- Niigata Research Laboratory, Mitsubishi Gas Chemical Company, Inc., Niigata, Japan
Obesity is a major health concern worldwide, and its prevalence continues to increase in several countries. Pyrroloquinoline quinone (PQQ) is naturally found in some foods and is available as a dietary supplement in its disodium crystal form. The potential health benefits of PQQ have been studied, considering its antioxidant and anti-inflammatory properties. Furthermore, PQQ has been demonstrated to significantly influence the functions of mitochondria, the organelles responsible for energy production within cells, and their dysfunction is associated with various health conditions, including obesity complications. Here, we explore PQQ properties that can be exploited in obesity treatment and highlight the underlying molecular mechanisms. We review animal and cell culture studies demonstrating that PQQ is beneficial for reducing the accumulation of visceral and hepatic fat. In addition to inhibiting lipogenesis, PQQ can increase mitochondria number and function, leading to improved lipid metabolism. Besides diet-induced obesity, PQQ ameliorates programing obesity of the offspring through maternal supplementation and alters gut microbiota, which reduces obesity risk. In obesity progression, PQQ mitigates mitochondrial dysfunction and obesity-associated inflammation, resulting in the amelioration of the progression of obesity co-morbidities, including non-alcoholic fatty liver disease, chronic kidney disease, and Type 2 diabetes. Overall, PQQ has great potential as an anti-obesity and preventive agent for obesity-related complications. Although human studies are still lacking, further investigations to address obesity and associated disorders are still warranted.
1 Introduction
Pyrroloquinoline-quinone (PQQ) was discovered as a bacterial coenzyme for dehydrogenase (Hauge, 1964), and its structure was later determined by derivatized crystallography (Salisbury et al., 1979). PQQ is also present in small amounts of everyday food, including fruits, vegetables, fermented foods, and breast milk (Kumazawa et al., 1995; Mitchell et al., 1999). PQQ is required for normal growth and maintenance (Killgore et al., 1989). Moreover, PQQ functions as a vitamin (Kasahara and Kato, 2003) and is vital for good health (Jonscher et al., 2021).
Redox reactions are essential to PQQ given their quinone structure. PQQ can be reduced by various substances and converted back to its oxidized state by oxygen. This reaction describes how PQQ functions as the active site of glucose dehydrogenase and can be used as a glucose sensor to manage diabetes (Teymourian et al., 2020). Furthermore, PQQ functions as an antioxidant. Although PQQ in food is in its oxidized form, it is easily reduced by reacting with biological materials. Reduced PQQ scavenges free radicals and reduces oxidative stress, causing cellular damage and contributing to developing chronic diseases (Ouchi et al., 2013). The antioxidant capacity of PQQ contributes to its longevity and anti-inflammatory effects (Sasakura et al., 2017; Yin et al., 2019). Moreover, owing to its ability to increase nerve growth factor production (Yamaguchi et al., 1996) and protect against oxidative damage and encephalitis (Scanlon et al., 1997), PQQ improves cognitive function (Itoh et al., 2016; Tamakoshi et al., 2023).
PQQ can stimulate new mitochondrial growth in cells by increasing peroxisome proliferator-activated receptor-gamma coactivator 1 alpha (PGC-1α) and activating the transcriptional networks (Chowanadisai et al., 2010). Such signaling, often including the AMP-activated protein kinase (AMPK) pathway, is linked to cellular increases in the NAD+/NADH ratio and increased sirtuins expression. In this regard, PQQ improves energy regulation, where genes essential for fatty acid metabolism and mitochondrial function are particularly enhanced (Jonscher et al., 2021). Thus, PQQ could be an alternative food ingredient to prevent obesity. However, human studies on the treatment of obesity with PQQ are lacking.
PQQ was launched as a food ingredient in the United States in 2008. Mitsubishi Gas Chemical Co., Ltd. produced PQQ as pyrroloquinoline quinone disodium (product named BioPQQ®, C14H10N2Na2O11, formula weight: 428.22 amu) by fermentation. PQQ has been certified as a functional food and a new dietary ingredient. In 2014, the Japanese Ministry of Health, Labour, and Welfare approved it as a health food ingredient. In addition, the European Commission approved the product in 2018 as a novel food ingredient named MGCPQQ®, after passing the European Food Safety assessment (Turck et al., 2017). Long-term human experiments with PQQ have not revealed complications, making it food-grade. The product, disodium 9-carboxy-4,5-dioxo-4,5-dihydro-1H-pyrrolo[2,3-f] quinoline-2,7-dicarboxylate, was a crystalline red trihydrate (Ikemoto et al., 2012) distinct from the free form, 4,5-dioxo-4,5-dihydro-1H-pyrrolo[2,3-f] quinoline-2,7,9-tricarboxylic acid. The free form does not exist in nature, and its physical properties are distinct from those of the food ingredient disodium salt (Ikemoto et al., 2017). However, there is no distinction in the use of free-form PQQ and PQQ disodium in most academic literature and in the present review.
Obesity and its related co-morbidities are increasing globally. Hence, finding a therapeutic agent to prevent and treat obesity is crucial. In this review, we specifically focus on the properties of PQQ that can be used in obesity management.
As PQQ supplementation has been studied for myriad potential health benefits (Akagawa et al., 2016), here we described the findings of recent studies on how it ameliorates obesity-related diseases.
2 Factors contributing to obesity development
Obesity is a medical condition and is often the result of a complex interplay among genetics, the environment, developmental programming, and gut microbiome factors. Although numerous factors contribute to obesity, it is eventually caused by an energy imbalance. When more calories are consumed than burned, the excess energy is stored in the body as fat. Excess accumulated body fat causes mitochondrial dysfunction, inflammation, hyperlipidemia, and insulin resistance, leading to various metabolic health problems (Jung et al., 2014). Such conditions contribute to co-morbidities such as Type 2 diabetes, high cholesterol, and cardiovascular disease (Guh et al., 2009; Tsatsoulis and Paschou, 2020). Treating obesity often involves a combination of lifestyle changes, particularly improving diet and increasing physical activity, as well as medical interventions, such as drugs and surgery (Wiechert and Holzapfel, 2021). Here, we propose PQQ as a preventive measure against obesity and its associated health conditions. PQQ reportedly reduces fat accumulation (Mohamad Ishak et al., 2021), and alters lipid metabolism (Qiu et al., 2021; Zhang et al., 2022) and adipokine production (Devasani et al., 2020). Hence, herein, we discuss the effects of PQQ on obesity and its mode of action, primarily based on recent research based on multiple animal and cell culture studies.
