ADAM19-dependent Wnt signalling in osteoarthritis rats. Inflammopharmacology 31, 915–926. doi:10.1007/s10787-023-01142-y
Krut, Z., Pelled, G., Gazit, D., and Gazit, Z. (2021). Stem cells and exosomes: new therapies for intervertebral disc degeneration. Cells 10, 2241. doi:10.3390/cells10092241
Kubiatowicz, L. J., Mohapatra, A., Krishnan, N., Fang, R. H., and Zhang, L. (2022). mRNA nanomedicine: design and recent applications. Explor. (Beijing) 2, 20210217. doi:10.1002/EXP.20210217
Kushioka, J., Chow, S. K., Toya, M., Tsubosaka, M., Shen, H., Gao, Q., et al. (2023). Bone regeneration in inflammation with aging and cell-based immunomodulatory therapy. Inflamm. Regen. 43, 29. doi:10.1186/s41232-023-00279-1
Kwon, D. G., Kim, M. K., Jeon, Y. S., Nam, Y. C., Park, J. S., and Ryu, D. J. (2022). State of the art: the immunomodulatory role of MSCs for osteoarthritis. Int. J. Mol. Sci. 23, 1618. doi:10.3390/ijms23031618
Lai, G., Zhao, R., Zhuang, W., Hou, Z., Yang, Z., He, P., et al. (2022). BMSC-derived exosomal miR-27a-3p and miR-196b-5p regulate bone remodeling in ovariectomized rats. PeerJ 10, e13744. doi:10.7717/peerj.13744
Leung, V. Y., Aladin, D. M., Lv, F., Tam, V., Sun, Y., Lau, R. Y., et al. (2014). Mesenchymal stem cells reduce intervertebral disc fibrosis and facilitate repair. Stem Cells 32, 2164–2177. doi:10.1002/stem.1717
Li, F., Xu, Z., Xie, Z., Sun, X., Li, C., Chen, Y., et al. (2023). Adipose mesenchymal stem cells-derived exosomes alleviate osteoarthritis by transporting microRNA -376c-3p and targeting the WNT-beta-catenin signaling axis. Apoptosis 28, 362–378. doi:10.1007/s10495-022-01787-0
Li, L., Zhou, X., Zhang, J. T., Liu, A. F., Zhang, C., Han, J. C., et al. (2021). Exosomal miR-186 derived from BMSCs promote osteogenesis through hippo signaling pathway in postmenopausal osteoporosis. J. Orthop. Surg. Res. 16, 23. doi:10.1186/s13018-020-02160-0
Li, P., Lv, S., Jiang, W., Si, L., Liao, B., Zhao, G., et al. (2022). Exosomes derived from umbilical cord mesenchymal stem cells protect cartilage and regulate the polarization of macrophages in osteoarthritis. Ann. Transl. Med. 10, 976. doi:10.21037/atm-22-3912
Li, Q. C., Li, C., Zhang, W., Pi, W., and Han, N. (2022). Potential effects of exosomes and their MicroRNA carrier on osteoporosis. Curr. Pharm. Des. 28, 899–909. doi:10.2174/1381612828666220128104206
Li, W., Li, L., Cui, R., Chen, X., Hu, H., and Qiu, Y. (2023). Bone marrow mesenchymal stem cells derived exosomal Lnc TUG1 promotes bone fracture recovery via miR-22-5p/Anxa8 axis. Hum. Cell 36, 1041–1053. doi:10.1007/s13577-023-00881-y
Li, W., Liu, Y., Zhang, P., Tang, Y., Zhou, M., Jiang, W., et al. (2018). Tissue-engineered bone immobilized with human adipose stem cells-derived exosomes promotes bone regeneration. ACS Appl. Mater Interfaces 10, 5240–5254. doi:10.1021/acsami.7b17620
Li, W., Xu, Y., and Chen, W. (2022). Bone mesenchymal stem cells deliver exogenous lncRNA CAHM via exosomes to regulate macrophage polarization and ameliorate intervertebral disc degeneration. Exp. Cell Res. 421, 113408. doi:10.1016/j.yexcr.2022.113408
Li, Y., Jin, D., Xie, W., Wen, L., Chen, W., Xu, J., et al. (2018). Mesenchymal stem cells-derived exosomes: a possible therapeutic strategy for osteoporosis. Curr. Stem Cell Res. Ther. 13, 362–368. doi:10.2174/1574888x13666180403163456
