- 1GSK Vaccines Institute for Global Health, Siena, Italy
- 2Department of Biomolecular Sciences, University of Urbino Carlo Bo, Urbino, Italy
- 3Enteric and Diarrheal Diseases, Global Health, Bill and Melinda Gates Foundation, Seattle, WA, United States
- 4The Jenner Institute, Nuffield Department of Medicine, University of Oxford, Oxford, United Kingdom
- 5The Institute of Immunology and Immunotherapy, University of Birmingham, Birmingham, United Kingdom
Vaccines are cost-effective tools for reducing morbidity and mortality caused by infectious diseases. The rapid evolution of pneumococcal conjugate vaccines, the introduction of tetravalent meningococcal conjugate vaccines, mass vaccination campaigns in Africa with a meningococcal A conjugate vaccine, and the recent licensure and introduction of glycoconjugates against S. Typhi underlie the continued importance of research on glycoconjugate vaccines. More innovative ways to produce carbohydrate-based vaccines have been developed over the years, including bioconjugation, Outer Membrane Vesicles (OMV) and the Multiple antigen-presenting system (MAPS). Several variables in the design of these vaccines can affect the induced immune responses. We review immunogenicity studies comparing conjugate vaccines that differ in design variables, such as saccharide chain length and conjugation chemistry, as well as carrier protein and saccharide to protein ratio. We evaluate how a better understanding of the effects of these different parameters is key to designing improved glycoconjugate vaccines.
1 Introduction
Glycoconjugate vaccines began when Avery and Goebel found that the immunogenicity of polysaccharides (PS) and oligosaccharides (OS) could be enhanced by coupling to carrier proteins and that antibody concentrations increased after reinjection of such conjugates (Avery and Goebel, 1929; Goebel and Avery, 1931; Goebel, 1939). The introduction of glycoconjugate vaccines has greatly reduced the incidence of disease caused by Haemophilus influenzae type b (Hib), Neisseria meningitidis and Streptococcus pneumoniae (Ada and Isaacs, 2003; Trotter et al., 2008). The research on glycoconjugates has never stopped as shown by the evolution of pneumococcal conjugate vaccines (Micoli et al., 2023), the introduction of tetravalent meningococcal conjugate vaccines (Cooper et al., 2011), vaccination campaigns in Africa with the MenAfriVac meningococcal serogroup A vaccine (Frasch et al., 2012) and most recently the licensure of Vi glycoconjugate vaccines against S. Typhi (Hancuh et al., 2023).
Many bacteria are surrounded by capsular PS or O-specific polysaccharide (O-SP) which are virulence factors and targets of protective antibodies. The structure of the repeating unit of some of these PS and O-SP are shown in Table 1. Most PS are T-independent type 2 antigens that can directly stimulate B cells without the help of T cells (Mond et al., 1995; Snapper and Mond, 1996; Lesinski and Westerink, 2001). Cross-linking of multiple surface immunoglobulins on B cells by PS activates the B cells which differentiate into antibody-secreting plasma cells. However, PS can be poorly immunogenic, particularly in children below 2 years (Käyhty et al., 1984; Peltola et al., 1984; Austrian, 1989; Peter, 1998). Re-vaccination is often required, since antibody levels decline rapidly, but does not elicit a booster effect indicating that differentiation into memory B cells does not occur (Kelly et al., 2006). Indeed, immunization with unconjugated PS can deplete a preexisting memory B cell pool leading to hyporesponsiveness to subsequent immunization (Granoff and Pollard, 2007; Pollard et al., 2009; Poolman and Borrow, 2011). The antibody response to PS is little affected by adjuvants (Barrett, 1985; Rappuoli, 2018) and only glycoconjugate vaccines have been shown to induce mucosal IgG, which is key to achieve herd immunity.
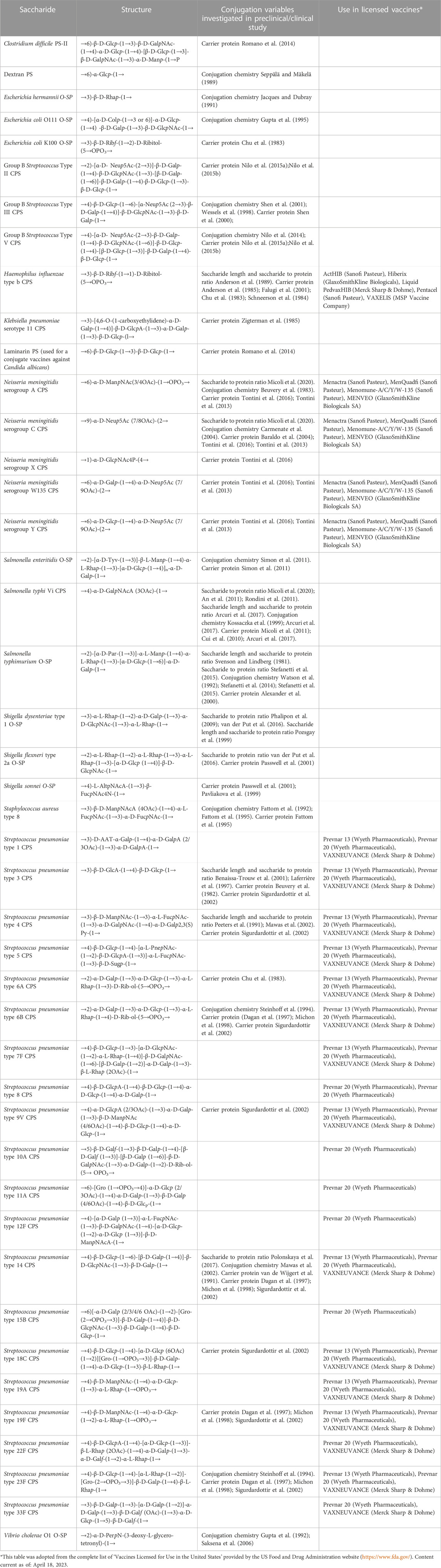
TABLE 1. Polysaccharides for which the impact of certain variables on the immunogenicity of corresponding glycoconjugates has been studied. Some of these saccharides are in licensed vaccines.
Conjugation of a saccharide to a suitable carrier protein converts it into a T-dependent antigen (Mäkelä and Käyhty, 2002; Weintraub, 2003) with B and T cells now able to interact in the generation of the antibody response (Mitchison, 1971a; b;2004). Importantly, conjugate vaccines can induce strong immune responses from birth (Lindberg, 1999; Pozsgay, 2000; Ravenscroft and Jones, 2000; Lesinski and Westerink, 2001; Mäkelä and Käyhty, 2002; Jones, 2005; Lucas et al., 2005). The PS component of the vaccine binds to surface immunoglobulin of PS-specific B cells. Following internalization of the vaccine, the protein component is processed and resulting peptide fragments are presented to the T cell receptor of CD4+ peptide-specific T cells in the peptide-binding groove of major histocompatibility complex class II molecules (MHCII). In addition to this cognate interaction, further signals are essential in eliciting CD4+ T cell help for the B cell (Borriello et al., 1997; Guttormsen et al., 1999). An alternative mechanism for the activation of the immune response by glycoconjugate vaccines has been also proposed, with the carbohydrate fragments of glycopeptides, produced by glycoconjugate vaccine processing within antigen-presenting cells, being recognized by CD4+ T cells while the peptide moieties allow binding to MHCII molecules (Lai and Schreiber, 2009; Avci et al., 2011). In this regard, a recent study demonstrated that the mechanisms of antigen presentation to T cells may also depend on the structure of the carbohydrate itself, and peptide or glycopeptide presentation to T cells could be of varying importance (Sun et al., 2019).
When B cells receive T cell help, they proliferate and differentiate into plasma cells, with class switching, particularly to IgG, and memory B cells. Memory B cells can rapidly proliferate and differentiate into plasma cells on subsequent encounter with the specific antigen producing high antibody titers (Insel and Anderson, 1986; Guttormsen et al., 1998; Tangye et al., 2003; Guirola et al., 2006; Pichichero, 2009). Antibody avidity is increased through affinity maturation in germinal centers (Goldblatt et al., 1998). Conjugate vaccines are normally not associated with hyporesponsiveness (Poolman and Borrow, 2011).
Persistence of antibodies following immunization, immunological memory and herd immunity (John and Samuel, 2000; Ramsay et al., 2003; Lexau et al., 2005) are important factors for long-term protection against invasive diseases from encapsulated bacteria (Pollard et al., 2009; Trück and Pollard, 2010; Blanchard-Rohner and Pollard, 2011). Despite induction of immunological memory, serum antibody levels and vaccine effectiveness can wane following infant immunization (MacLennan et al., 2000; Rennels et al., 2001; Borrow et al., 2002; Dagan et al., 2004; Trotter et al., 2004; Snape et al., 2005; Auckland et al., 2006; Tsai et al., 2006; Blanchard Rohner et al., 2008; Perrett et al., 2010). Booster doses of vaccine can maintain serum antibody levels above the protective threshold (Clutterbuck et al., 2006). Strategies to enhance the initial B cell response to immunization, using adjuvants and adjusting immunization schedules, can improve antibody persistence, although this needs further investigation (Borrow et al., 2003; Kelly et al., 2006; Blanchard Rohner et al., 2008; Clutterbuck et al., 2008; Kelly et al., 2009; Rappuoli, 2018).
2 Traditional and newer technologies for glycoconjugates
Traditionally, the synthesis of glycoconjugate vaccines requires a covalent linkage between the saccharide and the carrier protein. Many different conjugation chemistries have been proposed (Pozsgay, 2000; Frasch, 2009; Costantino et al., 2011; Micoli et al., 2019), but all follow two main approaches: 1) random linkage along the PS or OS chain (Figures 1, 2A); 2) selective attachment at the terminal end of OS (Figures 1, 2B). The random chemistry approach results in high molecular weight (MW), and cross-linked and rather undefined heterogeneous structures, while the selective approach produces better-defined structures, avoiding chemical modification of the saccharide chain (Pozsgay, 2000; Wang et al., 2003; Jones, 2005; Lucas et al., 2005). The possibility to conjugate chemically the PS at specific sites on the carrier protein has also been explored. These approaches facilitate structure-activity relationship investigation and preservation of key protein epitopes allowing a dual role of the protein component as carrier and antigen. Spacer molecules are often introduced between the saccharide and protein to reduce steric hindrance and facilitate interaction between both moieties (Kubler-Kielb and Pozsgay, 2005; Lees et al., 2006; Floyd et al., 2009; Costantino et al., 2011).
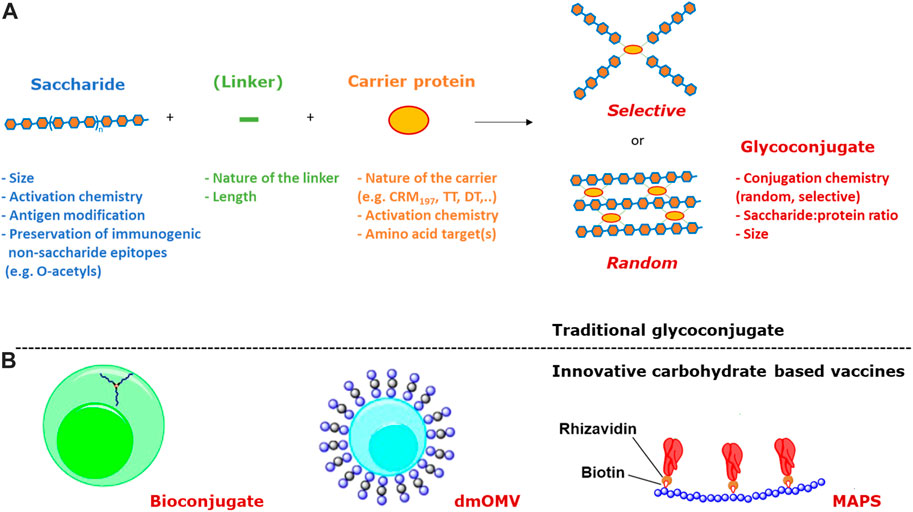
FIGURE 1. (A) The two main approaches to traditional conjugation of saccharides to proteins (linkers are not always present) and main variables impacting immunogenicity; (B) Innovative carbohydrate based vaccines based on bioconjugation, dmOMV and MAPS technologies.
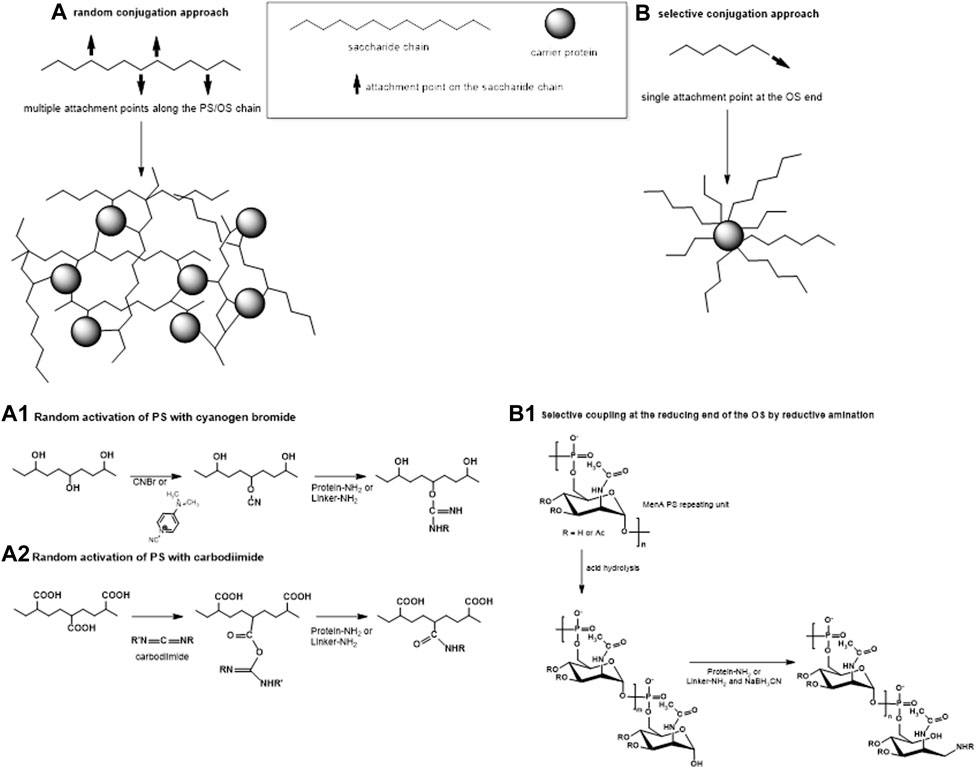
FIGURE 2. Two main approaches of conjugation: (A) random linkage sites along the PS or OS chain, resulting in high molecular weight cross-linked and rather undefined and heterogeneous conjugate structures and (B) selective attachment at the terminal end of the OS, resulting in better-defined conjugates without chemical modification of the saccharide chain. (A1–A2) Some commonly used methods for random activation of the PS using OH groups (1) or COOH groups (2) along the saccharide chain. (B1) An example of selective conjugation after depolymerization of the PS by mild acid hydrolysis. Mild hydrolysis, for example, of MenA PS, generates one terminal aldehyde group per shorter saccharide chain. Resulting aldehyde groups are linked to the protein or to the spacer by reductive amination.