3 PQQ reduces body fat accumulation to prevent obesity
Obesity can be evaluated based on body fat content because elevated visceral and hepatic fat levels increase the risk of developing chronic diseases (Thomas et al., 2012). We summarized animal and cell studies on PQQ roles in reducing body fat accumulation in Table 1. These results indicated that PQQ could attenuate body fat, especially visceral and hepatic fat accumulation to prevent dietary obesity (Figure 1A). As obesity progresses with increased fat cell number and size, increasing adipose tissue mass (Sakai et al., 2007), PQQ reduced lipid content and droplet size in mouse adipocytes (Mohamad Ishak et al., 2021). Moreover, in condition to exacerbate obesity and diabetes with benzyl butyl phthalate (BBP) exposure (Zhang et al., 2020), a common endocrine-disrupting chemical (EDC), PQQ normalized the increased liver weight in HFD- and BBP-treated male mice, suggesting that PQQ prevented hepatic fat accumulation. However, female mice showed inconsistent data owing to hormonal changes and reduced EDC susceptibility (Nickelson et al., 2012; Zhang et al., 2022).
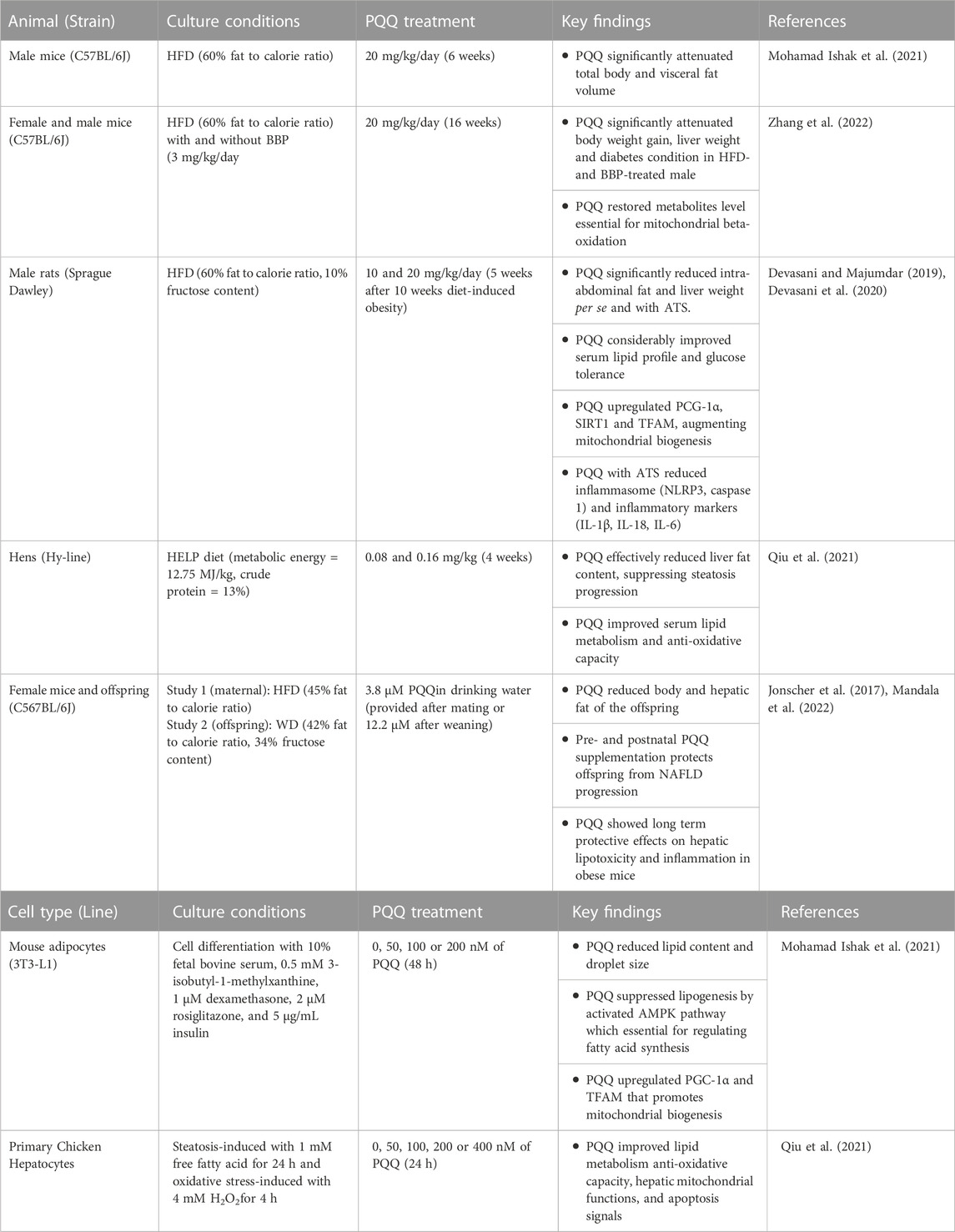
TABLE 1. Summary of animal and cell culture studies and their key findings on PQQ roles associated to obesity.