Liang, B., Liang, J. M., Ding, J. N., Xu, J., Xu, J. G., and Chai, Y. M. (2019). Dimethyloxaloylglycine-stimulated human bone marrow mesenchymal stem cell-derived exosomes enhance bone regeneration through angiogenesis by targeting the AKT/mTOR pathway. Stem Cell Res. Ther. 10, 335. doi:10.1186/s13287-019-1410-y
Liang, W., Han, B., Hai, Y., Sun, D., and Yin, P. (2021). Mechanism of action of mesenchymal stem cell-derived exosomes in the intervertebral disc degeneration treatment and bone repair and regeneration. Front. Cell Dev. Biol. 9, 833840. doi:10.3389/fcell.2021.833840
Liao, Q., Li, B. J., Li, Y., Xiao, Y., Zeng, H., Liu, J. M., et al. (2021). Low-intensity pulsed ultrasound promotes osteoarthritic cartilage regeneration by BMSC-derived exosomes via modulating the NF-κB signaling pathway. Int. Immunopharmacol. 97, 107824. doi:10.1016/j.intimp.2021.107824
Liao, Z., Luo, R., Li, G., Song, Y., Zhan, S., Zhao, K., et al. (2019). Exosomes from mesenchymal stem cells modulate endoplasmic reticulum stress to protect against nucleus pulposus cell death and ameliorate intervertebral disc degeneration in vivo. Theranostics 9, 4084–4100. doi:10.7150/thno.33638
Liu, S., Xu, X., Liang, S., Chen, Z., Zhang, Y., Qian, A., et al. (2020). The application of MSCs-derived extracellular vesicles in bone disorders: novel cell-free therapeutic strategy. Front. Cell Dev. Biol. 8, 619. doi:10.3389/fcell.2020.00619
Liu, W., Li, L., Rong, Y., Qian, D., Chen, J., Zhou, Z., et al. (2020). Hypoxic mesenchymal stem cell-derived exosomes promote bone fracture healing by the transfer of miR-126. Acta Biomater. 103, 196–212. doi:10.1016/j.actbio.2019.12.020
Lo, J., Chan, L., and Flynn, S. (2021). A systematic review of the incidence, prevalence, costs, and activity and work limitations of amputation, osteoarthritis, rheumatoid arthritis, back pain, multiple sclerosis, spinal cord injury, stroke, and traumatic brain injury in the United States: a 2019 update. Arch. Phys. Med. Rehabil. 102, 115–131. doi:10.1016/j.apmr.2020.04.001
Lu, G. D., Cheng, P., Liu, T., and Wang, Z. (2020). BMSC-derived exosomal miR-29a promotes angiogenesis and osteogenesis. Front. Cell Dev. Biol. 8, 608521. doi:10.3389/fcell.2020.608521
Lu, L., Xu, A., Gao, F., Tian, C., Wang, H., Zhang, J., et al. (2021). Mesenchymal stem cell-derived exosomes as a novel strategy for the treatment of intervertebral disc degeneration. Front. Cell Dev. Biol. 9, 770510. doi:10.3389/fcell.2021.770510
Luo, Z. W., Li, F. X., Liu, Y. W., Rao, S. S., Yin, H., Huang, J., et al. (2019). Aptamer-functionalized exosomes from bone marrow stromal cells target bone to promote bone regeneration. Nanoscale 11, 20884–20892. doi:10.1039/c9nr02791b
Ma, S., Wu, J., Hu, H., Mu, Y., Zhang, L., Zhao, Y., et al. (2022). Novel fusion peptides deliver exosomes to modify injectable thermo-sensitive hydrogels for bone regeneration. Mater Today Bio 13, 100195. doi:10.1016/j.mtbio.2021.100195
Ma, W., Tang, F., Xiao, L., Han, S., Yao, X., Zhang, Q., et al. (2022). miR-205-5p in exosomes divided from chondrogenic mesenchymal stem cells alleviated rheumatoid arthritis via regulating MDM2 in fibroblast-like synoviocytes. J. Musculoskelet. Neuronal Interact. 22, 132–141.
