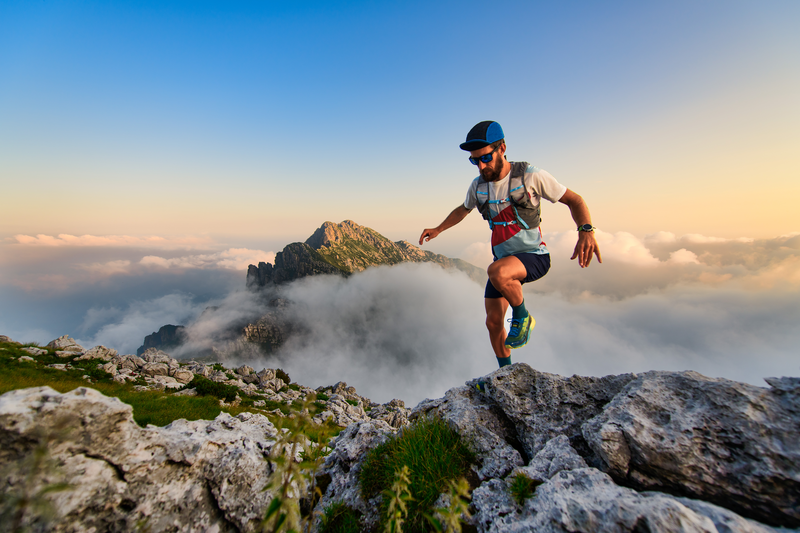
95% of researchers rate our articles as excellent or good
Learn more about the work of our research integrity team to safeguard the quality of each article we publish.
Find out more
MINI REVIEW article
Front. Mol. Biosci. , 09 May 2023
Sec. Structural Biology
Volume 10 - 2023 | https://doi.org/10.3389/fmolb.2023.1195010
This article is part of the Research Topic Functions, Working Mechanisms, and Regulation of Rotary ATPases and Ductin Proteins View all 9 articles
Ductins are a family of homologous and structurally similar membrane proteins with 2 or 4 trans-membrane alpha-helices. The active forms of the Ductins are membranous ring- or star-shaped oligomeric assemblies and they provide various pore, channel, gap-junction functions, assist in membrane fusion processes and also serve as the rotor c-ring domain of V-and F-ATPases. All functions of the Ductins have been reported to be sensitive to the presence of certain divalent metal cations (Me2+), most frequently Cu2+ or Ca2+ ions, for most of the better known members of the family, and the mechanism of this effect is not yet known. Given that we have earlier found a prominent Me2+ binding site in a well-characterised Ductin protein, we hypothesise that certain divalent cations can structurally modulate the various functions of Ductin assemblies via affecting their stability by reversible non-covalent binding to them. A fine control of the stability of the assembly ranging from separated monomers through a loosely/weakly to tightly/strongly assembled ring might render precise regulation of Ductin functions possible. The putative role of direct binding of Me2+ to the c-ring subunit of active ATP hydrolase in autophagy and the mechanism of Ca2+-dependent formation of the mitochondrial permeability transition pore are also discussed.
In the ‘80s a 16-kDa protein was purified from the presynaptic plasma membranes of the electric organ of Torpedo marmorata. The protein was called “mediatophore” because it was shown to mediate membrane translocation of acetylcholine (ACh) in a calcium-dependent manner. The active form of the mediatophore was an oligomeric ring, not linked by disulphide bonds but it required certain native lipids for function (Israël et al., 1986; Israël et al., 1988). It showed high sequence homology with the bovine chromaffin granule protonophore and subunits of the proteolipid c-ring of the yeast vacuolar proton-ATPase (V-ATPase) (Birman et al., 1990). It was also demonstrated that calcium-induced occurrence of intramembranous particles was conditional to ACh release from proteoliposomes equipped with mediatophore (Brochier et al., 1992). Although N,N’-dicyclohexylcarbodiimide (DCCD, a proton-translocation blocker of V-ATPase, which targets carboxyl groups within the membrane) was shown to bind to mediatophore, it still had the ability of calcium-dependent ACh translocation, suggesting that different protein domains were involved in ACh and proton transport functions (Sbia et al., 1992). Calcium dependence of mediatophore assembly and function seems well supported (Israël et al., 1993; Morel and Israël, 2000; Malo and Israël, 2003; Dunant et al., 2009; Fujii et al., 2012). However, recent studies challenge the original c-ring fusion pore model about the role of V-ATPase in membrane fusion processes (Poea-Guyon et al., 2013; Bodzęta et al., 2017), suggesting a more complex scenario. Gap-junction proteins from mouse liver plasma membrane and the hepatopancreas of Nephrops norvegicus (arthropod) showed both high sequence and structural similarity to the above proteins, and their different relative orientation relative to the cytoplasm was observed (Finbow and Meagher, 1992; Finbow et al., 1993). In addition, the same proteolipid was found to be a constituent of both the ACh releasing mediatophore and the V-ATPase in Torpedo (Brochier and Morel, 1993), indicating more than one function of some of the related proteins. Based on microscopic and spectroscopic data we have provided evidence for a common structure for a class of membrane channels, that we named Ductins: The gap-junction-like structures isolated from Nephrops norvegicus were composed of a 16-kDa polypeptide, and the functional assembly was a star-shaped hexamer of the protein, of a four trans-membrane alpha helix (TMH) per monomer topology, arranged around a central channel (Holzenburg et al., 1993) [see left drawing in Figure 1 for an illustration, based on (Pali et al., 1995; Pali et al., 1997; Harrison et al., 1999; Pali et al., 1999)]. It was also shown that the Nephrops 16-kDa protein could substitute for the subunit c of V-ATPase in yeast yielding a functional hybrid enzyme (Holzenburg et al., 1993; Finbow et al., 1994). It had been long disputed but now it is established that subunit c (a Ductin protein) and other subunits of the Vo domain of V-ATPase play direct roles in some vesicle transport processes by facilitating membrane fusion, via intra- and inter-membrane subunit rearrangement and interaction with other fusion proteins, unrelated to the acidification role of V-ATPase (Galli et al., 1996; Shiff et al., 1996; Israël and Dunant, 1999; Di et al., 2010; El Far and Seagar, 2011; Strasser et al., 2011; Amendola et al., 2015; Couoh-Cardel et al., 2016; Rama et al., 2019).