An innovative methodology, known as bioconjugation (Figure 1B), has been developed for in vivo production of glycoproteins, where both the saccharide antigen and the carrier protein are expressed in Escherichia coli cells and coupled in vivo (Wacker et al., 2002). Glycoconjugate production in E. coli requires the presence of genome clusters encoding the bacterial polysaccharide, a plasmid encoding the carrier protein, and the oligosaccharyl transferase (OTase; traditionally the enzyme PglB from C. jejuni). The lipid undecaprenyl pyrophosphate (Und-PP), present in the cytoplasmic membrane of E. coli, works as an anchor for the construction of oligo- and polysaccharides. Then, the glycan moiety is flipped in the periplasmatic space and elongated by a polymerase. The PglB transfers Und-PP-linked glycans to the appropriate consensus acceptor sequence (i.e., D/E-X1-N-X2-S/T, where X1 and X2 can be any amino acid except proline) inserted in the carrier protein. This allows for precise control of where saccharide antigens are attached to the protein.
Alternative carrier systems, such as Outer Membrane Vesicles (OMV) have also been explored, which can facilitate antigen uptake by APCs, ensure display of the carbohydrate in multiple copies, and confer self-adjuvanticity through the presence of Toll-like receptor stimulatory molecules in the case of OMV (Berti and Micoli, 2020). Mutant-derived OMV (mdOMV), also called GMMA (Generalized Modules for Membrane Antigen) are OMV produced by Gram-negative bacteria which have been genetically manipulated to increase OMV release and reduce reactogenicity of lipid A. mdOMV (Figure 1B) have been proposed as delivery vehicles for O-antigens naturally present on their surface, or carrier for chemical conjugation of heterologous polysaccharides (Micoli and MacLennan, 2020). Also E. coli strains not expressing the O-SP portion of LPS molecules have been genetically manipulated for the biosynthesis and surface expression of heterologous polysaccharides anchored to lipid A-core as acceptors (Valguarnera and Feldman, 2017).
Covalent linkage of PS to the carrier protein has been thought fundamental to the immunological properties of conjugate vaccines. However, alternative approaches, such as the affinity-based coupling approach of the Multiple antigen-presenting system (MAPS) technology, have tested the co-delivery of the two components through non-conventional biotin-rhizavadin connection (Zhang et al., 2013) (Figure 1B).
The physical characteristics of traditional and innovative glycoconjugate vaccines (Figure 1), including saccharide length, saccharide to protein ratio, carrier protein, conjugation method and linker, can affect the magnitude, quality and persistence of the antibody response elicited, as well as cellular responses. Thus, these variables can have a marked influence on the success of a vaccine and a better understanding of the influence of these parameters can facilitate the rational design of glycoconjugate vaccines with improved immunogenicity and enhanced ability to protect. Here, we have conducted a comprehensive review of studies that compare the immunogenicity of conjugate vaccines differing in such characteristics. Table 1 reports the structure of the polysaccharides for which the impact of certain variables on the immunogenicity of corresponding glycoconjugates has been investigated. Saccharides used in licensed vaccines are highlighted.
3 Saccharide length and saccharide to protein ratio
We have recently reviewed the impact that PS chain length can have on the immune response elicited by glycoconjugate vaccines (Stefanetti et al., 2022). Saccharides can be isolated from bacterial cultures and are often reduced in size through different methodologies in order to simplify manufacture; improve conjugation yields, purification and consistency; and facilitate conjugate characterization. Shorter OS can be produced by organic synthesis and/or enzymatic approaches as well. According to the length of the conjugated sugars, different optimal saccharide to protein ratios can be identified, these two parameters appearing to be strongly interconnected. A high glycan loading could be needed for conjugation of short OS to result in an optimal immune response, while a lower density could be sufficient for longer PS. More recently nanoparticle carrier systems have been explored for the display of carbohydrates, offering multimeric presentation of glycan epitopes coupled with special chemico-physical properties of nano-sized particles. Control of saccharide loading on the protein can be critical when the protein plays a double role of carrier and antigen and, despite conjugation, the antigenicity of the protein needs to be preserved. Below, examples of saccharide loading impacting the immune response elicited by saccharide of defined chain length are reported.
3.1 Haemophilus influenzae type b
Anderson et al. (1989), studying Hib OS directly coupled at one end to CRM197 in human adults and 1-year-old infants, found that hapten loading (in a range of 0.02–0.1 μg ribose/μg protein) was a more critical variable than OS length, in the range of 4–12 repeating units. Increased saccharide loading produced enhanced antibody response.
3.2 Meningococcus
OMVs combine antigen presentation with the immunopotentiator effect of the Toll-like receptor agonists naturally present on these nanoparticles. When different average numbers of MenA or MenC OS (average size of 4.5 kDa) were conjugated to OMV membrane proteins, no major impact of OS density (in the range of 650–4,500 sugar chains per OMV) was observed on anti-PS IgG response and functionality of antibodies elicited in mice. However, the control of glycosylation density could be useful to fully preserve the immune response induced by protein components of the OMV (Micoli et al., 2020).
3.3 Salmonella
An et al. (2011) studied impact of full-length Vi loading on a traditional carrier protein like Diphtheria Toxoid (DT). Conjugates produced using random chemistry with ADH linker were tested in mice. The anti-Vi antibody response progressively increased with increasing DT to PS content. Rondini et al. (2011), using a similar conjugation approach as An et al., but with CRM197 as carrier, found that a weight to weight ratio of full length Vi to CRM197 of 0.9 and 2.1 produced more antibodies than a ratio of 10. Recently the impact of saccharide to protein ratio on the immunogenicity of full length (165 kDa) and fragmented Vi (43 kDa) randomly conjugated to CRM197 was evaluated, albeit over a limited range of ratios. Altering the ratio of Vi to protein from 0.8 to 1.4 (for full length Vi) and from 0.26 to 0.85 (for fragmented Vi) did not influence the anti-Vi IgG response in mice (Arcuri et al., 2017).
Conjugates differing for number of PS chains to OMV were produced by conjugation of S. Typhi Vi PS of 48.5 kDa (93 or 17 chains per OMV) or 3.8 kDa (138 or 55 chains per OMV) to S. Typhimurium OMV. No impact of antigen density on Vi-specific serum IgG response in mice was observed (Micoli et al., 2020).
Svenson and Lindberg (1981) found that immunogenicity of Salmonella Typhimurium octasaccharide-BSA conjugates in mice was enhanced by increasing the molar ratio of saccharide to protein from 7 to 23. However, with a longer Salmonella Typhimurium O-SP, composed of approximately 25 repeating units, robust immunogenicity was obtained even with a single attachment site, depending on the targeted amino acid on CRM197 (Stefanetti et al., 2015). Indeed the conjugate elicited similar bactericidal antibody response compared to a random conjugate with multiple saccharide chains in mice when the O-SP chain was linked to one of the disulfide bonds of CRM197, but not to specific lysine residues.
3.4 Shigella
For Shigella flexneri 2a conjugates with synthetic pentadecasaccharide, an average molar ratio of two saccharide chains per protein induced no anti-LPS antibodies in mice, and an average ratio of 14 was more immunogenic than eight (Phalipon et al., 2009). More recently (van der Put et al., 2016), comparison of four S. flexneri 2a glycoconjugates only differing by their pentadecasaccharide to Tetanus Toxoid (TT) ratio confirmed that hapten loading is critical for immunogenicity with an optimal saccharide to protein ratio in the range of 17 ± 5, supporting the selection of a synthetic conjugate with average loading of 15 OS per TT which was subsequently tested in a Phase 1 clinical study (Cohen et al., 2021). Pozsgay et al. (1999), testing immunogenicity of Shigella dysenteriae type 1 conjugates in mice, found that the optimal carbohydrate chain density differed with OS length, highlighting the interrelatedness of these parameters.
3.5 Streptococcus pneumoniae
Benaissa-Trouw et al., 2001) found that immunogenicity in mice of synthetic di-, tri-, and tetrasaccharides of S. pneumoniae serotype (PnPS) 3 conjugated to CRM197 was not influenced by the carbohydrate to protein ratio over a molar range of 2.9–12. Similar results were obtained in rabbits by Laferrière et al. (1997), who compared PnPS 3 of 8 repeating units differing for saccharide to protein molar ratio of 6.3, 8.1, and 12.5, and PnPS 19F OS of 17 repeats with 2.8 or 5.5 chains terminally linked to TT. However, the majority of studies have found that saccharide to protein ratio does affect immunogenicity of glycoconjugates.
Peeters et al. (1991) showed that PnPS 4 (4–120 kDa)-TT conjugates in the range of 0.3–2.8 saccharide to protein molar ratio were more immunogenic in mice than conjugates with a lower saccharide loading, while the immunogenicity of OS (12 repeating units, 9.6 kDa)-TT conjugates increased with higher saccharide density (PnPS:TT molar ratio 50 > 25 > 14). Also, for PnPS 14 synthetic tetrasaccharides coupled to CRM197, over the molar ratio ranges of 5–24, the highest OS loading corresponded to the highest level of antibodies in mice (Mawas et al., 2002).
Controlled reproducible loading of approximately 20–200 synthetic tetrasaccharides of PnPS 14 was achieved with nanoparticles made of 180 repetitive units assembled in a virus-like particle (VLP) (Polonskaya et al., 2017). Mice were immunized with conjugates bearing on average 20, 40, 80, or 200 OS per particle. No detectable anti-glycan antibody response was produced in mice immunized with VLPs containing an average of 20 OS, and a certain variability in both the primary and the secondary antibody responses was obtained with VLPs with an average of 40 OS. In contrast, conjugates with higher glycan densities produced similarly potent antibody responses in animals.
4 Conjugation chemistry
Conjugation chemistry plays an important role in determining the immunogenicity of glycoconjugate vaccines. Different approaches can be used to covalently attach the carbohydrate antigen to a carrier protein, and both the efficiency of the chemistry chosen, and the availability of reacting sites in the two molecules can influence critical immunological variables such as sugar to protein ratio and molecular size of the resulting vaccine (Jones, 2005; Costantino et al., 2011; Berti and Adamo, 2018). In addition, the use of linkers between the saccharide antigen and the carrier protein can facilitate the conjugation between those high-molecular weight biomolecules and can also affect the immunogenicity of these vaccines.
The method of carbohydrate coupling to the protein depends on the saccharide structure. Hydroxyl groups along the saccharide can be randomly activated by cyanylation (Schneerson et al., 1980; Chu et al., 1983; Lees et al., 1996; Shafer et al., 2000) (Figure 2A1). Sodium periodate can be used to oxidize two adjacent carbons with hydroxyl groups generating two aldehyde groups and breaking the C–C bond in the process. For some saccharides, like Hib and meningococcal serogroup C (MenC) PS, this produces shorter saccharides with reactive terminal aldehyde groups (Anderson et al., 1986), while for other saccharides, like MenA PS (Lee et al., 2009), this opens the sugar ring, without PS fragmentation. In both cases, the resulting carbonyl groups can be used for reductive amination with the protein directly or with a linker. Some PS, like Hib and meningococcal PS, can be fragmented by mild acid hydrolysis while maintaining the repeating unit motif, generating an aldehyde group at the reducing end only which is then available for conjugation (Costantino et al., 1992; Costantino et al., 1999; Ravenscroft et al., 1999; Matjila et al., 2004) (Figure 2B1). Salmonella Typhi and Staphylococcus aureus PS are resistant to acid hydrolysis and their conjugation has been achieved by activating carboxyl groups with carbodiimide (Szu et al., 1987; Fattom et al., 1995; Kossaczka et al., 1997) (Figure 2A2).
Amino groups on lysine residues or carboxyl groups on aspartic or glutamic acid residues are the main linkage points used on proteins for conjugation, sometimes after modification with linkers exposing chemical groups for conjugation. Recent years have seen the rise of novel site-selective protein glycosylation methods aiming to provide more homogeneous and well-defined glycoconjugate constructs, facilitating both conjugate physico-chemical characterization and the investigation of structure-activity relationship. Chemical, by targeting cysteine or tyrosine residues, or enzymatic, by modifying glutamine, glycine or lysine residues, approaches have been explored. Recombinant proteins have also been engineered by inserting unnatural amino acids, non-proteinogenic amino acids that either occur naturally or are chemically synthesized, in specific regions of the protein sequence (Hu et al., 2016). This strategy can enhance the functionality of proteins or peptides by introducing specific chemical groups or reactive moieties at specific sites along the protein sequence.
Significant progress has been made in recent years in identifying enzymes involved in natural glycan conjugation in bacteria, creating new opportunities for glycoconjugate vaccine production (Terra et al., 2012; Cuccui and Wren, 2015; Moeller et al., 2021). The oligosaccharyltransferase (OST) enzyme PglB from Campylobacter jejuni has been used to connect bacterial PS to carrier proteins through both chemoenzymatic and in vivo methods, providing an effective way to directly link sugars to a specific amino acid sequence of the protein. Both N-linked and O-linked glycosylation techniques have been employed, and ongoing efforts aim to expand these capabilities.
The use of peptides as carriers instead of proteins can result in conjugates where multiple peptides are attached only in one position (generally the C- or N-terminus) to multiple positions along the saccharide chain (Alexander et al., 2000; Alexander et al., 2004; Ingale et al., 2007; Xin et al., 2008). The recent finding that glycopeptide fragments may be involved in the immunological synapse and presented to TCR by MHC-II molecules (Avci et al., 2011) has focused attention on the fine details of the glycan-protein linkage, in particular the specific amino acid residue(s) involved. Targeting different amino acids for conjugation results in the formation of distinct glycopeptides with potentially different abilities to raise a specific T cell-dependent immune response against the carbohydrate portion. Interestingly, the need for covalent conjugation has been challenged recently by new technologies. These include the Protein Capsular Matrix Vaccine (PCMV; Matrivax) platform which enables the capture of both the sugar antigen and the carrier protein in a polymer matrix (Thanawastien et al., 2015), and the ‘MAPS’ (Multiple Antigen Presenting System) technology, where covalent binding is substituted by affinity-based coupling of proteins engineered with rhizavidin moieties and biotynilated PS (Zhang et al., 2013).
4.1 Dextrans
Seppala et al. (Seppälä and Mäkelä, 1989) studied the effect of random and selective (with and without ADH linker) chemistries to conjugate dextrans to Chicken Serum Albumin (CSA). Selective chemistry using short saccharide molecules (4–10 kDa) induced much higher antibody responses than random activation, and linker presence did not affect immunogenicity. For 40 kDa dextrans, conjugates prepared with random chemistry were more immunogenic than with selective methods.