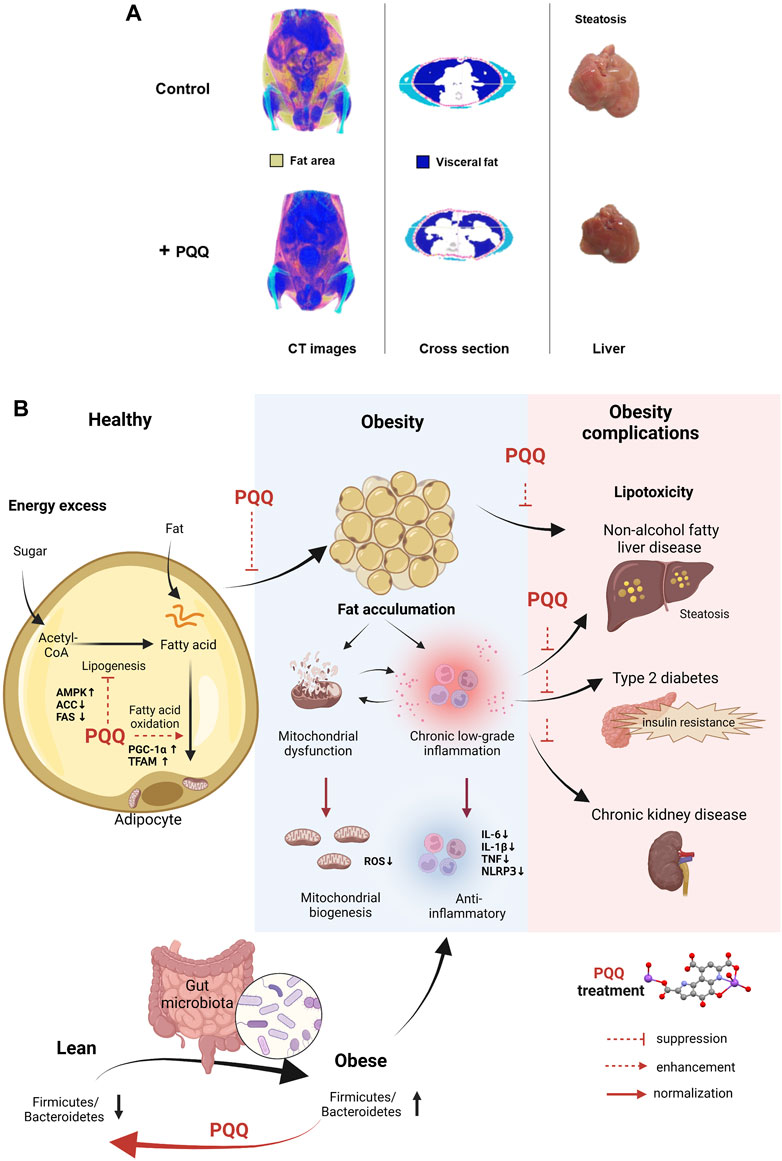
FIGURE 1. PQQ in reducing fat accumulation and ameliorating obesity progression. (A) PQQ reduced visceral and hepatic fats in HFD-induced obese mice. Control mice have accumulated fat, especially visceral fat. The steatosis condition caused by fats building up in the liver was observed in control mice. The images were obtained and edited from our previous study (Mohamad Ishak et al., 2021). (B) PQQ action in reducing fat accumulation and improving obesity conditions. Fat accumulation occurs when excess energy, such as sugar (glucose), is converted into fatty acids via lipogenesis and stored in fat cells (adipose tissue) as triglycerides, leading to obesity. When necessary, fatty acids are metabolized through beta-oxidation (or fatty acid oxidation) in the mitochondria, and energy is produced in the form of adenosine triphosphate. PQQ attenuates fat accumulation by suppressing fatty acid synthesis (lipogenesis) and enhances beta-oxidation by boosting mitochondrial biogenesis. Mitochondrial dysfunction and inflammation occur during obesity progression. Mitochondrial dysfunction causes ROS accumulation and activates inflammatory pathways, releasing pro-inflammatory cytokines and inflammasomes. Inflammation further impairs mitochondrial function, creating a cycle of dysfunction and inflammation. When inflammation becomes chronic, it can lead to tissue damage and contribute to the development of insulin resistance. These events cause various health conditions, including NAFLD, type 2 diabetes, and CKD. With PQQ treatment, the enhancement of mitochondrial biogenesis mitigates mitochondrial dysfunction complications and its anti-inflammatory properties aid in resolving inflammation. Additionally, PQQ improved insulin resistance, and alleviates lipotoxicity in the fetal kidney and liver by decreasing ROS levels. The anti-obesity effects of PQQ have been shown to improve obesity complications. The microbiota Firmicutes/Bacteroidetes (F/B) ratio refers to the ratio of two major phyla of bacteria in the gut microbiome. Studies have shown that an increased F/B ratio is associated with obesity and other metabolic disorders. PQQ reversed F/B ratio in HFD animals and reduced inflammatory markers. The illustration was created with BioRender.com.
Adipose tissue stores excess energy inside fat cells as triglycerides (TG). When necessary, stored TG are broken down and released as free fatty acids outside the cells to provide energy. This process occurs in the mitochondria and involves a series of chemical reactions that break down fatty acids into acetyl-CoA (beta-oxidation). As PQQ is known to enhance mitochondrial function, it attenuated diet-induced fat accumulation primarily by improving beta-oxidation through mitochondrial biogenesis and suppressing lipogenesis (Figure 1B). PGC-1α is a critical factor that stimulates mitochondrial oxidative metabolism and promotes mitochondrial biogenesis (Wu et al., 1999; Puigserver and Spiegelman, 2003). Specific conditions such as caloric restriction and exercise can increase PGC-1α expression (Wu et al., 1999; Russell et al., 2003). PQQ enhances PGC-1α expression, eventually improving mitochondrial biogenesis (Chowanadisai et al., 2010; Saihara et al., 2017), suggesting that PQQ could also stimulate PGC-1α expression similar to exercise or caloric restriction. Recent diet-induced obesity studies also showed that PQQ supplementation enhanced mitochondria biogenesis by regulating PGC-1α, NRF1, and TFAM through the AMPK (adenosine monophosphate-activated protein kinase) signaling pathway (Devasani et al., 2020; Mohamad Ishak et al., 2021; Qiu et al., 2021). Moreover, studies revealed PQQ promoted lipid metabolism as PQQ treatment considerably reduced TG, total cholesterol, and low-density lipoprotein cholesterol levels in the serum lipid profile of diet-induced obese animals (Devasani and Majumdar, 2019) and hens (Qiu et al., 2021)and adipocytes (Mohamad Ishak et al., 2021). Diet-induced obesity in mice lowered acetyl-L-carnitine, propionylcarnitine, and stachydrine levels, metabolites essential for mitochondrial energy production. This condition was restored by PQQ treatment, suggesting beneficial metabolic improvement (Zhang et al., 2022). These results indicate that PQQ improves beta-oxidation and enhances mitochondrial biogenesis, reducing fat accumulation.
PQQ suppresses lipogenesis, through which the body synthesizes new fat molecules from non-fat sources. This process occurs primarily in the liver and adipose tissue and uses acetyl-CoA for fatty acid synthesis, forming TG (Semenkovich and Goldberg, 2020). PQQ treatment activated AMPK, which increased the phosphorylation of acetyl-CoA carboxylase 1 and sterol regulatory element-binding protein 1c in adipocytes (Mohamad Ishak et al., 2021). Such events eventually decreased the lipogenic gene expression and fatty acid synthase, essential for regulating the fatty acid synthesis and suppressing malonyl-CoA conversion to palmitate. The events reduced lipogenesis during fat storage. These findings suggest that PQQ inhibits lipogenesis.