FIGURE 1. An illustration of the top view of the hexameric ring assembly (left) and separated monomers (right) of 16-kDa, 4 trans-membrane alpha-helix (TMH) Ductin proteins in a membrane. The central pore, which can only form in the ring assembly, is shown with an outlined blue circle (left). The numbers indicate the TMHs of the monomers in the sequential order. Lipids are shown as green circles, and the first shell or annular lipids contacting the protein surface are shown in darker green. According to the hypothesis of this paper, binding of specific divalent cations shifts the equilibrium towards the stable hexameric ring assembly (left) needed for both the pore, gap-junction and rotor functions of these Ductin proteins. However, inter- and intra-membrane rearrangement, which is needed for putative membrane fusion-related functions, is only possible in a loosely assembled or fully disassembled state, assuming low concentration of the divalent cations.
Intracellular and extracellular material destined for degradation are transported along the autophagy, endocytic, and phagocytic pathways, respectively. Their shared endpoint is the many lysosomes (in animal cells) and usually a single vacuole (in plants and fungi), where acidic hydrolases break down proteins, lipids, nucleic acids, and carbohydrates into building blocks for subsequent recycling and reuse in the cytosol. Lysosomal/vacuolar hydrolases function optimally in an acidic internal milieu (pH: 4.5-5.0), which is generated and maintained by the V-ATPase complex. Considering the fundamental, homeostatic role of autophagy and endocytosis both at the cellular and organismal levels, the most important function of V-ATPase is to promote these vesicle-mediated degradation pathways, but very little is known about the regulation of V-ATPase during these scenarios. Although the non-ubiquitously expressed V-ATPase isoform ATP6V0D2/subunit d2 was found to bind to Syntaxin 17, the autophagosomal SNARE that others and we have identified as a key competence factor enabling lysosomal fusion (Itakura et al., 2012; Takáts et al., 2013), this Syntaxin 17-binding ATP6V0D2/subunit d2 was found to be dispensable for lysosome acidification as it promotes autophagosome-lysosomal fusion (Xia et al., 2019). On the other hand since, at least in the above referred context, a physical and functional interaction of Syntaxin 17 and V-ATPase is firmly established and because ATPV0D is closely situated to the c-ring of the Vo subunit, interaction of the latter with Ca2+ may have a structural impact providing a mean for regulation. Unlike Mg2+, which supports both ATP hydrolysis and coupled vectorial proton transport of the purified holoenzyme, Ca2+ has been shown to facilitate only its ATPase activity. However, Ca2+ supports a coupled reaction having given that the holoenzyme is membrane embedded and there is a favourable membrane potential difference. There is no solid explanation for these findings, but Ca2+ binding to the holoenzyme most likely have a structural impact on the V-ATPase, and may serve thereby as a mean for quality control to support the coupled reaction only if the holoenzyme is properly positioned into a membrane, a regulatory role could not be exerted by Mg2+. This putative mechanism may act on some of the Vo subunits such as Voc (a Ductin, c-ring protein), as it is the part of the complex to interact with the internal side of the membrane and may be affected by membrane potential. We found that the V-ATPase complex itself is dispensable for autophagosome-lysosome fusion in Drosophila fat cells (even though its loss inhibits lysosome acidification), and interestingly, the vesicle fusion blocking effect of the commonly used V-ATPase inhibitor bafilomycin A1 could be attributed to Ca2+ dyshomeostasis within cells (Mauvezin et al., 2015). Thus, these data leave the question still open whether Me2+ (or Ca2+ in particular) have any direct effect on the V-ATPase in autophagy.