Malekpour, K., Hazrati, A., Zahar, M., Markov, A., Zekiy, A. O., Navashenaq, J. G., et al. (2022). The potential use of mesenchymal stem cells and their derived exosomes for orthopedic diseases treatment. Stem Cell Rev. Rep. 18, 933–951. doi:10.1007/s12015-021-10185-z
Pang, L., Jin, H., Lu, Z., Xie, F., Shen, H., Li, X., et al. (2023). Treatment with mesenchymal stem cell-derived nanovesicle-containing gelatin methacryloyl hydrogels alleviates osteoarthritis by modulating chondrogenesis and macrophage polarization. Adv. Healthc. Mater 12, e2300315. doi:10.1002/adhm.202300315
Pourakbari, R., Khodadadi, M., Aghebati-Maleki, A., Aghebati-Maleki, L., and Yousefi, M. (2019). The potential of exosomes in the therapy of the cartilage and bone complications; emphasis on osteoarthritis. Life Sci. 236, 116861. doi:10.1016/j.lfs.2019.116861
Qi, H., Shen, E., Shu, X., Liu, D., and Wu, C. (2023). ERK-estrogen receptor α signaling plays a role in the process of bone marrow mesenchymal stem cell-derived exosomes protecting against ovariectomy-induced bone loss. J. Orthop. Surg. Res. 18, 250. doi:10.1186/s13018-023-03660-5
Qi, X., Zhang, J., Yuan, H., Xu, Z., Li, Q., Niu, X., et al. (2016). Exosomes secreted by human-induced pluripotent stem cell-derived mesenchymal stem cells repair critical-sized bone defects through enhanced angiogenesis and osteogenesis in osteoporotic rats. Int. J. Biol. Sci. 12, 836–849. doi:10.7150/ijbs.14809
Rosini, S., Saviola, G., Comini, L., and Molfetta, L. (2023). Mesenchymal cells are a promising -but still unsatisfying- anti- inflammatory therapeutic strategy for osteoarthritis: a narrative review. Curr. Rheumatol. Rev. 19, 287–293. doi:10.2174/1573397118666220928141624
Rui, K., Tang, X., Shen, Z., Jiang, C., Zhu, Q., Liu, S., et al. (2023). Exosome inspired photo-triggered gelation hydrogel composite on modulating immune pathogenesis for treating rheumatoid arthritis. J. Nanobiotechnology 21, 111. doi:10.1186/s12951-023-01865-8
Salari, N., Ghasemi, H., Mohammadi, L., Behzadi, M. H., Rabieenia, E., Shohaimi, S., et al. (2021). The global prevalence of osteoporosis in the world: a comprehensive systematic review and meta-analysis. J. Orthop. Surg. Res. 16, 609. doi:10.1186/s13018-021-02772-0
Shang, F., Yu, Y., Liu, S., Ming, L., Zhang, Y., Zhou, Z., et al. (2021). Advancing application of mesenchymal stem cell-based bone tissue regeneration. Bioact. Mater 6, 666–683. doi:10.1016/j.bioactmat.2020.08.014
Smolinska, V., Csobonyeiova, M., Zamborsky, R., and Danisovic, L. (2023). Stem cells and their derivatives: an implication for the regeneration of nonunion fractures. Cell Transpl. 32, 9636897231183530. doi:10.1177/09636897231183530
Song, H., Zhao, J., Cheng, J., Feng, Z., Wang, J., Momtazi-Borojeni, A. A., et al. (2021). Extracellular vesicles in chondrogenesis and cartilage regeneration. J. Cell Mol. Med. 25, 4883–4892. doi:10.1111/jcmm.16290
Sun, X., Mao, Y., Liu, B., Gu, K., Liu, H., Du, W., et al. (2023). Mesenchymal stem cell-derived exosomes enhance 3D-printed scaffold functions and promote alveolar bone defect repair by enhancing angiogenesis. J. Pers. Med. 13, 180. doi:10.3390/jpm13020180
