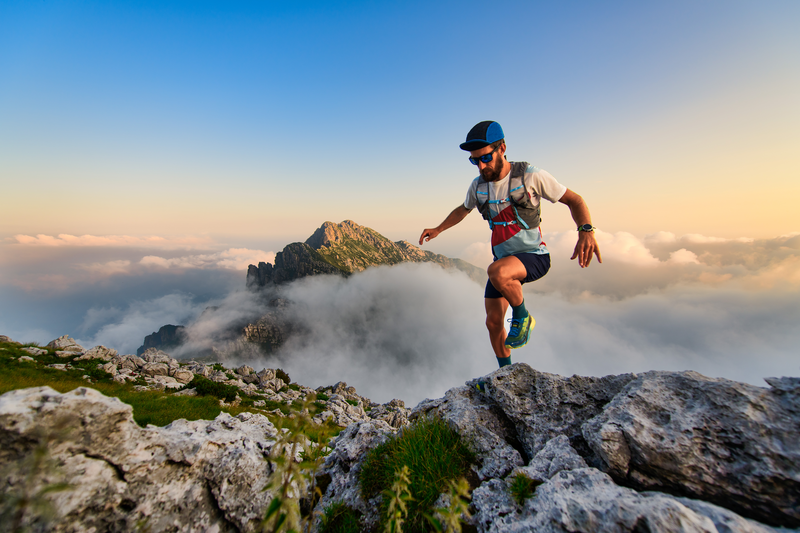
94% of researchers rate our articles as excellent or good
Learn more about the work of our research integrity team to safeguard the quality of each article we publish.
Find out more
REVIEW article
Front. Mol. Biosci. , 04 July 2023
Sec. Molecular Diagnostics and Therapeutics
Volume 10 - 2023 | https://doi.org/10.3389/fmolb.2023.1223493
This article is part of the Research Topic Ferroptosis in Cancer and Beyond, volume II View all 14 articles
Ferroptosis is a novel types of regulated cell death and is widely studied in cancers and many other diseases in recent years. It is characterized by iron accumulation and intense lipid peroxidation that ultimately inducing oxidative damage. So far, signaling pathways related to ferroptosis are involved in all aspects of determining cell fate, including oxidative phosphorylation, metal-ion transport, energy metabolism and cholesterol synthesis progress, et al. Recently, accumulated studies have demonstrated that ferroptosis is associated with gynecological oncology related to steroid hormone signaling. This review trends to summarize the mechanisms and applications of ferroptosis in cancers related to estrogen and progesterone, which is expected to provide a theoretical basis for the prevention and treatment of gynecologic cancers.
Ferroptosis is a unique iron-dependent non-apoptotic cell death and is considered as one of the most widely studied regulated cell death types in the last decade. The concept of ferroptosis is first proposed by Dixon et al., in 2012, they triggered ferroptosis with erastin (a selective lethal small molecule drug targeting the oncogenic RAS) and described its major features (Dixon et al., 2012). During ferroptosis, the outer mitochondrial membrane is ruptured and the mitochondrial cristae reduction is commonly observed in mitochondrial morphology. The regulation of ferroptosis is associated with iron homeostasis and lipid metabolism (Li et al., 2020). Multiple factors caused Fe2+ accumulation that generates numerous reactive oxygen species (ROS) from hydrogen peroxide through the Fenton reaction and lead to ferroptosis. Lipid peroxidation is considered as the primary driver of ferroptosis (Gaschler and Stockwell, 2017; Kagan et al., 2017). Either the depletion of glutathione (GSH) or reduction of glutathione peroxidase 4 (GPX4) activity would attenuate lipid peroxide metabolism, increase ROS level and cause ferroptosis. The molecular mechanism of ferroptosis is involved in a complex regulation network such as system xc−-GSH-GPX4 pathway, serotransferrin-mediated iron uptake, unsaturated fatty acid-mediated lipid peroxidation, and cholesterol synthesis related mevalonate pathway, et al. (Figure 1). We are interested in the crosstalk between ferroptosis and steroid hormone signaling pathway in gynecologic cancers. Here this review trends to summarize the molecular mechanisms of ferroptosis on steroid hormone signaling pathway and its applications in gynecologic cancers.
FIGURE 1. Regulation mechanisms of estrogen and progesterone on ferroptosis in gynecologic cancers. Ferroptosis is driven by iron-dependent lipid peroxidation. There are multiple molecular mechanisms involved in the regulation of ferroptosis. Iron-loaded TF-TFRC complexes releases Fe2+ into the cytoplasm via SLC11A2. The intracellular free iron is stored as Fe3+-ferritin complex. The autophagy-dependent ferritinophagy is mediated by NCOA4 for degradation of ferritin at lysosome to release Fe2+. The excess Fe2+ induces lipid peroxidation via Fenton reaction. PUFAs, which are mainly from ACAC-mediated fatty acid synthesis or by the lipophagy, also can induce ferroptosis. PUFAs convert to PUFA-PL by ACSL4 and LPCAT3, finally induce lipid peroxidation. The system xc−-GSH-GPX4 pathway mainly acts as a defense system so as to antiferroptosis. In this pathway, cystine enters the cell and is oxidized to cysteine, with the action of GCL and GSS, GSH is synthesized and catalyzed by GPX4 to antiferroptosis. IPP, a metabolic intermediate from MVA pathway related to cholesterol synthesis, can enhance GPX4 activity and cause antiferroptotic effect. Transsulfuration pathway, GCH1-BH4 pathway and AIFM 2-CoQ10 pathway also have unique mechanisms to antiferroptosis. Estrogen binds to ESR and reduces free iron by inhibition of TFRC and ferritin or by promotion of ferroportin. Estrogen can also suppress lipid peroxidation through Wnt/β-catenin pathway. Progesterone enhances lipid peroxidation via its receptor PR-A. The other progesterone receptor PGRMC1 also mediated lipophagy to increase ROS generation. Both estrogen and progesterone supplement can inhibit cholesterol synthesis, which regulates GPX4 in turn.
Ferroptosis occurs mainly by targeting two pathways (extrinsic and intrinsic pathways) (Tang and Kroemer, 2020). In the extrinsic pathway, ferroptosis begins with the inhibition of cystine/glutamic acid transporter (system xc−) or with the activity of the serotransferrin (TF)-mediated iron uptake. In the intrinsic pathway, it is activated by blocking intracellular antioxidant enzyme such as GPX 4 (Chen et al., 2021a).
System xc−-GSH-GPX4 pathway is the main defense system to antiferroptosis. The system xc− is comprised of two subunits: solute carrier family 7 member 11(SLC7A11) and solute carrier family 3 members 2 (SLC3A2) (Sato et al., 2000). After the exchange of Cysteine and Cystine, system xc− maintains the GSH generation in a continuous reactions (Lewerenz et al., 2013). In mammalian cells, one of the most important functions of system xc− is mediating Cystine transport by glutamate reverse transportation. Cystine is convert to cysteine, then cysteine is catalyzed by glutamate-cysteine ligase (GCL) and glutathione synthetase (GSS) for GSH synthesis (Bayır et al., 2020). SLC7A11 is commonly used as the target of system xc−. SLC7A11 promotes the expression of GPX4 through the mTORC-4EBP1 signaling pathway (Zhang YY. et al., 2021). Up-regulating the expression of system xc− is involved in the enhanced chemoresistance and tumor growth (Ishimoto et al., 2011; Habib et al., 2015). Inhibiting SLC7A11 causes GSH depletion (Dixon et al., 2012). Down-regulating system xc− by targeting TP53 (Jiang et al., 2015; Liu S. et al., 2022), NFE2L2 (Chen et al., 2017), BAP1 (Zhang et al., 2018), BECN1 or OTUB1(Song et al., 2018), can reduce GSH synthesis, enhance ROS generation, and result in ferroptosis. GPX4 belongs to GSH peroxidases and it is an antiferroptotic molecular (Seibt et al., 2019). It reduces the generation of phospholipid hydroperoxide and converts it to phospholipid alcohol. The GPX4 activity is depended on the presence of GSH and selenium, which finally affect ferroptosis (Ingold et al., 2018). Some small molecular compounds can inhibit GPX4 activity directly or indirectly to induce ferroptosis, and some other compounds can lead to the degradation of GPX4 (Yang et al., 2014; Shimada et al., 2016). High expression level of GPX4 is correlated with bad prognosis in breast cancer (BC) patients, and the GPX4 reduction enhances the sensitivity of cancer cells to cisplatin (Zhang et al., 2020).
Intracellular Fe2+ accumulation is one of central events to induce ferroptosis. The increased iron uptake and the reduced iron storage as well as the limited iron efflux can induce ferroptosis (Chen X. et al., 2020). Iron metabolism capability determines cell susceptibility to ferroptosis by regulating cell labile iron (LIP). Increasing LIP could enhance the Fenton reaction so as to produce more hydroxyl radicals (Feng et al., 2020). The iron-loaded Serotransferrin (TF) binds to Transferrin receptor protein 1 (TFRC) locating in cytomembrane and forms a TF-TFRC complex (Yang and Stockwell, 2008; Wang Y. et al., 2020). The complex releases iron (Fe2+) into the cytoplasm mediated by solute carrier family 11 member 2 (SLC11A2) (Montalbetti et al., 2013; Gao et al., 2015). The intracellular free iron is stored as a ferritin-Fe3+ complex (Muhoberac and Vidal, 2019) and this complex releases Fe2+ through a ferritinophagy manner (Park and Chung, 2019). Ferritinophagy is an autophagy-dependent degradation of ferritin progress. During ferritinophagy, the ferritin-Fe3+ complex is mediated by nuclear receptor coactivator 4 (NCOA4) to degrade in autolysosome and releases Fe2+, which increases cell sensitivity to ferroptosis (Mancias et al., 2014; Mancias et al., 2015; Gatica et al., 2018; Liang et al., 2022). Enhancing iron output or increasing ferritin output can suppress ferritinophagy. The iron output is mediated by solute carrier family 40 member 1 (SLC40A1) in cytomembrane, the ferritin output is mediated by exosome, both of them are able to inhibit ferroptosis (Geng et al., 2018; Brown et al., 2019).
The unsaturated fatty acids-mediated lipid peroxidation is an important pathway to induce lipid peroxidation and ferroptosis. The intracellular free fatty acids are mainly generated from two ways: the first way is the fatty acid de novo synthesis mediated by Acetyl-CoA carboxylase (ACAC); the second way is the fatty acid release derived from lipid droplet (LD). In the fatty acid de novo synthesis progress, acetyl-CoA is catalyzed by ACAC to malonyl-CoA, and then is subjected to a continuous polymerization into fatty acids (Batchuluun et al., 2022). In this progress, several major enzymes (including ACLY, ACSs, ACC, FASN, and SCD1) are involved in fatty acid generation (Li et al., 2022). The excess free fatty acids are usually synthesized into triacylglycerols (TAGs), which are mainly stored in LD. Multiple enzymes regulate LD formation and LD catabolism: during LD formation, GPAT, AGPAT, Lipin and DGAT are required (Tan et al., 2014; Onal et al., 2017); during LD catabolism, ATGL, HSL and MGL are the rate-limiting enzymes of lipolysis that catalyze LD into fatty acids step-by-step (Zechner et al., 2012; Missaglia et al., 2019; Wang T. et al., 2020); in addition to lipolysis, an autophagy-dependent progress referred to lipophagy also has a function of LD breakdown mediated by acid lipases in the autolysosome (Kaur and Debnath, 2015; Schott et al., 2019). The polyunsaturated fatty acids (PUFAs), either from ACAC-mediated de novo synthesis or LD breakdown, are able to trigger ferroptosis. In this progress, long-chain fatty acid–CoA ligase 4 (ACSL4) and lysophospholipid acyltransferase 5 (LPCAT3) are required. Combining with CoA, ACSL4 catalyzes the free arachidonic acid (AA) or adrenergic acid (Ada), which is most likely to undergo peroxidation, to form AA-CoA or Ada-CoA (Yuan et al., 2016; Doll et al., 2017; Kagan et al., 2017). LPCAT3 promotes PUFA-CoA and phospholipid (PL) to form into PUFA-PL, enhances lipid peroxidation and induces ferroptosis (Dixon et al., 2015; Chen et al., 2021a).
Cholesterol can be produced by receptor-mediated LDL-cholesterol uptake or cholesterol de novo synthesis. In cholesterol biosynthesis pathway, three molecules of acetyl-CoA are catalyzed by HMG-CoA synthase into 3-hydroxy-3-methylglutaryl-CoA (HMG-CoA). This CoA-derivative is then converted to Mevalonate (MVA) through a reduction reaction by HMG-CoA reductase (HMGCR), which is a rate-limiting enzyme for cholesterol biosynthesis (Liao et al., 2016; Ni et al., 2021). In the next step, MVA forms isopentenyl diphosphate (IPP) via phosphorylation and decarboxylation (Kuzuyama and Seto, 2012; Bathaie et al., 2017). With catalytic action of the endoplasmic reticulum cyclase and oxygenase, IPP is catalyzed into squalene, then into lanosterol, and is finally converted to cholesterol through multiple steps. In addition, IPP also has a function of antiferroptosis, it promotes the expression of GPX4 to defend lipid peroxidation so as to inhibit ferroptosis (Warner et al., 2000; Ingold et al., 2018). Statins can also reduce GPX4 and inhibit ferroptosis by targeting HMGCR activity through MVA pathway (Yu et al., 2017).
Besides the system xc−, cysteine can also be produced through the thiolation. The transsulfuration pathway is another antioxidant process that inhibits lipid peroxidation. In this pathway, methionine is converted by methionine adenosyltransferase to S-adenosylmethionine and is further converted to S-adenosylhomocysteine (SAH). The S-adenosylhomocysteine hydrolase (SAHH) hydrolyzed SAH to the cysteine precursor homocysteine (HCY) (Chen et al., 2016). It is reported that increasing DJ-1 (an oxidative stress-related protein) promotes the stability of SAHH activity and HCY synthesis through this pathway (Cao et al., 2020). Knockdown of Cysteine-tRNA synthetases (CARS), which is a molecular that links cysteine to tRNA for protein translation, promotes transsulfuration, enhances cysteine synthesis, increases GSH, and inhibits ferroptosis (Yao and Fox, 2013; Hayano et al., 2016).
