- 1Department of Nutrition and Integrative Physiology, College of Health and Human Sciences, Florida State University, Tallahassee, FL, United States
- 2Department of Biomedical and Chemical Engineering and Sciences, Florida Institute of Technology, Melbourne, FL, United States
Emerging evidence reveals the fundamental role of the gut microbiome in human health. Among various factors regulating our gut microbiome, diet is one of the most indispensable and prominent one. Inulin is one of the most widely-studied dietary fiber for its beneficial prebiotic effects by positively modulating the gut microbiome and microbial metabolites. Recent research underscores sexual dimorphism and sex-specific disparities in microbiome and also diet-microbiome interactions. However, whether and how the prebiotic effects of dietary fiber differ among sexes remain underexplored. To this end, we herein examine sex-specific differences in the prebiotic effects of inulin on gut microbiome and metabolome in a humanized murine model of aging i.e., aged mice carrying human fecal microbiota. The findings demonstrate that inulin exerts prebiotic effects, but in a sex-dependent manner. Overall, inulin increases the proportion of Bacteroides, Blautia, and glycine, while decreasing Eggerthella, Lactococcus, Streptococcus, trimethylamine, 3-hydroxyisobutyrate, leucine and methionine in both sexes. However, we note sex-specific effects of inulin including suppression of f_Enteroccaceae:_, Odoribacter, bile acids, malonate, thymine, valine, acetoin, and ethanol while promotion of Dubosiella, pyruvate, and glycine in males. Whereas, suppression of Faecalibaculum, Lachnoclostridium, Schaedlerella, phenylalanine and enhancement of Parasutterella, Phocaeicola, f_Lachnospiraceae;_, Barnesiella, Butyricimonas, glycine, propionate, acetate and glutamate are observed in females. Altogether, the study reveals that prebiotic mechanisms of dietary fiber vary in a sex-dependent manner, underscoring the importance of including both sexes in preclinical/clinical studies to comprehend the mechanisms and functional aspects of dietary interventions for effective extrapolation and translation in precision nutrition milieus.
1 Introduction
Emerging evidence has highlighted the profound impact of the gut microbiome on our physiology and health (Jovel et al., 2018). Our gut is densely packed with a highly diverse and complex community of microorganisms, which via their specific metabolic activities continuously interact with the intestinal epithelium leading to distinct physiological, metabolic, and immunomodulatory responses either locally or systemically within the host (Nagpal et al., 2016). The diversity and metabolic capacity of the gut microbiome are strongly and tightly regulated by the dietary lifestyle of the host throughout the lifecycle. Moreover, the evolution of gut microbiome with the aging process is closely linked to dietary patterns with a fiber-deficit diet being often correlated with reduced gut bacterial diversity and increased propensity to various intestinal, cardiometabolic, and neurobehavioral disorders (Nagpal et al., 2018a; Ghosh et al., 2022). Recent studies by us and others have also highlighted the existence of considerable sexual dimorphism in the gut microbiome, indicating that dietary interventions may influence gut microbiota in a sex-dependent manner (Bolnick et al., 2014; Suzuki et al., 2017). Despite the significant influence of sex-diet interactions on the gut microbiome, most clinical studies examining the effects of dietary fiber intake have overlooked the phenomenon of sexual dimorphism in the gut microbiome (Dominianni et al., 2015; Chu et al., 2019; Reimer et al., 2020; Vijay et al., 2021), with few noted exceptions (Trimigno et al., 2017; Reider et al., 2020; Benítez-Páez et al., 2021). Besides, dietary studies involving preclinical models mostly use only males to avoid confounding effects of the estrus cycle in females and thus fail to examine sex-based differences (Shastri et al., 2015). Since the precise mechanisms underlying the relationship between diet, sex and microbiome/metabolome are only beginning to be understood, it becomes imperative to investigate the mechanisms of dietary components including fibers and prebiotics to validate the universality of their health effects while avoiding the extrapolation of the prebiotic effects of males on females or vice versa.
Of all the dietary fibers, inulin is the most extensively studied one for its prebiotic properties and beneficial modulation of the gut microbiome by promoting short-chain fatty acids (SCFAs) in the distal gut leading to enhanced intestinal homeostasis, immune-stimulation, blood glucose, and lipid regulation (Tawfick et al., 2022). Inulin is composed of 2–60 linear fructose units joined together via β-(1 → 2)-D-fructosyl-fructose glycosidic bonds along with a glucose unit at the terminal end attached via α-(1 → 2) linkage. Despite the increasing recognition of host sex as a major confounder in microbiome studies, the mechanistic preclinical studies exploring the health effects of inulin are largely confined to male rodents (Zhang et al., 2017; Du et al., 2020; Li et al., 2020; Shao et al., 2020; Régnier et al., 2023). Animal models are widely used for understanding the mechanistic and functional basis of health benefits imparted by dietary fibers but suffer from limitations of differences in their gut microbial community structure compared to humans (Li and Zhang, 2022). For instance, the relative abundance of 79 genera found in mouse and human gut varies greatly in their relative abundance patterns (Nguyen et al., 2015; Nagpal et al., 2018b). Animal models colonized with human microbiota offer an attractive avenue to address this problem and may provide close reflections of dietary impacts which are ecologically similar to the human gut (Lundberg, 2019). However, there is a lack of evidence elucidating the prebiotic effects of inulin on gut microbiome-metabolome arrays in humanized murine models of aging, particularly taking into consideration the indirect influence of a sexually dimorphic gut. Keeping these knowledge gaps in view, we herein aim to determine the extent to which inulin supplementation within a western-style diet ameliorates gut microbiome and metabolome pool towards a healthy ecosystem in a humanized mouse model of aging and whether and how these prebiotic effects differ according to host sex. The study reveals that inulin modulates gut microbiome and its metabolites in a sex-dependent manner, thereby signifying the prevalence of sexual dimorphism in the gut microbiome which may differentially regulate the diet-microbiome interface as well as the therapeutic attributes delivered by dietary fiber.
2 Material and methods
2.1 Study design
Animal experiments were executed as per our previously described protocol (Kadyan et al., 2023). Briefly, old male and female C57BL/6J mice (55 weeks old) were subjected to a gut-depletion procedure using the antibiotic cocktail (ampicillin [1 g], Metronidazole [1 g], Neomycin [1 g], and Vancomycin [0.5 g] per liter drinking water) regimen for 4 days followed by polyethylene glycol treatment (200 μL per dose per mouse; 425 g/L) to cleanse remnant gut microbiota. Pooled fecal samples from five healthy older adult human donors (3M, 2F; 50–55 years old) were transplanted (via oral gavage) in mice after followed by their random allocation into two experimental groups (N = six to eight per group per sex): CTL (western-style high-fat diet control) and INU (high-fat diet supplemented with inulin (Biosynth) @ 5% w/w). The dietary regimen continued for 20 weeks. The fecal samples for metabolome and microbiome analysis were collected and stored at −80°C till further analysis. The study was carried out in accordance with the recommendations and guidelines of the Institutional Animal Care and Use Committee. The protocol was approved by the Institutional Animal Care and Use Committee at Florida State University (PROTO202100008).
2.2 Gut microbiome analysis
The gut microbiome was measured as per our previously described methods (Nagpal et al., 2018a; Ahmadi et al., 2019; Nagpal et al., 2019; Nagpal et al., 2021; Saccon et al., 2021; Clark et al., 2022; Munley et al., 2022). Briefly, genomic DNA from 200 mg of the fecal specimen was extracted using the QIAmp PowerFecal Pro DNA Kit (Qiagen) according to the manufacturer’s instructions. The amplification of the hypervariable V4 region of bacterial 16S rRNA gene using Universal primers 515F (barcoded) and 806R was done in accordance with the Earth Microbiome Project benchmark protocol (https://earthmicrobiome.org/). Equal molar concentrations of the library were pooled and sequenced for paired-end (2 × 300 bp) sequencing using an Illumina MiSeq sequencer (using Miseq reagent kit v3; Illumina Inc., San Diego, USA). QIIME2 (ver. 2–2022.11) was used for microbiome bioinformatics analysis (Bolyen et al., 2019). The raw sequences were quality-filtered, trimmed, and denoised through DADA2 pipeline (Callahan et al., 2016). All identified amplicon sequence variants (ASVs) were aligned with the MAFFT (Katoh et al., 2002). ASVs were assigned with a naïve Bayes taxonomy classifier developed for the sklearn classifier against the pre-built from the most recent version of Greengenes2 database (ver. 2022.10) (Bokulich et al., 2018; McDonald et al., 2022).