4 PQQ and epigenetic obesity
Maternal obesity during pregnancy is a risk factor for programming obesity in the offspring (Reinehr and Wabitsch, 2011). Programming refers to how early life experiences, including prenatal and early postnatal nutrition, can have long-lasting effects on metabolism, body composition, and health outcomes later in life (Oben et al., 2010; Schack-Nielsen et al., 2010). The impact of maternal PQQ supplementation on offspring was investigated in mice. Pre- and postnatal supplementation of 3.8 µM of PQQ in water given to diet-induced obese mice reduced the body and hepatic fat of the offspring compared to that of the untreated obese offspring (Jonscher et al., 2017; Mandala et al., 2022). Maternal PQQ treatment also elevated beta-oxidation genes in the offspring, indicating improved lipid metabolism that decreased uterine TG accumulation (Mandala et al., 2022). PQQ treatment in mice substantially reduced steatosis induced by a Western diet (42% kcal from fat, 15% protein, 43% carbohydrates, 34%). These results demonstrate that PQQ also works epigenetically to attenuate fat accumulation and combat obesity in offspring. However, due to limited clinical studies, we stress here that PQQ is not yet intended for pregnant and lactating women, or for children, as a food supplement (Turck et al., 2017).
5 PQQ and the gut microbiota
The gut microbiota composition can influence obesity risk and overall health (Liu et al., 2021; Régnier et al., 2021). In mammals, obese and lean individuals have different microbiota compositions, functional genes, and metabolic activities, indicating that the gut microbiota may play a role in these phenotypes (Gérard, 2016). PQQ may benefit gut microbiota by increasing the abundance of certain beneficial bacteria. Supplementation with PQQ improved the gut microbiota composition and reduced inflammation markers associated with obesity in mice (Friedman et al., 2018). PQQ supplementation significantly reversed the diet-induced decrease in Bacteroidetes and increase in Firmicutes observed in the offspring (Figure 1B). Although few studies have specifically examined the impact of PQQ on microbiota composition or function, some gut bacteria may require PQQ for optimal function. However, more research is needed to fully understand the effects of PQQ on gut microbiota and obesity in humans.
6 PQQ mitigates mitochondrial dysfunction and inflammation
Obesity causes various changes in the body and contributes to health problems. Mitochondrial dysfunction and inflammation, implicated in obesity progression, are interconnected processes (de Mello et al., 2018). Existing inflammatory processes generate high levels of reactive oxygen species (ROS), resulting in oxidative stress that causes mitochondrial dysfunction, subsequently leading to decreased energy production and ROS accumulation, exacerbating oxidative damage. Moreover, mitochondrial dysfunction activates inflammatory pathways, producing pro-inflammatory cytokines and chemokines (de Mello et al., 2018). Prolonged inflammation decreases immune defense, a precursor of several diseases (Castro et al., 2017). During obesity, macrophages infiltrate and produce cytokines such as tumor necrosis factor-alpha (TNF-α), interleukin 1 beta (IL-1β), IL-18, IL-6, and interferon-gamma (IFN-γ). Secretion of these molecules, in addition to adipocyte destruction, triggers insulin resistance and metabolic syndrome development (Castro et al., 2017; Saltiel and Olefsky, 2017). Moreover, obesity activates inflammasomes such as NOD-like receptor family pyrin domain-containing 3 (NLRP3), which is involved in diseases such as non-alcoholic fatty liver disease (NAFLD) (Yu et al., 2022), Type 2 diabetes (Gora et al., 2021), and chronic kidney disease (CKD) (Ke et al., 2018)). Hence, maintaining healthy mitochondrial function and reducing inflammation are crucial strategies for promoting overall health and managing various health conditions.
In addition to improving mitochondrial biogenesis, PQQ exhibits anti-inflammatory properties. PQQ acts as an antioxidant by scavenging ROS and inhibiting lipid peroxidation (Miyauchi et al., 1999). PQQ also reduces inflammation by modulating inflammation regulators through NF-kB and MAPK pathways (Kumar and Kar, 2015). By inhibiting NF-κB activation, PQQ decreases IL-6 and TNF-α production (Liu et al., 2016). Consequently, researchers have predicted that PQQ can mitigate obesity-associated inflammation. Indeed, the serum levels of IL-18, IL-1, TNF-α, and IL-6 decreased after 5 weeks PQQ treatment in diet-induced obese rats (Devasani and Majumdar, 2019). Conversely, PQQ decreased NLRP3 inflammasome and caspase 1 expression, suggesting that PQQ managed mitochondrial dysfunction and low-grade inflammation caused by obesity by improving mitochondrial biogenesis (Devasani et al., 2020). In a mouse model study, PQQ supplementation reduced pro-inflammatory cytokine expression, particularly NLRP3, NOS-2, and IL-6. PQQ was more effective on IL-6 than on TNF and IL-1β (Jonscher et al., 2017). These results suggest that the action of PQQ on mitochondrial biogenesis is beneficial for treating mitochondrial dysfunction and inflammation caused by obesity.
7 PQQ and obesity-associated metabolic diseases
AMPK is a key cellular energy regulator that plays a crucial role in maintaining cellular energy homeostasis (Hardie, 2014). Given its impact on AMPK and mitochondrial function described in Section 3, PQQ has considerable potential for treating numerous metabolic diseases including diabetes, NAFLD, and CKD. Moreover, PQQ influences insulin signaling via multiple pathways and increases glucose absorption via glucose transporter translocation. Thus, studies suggest that PQQ may be beneficial in insulin resistance and type 2 diabetes (Akagawa et al., 2016). In a cell culture study, the HK-1 human proximal tubular epithelial cell line modeled the progression of diabetes in the kidney following exposure to high glucose (HG) levels. Diabetes progressing HK-1 cells had an increase in ROS and pro-inflammatory gene expression, and factor erythroid 2-related factor 2 (NFE2L2) and its target inhibition. PQQ supplementation of HG-treated HK-1 cells activated NFE2L2 signaling by increasing NFE2L2 translocation to the nucleus (Wang et al., 2019). PQQ also reduces pro-inflammatory signaling in vivo and in vitro, regulated by the NLRP3 inflammasome (Lin et al., 2020).