In addition, Apoptosis-inducing factor mitochondrion-associated 2 (AIFM2)-Coenzyme Q10 (CoQ10) axis is another antiferroptosis pathway. AIFM2 (also known as FSP1) is a NADP-dependent oxidoreductase of CoQ10 (Wei et al., 2020). CoQ10 is a lipophilic compound and is considered as a lipophilic free radical scavenger. It is reported that overexpressing AIFM2 inhibited ferroptosis and positively correlated with ferroptosis resistance in many cancers (Bersuker et al., 2019).
Similarly, the GCH1-BH4 pathway is reported to inhibit lipid peroxidation and defend ferroptosis (Kraft et al., 2020). Tetrahydrobiopterin (BH4) is an integral part of the antioxidant system and GTP cyclohydrolase-1 (GCH1) is the rate-limiting enzyme in the synthesis of BH4 (Latremoliere and Costigan, 2011; Cronin et al., 2018). GCH1 selectively inhibited the peroxidation of certain PUFA-PL, overexpressing GCH1 rescues ferroptosis induced by RSL-3 (a GPX4 inhibitor) in mouse fibroblasts.
It is well known that the main substrate for estrogen and progesterone synthesis is cholesterol. The receptor-mediated LDL-cholesterol uptake and cholesterol de novo synthesis is the major source of cholesterol. In the progress of receptor-mediated LDL-cholesterol uptake, after endocytosis, the cholesterol ester is cleaved by acid lipase in lysosome, free cholesterol is then transferred onto NPC intracellular cholesterol transporter 1 (NPC1) which is located in lysosomal membrane, and followed by a further transport to other organelles (Pfeffer, 2019). Cholesterol also could be generated through cholesterol de novo synthesis pathway. In this pathway, HMG-CoA, which is condensed from acetyl-CoA, is then reduced to MVA by HMGCR, and is further phosphorylated and decarboxylated into IPP. IPP is used to generate cholesterol through multiple steps (Kuzuyama and Seto, 2012; Liao et al., 2016; Bathaie et al., 2017; Ni et al., 2021). Then, as for substrate of steroid hormone synthesis, cholesterol could be catalyzed into estrogen and progesterone. Cholesterol enters into mitochondria, it is then catalyzed to pregnenolone by a cytochrome P450 monooxygenase referred to Cholesterol side-chain cleavage enzyme (CYP11A1 or P450scc) through hydroxylation of the side-chain and cleavage (Strushkevich et al., 2011). Pregnenolone or progesterone could be hydroxylated by Steroid 17-alpha-hydroxylase/17,20 lyase (CYP17A1 or P450c17) to form 17-alpha hydroxy metabolites. Then 17-OH pregnenolone is converted to dehydroepiandrosterone (DHEA) and finally forms estrogens (Auchus et al., 1998; Miller, 2002; DeVore and Scott, 2012; Petrunak et al., 2014; Yoshimoto et al., 2016) (Figure 2). Steroid hormones also have the capability to regulate intracellular cholesterol level in turn. A previously study referred to the effect of steroid hormone on cholesterol synthesis, has demonstrated that the inhibition of cholesterol synthesis could be found in the treatment of many steroids (DHEA, beta-estradiol, pregnenolone, progesterone and deoxycorticosterone included) (Metherall et al., 1996).
FIGURE 2. The relationship between ferroptosis and the synthesis of estrogen and progesterone. The synthesis of estrogen and progesterone depends on cholesterol. Three molecules of acetyl-CoA condense successively to form HMG-CoA. HMG-CoA is then reduced to MVA by HMGCR, and is further phosphorylated and decarboxylated to form IPP. IPP is condensed to produce squalene and then squalene forms lanosterol by the catalysis of endoplasmic reticulum cyclase and oxygenase, finally lanosterol is converted to cholesterol. Cholesterol enters into mitochondria and converts to pregnenolone by P450scc. Then 17-OH pregnenolone is converted to DHEA and finally forms estrogen.
Estrogen is mainly generated by granulosa cells of ovaries (Fuentes and Silveyra, 2019). It maintains normal physiological function such as reproductive system development, body metabolism level regulation, immune regulation and a variety of sex hormone-driven cancers (Baker et al., 2017; Kumar and Goyal, 2021). Estrogen encompasses four estrogenic steroid hormones (estrone, 17-beta-estradiol (E2), estriol and estetrol). Among the four terms, E2 has the highest affinity of estrogen receptors (ERs) (Russell et al., 2019). ERs are divided into two categories: nuclear receptor type and membranous receptor type. The nuclear receptors mainly contain ESR1 (also called ERα) and ESR2 (also named ERβ), while the membranous receptor is G protein-coupled estrogen receptor (GPER1). ESR1 and ESR2 are highly homologous in the amino acid sequence of their DNA binding domain (96%) and the ligand binding domain (55%) (Kuiper and Gustafsson, 1997). ESR1 locates in epithelial and muscle cells of the uterus and vagina, epithelial and stromal cells of the breast, germinal epithelium of the ovary, and testicular interstitial cells (Pelletier and El-Alfy, 2000). ESR2 has a broad distribution of tissues, including the gastrointestinal tract, lung, and brain (Younes and Homma, 2011). They trigger different transcriptional responses and have opposite effects to determine the cell fate (Kwon et al., 2020). In the genomic effects of estrogen-mediated signaling, estrogen binds to ESR1 or ESR2, forms a estrogen-receptor complex, and regulates the transcription of downstream genes in the nucleus by interaction with estrogen response elements (EREs) directly or by tethering to Sp-1 and Ap-1 (Fuentes and Silveyra, 2019; Chen P. et al., 2022). Under physiological condition, estrogen regulates a variety of cellular processes such as autophagy, proliferation, apoptosis, survival, differentiation, and vasodilation. It also regulates Ca2+ mobilization, PI3K signaling, and MAPK pathway through membrane-bound ERs with a non-genomic effect (Chen YC. et al., 2022).
In addition to estrogen, progesterone also plays an important role in gynecologic cancers (Kim et al., 2013). Progesterone is a natural progestin, it is produced from follicular granulosa cells after reaching the luteinizing hormone (LH) peak in the middle of the menstrual cycle, and is mainly produced by the corpus luteum and placenta (Bulletti et al., 2022). Besides the functions of maintaining the implantation of embryonic endometrium and sustain pregnancy, progesterone has multiple biological effects such as utero-relaxation and neuroprotection (Bulletti et al., 1993; Jodhka et al., 2009). In animal models, progesterone affects cognitive ability and suggests its potential role of cognitive ability in human (particularly more relevant for women) (Henderson, 2018). Classical progesterone signaling pathway can be activated by the steroid hormone progesterone through nuclear progesterone receptor (nPR), which has two major isoforms (PR-A and PR-B) (Ali et al., 2023). PR-A is necessary for uterine development and PR-B is necessary for mammary gland development (Conneely and Lydon, 2000). The two isoforms can form a homodimer or heterodimer, and regulate the transcription of downstream genes in the nucleus by interaction with progesterone response elements directly or by tethering to Sp-1 and Ap-1 (Tsai et al., 1988; Tseng et al., 2003; Daniel et al., 2011). Progesterone also activates non-classical pathway via non-nuclear PR containing progesterone receptor membrane component 1 (PGRMC1) and progesterone receptor membrane component 2 (PGRMC2). Numerous studies in endometriosis (EMs) have shown the association of progesterone and iron overload (Van Langendonckt et al., 2002; Van Langendonckt et al., 2004; Lousse et al., 2009). In addition, progesterone takes part in ferroptosis by affecting protein-protein interaction. Progesterone targets Fibulin-1 (FBLN1) in the process of endometrial stromal cells (ESCs) decidualization, FBLN1 interacts with EGF-containing fibrin-like extracellular matrix protein 1 (EFEMP1) and affects the stability of EFEMP1. Silencing of EFEMP1 inhibits the effect of FBLN1 on ferroptosis (Wan et al., 2022).
Ovarian cancer (OVCA) is one of the most lethal malignancies, including epithelial tumor, sexual cord-mesenchymal tumor and germ cell tumor. The epithelial OVCA is most common among all the OVCA types with less than 50% on the 5-year relative survival rate (Torre et al., 2018). High estrogen level is often observed in patients with OVCA, and the estrogen receptor is also high expressed in OVCAs (Mungenast and Thalhammer, 2014; Englert-Golon et al., 2021; Xu et al., 2022). High progesterone level also increases the risk of OVCA. The progesterone signaling promotes the development of high-grade serous carcinoma into metastatic OVCA via BRCA1/DNA repair signaling pathway (Kim et al., 2020).
Endometrial cancer (EC) is the sixth most common cancer in the world. In the United States, its incidence rate increases year by year. The prognosis of patients with recurrent EC and clinical histological detection of aggressive EC is usually limited (Siegel et al., 2019). Surgery, radiotherapy and chemotherapy are the current approach for EC treatment, but have unsatisfactory effects. Estrogen stimulates EC proliferation, while progesterone inhibits it. In estrogen-dependent EC, ESR1 is high expressed, it interacts with GPER and promotes the proliferation of EC cells via PI3K signaling pathway and MAPK signaling pathway (Yu et al., 2022). Progesterone acts to antagonize estrogen in EC. PR is mainly expressed in epithelial cells and stromal cells. It inhibits the proliferation of EC cells with a paracrine manner (Kim et al., 2013; Gompel, 2020).
Cervical cancer (CC) is also most common in women, and almost all cases of cervical squamous cell carcinoma can be attributed to the infection of human papillomavirus (HPV). Basing on their carcinogenic potential, HPVs are classified as low-risk HPV (lrHPV) or high-risk HPV (hrHPV) (Bedell et al., 2020). About 67% of HPV infections will be eliminated within 12 months without intervention and the eliminated rate would reach 90% within 24 months, however, the remaining HPV infections might have high persistent potential (Plummer et al., 2007). Persistent HPV infection will lead to an abnormal proliferation in the lesion region named cervical intraepithelial neoepithelial neoplasia (CIN). According to pathological grade, CIN is classified as CINI, II, and III, or is classified as low-grade squamous intraepithelial lesion (LSIL) and high-grade squamous intraepithelial lesion (HSIL). CINI corresponds to LSIL or dysplasia, CINII and most CINII correspond to HSIL or moderate and severe dysplasia (Jayshree, 2021). CC is a non-estrogen-dependent cancer. But estrogen coordinates with HPV by increasing the DNA double-strand breaks (DSBs), then it promotes proliferation of CC cells through G protein-coupled receptor 30 (GPR30) signaling pathway (Ogawa et al., 2023). The estrogen receptor ESR1 is expressed higher in the nucleus of squamous epithelium than that in cervical lesions. And the expression of ESR1 in squamous epithelium is higher than that in the cervical glands. Progesterone receptor is important for suppression of CC occurrence, low expression of PR may increase the risk of CC, activating PR by medroxyprogesterone acetate reduces the occurrence of CC (Park et al., 2021; Baik et al., 2022). Notably, PGRMC1 has been reported to promote migration of CC cells, siRNA-mediated PGRMC1 knockdown reduces the proliferation of CC cells and inhibits the migration ability of CC cells (Shih et al., 2019).
Besides to gynecologic cancers, breast cancer (BC) is considered to be the most relative cancer of steroid hormone signaling. BC is one of the highest incidence cancers in the world (Duggan et al., 2021). The breast has a unique microenvironment containing a large number of adipocytes. Thus, hormone signaling and lipid metabolism capability may be involved in the invasion and metastasis of BC. In hormone-dependent BC, estrogen has a high level in BC and it can induce DNA damage by metabolites (Starek-Świechowicz et al., 2021). Estrogen activates PI3K signaling pathway by ERs to promote cell proliferation of BC cells where ERs is highly expressed (Vasan et al., 2019). Progesterone influences early events in the occurrence of BC. PR-B mediates the proliferation of BC cells related to progesterone and it also regulates the actions of extranuclear signaling of PRs (Trabert et al., 2020).
The relation between ferroptosis and steroid hormone signaling has been studied in a variety of cancer types from molecular mechanism to the development of targeted therapeutic drug. Activation of estrogen-related receptor gamma (ESRRG) may enhance the effect of ferroptosis in HCC cells with sorafenib-resistant (Kim et al., 2022). Increasing PGRMC1 expression promotes fatty acid oxidation and enhances the sensitivity of paclitaxel-tolerant cancer cells (PCC) to ferroptosis in head and neck cancer (You JH. et al., 2021). It is well known that most gynecologic cancers (including EC, CC and OVCA) are driven by sex hormone (Baker et al., 2017), so deeply understanding the crosstalk between ferroptosis and steroid hormone signaling is helpful for tumor-targeted therapy in gynecologic cancers. In molecular mechanism, the effect of steroid hormone signaling on ferroptosis is complex (Figure 1). Firstly, steroid hormone signaling is able to regulate iron homeostasis. Secondly, abnormal steroid hormone level may affect the endogenous antioxidant capacity.
In most of gynecologic cancers, estrogen disrupts intracellular iron level and promotes free iron export into systemic circulation. Hepcidin is an important regulator of systemic iron balance. A previous study on female mouse model has reported that estrogen can reduce the expression of hepcidin (Yang et al., 2020). An in vivo study has reported that serum hepcidin levels declines more than 40% after E2 treatment in females (Lehtihet et al., 2016). Estrogen represses hepcidin expression via ERE of its promoter region; ovariectomizing reduces serum iron level in mice, but elevates the tissue iron level (Hou et al., 2012). Besides to hepcidin, estrogen also affects iron uptake and iron export in multiple gynecologic cancer types (Bajbouj et al., 2019; Riera Leal et al., 2020). Ovarian clear cell carcinoma (CCC) is a most common OVCA type. Among endometriosis-associated OVCA (EAOC), the positive percentage of ESR in CCC is only 8%, which is lower than the other OVCA types (Lai et al., 2013). In a previously study of CCC, it is found that free iron levels in endometriotic cysts and CCCs are both higher than that in nonendometriotic benign cysts (Yamaguchi et al., 2008). In doxorubicin-treated OVCA cells and BC cells, E2 inhibits the expression of TFRC but promotes that of ferroportin and ferritin (Bajbouj et al., 2019). In BCs, from a study of ferroptosis induced by sulfasalazine, it is reported that the expression level of TFRC in ER-positive BCs is much lower than that in the ER-negative BCs, and ESR1 knockdown increases TFRC expression (Yu et al., 2019). From a meta-analysis of CC patients in China, the result indicates that high serum iron levels may have a protective function for CC patients (Chen S. et al., 2020). In CC cell lines, the effect of E2 that it reduces free iron and intracellular ferritin level is only found in HaCaT cells (Riera Leal et al., 2020). However, this effect is not found in some other CC cell types (HeLa, SiHa and C33A) (Riera Leal et al., 2020). Progesterone is another important steroid sex hormone with a function of maintaining iron level, but sometimes its effect on iron regulation is contradictory. In zebrafish model, progesterone promotes the degradation of ferroportin and enhances the transcriptional expression of hepcidin (Li et al., 2016). PGRMC1, rather than the classical PRs, mediates the activity of SRC family kinases to promote hepcidin biosynthesis, and this effect can be rescued by the inhibition of SRC family kinase (Li et al., 2016). Moreover, PGRMC1 is found expressed higher in triple-negative BC (TNBC) than that in the other BC subtypes. Overexpression of PGRMC1 reduces free iron level and inhibits ferroptosis by binding to intracellular iron; the inhibition of PGRMC1 enhances sensitivity of BC cells (MDA-MB231) to ferroptosis inducer (Zhao et al., 2023).