2.3 Global untargeted metabolomics analysis
The collected fecal samples were processed as per the previously described protocol (Gratton et al., 2016) with slight modifications. Briefly, samples were extracted using deionized water by vertexing for 5 min. The extracted samples were mixed with a phosphate buffer (pH = 7.4) in D2O which made the final solution with 10% D2O, 0.1 M phosphate, and 0.1 mM Trimethylsilyl propionate (TSP). The samples were centrifuged and transferred to 5 mm NMR tubes for further data acquisition by a Bruker Ascend 400 MHz high-resolution NMR (Bruker Biospin, Germany. The 1D first increment of a NOESY (noesygppr1d) experiment with water suppression was applied for all samples with 64 scans. All NMR spectra were phased and referenced to TSP in TopSpin 4.06 (Bruker BioSpin, Germany). All the NMR processing will be carried out in Amix 4.0 (Bruker BioSpin) and the NMR spectra will be bucketed using our previously reported automatic method (Wang et al., 2020) to minimize peak overlap and splitting. Metabolite indentation will be carried out using Chenomx 8.6 (Chenomx Inc.). Total intensity normalization was applied before further data analysis.
2.4 Bioinformatics and statistical analysis
‘R’ or ‘Python’ packages were used for downstream microbiome and metabolome analyses and visualization. Observed features (ASVs), Faith PD (phylogenetic diversity), and Shannon (species richness and evenness) index were used as alpha-diversity metrics, and weighted UniFrac distance and Bray-Curtis dissimilarity index were used for the analysis of beta-diversity in the form of the principal coordinate analysis (PCoA). To identify differentially abundant taxa and/or metabolites between CTL and INU groups, the linear discriminant analysis (LDA) effect size (LefSe) (Segata et al., 2011), ANOVA-Like differential expression (ALDEx2) method (Fernandes et al., 2014), and multivariate analysis by linear models (MaAsLin2) (Mallick et al., 2021) were used. p-values were corrected using the Benjamini–Hochberg procedure. Cladograms were generated using differential features with LDA>2.0. For the prediction of microbiome and metabolites via supervised classification, a q2-sample-classifier plugin for the QIIME2 via nested stratified 4-fold cross-validation with Random Forest classifier grown with 2,000 trees was used. Spearman’s correlation was used to identify significant correlations between groups.
3 Results
3.1 Inulin modulates gut microbiome diversity and metabolomic profiles in a sex-dependent manner
The intra-individual diversity (⍺-diversity; microbial species richness) in males and females is evaluated using observed features, Faith PD, and Shannon indices (Figure 1A). No significant differences are observed in the INU group of both sexes; however, Faith PD scores are significantly higher in females than males and are subsequently decreased after INU treatment. The inter-individual diversity (β-diversity), assessed using PCoA based on weighted UniFrac distances, shows significantly distinct arrays between CON vs. INU groups in both sexes (Figures 1B, C). However, fecal metabolome arrays, as assessed via PCoA of the Bray-Curtis dissimilarity index, exhibit relatively more distinct signatures in females than males after INU treatment (Figure 1D). INU treatment shows marked differences (p < 0.014) in the metabolite profiles in females while a similar differential trend (p < 0.052) is also observed in males relative to their CTL groups.
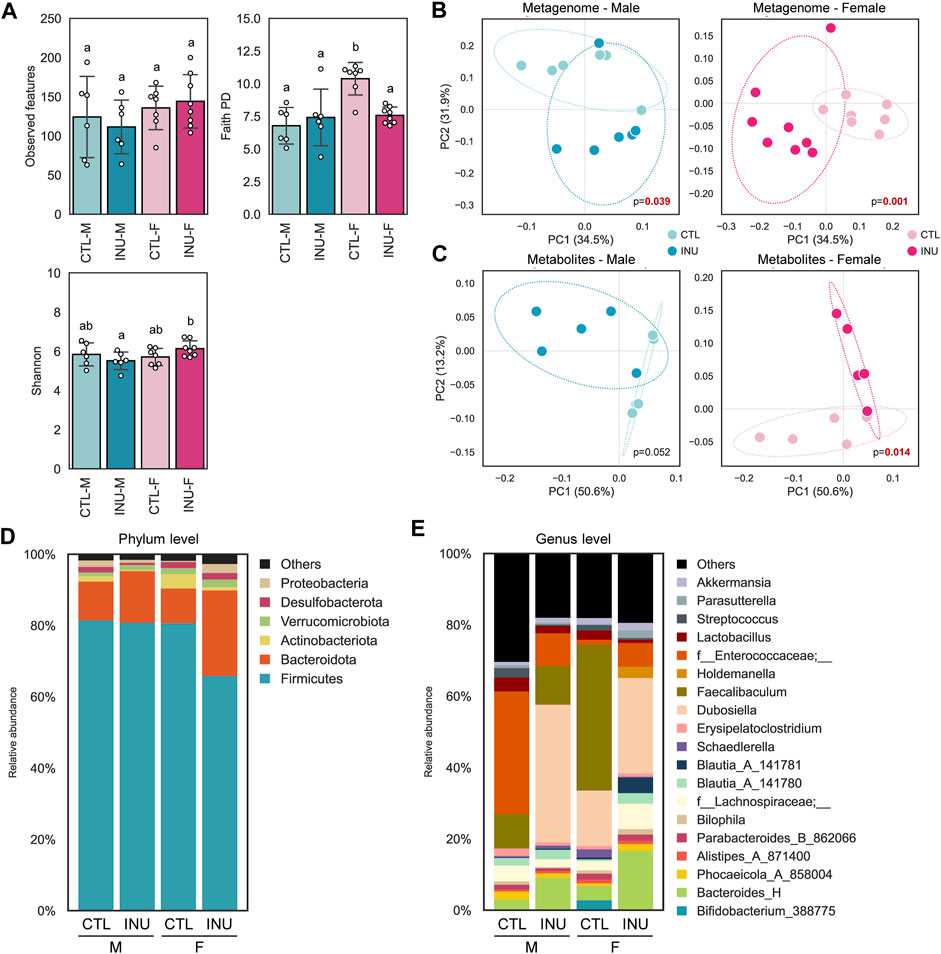
FIGURE 1. Sex-specific effects of inulin on gut microbiome diversity, fecal metabolites, and taxonomic hierarchies. (A) Microbial diversity of each group determined using the number of observed features, Faith PD, and Shannon index with differences between groups assessed using the pairwise Kruskal–Wallis test and different letters indicate significant differences (p < 0.05). PCoA plots of (B) weighted UniFrac distances between samples based on ASVs and (C) Bray-Curtis dissimilarity between samples based on metabolites composition. Bacterial composition at (D) phylum and (E) genus level.
Sex-specific differences in the microbiome modulation by INU treatment are further observed using altered bacterial abundance at bacterial phylum (Figure 1E) and genus level (Figure 1F). At the phylum level, nearly 90% of relative abundance is contributed by Firmicutes and Bacteroidota for all groups. Amongst all, the INU-F group differs from others by exhibiting enhanced and reduced abundance of phyla Bacteroidota and Firmicutes, respectively. Further analysis of genus level reveals interesting patterns of bacterial abundance in both sexes. In males, relative abundance of genera Bacteroides_H and Dubosiella are increased, whereas f_Enterococcaceae;_, f-Lachnospiraceae; _, Erysipelatoclostridium and Streptococcus are decreased after INU intervention. In contrast, INU treatment in females promotes the prevalence of Bacteroides_H, f-Lachnospiraceae;_, Blautia_A, Dubosiella, f_Enterococcaceae;_ and Parasutterella while that of Bifidobacterium_388775, Schaederella, and Faecalibaculum are reduced compared to CTL-F. Altogether, these findings demonstrate that inulin modulates the gut microbiome and metabolome in a sex-dependent manner.
3.2 Inulin exerts sex-specific modulation in gut microbiome-metabolome arrays and metabolic pathways
Unique gut microbiome signatures induced by inulin supplementation in males and females are assessed using two benchmark statistical approaches: LEfSe and ALDEx2. The taxonomic hierarchy of differentially abundant features, as analyzed using LEfSe cladograms (Figure 2A) clearly demonstrate that INU treatment exerts a more profound effect in females than males. Subsequent execution of the more conservative and precise statistical algorithm ALDEx2 for differential abundance assessment of effect size (Nearing et al., 2022) depicts several key taxa that are increased or decreased by INU relative to control (Figure 2B). While no significant differences in the abundance of key taxa are seen in males, females show a significant promotion in the abundance of Parasutterella (q < 0.05), Blautia_A_141781 (q < 0.1) and Bacteroides_H (q < 0.1) in addition to significantly (q < 0.05) decreased abundance of Evtepia, Schaedlerella, Faecalibaculum, and Lachnoclostridium_B. Likewise, key metabolites modulated by INU treatment also vary among sexes (Figure 2C). Considerable variation (q < 0.1) in metabolites abundance pattern is exhibited only in males, which includes a reduction in metabolites including trimethylamine, ethanol, thymine, acetoin, butyrate, 3-hydroxyisobutyrate, 3-methyl-2-oxovalerate, malonate, threonine, methionine, lactate, leucine, xanthine, total bile acids (TBA) and cholesterol. Though insignificant, trimethylamine also follows a reducing trend in females. Subsequently, to predict and assess modulations in the metabolic processes originating from differences in the gut microbiome in both sexes, we execute and analyze experimentally elucidated MetaCyc pathway database. As summarized in Figure 2D, overall, in line with metabolomics data, the MeTaCyc functional pathways also modulated differently in males and females; however, only females show significantly (q < 0.05) affected pathways including upregulation of anhydromuropeptides recycling and GDP-D-glycero-α-D-manno-heptose biosynthesis together with downregulation of heme biosynthesis I (aerobic), fatty acid β-oxidation, superpathway of Clostridium acetobutylicum acidogenic fermentation and pyruvate fermentation to butanoate.