PQQ showed protective effects on lipotoxicity and inflammation caused by maternal obesity, and intervention on the risk of NAFLD (Jonscher et al., 2017) and CKD (Zhou et al., 2021). Maternal obesity induced the NLRP3 inflammasome activation, leading to pro-inflammatory factor secretion in the offspring. Maternal supplementation of PQQ alleviates lipotoxicity in the fetal kidney and liver by decreasing ROS levels and inhibiting inflammasome NLRP3 activation. Taken together, PQQ could mitigate the progressive development of obesity-related complications, including diabetes, NAFLD, and CKD. However, further research is needed to fully understand its mechanism of action and potential therapeutic applications in obesity.
8 PQQ with other drugs
Statins are antihyperlipidemic drugs prescribed to those at high risk of cardiovascular disease. Unfortunately, statin therapy is clinically limited for various reasons, including inadequate treatment outcomes (Barry et al., 2018). Furthermore, 20% of people cannot take statins due to intolerance, and 40%–75% of patients discontinue statin treatment within 1–2 years of starting treatment (Toth et al., 2018). Moreover, high statin doses worsen glycemic control in people with diabetes mellitus and increase the chances of developing the disease (Angelidi et al., 2018). The application of PQQ with atorvastatin (ATS) (10 or 20 mg/kg of both molecules) to improve clinical outcomes in obesity and associated low-grade inflammation was investigated. PQQ was expected to counteract the adverse effects of ATS, lessen its deleterious effects, and enhance metabolic results. This study was the first to examine how PQQ affects the ATS treatment results and revealed that combining PQQ with ATS reduced hyperlipidemia and inflammatory reactions and boosted mitochondrial biogenesis (Devasani et al., 2020). Although the positive action of PQQ in alleviating the adverse effects of statins was shown, more research is needed before it can be recommended as a treatment.
9 Related clinical studies
Although animal studies have highlighted PQQ’s potential for treating obesity, no clinical studies have been conducted. However, PQQ has been demonstrated to enhance energy metabolism in humans. When a single dose (0.2 mg/kg) daily for 3 days (0.3 mg/kg) of PQQ was administered to 10 young participants (five males and five females), the levels of trimethylamine N-oxide, a marker of perturbed energy metabolism, decreased following PQQ intake. The ratio of blood lactate to pyruvate and the profile of urinary metabolites were consistent with enhanced mitochondrial oxidation. Additionally, PQQ reduced inflammation indices, C-reactive protein, IL-6 levels, and plasma malondialdehyde levels (Harris et al., 2013). Thus, PQQ positively influences mitochondrial efficiency and attenuates human inflammation.
Another study was conducted on 29 healthy adults with normal-to-moderately high TG levels (110–300 mg/dL) to investigate the effects of PQQ on serum TG and cholesterol levels (Nakano et al., 2015). At an oral dosage of 20 mg/day for 12 weeks, LDL-cholesterol levels substantially decreased in individuals with high LDL-cholesterol (≥140 mg/dL) at baseline compared to the placebo group. However, the serum TG levels did not change. Although the sample size was small, this result regarding the benefits of PQQ for cholesterolemia is promising and warrants further investigation. Investigating the impact of PQQ on obese participants would address some of the limitations.
10 Conclusion
PQQ has emerged as a novel factor that contributes to obesity management. Dietary supplementation with PQQ reduced visceral and hepatic fat accumulation by enhancing mitochondria-related oxidative metabolism and suppressing lipogenesis. Notably, administering PQQ has shown beneficial effects in attenuating clinically relevant dysfunctions, such as mitochondrial dysfunction, inflammation, hyperlipidemia, and lipotoxicity. Evidence from multiple animal lines and cell cultures supports PQQ as a promising therapeutic agent for obesity and its associated complications, such as NAFLD, diabetes, and CKD. However, further research into the anti-obesity potential of PQQ is required. Overall, PQQ may facilitate obesity treatment as research in the field of study progresses.
Author contributions
NI wrote the manuscript and prepared the figures. KI checked and edited the manuscript.
Acknowledgments
We thank Frontiers in Molecular Biosciences for their invitation to publish our manuscript. This opportunity allowed us to deepen and update our knowledge in the obesity research field. We would also like to thank Editage (www.editage.com) for English language editing.
Conflict of interest
NI and KI were employed the Niigata Research Laboratory, Mitsubishi Gas Chemical Company, Inc.
Publisher’s note
All claims expressed in this article are solely those of the authors and do not necessarily represent those of their affiliated organizations, or those of the publisher, the editors and the reviewers. Any product that may be evaluated in this article, or claim that may be made by its manufacturer, is not guaranteed or endorsed by the publisher.
Abbreviations
AMPK, AMP-activated protein kinase; ATS, Atorvastatin; BBP, Benzyl butyl phthalate; CKD, Chronic kidney disease; EDC, Endocrine disrupting chemical; F/B, Firmicutes/Bacteroidetes; HFD, High-fat diet; IL, Interleukin; NAD, Nicotinamide adenine dinucleotide; NADH, Reduced form of nicotinamide adenine dinucleotide; NAFLD, Non-alcohol fatty liver disease; NFE2L2, Factor erythroid 2-related factor 2; NLRP3, NOD-like receptor family pyrin domain containing 3; PGC-1α, Peroxisome proliferator-activated receptor-gamma coactivator 1 alpha; PQQ, Pyrroloquinoline quinone; ROS, Reactive oxygen species; TG, Triglyceride; TNF, Tumor necrosis factor.