The Ductin family also includes the c-ring protein subunit (c) of the ATP synthase (F-ATPase). A 16-kDa Ductin protein (such as, e.g., the 4TM Voc subunit) is basically a tandem repeat of the 8-kDa subunit c of F-ATPase (with 2 TMHs) based on sequence and structure similarity between subunit c of F-ATPase and the Nephrops and other 16-kDa Ductin proteins from different species (Holzenburg et al., 1993). V-ATPase works in the opposite sense as the better known F-ATP synthase, which normally synthesises ATP on the cost of trans-membrane delta pH (Borsch, 2013; Junge and Nelson, 2015; Nakanishi et al., 2019; Noji et al., 2020). F- and V-ATPases are true molecular motors, and the catalytic process (ATP hydrolysis or synthesis) and proton transport are strongly coupled via the rotary mechanism in both enzymes (Rawson et al., 2015; Sugawa et al., 2016; Yamato et al., 2016; Ferencz et al., 2017; Yanagisawa and Frasch, 2017; Kühlbrandt, 2019; Murphy et al., 2019; Kubo et al., 2020; Noji et al., 2020; Pinke et al., 2020; Frasch et al., 2022; Kishikawa et al., 2022). Mitochondria tightly regulate the permeability of their inner membrane to maintain efficient ATP synthesis. Stress events lead to dys-regulation of cellular [Ca2+], which causes loss of the inner membrane potential, and the process results in non-specific permeability transition pores, metabolic dysfunction and ultimately cell death (Carraro and Bernardi, 2016; Giorgio et al., 2018; Nesci, 2020). It is now established that if mitochondrial F-ATPase becomes a molecular target of Ca2+ it is one of the key events in the formation of the mitochondrial permeability transition pore (mPTP) (Nath, 2020; Algieri et al., 2021; Nesci, 2022a; Nesci, 2022b). The process of mPTP formation is complex and probably involves dissociation of F-ATPase dimers, then the F1-Fo domains, and it depends on many factors (Amodeo et al., 2017; Bonora et al., 2017; Mnatsakanyan and Jonas, 2020; Nath, 2020). Nevertheless, F-ATPase seems to be able to accommodate all the key factors (including Ca2+, Mg2+, adenine nucleotides, membrane potential, matrix pH, SH oxidants and reductants, etc.) that regulate mPTP activity (Giorgio et al., 2018). Several putative Ca2+ binding locations on the F-ATPase and related effects had been proposed (Giorgio et al., 2017; Mnatsakanyan and Jonas, 2020; Nath, 2020), but the [Ca2+]-dependent c-ring assembly appears to be a key step in the actual pore formation (Alavian et al., 2014; Halestrap, 2014; Bonora et al., 2017; Neginskaya et al., 2019; Mnatsakanyan and Jonas, 2020; Amodeo et al., 2021; Nesci, 2022a). (This c-ring pore assembly is somewhat similar to the gap-junctional arrangement of the V-ATPase Vo subunit c homologue of lobster hepatopancreas (Holzenburg et al., 1993; Pali et al., 1995).)
Ductins can be defined as a family of homologous and structurally similar membrane proteins with 2 or 4 trans-membrane alpha-helices whose active forms are membranous ring- or star-shaped oligomeric assemblies and they provide various pore, channel, gap-junction functions, assist membrane fusion processes and also serve as the rotor c-ring domain of F- and V-ATPases. The multifunctional character puts the Ductins at the crossroads of a number of key biological processes (Dunlop et al., 1995; Finbow et al., 1995; Lautemann and Bohrmann, 2016). In a series of studies, we have characterised the 16-kDa lobster gap-junction protein as concern its membranous hexameric assembly, interaction with lipids and inhibitors, and the membrane location of some key residues (Jones et al., 1995; Pali et al., 1995; Pali et al., 1997; Pali et al., 1999; Pali et al., 2004). Most importantly, we have also shown that, as purified from the hepatopancreas of Nephrops norvegicus, the stable c-ring form of this Ductin protein contained a Me2+ binding site that was occupied by Cu2+, that could be removed by washing with EDTA. Titration with NiCl2 then yielded Ni2+ bound to the exchangeable Me2+ site (Pali et al., 2006). This site was found to be situated closer to Cys54 (of TMH2) and the C5 position of the lipid chains than to the C9-C14 fatty acid chain positions, possibly in the outer ring of TMHs. Back then we had not identified the function of this Me2+ binding site, but suggested that it might be involved in copper homeostasis. Further experiments with inhibitors and chelators (unpublished) suggested that Me2+ binding to the lobster protein might have a stabilising effect on the ring- or star-shaped assembly. It has been observed recently that excess Cu2+ caused the inhibition of vacuole fusion and V-ATPase function in yeast. In addition, a Cu2+-specific chelator rescued fusion, whereas a Cu1+-specific chelator had no effect on the inhibited fusion (Miner et al., 2019) (although the chelators used might not have penetrated inside the cells, which prevents excluding the possibility of the effect of intracellular Cu1+). This observation is in an apparent contradiction with earlier observations made on cucumber roots where both the hydrolytic and proton-transport activity of V-ATPase increased under copper stress (Kabała et al., 2013). Importantly, copper induced transcription of subunit c of V-ATPase was also observed, hinting that this subunit is a direct target of Cu2+ and that the effect of copper treatment on V-ATPase activity was actually the result of a compensatory mechanism provoked by copper toxicity in roots (Kabała et al., 2014). In addition, it is likely that the stabilising/destabilising [Me2+] effects are not unidirectional and uniform for all states of the enzyme and the c-ring (see below). For instance, in this case low effective [Cu2+] is probably sufficient for shifting the equilibrium towards assembled c-rings (ie., when Vo and V1 are dissociated) from monomeric c subunits, and low [Cu2+] is conditional to structural flexibility of the c-ring needed for assisting membrane fusion processes. On the other hand, high(er) [Cu2+] might over-stabilise the c-ring preventing its role in membrane fusion but promoting the formation of functional Vo, but very high [Cu2+] might interfere with the intact enzyme possibly through different binding sites/mechanism (for instance by perturbing the Vo-V1 association). These observations also further point toward a role of the V-ATPase in copper homeostasis under un-perturbed, none-stressed conditions (Eide et al., 1993; Pali et al., 2006; Schlecht et al., 2014). There have been further convincing arguments provided for Me2+ binding sites present in the c-ring (Ductin) assembly of the F- and V-ATPases, and it has also been proposed that different cations can bind (Pali et al., 2006) and even compete for binding sites on the F-ATPase under certain conditions (Van Walraven et al., 2002; Giorgio et al., 2017; Nath, 2020; Nesci, 2022a). Therefore it appears that the Ductin Me2+ binding sites are structurally flexible and not very specific.