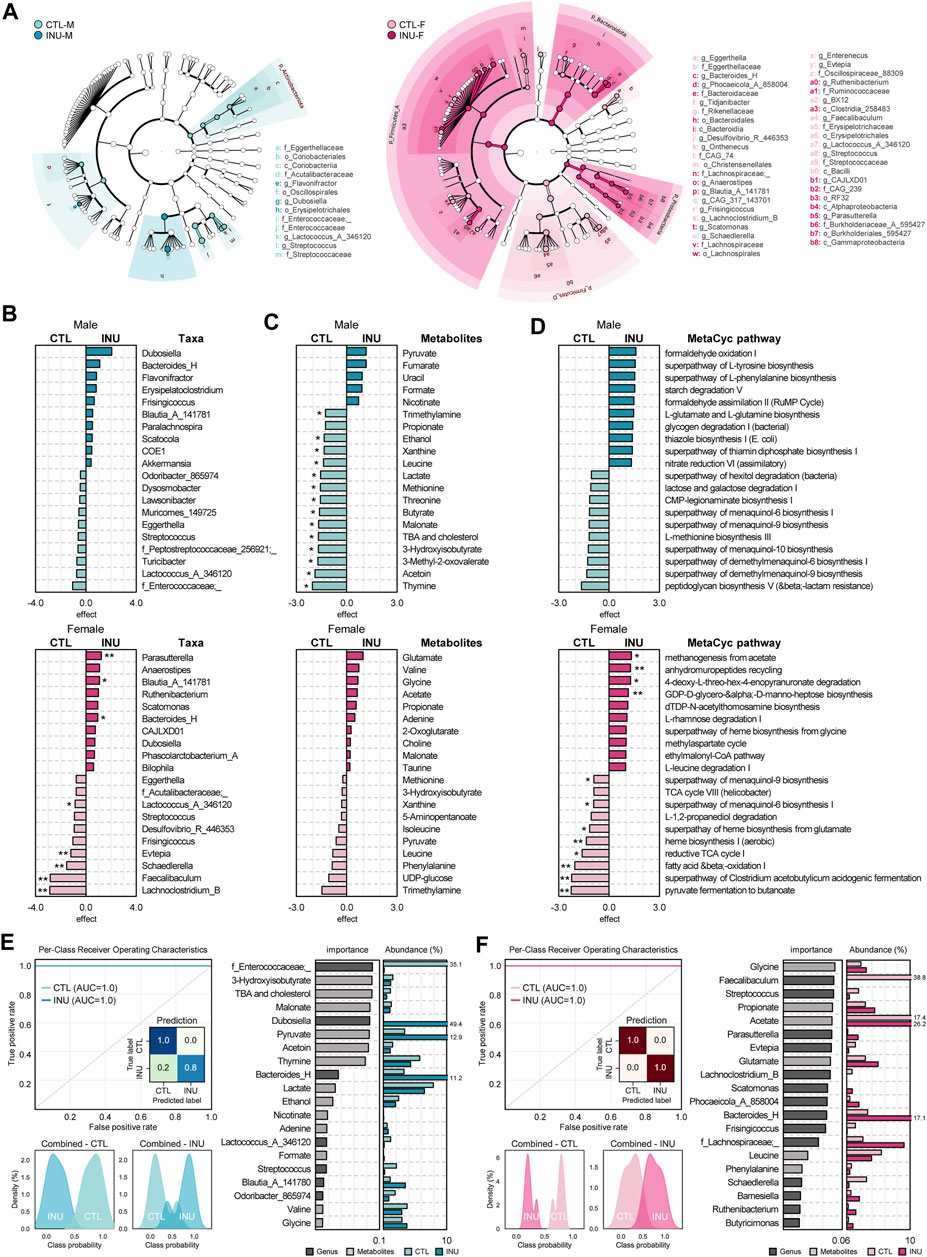
FIGURE 2. Sex-dependent disparities in the effects of inulin on unique features of microbiome, metabolome, and metabolic pathways. (A) Bacterial composition differences between groups were detected using LEfSe analysis (LDA >3.0) and depicted in a cladogram. Top 10 (B) taxa, (C) metabolites, and (D) MetaCyc pathways for each sex determined using ALDEx2 (* and ** indicate q < 0.1, and q < 0.05 respectively). Random forest prediction between groups in each (E) male and (F) female using the combined abundance data of bacterial genera and metabolites. Receiver Operating Characteristic (ROC) curve and prediction rates heatmap represents the classification accuracy, and probability histograms, respectively along with top 20 most strongly predictive genera and metabolites by relative importance score and their average abundance (%) for each group.
We subsequently also execute machine-learning algorithm based on random-forest prediction model of integrated microbiome and metabolite profiles, which clearly predicts differences in CTL vs. INU groups among both sexes. The top 20 strongly predictive genera and metabolites each for males and females are presented in Figures 2E, F. Among males, f_Enterococcaceae;_, Streptococcus, Odoribacter_865974, Lactococcus_A_346120, 3-hydroxyisobutyrate, thymine, acetoin, lactate, ethanol, valine, TBA and cholesterol are more predictive of CTL group, whereas, Dubosiella, Bacteroides_H, Blautia_A_141780, pyruvate, adenine, and glycine are suggestive for INU group. Similarly, for females, Faecalibaculum, Streptococcus, Evtepia, Lachnoclostridium_B, Frisingicoccus, Schaedlerella, leucine, and phenylalanine predict CTL while Parasutterella, Scatomonas, Bacteroides_H, f_Lachnospiraceae;_, Barnesiella, Ruthenibacterium, Butyricimonas, glycine, propionate, acetate, and glutamate predict post INU intervention. Overall, these data infer that inulin modulates the gut microbiome structure and its functional metabolic pathways more prominently in females while its prebiotic effects on fecal metabolites are more marked in males.
3.3 Integrated co-regulation networks reveal sex-specific effects of inulin on microbiome, metabolome, and physiological responses
The variations in the metagenome (Figure 3A) and metabolome (Figure 3B) post-inulin intervention is evaluated using 2-way ANOVA using Group (CTL vs. INU) and Sex (males vs. females) as two independent factors as well using the interaction effects among these two factors (Group x Sex). Bacteroides_H is the only taxa significantly influenced by group alone. In addition to the group, the interaction effects show modulation in the abundance of Parasutterella, Blautia_A, Ruthenibacterium, and f_Lachnospiraceae. Turcibacter and f_Enterococcaceae are only affected by sex and group-sex interaction effects, while Bilophila is marginally influenced by interaction effects. The abundance of Faecalibaculum and Schaedlerella is affected by both factors and their interaction. From a metabolome viewpoint, the abundance of phenylalanine is solely explained by group, while glutamate, acetate, and propionate are influenced by group and interaction effects. The variation in glycine, TBA, and cholesterol is governed by group and sex, while the abundance of valine, lysine, and 5-aminopentanoate is only explained by sex.