Abnormal steroid hormone level also affects antioxidant capacity of cancer cells so as to regulate their sensitivity to ferroptosis. For instance, IPP, an intermediate product in cholesterol synthesis, could defend ferroptosis by promoting GPX4 activity (Warner et al., 2000; Ingold et al., 2018); while inhibiting HMGCR activity by statins could also inhibit GPX4 through the same pathway (Yu et al., 2017). Moreover, increasing Sterol regulatory element-binding protein 2 (SREBP2), which promotes cholesterol synthesis by targeting HMGCR, could also suppress ferroptosis (Hong et al., 2021). Because steroid hormones (E2 and progesterone) have been proven to inhibit cholesterol synthesis (Metherall et al., 1996), thus, steroid hormone may act to antiferroptosis. Estrogen has been reported to protect against oxidative stress by promoting the expression of mitochondrial antioxidant enzymes (SOD2, GPXs), increasing antioxidants and reducing free radicals in many organs and cells (Irwin et al., 2008; Vina and Borras, 2010). In CC cells, E2 reduces NO level and MDA level (final product of the lipid peroxidation). This effect could be reversed by metformin treatment (Riera Leal et al., 2020). It can also inhibit oxidative stress through Wnt/β-catenin signaling pathway in ovarian endometrioid adenocarcinoma (Wu et al., 2007). Progesterone is reported to increase ROS level in sperm and fallopian tube fibroblasts (Gimeno-Martos et al., 2020; Wu et al., 2023). Progesterone induces ROS generation and suppresses OVCA via its receptor PR-A (Wu et al., 2017). Fallopian tube (FT) is well known as the origin of high-grade serous ovarian cancer, and defective p53 is considered as an early event in the FT epithelium-to-OVCA transition. After progesterone treatment, combining with more ROS generation, necroptosis is activated via TNF-α/RIPK1/RIPK3/MLKL pathway in p53-defective human FT fimbrial epithelial cell line (FE25 cells). The antioxidant Necrox-2 and acetylcysteine could rescue this effect (Wu et al., 2017).
Simply elevating iron level may lead to an unexpected effect. From a study of ECOA, the authors indicate that iron-induced oxidative stress may promote the production of the antioxidants, and follow by apoptosis-resistance malignant transformation of endometriosis (Kobayashi et al., 2019). A recent study also reports that persistent and mild ferroptosis increases the expression of antioxidant genes and promotes initiation of HPV-positive CC (Wang T. et al., 2022). Iron deprivation with iron chelators represses HPV E6/E7 oncogene expression and has profound antiproliferative effects in HPV-positive CC cells (HeLa and SiHa) (Braun et al., 2020). Thus, targeting ferroptosis for killing serous gynecologic cancer cells should consider their respective features (steroid hormones and the expression levels of their receptors).
Ferroptosis is important for repressing the occurrence, development and metastasis of gynecologic cancers (Fan et al., 2022). In OVCA, a lot of studies have proven that enhancing lipid peroxidation is important for inhibiting OVCA cells, providing us with an emerging strategy for the OVCA treatment (Park et al., 2018; Zhao et al., 2019; Zhao X. et al., 2022). Resent study has indicated that drug-resistant OVCA cells are vulnerable to GPX4 inhibition, the ferroptosis inducer (RSL3) suppress the viability of drug-resistant OVCA cells but less affect the parental cells (Hangauer et al., 2017). Another study has proven that inhibition of monounsaturated fatty acids generation by the blockage of SCD1 sensitizes OVCA cells to RSL3 (Tesfay et al., 2019). Moreover, the inhibition of ROS generation through the Nrf2/heme oxygenase 1 (HMOX1) signaling pathway, promotes the cell proliferation of cisplatin-resistant OVCA cells (Sun et al., 2019). All the studies suggest that the drug resistance of OVCA cells depends on the endogenic antioxidant system. Ferroptosis is also proved to have a synergistic effect with chemotherapy, radiotherapy and immunotherapy in killing OVCA cells (Zhao H. et al., 2022). Ferroptosis can be induced in OVCA cells by artesunate in a ROS-dependent manner, transferrin pretreatment enhances this effect and ferrostatin-1 can rescue (Greenshields et al., 2017). Basing on the effect of ferroptosis in OVCA, a scoring system related to ferroptosis genes is used to predict the prognosis of OVCA patients (You Y. et al., 2021). Thus, there are convincing evidences to show a closely relationship of OVCA and ferroptosis.
Ferroptosis is found in SILs from patients with hrHPV infection and persistent ferroptosis contributes to an anti-ferroptosis effect in CC (Wang T. et al., 2022). In addition, more ROS generation is found in cancer cells because the cancer cells require large amount of nutrients for rapid proliferation (Wang et al., 2019). So, cancer cells increase the antioxidant activity to maintain the redox balance and prevent from cell death caused by high level of ROS. Lipid peroxidation is one of the most obvious features of ferroptosis, and its importance in CC has been proven in many studies, providing us with new insight for CC treatment by targeting ferroptosis (Jelić et al., 2018; Jelic et al., 2021).
Recently, the study on the relation between ferroptosis and EC gets many attentions. Basing on the expression profiles of cancer genomic database, some research teams have described the characteristics of ferroptosis-related genes in EC, and further provided evidences to describe the relationship between ferroptosis and the immune microenvironment, suggesting that ferroptosis-related genes could be used for the prognosis prediction of EC (Liu J. et al., 2021; Weijiao et al., 2021; Liu L. et al., 2022). A recently study has identified a centrosome microtubule-binding protein Centrosome spindle pole-associated protein (CSPP1), which functions in cell cycle-dependent cytoskeletal tissue and ciliation, to be a potential biomarker of ferroptosis, providing a novel target for the diagnosis, prognosis and therapy of EC (Wang W. et al., 2022). Another study has shown that ETS transcription factor ELK1 (ELK1) is upregulated in EC cells, and it binds to the promoter of GPX4 to antiferroptosis, indicating the ELK1/GPX4 axis might be a potential therapeutic target to develop drugs for EC (Wei et al., 2022).
Besides to gynecologic cancers, numerous studies of ferroptosis on the development and treatment of BC have been reported. And ferroptosis is considered to be a potential and valuable research direction for the treatment of BC (Sui et al., 2022). Some randomized controlled studies have reported that TF level is positively related to the incidence of ER-negative BC (Hou et al., 2021); the dietary iron supplementation is negatively correlated with the risk of BC; but in the postmenopausal women, heme iron is positively correlated with the risk of ER-positive and/or PR-positive BC (Chang et al., 2020). Iron transport protein and hepcidin have protective functions for BC patients (Pinnix et al., 2010), and the expression of TFRC is positively related to the quantity of immunocytes in BC patients (Chen et al., 2021b). Interferon -γ (IFN-γ) secreted from immunocytes suppress the cystine-uptake by reducing SLC7A11 (a ferroptosis related gene) in BC cells, followed by a lipid peroxidation and ferroptosis (Wang W. et al., 2019). In BC tissues, SLC7A11 expresses higher than that in adjacent normal tissues. From a study of IR on BC, it is positively correlated with ESR1. ESR1 promotes SLC7A11 expression early after IR, either ESR1 or SLC7A11 knockdown enhances ferroptosis induced by IR in the ER-positive BC cells (Liu R. et al., 2021). It is reported that ESR1 inhibition enhances the sensitivity of ER-positive BC cells to ionizing radiation (IR) by inducing ferroptosis (Liu and Gu 2022). Drugs such as siramesine and lapatinib can reduce GSH level, increase ROS generation, and induce ferroptosis in BC cells (Ma et al., 2017). MUC1-C is able to interact with CD44v (CD44 variant) to stable the system xc−, increase GSH level and result in antiferroptosis in BC cells (Hasegawa et al., 2016). Notably, drug-resistant BC cells exhibits a dependence of GPX4 activity, thus, targeting GPX4 to induce ferroptosis might potentially overcome drug-resistant BC (Hangauer et al., 2017). A recently study has developed a series of small molecules that trigger to ferroptosis, and verified the effect on selectively killing drug-resistant BC stem cell-like cells (bCSC) with mesenchymal phenotypes in vitro (Taylor et al., 2019).
The hormone-targeted therapy in BC can be divided into three broad categories. The first category is selective estrogen receptor modulator (SERM), which functions by binding to ER to block estrogen, including Tamoxifen, Toremifene and Fulvestrant. Tamoxifen is most commonly used in ER + BC (Legha, 1988; Yang et al., 2013), Toremifene has a comparable efficacy of Tamoxifen (Zhou et al., 2011), and Fulvestrant is the latest generation of ER inhibitor for the treatment of ER + BC (Chen P. et al., 2022). The second category is aromatase inhibitors, which functions to inhibit estrogen synthesis, including Letrozole (Mukherjee et al., 2022), Anastrozole (Nabholtz, 2006), and Exemestane (Wang Y. et al., 2022). The third category is the progesterone analogue, which functions to active PRs, including megestrol and medroxyprogesterone. Megestrol acetate (MA) is one of the first pregnancy promotors to be evaluated for hormonal treatment of advanced BC (Sedlacek, 1988). Some clinical trials have shown that luteinizing hormone releasing hormone receptor antagonists, such as Goserrelin, are also effective for BC (Moore et al., 2015).
Basing on the steroid hormone signaling characteristics of gynecological cancers, there are a lot of steroid hormone-targeted drugs applied for cancer therapy. Letrozole (an aromatase inhibitors) is used for the treatment of low-grade serous ovarian cancer and high-grade serous ovarian cancer by inhibition of estrogen generation (Heinzelmann-Schwarz et al., 2018). Besides Letrozole, there are some the third-generation aromatase inhibitors such as Anastrozole and Exemestane. Fulvestrant has a high affinity of ER and downregulates its expression. Fluvestrant is effective for in patients with disease recurrence after endocrine therapy, some ongoing clinical trials suggest that Fluvestrant may be effective in OVCA (Bidard et al., 2022; Cristofanilli et al., 2022).
Because CC is a non-hormone-responsive cancer, steroid hormone-targeted tumor therapy is not common in CC. But there are some studies which have reported the relation between long-term oral contraceptives and the increasing risk of CC (Chung et al., 2010), which reminds us to concerned about the use of hormone replacement therapy in patients with CC.
For the treatment of EC, steroid hormone-targeted drugs are usually applied in well-differentiated endometrioid adenocarcinoma, young women with early stage EC who need to maintain fertility, and patients with advanced, recurrent, or inoperable EC. Medroxyprogesterone acetate (MPA) and MA are high-potency progesterone drugs that commonly used in EC, Levonorgestrel-releasing intrauterine device (LNG-IUD) also appears to be an alternative therapy in patients who do not tolerate oral therapy (Garzon et al., 2021; Zhao H. et al., 2022; Markowska et al., 2022). The application of SERM, gonadotropin-releasing hormone agonist (GnRH agonist) (Emons and Gründker, 2021) or aromatase inhibitors is good option for the treatment of EC (Zhang et al., 2019; Markowska et al., 2022). Tamoxifen is a selective estrogen receptor modulator that is effective for low-toxicity advanced or recurrent EC (Emons et al., 2020). Some clinical trials suggest that Fluvestrant is effective for EC (Battista and Schmidt, 2016; Bogliolo et al., 2017) and other aromatase inhibitors (letrozole, anastrozole) also have meaningful efficacy in patients with recurrent EC (Slomovitz et al., 2015; Heudel et al., 2022; Slomovitz et al., 2022).
There is a good application prospect of ferroptosis induced by the blockage of endogenous antioxidant system or by the regulation of intracellular free iron level in immunotherapy, radiation therapy and drug treatment of gynecologic cancers (Table 1). For example, MA-resistant EC cells are susceptible to ferroptosis (Murakami et al., 2023); Carboplatin is effective for estrogen-resistant BC (Larsen et al., 2012). Immune checkpoint inhibitor (ICI) has made unprecedented breakthrough in some cancer types (Hernandez et al., 2022), however, due to lack of tumor-infiltrating lymphocytes, numerous cancer types with poor prognosis after ICI immunotherapy remain. A recent study has reported that CD8+T cells release cytokines to induce ferroptosis in OVCA cells (ID8) by suppressing system xc−, restraining cystine uptake, and enhancing lipid peroxidation (Wang K. et al., 2019). For radiation therapy (RT), there are plenty of evidences supporting its association with ferroptosis in cancers from multiple organs, including breast, ovarian, vulvar, and melanoma (Lang et al., 2019; Lei et al., 2020; Ye et al., 2020). Ionizing radiation (IR) inhibits system xc− in an ATM-dependent manner, which is a core component of DNA damage/repair systems (Matsuoka et al., 2007). In addition, Olaparib (a PARP inhibitor) can repress system xc− and induces ferroptosis in OVCA cells (Zhang et al., 2021a).
Artemisinin is proposed to promote free radical generation by Fe2+ (Li and Zhou, 2010). Its safety on intravenous or intravaginal administration in patients with advanced solid tumors (NCT 02353026) and cervical intraepithelial neoplasias (NCT 02354534) have been evaluated (Chen et al., 2021c; von Hagens et al., 2017). Artesunate (ART) can promote ferritinophagy to release free iron, and thus induce ferroptosis (Eling et al., 2015; Ooko et al., 2015; Lin et al., 2016; Du et al., 2019). ART-conjugated phosphorescence rhenium (I) complexes can promote ROS generation and induce ferroptosis in OVCA and CC cells (Shield et al., 2009; Greenshields et al., 2017; Ye et al., 2021). Sorafenib is able to inhibit GSH and promote ROS generation in CC cells (Wang C. et al., 2021). Quinones inhibits cell growth of EC cells by inducing ferroptosis through both iron regulation and blockage of endogenous antioxidant system (Zhang et al., 2021b).