Systematic studies on the affinity profile of Me2+ binding to Ductin proteins are still missing but strongly needed for a better understanding of Me2+ effects on the function of these proteins. Nevertheless, it is clear from the above overview that all the various functions of the Ductin proteins have been documented to be sensitive to the presence of certain divalent cations, most frequently Cu2+ or Ca2+ ions, for most of the better known members of the family. Given that we found a prominent Me2+ binding site in a well-characterised Ductin protein, and in view of the great sequence and structure similarity within the family, we hypothesise that, in addition to the already known regulatory factors, Me2+ can structurally modulate the various functions of oligomeric Ductin assemblies via affecting their stability by reversible non-covalent binding to them (Figure 1). Other binding site(s) are still mostly un-identified and the detailed mechanism of Me2+ effects on Ductin assemblies are not yet known. However, it can be concluded that the sign of the—stabilising or destabilising—effect of Me2+ binding to Ductin assemblies depend on [Me2+] and on wether these assemblies are part of an intact rotary ATPase or their proton-conducting membrane domain, or the Ductins are free in a monomeric or oligomeric form in the membrane. For instance, the activity of F- and V-ATPases decrease at high [Me2+], probably because of interference with subunit-subunit interactions, and high [Me2+] might promote dissociation of the catalytic (F1, V1) from the transport (Fo, Vo) domains in these rotary enzymes. On the other hand, low [Me2+] is probably needed for assembling and stabilising the c ring from monomers. Indeed, a fine control of the stability of the assembly ranging from disassembled monomers through a loosely to tightly assembled c-ring is expected to render precise regulation of Ductin functions possible. For instance, in V-ATPase and F-ATPase loosening or tightening the c-ring of the rotor may decrease or increase, respectively, the efficiency of the catalytic function and the rate of the proton translocation. In order to posses a central pore, mediatophore and gap-junction Ductin proteins must be in the assembled c-ring form (Figure 1, left), which is promoted by binding of divalent cations. On the other hand, Ductins participating in membrane fusion processes need intra- and inter-membrane rearrangements and exchange of monomers, which assumes, at least temporarily, a loose c-ring form or monomeric Ductins (Figure 1, right), which in turn assumes low concentration of any structural Me2+, whereas high [Me2+] prevents rearrangement of the monomers. In addition, in the loose c-ring or monomeric state the lipid-protein interface is different from the sealed stable c-ring state (Figure 1), which renders different lipid-specific regulatory effects possible. It is very likely that there is an affinity profile of the binding site(s) for different divalent metal cations, but so far mostly Cu2+ and Ca2+ were reported to affect the various Ductin functions. It should be noted that a direct Me2+ binding effect on Ductin assemblies might be masked in vivo by other processes sensitive to the Me2+. Therefore reconstituted systems should be initially preferred for testing the above hypothesis and for further studies on the details of Me2+ binding to Ductins assemblies. Finally, since un-plugged c-ring pores in biomembranes are lethal to the host cells, expression systems aiming at purification of Ductin proteins should prefer low [Me2+], in order to prevent pore formation of the over-expressed monomers.
KS-N contributed to the analysis, selection and organisation of literature references, checked the text for consistency and improved the chemistry aspects. AB contributed to the section about autophagy and improved the section about putative Ductin binding site(s) from the biochemical point of view. GJ made the first draft of the section about autophagy and then improved it in the final stage. TP developed the idea, conception and design of the review, made the figure and the first complete draft, and finalised the manuscript. All authors contributed to manuscript revision, read, and approved the submitted version.
This work was supported by the Hungarian National Research Development and Innovation Fund (K 101633, K 112716, KKP129797) and the GINOP-2.3.2-15-2016-00001 program.
The authors declare that the research was conducted in the absence of any commercial or financial relationships that could be construed as a potential conflict of interest.
All claims expressed in this article are solely those of the authors and do not necessarily represent those of their affiliated organizations, or those of the publisher, the editors and the reviewers. Any product that may be evaluated in this article, or claim that may be made by its manufacturer, is not guaranteed or endorsed by the publisher.