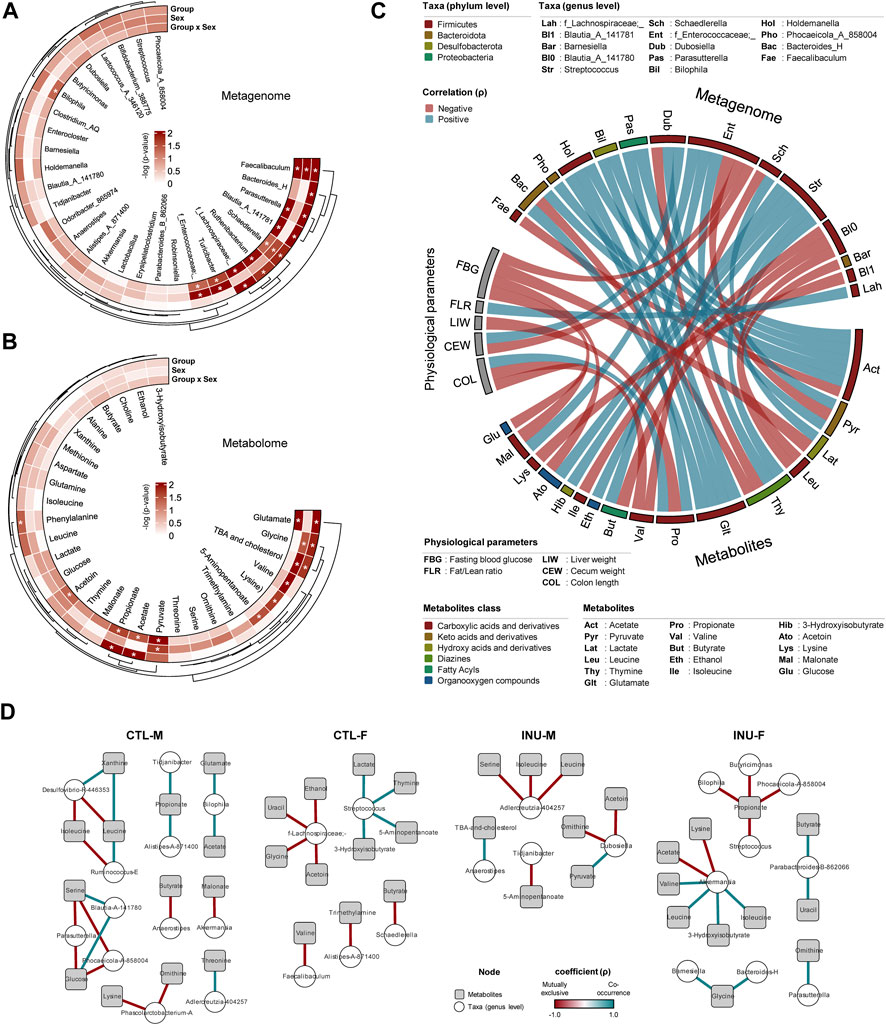
FIGURE 3. Inulin differently modulates gut microbiome-metabolome interactions together with physiological responses in male versus female mice. Circular heatmap shows differences of dominant (A) bacterial genera and (B) metabolites by treatment group and sex determined using two-way ANOVA models. (C) Correlation between most abundant 20 genera, 20 metabolites, and physiological parameters (Spearman’s rank correlation coefficient (ρ) > 0.55). (D) Bacterial genera-metabolites correlation network of each group. Each node represents one genus (white circle) and metabolites (gray rectangle), and only significant links are shown (Spearman’s rank correlation coefficient (ρ) > 1.0, Benjamini–Hochberg corrected p-value <0.05).
The Spearman correlation network analyses reveal the intricate relationship between gut metagenome, metabolome, and host physiological responses associated with inulin intervention (Figure 3C). The details of physiological parameters evaluated for the current study are presented in Supplementary Figure S1. Intestinal tissue measures i.e., total intestine length, colon length, cecum weight, and liver weight were adjusted by normalization with bodyweight. The data show that fasting blood glucose is negatively correlated with f_Enterococcaceae;_, lactate, malonate, and acetoin and is significantly higher (p < 0.048) for the INU-M group (Supplementary Figure S1). Fat/lean mass ratio correlates positively only with f_Lachnospiraceae;_. Liver weight is negatively correlated with propionate. Cecum weight, which is consistently higher for both sexes of the INU group (Supplementary Figure S1), is positively and negatively associated with Dubosiella and f_Enterococcaceae;_, respectively. Colon length is positively associated with propionate but negatively with valine and glucose. Acetate and glutamate are directly associated with Bacteroides_H, Phocaeicola_A, Bilophila, and Holdemanella, the latter being also positively linked to propionate. Lactate is positively associated with Streptococcus only. The abundance of Blautia_A_141780 is negatively linked to leucine, butyrate, and isoleucine. Blautia_A_141781 and Faecalibaculum inversely correlate with lysine and acetate, respectively.
Further insights into microbiome-metabolome interplay are assessed using cooccurrence networks within each group (Figure 3D). In the CTL-M group, Desulfovibrio-R-446353 exhibits a positive association with xanthine and an inverse association with leucine and isoleucine. The presence of serine and glucose directly correlates with Blautia-A-141780 but inversely with Parasutterella and Phocoaeicola-A-858004. In the CTL-F group, the abundance of f-Lachnospiraceae;_ is mutually exclusive for the presence of ethanol, uracil, glycine, and acetoin, while the abundance of Streptococcus co-occurs with the presence of lactate, thymine, 5-aminopentanoate, and 3-hydroxyisobutyrate. INU treatment marks a change of association between microbiome and metabolites for both sexes. Anaerostipes, which was negatively associated with butyrate in the CTL-M group, is positively associated with TBA and cholesterol in the INU-M group. Besides, serine, isoleucine, and leucine show a negative association with Adlercreutzia-404257 post-INU treatment. Correlation network analyses for INU-F mainly center around Akkermansia and propionate. Akkermansia is positively associated with valine, leucine, 3-hydroisobutyrate, and isoleucine while negatively with acetate and lysine. Propionate abundance is inversely associated with Bilophila, Butyricimonas, Streptococcus, and Phocaeicola A-858094. Altogether, inulin significantly modulates the gut bacterial architecture and associated pool of metabolites but with distinct arrays of microbiome-metabolome cooccurrence in males versus females.
4 Discussion
Rodent models are invariably used to validate as well as understand the mechanisms and health effects of functional foods including dietary fibers. Given the increasing awareness of the sex-based differences in microbiome, metabolism and immunity, the validation of dietary fibers merits evaluation in rodents of both sexes to establish the universality of prebiotic effects. Accordingly, we herein apply ecosystem-wide gut microbiome and metabolome profiling to assess the prebiotic attributes of inulin fiber in modulating the microbiome-metabolome arrays in western-style diet-fed C57BL/6 aged mice carrying human gut microbiota wherein we find that these effects vary between males and females, further emphasizing the consideration and inclusion of sex-effects for extrapolation of health effects of dietary fibers.
Age, along with sex, is a confounding factor in gut microbiome studies due to the transition of the intestinal microbial community towards ‘dysbiosis’ as individuals age. While inulin had demonstrated its responsiveness in elderly population (55–80 years) by promoting abundance of beneficial taxa like Bifidobacterium, Parabacteroides and Anaerostipes together with reductions in fecal isobutyric acid levels (Kiewiet et al., 2021), emerging studies have revealed its age-dependent response in modulating gut microbiome and SCFAs composition. Recent preclinical studies have demonstrated diminished potential of inulin-induced microbiome shifts with age, which includes less diverse microbiomes compared to adult mice, age-dependent decline of fecal propionate acids and no significant effects on systemic inflammatory and gut health markers (Muthyala et al., 2022; Hutchinson et al., 2023). Nevertheless, future studies can overcome these deficits in the efficacy of inulin by investigating the dose-dependency of inulin supplementation and/or incorporating dietary fibers earlier in life, before reaching old age. In our current investigation, we further emphasize that sex is an important contributing factor that should be taken into account when targeting precision nutrition in old age.
Overall, inulin has insignificant effect on the combined estimators of microbial diversity and richness in either sex. A previous study also indicated no such effect on fecal community diversity in both sexes of rats fed with oligofructans supplemented standard chow diet (Shastri et al., 2015). However, another study demonstrated higher Shannon diversity in female gnotobiotic mice than males after fecal transplantation with male human donors, which also partly aligns with our study wherein CTL-F show high phylogenetic diversity than CTL-M even though there are no visible differences in Shannon diversity (Wang et al., 2020). This further posits that ecologically distinct environments between males and females eventually influence their gut microbiomes. For instance, the driving effect of sex in shaping the microbiota colonization pattern in gnobiotic mice after fecal transplantation from human donor on inulin-enriched diet had been acknowledged before (Wang et al., 2016). Although the precise mechanisms underlying sexual dimorphism in microbiome remain unclear, sex-specific differences in immune responses and hormones, and their direct or indirect effects on gut microbes have begun to unravel these phenomena (Bolnick et al., 2014). Sex hormones can interact with the gut microbiome in a bidirectional manner (Yoon and Kim, 2021). Firstly, the deconjugation of estrogens and androgens by gut microbiome alters their abundance profile in the intestine and systemic circulation. Secondly, estrogens and androgens demonstrate varying effects on the immune response, which in turn impacts the composition of the gut microbiota and intestinal permeability differently in males and females. High levels of estradiol in females and testosterone in males were associated with diverse gut microbiome (Shin et al., 2019). Thus, it is reasonable to assume that the alteration in microbial colonization patterns mediated by host sex hormones and immune system also modulate diet-microbe interactions in the gut ecosystem in response to intake of dietary fibers.