In addition, unsaturated fatty acid-mediated lipid peroxidation could be activated by IR, leading to ferroptosis in BC cells (MCF-7) (Lei et al., 2020). Sulfasalazine (SSZ) has recently been recognized as a system xc− inhibitor (Babu and Muckenthaler, 2016). It affects iron metabolism by disruption of circadian rhythms of TFRC expression (Yoshida, 2015; Okazaki et al., 2017; Yoshida, 2018). SSZ has been planned to be administered for clinical therapy of patients with BC and chronic pain from a Phase I clinical trial (NCT03847311). Statins can inhibit GPX4 and CoQ10 through MVA pathway, which triggers ferroptosis (Shimada et al., 2016; Viswanathan et al., 2017). Atorvastatin and fluvastatin also have antiproliferative effects on the BC cells with high expressing HMGCR (Garwood et al., 2010; Bjarnadottir et al., 2013). Lapatinib (a tyrosine kinase inhibitor) is reported to induce ferroptosis by activation of autophagy-dependent ferritinophagy in BC cells (Ma et al., 2017). Vitamin C can promote ferritinophagy for the release of free iron and to increase ROS level by Fenton reaction (Wang C. et al., 2021). It is reported to inhibit BC by targeting miR-93-Nrf2 axis (Singh et al., 2013).
Furthermore, steroid hormone-targeted therapy combining with ferroptosis inducer is applied in some gynecologic cancers with drug resistance. More than 30 years ago, the treatment of cisplatin, doxorubicin, cyclophosphamide, and MA is used for recurrent and metastatic EC (Hoffman et al., 1989). Besides the effect on ferroptosis, Artemisinin selectively reduces ESR1 level. The tamoxifen–artemisinin hybrids and estrogen–artemisinin hybrid compounds are highly active against BC cells (MCF-7) (Fröhlich et al., 2020).
Gynecologic cancers are the most common malignant cancers in women. They affect thousands of lives and have attracted public attention due to the increased incidence rate worldwide. As a new type of RCD, ferroptosis has become a hot-spot issue in cancer research. In the past decade, there have been many studies on various aspects of ferroptosis, including molecular mechanisms, metabolic pathways, regulatory factors and tumor-related signaling pathways. Although some studies have proposed the importance of ferroptosis in gynecologic cancers, however, the underlying molecular mechanisms involved in ferroptosis and the occurrence and development of gynecological cancers have not yet been fully elucidated. We should also notice the relationship between steroid hormone signaling and ferroptosis in gynecologic cancers. The steroid hormone levels are distinct due to gynecologic cancer types, and the expression levels of steroid hormone receptors also have great difference. We examined the expression levels of steroid hormone signaling-related genes (ESR1, ESR2, PR and PGRMC1) in gynecologic cancer tissues and their adjacent samples from TCGA RNA seq data (Supplementary Figure S1). From the TCGA RNA seq data of CC with 317 patients documented, we found that ESR1 and PR were decreased in primary tumors and metastatic tumors compared to that in normal tissues, while ESR2 and PGRMC1 were increased in metastatic tumors compared to that in normal tissues and primary tumors. From the TCGA RNA seq data of OVCA with 758 patients documented, we did not find significant difference of the four genes between primary tumors and recurrent tumors. From the TCGA RNA seq data of EC with 606 patients documented, PR was decreased in primary tumors compared to that in normal tissues, while the other genes were not changed. From the TCGA RNA seq data of BC with 1,284 patients documented, ESR1 was increased in primary tumors and metastatic tumors compared to that in normal tissues, while ESR2 was decreased in primary tumors and metastatic tumors. In addition, ESR2 was found a low expression level in CC, OVCA and EC tissues. All the four genes were expressed much higher in BC tissues than that of CC, OVCA and EC tissues (Supplementary Figure S1). We next examined the expression levels of ferroptosis-related genes (GPX4, TFRC, HMGCR and ACSL4) in gynecologic cancer tissues and their adjacent samples from TCGA RNA seq data. From the TCGA RNA seq data of CC, we found that GPX4, TFRC and HMGCR were increased in primary tumors and metastatic tumors compared to that in normal tissues, while ACSL4 was decreased (Supplementary Figure S2). From the TCGA RNA seq data of OVCA, only ACSL4 was increased in recurrent tumors compared to that in primary tumors. From the TCGA RNA seq data of EC, we found that GPX4, TFRC and HMGCR were increased in primary tumors compared to that in normal tissues, while ACSL4 was not changed. From the TCGA RNA seq data of BC, only ACSL4 was decreased in primary tumors and metastatic tumors compared to that in normal tissues, while the other genes were not changed (Supplementary Figure S2).
It is important to select the best manner to trigger ferroptosis basing on the expression levels of ferroptosis-genes and characteristic of steroid hormone signaling in gynecologic cancers. In OVCA, due to the high expression of ER and low expression of PR, inhibition of estrogen signaling or activation of non-classical progesterone signaling pathway mediated by PGRMC1 are beneficial to ferroptosis. PGRMC1 has been reported to induce ferroptosis by enhancing LD lipolysis in other cell type, thus increasing unsaturated fatty acid generation to enhance lipid peroxidation and inhibiting GPX4 to reduce the antioxidant ability of OVCA cells may induce ferroptosis.
During CC development, we should also notice the function of PGRMC1 rather than ESR1 and PR. Although serotransferrin-mediated iron uptake is increased and PGRMC1 can promote LD lipolysis, but the decrease of ACSL4 may weaken this effect. The activation of the MVA pathway and the elevated GPX4 expression may constitute a strong antioxidant defense system against ferroptosis. Thus, stains and GPX4-targeted drugs may have a better treatment effect to trigger ferroptosis in CC.
In EC, due to the high expression of ESR1, PR and PGRMC1, inhibition of estrogen signaling or activation of progesterone signaling may have good effect for inducing ferroptosis. Basing on the elevated expression of TFRC and HMGCR in EC, drugs enhancing ferritinophagy and inhibiting the MVA pathway may induce ferroptosis of EC cells.
During the progress of BC development, both inhibition of estrogen signaling and enhance of progesterone signaling are necessary. Although the decrease of ACSL4 may weaken ferroptosis, enhanced LD lipolysis is still beneficial in promoting ferroptosis because the breast is rich in fat. In addition, stains and GPX4-targeted drugs may also have good treatment effect in BC. Thus, basing on the features of ferroptosis and steroid hormone signaling, the combination of steroid hormone-targeted tumor therapy and ferroptosis-targeted tumor therapy is expected to solve the problem of drug-resistance and may further enhance therapeutic efficiency of gynecologic cancers.
How to use the ferroptosis mechanism for tumor-targeted therapy still has a long way to go in gynecologic cancers. In the future, we hope to investigate the regulatory mechanism of ferroptosis on the estrogen and progesterone signaling pathways in order to provide a theoretical basis for the prevention and treatment of gynecological cancers.
WL and TW wrote the manuscript and were involved with submission; JC and QL were involved with project concept; WL, JC, and QL performed data collection; WL and TW revised the manuscript and were responsible for final approval; All authors contributed to the article and approved the submitted version.
This work is supported by Open Project of Jiangsu Biobank of Clinical Resources (Grant/Award Number: TC2021B013) and the Nanjing Healthcare Science and Technology Development Special Funded Project (Grant/Award Number: YKK20197).
The authors declare that the research was conducted in the absence of any commercial or financial relationships that could be construed as a potential conflict of interest.
All claims expressed in this article are solely those of the authors and do not necessarily represent those of their affiliated organizations, or those of the publisher, the editors and the reviewers. Any product that may be evaluated in this article, or claim that may be made by its manufacturer, is not guaranteed or endorsed by the publisher.
The Supplementary Material for this article can be found online at: https://www.frontiersin.org/articles/10.3389/fmolb.2023.1223493/full#supplementary-material
Ali, M., Ciebiera, M., Vafaei, S., Alkhrait, S., Chen, H. Y., Chiang, Y. F., et al. (2023). Progesterone signaling and uterine fibroid pathogenesis; molecular mechanisms and potential therapeutics. Cells 12 (8), 1117. doi:10.3390/cells12081117
Auchus, R. J., Lee, T. C., and Miller, W. L. (1998). Cytochrome b5 augments the 17,20-lyase activity of human P450c17 without direct electron transfer. J. Biol. Chem. 273 (6), 3158–3165. doi:10.1074/jbc.273.6.3158
Babu, K. R., and Muckenthaler, M. U. (2016). miR-20a regulates expression of the iron exporter ferroportin in lung cancer. J. Mol. Med. Berl. 94 (3), 347–359. doi:10.1007/s00109-015-1362-3
Baik, S., Mehta, F. F., Unsal, E., Park, Y., and Chung, S. H. (2022). Estrogen inhibits epithelial progesterone receptor-dependent progestin therapy efficacy in a mouse model of cervical cancer. Am. J. Pathol. 192 (2), 353–360. doi:10.1016/j.ajpath.2021.10.008
Bajbouj, K., Shafarin, J., and Hamad, M. (2019). Estrogen-dependent disruption of intracellular iron metabolism augments the cytotoxic effects of doxorubicin in select breast and ovarian cancer cells. Cancer Manag. Res. 11, 4655–4668. doi:10.2147/CMAR.S204852
Baker, J. M., Al-Nakkash, L., and Herbst-Kralovetz, M. M. (2017). Estrogen-gut microbiome axis: Physiological and clinical implications. Maturitas 103, 45–53. doi:10.1016/j.maturitas.2017.06.025
Batchuluun, B., Pinkosky, S. L., and Steinberg, G. R. (2022). Lipogenesis inhibitors: Therapeutic opportunities and challenges. Nat. Rev. Drug Discov. 21 (4), 283–305. doi:10.1038/s41573-021-00367-2
Bathaie, S. Z., Ashrafi, M., Azizian, M., and Tamanoi, F. (2017). Mevalonate pathway and human cancers. Curr. Mol. Pharmacol. 10 (2), 77–85. doi:10.2174/1874467209666160112123205
Battista, M. J., and Schmidt, M. (2016). Fulvestrant for the treatment of endometrial cancer. Expert Opin. Investig. Drugs 25 (4), 475–483. doi:10.1517/13543784.2016.1154532
Bayır, H., Anthonymuthu, T. S., Tyurina, Y. Y., Patel, S. J., Amoscato, A. A., Lamade, A. M., et al. (2020). Achieving life through death: Redox biology of lipid peroxidation in ferroptosis. Cell Chem. Biol. 27 (4), 387–408. doi:10.1016/j.chembiol.2020.03.014
Bedell, S. L., Goldstein, L. S., and Goldstein, A. R. (2020). Cervical cancer screening: Past, present, and future. Sex. Med. Rev. 8 (1), 28–37. doi:10.1016/j.sxmr.2019.09.005
Bersuker, K., Hendricks, J. M., Li, Z., Magtanong, L., Ford, B., Tang, P. H., et al. (2019). The CoQ oxidoreductase FSP1 acts parallel to GPX4 to inhibit ferroptosis. Nature 575 (7784), 688–692. doi:10.1038/s41586-019-1705-2
Bidard, F. C., Hardy-Bessard, A. C., Dalenc, F., Bachelot, T., Pierga, J. Y., de la Motte Rouge, T., et al. (2022). Switch to fulvestrant and palbociclib versus no switch in advanced breast cancer with rising ESR1 mutation during aromatase inhibitor and palbociclib therapy (PADA-1): A randomised, open-label, multicentre, phase 3 trial. Lancet Oncol. 23 (11), 1367–1377. doi:10.1016/S1470-2045(22)00555-1
Bjarnadottir, O., Romero, Q., Bendahl, P. O., Jirström, K., Rydén, L., Loman, N., et al. (2013). Targeting HMG-CoA reductase with statins in a window-of-opportunity breast cancer trial. Breast Cancer Res. Treat. 138 (2), 499–508. doi:10.1007/s10549-013-2473-6
Bogliolo, S., Cassani, C., Dominoni, M., Orlandini, A., Ferrero, S., Iacobone, A. D., et al. (2017). The role of fulvestrant in endometrial cancer. Expert Opin. Drug Metab. Toxicol. 13 (5), 537–544. doi:10.1080/17425255.2016.1244264