References
Akagawa, M., Nakano, M., and Ikemoto, K. (2016). Recent progress in studies on the health benefits of pyrroloquinoline quinone. Biosci. Biotechnol. Biochem. 80, 13–22. doi:10.1080/09168451.2015.1062715
Angelidi, A. M., Stambolliu, E., Adamopoulou, K. I., and Kousoulis, A. A. (2018). Is atorvastatin associated with new onset diabetes or deterioration of glycemic control? Systematic review using data from 1.9 million patients. Int. J. Endocrinol. 2018, 8380192. doi:10.1155/2018/8380192
Barry, A. R., Beach, J. E., and Pearson, G. J. (2018). Prevention and management of statin adverse effects: A practical approach for pharmacists. Can. Pharm. J./Revue des Pharm. du Can. 151, 179–188. doi:10.1177/1715163518768534
Castro, A. M., Macedo-de la Concha, L. E., and Pantoja-Meléndez, C. A. (2017). Low-grade inflammation and its relation to obesity and chronic degenerative diseases. Rev. Medica del Hosp. Gen. Mex. 80, 101–105. doi:10.1016/j.hgmx.2016.06.011
Chowanadisai, W., Bauerly, K. A., Tchaparian, E., Wong, A., Cortopassi, G. A., and Rucker, R. B. (2010). Pyrroloquinoline quinone stimulates mitochondrial biogenesis through cAMP response element-binding protein phosphorylation and increased PGC-1alpha expression. J. Biol. Chem. 285, 142–152. doi:10.1074/jbc.M109.030130
de Mello, A. H., Costa, A. B., Engel, J. D. G., and Rezin, G. T. (2018). Mitochondrial dysfunction in obesity. Life Sci. 192, 26–32. doi:10.1016/j.lfs.2017.11.019
Devasani, K., Kaul, R., and Majumdar, A. (2020). Supplementation of pyrroloquinoline quinone with atorvastatin augments mitochondrial biogenesis and attenuates low grade inflammation in obese rats. Eur. J. Pharmacol. 881, 173273. doi:10.1016/j.ejphar.2020.173273
Devasani, K., and Majumdar, A. (2019). Pyrroloquinoline quinone attenuates obesity associated low grade inflammation. Obes. Med. 16, 100134. doi:10.1016/j.obmed.2019.100134
Friedman, J. E., Dobrinskikh, E., Alfonso-Garcia, A., Fast, A., Janssen, R. C., Soderborg, T. K., et al. (2018). Pyrroloquinoline quinone prevents developmental programming of microbial dysbiosis and macrophage polarization to attenuate liver fibrosis in offspring of obese mice. Hepatol. Commun. 2, 313–328. doi:10.1002/hep4.1139
Gérard, P. (2016). Gut microbiota and obesity. Cell. Mol. Life Sci. 73, 147–162. doi:10.1007/s00018-015-2061-5
Gora, I. M., Ciechanowska, A., and Ladyzynski, P. (2021). NLRP3 inflammasome at the interface of inflammation, endothelial dysfunction, and type 2 diabetes. Cells 10, 314. doi:10.3390/cells10020314
Guh, D. P., Zhang, W., Bansback, N., Amarsi, Z., Birmingham, C. L., and Anis, A. H. (2009). The incidence of co-morbidities related to obesity and overweight: A systematic review and meta-analysis. BMC Public Health 9, 88. doi:10.1186/1471-2458-9-88
Hardie, D. (2014). AMP-Activated protein kinase: A key regulator of energy balance with many roles in human disease. J. Intern. Med. 276, 543–559. doi:10.1111/joim.12268
Harris, C. B., Chowanadisai, W., Mishchuk, D. O., Satre, M. A., Slupsky, C. M., and Rucker, R. B. (2013). Dietary pyrroloquinoline quinone (PQQ) alters indicators of inflammation and mitochondrial-related metabolism in human subjects. J. Nutr. Biochem. 24, 2076–2084. doi:10.1016/j.jnutbio.2013.07.008
Hauge, J. G. (1964). Glucose dehydrogenase of Bacterium anitratum: An enzyme with a novel prosthetic group. J. Biol. Chem. 239, 3630–3639. doi:10.1016/S0021-9258(18)91183-X
Ikemoto, K., Sakamoto, H., and Nakano, M. (2012). Crystal structure and characterization of pyrroloquinoline quinone disodium trihydrate. Chem. Cent. J. 6, 57. doi:10.1186/1752-153X-6-57
Ikemoto, K., Sakamoto, Y., Tanaka, R., Ogata, K., Matsushita, N., and Nakamura, S. (2017). Unusual ionic bond and solubility mechanism of Na<i>n</i>PQQ (n = 0–4) crystals. Cryst. Growth Des. 17, 4118–4123. doi:10.1021/acs.cgd.7b00324
Itoh, Y., Hine, K., Miura, H., Uetake, T., Nakano, M., Takemura, N., et al. (2016). Effect of the antioxidant supplement pyrroloquinoline quinone disodium salt (BioPQQTM) on cognitive functions. Adv. Exp. Med. Biol. 876, 319–325. doi:10.1007/978-1-4939-3023-4_40
Jonscher, K. R., Chowanadisai, W., and Rucker, R. B. (2021). Pyrroloquinoline-quinone is more than an antioxidant: A vitamin-like accessory factor important in health and disease prevention. Biomolecules 11, 1441. doi:10.3390/biom11101441
Jonscher, K. R., Stewart, M. S., Alfonso-Garcia, A., DeFelice, B. C., Wang, X. X., Luo, Y., et al. (2017). Early PQQ supplementation has persistent long-term protective effects on developmental programming of hepatic lipotoxicity and inflammation in obese mice. FASEB J. 31, 1434–1448. doi:10.1096/fj.201600906R
Jung, S., Lee, M.-S., Shin, Y., Kim, C.-T., Kim, I.-H., Kim, Y. S., et al. (2014). A case of thyrotoxic periodic paralysis as initial manifestation of Graves' disease in a 16-year-old Korean adolescent. J. Funct. Foods 10, 169–173. doi:10.6065/apem.2014.19.3.169
Kasahara, T., and Kato, T. (2003). Nutritional biochemistry: A new redox-cofactor vitamin for mammals. Nature 422, 832. doi:10.1038/422832a
Ke, B., Shen, W., Fand, X., and Wu, Q. (2018). The NLPR3 inflammasome and obesity-related kidney disease. J. Cell Mol. Med. 22, 16–24. doi:10.1111/jcmm.13333
Killgore, J., Smidt, C., Duich, L., Romero-Chapman, N., Tinker, D., Reiser, K., et al. (1989). Nutritional importance of pyrroloquinoline quinone. Science 245, 850–852. doi:10.1126/science.2549636
Kumar, N., and Kar, A. (2015). Pyrroloquinoline quinone (PQQ) has potential to ameliorate streptozotocin-induced diabetes mellitus and oxidative stress in mice: A histopathological and biochemical study. Chem. Biol. Interact. 240, 278–290. doi:10.1016/j.cbi.2015.08.027
Kumazawa, T., Sato, K., Seno, H., Ishii, A., and Suzuki, O. (1995). Levels of pyrroloquinoline quinone in various foods. Biochem. J. 307, 331–333. doi:10.1042/bj3070331