Sex-specific microbiome alterations in vertebrates (humans, laboratory fish and mice) in response to diet have been reported earlier (Bolnick et al., 2014). We observe higher Bacteroidota abundance post-INU treatment in females, which is consistent with previous findings (Shastri et al., 2015). These selective effects might also be ascribed to differences in the availability of peptidyl-nitrogen in the distal gut of males and females, which in turn act as a source of ammonia to selectively stimulate certain bacterial taxa viz., Prevotella and Barnesiella within Bacteroidota phylum (Kalmokoff et al., 2015; Shastri et al., 2015). Nonetheless, within the same phylum, we also observe an abundance of genus Bacteroides as a function of the diet without any influence of sex. Inulin-induced increase in Bacteroides is closely associated with its ingenious ability to catabolize complex polysaccharides (Du et al., 2020; Shao et al., 2020). Likewise, increased Parasutterella and Dubosiella abundance after inulin intake with their direct association with SCFAs levels has been reported before (Li et al., 2020). Conversely, in line with this study, sex dependency of Turicibacter and Enterococcus genera in C57BJ/6 mice had been documented (Elderman et al., 2018). Although Faecalibaculum and Lactococcus abundance is decreased more markedly in females, both taxa have been reported to decline in both sexes of mice fed on high-amylose wheat diet (Lim et al., 2021). However, such sex-specific effects are strikingly evident at high fiber doses, suggesting dose-dependency as another variable for exerting universal benefits. Moreover, inulin mediated sex-specific impacts gut microbiome is not only confined in males but also reported in other experimental animal models like adult Wister rats (Massot-Cladera et al., 2020) and Drosophila melanogaster (Dong et al., 2019). Besides, inulin supplementation to neonatal piglets had also shown sexual dimorphism in immune development, with females exhibiting higher expression of E-cadherin, CD172+ and CD4+ antigen-presenting cells compared to males in the distal jejunum (Christoforidou et al., 2019). Pronounced restructuring in the microbiome of females compared to males also reflects in the upregulation of key pathways such as anhydromuropeptides recycling and heptose biosynthesis, which are associated with the formation of new peptidoglycan and lipopolysaccharide moieties, respectively. Besides, some pathways exhibit considerable connection with metabolites’ abundance in both sexes such as reduced pyruvate fermentation to butanoate pathway with reduced butyrate, increased L-leucine degradation with reduced leucine, and reduced L-methionine biosynthesis with reduced methionine.
Even though the gut microbiome and metabolome are modulated differently by inulin among sexes in this study, the machine-learning approach predicts prebiotic traits conferred by inulin in western-style HFD fed mice. Inulin suppresses the abundance of diet-induced obesity-linked taxa reported earlier such as Enterococcus, Streptococcus (Zhang et al., 2017), Faecalibaculum (Brennan and Garrett, 2019) and Odoribacter (Li et al., 2020; Shao et al., 2020) while fostering certain benign taxa which are suppressed under high-fat dietary regimens such as Dubosiella, Bacteroides, Butyricimonas and Parasutterella, which are known to be enhanced by short-chain and long-chain inulin-type fructans (Li et al., 2020) and neoagarotetraose, a marine oligosaccharide (Zhang et al., 2022). Barnesiella and Lachnospiraceae are also documented to be increased by inulin and xylooligosaccharides incorporation in HFD-fed male mice, respectively (Du et al., 2020). Phocaeicola, a strict anaerobe discovered recently in the human gut ecosystem, could be involved in prebiotic metabolisms as it encodes glycosyl hydrolyses for degrading complex plant-derived polysaccharides and producing propionate and succinate (Wang et al., 2021; Borgonovi et al., 2022). Although these studies were conducted only in males, it still infers that inulin promotes beneficial taxa in a sex-dependent manner, warranting the validation of preclinical/clinical studies to understand sex-related differences for precision nutritional targeting. Similar outputs are also observed in metabolite profiles with suppression of obesity-associated harmful metabolites including malonate, total bile acids, isobutyrate, isovalerate, and trimethylamine together with the promotion of beneficial metabolites including propionate, acetate, glutamate, and glycine, suggesting the sex-specific functional consequences of the microbiome.
Reduction in branched-chain fatty acids (e.g., isobutyrate and isovalerate) in males’ INU group implies reduced toxic effects on the gut as protein fermentation favors the promotion of these acids under limited supply of fermentable fibers (Koh et al., 2016). Moreover, higher levels of these acids together with malonate are seen in T1D patients (Del Chierico et al., 2022). Reduction in total bile acids is another positive feature of fiber as a higher bile acid pool is associated with the risk of colorectal cancer (Degirolamo et al., 2011). A decrease in trimethylamine (TMA) indicates a favorable outcome as T2D patients are found to have elevated plasma levels of TMA-derived trimethylamine-N-oxide (TMAO) (Tang et al., 2017). The abundance of pyruvate is in concert with previous findings whereby high-fat feeding impaired energy metabolism by causing the metabolic disturbance in the tricarboxylic acid (TCA) cycle associated with competitive inhibition of glucose conversion into pyruvate and energy, which in turn was ameliorated by long-term supplementation of dietary polysaccharides (Chen et al., 2018). The abundance of acetoin is also altered by inulin, implying the remodeling of host energy metabolisms because acetoin (another intermediate product of the TCA cycle) concentration is also affected by aerobic and anaerobic microbes during intestinal fermentation processes (Peng et al., 2020). Methionine, an essential amino acid, is significantly decreased in males compared to the control. Despite its crucial role in regulating cellular function for normal growth and development, excessive methionine levels as typically found in the western diet have been shown to alter the intestine microbiome unfavorably by promoting gut pathogens and compromising mucosal structural integrity (Miousse et al., 2017). Contrarily, dietary restriction of methionine in males correlated with enhanced abundance of Bacteroidaceae and Verrucoccaceae (Wallis et al., 2020); respective members namely, Bacteroides and Akkermansia of these families also show an increasing trend in males in the current study. Differences in gut microbiome shift the SCFAs abundance differently among sexes, with butyrate decreasing significantly in males while acetate and propionate increase insignificantly in females. Differences in gut microbiota-associated SCFAs production in male and female mice have also been reported previously following antibiotic exposure (Gao et al., 2019) and fiber supplementation (Zhang et al., 2020; Hutchinson et al., 2023).
The disparate responses of gut microbiome-metabolome interaction to diet and sex are evident based on correlational networks, and this interaction also affected physiological responses. The positive association of fat/lean mass ratio with Lachnospiraceae appears to be strain-dependent as some studies link its positive association with obesity (Kameyama and Itoh, 2014) while some reported otherwise (Companys et al., 2021). The positive and negative association of propionate with colon length and liver weight, respectively, complies with earlier studies whereby propionate repressed hepatic lipogenesis (Weitkunat et al., 2016) and ameliorated dextran-sulfate-induced colitis (Tong et al., 2016). An increase in lactic-acid-producing genus Streptococcus after high-fat feeding is well documented (Liu et al., 2018), which explains its direct association with lactate as observed in our study. Besides, microbiome-metabolome interplay exhibits considerable modulation before and after dietary intervention for males and females. For instance, the relationship of Anaerostipes with butyrate and TBA in males pre- and post-inulin regimen indicates inulin-mediated modulation in microbiome and metabolomic pools. Amongst all, alterations in amino acid metabolism exhibit the widest arrays of correlations with the microbiome. Notably, fecal branched-chain amino acids (BCAAs) such as leucine, isoleucine, and valine closely associate with different taxa including Desulfovibrio, Ruminococcus, Faecalibaculum, Adlercreutzia, and Akkermansia. Cumulative effects of gut bacterial metabolic activity resulted in a net increase in the abundance of BCAAs in the CTL group, which aligns well with earlier studies reporting increased fecal BCAAs by HFD-feeding (Xu et al., 2020). Circulating levels of BCAAs are reported to increase in T2D cases wherein insulin resistance is promoted via activation of the leucine-induced mTORC1 pathway (Lynch and Adams, 2014). The promotion of glycine in both sexes and its association with Barnesiella and Bacteroides in INU-F group also hints beneficial effect on the host as its supplementation attenuated insulin resistance and oxidative stress in sucrose-fed rats (El-Hafidi et al., 2018).
In summary, our study corroborates how western-style diet induces sex-specific changes in the gut microbiome-metabolome arrays which appears to correlate with impaired gut and metabolic health. Incorporation of inulin, however, ameliorates these fingerprints by fostering beneficial bacterial taxa together with metabolites which are linked with a healthier metabolic state and function of the host. Importantly, inulin-mediated prebiotic effects in microbiome-metabolome arrays differ significantly between sexes, further emphasizing the need to consider sexual dimorphism in future diet-microbiome studies—which has otherwise been often ignored to date—to validate and comprehend the health effects of functional foods as well as for the development of personalized nutrition strategies.