Swanson, W. B., Zhang, Z., Xiu, K., Gong, T., Eberle, M., Wang, Z., et al. (2020). Scaffolds with controlled release of pro-mineralization exosomes to promote craniofacial bone healing without cell transplantation. Acta Biomater. 118, 215–232. doi:10.1016/j.actbio.2020.09.052
Takeuchi, R., Katagiri, W., Endo, S., and Kobayashi, T. (2019). Exosomes from conditioned media of bone marrow-derived mesenchymal stem cells promote bone regeneration by enhancing angiogenesis. PLoS One 14, e0225472. doi:10.1371/journal.pone.0225472
Tan, S. H. S., Wong, J. R. Y., Sim, S. J. Y., Tjio, C. K. E., Wong, K. L., Chew, J. R. J., et al. (2020). Mesenchymal stem cell exosomes in bone regenerative strategies-a systematic review of preclinical studies. Mater Today Bio 7, 100067. doi:10.1016/j.mtbio.2020.100067
Tao, S. C., Yuan, T., Zhang, Y. L., Yin, W. J., Guo, S. C., and Zhang, C. Q. (2017). Exosomes derived from miR-140-5p-overexpressing human synovial mesenchymal stem cells enhance cartilage tissue regeneration and prevent osteoarthritis of the knee in a rat model. Theranostics 7, 180–195. doi:10.7150/thno.17133
Tao, Y., Zhou, J., Wang, Z., Tao, H., Bai, J., Ge, G., et al. (2021). Human bone mesenchymal stem cells-derived exosomal miRNA-361-5p alleviates osteoarthritis by downregulating DDX20 and inactivating the NF-κB signaling pathway. Bioorg Chem. 113, 104978. doi:10.1016/j.bioorg.2021.104978
Tavasolian, F., Hosseini, A. Z., Soudi, S., and Naderi, M. (2020). miRNA-146a improves immunomodulatory effects of MSC-derived exosomes in rheumatoid arthritis. Curr. Gene Ther. 20, 297–312. doi:10.2174/1566523220666200916120708
Tian, X., Wei, W., Cao, Y., Ao, T., Huang, F., Javed, R., et al. (2022). Gingival mesenchymal stem cell-derived exosomes are immunosuppressive in preventing collagen-induced arthritis. J. Cell Mol. Med. 26, 693–708. doi:10.1111/jcmm.17086
Torrecillas-Baena, B., Pulido-Escribano, V., Dorado, G., Gálvez-Moreno, M., Camacho-Cardenosa, M., and Casado-Díaz, A. (2023). Clinical potential of mesenchymal stem cell-derived exosomes in bone regeneration. J. Clin. Med. 12, 4385. doi:10.3390/jcm12134385
Vig, S., and Fernandes, M. H. (2022). Bone cell exosomes and emerging strategies in bone engineering. Biomedicines 10, 767. doi:10.3390/biomedicines10040767
Vitha, A. E., Kollefrath, A. W., Huang, C. C., and Garcia-Godoy, F. (2019). Characterization and therapeutic uses of exosomes: a new potential tool in orthopedics. Stem Cells Dev. 28, 141–150. doi:10.1089/scd.2018.0205
Wang, C., Xu, M., Fan, Q., Li, C., and Zhou, X. (2023). Therapeutic potential of exosome-based personalized delivery platform in chronic inflammatory diseases. Asian J. Pharm. Sci. 18, 100772. doi:10.1016/j.ajps.2022.100772
Wang, D., Cao, H., Hua, W., Gao, L., Yuan, Y., Zhou, X., et al. (2022). Mesenchymal stem cell-derived extracellular vesicles for bone defect repair. Membr. (Basel) 12, 716. doi:10.3390/membranes12070716
Wang, L., Wang, D., Ye, Z., and Xu, J. (2023). Engineering extracellular vesicles as delivery systems in therapeutic applications. Adv. Sci. (Weinh) 10, e2300552. doi:10.1002/advs.202300552
Wang, L., Wang, J., Zhou, X., Sun, J., Zhu, B., Duan, C., et al. (2020). A new self-healing hydrogel containing hucMSC-derived exosomes promotes bone regeneration. Front. Bioeng. Biotechnol. 8, 564731. doi:10.3389/fbioe.2020.564731