4.2 Escherichia coli
For the design of vaccines to prevent against enteritis caused by Escherichia coli, four E. coli O111 glycoconjugates were synthesized and compared in mice. The saccharide was generated through the processing of its LPS using either acetic acid (O-SP) or the organic base hydrazine (DeA-LPS).
The O-SP had a reduced concentration of colitose. The products (O-SP or DeA-LPS) underwent derivatization with ADH (random derivatization) or thiolation using N-succinimidyl-3 (2-pyridyldithio) propionate (SPDP) (selective derivation on the aminoethanol moiety of the core region). The resulting four derivatives were then covalently attached to TT. Immunization studies in mice revealed that, out of the four conjugates tested, DeA-LPS–TT random conjugate produced using ADH as linker induced the most significant amount of anti-LPS antibodies, despite all conjugates tested having similar sugar to protein ratio (0.7–1.4). The authors suggested that the superior immunogenicity of this glycoconjugate over the other three conjugates tested can be attributed to several factors, including the increased retention of colitose, higher molecular weight (O-SP molecular weight population possesses two peaks with Kds of 0.2 and 0.5, while DeA-LPS had three peaks with Kd < 0.1, 0.4 and 0.6), the attachment of DeA-LPS to TT at multiple points, and the physicochemical characteristics of ADH (Gupta et al., 1995).
4.3 Escherichia hermannii
Escherichia hermannii O-SP linked directly to BSA gave earlier and more persistent antibody response in mice than when ADH linker was used, both approaches using random conjugation (Jacques and Dubray, 1991). Specifically, the direct conjugation required a lower dosage to induce a similar IgG response, that was sustained for at least 4 months.
4.4 Group B Streptococcus
Shen et al. compared the direct conjugation of GBS type III capsular PS, activated by random periodate oxidation, to recombinant cholera toxin B subunit (rCTB) via reductive amination, with two other random conjugation chemistries that employed cystamine and SPDP, or ADH, respectively. They also evaluated the impact of glycoconjugate size on immunogenicity by separating the conjugates into large and small molecular weight batches before intranasal immunization in mice. Sugar to protein ratios were comparable among the constructs (respectively around 0.9 for large size conjugates and around 1.1–1.5 for small size conjugates). Results showed that the large and small conjugates made by reductive amination or via ADH linking induced high capsular PS-specific IgG levels in serum, lungs, and vagina, with the larger batches eliciting higher IgA titers. In contrast, the conjugates made via SPDP linking were less immunogenic. When comparing sizes, the immunogenicity of smaller-molecular weight conjugates was generally lower than that of the larger-molecular weight conjugates, as evidenced by a reduced anti-capsular PS IgA immune response. Notably, the larger-molecular weight conjugate made by direct conjugation via reductive amination elicited the highest anti-capsular PS IgA titers in both tissues and serum, indicating that the molecular size of the conjugates influences the immune response (Shen et al., 2001).
Wessels et al. conducted a study on GBS type III capsular PS-TT conjugates, which were obtained through the reductive amination of sodium periodate oxidized-capsular PS (Wessels et al., 1998). The researchers identified an optimal threshold for PS-protein cross-linking to induce high titers of functional antibodies in mice. To examine the effect of PS-protein cross-linking on immunogenicity, the researchers constructed a series of vaccine candidates using type III GBS PS with various proportions of sialic acid residues modified by periodate oxidation (18%, 35%, 66%, or 89%). All four conjugates had a comparable sugar to protein ratio (total saccharide content 42%–50%). Immunogenicity studies in mice showed a significant direct relationship between the extent of PS-protein cross-linking and PS-specific antibody response to the conjugate. III66%-TT elicited significantly greater opsonic killing activity in antiserum than III18%-TT, III35%-TT, or III89%-TT, suggesting that some antibodies evoked by highly cross-linked conjugates were directed to a nonprotective epitope. Indeed, some antibodies recognized a new epitope induced by extensive oxidation of the PS, but not present on the native PS and therefore unlikely to be relevant for protective immunity (Cox et al., 2017).
The impact of conjugating GBS type V capsular PS, again activated randomly by oxidation, to specific lysine or tyrosine residues of the GBS67 pilus protein, which serves both as protein carrier and antigen, was evaluated. Protein-selective conjugates, made by click chemistry, thiol-maleimide addition or microbial Transglutaminase (mTGase) catalyzed insertion, were compared to GBS type V conjugates created by attaching the PS to either GBS67 or CRM197 proteins by random chemistry. Immunological study in mice revealed that all the site-directed constructs effectively induced high levels of anti-PS and anti-protein IgG, resulting in osponophagocytic killing of bacterial strains expressing either PSV or GBS67. Among the different site-directed conjugates tested, the tyrosine-directed thiol-maleimide addition was found to be the most effective in generating functional IgG against both PS and protein (as evidenced by in vitro opsonophagocytic killing titers), comparable to controls prepared by random conjugation, without inducing anti-linker antibodies (Nilo et al., 2015b).
4.5 Neisseria meningitidis
With conjugates of MenA PS linked to TT by random chemistries, use of glycine or 6-amino-n-hexanoic acid spacers led to four-fold higher protein to PS ratios compared with no spacer (Beuvery et al., 1983). Conjugates with a spacer were more immunogenic after the second, but not the first immunization in mice. With MenC PS (60 kDa) coupled to the meningococcal P64k protein, a random conjugate obtained by direct reductive amination after saccharide oxidation with NaIO4 (protein to saccharide ratio of 1.43) elicited higher anti-PS IgG titers and higher bactericidal activity than when carbodiimide condensation following saccharide derivatization with ADH was used (protein to saccharide ratio of 0.14) (Carmenate et al., 2004). However, the two conjugates had very different sugar to protein ratio, which complicates the evaluation of the impact of the conjugation chemistry on the immunogenicity response. Some studies have reported induction of high levels of linker-specific antibodies (Peeters et al., 1991; Buskas et al., 2004). In the presence of a strongly immunogenic glycan such as MenC PS, the chemical link between ADH and the PS is immunologically silent, but becomes immunodominant in the presence of a poor immunogen such as MenB PS (Bartoloni et al., 1995).
4.6 Salmonella
For Salmonella Enteritidis O-SP, the direct conjugation to flagellin monomers by random activation (two conjugates tested with saccharide to protein ratios of 0.97 and 0.26) induced the same IgG levels and protection against subsequent challenge in mice as with selective aminooxyoxime thioether chemistry using diaminooxy cysteamine and N-(γ-maleimidobutyloxy)-sulfosuccinimide ester linkers (saccharide to protein ratio = 0.45) (Simon et al., 2011).
For Salmonella Typhi Vi conjugates, PS linked to recombinant Pseudomonas aeruginosa exotoxin A (rEPA) by random chemistry was more immunogenic using an ADH linker (saccharide to protein ratio of 1.12) than with cystamine and SPDP (saccharide to protein ratio of 1.06) in humans (Kossaczka et al., 1999). More recently, different chemistries have been tested to link Vi of 43 kDa to CRM197 carrier protein, using -Ethyl-3-(3-Dimethylaminopropyl)carbodiimide, Hydrochloride (EDAC) or click chemistry for random conjugates, and EDAC chemistry or thiol chemistry with linkers with different lengths for selective conjugates, targeting different amino acids (Asp/Glu or Lys). No significant differences were observed in the anti-Vi IgG response induced in mice between the difference glycoconjugate constructs (Arcuri et al., 2017).
Recently, a classical glycoconjugate was compared with a MAPS construct for a carbohydrate-based vaccine against Salmonella Typhi. To assess the efficacy of the different vaccines, adoptive transfer of B and T cells from immunized wild-type C57BL/6 mice to Rag−/− mice were conducted. Specifically, the recall of memory response for a classical Vi-DT conjugate and a rEPA-Vi MAPS complex was compared. The results showed that the anti-Vi IgG antibody titers induced by the two conjugate vaccines were comparable (Zhang et al., 2022).
Watson et al. (1992) found that Salmonella Typhimurium O-SP-TT conjugates, produced by random activation and ADH linker (saccharide to protein ratio of 0.6) were more immunogenic in mice than when selective chemistry with ADH (saccharide to protein weight ratio of 0.1) was used, starting with O-SP having a bimodal molecular weight population with a major peak at 30 kDa and a minor peak at 119 kDa. Immunogenicity was also positively affected by the amount of linker incorporated, which had a positive linear relationship with the sugar to protein ratio in the final conjugate. However, for O-SP-CT conjugates produced by random (saccharide to protein ratio of 1.06) compared with two conjugates made by selective activation (saccharide to protein ratio of 0.23 and 0.5), using SPDP linker, there was no difference in the elicited immunogenicity. Using CRM197 as carrier protein (Stefanetti et al., 2014), Salmonella Typhimurium O-SP (20.5 kDa) was randomly activated by oxidation, respectively using a mild oxidizing agent, TEMPO (two conjugates with saccharide to protein ratio of 0.56 and 0.38), or NaIO4 (saccharide to protein ratio of 0.72), which opens the sugar units impacting O-SP epitopes and conformation. Selective activation at the reducing end of the saccharide was obtained by using two different lengths of the spacer (NH2-SIDEA (saccharide to protein ratio of 1.42) and ADH-SIDEA (saccharide to protein ratio of 1.74)) between the carrier protein and the sugar reducing end. The random and selective conjugates induced similar anti-OAg IgG responses in mice; however, the random conjugates induced antibodies with higher bactericidal activity. Notably, the use of TEMPO oxidation resulted in antibodies with the highest bactericidal activity. Another study involved synthesis of glycoconjugates with defined connectivity by targeting different amino acids on CRM197, including two novel methods for site-selective glycan conjugation (Stefanetti et al., 2015). Conjugation at the cysteine residues C186-201 bond resulted in higher anti O-SP bactericidal antibodies than coupling to lysines K37/39.
4.7 Staphylococcus aureus
Similar to Salmonella Typhi Vi conjugates, S. aureus type 8 capsular PS linked with ADH linker via random chemistry to rEPA (saccharide to protein ratio of 0.87) or DT (saccharide to protein ratio of 1.08) was more immunogenic in mice than the corresponding random conjugates with cystamine and SPDP (rEPA conjugate saccharide to protein ratio of 0.85, DT conjugate saccharide to protein ratio of 0.94). (Fattom et al., 1992; Fattom et al., 1995).
4.8 Streptococcus pneumoniae
Synthetic tetrasaccharides of PnPS 14 terminally conjugated to CRM197 using N-hydroxysuccinimide-activated adipic acid diester (average number of OS units per protein of 4) were more immunogenic in mice than when a diethyl squarate spacer (average number of OS units per protein of 4.8) was used (Mawas et al., 2002). Similar immunogenicity was obtained in young children when two bivalent pneumococcal conjugate vaccines were compared, consisting of serotype 6B and 23F OS conjugated to CRM197 directly or by a 6-carbon adipic acid spacer (Steinhoff et al., 1994).
4.9 Vibrio cholerae
Gupta et al. (Gupta et al., 1992) found that Vibrio cholerae O1 serotype Inaba deacylated lipopolysaccharides (LPS) (bimodal molecular weight population with two peaks at 13 kDa and 6 kDa) conjugated to cholera toxin (CT) were more immunogenic when obtained by random chemistry with ADH (saccharide to protein ratio of 0.8) than when produced by single-point attachment, using SPDP linkers (saccharide to protein ratio of 0.72). In another study, linkers of three different chain length (linkers extended by two (H2N(CH2)2NH2)- and six (H2N(CH2)6NH2)-carbon chains, respectively, compared to the use of an hydrazide linker (H2NNH2)) did not impact on the immunogenicity of V. cholerae O1 Ogawa serotype hexasaccharide-BSA selective conjugates tested in mice (Saksena et al., 2006).
5 Carrier protein
Theoretically, any protein can be used as a carrier protein. In addition to the ability to activate T-cells immune responses, important features to consider when choosing a carrier protein are purity and safety, manufacturability at industrial scale, and the possibility of being fully characterized by analytical methods. Additionally, a low likelihood of generating an antibody response to the carrier protein’s specific B cell epitopes can help to focus the immune response on the sugar hapten and allow for easy combination with other possible antigens with minimal immune interference. However, the possibility that the carrier protein originates from the pathogen of interest, thus also having a role as a protective antigen in addition to serving as a carrier, is an attractive possibility (Micoli et al., 2018).
Currently, five carrier proteins have been approved for use: diphtheria toxoid (DT), tetanus toxoid (TT), CRM197 (a non-toxic variant of diphtheria toxin), Haemophilus protein D (PD), and the outer membrane protein complex of serogroup B meningococcus (OMPC) (Pichichero, 2013; Micoli et al., 2018). TT and DT have traditionally been used because of safety data collected with tetanus and diphtheria vaccination. CRM197 does not require chemical detoxification, facilitating production and resulting in homogeneous preparations (Broker et al., 2011). Merck has used OMPC for its Hib conjugate vaccine (Donnelly et al., 1990) and GSK has used Hib-related protein D in a 10-valent pneumococcal conjugate vaccine (Forsgren et al., 2008). Other proteins have been used in conjugate vaccines under development, including rEPA as carrier for S. aureus types 5 and 8, Salmonella Typhi Vi, Shigella conjugate vaccines (Kossaczka et al., 1997; Fattom et al., 2004; Passwell et al., 2010) and several bioconjugates directly synthesized in E. coli (Wacker et al., 2014; Hatz et al., 2015; Riddle et al., 2016; Huttner et al., 2017). Investigating new carrier proteins is important due to factors such as pre-exposure and co-exposure to certain carriers leading to reduced anti-carbohydrate immune response and for a potential dual role as both a carrier and protective antigen. In this, case site-specific conjugation chemistries and glycoengineering are preferable to prevent detrimental effects on protein immunogenicity. Novel multivalent carrier systems, such as nanoparticles and mdOMV/GMMA, are being explored for their potential to present multiple copies of carbohydrate antigens and for their innate adjuvant properties (Micoli et al., 2018).
Below we report those studies in which different carrier proteins have been compared.
5.1 Candida albicans
Laminarin (Lam) from the brown alga Laminaria digitata was the model saccharide used for an immunogenicity study comparing different and novel carrier proteins for glycoconjugate vaccines against Candida albicans. Twenty-eight proteins from various pathogens were selected for recombinant expression in E. coli based on criteria such as lack of toxicity, solubility in physiological buffers, sufficient number of lysines for conjugation, and molecular weight between 40 and 100 kDa. The proteins were conjugated to Lam via reducing end, using the same active ester/protein molar ratio, and compared to a glycoconjugate using CRM197 as carrier (saccharide to protein ratio varied from 1.7 to 0.1 w/w). The pneumococcal protein spr96/2021 was shown to be a promising carrier inducing IgG titers significantly higher than CRM197-Lam (Tontini et al., 2016).
5.2 Clostridium difficile
In a recent study, selective chemistry was used to conjugate recombinant fragments of the toxins TcdA_B2 and TcdB_GT from Clostridium difficile to the PS PSII from the same organism (13 kDa). It was found that only the TcdB_GT conjugate was able to generate anti-PSII IgG levels in mice that were similar to those produced by a CRM197-PSII conjugate, with all conjugates bearing comparable saccharide to protein ratios (0.28–0.33 w/w). Both conjugates elicited anti-carrier IgG with toxin-neutralizing activity that were comparable to those of the non-conjugated proteins (Romano et al., 2014).