Alavian, K. N., Beutner, G., Lazrove, E., Sacchetti, S., Park, H. A., Licznerski, P., et al. (2014). An uncoupling channel within the c-subunit ring of the F1FO ATP synthase is the mitochondrial permeability transition pore. Proc. Natl. Acad. Sci. U. S. A. 111 (29), 10580–10585. doi:10.1073/pnas.1401591111
Algieri, V., Algieri, C., Maiuolo, L., De Nino, A., Pagliarani, A., Tallarida, M. A., et al. (2021). 1,5-Disubstituted-1,2,3-triazoles as inhibitors of the mitochondrial Ca2+ -activated F1 FO -ATP(hydrol)ase and the permeability transition pore. Ann. N. Y. Acad. Sci. 1485 (1), 43–55. doi:10.1111/nyas.14474
Amendola, J., Boumedine, N., Sangiardi, M., and El Far, O. (2015). Optimization of neuronal cultures from rat superior cervical ganglia for dual patch recording. Sci. Rep. 514455, 14455. doi:10.1038/srep14455
Amodeo, G. F., Lee, B. Y., Krilyuk, N., Filice, C. T., Valyuk, D., Otzen, D. E., et al. (2021). C subunit of the ATP synthase is an amyloidogenic calcium dependent channel-forming peptide with possible implications in mitochondrial permeability transition. Sci. Rep. 11 (1), 8744. doi:10.1038/s41598-021-88157-z
Amodeo, G. F., Solesio, M. E., and Pavlov, E. V. (2017). From ATP synthase dimers to C-ring conformational changes: Unified model of the mitochondrial permeability transition pore. Cell Death Dis. 8 (12), 1. doi:10.1038/s41419-017-0042-3
Birman, S., Meunier, F. M., Lesbats, B., Le Caer, J. P., Rossier, J., and Israël, M. (1990). A 15 kDa proteolipid found in mediatophore preparations from Torpedo electric organ presents high sequence homology with the bovine chromaffin granule protonophore. FEBS Lett. 261 (2), 303–306. doi:10.1016/0014-5793(90)80577-6
Bodzęta, A., Kahms, M., and Klingauf, J. (2017). The presynaptic v-ATPase reversibly disassembles and thereby modulates exocytosis but is not part of the fusion machinery. Cell Rep. 20 (6), 1348–1359. doi:10.1016/j.celrep.2017.07.040
Bonora, M., Morganti, C., Morciano, G., Pedriali, G., Lebiedzinska-Arciszewska, M., Aquila, G., et al. (2017). Mitochondrial permeability transition involves dissociation of F1FO ATP synthase dimers and C-ring conformation. EMBO Rep. 18 (7), 1077–1089. doi:10.15252/embr.201643602
Borsch, M. (2013). Microscopy of single F(o) F(1) -ATP synthases--the unraveling of motors, gears, and controls. IUBMB Life 65 (3), 227–237. doi:10.1002/iub.1149
Brochier, G., Gulik-Krzywicki, T., Lesbats, B., Dedieu, J. C., and Israël, M. (1992). Calcium-induced acetylcholine release and intramembrane particle occurrence in proteoliposomes equipped with mediatophore. Biol. Cell 74 (2), 225–230. doi:10.1016/0248-4900(92)90029-z
Brochier, G., and Morel, N. (1993). The same 15 kDa proteolipid subunit is a constituent of two different proteins in Torpedo, the acetylcholine releasing protein mediatophore and the vacuolar H+ ATPase. Neurochem. Int. 23 (6), 525–539. doi:10.1016/0197-0186(93)90100-j
Carraro, M., and Bernardi, P. (2016). Calcium and reactive oxygen species in regulation of the mitochondrial permeability transition and of programmed cell death in yeast. Cell Calcium 60 (2), 102–107. doi:10.1016/j.ceca.2016.03.005
Couoh-Cardel, S., Hsueh, Y. C., Wilkens, S., and Movileanu, L. (2016). Yeast V-ATPase proteolipid ring acts as a large-conductance transmembrane protein pore. Sci. Rep. 6, 624774. doi:10.1038/srep24774
Di, G., J., Boudkkazi, S., Mochida, S., Bialowas, A., Samari, N., Leveque, C., et al. (2010). V-ATPase membrane sector associates with synaptobrevin to modulate neurotransmitter release. Neuron 67 (2), 268–279. doi:10.1016/j.neuron.2010.06.024
Dunant, Y., Cordeiro, J. M., and Gonçalves, P. P. (2009). Exocytosis, mediatophore, and vesicular Ca2+/H+ antiport in rapid neurotransmission. Ann. N. Y. Acad. Sci. 1152, 1152100–1152112. doi:10.1111/j.1749-6632.2008.04000.x
Dunlop, J., Jones, P. C., and Finbow, M. E. (1995). Membrane insertion and assembly of ductin: a polytopic channel with dual orientations. EMBO J. 14 (15), 3609–3616. doi:10.1002/j.1460-2075.1995.tb00030.x
Eide, D. J., Bridgham, J. T., Zhao, Z., and Mattoon, J. R. (1993). The vacuolar H(+)-ATPase of Saccharomyces cerevisiae is required for efficient copper detoxification, mitochondrial function, and iron metabolism. Mol. Gen. Genet. 241 (3-4), 447–456. doi:10.1007/BF00284699
El Far, O., and Seagar, M. (2011). A role for V-ATPase subunits in synaptic vesicle fusion? J. Neurochem. 117 (4), 603–612. doi:10.1111/j.1471-4159.2011.07234.x
Ferencz, C. M., Petrovszki, P., Dér, A., Sebők-Nagy, K., Kóta, Z., and Páli, T. (2017). Oscillating electric field measures the rotation rate in a native rotary enzyme. Sci. Rep. 7, 745309. doi:10.1038/srep45309
Finbow, M. E., Goodwin, S. F., Meagher, L., Lane, N. J., Keen, J., Findlay, J. B., et al. (1994). Evidence that the 16 kDa proteolipid (subunit c) of the vacuolar H(+)-ATPase and ductin from gap junctions are the same polypeptide in Drosophila and manduca: molecular cloning of the Vha16k gene from Drosophila. J. Cell Sci. 107, 1817–1824. doi:10.1242/jcs.107.7.1817