Wang, L., Yu, W., Yin, X., Cui, L., Tang, S., Jiang, N., et al. (2021). Prevalence of osteoporosis and fracture in China: the China osteoporosis prevalence study. JAMA Netw. Open 4, e2121106. doi:10.1001/jamanetworkopen.2021.21106
Wang, X., and Thomsen, P. (2021). Mesenchymal stem cell-derived small extracellular vesicles and bone regeneration. Basic Clin. Pharmacol. Toxicol. 128, 18–36. doi:10.1111/bcpt.13478
Wang, Y., Kong, B., Chen, X., Liu, R., Zhao, Y., Gu, Z., et al. (2022). BMSC exosome-enriched acellular fish scale scaffolds promote bone regeneration. J. Nanobiotechnology 20, 444. doi:10.1186/s12951-022-01646-9
Wang, Y., Yu, D., Liu, Z., Zhou, F., Dai, J., Wu, B., et al. (2017). Exosomes from embryonic mesenchymal stem cells alleviate osteoarthritis through balancing synthesis and degradation of cartilage extracellular matrix. Stem Cell Res. Ther. 8, 189. doi:10.1186/s13287-017-0632-0
Wang, Z., Zhao, Z., Gao, B., and Zhang, L. (2022). Exosome mediated biological functions within skeletal microenvironment. Front. Bioeng. Biotechnol. 10, 953916. doi:10.3389/fbioe.2022.953916
Widjaja, G., Jalil, A. T., Budi, H. S., Abdelbasset, W. K., Efendi, S., Suksatan, W., et al. (2022). Mesenchymal stromal/stem cells and their exosomes application in the treatment of intervertebral disc disease: a promising frontier. Int. Immunopharmacol. 105, 108537. doi:10.1016/j.intimp.2022.108537
Wu, J., Kuang, L., Chen, C., Yang, J., Zeng, W. N., Li, T., et al. (2019). miR-100-5p-abundant exosomes derived from infrapatellar fat pad MSCs protect articular cartilage and ameliorate gait abnormalities via inhibition of mTOR in osteoarthritis. Biomaterials 206, 87–100. doi:10.1016/j.biomaterials.2019.03.022
Xia, C., Zeng, Z., Fang, B., Tao, M., Gu, C., Zheng, L., et al. (2019). Mesenchymal stem cell-derived exosomes ameliorate intervertebral disc degeneration via anti-oxidant and anti-inflammatory effects. Free Radic. Biol. Med. 143, 1–15. doi:10.1016/j.freeradbiomed.2019.07.026
Xia, Y., Yang, R., Hou, Y., Wang, H., Li, Y., Zhu, J., et al. (2022). Application of mesenchymal stem cell-derived exosomes from different sources in intervertebral disc degeneration. Front. Bioeng. Biotechnol. 10, 1019437. doi:10.3389/fbioe.2022.1019437
Xiao, Q., Zhao, Z., Teng, Y., Wu, L., Wang, J., Xu, H., et al. (2022). BMSC-derived exosomes alleviate intervertebral disc degeneration by modulating AKT/mTOR-Mediated autophagy of nucleus pulposus cells. Stem Cells Int. 2022, 9896444. doi:10.1155/2022/9896444
Xie, L., Chen, Z., Liu, M., Huang, W., Zou, F., Ma, X., et al. (2020). MSC-derived exosomes protect vertebral endplate chondrocytes against apoptosis and calcification via the miR-31-5p/ATF6 Axis. Mol. Ther. Nucleic Acids 22, 601–614. doi:10.1016/j.omtn.2020.09.026
Xie, X., Xiong, Y., Panayi, A. C., Hu, L., Zhou, W., Xue, H., et al. (2020). Exosomes as a novel approach to reverse osteoporosis: a review of the literature. Front. Bioeng. Biotechnol. 8, 594247. doi:10.3389/fbioe.2020.594247
Xu, H., and Xu, B. (2021). BMSC-derived exosomes ameliorate osteoarthritis by inhibiting pyroptosis of cartilage via delivering miR-326 targeting HDAC3 and STAT1//NF-κB p65 to chondrocytes. Mediat. Inflamm. 2021, 9972805. doi:10.1155/2021/9972805