5.3 Escherichia coli
Conjugates were created by random carbodiimide-mediated coupling of ADH-derivatives of E. coli K100 PS respectively with TT (protein to saccharide ratio = 2.3 w/w) and horseshoe crab hemocyanin (HCH) (protein to saccharide ratio = 2 w/w). The E. coli K 100-HCH conjugate was more immunogenic than the E. coli K100-TT conjugate in mice (Chu et al., 1983).
5.4 B Streptococcus
GBS type III PS (460 kDa) conjugated to rCTB by random chemistry (saccharide to protein ratio of 0.78 w/w) induced higher IgG and IgA response in the lungs and vagina in mice after intranasal immunization, than with TT (saccharide to protein ratio of 0.8 w/w) as carrier protein (Shen et al., 2000).
In another study (Nilo et al., 2015b), GBS type V capsular PS activated by oxidation and randomly conjugated to CRM197 (saccharide to protein ratio of 1.9 w/w) or GBS67 (saccharide to protein ratio of 1.8 w/w), a pathogen-specific pilus protein with the dual role of T cell carrier for the PS and antigen, showed comparable immune responses in mice. Similarly, GBS type II capsular PS conjugated via reductive amination to another GBS-specific pilus protein, GBS80, by random conjugation (saccharide to protein ratio of 1.8 w/w) showed comparable immunogenicity than a random glycoconjugate using CRM197 (saccharide to protein ratio of 1.1 w/w) (Nilo et al., 2015a).
5.5 Haemophilus influenzae type b
While Hib OS (3–10 repeating units) conjugated to CRM197 or DT via reducing end produced the same primary response in infants 19–23 months of age, on boosting the CRM197 conjugate elicited higher antibody levels than the DT conjugate. Five months later the antibody levels induced by the CRM197 conjugate had declined faster, so that levels for both conjugates no longer differed significantly. In 12–16 months old infants, only the CRM197 conjugate elicited strong primary and secondary antibody responses (Anderson et al., 1985).
Some studies have investigated T cell epitope carriers (de Velasco et al., 1995; Könen-Waisman et al., 1999; Amir-Kroll et al., 2003; Avci et al., 2011). Falugi et al. (Falugi et al., 2001) used three recombinant carrier proteins (N6, N10, N19) consisting of strings of 6, 10 or 19 human CD4+ T cell epitopes from various pathogen-derived antigens, including TT and proteins from Plasmodium falciparum, influenza virus and hepatitis B virus. When conjugated to Hib OS (5.5 KDa) via reducing end, the helper effect of the carrier was proportional to its size with Hib-N19 eliciting an anti-Hib IgG response greater than Hib-CRM197 (Falugi et al., 2001).
In another mouse study, the use of TT or horseshoe crab hemocyanin (HCH), respectively as carrier proteins, was found to similarly affect the immunogenicity of random Hib conjugates made by cyanogen bromide activation with ADH and conjugation to the protein (Chu et al., 1983). However, when Hib conjugates, prepared with the same chemistry (Chu et al., 1983) using TT, HCH or Cholera Toxin (CT) as carrier proteins, were compared in rhesus monkeys (Schneerson et al., 1984), Hib-HCH was less immunogenic than Hib-TT in juvenile monkeys and Hib-CT more immunogenic than Hib-TT in infant monkeys.
5.6 Klebsiella pneumoniae
Klebsiella serotype 11 octasaccharides were conjugated to KLH (molar ratio saccharide to protein of 18) or BSA (molar ratio saccharide to protein of 5) carrier proteins and their immunogenicity was compared in mice. The two conjugates induced a comparable immune response in BALB/c mice, while only the KLH conjugate was able to induce an immune response in (CBA/N x C3H/HeN)F1 mice, possessing an immature set of B cells. This suggests that certain carrier determinants present in KLH, but not in BSA, are likely to be required for the induction of antibodies with OS-protein conjugates in animal models with immature B cells (Zigterman et al., 1985).
5.7 Neisseria meningitidis
Similarly to Hib glycoconjugates (Falugi et al., 2001), the polyepitope carrier N19 (saccharide to protein ratio of 0.4) exerted a stronger carrier effect than CRM197 (saccharide to protein ratio of 0.49) in mice when conjugated to MenC OS (MW around 4 kDa) (Baraldo et al., 2004). Indeed, after two immunizations, the N19-conjugate induced anti-MenC antibody and protective bactericidal antibody titers higher than those induced by three doses of the CRM197-conjugate. In addition, after three doses, lower amounts of N19-based conjugate induced levels of anti-MenC IgG antibodies significantly higher than those induced by the CRM197-conjugate (e.g., N19-MenC at 0.156 μg versus CRM197-MenC at 0.625 μg).
In another study in mice, 8 recombinant proteins from various pathogens were evaluated as carriers for meningococcal saccharide antigens in comparison to CRM197 for selective conjugate vaccines. Some of the proteins, such as the pneumococcal spr 96/2021 and spr1875 from pneumococcus, and Upec-5211 and Orf3526 from Extra Intestinal Pathogenic E. coli (saccharide to protein ratios of 0.1–0.6 w/w), showed good promise as carriers as the corresponding conjugates elicited in mice anti-meningococcal serogroup C IgG titers similar to those obtained with the CRM197-based glycoconjugate (MenC OS MW around 4 kDa). Men A, Men W, Men Y and Men X - spr96/2021 conjugates, after three doses, induced specific anti-Men IgG and bactericidal titers comparable to corresponding CRM197 conjugates (Tontini et al., 2016).
It is important to evaluate the effect of prior exposure to carrier proteins on the anti-carbohydrate response to detect potential immune interference in individuals who have previously received vaccines containing DT and TT carrier proteins. Comparing the immunogenicity of Men OS (MW around 4 kDa) conjugated to CRM197, DT, and TT using a selective chemistry (comparable saccharide to protein ratio of = 0.1—0.4 w/w), the three carriers were equally effective in inducing an immune response against the carbohydrate moiety in mice without prior immunization, for all the serogroups tested (A, C, W-135 and Y). However, using Men A as a model, CRM197 priming had a stronger ability to enhance the anti-carbohydrate response elicited by CRM197 conjugates or conjugates with the antigenically related carrier DT, while TT priming did not have any effect. Additionally, repeated exposure of mice to TT or CRM197 before immunization with the respective MenA conjugates resulted in a significant suppression of the anti-carbohydrate response with TT conjugate and only a slight reduction with CRM197 conjugate. The effect of carrier priming on the anti-MenA response of DT-based conjugates varied depending on the carbohydrate to protein ratio (Tontini et al., 2013).
5.8 Salmonella
Salmonella Enteritidis O-SP conjugates were similarly immunogenic in mice when coupled to S. Enteritidis flagellin monomers, polymers or CRM197 by random conjugation with no linker (Simon et al., 2011). Simon et al. proposed flagellin as the carrier protein for Salmonella Enteritidis O-SP, both being a virulence factor and protective antigens with the potential to achieve enhanced protection by the additive effect of anti-O-SP and anti-flagellin immune responses. Mice immunized with core polysaccharide-OPS (COPS) respectively conjugated to CRM197 or flagellin polymer by random chemistry with the same PS/protein ratio elicited similar anti-LPS IgG levels and were significantly protected from lethal challenge with wild-type S. Enteritidis (80%–100% vaccine efficacy) (Simon et al., 2011).
Conjugates of the 13 amino acids peptide non-natural pan DR epitope (PADRE) with the human milk oligosaccharide, lacto-N-fucopentose II or a dodecasaccharide derived from Salmonella Typhimurium O-SP induced high glycan IgG titers in mice, which were comparable to glycoconjugates employing human serum albumin (HSA) as the carrier protein (Alexander et al., 2000).
Studies comparing Vi conjugates against Salmonella Typhi, obtained by random chemistry with ADH linker and different carrier protein, CRM197 and TT (Micoli et al., 2011), or DT and rEPA (Cui et al., 2010) found no effect of the carrier protein on immunogenicity in mice. In another study, full length Vi (165 kDa) conjugates induced strong IgG responses after just one dose, regardless of the carrier protein used (CRM197, DT, or TT), and a second dose did not increase the response. Fragmented Vi (43 kDa) conjugated to CRM197 and DT (but not TT) resulted in lower anti-Vi IgG responses after the first dose compared with full-length Vi conjugates. However, after a second dose, IgG levels were similar to those induced by full-length Vi conjugates, regardless of carrier protein (Arcuri et al., 2017).
5.9 Shigella
Vaccination of healthy adults using O-SP of Shigella sonnei conjugated to succinylated rEPA (saccharide to protein ratio of 2.02 w/w) or to native (saccharide to protein ratio of 1.35 w/w) or succinylated (saccharide to protein ratio of 2.06 w/w) Corynebacterium diphtheriae toxin mutant CRM9 by random chemistry and ADH, gave the same anti-LPS IgG levels (Passwell et al., 2001). With S. flexneri 2a O-SP, the rEPAsucc conjugate (saccharide to protein ratio of 2.58 w/w) gave significantly higher antibody levels than the CRM9succ conjugate (saccharide to protein ratio of 2.58 w/w) (Passwell et al., 2001). The opposite result was obtained in mice, with S. flexneri 2a-CRM9 (saccharide to protein ratio of 1.70 w/w) being more immunogenic than S. flexneri 2a-rEPA (saccharide to protein ratio of 0.60 w/w) (Pavliakova et al., 1999).
5.10 Staphylococcus aureus
Staphylococcus aureus type 8 capsular PS conjugated via random chemistry to DT with SPDP linker (saccharide to protein ratio of 0.94 w/w) was more immunogenic in mice than when rEPA was used (saccharide to protein ratio of 0.85 w/w), but there was no difference when the linker was ADH (DT conjugate saccharide to protein ratio of 1.08 w/w, rEPA conjugate saccharide to protein ratio of 0.87 w/w) (Fattom et al., 1995).
5.11 Streptococcus pneumoniae
Pneumococcal type 3 PS conjugated by random chemistry to TT (saccharide to protein ratio of 1.24 w/w) induced higher PS-specific IgG antibody levels in mice than BSA (saccharide to protein ratio of 0.52 w/w) and DT (saccharide to protein ratio of 1.17 w/w) conjugates prepared by the same method (Beuvery et al., 1982). In another study, the total IgG and IgM antibody concentrations induced in mice by PnPS 14 conjugated by random chemistry to BSA (saccharide to protein ratio of 0.85 w/w) were higher than those induced by Salmonella Typhi flagellin conjugate (saccharide to protein ratio of 1.4 w/w) (van de Wijgert et al., 1991), obtained using the same carbodiimide conjugation chemistry. Comparing two tetravalent vaccines containing pneumococcal type 6B, 14, 19F and 23F PS in a double-blinded study of young infants, antibody concentrations elicited by PnPS-TT were generally higher than those elicited by PnPS-DT (Dagan et al., 1997). However, there was a significant booster response to each serotype in both the PnPS-TT and PnPS-DT groups using unconjugated PS 6 months after priming.
A genetically detoxified pneumolysin, pneumolysoid (PLD), was investigated as a carrier protein for pneumococcal capsular PS, serotypes 6B, 14, 19F, and 23F and compared to TT conjugates made by random activation. Pneumolysin is a toxin produced by all types of S. pneumoniae and is a major virulence factor. The PLD conjugates were shown to be equivalent to or better in terms of PS-specific responses than the TT conjugates and elicited high levels of pneumolysin-specific IgG able to neutralize pneumolysin-induced hemolytic activity in vitro (Michon et al., 1998).
Sigurdardottir et al. compared DT and TT as carrier for an octavalent (serotypes 3, 4, 6B, 9V, 14, 18C, 19F and 23F) pneumococcal random conjugates formulation, finding that serotype-specific immune responses depended on the carrier protein used: DT gave better primary responses to serotypes 3, 9V and 18C, whereas TT gave better response to serotype 4 (Sigurdardottir et al., 2002). Similar responses were induced to the other serotypes. Good booster IgG responses were obtained in all vaccine groups.
Chu et al., comparing TT with the carrier horseshoe crab hemocyanin (HCH) for random PnPS 6A glycoconjugate vaccines in mice, found that PnPS 6A-TT (saccharide to protein ratio of 0.59 w/w) induced higher anti PS specific IgG levels than PnPS 6A-HCH (saccharide to protein ratio of 0.36 w/w) (Chu et al., 1983).
6 Conclusion
Many animal studies have investigated the influence of saccharide chain length, saccharide to protein ratio, conjugation method and carrier protein on the immunogenicity of traditional glycoconjugate vaccines (Table 1). Although in some studies more than one vaccine variable is altered at a time and the effects can be saccharide-specific, the importance of these parameters in determining overall immunogenicity is evident and some general principles emerge. Few studies have been performed with the newer technologies such as bioconjugates, dmOMVs and MAPS. Lessons learned from traditional glycoconjugates may also be relevant for the design of these next-generation polysaccharide and OS-based vaccines.
Synthetic OS have facilitated careful study of the influence of saccharide chain length and saccharide density on the immunogenicity of glycoconjugates (Pozsgay et al., 1999; Mawas et al., 2002; Pozsgay et al., 2007), but they are generally small in size. Anti-saccharide responses in animals can potentially be induced by conjugates with short OS comprising only one repeating unit of the corresponding PS or even only fragments thereof (Alonso de Velasco et al., 1993; Benaissa-Trouw et al., 2001; Jansen et al., 2001; Mawas et al., 2002; Jansen and Snippe, 2004). However, human studies in infants evaluating Hib or pneumococcal conjugates that differ in the length of OS indicate that increased immunogenicity occurs with longer OS (Anderson et al., 1986; Pichichero et al., 1998).
The effect of saccharide length on immunogenicity is related to other parameters, in particular hapten loading and conjugation chemistry. The use of low MW OS may maximize the T cell help offered by the carrier protein, but such glycoconjugates may fail to develop functional anti-PS antibodies if key conformational epitopes on the PS are lost (Peeters et al., 1991; Pozsgay, 2000; Peeters et al., 2003). It is likely that a minimal chain length is required to induce a strong immunological response that varies for each saccharide type (Peeters et al., 1992; Chong et al., 1997).
Some studies do not report an influence of the saccharide to protein ratio on the immunogenicity of conjugate vaccines (Laferrière et al., 1997; Benaissa-Trouw et al., 2001). The effect of this parameter is strongly influenced by saccharide size and conjugation chemistry. In general, lower saccharide to protein ratios increase the immunogenicity of native PS conjugated by random chemistry. This may be related to the large size and high degree of cross-linking of such conjugates, with a critical amount of protein required to stimulate an optimal T-dependent response. With terminally linked OS, the optimal saccharide to protein ratio depends on saccharide length, but generally immunogenicity is directly correlated to the saccharide to protein ratio. This is likely to result from better cross-linking and activation of saccharide-specific B cells with increased OS loading. Nonetheless, with high saccharide-to-protein ratios, essential carrier epitopes may be hidden from the immune system, hindering recognition of the conjugate vaccine as a T-dependent antigen (Pozsgay et al., 1999; Peeters et al., 2003).