Braun, J. A., Herrmann, A. L., Blase, J. I., Frensemeier, K., Bulkescher, J., Scheffner, M., et al. (2020). Effects of the antifungal agent ciclopirox in HPV-positive cancer cells: Repression of viral E6/E7 oncogene expression and induction of senescence and apoptosis. Int. J. cancer 146 (2), 461–474. doi:10.1002/ijc.32709
Brown, C. W., Amante, J. J., Chhoy, P., Elaimy, A. L., Liu, H., Zhu, L. J., et al. (2019). Prominin2 drives ferroptosis resistance by stimulating iron export. Dev. Cell 51 (5), 575–586.e4. doi:10.1016/j.devcel.2019.10.007
Bulletti, C., Bulletti, F. M., Sciorio, R., and Guido, M. (2022). Progesterone: The key factor of the beginning of life. Int. J. Mol. Sci. 23 (22), 14138. doi:10.3390/ijms232214138
Bulletti, C., Prefetto, R. A., Bazzocchi, G., Romero, R., Mimmi, P., Polli, V., et al. (1993). Electromechanical activities of human uteri during extra-corporeal perfusion with ovarian steroids. Hum. Reprod. 8 (10), 1558–1563. doi:10.1093/oxfordjournals.humrep.a137891
Cao, J., Chen, X., Jiang, L., Lu, B., Yuan, M., Zhu, D., et al. (2020). DJ-1 suppresses ferroptosis through preserving the activity of S-adenosyl homocysteine hydrolase. Nat. Commun. 11 (1), 1251. doi:10.1038/s41467-020-15109-y
Chang, V. C., Cotterchio, M., Bondy, S. J., and Kotsopoulos, J. (2020). Iron intake, oxidative stress-related genes and breast cancer risk. Int. J. Cancer 147 (5), 1354–1373. doi:10.1002/ijc.32906
Chen, D., Tavana, O., Chu, B., Erber, L., Chen, Y., Baer, R., et al. (2017). NRF2 is a major target of ARF in p53-independent tumor suppression. Mol. Cell 68 (1), 224–232.e4. doi:10.1016/j.molcel.2017.09.009
Chen, F., Fan, Y., Hou, J., Liu, B., Zhang, B., Shang, Y., et al. (2021c). Integrated analysis identifies TfR1 as a prognostic biomarker which correlates with immune infiltration in breast cancer. Aging (Albany NY) 13 (17), 21671–21699. doi:10.18632/aging.203512
Chen, H., Liu, S., Ji, L., Wu, T., Ji, Y., Zhou, Y., et al. (2016). Folic acid supplementation mitigates alzheimer's disease by reducing inflammation: A randomized controlled trial. Mediat. Inflamm. 2016, 5912146. doi:10.1155/2016/5912146
Chen, P., Li, B., and Ou-Yang, L. (2022a). Role of estrogen receptors in health and disease. Front. Endocrinol. 13, 839005. doi:10.3389/fendo.2022.839005
Chen, S., Shen, L., Luo, S., Lan, X., and Wang, L. (2020b). Association between serum iron levels and the risk of cervical cancer in Chinese: A meta-analysis. J. Int. Med. Res. 48 (3), 300060519882804. doi:10.1177/0300060519882804
Chen, X., Kang, R., Kroemer, G., and Tang, D. (2021b). Broadening horizons: The role of ferroptosis in cancer. Nat. Rev. Clin. Oncol. 18 (5), 280–296. doi:10.1038/s41571-020-00462-0
Chen, X., Li, J., Kang, R., Klionsky, D. J., and Tang, D. (2021a). Ferroptosis: Machinery and regulation. Autophagy 17 (9), 2054–2081. doi:10.1080/15548627.2020.1810918
Chen, X., Yu, C., Kang, R., and Tang, D. (2020a). Iron metabolism in ferroptosis. Front. Cell Dev. Biol. 8, 590226. doi:10.3389/fcell.2020.590226
Chen, Y. C., Yu, J., Metcalfe, C., De Bruyn, T., Gelzleichter, T., Malhi, V., et al. (2022b). Latest generation estrogen receptor degraders for the treatment of hormone receptor-positive breast cancer. Expert Opin. Investig. Drugs 31 (6), 515–529. doi:10.1080/13543784.2021.1983542
Chung, S. H., Franceschi, S., and Lambert, P. F. (2010). Estrogen and ERalpha: Culprits in cervical cancer? Trends Endocrinol. metabolism TEM 21 (8), 504–511. doi:10.1016/j.tem.2010.03.005
Conneely, O. M., and Lydon, J. P. (2000). Progesterone receptors in reproduction: Functional impact of the A and B isoforms. Steroids 65 (10-11), 571–577. doi:10.1016/s0039-128x(00)00115-x
Cristofanilli, M., Rugo, H. S., Im, S. A., Slamon, D. J., Harbeck, N., Bondarenko, I., et al. (2022). Overall survival with palbociclib and fulvestrant in women with HR+/HER2- ABC: Updated exploratory analyses of PALOMA-3, a double-blind, phase III randomized study. Clin. cancer Res. official J. Am. Assoc. Cancer Res. 28 (16), 3433–3442. doi:10.1158/1078-0432.CCR-22-0305
Cronin, S. J. F., Seehus, C., Weidinger, A., Talbot, S., Reissig, S., Seifert, M., et al. (2018). The metabolite BH4 controls T cell proliferation in autoimmunity and cancer. Nature 563 (7732), 564–568. doi:10.1038/s41586-018-0701-2
Daniel, A. R., Hagan, C. R., and Lange, C. A. (2011). Progesterone receptor action: Defining a role in breast cancer. Expert Rev. Endocrinol. metabolism 6 (3), 359–369. doi:10.1586/eem.11.25
DeVore, N. M., and Scott, E. E. (2012). Structures of cytochrome P450 17A1 with prostate cancer drugs abiraterone and TOK-001. Nature 482 (7383), 116–119. doi:10.1038/nature10743
Dixon, S. J., Lemberg, K. M., Lamprecht, M. R., Skouta, R., Zaitsev, E. M., Gleason, C. E., et al. (2012). Ferroptosis: An iron-dependent form of nonapoptotic cell death. Cell 149 (5), 1060–1072. doi:10.1016/j.cell.2012.03.042
Dixon, S. J., Winter, G. E., Musavi, L. S., Lee, E. D., Snijder, B., Rebsamen, M., et al. (2015). Human haploid cell genetics reveals roles for lipid metabolism genes in nonapoptotic cell death. ACS Chem. Biol. 10 (7), 1604–1609. doi:10.1021/acschembio.5b00245
Doll, S., Proneth, B., Tyurina, Y. Y., Panzilius, E., Kobayashi, S., Ingold, I., et al. (2017). ACSL4 dictates ferroptosis sensitivity by shaping cellular lipid composition. Nat. Chem. Biol. 13 (1), 91–98. doi:10.1038/nchembio.2239
Du, J., Wang, T., Li, Y., Zhou, Y., Wang, X., Yu, X., et al. (2019). DHA inhibits proliferation and induces ferroptosis of leukemia cells through autophagy dependent degradation of ferritin. Free Radic. Biol. Med. 131, 356–369. doi:10.1016/j.freeradbiomed.2018.12.011
Duggan, C., Trapani, D., Ilbawi, A. M., Fidarova, E., Laversanne, M., Curigliano, G., et al. (2021). National health system characteristics, breast cancer stage at diagnosis, and breast cancer mortality: A population-based analysis. Lancet Oncol. 22 (11), 1632–1642. doi:10.1016/S1470-2045(21)00462-9
Eling, N., Reuter, L., Hazin, J., Hamacher-Brady, A., and Brady, N. R. (2015). Identification of artesunate as a specific activator of ferroptosis in pancreatic cancer cells. Oncoscience 2 (5), 517–532. doi:10.18632/oncoscience.160
Emons, G., and Gründker, C. (2021). Role of gonadotropin-releasing hormone (GnRH) in ovarian cancer. Cells 10 (2), 437. doi:10.3390/cells10020437
Emons, G., Mustea, A., and Tempfer, C. (2020). Tamoxifen and endometrial cancer: A janus-headed drug. Cancers (Basel) 12 (9), 2535. doi:10.3390/cancers12092535
Englert-Golon, M., Andrusiewicz, M., Żbikowska, A., Chmielewska, M., Sajdak, S., and Kotwicka, M. (2021). Altered expression of ESR1, ESR2, PELP1 and c-SRC genes is associated with ovarian cancer manifestation. Int. J. Mol. Sci. 22 (12), 6216. doi:10.3390/ijms22126216
Fan, R., Sun, Y., Wang, M., Wang, Q., Jiang, A., and Yang, T. (2022). New insights on ferroptosis and gynecological malignancies. Front. Mol. Biosci. 9, 921298. doi:10.3389/fmolb.2022.921298
Feng, H., Schorpp, K., Jin, J., Yozwiak, C. E., Hoffstrom, B. G., Decker, A. M., et al. (2020). Transferrin receptor is a specific ferroptosis marker. Cell Rep. 30 (10), 3411–3423.e7. doi:10.1016/j.celrep.2020.02.049
Fröhlich, T., Mai, C., Bogautdinov, R. P., Morozkina, S. N., Shavva, A. G., Friedrich, O., et al. (2020). Synthesis of tamoxifen-artemisinin and estrogen-artemisinin hybrids highly potent against breast and prostate cancer. ChemMedChem 15 (15), 1473–1479. doi:10.1002/cmdc.202000174
Fuentes, N., and Silveyra, P. (2019). Estrogen receptor signaling mechanisms. Adv. protein Chem. Struct. Biol. 116, 135–170. doi:10.1016/bs.apcsb.2019.01.001
Gao, M., Monian, P., Quadri, N., Ramasamy, R., and Jiang, X. (2015). Glutaminolysis and transferrin regulate ferroptosis. Mol. Cell 59 (2), 298–308. doi:10.1016/j.molcel.2015.06.011
Garwood, E. R., Kumar, A. S., Baehner, F. L., Moore, D. H., Au, A., Hylton, N., et al. (2010). Fluvastatin reduces proliferation and increases apoptosis in women with high grade breast cancer. Breast Cancer Res. Treat. 119 (1), 137–144. doi:10.1007/s10549-009-0507-x
Garzon, S., Uccella, S., Zorzato, P. C., Bosco, M., Franchi, M. P., Student, V., et al. (2021). Fertility-sparing management for endometrial cancer: Review of the literature. Minerva Med. 112 (1), 55–69. doi:10.23736/S0026-4806.20.07072-X
Gaschler, M. M., and Stockwell, B. R. (2017). Lipid peroxidation in cell death. Biochem. Biophys. Res. Commun. 482 (3), 419–425. doi:10.1016/j.bbrc.2016.10.086
Gatica, D., Lahiri, V., and Klionsky, D. J. (2018). Cargo recognition and degradation by selective autophagy. Nat. Cell Biol. 20 (3), 233–242. doi:10.1038/s41556-018-0037-z
Geng, N., Shi, B. J., Li, S. L., Zhong, Z. Y., Li, Y. C., Xua, W. L., et al. (2018). Knockdown of ferroportin accelerates erastin-induced ferroptosis in neuroblastoma cells. Eur. Rev. Med. Pharmacol. Sci. 22 (12), 3826–3836. doi:10.26355/eurrev_201806_15267
Gimeno-Martos, S., Miguel-Jimenez, S., Casao, A., Cebrián-Pérez, J. A., Muiño-Blanco, T., and Pérez-Pe, R. (2020). Underlying molecular mechanism in the modulation of the ram sperm acrosome reaction by progesterone and 17β-estradiol. Animal reproduction Sci. 221, 106567. doi:10.1016/j.anireprosci.2020.106567
Gompel, A. (2020). Progesterone and endometrial cancer. Best. Pract. Res. Clin. Obstet. Gynaecol. 69, 95–107. doi:10.1016/j.bpobgyn.2020.05.003
Greenshields, A. L., Shepherd, T. G., and Hoskin, D. W. (2017). Contribution of reactive oxygen species to ovarian cancer cell growth arrest and killing by the anti-malarial drug artesunate. Mol. Carcinog. 56 (1), 75–93. doi:10.1002/mc.22474
Habib, E., Linher-Melville, K., Lin, H. X., and Singh, G. (2015). Expression of xCT and activity of system xc(-) are regulated by NRF2 in human breast cancer cells in response to oxidative stress. Redox Biol. 5, 33–42. doi:10.1016/j.redox.2015.03.003
Hangauer, M. J., Viswanathan, V. S., Ryan, M. J., Bole, D., Eaton, J. K., Matov, A., et al. (2017). Drug-tolerant persister cancer cells are vulnerable to GPX4 inhibition. Nature 551 (7679), 247–250. doi:10.1038/nature24297
Hasegawa, M., Takahashi, H., Rajabi, H., Alam, M., Suzuki, Y., Yin, L., et al. (2016). Functional interactions of the cystine/glutamate antiporter, CD44v and MUC1-C oncoprotein in triple-negative breast cancer cells. Oncotarget 7 (11), 11756–11769. doi:10.18632/oncotarget.7598
Hayano, M., Yang, W. S., Corn, C. K., Pagano, N. C., and Stockwell, B. R. (2016). Loss of cysteinyl-tRNA synthetase (CARS) induces the transsulfuration pathway and inhibits ferroptosis induced by cystine deprivation. Cell Death Differ. 23 (2), 270–278. doi:10.1038/cdd.2015.93
Heinzelmann-Schwarz, V., Knipprath Meszaros, A., Stadlmann, S., Jacob, F., Schoetzau, A., Russell, K., et al. (2018). Letrozole may be a valuable maintenance treatment in high-grade serous ovarian cancer patients. Gynecol. Oncol. 148 (1), 79–85. doi:10.1016/j.ygyno.2017.10.036
Henderson, V. W. (2018). Progesterone and human cognition. Climacteric 21 (4), 333–340. doi:10.1080/13697137.2018.1476484
Hernandez, R., Põder, J., LaPorte, K. M., and Malek, T. R. (2022). Engineering IL-2 for immunotherapy of autoimmunity and cancer. Nat. Rev. Immunol. 22 (10), 614–628. doi:10.1038/s41577-022-00680-w
Heudel, P., Frenel, J. S., Dalban, C., Bazan, F., Joly, F., Arnaud, A., et al. (2022). Safety and efficacy of the mTOR inhibitor, vistusertib, combined with anastrozole in patients with hormone receptor-positive recurrent or metastatic endometrial cancer: The VICTORIA multicenter, open-label, phase 1/2 randomized clinical trial. JAMA Oncol. 8 (7), 1001–1009. doi:10.1001/jamaoncol.2022.1047
Hoffman, M. S., Roberts, W. S., Cavanagh, D., Praphat, H., Solomon, P., and Lyman, G. H. (1989). Treatment of recurrent and metastatic endometrial cancer with cisplatin, doxorubicin, cyclophosphamide, and megestrol acetate. Gynecol. Oncol. 35 (1), 75–77. doi:10.1016/0090-8258(89)90016-4