Finbow, M. E., Harrison, M., and Jones, P. (1995). Ductin--a proton pump component, a gap junction channel and a neurotransmitter release channel. Bioessays 17 (3), 247–255. doi:10.1002/bies.950170311
Finbow, M. E., John, S., Kam, E., Apps, D. K., and Pitts, J. D. (1993). Disposition and orientation of ductin (DCCD-reactive vacuolar H(+)-ATPase subunit) in mammalian membrane complexes. Exp. Cell Res. 207 (2), 261–270. doi:10.1006/excr.1993.1192
Finbow, M. E., and Meagher, L. (1992). Connexins and the vacuolar proteolipid-like 16-kDa protein are not directly associated with each other but may be components of similar or the same gap junctional complexes. Exp. Cell Res. 203 (1), 280–284. doi:10.1016/0014-4827(92)90066-h
Frasch, W. D., Bukhari, Z. A., and Yanagisawa, S. (2022). F1FO ATP synthase molecular motor mechanisms. Front. Microbiol. 13, 13965620. doi:10.3389/fmicb.2022.965620
Fujii, T., Takada-Takatori, Y., Horiguchi, K., and Kawashima, K. (2012). Mediatophore regulates acetylcholine release from T cells. J. Neuroimmunol. 244 (1-2), 16–22. doi:10.1016/j.jneuroim.2011.12.022
Galli, T., McPherson, P. S., and De Camilli, P. (1996). The V0 sector of the V-ATPase, synaptobrevin, and synaptophysin are associated on synaptic vesicles in a Triton X-100-resistant, freeze-thawing sensitive, complex. J. Biol. Chem. 271 (4), 2193–2198. doi:10.1074/jbc.271.4.2193
Giorgio, V., Burchell, V., Schiavone, M., Bassot, C., Minervini, G., Petronilli, V., et al. (2017). Ca2+ binding to F-ATP synthase β subunit triggers the mitochondrial permeability transition. EMBO Rep. 18 (7), 1065–1076. doi:10.15252/embr.201643354
Giorgio, V., Guo, L., Bassot, C., Petronilli, V., and Bernardi, P. (2018). Calcium and regulation of the mitochondrial permeability transition. Cell Calcium 22, 7056–7063. doi:10.1016/j.ceca.2017.05.004
Halestrap, A. P. (2014). The C ring of the F1Fo ATP synthase forms the mitochondrial permeability transition pore: A critical appraisal. Front. Oncol. 4234, 234. doi:10.3389/fonc.2014.00234
Harrison, M., Murray, J., Powell, B., Kim, Y., Finbow, M., and Findlay, J. (1999). Helical interactions and membrane disposition of the 16-kDa proteolipid subunit of the vacuolar H+ ATPase analyzed by cysteine replacement mutagenesis. J. Biol. Chem. 274, 27425461–27425470. doi:10.1074/jbc.274.36.25461
Holzenburg, A., Jones, P. C., Franklin, T., Pali, T., Heimburg, T., Marsh, D., et al. (1993). Evidence for a common structure for a class of membrane channels. Eur. J. Biochem. 213, 21321–21330. doi:10.1111/j.1432-1033.1993.tb17730.x
Israël, M., and Dunant, Y. (1999). Mediatophore, a protein supporting quantal acetylcholine release. Can. J. Physiol. Pharmacol. 77 (9), 689–698. doi:10.1139/y99-080
Israël, M., Lesbats, B., and Bruner, J. (1993). Glutamate and acetylcholine release from cholinergic nerve terminals, a calcium control of the specificity of the release mechanism. Neurochem. Int. 22 (1), 53–58. doi:10.1016/0197-0186(93)90068-g
Israël, M., Lesbats, B., Morel, N., Manaranche, R., and Birman, S. (1988). The lipid requirements of mediatophore for acetylcholine release activity. Large-scale purification of this protein in a reactive form. Neurochem. Int. 13 (2), 199–205. doi:10.1016/0197-0186(88)90055-1
Israël, M., Morel, N., Lesbats, B., Birman, S., and Manaranche, R. (1986). Purification of a presynaptic membrane protein that mediates a calcium-dependent translocation of acetylcholine. Proc. Natl. Acad. Sci. U. S. A. 83, 839226–839230. doi:10.1073/pnas.83.23.9226
Itakura, E., Kishi-Itakura, C., and Mizushima, N. (2012). The hairpin-type tail-anchored SNARE syntaxin 17 targets to autophagosomes for fusion with endosomes/lysosomes. Cell 151 (6), 1256–1269. doi:10.1016/j.cell.2012.11.001
Jones, P. C., Harrison, M. A., Kim, Y., Finbow, M. E., and Findlay, J. B. C. (1995). The first putative transmembrane helix of the 16 kDa proteolipid lines a pore in the Vo sector of the vacuolar H(+)-ATPase. Biochem. J. 312, 739–747. doi:10.1042/bj3120739
Junge, W., and Nelson, N. (2015). ATP synthase. Annu. Rev. Biochem. 84, 84631–84657. doi:10.1146/annurev-biochem-060614-034124
Kabała, K., Janicka-Russak, M., and Anklewicz, A. (2013). Mechanism of Cd and Cu action on the tonoplast proton pumps in cucumber roots. Physiol. Plant 147 (2), 207–217. doi:10.1111/j.1399-3054.2012.01655.x
Kabała, K., Janicka-Russak, M., Reda, M., and Migocka, M. (2014). Transcriptional regulation of the V-ATPase subunit c and V-PPase isoforms in Cucumis sativus under heavy metal stress. Physiol. Plant 150 (1), 32–45. doi:10.1111/ppl.12064
Kishikawa, J., Nakanishi, A., Nakano, A., Saeki, S., Furuta, A., Kato, T., et al. (2022). Structural snapshots of V/A-ATPase reveal the rotary catalytic mechanism of rotary ATPases. Nat. Commun. 13 (1), 1213. doi:10.1038/s41467-022-28832-5