Xu, T., Hua, Y., Mei, P., Zeng, D., Jiang, S., and Liao, C. (2023). Black phosphorus thermosensitive hydrogels loaded with bone marrow mesenchymal stem cell-derived exosomes synergistically promote bone tissue defect repair. J. Mater Chem. B 11, 4396–4407. doi:10.1039/d3tb00341h
Xu, T., Luo, Y., Wang, J., Zhang, N., Gu, C., Li, L., et al. (2020). Exosomal miRNA-128-3p from mesenchymal stem cells of aged rats regulates osteogenesis and bone fracture healing by targeting Smad5. J. Nanobiotechnology 18, 47. doi:10.1186/s12951-020-00601-w
Xu, W., Liu, X., Qu, W., Wang, X., Su, H., Li, W., et al. (2022). Exosomes derived from fibrinogen-like protein 1-overexpressing bone marrow-derived mesenchymal stem cells ameliorates rheumatoid arthritis. Bioengineered 13, 14545–14561. doi:10.1080/21655979.2022.2090379
Xu, X., Liang, Y., Li, X., Ouyang, K., Wang, M., Cao, T., et al. (2021). Exosome-mediated delivery of kartogenin for chondrogenesis of synovial fluid-derived mesenchymal stem cells and cartilage regeneration. Biomaterials 269, 120539. doi:10.1016/j.biomaterials.2020.120539
Xu, Y., Wang, Q., Wang, X. X., Xiang, X. N., Peng, J. L., He, C. Q., et al. (2022). The effect of different frequencies of pulsed electromagnetic fields on cartilage repair of adipose mesenchymal stem cell-derived exosomes in osteoarthritis. Cartilage 13, 200–212. doi:10.1177/19476035221137726
Yahao, G., and Xinjia, W. (2021). The role and mechanism of exosomes from umbilical cord mesenchymal stem cells in inducing osteogenesis and preventing osteoporosis. Cell Transpl. 30, 9636897211057465. doi:10.1177/09636897211057465
Yang, B. C., Kuang, M. J., Kang, J. Y., Zhao, J., Ma, J. X., and Ma, X. L. (2020). Human umbilical cord mesenchymal stem cell-derived exosomes act via the miR-1263/Mob1/Hippo signaling pathway to prevent apoptosis in disuse osteoporosis. Biochem. Biophys. Res. Commun. 524, 883–889. doi:10.1016/j.bbrc.2020.02.001
Yang, Y., Yuan, L., Cao, H., Guo, J., Zhou, X., and Zeng, Z. (2022). Application and molecular mechanisms of extracellular vesicles derived from mesenchymal stem cells in osteoporosis. Curr. Issues Mol. Biol. 44, 6346–6367. doi:10.3390/cimb44120433
Yao, X. W., Liu, Z. Y., Ma, N. F., Jiang, W. K., Zhou, Z., Chen, B., et al. (2023). Exosomes from adipose-derived stem cells alleviate dexamethasone-induced bone loss by regulating the Nrf2/HO-1 Axis. Oxid. Med. Cell Longev. 2023, 3602962. doi:10.1155/2023/3602962
Ying, C., Wang, R., Wang, Z., Tao, J., Yin, W., Zhang, J., et al. (2020). BMSC-exosomes carry mutant HIF-1α for improving angiogenesis and osteogenesis in critical-sized calvarial defects. Front. Bioeng. Biotechnol. 8, 565561. doi:10.3389/fbioe.2020.565561
You, D. G., Lim, G. T., Kwon, S., Um, W., Oh, B. H., Song, S. H., et al. (2021). Metabolically engineered stem cell-derived exosomes to regulate macrophage heterogeneity in rheumatoid arthritis. Sci. Adv. 7, eabe0083. doi:10.1126/sciadv.abe0083
Yu, H., Huang, Y., and Yang, L. (2022). Research progress in the use of mesenchymal stem cells and their derived exosomes in the treatment of osteoarthritis. Ageing Res. Rev. 80, 101684. doi:10.1016/j.arr.2022.101684
Yu, H., Zhang, J., Liu, X., and Li, Y. (2021). microRNA-136-5p from bone marrow mesenchymal stem cell-derived exosomes facilitates fracture healing by targeting LRP4 to activate the Wnt/β-catenin pathway. Bone Jt. Res. 10, 744–758. doi:10.1302/2046-3758.1012.Bjr-2020-0275.R2