The choice of conjugation strategy and linker has an important effect on conjugation efficiency, saccharide to protein ratio and vaccine size, resulting in an impact on immunogenicity (Beuvery et al., 1983; Carmenate et al., 2004). Different moieties of the saccharide and different amino acids of the protein can be involved in the conjugation process influencing which epitopes are exposed. In general, with short OS, selective chemistries are better than random ones and the converse applies with long PS. Linkers can differ in length and flexibility affecting the distance between saccharide and protein and the access of antigen binding sites to surface immunoglobulin on B cells.
Most animal studies indicate that the choice of carrier protein has an impact on conjugate vaccine immunogenicity. Overall, it is not straightforward to draw conclusions about which protein carrier has the optimal impact on the immunogenicity of glycoconjugate vaccines. This parameter is likely to be antigen-specific and interdependent with other factors such as conjugation chemistry, the presence of a spacer, and the size and degree of conjugation of the saccharide. Furthermore, the valency of the administered glycoconjugate (i.e., monovalent vs. multivalent) is likely to play a role, particularly in clinical settings where immune interference caused by (pre)exposure to the same carrier protein can result in a positive or negative effect on the immune response to the conjugated carbohydrate antigen.
Predicting successful vaccine design strategies for humans, especially infants, is not straightforward. Most of the published data on the influence of variables of conjugation on vaccine immunogenicity comes from animal studies. Clinical trials investigating conjugates differing in only one parameter are rare (Anderson et al., 1989; Steinhoff et al., 1994; Daum et al., 1997; Kossaczka et al., 1999; Passwell et al., 2001) and only a few studies have evaluated whether the results obtained on animals are reproducible in humans (Anderson et al., 1986; Pichichero et al., 1998). The determination of vaccine structure-immunogenicity relationships for humans, and especially for infants, is limited by ethical issues related to testing candidate vaccines in humans that are poorly immunogenic in animals, and by the cost of clinical trials. Good animal models are important for prioritizing which vaccines move into clinical trials. For example, Siber et al. showed that a guinea pig model could successfully predict the immunogenicity of Hib conjugate vaccines in humans (Siber et al., 1995).
Furthermore, animal studies usually focus on the influence of conjugation variables on the immediate immune response. Studies investigating the effect of parameters on antibody persistence and memory response are also needed. Current licensed conjugate vaccines are often characterized by a marked decline in antibody levels over time, particularly in infants, with multiple primary doses and/or boosters required to ensure protection. Improving the longevity of the immune response would increase the benefit-cost ratio of conjugate vaccines.
In conclusion, conjugation parameters are strongly interconnected in determining vaccine efficacy and the best candidate glycoconjugate vaccines will result from an optimal combination of each of these variables. Studies that use a matrix approach to examine the influence of different combinations of variables may provide the fastest route to identifying the most promising vaccines.
Author contributions
All authors wrote the main manuscript text, prepared figures and tables, reviewed and approved this manuscript.
Conflict of interest
FM is employee of the GSK group of companies and owner of GSK shares.
The remaining authors declare that the research was conducted in the absence of any commercial or financial relationships that could be construed as a potential conflict of interest.
Publisher’s note
All claims expressed in this article are solely those of the authors and do not necessarily represent those of their affiliated organizations, or those of the publisher, the editors and the reviewers. Any product that may be evaluated in this article, or claim that may be made by its manufacturer, is not guaranteed or endorsed by the publisher.
References
Ada, G., and Isaacs, D. (2003). Carbohydrate-protein conjugate vaccines. Clin. Microbiol. Infect. 9, 79–85. doi:10.1046/j.1469-0691.2003.00530.x
Alexander, J., Del Guercio, M.F., Frame, B., Maewal, A., Sette, A., Nahm, M. H., et al. (2004). Development of experimental carbohydrate-conjugate vaccines composed of Streptococcus pneumoniae capsular polysaccharides and the universal helper T-lymphocyte epitope (PADRE). Vaccine 22, 2362–2367. doi:10.1016/j.vaccine.2003.11.061
Alexander, J., Del Guercio, M. F., Maewal, A., Qiao, L., Fikes, J., Chesnut, R. W., et al. (2000). Linear PADRE T helper epitope and carbohydrate B cell epitope conjugates induce specific high titer IgG antibody responses. J. Immunol. 164, 1625–1633. doi:10.4049/jimmunol.164.3.1625
Alonso De Velasco, E., Verheul, A. F., Veeneman, G. H., Gomes, L. J., Van Boom, J. H., Verhoef, J., et al. (1993). Protein-conjugated synthetic di- and trisaccharides of pneumococcal type 17F exhibit a different immunogenicity and antigenicity than tetrasaccharide. Vaccine 11, 1429–1436. doi:10.1016/0264-410x(93)90172-t
Amir-Kroll, H., Nussbaum, G., and Cohen, I. R. (2003). Proteins and their derived peptides as carriers in a conjugate vaccine for Streptococcus pneumoniae: Self-heat shock protein 60 and tetanus toxoid. J. Immunol. 170, 6165–6171. doi:10.4049/jimmunol.170.12.6165
An, S. J., Yoon, Y. K., Kothari, S., Kothari, N., Kim, J. A., Lee, E., et al. (2011). Physico-chemical properties of Salmonella typhi Vi polysaccharide-diphtheria toxoid conjugate vaccines affect immunogenicity. Vaccine 29, 7618–7623. doi:10.1016/j.vaccine.2011.08.019
Anderson, P., Pichichero, M. E., and Insel, R. A. (1985). Immunogens consisting of oligosaccharides from the capsule of Haemophilus influenzae type b coupled to diphtheria toxoid or the toxin protein CRM197. J. Clin. Invest. 76, 52–59. doi:10.1172/JCI111976
Anderson, P. W., Pichichero, M. E., Insel, R. A., Betts, R., Eby, R., and Smith, D. H. (1986). Vaccines consisting of periodate-cleaved oligosaccharides from the capsule of Haemophilus influenzae type b coupled to a protein carrier: Structural and temporal requirements for priming in the human infant. J. Immunol. 137, 1181–1186. doi:10.4049/jimmunol.137.4.1181
Anderson, P. W., Pichichero, M. E., Stein, E. C., Porcelli, S., Betts, R. F., Connuck, D. M., et al. (1989). Effect of oligosaccharide chain length, exposed terminal group, and hapten loading on the antibody response of human adults and infants to vaccines consisting of Haemophilus influenzae type b capsular antigen unterminally coupled to the diphtheria protein CRM197. J. Immunol. 142, 2464–2468. doi:10.4049/jimmunol.142.7.2464
Arcuri, M., Di Benedetto, R., Cunningham, A. F., Saul, A., Maclennan, C. A., and Micoli, F. (2017). The influence of conjugation variables on the design and immunogenicity of a glycoconjugate vaccine against Salmonella Typhi. Plos One 12, e0189100. doi:10.1371/journal.pone.0189100
Auckland, C., Gray, S., Borrow, R., Andrews, N., Goldblatt, D., Ramsay, M., et al. (2006). Clinical and immunologic risk factors for meningococcal C conjugate vaccine failure in the United Kingdom. J. Infect. Dis. 194, 1745–1752. doi:10.1086/509619
Austrian, R. (1989). Pneumococcal polysaccharide vaccines. Rev. Infect. Dis. 11 (3), S598–S602. doi:10.1093/clinids/11.supplement_3.s598
Avci, F. Y., Li, X., Tsuji, M., and Kasper, D. L. (2011). A mechanism for glycoconjugate vaccine activation of the adaptive immune system and its implications for vaccine design. Nat. Med. 17, 1602–1609. doi:10.1038/nm.2535
Avery, O. T., and Goebel, W. F. (1929). Chemo-immunological studies on conjugated carbohydrate-proteins: II. Immunological specificity of synthetic sugar-protein antigens. J. Exp. Med. 50, 533–550. doi:10.1084/jem.50.4.533
Baraldo, K., Mori, E., Bartoloni, A., Petracca, R., Giannozzi, A., Norelli, F., et al. (2004). N19 polyepitope as a carrier for enhanced immunogenicity and protective efficacy of meningococcal conjugate vaccines. Infect. Immun. 72, 4884–4887. doi:10.1128/IAI.72.8.4884-4887.2004
Barrett, D. J. (1985). Human immune responses to polysaccharide antigens: An analysis of bacterial polysaccharide vaccines in infants. Adv. Pediatr. 32, 139–158.
Bartoloni, A., Norelli, F., Ceccarini, C., Rappuoli, R., and Costantino, P. (1995). Immunogenicity of meningococcal B polysaccharide conjugated to tetanus toxoid or CRM197 via adipic acid dihydrazide. Vaccine 13, 463–470. doi:10.1016/0264-410x(94)00007-a
Benaissa-Trouw, B., Lefeber, D. J., Kamerling, J. P., Vliegenthart, J. F., Kraaijeveld, K., and Snippe, H. (2001). Synthetic polysaccharide type 3-related di-tri-and tetrasaccharide-CRM(197) conjugates induce protection against Streptococcus pneumoniae type 3 in mice. Infect. Immun. 69, 4698–4701. doi:10.1128/IAI.69.7.4698-4701.2001
Berti, F., and Adamo, R. (2018). Antimicrobial glycoconjugate vaccines: An overview of classic and modern approaches for protein modification. Chem. Soc. Rev. 47, 9015–9025. doi:10.1039/c8cs00495a
Berti, F., and Micoli, F. (2020). Improving efficacy of glycoconjugate vaccines: From chemical conjugates to next generation constructs. Curr. Opin. Immunol. 65, 42–49. doi:10.1016/j.coi.2020.03.015
Beuvery, E. C., Van Rossum, F., and Nagel, J. (1982). Comparison of the induction of immunoglobulin M and G antibodies in mice with purified pneumococcal type 3 and meningococcal group C polysaccharides and their protein conjugates. Infect. Immun. 37, 15–22. doi:10.1128/IAI.37.1.15-22.1982
Beuvery, E. C., Vd Kaaden, A., Kanhai, V., and Leussink, A. B. (1983). Physicochemical and immunological characterization of meningococcal group A polysaccharide-tetanus toxoid conjugates prepared by two methods. Vaccine 1, 31–36. doi:10.1016/0264-410x(83)90010-5
Blanchard Rohner, G., Snape, M. D., Kelly, D. F., John, T., Morant, A., Yu, L. M., et al. (2008). The magnitude of the antibody and memory B cell responses during priming with a protein-polysaccharide conjugate vaccine in human infants is associated with the persistence of antibody and the intensity of booster response. J. Immunol. 180, 2165–2173. doi:10.4049/jimmunol.180.4.2165
Blanchard-Rohner, G., and Pollard, A. J. (2011). Long-term protection after immunization with protein-polysaccharide conjugate vaccines in infancy. Expert Rev. Vaccines 10, 673–684. doi:10.1586/erv.11.14
Borriello, F., Sethna, M. P., Boyd, S. D., Schweitzer, A. N., Tivol, E. A., Jacoby, D., et al. (1997). B7-1 and B7-2 have overlapping, critical roles in immunoglobulin class switching and germinal center formation. Immunity 6, 303–313. doi:10.1016/s1074-7613(00)80333-7
Borrow, R., Goldblatt, D., Andrews, N., Southern, J., Ashton, L., Deane, S., et al. (2002). Antibody persistence and immunological memory at age 4 years after meningococcal group C conjugate vaccination in children in the United Kingdom. J. Infect. Dis. 186, 1353–1357. doi:10.1086/344324
Borrow, R., Goldblatt, D., Finn, A., Southern, J., Ashton, L., Andrews, N., et al. (2003). Immunogenicity of, and immunologic memory to, a reduced primary schedule of meningococcal C-tetanus toxoid conjugate vaccine in infants in the United Kingdom. Infect. Immun. 71, 5549–5555. doi:10.1128/iai.71.10.5549-5555.2003
Broker, M., Costantino, P., Detora, L., Mcintosh, E. D., and Rappuoli, R. (2011). Biochemical and biological characteristics of cross-reacting material 197 CRM197, a non-toxic mutant of diphtheria toxin: Use as a conjugation protein in vaccines and other potential clinical applications. Biologicals 39, 195–204. doi:10.1016/j.biologicals.2011.05.004
Buskas, T., Li, Y., and Boons, G. J. (2004). The immunogenicity of the tumor-associated antigen Lewis(y) may be suppressed by a bifunctional cross-linker required for coupling to a carrier protein. Chemistry 10, 3517–3524. doi:10.1002/chem.200400074