Hong, X., Roh, W., Sullivan, R. J., Wong, K. H. K., Wittner, B. S., Guo, H., et al. (2021). The lipogenic regulator SREBP2 induces transferrin in circulating melanoma cells and suppresses ferroptosis. Cancer Discov. 11 (3), 678–695. doi:10.1158/2159-8290.CD-19-1500
Hou, C., Hou, Q., Xie, X., Wang, H., Chen, Y., Lu, T., et al. (2021). Serum iron status and the risk of breast cancer in the European population: A two-sample mendelian randomisation study. Genes Nutr. 16 (1), 9. doi:10.1186/s12263-021-00691-7
Hou, Y., Zhang, S., Wang, L., Li, J., Qu, G., He, J., et al. (2012). Estrogen regulates iron homeostasis through governing hepatic hepcidin expression via an estrogen response element. Gene 511 (2), 398–403. doi:10.1016/j.gene.2012.09.060
Ingold, I., Berndt, C., Schmitt, S., Doll, S., Poschmann, G., Buday, K., et al. (2018). Selenium utilization by GPX4 is required to prevent hydroperoxide-induced ferroptosis. Cell 172 (3), 409–422.e21. doi:10.1016/j.cell.2017.11.048
Irwin, R. W., Yao, J., Hamilton, R. T., Cadenas, E., Brinton, R. D., and Nilsen, J. (2008). Progesterone and estrogen regulate oxidative metabolism in brain mitochondria. Endocrinology 149 (6), 3167–3175. doi:10.1210/en.2007-1227
Ishimoto, T., Nagano, O., Yae, T., Tamada, M., Motohara, T., Oshima, H., et al. (2011). CD44 variant regulates redox status in cancer cells by stabilizing the xCT subunit of system xc(-) and thereby promotes tumor growth. Cancer Cell 19 (3), 387–400. doi:10.1016/j.ccr.2011.01.038
Jayshree, R. S. (2021). The immune microenvironment in human papilloma virus-induced cervical lesions-evidence for estrogen as an immunomodulator. Front. Cell Infect. Microbiol. 11, 649815. doi:10.3389/fcimb.2021.649815
Jelić, M., Mandić, A., Kladar, N., Sudji, J., Božin, B., and Srdjenović, B. (2018). Lipid peroxidation, antioxidative defense and level of 8-hydroxy-2-deoxyguanosine in cervical cancer patients. J. Med. Biochem. 37 (3), 336–345. doi:10.1515/jomb-2017-0053
Jelic, M. D., Mandic, A. D., Maricic, S. M., and Srdjenovic, B. U. (2021). Oxidative stress and its role in cancer. J. Cancer Res. Ther. 17 (1), 22–28. doi:10.4103/jcrt.JCRT_862_16
Jiang, L., Kon, N., Li, T., Wang, S. J., Su, T., Hibshoosh, H., et al. (2015). Ferroptosis as a p53-mediated activity during tumour suppression. Nature 520 (7545), 57–62. doi:10.1038/nature14344
Jodhka, P. K., Kaur, P., Underwood, W., Lydon, J. P., and Singh, M. (2009). The differences in neuroprotective efficacy of progesterone and medroxyprogesterone acetate correlate with their effects on brain-derived neurotrophic factor expression. Endocrinology 150 (7), 3162–3168. doi:10.1210/en.2008-1247
Kagan, V. E., Mao, G., Qu, F., Angeli, J. P. F., Doll, S., Croix, C. S., et al. (2017). Oxidized arachidonic and arsenic PEs navigate cells to ferroptosis. Nat. Chem. Biol. 13 (1), 81–90. doi:10.1038/nchembio.2238
Kaur, J., and Debnath, J. (2015). Autophagy at the crossroads of catabolism and anabolism. Nat. Rev. Mol. Cell Biol. 16 (8), 461–472. doi:10.1038/nrm4024
Kim, D. H., Kim, M. J., Kim, N. Y., Lee, S., Byun, J. K., Yun, J. W., et al. (2022). DN200434, an orally available inverse agonist of estrogen-related receptor γ, induces ferroptosis in sorafenib-resistant hepatocellular carcinoma. BMB Rep. 55 (11), 547–552. doi:10.5483/BMBRep.2022.55.11.089
Kim, J. J., Kurita, T., and Bulun, S. E. (2013). Progesterone action in endometrial cancer, endometriosis, uterine fibroids, and breast cancer. Endocr. Rev. 34 (1), 130–162. doi:10.1210/er.2012-1043
Kim, O., Park, E. Y., Kwon, S. Y., Shin, S., Emerson, R. E., Shin, Y. H., et al. (2020). Targeting progesterone signaling prevents metastatic ovarian cancer. Proc. Natl. Acad. Sci. U. S. A. 117 (50), 31993–32004. doi:10.1073/pnas.2013595117
Kobayashi, H., Yamada, Y., Kawahara, N., Ogawa, K., and Yoshimoto, C. (2019). Integrating modern approaches to pathogenetic concepts of malignant transformation of endometriosis. Oncol. Rep. 41 (3), 1729–1738. doi:10.3892/or.2018.6946
Kraft, V. A. N., Bezjian, C. T., Pfeiffer, S., Ringelstetter, L., Müller, C., Zandkarimi, F., et al. (2020). GTP cyclohydrolase 1/tetrahydrobiopterin counteract ferroptosis through lipid remodeling. ACS Cent. Sci. 6 (1), 41–53. doi:10.1021/acscentsci.9b01063
Kuiper, G. G., and Gustafsson, J. A. (1997). The novel estrogen receptor-beta subtype: Potential role in the cell- and promoter-specific actions of estrogens and anti-estrogens. FEBS Lett. 410 (1), 87–90. doi:10.1016/s0014-5793(97)00413-4
Kumar, R. S., and Goyal, N. (2021). Estrogens as regulator of hematopoietic stem cell, immune cells and bone biology. Life Sci. 269, 119091. doi:10.1016/j.lfs.2021.119091
Kuzuyama, T., and Seto, H. (2012). Two distinct pathways for essential metabolic precursors for isoprenoid biosynthesis. Proc. Jpn. Acad. Ser. B Phys. Biol. Sci. 88 (3), 41–52. doi:10.2183/pjab.88.41
Kwon, S., Ahn, S. H., Jeong, W. J., Jung, Y. H., Bae, Y. J., Paik, J. H., et al. (2020). Estrogen receptor α as a predictive biomarker for survival in human papillomavirus-positive oropharyngeal squamous cell carcinoma. J. Transl. Med. 18 (1), 240. doi:10.1186/s12967-020-02396-8
Lai, C. R., Hsu, C. Y., Chen, Y. J., Yen, M. S., Chao, K. C., and Li, A. F. Y. (2013). Ovarian cancers arising from endometriosis: A microenvironmental biomarker study including ER, HNF1ß, p53, PTEN, BAF250a, and COX-2. J. Chin. Med. Assoc. JCMA 76 (11), 629–634. doi:10.1016/j.jcma.2013.07.008
Lang, X., Green, M. D., Wang, W., Yu, J., Choi, J. E., Jiang, L., et al. (2019). Radiotherapy and immunotherapy promote tumoral lipid oxidation and ferroptosis via synergistic repression of SLC7A11. Cancer Discov. 9 (12), 1673–1685. doi:10.1158/2159-8290.CD-19-0338
Larsen, M. S., Yde, C. W., Christensen, I. J., and Lykkesfeldt, A. E. (2012). Carboplatin treatment of antiestrogen-resistant breast cancer cells. Int. J. Oncol. 41 (5), 1863–1870. doi:10.3892/ijo.2012.1623
Latremoliere, A., and Costigan, M. (2011). GCH1, BH4 and pain. Curr. Pharm. Biotechnol. 12 (10), 1728–1741. doi:10.2174/138920111798357393
Legha, S. S. (1988). Tamoxifen in the treatment of breast cancer. Ann. Intern Med. 109 (3), 219–228. doi:10.7326/0003-4819-109-3-219
Lehtihet, M., Bonde, Y., Beckman, L., Berinder, K., Hoybye, C., Rudling, M., et al. (2016). Circulating hepcidin-25 is reduced by endogenous estrogen in humans. PloS one 11 (2), e0148802. doi:10.1371/journal.pone.0148802
Lei, G., Zhang, Y., Koppula, P., Liu, X., Zhang, J., Lin, S. H., et al. (2020). The role of ferroptosis in ionizing radiation-induced cell death and tumor suppression. Cell Res. 30 (2), 146–162. doi:10.1038/s41422-019-0263-3
Lewerenz, J., Hewett, S. J., Huang, Y., Lambros, M., Gout, P. W., Kalivas, P. W., et al. (2013). The cystine/glutamate antiporter system x(c)(-) in health and disease: From molecular mechanisms to novel therapeutic opportunities. Antioxid. Redox Signal 18 (5), 522–555. doi:10.1089/ars.2011.4391
Li, C., Zhang, L., Qiu, Z., Deng, W., and Wang, W. (2022). Key molecules of fatty acid metabolism in gastric cancer. Biomolecules 12 (5), 706. doi:10.3390/biom12050706
Li, J., Cao, F., Yin, H. L., Huang, Z. J., Lin, Z. T., Mao, N., et al. (2020). Ferroptosis: Past, present and future. Cell death Dis. 11 (2), 88. doi:10.1038/s41419-020-2298-2
Li, J., and Zhou, B. (2010). Biological actions of artemisinin: Insights from medicinal chemistry studies. Molecules 15 (3), 1378–1397. doi:10.3390/molecules15031378
Li, X., Rhee, D. K., Malhotra, R., Mayeur, C., Hurst, L. A., Ager, E., et al. (2016). Progesterone receptor membrane component-1 regulates hepcidin biosynthesis. J. Clin. investigation 126 (1), 389–401. doi:10.1172/JCI83831
Liang, L., Wang, H., Yao, J., Wei, Q., Lu, Y., Wang, T., et al. (2022). NPC1 deficiency contributes to autophagy-dependent ferritinophagy in HEI-OC1 auditory cells. Front. Mol. Biosci. 9, 952608. doi:10.3389/fmolb.2022.952608
Liao, P., Hemmerlin, A., Bach, T. J., and Chye, M. L. (2016). The potential of the mevalonate pathway for enhanced isoprenoid production. Biotechnol. Adv. 34 (5), 697–713. doi:10.1016/j.biotechadv.2016.03.005
Lin, R., Zhang, Z., Chen, L., Zhou, Y., Zou, P., Feng, C., et al. (2016). Dihydroartemisinin (DHA) induces ferroptosis and causes cell cycle arrest in head and neck carcinoma cells. Cancer Lett. 381 (1), 165–175. doi:10.1016/j.canlet.2016.07.033
Liu, J., Wang, Y., Meng, H., Yin, Y., Zhu, H., and Ni, T. (2021a). Identification of the prognostic signature associated with tumor immune microenvironment of uterine corpus endometrial carcinoma based on ferroptosis-related genes. Front. Cell Dev. Biol. 9, 735013. doi:10.3389/fcell.2021.735013
Liu, L., Zhang, C., Qu, S., Liu, R., Chen, H., Liang, Z., et al. (2022b). ESR1 inhibits ionizing radiation-induced ferroptosis in breast cancer cells via the NEDD4L/CD71 pathway. Arch. Biochem. Biophys. 725, 109299. doi:10.1016/j.abb.2022.109299
Liu, R., Liu, L., Bian, Y., Zhang, S., Wang, Y., Chen, H., et al. (2021b). The dual regulation effects of ESR1/nedd4l on SLC7A11 in breast cancer under ionizing radiation. Front. Cell Dev. Biol. 9, 772380. doi:10.3389/fcell.2021.772380
Liu, S., Zhang, Q., Liu, W., and Huang, X. (2022a). Prediction of prognosis in patients with endometrial carcinoma and immune microenvironment estimation based on ferroptosis-related genes. Front. Mol. Biosci. 9, 916689. doi:10.3389/fmolb.2022.916689
Liu, Y., and Gu, W. (2022). p53 in ferroptosis regulation: the new weapon for the old guardian. Cell Death Differ. 29 (5), 895–910. doi:10.1038/s41418-022-00943-y
Lousse, J. C., Defrere, S., Van Langendonckt, A., Gras, J., González-Ramos, R., Colette, S., et al. (2009). Iron storage is significantly increased in peritoneal macrophages of endometriosis patients and correlates with iron overload in peritoneal fluid. Fertil. Steril. 91 (5), 1668–1675. doi:10.1016/j.fertnstert.2008.02.103
Ma, S., Dielschneider, R. F., Henson, E. S., Xiao, W., Choquette, T. R., Blankstein, A. R., et al. (2017). Ferroptosis and autophagy induced cell death occur independently after siramesine and lapatinib treatment in breast cancer cells. PLoS One 12 (8), e0182921. doi:10.1371/journal.pone.0182921
Mancias, J. D., Pontano Vaites, L., Nissim, S., Biancur, D. E., Kim, A. J., Wang, X., et al. (2015). Ferritinophagy via NCOA4 is required for erythropoiesis and is regulated by iron dependent HERC2-mediated proteolysis. Elife 4, e10308. doi:10.7554/eLife.10308
Mancias, J. D., Wang, X., Gygi, S. P., Harper, J. W., and Kimmelman, A. C. (2014). Quantitative proteomics identifies NCOA4 as the cargo receptor mediating ferritinophagy. Nature 509 (7498), 105–109. doi:10.1038/nature13148
Markowska, A., Chudecka-Głaz, A., Pityński, K., Baranowski, W., Markowska, J., and Sawicki, W. (2022). Endometrial cancer management in young women. Cancers (Basel) 14 (8), 1922. doi:10.3390/cancers14081922
Matsuoka, S., Ballif, B. A., Smogorzewska, A., McDonald, E. R., Hurov, K. E., Luo, J., et al. (2007). ATM and ATR substrate analysis reveals extensive protein networks responsive to DNA damage. Science 316 (5828), 1160–1166. doi:10.1126/science.1140321
Metherall, J. E., Li, H., and Waugh, K. (1996). Role of multidrug resistance P-glycoproteins in cholesterol biosynthesis. J. Biol. Chem. 271 (5), 2634–2640. doi:10.1074/jbc.271.5.2634
Miller, W. L. (2002). Androgen biosynthesis from cholesterol to DHEA. Mol. Cell Endocrinol. 198 (1-2), 7–14. doi:10.1016/s0303-7207(02)00363-5
Missaglia, S., Coleman, R. A., Mordente, A., and Tavian, D. (2019). Neutral lipid storage diseases as cellular model to study lipid droplet function. Cells 8 (2), 187. doi:10.3390/cells8020187
Montalbetti, N., Simonin, A., Kovacs, G., and Hediger, M. A. (2013). Mammalian iron transporters: Families SLC11 and SLC40. Mol. Asp. Med. 34 (2-3), 270–287. doi:10.1016/j.mam.2013.01.002
Moore, H. C., Unger, J. M., Phillips, K. A., Boyle, F., Hitre, E., Porter, D., et al. (2015). Goserelin for ovarian protection during breast-cancer adjuvant chemotherapy. N. Engl. J. Med. 372 (10), 923–932. doi:10.1056/NEJMoa1413204