Data availability statement
All datasets generated for this study are included in the manuscript/Supplementary Materials. All the raw sequencing datasets have been submitted to the NCBI Sequence Read Archive (SRA) public repository database under SRA BioProject number PRJNA902407.
Ethics statement
The animal study was reviewed and approved by Florida State University Institutional Animal Care and Use Committee.
Author contributions
SK performed experiments, analyzed data, wrote the manuscript; GP analyzed data, revised and edited manuscript; BW performed metabolomics assays; RN conceived the idea, supervised the study, analyzed and interpreted data, revised and edited the manuscript. All authors contributed to the article and approved the submitted version.
Funding
This work was supported by funding from the Pulse Crop Health Initiative program of the United States Department of Agriculture (USDA-ARS, Accession No. 440658) to RN. The findings and conclusions in this publication have not been formally disseminated by the USDA and should not be construed to represent any agency determination or policy. The Florida State University startup funds and FSU Council on Research and Creativity (CRC) First-Year Assistant Professor grant award (to RN) are also duly acknowledged. BW would like to thank the National Science Foundation (NSF; Grant No. 2245530) for support in metabolomics studies.
Acknowledgments
The authors wish to thank all the lab members, the Institutional Animal Care and Use Committees members, and fellow colleagues for their help and cooperation in these studies. The help provided by Ngan Nguyen (North Carolina A&T State University) in metabolomics analysis is also appreciated.
Conflict of interest
The authors declare that the research was conducted in the absence of any commercial or financial relationships that could be construed as a potential conflict of interest.
Publisher’s note
All claims expressed in this article are solely those of the authors and do not necessarily represent those of their affiliated organizations, or those of the publisher, the editors and the reviewers. Any product that may be evaluated in this article, or claim that may be made by its manufacturer, is not guaranteed or endorsed by the publisher.
Supplementary material
The Supplementary Material for this article can be found online at: https://www.frontiersin.org/articles/10.3389/fmolb.2023.1182643/full#supplementary-material
References
Ahmadi, S., Nagpal, R., Wang, S., Gagliano, J., Kitzman, D. W., Soleimanian-Zad, S., et al. (2019). Prebiotics from acorn and sago prevent high-fat-diet-induced insulin resistance via microbiome–gut–brain axis modulation. J. Nutr. Biochem. 67, 1–13. doi:10.1016/j.jnutbio.2019.01.011
Benítez-Páez, A., Hess, A. L., Krautbauer, S., Liebisch, G., Christensen, L., Hjorth, M. F., et al. (2021). Sex, food, and the gut microbiota: Disparate response to caloric restriction diet with fiber supplementation in women and men. Mol. Nutr. Food Res. 65, 2000996. doi:10.1002/mnfr.202000996
Bokulich, N. A., Kaehler, B. D., Rideout, J. R., Dillon, M., Bolyen, E., Knight, R., et al. (2018). Optimizing taxonomic classification of marker-gene amplicon sequences with QIIME 2’s q2-feature-classifier plugin. Microbiome 6, 90–17. doi:10.1186/s40168-018-0470-z
Bolnick, D. I., Snowberg, L. K., Hirsch, P. E., Lauber, C. L., Org, E., Parks, B., et al. (2014). Individual diet has sex-dependent effects on vertebrate gut microbiota. Nat. Commun. 5, 4500. doi:10.1038/ncomms5500
Bolyen, E., Rideout, J. R., Dillon, M. R., Bokulich, N. A., Abnet, C. C., Al-Ghalith, G. A., et al. (2019). Reproducible, interactive, scalable and extensible microbiome data science using QIIME 2. Nat. Biotechnol. 37, 852–857. doi:10.1038/s41587-019-0209-9
Borgonovi, T. F., Salgaço, M. K., Oliveira, G. L. V. de, Carvalho, L. A. L. de, Pinheiro, D. G., Todorov, S. D., et al. (2022). Functional fermented milk with fruit pulp modulates the in vitro intestinal microbiota. Foods 11, 4113. doi:10.3390/foods11244113
Brennan, C. A., and Garrett, W. S. (2019). Fusobacterium nucleatum—Symbiont, opportunist and oncobacterium. Nat. Rev. Microbiol. 17, 156–166. doi:10.1038/s41579-018-0129-6
Callahan, B. J., McMurdie, P. J., Rosen, M. J., Han, A. W., Johnson, A. J. A., and Holmes, S. P. (2016). DADA2: High-resolution sample inference from Illumina amplicon data. Nat. Methods 13, 581–583. doi:10.1038/nmeth.3869
Chen, M., Lu, B., Li, Y., Wang, Y., Zheng, H., Zhong, D., et al. (2018). Metabolomics insights into the modulatory effects of long-term compound polysaccharide intake in high-fat diet-induced obese rats. Nutr. Metab. 15, 8–15. doi:10.1186/s12986-018-0246-2
Christoforidou, Z., Mora Ortiz, M., Poveda, C., Abbas, M., Walton, G., Bailey, M., et al. (2019). Sexual dimorphism in immune development and in response to nutritional intervention in neonatal piglets. Front. Immunol. 10, 2705. doi:10.3389/fimmu.2019.02705
Chu, J. R., Kang, S-Y., Kim, S-E., Lee, S-J., Lee, Y-C., and Sung, M-K. (2019). Prebiotic UG1601 mitigates constipation-related events in association with gut microbiota: A randomized placebo-controlled intervention study. World J. Gastroenterol. 25, 6129–6144. doi:10.3748/wjg.v25.i40.6129
Clark, M., Centner, A. M., Ukhanov, V., Nagpal, R., and Salazar, G. (2022). Gallic acid ameliorates atherosclerosis and vascular senescence and remodels the microbiome in a sex-dependent manner in ApoE−/− mice. J. Nutr. Biochem. 110, 109132. doi:10.1016/j.jnutbio.2022.109132
Companys, J., Gosalbes, M. J., Pla-Pagà, L., Calderón-Pérez, L., Llauradó, E., Pedret, A., et al. (2021). Gut microbiota profile and its association with clinical variables and dietary intake in overweight/obese and lean subjects: A cross-sectional study. Nutrients 13, 2032. doi:10.3390/nu13062032
Degirolamo, C., Modica, S., Palasciano, G., and Moschetta, A. (2011). Bile acids and colon cancer: Solving the puzzle with nuclear receptors. Trends Mol. Med. 17, 564–572. doi:10.1016/j.molmed.2011.05.010
Del Chierico, F., Conta, G., Matteoli, M. C., Fierabracci, A., Reddel, S., Macari, G., et al. (2022). Gut microbiota functional traits, blood PH, and anti-GAD antibodies concur in the clinical characterization of T1D at onset. Int. J. Mol. Sci. 23, 10256. doi:10.3390/ijms231810256
Dominianni, C., Sinha, R., Goedert, J. J., Pei, Z., Yang, L., Hayes, R. B., et al. (2015). Sex, body mass index, and dietary fiber intake influence the human gut microbiome. PloS One 10, e0124599. doi:10.1371/journal.pone.0124599
Dong, Y., Sun, H., Yang, W., Ma, S., Du, B., and Xu, H. (2019). The effect of inulin on lifespan, related gene expression and gut microbiota in InR p5545/TM3 mutant Drosophila melanogaster: A preliminary study. Nutrients 11, 636. doi:10.3390/nu11030636
Du, H., Zhao, A., Wang, Q., Yang, X., and Ren, D. (2020). Supplementation of inulin with various degree of polymerization ameliorates liver injury and gut microbiota dysbiosis in high fat-fed obese mice. J. Agric. Food Chem. 68, 779–787. doi:10.1021/acs.jafc.9b06571
El-Hafidi, M., Franco, M., Ramírez, A. R., Sosa, J. S., Flores, J. A. P., Acosta, O. L., et al. (2018). Glycine increases insulin sensitivity and glutathione biosynthesis and protects against oxidative stress in a model of sucrose-induced insulin resistance. Oxid. Med. Cell Longev. 2018, 2101562. doi:10.1155/2018/2101562
Elderman, M., Hugenholtz, F., Belzer, C., Boekschoten, M., van Beek, A., de Haan, B., et al. (2018). Sex and strain dependent differences in mucosal immunology and microbiota composition in mice. Biol. Sex. Differ. 9, 26–18. doi:10.1186/s13293-018-0186-6