Carmenate, T., Canaán, L., Alvarez, A., Delgado, M., González, S., Menéndez, T., et al. (2004). Effect of conjugation methodology on the immunogenicity and protective efficacy of meningococcal group C polysaccharide-P64k protein conjugates. FEMS Immunol. Med. Microbiol. 40, 193–199. doi:10.1016/S0928-8244(03)00346-8
Chong, P., Chan, N., Kandil, A., Tripet, B., James, O., Yang, Y. P., et al. (1997). A strategy for rational design of fully synthetic glycopeptide conjugate vaccines. Infect. Immun. 65, 4918–4925. doi:10.1128/IAI.65.12.4918-4925.1997
Chu, C., Schneerson, R., Robbins, J. B., and Rastogi, S. C. (1983). Further studies on the immunogenicity of Haemophilus influenzae type b and pneumococcal type 6A polysaccharide-protein conjugates. Infect. Immun. 40, 245–256. doi:10.1128/IAI.40.1.245-256.1983
Clutterbuck, E. A., Oh, S., Hamaluba, M., Westcar, S., Beverley, P. C., and Pollard, A. J. (2008). Serotype-specific and age-dependent generation of pneumococcal polysaccharide-specific memory B-cell and antibody responses to immunization with a pneumococcal conjugate vaccine. Clin. Vaccine Immunol. 15, 182–193. doi:10.1128/CVI.00336-07
Clutterbuck, E. A., Salt, P., Oh, S., Marchant, A., Beverley, P., and Pollard, A. J. (2006). The kinetics and phenotype of the human B-cell response following immunization with a heptavalent pneumococcal-CRM conjugate vaccine. Immunology 119, 328–337. doi:10.1111/j.1365-2567.2006.02436.x
Cohen, D., Atsmon, J., Artaud, C., Meron-Sudai, S., Gougeon, M. L., Bialik, A., et al. (2021). Safety and immunogenicity of a synthetic carbohydrate conjugate vaccine against Shigella flexneri 2a in healthy adult volunteers: A phase 1, dose-escalating, single-blind, randomised, placebo-controlled study. Lancet Infect. Dis. 21, 546–558. doi:10.1016/S1473-3099(20)30488-6
Cooper, B., Detora, L., and Stoddard, J. (2011). Menveo®): A novel quadrivalent meningococcal CRM197 conjugate vaccine against serogroups A, C, W-135 and Y. Expert Rev. Vaccines 10, 21–33. doi:10.1586/erv.10.147
Costantino, P., Norelli, F., Giannozzi, A., D'ascenzi, S., Bartoloni, A., Kaur, S., et al. (1999). Size fractionation of bacterial capsular polysaccharides for their use in conjugate vaccines. Vaccine 17, 1251–1263. doi:10.1016/s0264-410x(98)00348-x
Costantino, P., Rappuoli, R., and Berti, F. (2011). The design of semi-synthetic and synthetic glycoconjugate vaccines. Expert Opin. Drug Discov. 6, 1045–1066. doi:10.1517/17460441.2011.609554
Costantino, P., Viti, S., Podda, A., Velmonte, M. A., Nencioni, L., and Rappuoli, R. (1992). Development and phase 1 clinical testing of a conjugate vaccine against meningococcus A and C. Vaccine 10, 691–698. doi:10.1016/0264-410x(92)90091-w
Cox, A. D., Williams, D., Cairns, C., St Michael, F., Fleming, P., Vinogradov, E., et al. (2017). Investigating the candidacy of a capsular polysaccharide-based glycoconjugate as a vaccine to combat Haemophilus influenzae type a disease: A solution for an unmet public health need. Vaccine 35, 6129–6136. doi:10.1016/j.vaccine.2017.09.055
Cuccui, J., and Wren, B. (2015). Hijacking bacterial glycosylation for the production of glycoconjugates, from vaccines to humanised glycoproteins. J. Pharm. Pharmacol. 67, 338–350. doi:10.1111/jphp.12321
Cui, C., Carbis, R., An, S. J., Jang, H., Czerkinsky, C., Szu, S. C., et al. (2010). Physical and chemical characterization and immunologic properties of Salmonella enterica serovar typhi capsular polysaccharide-diphtheria toxoid conjugates. Clin. Vaccine Immunol. 17, 73–79. doi:10.1128/CVI.00266-09
Dagan, R., Kayhty, H., Wuorimaa, T., Yaich, M., Bailleux, F., Zamir, O., et al. (2004). Tolerability and immunogenicity of an eleven valent mixed carrier Streptococcus pneumoniae capsular polysaccharide-diphtheria toxoid or tetanus protein conjugate vaccine in Finnish and Israeli infants. Pediatr. Infect. Dis. J. 23, 91–98. doi:10.1097/01.inf.0000109221.50972.53
Dagan, R., Melamed, R., Zamir, O., and Leroy, O. (1997). Safety and immunogenicity of tetravalent pneumococcal vaccines containing 6B, 14, 19F and 23F polysaccharides conjugated to either tetanus toxoid or diphtheria toxoid in young infants and their boosterability by native polysaccharide antigens. Pediatr. Infect. Dis. J. 16, 1053–1059. doi:10.1097/00006454-199711000-00010
Daum, R. S., Hogerman, D., Rennels, M. B., Bewley, K., Malinoski, F., Rothstein, E., et al. (1997). Infant immunization with pneumococcal CRM197 vaccines: Effect of saccharide size on immunogenicity and interactions with simultaneously administered vaccines. J. Infect. Dis. 176, 445–455. doi:10.1086/514063
De Velasco, E. A., Merkus, D., Anderton, S., Verheul, A. F., Lizzio, E. F., Van Der Zee, R., et al. (1995). Synthetic peptides representing T-cell epitopes act as carriers in pneumococcal polysaccharide conjugate vaccines. Infect. Immun. 63, 961–968. doi:10.1128/IAI.63.3.961-968.1995
Donnelly, J. J., Deck, R. R., and Liu, M. A. (1990). Immunogenicity of a Haemophilus influenzae polysaccharide-Neisseria meningitidis outer membrane protein complex conjugate vaccine. J. Immunol. 145, 3071–3079. doi:10.4049/jimmunol.145.9.3071
Falugi, F., Petracca, R., Mariani, M., Luzzi, E., Mancianti, S., Carinci, V., et al. (2001). Rationally designed strings of promiscuous CD4(+) T cell epitopes provide help to Haemophilus influenzae type b oligosaccharide: A model for new conjugate vaccines. Eur. J. Immunol. 31, 3816–3824. doi:10.1002/1521-4141(200112)31:12<3816::AID-IMMU3816>3.0.CO;2-K
Fattom, A. I., Horwith, G., Fuller, S., Propst, M., and Naso, R. (2004). Development of StaphVAX, a polysaccharide conjugate vaccine against S. aureus infection: From the lab bench to phase III clinical trials. Vaccine 22, 880–887. doi:10.1016/j.vaccine.2003.11.034
Fattom, A., Li, X., Cho, Y. H., Burns, A., Hawwari, A., Shepherd, S. E., et al. (1995). Effect of conjugation methodology, carrier protein, and adjuvants on the immune response to Staphylococcus aureus capsular polysaccharides. Vaccine 13, 1288–1293. doi:10.1016/0264-410x(95)00052-3
Fattom, A., Shiloach, J., Bryla, D., Fitzgerald, D., Pastan, I., Karakawa, W. W., et al. (1992). Comparative immunogenicity of conjugates composed of the Staphylococcus aureus type 8 capsular polysaccharide bound to carrier proteins by adipic acid dihydrazide or N-succinimidyl-3-(2-pyridyldithio)propionate. Infect. Immun. 60, 584–589. doi:10.1128/IAI.60.2.584-589.1992
Floyd, N., Vijayakrishnan, B., Koeppe, J. R., and Davis, B. G. (2009). Thiyl glycosylation of olefinic proteins: S-Linked glycoconjugate synthesis. Angew. Chem. Int. Ed. Engl. 48, 7798–7802. doi:10.1002/anie.200903135
Forsgren, A., Riesbeck, K., and Janson, H. (2008). Protein D of Haemophilus influenzae: A protective nontypeable H. Influenzae antigen and a carrier for pneumococcal conjugate vaccines. Clin. Infect. Dis. 46, 726–731. doi:10.1086/527396
Frasch, C. E. (2009). Preparation of bacterial polysaccharide-protein conjugates: Analytical and manufacturing challenges. Vaccine 27, 6468–6470. doi:10.1016/j.vaccine.2009.06.013
Frasch, C. E., Preziosi, M. P., and Laforce, F. M. (2012). Development of a group A meningococcal conjugate vaccine, MenAfriVac(TM). Hum. Vaccin Immunother. 8, 715–724. doi:10.4161/hv.19619
Goebel, W. F., and Avery, O. T. (1931). Chemo-immunological studies on conjugated carbohydrate-proteins: IV. The synthesis of thep-aminobenzyl ether of the soluble specific substance of type III pneumococcus and its coupling with protein. J. Exp. Med. 54, 431–436. doi:10.1084/jem.54.3.431
Goebel, W. F. (1939). Studies on antibacterial immunity induced by artificial antigens: I. Immunity to experimental pneumococcal infection with an antigen containing cellobiuronic acid. J. Exp. Med. 69, 353–364. doi:10.1084/jem.69.3.353
Goldblatt, D., Vaz, A. R., and Miller, E. (1998). Antibody avidity as a surrogate marker of successful priming by Haemophilus influenzae type b conjugate vaccines following infant immunization. J. Infect. Dis. 177, 1112–1115. doi:10.1086/517407
Granoff, D. M., and Pollard, A. J. (2007). Reconsideration of the use of meningococcal polysaccharide vaccine. Pediatr. Infect. Dis. J. 26, 716–722. doi:10.1097/INF.0b013e3180cc2c25
Guirola, M., Urquiza, D., Alvarez, A., Cannan-Haden, L., Caballero, E., and Guillén, G. (2006). Immunologic memory response induced by a meningococcal serogroup C conjugate vaccine using the P64k recombinant protein as carrier. FEMS Immunol. Med. Microbiol. 46, 169–179. doi:10.1111/j.1574-695X.2005.00025.x
Gupta, R. K., Egan, W., Bryla, D. A., Robbins, J. B., and Szu, S. C. (1995). Comparative immunogenicity of conjugates composed of Escherichia coli O111 O-specific polysaccharide, prepared by treatment with acetic acid or hydrazine, bound to tetanus toxoid by two synthetic schemes. Infect. Immun. 63, 2805–2810. doi:10.1128/IAI.63.8.2805-2810.1995
Gupta, R. K., Szu, S. C., Finkelstein, R. A., and Robbins, J. B. (1992). Synthesis, characterization, and some immunological properties of conjugates composed of the detoxified lipopolysaccharide of Vibrio cholerae O1 serotype Inaba bound to cholera toxin. Infect. Immun. 60, 3201–3208. doi:10.1128/IAI.60.8.3201-3208.1992
Guttormsen, H. K., Sharpe, A. H., Chandraker, A. K., Brigtsen, A. K., Sayegh, M. H., and Kasper, D. L. (1999). Cognate stimulatory B-cell-T-cell interactions are critical for T-cell help recruited by glycoconjugate vaccines. Infect. Immun. 67, 6375–6384. doi:10.1128/IAI.67.12.6375-6384.1999
Guttormsen, H. K., Wetzler, L. M., Finberg, R. W., and Kasper, D. L. (1998). Immunologic memory induced by a glycoconjugate vaccine in a murine adoptive lymphocyte transfer model. Infect. Immun. 66, 2026–2032. doi:10.1128/IAI.66.5.2026-2032.1998
Hancuh, M., Walldorf, J., Minta, A. A., Tevi-Benissan, C., Christian, K. A., Nedelec, Y., et al. (2023). Typhoid fever surveillance, incidence estimates, and progress toward typhoid conjugate vaccine introduction - worldwide, 2018-2022. MMWR Morb. Mortal. Wkly. Rep. 72, 171–176. doi:10.15585/mmwr.mm7207a2
Hatz, C. F., Bally, B., Rohrer, S., Steffen, R., Kramme, S., Siegrist, C. A., et al. (2015). Safety and immunogenicity of a candidate bioconjugate vaccine against Shigella dysenteriae type 1 administered to healthy adults: A single blind, partially randomized phase I study. Vaccine 33, 4594–4601. doi:10.1016/j.vaccine.2015.06.102
Hu, Q. Y., Berti, F., and Adamo, R. (2016). Towards the next generation of biomedicines by site-selective conjugation. Chem. Soc. Rev. 45, 1691–1719. doi:10.1039/c4cs00388h
Huttner, A., Hatz, C., Van Den Dobbelsteen, G., Abbanat, D., Hornacek, A., Frölich, R., et al. (2017). Safety, immunogenicity, and preliminary clinical efficacy of a vaccine against extraintestinal pathogenic Escherichia coli in women with a history of recurrent urinary tract infection: A randomised, single-blind, placebo-controlled phase 1b trial. Lancet Infect. Dis. 17, 528–537. doi:10.1016/S1473-3099(17)30108-1
Ingale, S., Wolfert, M. A., Gaekwad, J., Buskas, T., and Boons, G. J. (2007). Robust immune responses elicited by a fully synthetic three-component vaccine. Nat. Chem. Biol. 3, 663–667. doi:10.1038/nchembio.2007.25
Insel, R. A., and Anderson, P. W. (1986). Oligosaccharide-protein conjugate vaccines induce and prime for oligoclonal IgG antibody responses to the Haemophilus influenzae b capsular polysaccharide in human infants. J. Exp. Med. 163, 262–269. doi:10.1084/jem.163.2.262
Jacques, I., and Dubray, G. (1991). Escherichia hermannii (ATCC 33651) polysaccharide-protein conjugates: Comparison of two conjugation methods for the induction of humoral responses in mice. Vaccine 9, 559–563. doi:10.1016/0264-410x(91)90242-x
Jansen, W. T., Hogenboom, S., Thijssen, M. J., Kamerling, J. P., Vliegenthart, J. F., Verhoef, J., et al. (2001). Synthetic 6B di-tri-and tetrasaccharide-protein conjugates contain pneumococcal type 6A and 6B common and 6B-specific epitopes that elicit protective antibodies in mice. Infect. Immun. 69, 787–793. doi:10.1128/IAI.69.2.787-793.2001
Jansen, W. T., and Snippe, H. (2004). Short-chain oligosaccharide protein conjugates as experimental pneumococcal vaccines. Indian J. Med. Res. 119, 7–12.