Muhoberac, B. B., and Vidal, R. (2019). Iron, ferritin, hereditary ferritinopathy, and neurodegeneration. Front. Neurosci. 13, 1195. doi:10.3389/fnins.2019.01195
Mukherjee, A. G., Wanjari, U. R., Nagarajan, D., K K, V., V, A., P, J. P., et al. (2022). Letrozole: Pharmacology, toxicity and potential therapeutic effects. Life Sci. 310, 121074. doi:10.1016/j.lfs.2022.121074
Mungenast, F., and Thalhammer, T. (2014). Estrogen biosynthesis and action in ovarian cancer. Front. Endocrinol. 5, 192. doi:10.3389/fendo.2014.00192
Murakami, H., Hayashi, M., Terada, S., and Ohmichi, M. (2023). Medroxyprogesterone acetate-resistant endometrial cancer cells are susceptible to ferroptosis inducers. Life Sci. 325, 121753. doi:10.1016/j.lfs.2023.121753
Nabholtz, J. M. (2006). Role of anastrozole across the breast cancer continuum: From advanced to early disease and prevention. Oncology 70 (1), 1–12. doi:10.1159/000091180
Ni, W., Mo, H., Liu, Y., Xu, Y., Qin, C., Zhou, Y., et al. (2021). Targeting cholesterol biosynthesis promotes anti-tumor immunity by inhibiting long noncoding RNA SNHG29-mediated YAP activation. Mol. Ther. 29 (10), 2995–3010. doi:10.1016/j.ymthe.2021.05.012
Ogawa, M., Hashimoto, K., Kitano, S., Yamashita, S., Toda, A., Nakamura, K., et al. (2023). Estrogen induces genomic instability in high-risk HPV-infected cervix and promotes the carcinogenesis of cervical adenocarcinoma. Biochem. Biophys. Res. Commun. 659, 80–90. doi:10.1016/j.bbrc.2023.04.009
Okazaki, F., Matsunaga, N., Hamamura, K., Suzuki, K., Nakao, T., Okazaki, H., et al. (2017). Administering xCT inhibitors based on circadian clock improves antitumor effects. Cancer Res. 77 (23), 6603–6613. doi:10.1158/0008-5472.CAN-17-0720
Onal, G., Kutlu, O., Gozuacik, D., and Dokmeci Emre, S. (2017). Lipid droplets in health and disease. Lipids health Dis. 16 (1), 128. doi:10.1186/s12944-017-0521-7
Ooko, E., Saeed, M. E., Kadioglu, O., Sarvi, S., Colak, M., Elmasaoudi, K., et al. (2015). Artemisinin derivatives induce iron-dependent cell death (ferroptosis) in tumor cells. Phytomedicine 22 (11), 1045–1054. doi:10.1016/j.phymed.2015.08.002
Park, E., and Chung, S. W. (2019). ROS-mediated autophagy increases intracellular iron levels and ferroptosis by ferritin and transferrin receptor regulation. Cell death Dis. 10 (11), 822. doi:10.1038/s41419-019-2064-5
Park, S., Lim, W., Jeong, W., Bazer, F. W., Lee, D., and Song, G. (2018). Sideroxylon (Callistemon lanceolatus) suppressed cell proliferation and increased apoptosis in ovarian cancer cells accompanied by mitochondrial dysfunction, the generation of reactive oxygen species, and an increase of lipid peroxidation. J. Cell Physiol. 233 (11), 8597–8604. doi:10.1002/jcp.26540
Park, Y., Baik, S., Ho, C., Lin, C. Y., and Chung, S. H. (2021). Progesterone receptor is a haploinsufficient tumor-suppressor gene in cervical cancer. Mol. Cancer Res. 19 (1), 42–47. doi:10.1158/1541-7786.MCR-20-0704
Pelletier, G., and El-Alfy, M. (2000). Immunocytochemical localization of estrogen receptors alpha and beta in the human reproductive organs. J. Clin. Endocrinol. Metab. 85 (12), 4835–4840. doi:10.1210/jcem.85.12.7029
Petrunak, E. M., DeVore, N. M., Porubsky, P. R., and Scott, E. E. (2014). Structures of human steroidogenic cytochrome P450 17A1 with substrates. J. Biol. Chem. 289 (47), 32952–32964. doi:10.1074/jbc.M114.610998
Pfeffer, S. R. (2019). NPC intracellular cholesterol transporter 1 (NPC1)-mediated cholesterol export from lysosomes. J. Biol. Chem. 294 (5), 1706–1709. doi:10.1074/jbc.TM118.004165
Pinnix, Z. K., Miller, L. D., Wang, W., D'Agostino, R., Kute, T., Willingham, M. C., et al. (2010). Ferroportin and iron regulation in breast cancer progression and prognosis. Sci. Transl. Med. 2 (43), 43ra56. doi:10.1126/scitranslmed.3001127
Plummer, M., Schiffman, M., Castle, P. E., Maucort-Boulch, D., and Wheeler, C. M. (2007). A 2-year prospective study of human papillomavirus persistence among women with a cytological diagnosis of atypical squamous cells of undetermined significance or low-grade squamous intraepithelial lesion. J. Infect. Dis. 195 (11), 1582–1589. doi:10.1086/516784
Riera Leal, A., Ortiz-Lazareno, P. C., Jave-Suarez, L. F., Ramírez De Arellano, A., Aguilar-Lemarroy, A., Ortiz-García, Y. M., et al. (2020). 17β-estradiol-induced mitochondrial dysfunction and Warburg effect in cervical cancer cells allow cell survival under metabolic stress. Int. J. Oncol. 56 (1), 33–46. doi:10.3892/ijo.2019.4912
Russell, J. K., Jones, C. K., and Newhouse, P. A. (2019). The role of estrogen in brain and cognitive aging. Neurother. J. Am. Soc. Exp. Neurother. 16 (3), 649–665. doi:10.1007/s13311-019-00766-9
Sato, H., Tamba, M., Kuriyama-Matsumura, K., Okuno, S., and Bannai, S. (2000). Molecular cloning and expression of human xCT, the light chain of amino acid transport system xc. Antioxid. Redox Signal 2 (4), 665–671. doi:10.1089/ars.2000.2.4-665
Schott, M. B., Weller, S. G., Schulze, R. J., Krueger, E. W., Drizyte-Miller, K., Casey, C. A., et al. (2019). Lipid droplet size directs lipolysis and lipophagy catabolism in hepatocytes. J. Cell Biol. 218 (10), 3320–3335. doi:10.1083/jcb.201803153
Sedlacek, S. M. (1988). An overview of megestrol acetate for the treatment of advanced breast cancer. Semin. Oncol. 15 (2), 3–13.
Seibt, T. M., Proneth, B., and Conrad, M. (2019). Role of GPX4 in ferroptosis and its pharmacological implication. Free Radic. Biol. Med. 133, 144–152. doi:10.1016/j.freeradbiomed.2018.09.014
Shield, K., Ackland, M. L., Ahmed, N., and Rice, G. E. (2009). Multicellular spheroids in ovarian cancer metastases: Biology and pathology. Gynecol. Oncol. 113 (1), 143–148. doi:10.1016/j.ygyno.2008.11.032
Shih, C. C., Chou, H. C., Chen, Y. J., Kuo, W. H., Chan, C. H., Lin, Y. C., et al. (2019). Role of PGRMC1 in cell physiology of cervical cancer. Life Sci. 231, 116541. doi:10.1016/j.lfs.2019.06.016
Shimada, K., Skouta, R., Kaplan, A., Yang, W. S., Hayano, M., Dixon, S. J., et al. (2016). Global survey of cell death mechanisms reveals metabolic regulation of ferroptosis. Nat. Chem. Biol. 12 (7), 497–503. doi:10.1038/nchembio.2079
Siegel, R. L., Miller, K. D., and Jemal, A. (2019). Cancer statistics. CA Cancer J. Clin. 69(1), 7–34. doi:10.3322/caac.21551
Singh, B., Ronghe, A. M., Chatterjee, A., Bhat, N. K., and Bhat, H. K. (2013). MicroRNA-93 regulates NRF2 expression and is associated with breast carcinogenesis. Carcinogenesis 34 (5), 1165–1172. doi:10.1093/carcin/bgt026
Slomovitz, B. M., Filiaci, V. L., Walker, J. L., Taub, M. C., Finkelstein, K. A., Moroney, J. W., et al. (2022). A randomized phase II trial of everolimus and letrozole or hormonal therapy in women with advanced, persistent or recurrent endometrial carcinoma: A gog foundation study. Gynecol. Oncol. 164 (3), 481–491. doi:10.1016/j.ygyno.2021.12.031
Slomovitz, B. M., Jiang, Y., Yates, M. S., Soliman, P. T., Johnston, T., Nowakowski, M., et al. (2015). Phase II study of everolimus and letrozole in patients with recurrent endometrial carcinoma. J. Clin. Oncol. 33 (8), 930–936. doi:10.1200/JCO.2014.58.3401
Song, X., Zhu, S., Chen, P., Hou, W., Wen, Q., Liu, J., et al. (2018). AMPK-mediated BECN1 phosphorylation promotes ferroptosis by directly blocking system X(c)(-) activity. Curr. Biol. 28 (15), 2388–2399.e5. doi:10.1016/j.cub.2018.05.094
Starek-Świechowicz, B., Budziszewska, B., and Starek, A. (2021). Endogenous estrogens-breast cancer and chemoprevention. Pharmacol. Rep. 73 (6), 1497–1512. doi:10.1007/s43440-021-00317-0
Strushkevich, N., MacKenzie, F., Cherkesova, T., Grabovec, I., Usanov, S., and Park, H. W. (2011). Structural basis for pregnenolone biosynthesis by the mitochondrial monooxygenase system. Proc. Natl. Acad. Sci. U. S. A. 108 (25), 10139–10143. doi:10.1073/pnas.1019441108
Sui, S., Xu, S., and Pang, D. (2022). Emerging role of ferroptosis in breast cancer: New dawn for overcoming tumor progression. Pharmacol. Ther. 232, 107992. doi:10.1016/j.pharmthera.2021.107992
Sun, X., Wang, S., Gai, J., Guan, J., Li, J., Li, Y., et al. (2019). SIRT5 promotes cisplatin resistance in ovarian cancer by suppressing DNA damage in a ROS-dependent manner via regulation of the Nrf2/HO-1 pathway. Front. Oncol. 9, 754. doi:10.3389/fonc.2019.00754
Tan, J. S., Seow, C. J., Goh, V. J., and Silver, D. L. (2014). Recent advances in understanding proteins involved in lipid droplet formation, growth and fusion. J. Genet. genomics = Yi chuan xue bao 41 (5), 251–259. doi:10.1016/j.jgg.2014.03.003
Tang, D., and Kroemer, G. (2020). Ferroptosis. Curr. Biol. Cb. 30 (21), R1292–R1297. doi:10.1016/j.cub.2020.09.068
Taylor, W. R., Fedorka, S. R., Gad, I., Shah, R., Alqahtani, H. D., Koranne, R., et al. (2019). Small-molecule ferroptotic agents with potential to selectively target cancer stem cells. Sci. Rep. 9 (1), 5926. doi:10.1038/s41598-019-42251-5
Tesfay, L., Paul, B. T., Konstorum, A., Deng, Z., Cox, A. O., Lee, J., et al. (2019). Stearoyl-CoA desaturase 1 protects ovarian cancer cells from ferroptotic cell death. Cancer Res. 79 (20), 5355–5366. doi:10.1158/0008-5472.CAN-19-0369
Torre, L. A., Trabert, B., DeSantis, C. E., Miller, K. D., Samimi, G., Runowicz, C. D., et al. (2018). Ovarian cancer statistics. CA Cancer J. Clin. 68(4):284–296. doi:10.3322/caac.21456
Trabert, B., Sherman, M. E., Kannan, N., and Stanczyk, F. Z. (2020). Progesterone and breast cancer. Endocr. Rev. 41 (2), 320–344. doi:10.1210/endrev/bnz001
Tsai, S. Y., Carlstedt-Duke, J., Weigel, N. L., Dahlman, K., Gustafsson, J. A., Tsai, M. J., et al. (1988). Molecular interactions of steroid hormone receptor with its enhancer element: Evidence for receptor dimer formation. Cell 55 (2), 361–369. doi:10.1016/0092-8674(88)90059-1
Tseng, L., Tang, M., Wang, Z., and Mazella, J. (2003). Progesterone receptor (hPR) upregulates the fibronectin promoter activity in human decidual fibroblasts. DNA Cell Biol. 22 (10), 633–640. doi:10.1089/104454903770238102
Van Langendonckt, A., Casanas-Roux, F., and Donnez, J. (2002). Iron overload in the peritoneal cavity of women with pelvic endometriosis. Fertil. Steril. 78 (4), 712–718. doi:10.1016/s0015-0282(02)03346-0
Van Langendonckt, A., Casanas-Roux, F., Eggermont, J., and Donnez, J. (2004). Characterization of iron deposition in endometriotic lesions induced in the nude mouse model. Hum. Reprod. 19 (6), 1265–1271. doi:10.1093/humrep/deh182
Vasan, N., Toska, E., and Scaltriti, M. (2019). Overview of the relevance of PI3K pathway in HR-positive breast cancer. Ann. Oncol. 30 (10), x3–x11. doi:10.1093/annonc/mdz281
Vina, J., and Borras, C. (2010). Women live longer than men: Understanding molecular mechanisms offers opportunities to intervene by using estrogenic compounds. Antioxidants redox Signal. 13 (3), 269–278. doi:10.1089/ars.2009.2952
Viswanathan, V. S., Ryan, M. J., Dhruv, H. D., Gill, S., Eichhoff, O. M., Seashore-Ludlow, B., et al. (2017). Dependency of a therapy-resistant state of cancer cells on a lipid peroxidase pathway. Nature 547 (7664), 453–457. doi:10.1038/nature23007
von Hagens, C., Walter-Sack, I., Goeckenjan, M., Osburg, J., Storch-Hagenlocher, B., Sertel, S., et al. (2017). Prospective open uncontrolled phase I study to define a well-tolerated dose of oral artesunate as add-on therapy in patients with metastatic breast cancer (ARTIC M33/2). Breast Cancer Res. Treat. 164 (2), 359–369. doi:10.1007/s10549-017-4261-1
Wan, Y., Song, Y., Chen, J., Kong, J., Gu, C., Huang, J., et al. (2022). Upregulated fibulin-1 increased endometrial stromal cell viability and migration by repressing EFEMP1-dependent ferroptosis in endometriosis. Biomed. Res. Int. 2022, 4809415. doi:10.1155/2022/4809415
Wang, C., Zeng, J., Li, L. J., Xue, M., and He, S. L. (2021a). Cdc25A inhibits autophagy-mediated ferroptosis by upregulating ErbB2 through PKM2 dephosphorylation in cervical cancer cells. Cell death Dis. 12 (11), 1055. doi:10.1038/s41419-021-04342-y