Kubo, S., Niina, T., and Takada, S. (2020). Molecular dynamics simulation of proton-transfer coupled rotations in ATP synthase FO motor. Sci. Rep. 10 (1), 8225. doi:10.1038/s41598-020-65004-1
Kühlbrandt, W. (2019). Structure and mechanisms of F-type ATP synthases. Annu. Rev. Biochem. 88, 88515–88549. doi:10.1146/annurev-biochem-013118-110903
Lautemann, J., and Bohrmann, J. (2016). Relating proton pumps with gap junctions: colocalization of ductin, the channel-forming subunit c of V-ATPase, with subunit a and with innexins 2 and 3 during Drosophila oogenesis. BMC Dev. Biol. 16 (1), 24. doi:10.1186/s12861-016-0124-y
Malo, M., and Israël, M. (2003). Expression of the acetylcholine release mechanism in various cells and reconstruction of the release mechanism in non-releasing cells. Life Sci. 72 (18-19), 2029–2038. doi:10.1016/s0024-3205(03)00066-3
Mauvezin, C., Nagy, P., Juhász, G., and Neufeld, T. P. (2015). Autophagosome-lysosome fusion is independent of V-ATPase-mediated acidification. Nat. Commun. 6, 67007. doi:10.1038/ncomms8007
Miner, G. E., Sullivan, K. D., Zhang, C., Hurst, L. R., Starr, M. L., Rivera-Kohr, D. A., et al. (2019). Copper blocks V-ATPase activity and SNARE complex formation to inhibit yeast vacuole fusion. Traffic 20 (11), 841–850. doi:10.1111/tra.12683
Mnatsakanyan, N., and Jonas, E. A. (2020). ATP synthase c-subunit ring as the channel of mitochondrial permeability transition: Regulator of metabolism in development and degeneration. J. Mol. Cell Cardiol. 144, 144109–144118. doi:10.1016/j.yjmcc.2020.05.013
Morel, N., and Israël, M. (2000). Role of mediatophore in connection with proteins of the active zone in synaptic transmission. Microsc. Res. Tech. 49 (1), 47–55. doi:10.1002/(SICI)1097-0029(20000401)49:1<47::AID-JEMT6>3.0.CO;2-W
Murphy, B. J., Klusch, N., Langer, J., Mills, D. J., Yildiz, Ö., and Kühlbrandt, W. (2019). Rotary substates of mitochondrial ATP synthase reveal the basis of flexible F1-Fo coupling. Science 364 (6446), eaaw9128. doi:10.1126/science.aaw9128
Nakanishi, A., Kishikawa, J. I., Mitsuoka, K., and Yokoyama, K. (2019). Cryo-EM studies of the rotary H+-ATPase/synthase from Thermus thermophilus. Biophys. Physicobiol 16, 16140–16146. doi:10.2142/biophysico.16.0_140
Nath, S. (2020). A novel conceptual model for the dual role of FOF1-ATP synthase in cell life and cell death. Biomol. Concepts 11 (1), 143–152. doi:10.1515/bmc-2020-0014
Neginskaya, M. A., Solesio, M. E., Berezhnaya, E. V., Amodeo, G. F., Mnatsakanyan, N., Jonas, E. A., et al. (2019). ATP synthase C-Subunit-Deficient mitochondria have a small cyclosporine A-sensitive channel, but lack the permeability transition pore. Cell Rep. 26 (1), 11–17. doi:10.1016/j.celrep.2018.12.033
Nesci, S. (2022b). Protein folding and unfolding: Proline cis-trans isomerization at the c subunits of F1 FO -ATPase might open a high conductance ion channel. Proteins 90, 2001–2005. doi:10.1002/prot.26383
Nesci, S. (2020). The mitochondrial permeability transition pore in cell death: A promising drug binding bioarchitecture. Med. Res. Rev. 40 (2), 811–817. doi:10.1002/med.21635
Nesci, S. (2022a). What happens when the mitochondrial H+-translocating F1FO-ATP(hydrol)ase becomes a molecular target of calcium? The pore opens. Biochimie 25, 19892–19895. doi:10.1016/j.biochi.2022.03.012
Noji, H., Ueno, H., and Kobayashi, R. (2020). Correlation between the numbers of rotation steps in the ATPase and proton-conducting domains of F- and V-ATPases. Biophys. Rev. 12 (2), 303–307. doi:10.1007/s12551-020-00668-7
Pali, T., Finbow, M. E., Holzenburg, A., Findlay, J. B. C., and Marsh, D. (1995). Lipid-protein interactions and assembly of the 16-kDa channel polypeptide from Nephrops norvegicus. Studies with spin-label electron spin resonance spectroscopy and electron microscopy. Biochemistry 34 (28), 9211–9218. doi:10.1021/bi00028a034
Pali, T., Finbow, M. E., and Marsh, D. (2006). A divalent-ion binding site on the 16-kDa proton channel from Nephrops norvegicus–revealed by EPR spectroscopy. Biochim. Biophys. Acta 1758 (2), 206–212. doi:10.1016/j.bbamem.2006.02.004
Pali, T., Finbow, M. E., and Marsh, D. (1999). Membrane assembly of the 16-kDa proteolipid channel from Nephrops norvegicus studied by relaxation enhancements in spin-label ESR. Biochemistry 38 (43), 14311–14319. doi:10.1021/bi991459c
Pali, T., Finbow, M. E., and Marsh, D. (1997). Membrane assembly of the 16-kDa V-ATPase proteolipid subunit from spin-lattice relaxation enhancements in spin label ESR. Biophysical J. 38, 14311–14319. 72TUAM7. doi:10.1021/bi991459c
Pali, T., Whyteside, G., Dixon, N., Kee, T. P., Ball, S., Harrison, M. A., et al. (2004). Interaction of inhibitors of the vacuolar H+-ATPase with the transmembrane V-o-sector. Biochemistry 43 (38), 12297–12305. doi:10.1021/bi0493867