John, T. J., and Samuel, R. (2000). Herd immunity and herd effect: New insights and definitions. Eur. J. Epidemiol. 16, 601–606. doi:10.1023/a:1007626510002
Jones, C. (2005). Vaccines based on the cell surface carbohydrates of pathogenic bacteria. Acad Bras Cienc 77, 293–324. doi:10.1590/s0001-37652005000200009
Käyhty, H., Karanko, V., Peltola, H., and Mäkelä, P. H. (1984). Serum antibodies after vaccination with Haemophilus influenzae type b capsular polysaccharide and responses to reimmunization: No evidence of immunologic tolerance or memory. Pediatrics 74, 857–865. doi:10.1542/peds.74.5.857
Kelly, D. F., Snape, M. D., Clutterbuck, E. A., Green, S., Snowden, C., Diggle, L., et al. (2006). CRM197-conjugated serogroup C meningococcal capsular polysaccharide, but not the native polysaccharide, induces persistent antigen-specific memory B cells. Blood 108, 2642–2647. doi:10.1182/blood-2006-01-009282
Kelly, D. F., Snape, M. D., Perrett, K. P., Clutterbuck, E. A., Lewis, S., Blanchard Rohner, G., et al. (2009). Plasma and memory B-cell kinetics in infants following a primary schedule of CRM 197-conjugated serogroup C meningococcal polysaccharide vaccine. Immunology 127, 134–143. doi:10.1111/j.1365-2567.2008.02934.x
Könen-Waisman, S., Cohen, A., Fridkin, M., and Cohen, I. R. (1999). Self heat-shock protein (hsp60) peptide serves in a conjugate vaccine against a lethal pneumococcal infection. J. Infect. Dis. 179, 403–413. doi:10.1086/314590
Kossaczka, Z., Bystricky, S., Bryla, D. A., Shiloach, J., Robbins, J. B., and Szu, S. C. (1997). Synthesis and immunological properties of Vi and di-O-acetyl pectin protein conjugates with adipic acid dihydrazide as the linker. Infect. Immun. 65, 2088–2093. doi:10.1128/IAI.65.6.2088-2093.1997
Kossaczka, Z., Lin, F. Y., Ho, V. A., Thuy, N. T., Van Bay, P., Thanh, T. C., et al. (1999). Safety and immunogenicity of Vi conjugate vaccines for typhoid fever in adults, teenagers, and 2- to 4-year-old children in Vietnam. Infect. Immun. 67, 5806–5810. doi:10.1128/IAI.67.11.5806-5810.1999
Kubler-Kielb, J., and Pozsgay, V. (2005). A new method for conjugation of carbohydrates to proteins using an aminooxy-thiol heterobifunctional linker. J. Org. Chem. 70, 6987–6990. doi:10.1021/jo050934b
Laferrière, C. A., Sood, R. K., De Muys, J. M., Michon, F., and Jennings, H. J. (1997). The synthesis of Streptococcus pneumoniae polysaccharide-tetanus toxoid conjugates and the effect of chain length on immunogenicity. Vaccine 15, 179–186. doi:10.1016/s0264-410x(96)00148-x
Lai, Z., and Schreiber, J. R. (2009). Antigen processing of glycoconjugate vaccines; the polysaccharide portion of the pneumococcal CRM(197) conjugate vaccine co-localizes with MHC II on the antigen processing cell surface. Vaccine 27, 3137–3144. doi:10.1016/j.vaccine.2009.03.064
Lee, C. H., Kuo, W. C., Beri, S., Kapre, S., Joshi, J. S., Bouveret, N., et al. (2009). Preparation and characterization of an immunogenic meningococcal group A conjugate vaccine for use in Africa. Vaccine 27, 726–732. doi:10.1016/j.vaccine.2008.11.065
Lees, A., Nelson, B. L., and Mond, J. J. (1996). Activation of soluble polysaccharides with 1 cyano-4-dimethylaminopyridinium tetrafluoroborate for use in protein-polysaccharide conjugate vaccines and immunological reagents. Vaccine 14, 190–198. doi:10.1016/0264-410x(95)00195-7
Lees, A., Sen, G., and Lopezacosta, A. (2006). Versatile and efficient synthesis of protein-polysaccharide conjugate vaccines using aminooxy reagents and oxime chemistry. Vaccine 24, 716–729. doi:10.1016/j.vaccine.2005.08.096
Lesinski, G. B., and Westerink, M. A. (2001). Vaccines against polysaccharide antigens. Curr. Drug Targets Infect. Disord. 1, 325–334. doi:10.2174/1568005014605964
Lexau, C. A., Lynfield, R., Danila, R., Pilishvili, T., Facklam, R., Farley, M. M., et al. (2005). Changing epidemiology of invasive pneumococcal disease among older adults in the era of pediatric pneumococcal conjugate vaccine. Jama 294, 2043–2051. doi:10.1001/jama.294.16.2043
Lindberg, A. A. (1999). Glycoprotein conjugate vaccines. Vaccine 17 (2), S28–S36. doi:10.1016/s0264-410x(99)00232-7
Lucas, A. H., Apicella, M. A., and Taylor, C. E. (2005). Carbohydrate moieties as vaccine candidates. Clin. Infect. Dis. 41, 705–712. doi:10.1086/432582
Maclennan, J. M., Shackley, F., Heath, P. T., Deeks, J. J., Flamank, C., Herbert, M., et al. (2000). Safety, immunogenicity, and induction of immunologic memory by a serogroup C meningococcal conjugate vaccine in infants: A randomized controlled trial. Jama 283, 2795–2801. doi:10.1001/jama.283.21.2795
Mäkelä, P. H., and Käyhty, H. (2002). Evolution of conjugate vaccines. Expert Rev. Vaccines 1, 399–410. doi:10.1586/14760584.1.3.399
Matjila, M. J., Phohu, T. C., Banzhoff, A., Viviani, S., Hoosen, A. A., Bianchini, M., et al. (2004). Safety and immunogenicity of two Haemophilus influenzae type b conjugate vaccines. S Afr. Med. J. 94, 43–46.
Mawas, F., Niggemann, J., Jones, C., Corbel, M. J., Kamerling, J. P., and Vliegenthart, J. F. (2002). Immunogenicity in a mouse model of a conjugate vaccine made with a synthetic single repeating unit of type 14 pneumococcal polysaccharide coupled to CRM197. Infect. Immun. 70, 5107–5114. doi:10.1128/iai.70.9.5107-5114.2002
Michon, F., Fusco, P. C., Minetti, C. A., Laude-Sharp, M., Uitz, C., Huang, C. H., et al. (1998). Multivalent pneumococcal capsular polysaccharide conjugate vaccines employing genetically detoxified pneumolysin as a carrier protein. Vaccine 16, 1732–1741. doi:10.1016/s0264-410x(98)00225-4
Micoli, F., Adamo, R., and Costantino, P. (2018). Protein carriers for glycoconjugate vaccines: History, selection criteria, characterization and new trends. Molecules 23, 1451. doi:10.3390/molecules23061451
Micoli, F., Alfini, R., Di Benedetto, R., Necchi, F., Schiavo, F., Mancini, F., et al. (2020). GMMA is a versatile platform to design effective multivalent combination vaccines. Vaccines (Basel) 8, 540. doi:10.3390/vaccines8030540
Micoli, F., Del Bino, L., Alfini, R., Carboni, F., Romano, M. R., and Adamo, R. (2019). Glycoconjugate vaccines: Current approaches towards faster vaccine design. Expert Rev. Vaccines 18, 881–895. doi:10.1080/14760584.2019.1657012
Micoli, F., and Maclennan, C. A. (2020). Outer membrane vesicle vaccines. Semin. Immunol. 50, 101433. doi:10.1016/j.smim.2020.101433
Micoli, F., Romano, M. R., Carboni, F., Adamo, R., and Berti, F. (2023). Strengths and weaknesses of pneumococcal conjugate vaccines. Glycoconj J. 40, 135–148. doi:10.1007/s10719-023-10100-3
Micoli, F., Rondini, S., Pisoni, I., Proietti, D., Berti, F., Costantino, P., et al. (2011). Vi-CRM 197 as a new conjugate vaccine against Salmonella Typhi. Vaccine 29, 712–720. doi:10.1016/j.vaccine.2010.11.022
Mitchison, N. A. (2004). T-cell-B-cell cooperation. Nat. Rev. Immunol. 4, 308–312. doi:10.1038/nri1334
Mitchison, N. A. (1971b). The carrier effect in the secondary response to hapten-protein conjugates. II. Cellular cooperation. Eur. J. Immunol. 1, 18–27. doi:10.1002/eji.1830010104
Mitchison, N. A. (1971a). The carrier effect in the secondary response to hapten-protein conjugates. I. Measurement of the effect with transferred cells and objections to the local environment hypothesis. Eur. J. Immunol. 1, 10–17. doi:10.1002/eji.1830010103
Moeller, T. D., Weyant, K. B., and Delisa, M. P. (2021). Interplay of carbohydrate and carrier in antibacterial glycoconjugate vaccines. Adv. Biochem. Eng. Biotechnol. 175, 355–378. doi:10.1007/10_2018_71
Mond, J. J., Lees, A., and Snapper, C. M. (1995). T cell-independent antigens type 2. Annu. Rev. Immunol. 13, 655–692. doi:10.1146/annurev.iy.13.040195.003255
Nilo, A., Allan, M., Brogioni, B., Proietti, D., Cattaneo, V., Crotti, S., et al. (2014). Tyrosine-directed conjugation of large glycans to proteins via copper-free click chemistry. Bioconjug Chem. 25, 2105–2111. doi:10.1021/bc500438h
Nilo, A., Morelli, L., Passalacqua, I., Brogioni, B., Allan, M., Carboni, F., et al. (2015a). Anti-group B Streptococcus glycan-conjugate vaccines using pilus protein GBS80 as carrier and antigen: Comparing lysine and tyrosine-directed conjugation. ACS Chem. Biol. 10, 1737–1746. doi:10.1021/acschembio.5b00247
Nilo, A., Passalacqua, I., Fabbrini, M., Allan, M., Usera, A., Carboni, F., et al. (2015b). Exploring the effect of conjugation site and chemistry on the immunogenicity of an anti-group B Streptococcus glycoconjugate vaccine based on GBS67 pilus protein and type V polysaccharide. Bioconjug Chem. 26, 1839–1849. doi:10.1021/acs.bioconjchem.5b00365
Passwell, J. H., Ashkenazi, S., Banet-Levi, Y., Ramon-Saraf, R., Farzam, N., Lerner-Geva, L., et al. (2010). Age-related efficacy of Shigella O-specific polysaccharide conjugates in 1-4-year-old Israeli children. Vaccine 28, 2231–2235. doi:10.1016/j.vaccine.2009.12.050
Passwell, J. H., Harlev, E., Ashkenazi, S., Chu, C., Miron, D., Ramon, R., et al. (2001). Safety and immunogenicity of improved Shigella O-specific polysaccharide-protein conjugate vaccines in adults in Israel. Infect. Immun. 69, 1351–1357. doi:10.1128/IAI.69.3.1351-1357.2001
Pavliakova, D., Chu, C., Bystricky, S., Tolson, N. W., Shiloach, J., Kaufman, J. B., et al. (1999). Treatment with succinic anhydride improves the immunogenicity of Shigella flexneri type 2a O-specific polysaccharide-protein conjugates in mice. Infect. Immun. 67, 5526–5529. doi:10.1128/IAI.67.10.5526-5529.1999
Peeters, C. C., Evenberg, D., Hoogerhout, P., Käyhty, H., Saarinen, L., Van Boeckel, C. A., et al. (1992). Synthetic trimer and tetramer of 3-beta-D-ribose-(1-1)-D-ribitol-5-phosphate conjugated to protein induce antibody responses to Haemophilus influenzae type b capsular polysaccharide in mice and monkeys. Infect. Immun. 60, 1826–1833. doi:10.1128/IAI.60.5.1826-1833.1992
Peeters, C. C., Lagerman, P. R., De Weers, O., Oomen, L. A., Hoogerhout, P., Beurret, M., et al. (2003). Preparation of polysaccharide-conjugate vaccines. Methods Mol. Med. 87, 153–174. doi:10.1385/1-59259-399-2:153
Peeters, C. C., Tenbergen-Meekes, A. M., Evenberg, D. E., Poolman, J. T., Zegers, B. J., and Rijkers, G. T. (1991). A comparative study of the immunogenicity of pneumococcal type 4 polysaccharide and oligosaccharide tetanus toxoid conjugates in adult mice. J. Immunol. 146, 4308–4314. doi:10.4049/jimmunol.146.12.4308
Peltola, H., Käyhty, H., Virtanen, M., and Mäkelä, P. H. (1984). Prevention of Hemophilus influenzae type b bacteremic infections with the capsular polysaccharide vaccine. N. Engl. J. Med. 310, 1561–1566. doi:10.1056/NEJM198406143102404
Perrett, K. P., Winter, A. P., Kibwana, E., Jin, C., John, T. M., Yu, L. M., et al. (2010). Antibody persistence after serogroup C meningococcal conjugate immunization of United Kingdom primary-school children in 1999-2000 and response to a booster: A phase 4 clinical trial. Clin. Infect. Dis. 50, 1601–1610. doi:10.1086/652765
Peter, C. G. (1998). Responses of children immunized with capsular polysaccharide of hemophilus influenzae type b, by david H. Smith, MD, et al, pediatrics, 1973;52:637-644;and Haemophilus influenzae type b capsular polysaccharide vaccine in children: A double-blind field study of 100 000 vaccinees 3 months to 5 years of age in Finland, by heikki Peltola, MD et al, pediatrics, 1977;60:730-737. Pediatrics 102, 252–254.
Phalipon, A., Tanguy, M., Grandjean, C., Guerreiro, C., Bélot, F., Cohen, D., et al. (2009). A synthetic carbohydrate-protein conjugate vaccine candidate against Shigella flexneri 2a infection. J. Immunol. 182, 2241–2247. doi:10.4049/jimmunol.0803141
Pichichero, M. E. (2009). Booster vaccinations: Can immunologic memory outpace disease pathogenesis? Pediatrics 124, 1633–1641. doi:10.1542/peds.2008-3645
Pichichero, M. E., Porcelli, S., Treanor, J., and Anderson, P. (1998). Serum antibody responses of weanling mice and two-year-old children to pneumococcal-type 6A-protein conjugate vaccines of differing saccharide chain lengths. Vaccine 16, 83–91. doi:10.1016/s0264-410x(97)00146-1
Pichichero, M. E. (2013). Protein carriers of conjugate vaccines: Characteristics, development, and clinical trials. Hum. Vaccin Immunother. 9, 2505–2523. doi:10.4161/hv.26109
Pollard, A. J., Perrett, K. P., and Beverley, P. C. (2009). Maintaining protection against invasive bacteria with protein-polysaccharide conjugate vaccines. Nat. Rev. Immunol. 9, 213–220. doi:10.1038/nri2494
Polonskaya, Z., Deng, S., Sarkar, A., Kain, L., Comellas-Aragones, M., Mckay, C. S., et al. (2017). T cells control the generation of nanomolar-affinity anti-glycan antibodies. J. Clin. Invest. 127, 1491–1504. doi:10.1172/JCI91192
Poolman, J., and Borrow, R. (2011). Hyporesponsiveness and its clinical implications after vaccination with polysaccharide or glycoconjugate vaccines. Expert Rev. Vaccines 10, 307–322. doi:10.1586/erv.11.8
Pozsgay, V., Chu, C., Pannell, L., Wolfe, J., Robbins, J. B., and Schneerson, R. (1999). Protein conjugates of synthetic saccharides elicit higher levels of serum IgG lipopolysaccharide antibodies in mice than do those of the O-specific polysaccharide from Shigella dysenteriae type 1. Proc. Natl. Acad. Sci. U. S. A. 96, 5194–5197. doi:10.1073/pnas.96.9.5194
Pozsgay, V., Kubler-Kielb, J., Schneerson, R., and Robbins, J. B. (2007). Effect of the nonreducing end of Shigella dysenteriae type 1 O-specific oligosaccharides on their immunogenicity as conjugates in mice. Proc. Natl. Acad. Sci. U. S. A. 104, 14478–14482. doi:10.1073/pnas.0706969104
Pozsgay, V. (2000). Oligosaccharide-protein conjugates as vaccine candidates against bacteria. Adv. Carbohydr. Chem. Biochem. 56, 153–199. doi:10.1016/s0065-2318(01)56004-7
Ramsay, M. E., Andrews, N. J., Trotter, C. L., Kaczmarski, E. B., and Miller, E. (2003). Herd immunity from meningococcal serogroup C conjugate vaccination in england: Database analysis. BMJ 326, 365–366. doi:10.1136/bmj.326.7385.365
Rappuoli, R. (2018). Glycoconjugate vaccines: Principles and mechanisms. Sci. Transl. Med. 10, eaat4615. doi:10.1126/scitranslmed.aat4615
Ravenscroft, N., Averani, G., Bartoloni, A., Berti, S., Bigio, M., Carinci, V., et al. (1999). Size determination of bacterial capsular oligosaccharides used to prepare conjugate vaccines. Vaccine 17, 2802–2816. doi:10.1016/s0264-410x(99)00092-4
Ravenscroft, N., and Jones, C. (2000). Glycoconjugate vaccines. Curr. Opin. Drug Discov. Devel 3, 222–231.