Fernandes, A. D., Reid, J. N., Macklaim, J. M., McMurrough, T. A., Edgell, D. R., and Gloor, G. B. (2014). Unifying the analysis of high-throughput sequencing datasets: Characterizing RNA-seq, 16S rRNA gene sequencing and selective growth experiments by compositional data analysis. Microbiome 2, 15–13. doi:10.1186/2049-2618-2-15
Gao, H., Shu, Q., Chen, J., Fan, K., Xu, P., Zhou, Q., et al. (2019). Antibiotic exposure has sex-dependent effects on the gut microbiota and metabolism of short-chain fatty acids and amino acids in mice. Msystems 4, e00048-19. doi:10.1128/mSystems.00048-19
Ghosh, T. S., Shanahan, F., and O’Toole, P. W. (2022). The gut microbiome as a modulator of healthy ageing. Nat. Rev. Gastroenterol. Hepatol. 19, 565–584. doi:10.1038/s41575-022-00605-x
Gratton, J., Phetcharaburanin, J., Mullish, B. H., Williams, H. R., Thursz, M., Nicholson, J. K., et al. (2016). Optimized sample handling strategy for metabolic profiling of human feces. Anal. Chem. 88, 4661–4668. doi:10.1021/acs.analchem.5b04159
Hutchinson, N. T., Wang, S. S., Rund, L. A., Caetano-Silva, M. E., Allen, J. M., Johnson, R. W., et al. (2023). Effects of an inulin fiber diet on the gut microbiome, colon, and inflammatory biomarkers in aged mice. Exp. Gerontol. 176, 112164. doi:10.1016/j.exger.2023.112164
Jovel, J., Dieleman, L. A., Kao, D., Mason, A. L., and Wine, E. (2018). The human gut microbiome in health and disease. Metagenomics 2018, 197–213. doi:10.1016/b978-0-08-102268-9.00010-0
Kadyan, S., Park, G., Singh, P., Arjmandi, B., and Nagpal, R. (2023). Prebiotic mechanisms of resistant starches from dietary beans and pulses on gut microbiome and metabolic health in a humanized murine model of aging. Front. Nutr. 10, 138. doi:10.3389/fnut.2023.1106463
Kalmokoff, M., Franklin, J., Petronella, N., Green, J., and Brooks, S. P. (2015). Phylum level change in the cecal and fecal gut communities of rats fed diets containing different fermentable substrates supports a role for nitrogen as a factor contributing to community structure. Nutrients 7, 3279–3299. doi:10.3390/nu7053279
Kameyama, K., and Itoh, K. (2014). Intestinal colonization by a Lachnospiraceae bacterium contributes to the development of diabetes in obese mice. Microbes Environ. 29, 427–430. doi:10.1264/jsme2.ME14054
Katoh, K., Misawa, K., Kuma, K., and Miyata, T. (2002). Mafft: A novel method for rapid multiple sequence alignment based on fast fourier transform. Nucleic Acids Res. 30, 3059–3066. doi:10.1093/nar/gkf436
Kiewiet, M. B., Elderman, M. E., El Aidy, S., Burgerhof, J. G., Visser, H., Vaughan, E. E., et al. (2021). Flexibility of gut microbiota in ageing individuals during dietary fiber long-chain inulin intake. Mol. Nutr. Food Res. 65, 2000390. doi:10.1002/mnfr.202000390
Koh, A., De Vadder, F., Kovatcheva-Datchary, P., and Bäckhed, F. (2016). From dietary fiber to host physiology: Short-chain fatty acids as key bacterial metabolites. Cell 165, 1332–1345. doi:10.1016/j.cell.2016.05.041
Li, C., and Zhang, X. (2022). Current in vitro and animal models for understanding foods: Human gut–microbiota interactions. J. Agric. Food Chem. 70, 12733–12745. doi:10.1021/acs.jafc.2c04238
Li, L-L., Wang, Y-T., Zhu, L-M., Liu, Z-Y., Ye, C-Q., and Qin, S. (2020). Inulin with different degrees of polymerization protects against diet-induced endotoxemia and inflammation in association with gut microbiota regulation in mice. Sci. Rep. 10, 978. doi:10.1038/s41598-020-58048-w
Lim, S. M., Choo, J. M., Li, H., O’rielly, R., Carragher, J., Rogers, G. B., et al. (2021). A high amylose wheat diet improves gastrointestinal health parameters and gut microbiota in male and female mice. Foods 10, 220. doi:10.3390/foods10020220
Liu, M., Ma, L., Chen, Q., Zhang, P., Chen, C., Jia, L., et al. (2018). Fucoidan alleviates dyslipidemia and modulates gut microbiota in high-fat diet-induced mice. J. Funct. Foods 48, 220–227. doi:10.1016/j.jff.2018.07.006
Lundberg, R. (2019). Humanizing the gut microbiota of mice: Opportunities and challenges. Lab. Anim. 53, 244–251. doi:10.1177/0023677218787554
Lynch, C. J., and Adams, S. H. (2014). Branched-chain amino acids in metabolic signalling and insulin resistance. Nat. Rev. Endocrinol. 10, 723–736. doi:10.1038/nrendo.2014.171
Mallick, H., Rahnavard, A., McIver, L. J., Ma, S., Zhang, Y., Nguyen, L. H., et al. (2021). Multivariable association discovery in population-scale meta-omics studies. PLoS Comput. Biol. 17, e1009442. doi:10.1371/journal.pcbi.1009442
Massot-Cladera, M., Azagra-Boronat, I., Franch, À., Castell, M., Rodríguez-Lagunas, M. J., and Pérez-Cano, F. J. (2020). Gut health-promoting benefits of a dietary supplement of vitamins with inulin and acacia fibers in rats. Nutrients 12, 2196. doi:10.3390/nu12082196
McDonald, D., Jiang, Y., Balaban, M., Cantrell, K., Zhu, Q., Gonzalez, A., et al. (2022). Greengenes2 enables a shared data universe for microbiome studies. bioRxiv, 2022-12.
Miousse, I. R., Pathak, R., Garg, S., Skinner, C. M., Melnyk, S., Pavliv, O., et al. (2017). Short-term dietary methionine supplementation affects one-carbon metabolism and DNA methylation in the mouse gut and leads to altered microbiome profiles, barrier function, gene expression and histomorphology. Genes Nutr. 12, 22–13. doi:10.1186/s12263-017-0576-0
Munley, J. A., Kelly, L. S., Pons, E. E., Kannan, K. B., Coldwell, P. S., Whitley, E. M., et al. (2022). Multicompartmental traumatic injury and the microbiome: Shift to a pathobiome. J. Trauma Acute Care Surg. 94, 15–22. doi:10.1097/TA.0000000000003803
Muthyala, S. D. V., Shankar, S., Klemashevich, C., Blazier, J. C., Hillhouse, A., and Wu, C-S. (2022). Differential effects of the soluble fiber inulin in reducing adiposity and altering gut microbiome in aging mice. J. Nutr. Biochem. 105, 108999. doi:10.1016/j.jnutbio.2022.108999
Nagpal, R., Indugu, N., and Singh, P. (2021). Distinct gut microbiota signatures in mice treated with commonly used food preservatives. Microorganisms 9, 2311. doi:10.3390/microorganisms9112311
Nagpal, R., Kumar, M., Yadav, A., Hemalatha, R., Yadav, H., Marotta, F., et al. (2016). Gut microbiota in health and disease: An overview focused on metabolic inflammation. Benef. Microbes 7, 181–194. doi:10.3920/bm2015.0062
Nagpal, R., Neth, B. J., Wang, S., Craft, S., and Yadav, H. (2019). Modified Mediterranean-ketogenic diet modulates gut microbiome and short-chain fatty acids in association with Alzheimer’s disease markers in subjects with mild cognitive impairment. EBioMedicine 47, 529–542. doi:10.1016/j.ebiom.2019.08.032
Nagpal, R., Newman, T. M., Wang, S., Jain, S., Lovato, J. F., and Yadav, H. (2018a). Obesity-linked gut microbiome dysbiosis associated with derangements in gut permeability and intestinal cellular homeostasis independent of diet. J. Diabetes Res. 2018, 3462092. doi:10.1155/2018/3462092
Nagpal, R., Wang, S., Solberg Woods, L. C., Seshie, O., Chung, S. T., Shively, C. A., et al. (2018b). Comparative microbiome signatures and short-chain fatty acids in mouse, rat, non-human primate, and human feces. Front. Microbiol. 9, 2897. doi:10.3389/fmicb.2018.02897
Nearing, J. T., Douglas, G. M., Hayes, M. G., MacDonald, J., Desai, D. K., Allward, N., et al. (2022). Microbiome differential abundance methods produce different results across 38 datasets. Nat. Commun. 13, 342. doi:10.1038/s41467-022-28034-z