Yu, Y., Li, W., Xian, T., Tu, M., Wu, H., and Zhang, J. (2023). Human embryonic stem-cell-derived exosomes repress NLRP3 inflammasome to alleviate pyroptosis in nucleus pulposus cells by transmitting miR-302c. Int. J. Mol. Sci. 24, 7664. doi:10.3390/ijms24087664
Yuan, W., Wu, Y., Huang, M., Zhou, X., Liu, J., Yi, Y., et al. (2022). A new frontier in temporomandibular joint osteoarthritis treatment: exosome-based therapeutic strategy. Front. Bioeng. Biotechnol. 10, 1074536. doi:10.3389/fbioe.2022.1074536
Zeng, J., Sun, P., Zhao, Y., Fang, X., Wu, Z., and Qi, X. (2023). Bone mesenchymal stem cell-derived exosomes involved co-delivery and synergism effect with icariin via mussel-inspired multifunctional hydrogel for cartilage protection. Asian J. Pharm. Sci. 18, 100799. doi:10.1016/j.ajps.2023.100799
Zeng, Z. L., and Xie, H. (2022). Mesenchymal stem cell-derived extracellular vesicles: a possible therapeutic strategy for orthopaedic diseases: a narrative review. Biomater. Transl. 3, 175–187. doi:10.12336/biomatertransl.2022.03.002
Zhang, D., Xiao, W., Liu, C., Wang, Z., Liu, Y., Yu, Y., et al. (2023). Exosomes derived from adipose stem cells enhance bone fracture healing via the activation of the wnt3a/β-catenin signaling pathway in rats with type 2 diabetes mellitus. Int. J. Mol. Sci. 24, 4852. doi:10.3390/ijms24054852
Zhang, J., Liu, X., Li, H., Chen, C., Hu, B., Niu, X., et al. (2016). Exosomes/tricalcium phosphate combination scaffolds can enhance bone regeneration by activating the PI3K/Akt signaling pathway. Stem Cell Res. Ther. 7, 136. doi:10.1186/s13287-016-0391-3
Zhang, J., Rong, Y., Luo, C., and Cui, W. (2020). Bone marrow mesenchymal stem cell-derived exosomes prevent osteoarthritis by regulating synovial macrophage polarization. Aging (Albany NY). 12, 25138–25152. doi:10.18632/aging.104110
Zhang, J., Zhang, J., Zhang, Y., Liu, W., Ni, W., Huang, X., et al. (2020). Mesenchymal stem cells-derived exosomes ameliorate intervertebral disc degeneration through inhibiting pyroptosis. J. Cell Mol. Med. 24, 11742–11754. doi:10.1111/jcmm.15784
Zhang, J., Zhang, Y., Ma, Y., Luo, L., Chu, M., and Zhang, Z. (2021). Therapeutic potential of exosomal circRNA derived from synovial mesenchymal cells via targeting circEDIL3/miR-485-3p/PIAS3/STAT3/VEGF functional module in rheumatoid arthritis. Int. J. Nanomedicine 16, 7977–7994. doi:10.2147/ijn.S333465
Zhang, L., Jiao, G., Ren, S., Zhang, X., Li, C., Wu, W., et al. (2020). Exosomes from bone marrow mesenchymal stem cells enhance fracture healing through the promotion of osteogenesis and angiogenesis in a rat model of nonunion. Stem Cell Res. Ther. 11, 38. doi:10.1186/s13287-020-1562-9
Zhang, L., Wang, Q., Su, H., and Cheng, J. (2021). Exosomes from adipose derived mesenchymal stem cells alleviate diabetic osteoporosis in rats through suppressing NLRP3 inflammasome activation in osteoclasts. J. Biosci. Bioeng. 131, 671–678. doi:10.1016/j.jbiosc.2021.02.007
Zhang, S., Teo, K. Y. W., Chuah, S. J., Lai, R. C., Lim, S. K., and Toh, W. S. (2019). MSC exosomes alleviate temporomandibular joint osteoarthritis by attenuating inflammation and restoring matrix homeostasis. Biomaterials 200, 35–47. doi:10.1016/j.biomaterials.2019.02.006