Rennels, M. B., Edwards, K. M., Keyserling, H. L., Reisinger, K., Blatter, M. M., Quataert, S. A., et al. (2001). Safety and immunogenicity of four doses of Neisseria meningitidis group C vaccine conjugated to CRM197 in United States infants. Pediatr. Infect. Dis. J. 20, 153–159. doi:10.1097/00006454-200102000-00007
Riddle, M. S., Kaminski, R. W., Di Paolo, C., Porter, C. K., Gutierrez, R. L., Clarkson, K. A., et al. (2016). Safety and immunogenicity of a candidate bioconjugate vaccine against Shigella flexneri 2a administered to healthy adults: A single-blind, randomized phase I study. Clin. Vaccine Immunol. 23, 908–917. doi:10.1128/CVI.00224-16
Romano, M. R., Leuzzi, R., Cappelletti, E., Tontini, M., Nilo, A., Proietti, D., et al. (2014). Recombinant Clostridium difficile toxin fragments as carrier protein for PSII surface polysaccharide preserve their neutralizing activity. Toxins (Basel) 6, 1385–1396. doi:10.3390/toxins6041385
Rondini, S., Micoli, F., Lanzilao, L., Hale, C., Saul, A. J., and Martin, L. B. (2011). Evaluation of the immunogenicity and biological activity of the Citrobacter freundii Vi-CRM197 conjugate as a vaccine for Salmonella enterica serovar Typhi. Clin. Vaccine Immunol. 18, 460–468. doi:10.1128/CVI.00387-10
Saksena, R., Ma, X., Wade, T. K., Kovác, P., and Wade, W. F. (2006). Length of the linker and the interval between immunizations influences the efficacy of Vibrio cholerae O1, Ogawa hexasaccharide neoglycoconjugates. FEMS Immunol. Med. Microbiol. 47, 116–128. doi:10.1111/j.1574-695X.2006.00071.x
Schneerson, R., Barrera, O., Sutton, A., and Robbins, J. B. (1980). Preparation, characterization, and immunogenicity of Haemophilus influenzae type b polysaccharide-protein conjugates. J. Exp. Med. 152, 361–376. doi:10.1084/jem.152.2.361
Schneerson, R., Robbins, J. B., Chu, C., Sutton, A., Vann, W., Vickers, J. C., et al. (1984). Serum antibody responses of juvenile and infant rhesus monkeys injected with Haemophilus influenzae type b and pneumococcus type 6A capsular polysaccharide-protein conjugates. Infect. Immun. 45, 582–591. doi:10.1128/IAI.45.3.582-591.1984
Seppälä, I., and Mäkelä, O. (1989). Antigenicity of dextran-protein conjugates in mice. Effect of molecular weight of the carbohydrate and comparison of two modes of coupling. J. Immunol. 143, 1259–1264. doi:10.4049/jimmunol.143.4.1259
Shafer, D. E., Toll, B., Schuman, R. F., Nelson, B. L., Mond, J. J., and Lees, A. (2000). Activation of soluble polysaccharides with 1-cyano-4-dimethylaminopyridinium tetrafluoroborate (CDAP) for use in protein-polysaccharide conjugate vaccines and immunological reagents. II. Selective crosslinking of proteins to CDAP-activated polysaccharides. Vaccine 18, 1273–1281. doi:10.1016/s0264-410x(99)00370-9
Shen, X., Lagergård, T., Yang, Y., Lindblad, M., Fredriksson, M., and Holmgren, J. (2001). Group B Streptococcus capsular polysaccharide-cholera toxin B subunit conjugate vaccines prepared by different methods for intranasal immunization. Infect. Immun. 69, 297–306. doi:10.1128/IAI.69.1.297-306.2001
Shen, X., Lagergård, T., Yang, Y., Lindblad, M., Fredriksson, M., and Holmgren, J. (2000). Preparation and preclinical evaluation of experimental group B streptococcus type III polysaccharide-cholera toxin B subunit conjugate vaccine for intranasal immunization. Vaccine 19, 850–861. doi:10.1016/s0264-410x(00)00226-7
Siber, G. R., Anderson, R., Habafy, M., and Gupta, R. K. (1995). Development of a Guinea pig model to assess immunogenicity of Haemophilus influenzae type b capsular polysaccharide conjugate vaccines. Vaccine 13, 525–531. doi:10.1016/0264-410x(94)00042-l
Sigurdardottir, S. T., Ingolfsdottir, G., Davidsdottir, K., Gudnason, T., Kjartansson, S., Kristinsson, K. G., et al. (2002). Immune response to octavalent diphtheria- and tetanus-conjugated pneumococcal vaccines is serotype- and carrier-specific: The choice for a mixed carrier vaccine. Pediatr. Infect. Dis. J. 21, 548–554. doi:10.1097/00006454-200206000-00013
Simon, R., Tennant, S. M., Wang, J. Y., Schmidlein, P. J., Lees, A., Ernst, R. K., et al. (2011). Salmonella enterica serovar enteritidis core O polysaccharide conjugated to H:g,m flagellin as a candidate vaccine for protection against invasive infection with S. enteritidis. Infect. Immun. 79, 4240–4249. doi:10.1128/IAI.05484-11
Snape, M. D., Kelly, D. F., Green, B., Moxon, E. R., Borrow, R., and Pollard, A. J. (2005). Lack of serum bactericidal activity in preschool children two years after a single dose of serogroup C meningococcal polysaccharide-protein conjugate vaccine. Pediatr. Infect. Dis. J. 24, 128–131. doi:10.1097/01.inf.0000151029.58752.27
Snapper, C. M., and Mond, J. J. (1996). A model for induction of T cell-independent humoral immunity in response to polysaccharide antigens. J. Immunol. 157, 2229–2233. doi:10.4049/jimmunol.157.6.2229
Stefanetti, G., Hu, Q. Y., Usera, A., Robinson, Z., Allan, M., Singh, A., et al. (2015). Sugar-protein connectivity impacts on the immunogenicity of site-selective Salmonella O-antigen glycoconjugate vaccines. Angew. Chem. Int. Ed. Engl. 54, 13198–13203. doi:10.1002/anie.201506112
Stefanetti, G., Maclennan, C. A., and Micoli, F. (2022). Impact and control of sugar size in glycoconjugate vaccines. Molecules 27, 6432. doi:10.3390/molecules27196432
Stefanetti, G., Rondini, S., Lanzilao, L., Saul, A., Maclennan, C. A., and Micoli, F. (2014). Impact of conjugation chemistry on the immunogenicity of S. Typhimurium conjugate vaccines. Vaccine 32, 6122–6129. doi:10.1016/j.vaccine.2014.08.056
Steinhoff, M. C., Edwards, K., Keyserling, H., Thoms, M. L., Johnson, C., Madore, D., et al. (1994). A randomized comparison of three bivalent Streptococcus pneumoniae glycoprotein conjugate vaccines in young children: Effect of polysaccharide size and linkage characteristics. Pediatr. Infect. Dis. J. 13, 368–372. doi:10.1097/00006454-199405000-00007
Sun, X., Stefanetti, G., Berti, F., and Kasper, D. L. (2019). Polysaccharide structure dictates mechanism of adaptive immune response to glycoconjugate vaccines. Proc. Natl. Acad. Sci. U. S. A. 116, 193–198. doi:10.1073/pnas.1816401115
Svenson, S. B., and Lindberg, A. A. (1981). Artificial Salmonella vaccines: Salmonella typhimurium O-antigen-specific oligosaccharide-protein conjugates elicit protective antibodies in rabbits and mice. Infect. Immun. 32, 490–496. doi:10.1128/IAI.32.2.490-496.1981
Szu, S. C., Stone, A. L., Robbins, J. D., Schneerson, R., and Robbins, J. B. (1987). Vi capsular polysaccharide-protein conjugates for prevention of typhoid fever. Preparation, characterization, and immunogenicity in laboratory animals. J. Exp. Med. 166, 1510–1524. doi:10.1084/jem.166.5.1510
Tangye, S. G., Avery, D. T., Deenick, E. K., and Hodgkin, P. D. (2003). Intrinsic differences in the proliferation of naive and memory human B cells as a mechanism for enhanced secondary immune responses. J. Immunol. 170, 686–694. doi:10.4049/jimmunol.170.2.686
Terra, V. S., Mills, D. C., Yates, L. E., Abouelhadid, S., Cuccui, J., and Wren, B. W. (2012). Recent developments in bacterial protein glycan coupling technology and glycoconjugate vaccine design. J. Med. Microbiol. 61, 919–926. doi:10.1099/jmm.0.039438-0
Thanawastien, A., Cartee, R. T., Griffin, T. J. T., Killeen, K. P., and Mekalanos, J. J. (2015). Conjugate-like immunogens produced as protein capsular matrix vaccines. Proc. Natl. Acad. Sci. U. S. A. 112, E1143–E1151. doi:10.1073/pnas.1425005112
Tontini, M., Berti, F., Romano, M. R., Proietti, D., Zambonelli, C., Bottomley, M. J., et al. (2013). Comparison of CRM197, diphtheria toxoid and tetanus toxoid as protein carriers for meningococcal glycoconjugate vaccines. Vaccine 31, 4827–4833. doi:10.1016/j.vaccine.2013.07.078
Tontini, M., Romano, M. R., Proietti, D., Balducci, E., Micoli, F., Balocchi, C., et al. (2016). Preclinical studies on new proteins as carrier for glycoconjugate vaccines. Vaccine 34, 4235–4242. doi:10.1016/j.vaccine.2016.06.039
Trotter, C. L., Andrews, N. J., Kaczmarski, E. B., Miller, E., and Ramsay, M. E. (2004). Effectiveness of meningococcal serogroup C conjugate vaccine 4 years after introduction. Lancet 364, 365–367. doi:10.1016/S0140-6736(04)16725-1
Trotter, C. L., Mcvernon, J., Ramsay, M. E., Whitney, C. G., Mulholland, E. K., Goldblatt, D., et al. (2008). Optimising the use of conjugate vaccines to prevent disease caused by Haemophilus influenzae type b, Neisseria meningitidis and Streptococcus pneumoniae. Vaccine 26, 4434–4445. doi:10.1016/j.vaccine.2008.05.073
Trück, J., and Pollard, A. J. (2010). Challenges in immunisation against bacterial infection in children. Early Hum. Dev. 86, 695–701. doi:10.1016/j.earlhumdev.2010.08.010
Tsai, T. F., Borrow, R., Gnehm, H. E., Vaudaux, B., Heininger, U., Desgrandchamps, D., et al. (2006). Early appearance of bactericidal antibodies after polysaccharide challenge of toddlers primed with a group C meningococcal conjugate vaccine: What is its role in the maintenance of protection? Clin. Vaccine Immunol. 13, 854–861. doi:10.1128/CVI.00059-06
Valguarnera, E., and Feldman, M. F. (2017). Glycoengineered outer membrane Vesicles as a platform for vaccine development. Meth Enzymol. 597, 285–310. doi:10.1016/bs.mie.2017.06.032
Van De Wijgert, J. H., Verheul, A. F., Snippe, H., Check, I. J., and Hunter, R. L. (1991). Immunogenicity of Streptococcus pneumoniae type 14 capsular polysaccharide: Influence of carriers and adjuvants on isotype distribution. Infect. Immun. 59, 2750–2757. doi:10.1128/IAI.59.8.2750-2757.1991
Van Der Put, R. M., Kim, T. H., Guerreiro, C., Thouron, F., Hoogerhout, P., Sansonetti, P. J., et al. (2016). A synthetic carbohydrate conjugate vaccine candidate against shigellosis: Improved bioconjugation and impact of alum on immunogenicity. Bioconjug Chem. 27, 883–892. doi:10.1021/acs.bioconjchem.5b00617
Wacker, M., Linton, D., Hitchen, P. G., Nita-Lazar, M., Haslam, S. M., North, S. J., et al. (2002). N-linked glycosylation in Campylobacter jejuni and its functional transfer into E. coli. Science 298, 1790–1793. doi:10.1126/science.298.5599.1790
Wacker, M., Wang, L., Kowarik, M., Dowd, M., Lipowsky, G., Faridmoayer, A., et al. (2014). Prevention of Staphylococcus aureus infections by glycoprotein vaccines synthesized in Escherichia coli. J. Infect. Dis. 209, 1551–1561. doi:10.1093/infdis/jit800
Wang, J. Y., Chang, A. H., Guttormsen, H. K., Rosas, A. L., and Kasper, D. L. (2003). Construction of designer glycoconjugate vaccines with size-specific oligosaccharide antigens and site-controlled coupling. Vaccine 21, 1112–1117. doi:10.1016/s0264-410x(02)00625-4
Watson, D. C., Robbins, J. B., and Szu, S. C. (1992). Protection of mice against Salmonella typhimurium with an O-specific polysaccharide-protein conjugate vaccine. Infect. Immun. 60, 4679–4686. doi:10.1128/IAI.60.11.4679-4686.1992
Weintraub, A. (2003). Immunology of bacterial polysaccharide antigens. Carbohydr. Res. 338, 2539–2547. doi:10.1016/j.carres.2003.07.008
Wessels, M. R., Paoletti, L. C., Guttormsen, H. K., Michon, F., D'ambra, A. J., and Kasper, D. L. (1998). Structural properties of group B streptococcal type III polysaccharide conjugate vaccines that influence immunogenicity and efficacy. Infect. Immun. 66, 2186–2192. doi:10.1128/IAI.66.5.2186-2192.1998
Xin, H., Dziadek, S., Bundle, D. R., and Cutler, J. E. (2008). Synthetic glycopeptide vaccines combining beta-mannan and peptide epitopes induce protection against candidiasis. Proc. Natl. Acad. Sci. U. S. A. 105, 13526–13531. doi:10.1073/pnas.0803195105
Zhang, F., Boerth, E. M., Gong, J., Ma, N., Lucas, K., Ledue, O., et al. (2022). A bivalent MAPS vaccine induces protective antibody responses against Salmonella typhi and paratyphi A. Vaccines (Basel) 11, 637
Zhang, F., Lu, Y. J., and Malley, R. (2013). Multiple antigen-presenting system (MAPS) to induce comprehensive B- and T-cell immunity. Proc. Natl. Acad. Sci. U. S. A. 110, 13564–13569. doi:10.1073/pnas.1307228110
Keywords: glycoconjugate, carbohydrate, vaccines, immune response, conjugation variables
Citation: Micoli F, Stefanetti G and MacLennan CA (2023) Exploring the variables influencing the immune response of traditional and innovative glycoconjugate vaccines. Front. Mol. Biosci. 10:1201693. doi: 10.3389/fmolb.2023.1201693
Received: 06 April 2023; Accepted: 28 April 2023;
Published: 16 May 2023.
Edited by:
Marcelo Lima, Keele University, United KingdomReviewed by:
Willie Vann, United States Food and Drug Administration, United StatesPumtiwitt McCarthy, Morgan State University, United States
Copyright © 2023 Micoli, Stefanetti and MacLennan. This is an open-access article distributed under the terms of the Creative Commons Attribution License (CC BY). The use, distribution or reproduction in other forums is permitted, provided the original author(s) and the copyright owner(s) are credited and that the original publication in this journal is cited, in accordance with accepted academic practice. No use, distribution or reproduction is permitted which does not comply with these terms.
*Correspondence: Francesca Micoli, ZnJhbmNlc2NhLngubWljb2xpQGdzay5jb20=