Wang, K., Jiang, J., Lei, Y., Zhou, S., Wei, Y., and Huang, C. (2019a). Targeting metabolic-redox circuits for cancer therapy. Trends Biochem. Sci. 44 (5), 401–414. doi:10.1016/j.tibs.2019.01.001
Wang, T., Gong, M., Cao, Y., Zhao, C., Lu, Y., Zhou, Y., et al. (2022a). Persistent ferroptosis promotes cervical squamous intraepithelial lesion development and oncogenesis by regulating KRAS expression in patients with high risk-HPV infection. Cell death Discov. 8 (1), 201. doi:10.1038/s41420-022-01013-5
Wang, T., Wei, Q., Liang, L., Tang, X., Yao, J., Lu, Y., et al. (2020b). OSBPL2 is required for the binding of COPB1 to ATGL and the regulation of lipid droplet lipolysis. iScience 23 (7), 101252. doi:10.1016/j.isci.2020.101252
Wang, W., Green, M., Choi, J. E., Gijón, M., Kennedy, P. D., Johnson, J. K., et al. (2019b). CD8(+) T cells regulate tumour ferroptosis during cancer immunotherapy. Nature 569 (7755), 270–274. doi:10.1038/s41586-019-1170-y
Wang, W., Zhang, J., Wang, Y., Xu, Y., and Zhang, S. (2022b). Identifies microtubule-binding protein CSPP1 as a novel cancer biomarker associated with ferroptosis and tumor microenvironment. Comput. Struct. Biotechnol. J. 20, 3322–3335. doi:10.1016/j.csbj.2022.06.046
Wang, X., Xu, S., Zhang, L., Cheng, X., Yu, H., Bao, J., et al. (2021b). Vitamin C induces ferroptosis in anaplastic thyroid cancer cells by ferritinophagy activation. Biochem. Biophys. Res. Commun. 551, 46–53. doi:10.1016/j.bbrc.2021.02.126
Wang, Y., Jing, F., and Wang, H. (2022c). Role of Exemestane in the treatment of estrogen-receptor-positive breast cancer: A narrative review of recent evidence. Adv. Ther. 39 (2), 862–891. doi:10.1007/s12325-021-01924-2
Wang, Y., Liu, Y., Liu, J., Kang, R., and Tang, D. (2020a). NEDD4L-mediated LTF protein degradation limits ferroptosis. Biochem. biophysical Res. Commun. 531 (4), 581–587. doi:10.1016/j.bbrc.2020.07.032
Warner, G. J., Berry, M. J., Moustafa, M. E., Carlson, B. A., Hatfield, D. L., and Faust, J. R. (2000). Inhibition of selenoprotein synthesis by selenocysteine tRNA[Ser]Sec lacking isopentenyladenosine. J. Biol. Chem. 275 (36), 28110–28119. doi:10.1074/jbc.M001280200
Wei, S., Yu, Z., Shi, R., An, L., Zhang, Q., Zhang, Q., et al. (2022). GPX4 suppresses ferroptosis to promote malignant progression of endometrial carcinoma via transcriptional activation by ELK1. BMC Cancer 22 (1), 881. doi:10.1186/s12885-022-09986-3
Wei, X., Yi, X., Zhu, X. H., and Jiang, D. S. (2020). Posttranslational modifications in ferroptosis. Oxid. Med. Cell Longev. 2020, 8832043. doi:10.1155/2020/8832043
Weijiao, Y., Fuchun, L., Mengjie, C., Xiaoqing, Q., Hao, L., Yuan, L., et al. (2021). Immune infiltration and a ferroptosis-associated gene signature for predicting the prognosis of patients with endometrial cancer. Aging 13 (12), 16713–16732. doi:10.18632/aging.203190
Wu, N., Zhang, X., Wang, Z., Fang, C., and Li, H. (2023). Progesterone prevents HGSOC by promoting precancerous cell pyroptosis via inducing fibroblast paracrine. iScience 26 (4), 106523. doi:10.1016/j.isci.2023.106523
Wu, N. Y., Huang, H. S., Chao, T. H., Chou, H. M., Fang, C., Qin, C. Z., et al. (2017). Progesterone prevents high-grade serous ovarian cancer by inducing necroptosis of p53-defective fallopian tube epithelial cells. Cell Rep. 18 (11), 2557–2565. doi:10.1016/j.celrep.2017.02.049
Wu, R., Hendrix-Lucas, N., Kuick, R., Zhai, Y., Schwartz, D. R., Akyol, A., et al. (2007). Mouse model of human ovarian endometrioid adenocarcinoma based on somatic defects in the Wnt/beta-catenin and PI3K/Pten signaling pathways. Cancer Cell 11 (4), 321–333. doi:10.1016/j.ccr.2007.02.016
Xu, X. L., Huang, Z. Y., Yu, K., Li, J., Fu, X. W., and Deng, S. L. (2022). Estrogen biosynthesis and signal transduction in ovarian disease. Front. Endocrinol. (Lausanne). 13, 827032. doi:10.3389/fendo.2022.827032
Yamaguchi, K., Mandai, M., Toyokuni, S., Hamanishi, J., Higuchi, T., Takakura, K., et al. (2008). Contents of endometriotic cysts, especially the high concentration of free iron, are a possible cause of carcinogenesis in the cysts through the iron-induced persistent oxidative stress. Clin. cancer Res. 14 (1), 32–40. doi:10.1158/1078-0432.CCR-07-1614
Yang, G., Nowsheen, S., Aziz, K., and Georgakilas, A. G. (2013). Toxicity and adverse effects of Tamoxifen and other anti-estrogen drugs. Pharmacol. Ther. 139 (3), 392–404. doi:10.1016/j.pharmthera.2013.05.005
Yang, L., Wang, H., Yang, X., Wu, Q., An, P., Jin, X., et al. (2020). Auranofin mitigates systemic iron overload and induces ferroptosis via distinct mechanisms. Signal Transduct. Target Ther. 5 (1), 138. doi:10.1038/s41392-020-00253-0
Yang, W. S., SriRamaratnam, R., Welsch, M. E., Shimada, K., Skouta, R., Viswanathan, V. S., et al. (2014). Regulation of ferroptotic cancer cell death by GPX4. Cell 156 (1-2), 317–331. doi:10.1016/j.cell.2013.12.010
Yang, W. S., and Stockwell, B. R. (2008). Synthetic lethal screening identifies compounds activating iron-dependent, nonapoptotic cell death in oncogenic-RAS-harboring cancer cells. Chem. Biol. 15 (3), 234–245. doi:10.1016/j.chembiol.2008.02.010
Yao, P., and Fox, P. L. (2013). Aminoacyl-tRNA synthetases in medicine and disease. EMBO Mol. Med. 5 (3), 332–343. doi:10.1002/emmm.201100626
Ye, L. F., Chaudhary, K. R., Zandkarimi, F., Harken, A. D., Kinslow, C. J., Upadhyayula, P. S., et al. (2020). Radiation-induced lipid peroxidation triggers ferroptosis and synergizes with ferroptosis inducers. ACS Chem. Biol. 15 (2), 469–484. doi:10.1021/acschembio.9b00939
Ye, R. R., Chen, B. C., Lu, J. J., Ma, X. R., and Li, R. T. (2021). Phosphorescent rhenium(I) complexes conjugated with artesunate: Mitochondrial targeting and apoptosis-ferroptosis dual induction. J. Inorg. Biochem. 223, 111537. doi:10.1016/j.jinorgbio.2021.111537
Yoshida, G. J. (2018). Emerging roles of Myc in stem cell biology and novel tumor therapies. J. Exp. Clin. Cancer Res. 37 (1), 173. doi:10.1186/s13046-018-0835-y
Yoshida, G. J. (2015). Metabolic reprogramming: The emerging concept and associated therapeutic strategies. J. Exp. Clin. Cancer Res. 34, 111. doi:10.1186/s13046-015-0221-y
Yoshimoto, F. K., Gonzalez, E., Auchus, R. J., and Guengerich, F. P. (2016). Mechanism of 17α,20-lyase and new hydroxylation reactions of human cytochrome P450 17A1: 18O labeling and oxygen surrogate evidence for a role of a perferryl oxygen. J. Biol. Chem. 291 (33), 17143–17164. doi:10.1074/jbc.M116.732966
You, J. H., Lee, J., and Roh, J. L. (2021a). PGRMC1-dependent lipophagy promotes ferroptosis in paclitaxel-tolerant persister cancer cells. J. Exp. Clin. Cancer Res. 40 (1), 350. doi:10.1186/s13046-021-02168-2
You, Y., Fan, Q., Huang, J., Wu, Y., Lin, H., and Zhang, Q. (2021b). Ferroptosis-related gene signature promotes ovarian cancer by influencing immune infiltration and invasion. J. Oncol. 2021, 9915312. doi:10.1155/2021/9915312
Younes, M., and Honma, N. (2011). Estrogen receptor β. Arch. Pathol. Lab. Med. 135 (1), 63–66. doi:10.5858/2010-0448-RAR.1
Yu, H., Guo, P., Xie, X., Wang, Y., and Chen, G. (2017). Ferroptosis, a new form of cell death, and its relationships with tumourous diseases. J. Cell Mol. Med. 21 (4), 648–657. doi:10.1111/jcmm.13008
Yu, H., Yang, C., Jian, L., Guo, S., Chen, R., Li, K., et al. (2019). Sulfasalazine-induced ferroptosis in breast cancer cells is reduced by the inhibitory effect of estrogen receptor on the transferrin receptor. Oncol. Rep. 42 (2), 826–838. doi:10.3892/or.2019.7189
Yu, K., Huang, Z. Y., Xu, X. L., Li, J., Fu, X. W., and Deng, S. L. (2022). Estrogen receptor function: Impact on the human endometrium. Front. Endocrinol. (Lausanne). 13, 827724. doi:10.3389/fendo.2022.827724
Yuan, H., Li, X., Zhang, X., Kang, R., and Tang, D. (2016). Identification of ACSL4 as a biomarker and contributor of ferroptosis. Biochem. Biophys. Res. Commun. 478 (3), 1338–1343. doi:10.1016/j.bbrc.2016.08.124
Zechner, R., Zimmermann, R., Eichmann, T. O., Kohlwein, S. D., Haemmerle, G., Lass, A., et al. (2012). FAT SIGNALS-lipases and lipolysis in lipid metabolism and signaling. Cell metab. 15 (3), 279–291. doi:10.1016/j.cmet.2011.12.018
Zhang, X., Sui, S., Wang, L., Li, H., Zhang, L., Xu, S., et al. (2020). Inhibition of tumor propellant glutathione peroxidase 4 induces ferroptosis in cancer cells and enhances anticancer effect of cisplatin. J. Cell Physiol. 235 (4), 3425–3437. doi:10.1002/jcp.29232
Zhang, Y., Shi, J., Liu, X., Feng, L., Gong, Z., Koppula, P., et al. (2018). BAP1 links metabolic regulation of ferroptosis to tumour suppression. Nat. Cell Biol. 20 (10), 1181–1192. doi:10.1038/s41556-018-0178-0
Zhang, Y., Swanda, R. V., Nie, L., Liu, X., Wang, C., Lee, H., et al. (2021a). mTORC1 couples cyst(e)ine availability with GPX4 protein synthesis and ferroptosis regulation. Nat. Commun. 12 (1), 1589. doi:10.1038/s41467-021-21841-w
Zhang, Y., Xia, M., Zhou, Z., Hu, X., Wang, J., Zhang, M., et al. (2021b). p53 promoted ferroptosis in ovarian cancer cells treated with human serum incubated-superparamagnetic iron oxides. Int. J. nanomedicine 16, 283–296. doi:10.2147/IJN.S282489
Zhang, Y. Y., Ni, Z. J., Elam, E., Zhang, F., Thakur, K., Wang, S., et al. (2021c). Juglone, a novel activator of ferroptosis, induces cell death in endometrial carcinoma Ishikawa cells. Food & Funct. 12 (11), 4947–4959. doi:10.1039/d1fo00790d
Zhang, Z., Huang, H., Feng, F., Wang, J., and Cheng, N. (2019). A pilot study of gonadotropin-releasing hormone agonist combined with aromatase inhibitor as fertility-sparing treatment in obese patients with endometrial cancer. J. Gynecol. Oncol. 30 (4), e61. doi:10.3802/jgo.2019.30.e61
Zhao, G., Cardenas, H., and Matei, D. (2019). Ovarian cancer-why lipids matter. Cancers (Basel) 11 (12), 1870. doi:10.3390/cancers11121870
Zhao, H., Xu, Y., and Shang, H. (2022a). Ferroptosis: A new promising target for ovarian cancer therapy. Int. J. Med. Sci. 19 (13), 1847–1855. doi:10.7150/ijms.76480
Zhao, X., Niu, J., Shi, C., and Liu, Z. (2022b). Levonorgestrel-releasing intrauterine device plus metformin, or megestrol acetate plus metformin for fertility-sparing treatment of atypical endometrial hyperplasia and early endometrial carcinoma: A prospective, randomized, blind-endpoint design trial protocol. Reprod. Health 19 (1), 206. doi:10.1186/s12978-022-01513-8
Zhao, Y., Ruan, X., Cheng, J., Xu, X., Gu, M., and Mueck, A. O. (2023). PGRMC1 promotes triple-negative breast cancer cell growth via suppressing ferroptosis. Climacteric 26 (2), 135–142. doi:10.1080/13697137.2023.2170225
Keywords: ferroptosis, lipid peroxidation, gynecologic cancers, estrogen, progesterone
Citation: Lai W, Chen J, Wang T and Liu Q (2023) Crosstalk between ferroptosis and steroid hormone signaling in gynecologic cancers. Front. Mol. Biosci. 10:1223493. doi: 10.3389/fmolb.2023.1223493
Received: 16 May 2023; Accepted: 22 June 2023;
Published: 04 July 2023.
Edited by:
Yanqing Liu, Columbia University, United StatesReviewed by:
Xudong Wang, University of Pennsylvania, United StatesCopyright © 2023 Lai, Chen, Wang and Liu. This is an open-access article distributed under the terms of the Creative Commons Attribution License (CC BY). The use, distribution or reproduction in other forums is permitted, provided the original author(s) and the copyright owner(s) are credited and that the original publication in this journal is cited, in accordance with accepted academic practice. No use, distribution or reproduction is permitted which does not comply with these terms.
*Correspondence: Qiaoling Liu, MTEzNDQ0NzgwNUBxcS5jb20=; Tianming Wang, d2FuZ3RpYW5taW5nQG5qbXUuZWR1LmNu
Disclaimer: All claims expressed in this article are solely those of the authors and do not necessarily represent those of their affiliated organizations, or those of the publisher, the editors and the reviewers. Any product that may be evaluated in this article or claim that may be made by its manufacturer is not guaranteed or endorsed by the publisher.
Research integrity at Frontiers
Learn more about the work of our research integrity team to safeguard the quality of each article we publish.