Nguyen, T. L. A., Vieira-Silva, S., Liston, A., and Raes, J. (2015). How informative is the mouse for human gut microbiota research? Dis. Model Mech. 8, 1–16. doi:10.1242/dmm.017400
Peng, Q., Zhao, X., Wen, J., Huang, M., Zhang, J., and Song, F. (2020). Transcription in the acetoin catabolic pathway is regulated by AcoR and CcpA in Bacillus thuringiensis. Microbiol. Res. 235, 126438. doi:10.1016/j.micres.2020.126438
Régnier, M., Van Hul, M., Roumain, M., Paquot, A., de Wouters d’Oplinter, A., Suriano, F., et al. (2023). Inulin increases the beneficial effects of rhubarb supplementation on high-fat high-sugar diet-induced metabolic disorders in mice: Impact on energy expenditure, Brown adipose tissue activity, and microbiota. Gut Microbes 15, 2178796. doi:10.1080/19490976.2023.2178796
Reider, S. J., Moosmang, S., Tragust, J., Trgovec-Greif, L., Tragust, S., Perschy, L., et al. (2020). Prebiotic effects of partially hydrolyzed guar gum on the composition and function of the human microbiota—results from the PAGODA trial. Nutrients 12, 1257. doi:10.3390/nu12051257
Reimer, R. A., Soto-Vaca, A., Nicolucci, A. C., Mayengbam, S., Park, H., Madsen, K. L., et al. (2020). Effect of chicory inulin-type fructan–containing snack bars on the human gut microbiota in low dietary fiber consumers in a randomized crossover trial. Am. J. Clin. Nutr. 111, 1286–1296. doi:10.1093/ajcn/nqaa074
Saccon, T. D., Nagpal, R., Yadav, H., Cavalcante, M. B., Nunes, A. D. de C., Schneider, A., et al. (2021). Senolytic combination of Dasatinib and Quercetin alleviates intestinal senescence and inflammation and modulates the gut microbiome in aged mice. J. Gerontol. Ser. A 76, 1895–1905. doi:10.1093/gerona/glab002
Segata, N., Izard, J., Waldron, L., Gevers, D., Miropolsky, L., Garrett, W. S., et al. (2011). Metagenomic biomarker discovery and explanation. Genome Biol. 12, R60–R18. doi:10.1186/gb-2011-12-6-r60
Shao, T., Yu, Q., Zhu, T., Liu, A., Gao, X., Long, X., et al. (2020). Inulin from Jerusalem artichoke tubers alleviates hyperglycaemia in high-fat-diet-induced diabetes mice through the intestinal microflora improvement. Br. J. Nutr. 123, 308–318. doi:10.1017/S0007114519002332
Shastri, P., McCarville, J., Kalmokoff, M., Brooks, S. P., and Green-Johnson, J. M. (2015). Sex differences in gut fermentation and immune parameters in rats fed an oligofructose-supplemented diet. Biol. Sex. Differ. 6, 13–12. doi:10.1186/s13293-015-0031-0
Shin, J-H., Park, Y-H., Sim, M., Kim, S-A., Joung, H., and Shin, D-M. (2019). Serum level of sex steroid hormone is associated with diversity and profiles of human gut microbiome. Res. Microbiol. 170, 192–201. doi:10.1016/j.resmic.2019.03.003
Suzuki, Y., Ikeda, K., Sakuma, K., Kawai, S., Sawaki, K., Asahara, T., et al. (2017). Association between yogurt consumption and intestinal microbiota in healthy young adults differs by host gender. Front. Microbiol. 8, 847. doi:10.3389/fmicb.2017.00847
Tang, W. W., Wang, Z., Li, X. S., Fan, Y., Li, D. S., Wu, Y., et al. (2017). Increased trimethylamine N-oxide portends high mortality risk independent of glycemic control in patients with type 2 diabetes mellitus. Clin. Chem. 63, 297–306. doi:10.1373/clinchem.2016.263640
Tawfick, M. M., Xie, H., Zhao, C., Shao, P., and Farag, M. A. (2022). Inulin fructans in diet: Role in gut homeostasis, immunity, health outcomes and potential therapeutics. Int. J. Biol. Macromol. 208, 948–961. doi:10.1016/j.ijbiomac.2022.03.218
Tong, L., Wang, Y., Wang, Z., Liu, W., Sun, S., Li, L., et al. (2016). Propionate ameliorates dextran sodium sulfate-induced colitis by improving intestinal barrier function and reducing inflammation and oxidative stress. Front. Pharmacol. 7, 253. doi:10.3389/fphar.2016.00253
Trimigno, A., Khakimov, B., Mejia, J. L. C., Mikkelsen, M. S., Kristensen, M., Jespersen, B. M., et al. (2017). Identification of weak and gender specific effects in a short 3 weeks intervention study using barley and oat mixed linkage β-glucan dietary supplements: A human fecal metabolome study by GC-MS. Metabolomics 13, 108–113. doi:10.1007/s11306-017-1247-2
Vijay, A., Astbury, S., Le Roy, C., Spector, T. D., and Valdes, A. M. (2021). The prebiotic effects of omega-3 fatty acid supplementation: A six-week randomised intervention trial. Gut Microbes 13, 1–11. doi:10.1080/19490976.2020.1863133
Wallis, K. F., Melnyk, S. B., and Miousse, I. R. (2020). Sex-specific effects of dietary methionine restriction on the intestinal microbiome. Nutrients 12, 781. doi:10.3390/nu12030781
Wang, B., Maldonado-Devincci, A. M., and Jiang, L. (2020). Evaluating line-broadening factors on a reference spectrum as a bucketing method for NMR based metabolomics. Anal. Biochem. 606, 113872. doi:10.1016/j.ab.2020.113872
Wang, C., Li, S., Zhang, Z., Yu, Z., Yu, L., Tian, F., et al. (2021). Phocaeicola faecalis sp. nov., a strictly anaerobic bacterial strain adapted to the human gut ecosystem. Ant. Van Leeuwenhoek 114, 1225–1235. doi:10.1007/s10482-021-01595-7
Wang, J., Wang, J., Pang, X., Zhao, L., Tian, L., and Wang, X. (2016). Sex differences in colonization of gut microbiota from a man with short-term vegetarian and inulin-supplemented diet in germ-free mice. Sci. Rep. 6, 36137. doi:10.1038/srep36137
Weitkunat, K., Schumann, S., Nickel, D., Kappo, K. A., Petzke, K. J., Kipp, A. P., et al. (2016). Importance of propionate for the repression of hepatic lipogenesis and improvement of insulin sensitivity in high-fat diet-induced obesity. Mol. Nutr. Food Res. 60, 2611–2621. doi:10.1002/mnfr.201600305
Xu, J., Li, M., Zhang, Y., Chu, S., Huo, Y., Zhao, J., et al. (2020). Huangjinya black tea alleviates obesity and insulin resistance via modulating fecal metabolome in high-fat diet-fed mice. Mol. Nutr. Food Res. 64, 2000353. doi:10.1002/mnfr.202000353
Yoon, K., and Kim, N. (2021). Roles of sex hormones and gender in the gut microbiota. J. Neurogastroenterol. Motil. 27, 314–325. doi:10.5056/jnm20208
Zhang, N., Wang, Q., Lin, F., Zheng, B., Huang, Y., Yang, Y., et al. (2022). Neoagarotetraose alleviates high fat diet induced obesity via white adipocytes browning and regulation of gut microbiota. Carbohydr. Polym. 296, 119903. doi:10.1016/j.carbpol.2022.119903
Zhang, S., Yang, J., Henning, S. M., Lee, R., Hsu, M., Grojean, E., et al. (2017). Dietary pomegranate extract and inulin affect gut microbiome differentially in mice fed an obesogenic diet. Anaerobe 48, 184–193. doi:10.1016/j.anaerobe.2017.08.017
Keywords: prebiotics, inulin, fiber, gut dysbiosis, metabolites, metabolomics, microbiota, sexual dimorphism
Citation: Kadyan S, Park G, Wang B and Nagpal R (2023) Dietary fiber modulates gut microbiome and metabolome in a host sex-specific manner in a murine model of aging. Front. Mol. Biosci. 10:1182643. doi: 10.3389/fmolb.2023.1182643
Received: 09 March 2023; Accepted: 05 June 2023;
Published: 15 June 2023.
Edited by:
Qi Xianghui, Jiangsu University, ChinaReviewed by:
Wen-Sen He, Jiangsu University, ChinaKiran Veer Sandhu, University College Cork, Ireland
Copyright © 2023 Kadyan, Park, Wang and Nagpal. This is an open-access article distributed under the terms of the Creative Commons Attribution License (CC BY). The use, distribution or reproduction in other forums is permitted, provided the original author(s) and the copyright owner(s) are credited and that the original publication in this journal is cited, in accordance with accepted academic practice. No use, distribution or reproduction is permitted which does not comply with these terms.
*Correspondence: Ravinder Nagpal, rnagpal@fsu.edu