Pinke, G., Zhou, L., and Sazanov, L. A. (2020). Cryo-EM structure of the entire mammalian F-type ATP synthase. Nat. Struct. Mol. Biol. 27 (11), 1077–1085. doi:10.1038/s41594-020-0503-8
Poea-Guyon, S., Ammar, M. R., Erard, M., Amar, M., Moreau, A. W., Fossier, P., et al. (2013). The V-ATPase membrane domain is a sensor of granular pH that controls the exocytotic machinery. J. Cell Biol. 203 (2), 283–298. doi:10.1083/jcb.201303104
Rama, S., Boumedine-Guignon, N., Sangiardi, M., Youssouf, F., Maulet, Y., Lévêque, C., et al. (2019). Chromophore-assisted light inactivation of the V-ATPase V0c subunit inhibits neurotransmitter release downstream of synaptic vesicle acidification. Mol. Neurobiol. 56 (5), 3591–3602. doi:10.1007/s12035-018-1324-1
Rawson, S., Phillips, C., Huss, M., Tiburcy, F., Wieczorek, H., Trinick, J., et al. (2015). Structure of the vacuolar H(+)-ATPase rotary motor reveals new mechanistic insights. Structure 23 (3), 461–471. doi:10.1016/j.str.2014.12.016
Sbia, M., Diebler, M. F., Morel, N., and Israël, M. (1992). Effect of N,N’-dicyclohexylcarbodiimide on acetylcholine release from Torpedo synaptosomes and proteoliposomes reconstituted with the proteolipid mediatophore. J. Neurochem. 59 (4), 1273–1279. doi:10.1111/j.1471-4159.1992.tb08437.x
Schlecht, U., Suresh, S., Xu, W., Aparicio, A. M., Chu, A., Proctor, M. J., et al. (2014). A functional screen for copper homeostasis genes identifies a pharmacologically tractable cellular system. BMC Genomics 15263, 263. doi:10.1186/1471-2164-15-263
Shiff, G., Synguelakis, M., and Morel, N. (1996). Association of syntaxin with SNAP 25 and VAMP (synaptobrevin) in Torpedo synaptosomes. Neurochem. Int. 29 (6), 659–667. doi:10.1016/s0197-0186(96)00026-5
Strasser, B., Iwaszkiewicz, J., Michielin, O., and Mayer, A. (2011). The V-ATPase proteolipid cylinder promotes the lipid-mixing stage of SNARE-dependent fusion of yeast vacuoles. EMBO J. 30 (20), 4126–4141. doi:10.1038/emboj.2011.335
Sugawa, M., Okazaki, K.-i., Kobayashi, M., Matsui, T., Hummer, G., Masaike, T., et al. (2016). F1-ATPase conformational cycle from simultaneous single-molecule FRET and rotation measurements. Proc. Natl. Acad. Sci. U. S. A. 113 (21), E2916–E2924. doi:10.1073/pnas.1524720113
Takáts, S., Nagy, P., Varga, Á., Pircs, K., Kárpáti, M., Varga, K., et al. (2013). Autophagosomal Syntaxin17-dependent lysosomal degradation maintains neuronal function in Drosophila. J. Cell Biol. 201 (4), 531–539. doi:10.1083/jcb.201211160
Van Walraven, H. S., Scholts, M. J., Zakharov, S. D., Kraayenhof, R., and Dilley, R. A. (2002). pH-dependent Ca2+ binding to the F0 c-subunit affects proton translocation of the ATP synthase from Synechocystis 6803. J. Bioenerg. Biomembr. 34 (6), 455–464. doi:10.1023/a:1022518109371
Xia, Y., Liu, N., Xie, X., Bi, G., Ba, H., Li, L., et al. (2019). The macrophage-specific V-ATPase subunit ATP6V0D2 restricts inflammasome activation and bacterial infection by facilitating autophagosome-lysosome fusion. Autophagy 15 (6), 960–975. doi:10.1080/15548627.2019.1569916
Yamato, I., Kakinuma, Y., and Murata, T. (2016). Operating principles of rotary molecular motors: differences between F1 and V1 motors. BIOPHYSICS 13 (0), 37–44. doi:10.2142/biophysico.13.0_37
Keywords: Ductin, V-ATPase, F-ATPase, ATP synthase, gap-junction, autophagy, mitochondrial permeability transition pore, divalent cation
Citation: Sebők-Nagy K, Blastyák A, Juhász G and Páli T (2023) Reversible binding of divalent cations to Ductin protein assemblies—A putative new regulatory mechanism of membrane traffic processes. Front. Mol. Biosci. 10:1195010. doi: 10.3389/fmolb.2023.1195010
Received: 27 March 2023; Accepted: 24 April 2023;
Published: 09 May 2023.
Edited by:
Enrico Ravera, University of Florence, ItalyReviewed by:
Nicolas Vitale, Centre National de la Recherche Scientifique (CNRS), FranceCopyright © 2023 Sebők-Nagy, Blastyák, Juhász and Páli. This is an open-access article distributed under the terms of the Creative Commons Attribution License (CC BY). The use, distribution or reproduction in other forums is permitted, provided the original author(s) and the copyright owner(s) are credited and that the original publication in this journal is cited, in accordance with accepted academic practice. No use, distribution or reproduction is permitted which does not comply with these terms.
*Correspondence: Tibor Páli, dHBhbGlAYnJjLmh1
Disclaimer: All claims expressed in this article are solely those of the authors and do not necessarily represent those of their affiliated organizations, or those of the publisher, the editors and the reviewers. Any product that may be evaluated in this article or claim that may be made by its manufacturer is not guaranteed or endorsed by the publisher.
Research integrity at Frontiers
Learn more about the work of our research integrity team to safeguard the quality of each article we publish.