Lin, X., Yang, F., Huang, J., Jiang, S., Tang, Y., and Li, J. (2020). Ameliorate effect of pyrroloquinoline quinone against cyclophosphamide-induced nephrotoxicity by activating the Nrf2 pathway and inhibiting the NLRP3 pathway. Life Sci. 256, 117901. doi:10.1016/j.lfs.2020.117901
Liu, B.-N., Liu, X.-T., Liang, Z.-H., and Wang, J.-H. (2021). Gut microbiota in obesity. World J. Gastroenterol. 27, 3837–3850. doi:10.3748/wjg.v27.i25.3837
Liu, Z., Sun, C., Tao, R., Xu, X., Xu, L., Cheng, H., et al. (2016). Pyrroloquinoline quinone decelerates rheumatoid arthritis progression by inhibiting inflammatory responses and joint destruction via modulating NF-κB and MAPK pathways. Inflammation 39, 248–256. doi:10.1007/s10753-015-0245-7
Mandala, A., Dobrinskikh, E., Janssen, R. C., Fiehn, O., D’Alessandro, A., Friedman, J. E., et al. (2022). Maternal pyrroloquinoline quinone supplementation improves offspring liver bioactive lipid profiles throughout the lifespan and protects against the development of adult NAFLD. Int. J. Mol. Sci. 23, 6043. doi:10.3390/ijms23116043
Mitchell, A. E., Jones, A. D., Mercer, R. S., and Rucker, R. B. (1999). Characterization of pyrroloquinoline quinone amino acid derivatives by electrospray ionization mass spectrometry and detection in human milk. Anal. Biochem. 269, 317–325. doi:10.1006/abio.1999.4039
Miyauchi, K., Urakami, T., Abeta, H., Shi, H., Noguchi, N., and Niki, E. (1999). Action of pyrroloquinolinequinol as an antioxidant against lipid peroxidation in solution. Antioxid. Redox Signal 1, 547–554. doi:10.1089/ars.1999.1.4-547
Mohamad Ishak, N. S., Ikemoto, K., Kikuchi, M., Ogawa, M., Akutagawa, K., and Akagawa, M. (2021). Pyrroloquinoline quinone attenuates fat accumulation in obese mice fed with a high-fat diet, Daphnia magna supplied with a high amount of food, and 3T3-L1 adipocytes. ACS Food Sci. Technol. 1, 1979–1989. doi:10.1021/acsfoodscitech.1c00301
Nakano, M., Kawasaki, Y., Suzuki, N., and Takara, T. (2015). Effects of pyrroloquinoline quinone disodium salt intake on the serum cholesterol levels of healthy Japanese adults. J. Nutr. Sci. Vitaminol. (Tokyo) 61, 233–240. doi:10.3177/jnsv.61.233
Nickelson, K. J., Stromsdorfer, K. L., Pickering, R. T., Liu, T.-W., Ortinau, L. C., Keating, A. F., et al. (2012). A comparison of inflammatory and oxidative stress markers in adipose tissue from weight-matched obese male and female mice. Exp. Diabetes Res. 2012, 859395. –8. doi:10.1155/2012/859395
Oben, J. A., Mouralidarane, A., Samuelsson, A.-M., Matthews, P. J., Morgan, M. L., Mckee, C., et al. (2010). Maternal obesity during pregnancy and lactation programs the development of offspring non-alcoholic fatty liver disease in mice. J. Hepatol. 52, 913–920. doi:10.1016/j.jhep.2009.12.042
Ouchi, A., Ikemoto, K., Nakano, M., Nagaoka, S., and Mukai, K. (2013). Kinetic study of aroxyl radical scavenging and α-tocopheroxyl regeneration rates of pyrroloquinolinequinol (PQQH2, a reduced form of pyrroloquinolinequinone) in dimethyl sulfoxide solution: Finding of synergistic effect on the reaction rate due to the coexistence of α-tocopherol and PQQH2. J. Agric. Food Chem. 61, 11048–11060. doi:10.1021/jf4040496
Puigserver, P., and Spiegelman, B. M. (2003). Peroxisome proliferator-activated receptor-gamma coactivator 1 alpha (PGC-1 alpha): Transcriptional coactivator and metabolic regulator. Endocr. Rev. 24, 78–90. doi:10.1210/er.2002-0012
Qiu, K., Zhao, Q., Wang, J., Qi, G. H., Wu, S. G., and Zhang, H. J. (2021). Effects of pyrroloquinoline quinone on lipid metabolism and anti-oxidative capacity in a high-fat-diet metabolic dysfunction-associated fatty liver disease chick model. Int. J. Mol. Sci. 22, 1458–1517. doi:10.3390/ijms22031458
Régnier, M., Van Hul, M., Knauf, C., and Cani, P. D. (2021). Gut microbiome, endocrine control of gut barrier function and metabolic diseases. J. Endocrinol. 248, R67–R82. doi:10.1530/JOE-20-0473
Reinehr, T., and Wabitsch, M. (2011). Childhood obesity. Curr. Opin. Lipidol. 22, 21–25. doi:10.1097/MOL.0b013e32833f9c37
Russell, A. P., Feilchenfeldt, J., Schreiber, S., Praz, M., Crettenand, A., Gobelet, C., et al. (2003). Endurance training in humans leads to fiber type-specific increases in levels of peroxisome proliferator-activated receptor-gamma coactivator-1 and peroxisome proliferator-activated receptor-alpha in skeletal muscle. Diabetes 52, 2874–2881. doi:10.2337/diabetes.52.12.2874
Saihara, K., Kamikubo, R., Ikemoto, K., Uchida, K., and Akagawa, M. (2017). Pyrroloquinoline quinone, a redox-active o-quinone, stimulates mitochondrial biogenesis by activating the SIRT1/PGC-1α signaling pathway. Biochemistry 56, 6615–6625. doi:10.1021/acs.biochem.7b01185
Sakai, T., Sakaue, H., Nakamura, T., Okada, M., Matsuki, Y., Watanabe, E., et al. (2007). Skp2 controls adipocyte proliferation during the development of obesity. J. Biol. Chem. 282, 2038–2046. doi:10.1074/jbc.M608144200
Salisbury, S. A., Forrest, H. S., Cruse, W. B. T., and Kennard, O. (1979). A novel coenzyme from bacterial primary alcohol dehydrogenases. Nature 280, 843–844. doi:10.1038/280843a0
Saltiel, A. R., and Olefsky, J. M. (2017). Inflammatory mechanisms linking obesity and metabolic disease. J. Clin. Investig. 127, 1–4. doi:10.1172/JCI92035
Sasakura, H., Moribe, H., Nakano, M., Ikemoto, K., Takeuchi, K., and Mori, I. (2017). Lifespan extension by peroxidase/dual oxidase-mediated ROS signaling through pyrroloquinoline quinone in C. elegans. J. Cell Sci. 130 (15), 2631–2643. doi:10.1242/jcs.202119
Scanlon, J. M., Aizenman, E., and Reynolds, I. J. (1997). Effects of pyrroloquinoline quinone on glutamate-induced production of reactive oxygen species in neurons. Eur. J. Pharmacol. 326, 67–74. doi:10.1016/S0014-2999(97)00137-4
Schack-Nielsen, L., Michaelsen, K. F., Gamborg, M., Mortensen, E. L., and Sørensen, T. I. A. (2010). Gestational weight gain in relation to offspring body mass index and obesity from infancy through adulthood. Int. J. Obes. 34, 67–74. doi:10.1038/ijo.2009.206
Semenkovich, C., and Goldberg, I. (2020). “Disorder of lipid metabolism,” in Williams textbook of endocrinology (Amsterdam: Elsevier), 1581–1620.