Zhang, Y., Hao, Z., Wang, P., Xia, Y., Wu, J., Xia, D., et al. (2019). Exosomes from human umbilical cord mesenchymal stem cells enhance fracture healing through HIF-1α-mediated promotion of angiogenesis in a rat model of stabilized fracture. Cell Prolif. 52, e12570. doi:10.1111/cpr.12570
Zhang, Y., Liu, L., Liu, K., Wang, M., Su, X., and Wang, J. (2022). Regulatory mechanism of circular RNA involvement in osteoarthritis. Front. Surg. 9, 1049513. doi:10.3389/fsurg.2022.1049513
Zhang, Y., Liu, Y., Liu, H., and Tang, W. H. (2019). Exosomes: biogenesis, biologic function and clinical potential. Cell Biosci. 9, 19. doi:10.1186/s13578-019-0282-2
Zhang, Y., Wang, X., Chen, J., Qian, D., Gao, P., Qin, T., et al. (2022). Exosomes derived from platelet-rich plasma administration in site mediate cartilage protection in subtalar osteoarthritis. J. Nanobiotechnology 20, 56. doi:10.1186/s12951-022-01245-8
Zhao, J., Zhang, B., Meng, W., and Hu, J. (2023). Elucidating a fresh perspective on the interplay between exosomes and rheumatoid arthritis. Front. Cell Dev. Biol. 11, 1177303. doi:10.3389/fcell.2023.1177303
Zhou, J., Liu, H. X., Li, S. H., Gong, Y. S., Zhou, M. W., Zhang, J. H., et al. (2019). Effects of human umbilical cord mesenchymal stem cells-derived exosomes on fracture healing in rats through the Wnt signaling pathway. Eur. Rev. Med. Pharmacol. Sci. 23, 4954–4960. doi:10.26355/eurrev_201906_18086
Zhu, Y., Wang, Y., Zhao, B., Niu, X., Hu, B., Li, Q., et al. (2017). Comparison of exosomes secreted by induced pluripotent stem cell-derived mesenchymal stem cells and synovial membrane-derived mesenchymal stem cells for the treatment of osteoarthritis. Stem Cell Res. Ther. 8, 64. doi:10.1186/s13287-017-0510-9
Zou, Z., Li, H., Yu, K., Ma, K., Wang, Q., Tang, J., et al. (2023). The potential role of synovial cells in the progression and treatment of osteoarthritis. Explor. (Beijing) 3, 20220132. doi:10.1002/EXP.20220132
Keywords: MSC-exosomes, skeletal disease, osteoarthritis, osteoporosis, fracture
Citation: Yang X, Zhang S, Lu J, Chen X, Zheng T, He R, Ye C and Xu J (2024) Therapeutic potential of mesenchymal stem cell-derived exosomes in skeletal diseases. Front. Mol. Biosci. 11:1268019. doi: 10.3389/fmolb.2024.1268019
Received: 27 July 2023; Accepted: 16 May 2024;
Published: 06 June 2024.
Edited by:
Alexander V. Glushakov, University of Virginia, United StatesReviewed by:
Fengyuan Zhao, Peking University Third Hospital, ChinaFlavia Rita Argento, University of Florence, Italy
Jessica J. Alm, Karolinska Institutet (KI), Sweden
Daniele Bellavia, Rizzoli Orthopedic Institute (IRCCS), Italy
Copyright © 2024 Yang, Zhang, Lu, Chen, Zheng, He, Ye and Xu. This is an open-access article distributed under the terms of the Creative Commons Attribution License (CC BY). The use, distribution or reproduction in other forums is permitted, provided the original author(s) and the copyright owner(s) are credited and that the original publication in this journal is cited, in accordance with accepted academic practice. No use, distribution or reproduction is permitted which does not comply with these terms.
*Correspondence: Rongxin He, aGVyb25neGluQHpqdS5lZHUuY24=; Chenyi Ye, eWVjaGVueWlAemp1LmVkdS5jbg==; Jianbin Xu, eHU5NzA5NDI2QHpqdS5lZHUuY24=
†These authors have contributed equally to this work