Tamakoshi, M., Suzuki, T., Nishihara, E., Nakamura, S., and Ikemoto, K. (2023). Pyrroloquinoline quinone disodium salt improves brain function in both younger and older adults. Food Funct. 14, 2496–2501. doi:10.1039/D2FO01515C
Teymourian, H., Barfidokht, A., and Wang, J. (2020). Electrochemical glucose sensors in diabetes management: An updated review (2010–2020). Chem. Soc. Rev. 49, 7671–7709. doi:10.1039/D0CS00304B
Thomas, E. L., Frost, G., Taylor-Robinson, S. D., and Bell, J. D. (2012). Excess body fat in obese and normal-weight subjects. Nutr. Res. Rev. 25, 150–161. doi:10.1017/S0954422412000054
Toth, P. P., Patti, A. M., Giglio, R. V., Nikolic, D., Castellino, G., Rizzo, M., et al. (2018). Management of statin intolerance in 2018: Still more questions than answers. Am. J. Cardiovasc. Drugs 18, 157–173. doi:10.1007/s40256-017-0259-7
Tsatsoulis, A., and Paschou, S. A. (2020). Metabolically healthy obesity: Criteria, epidemiology, controversies, and consequences. Curr. Obes. Rep. 9, 109–120. doi:10.1007/s13679-020-00375-0
Turck, D., Bresson, J., Burlingame, B., Dean, T., Fairweather-Tait, S., Heinonen, M., et al. (2017). Safety of pyrroloquinoline quinone disodium salt as a novel food pursuant to regulation (EC) No 258/97. EFSA J. 15, e05058. doi:10.2903/j.efsa.2017.5058
Wang, Z., Han, N., Zhao, K., Li, Y., Chi, Y., and Wang, B. (2019). Protective effects of pyrroloquinoline quinine against oxidative stress-induced cellular senescence and inflammation in human renal tubular epithelial cells via Keap1/Nrf2 signaling pathway. Int. Immunopharmacol. 72, 445–453. doi:10.1016/j.intimp.2019.04.040
Wiechert, M., and Holzapfel, C. (2021). Nutrition concepts for the treatment of obesity in adults. Nutrients 14, 169. doi:10.3390/nu14010169
Wu, Z., Puigserver, P., Andersson, U., Zhang, C., Adelmant, G., Mootha, V., et al. (1999). Mechanisms controlling mitochondrial biogenesis and respiration through the thermogenic coactivator PGC-1. Cell 98, 115–124. doi:10.1016/S0092-8674(00)80611-X
Yamaguchi, K., Tsuji, T., Uemura, D., and Kondo, K. (1996). Cyclooxygenase induction is essential for NGF synthesis enhancement by NGF inducers in L-M cells. Biosci. Biotechnol. Biochem. 60, 92–94. doi:10.1271/bbb.60.92
Yin, X., Ming, D., Bai, L., Wu, F., Liu, H., Chen, Y., et al. (2019). Effects of pyrroloquinoline quinone supplementation on growth performance and small intestine characteristics in weaned pigs. J. Anim. Sci. 97, 246–256. doi:10.1093/jas/sky387
Yu, L., Hong, W., Lu, S., Li, Y., Guan, Y., Weng, X., et al. (2022). The NLRP3 inflammasome in non-alcoholic fatty liver disease and steatohepatitis: Therapeutic targets and treatment. Front. Pharmacol. 13, 780496. doi:10.3389/fphar.2022.780496
Zhang, J., Powell, C. A., Kay, M. K., Park, M. H., Meruvu, S., Sonkar, R., et al. (2020). A moderate physiological dose of benzyl butyl phthalate exacerbates the high fat diet-induced diabesity in male mice. Toxicol. Res. (Camb) 9, 353–370. doi:10.1093/toxres/tfaa037
Zhang, J., Powell, C., Meruvu, S., Sonkar, R., and Choudhury, M. (2022). Pyrroloquinoline quinone attenuated benzyl butyl phthalate induced metabolic aberration and a hepatic metabolomic analysis. Biochem. Pharmacol. 197, 114883. doi:10.1016/j.bcp.2021.114883
Keywords: PQQ, pyrroloquinoline quinone, fat, obesity, lipogenesis, mitochondria, inflammation, metabolic syndrome
Citation: Mohamad Ishak NS and Ikemoto K (2023) Pyrroloquinoline-quinone to reduce fat accumulation and ameliorate obesity progression. Front. Mol. Biosci. 10:1200025. doi: 10.3389/fmolb.2023.1200025
Received: 04 April 2023; Accepted: 24 April 2023;
Published: 05 May 2023.
Edited by:
Julio Plaza-Diaz, Children’s Hospital of Eastern Ontario (CHEO), CanadaReviewed by:
Vijay Karkal Hegde, Texas Tech University, United StatesDan Gao, Xi’an Jiaotong University Health Science Center, China
Xiujing Feng, Shandong First Medical University, Jinan, China
Copyright © 2023 Mohamad Ishak and Ikemoto. This is an open-access article distributed under the terms of the Creative Commons Attribution License (CC BY). The use, distribution or reproduction in other forums is permitted, provided the original author(s) and the copyright owner(s) are credited and that the original publication in this journal is cited, in accordance with accepted academic practice. No use, distribution or reproduction is permitted which does not comply with these terms.
*Correspondence: Kazuto Ikemoto, kazuto-ikemoto@mgc.co.jp
†ORCID: Nur Syafiqah Mohamad Ishak, orcid.org/0000-0002-7818-428X; Kazuto Ikemoto, orcid.org/0000-0002-5708-1636