- 1Department of Dermatology and Venereology, Manisa Celal Bayar University, Manisa, Türkiye
- 2MEDICE Arzneimittel Pütter GmbH and Co. KG, Iserlohn, Germany
- 3Department of Dermatology-Venereology and Allergology, Universitätsklinikum Schleswig-Holstein, Kiel, Germany
Atopic dermatitis (AD) is a common, chronic and relapsing inflammatory skin disease with various clinical presentations and combinations of symptoms. The pathophysiology of AD is complex and multifactorial. There are several factors involved in the etiopathogenesis of AD including structural and immunological epidermal barrier defect, imbalance of the skin microbiome, genetic background and environmental factors. Alterations in structural proteins, lipids, proteases, and their inhibitors, lead to the impairment of the stratum corneum which is associated with the increased skin penetration and transepidermal water loss. The elevated serum immunoglobulin E levels and blood eosinophilia have been shown in the majority of AD patients. Type 2 T-helper cell immune pathway with increased expression of interleukin (IL)-4, IL-5, and IL-13, has an important role in the etiopathogenesis of AD. Both T cells and keratinocytes contribute to epidermal barrier impairment in AD via a dynamic interaction of cytokines and chemokines. The skin microbiome is another factor of relevance in the etiopathogenesis of AD. It has been shown that during AD flares, Staphylococcus aureus (S. aureus) colonization increased, while Staphylococcus epidermidis (S. epidermidis) decreased. On the contrary, S. epidermidis and species of Streptococcus, Corynebacterium and Propionibacterium increased during the remision phases. However, it is not clear whether skin dysbiosis is one of the symptoms or one of the causes of AD. There are several therapeutic options, targeting these pathways which play a critical role in the etiopathogenesis of AD. Although topical steroids are the mainstay of the treatment of AD, new biological therapies including IL-4, IL-13, and IL-31 inhibitors, as well as Janus kinase inhibitors (JAKi), increasingly gain more importance with new advances in the therapy of AD. In this review, we summarize the role of immunological and structural epidermal barrier dysfunction, immune abnormalities, impairment of lipids, filaggrin mutation and skin microbiome in the etiopathogenesis of AD, as well as the therapeutic options for AD and their effects on these abnormalities in AD skin.
1 Introduction
Atopic dermatitis (AD) is a common chronic and relapsing skin disease which usually occurs in the first years of life and affects ∼20% of children worldwide (Bylund et al., 2020). The prevalence of AD is increasing. in both children and adults (Fuxench et al., 2019; Lee et al., 2019). AD is characterized by chronic inflammation which is associated with animpaired immunological response and epidermal barrier dysfunction. This chronic inflammation leads to itching in AD patients due to dry skin, mechanical injury and allergic sensitization to environmental antigens (Oyoshi et al., 2009).
In general, AD is associated with other atopic comorbidities such as asthma, rhinitis, conjunctivitis, and food allergy (Paller et al., 2019a; Kim et al., 2019). The acute phase of AD is characterized by erythematous papules and vesicles, accompanied by itching. With the evolution to the chronic phase, lichenified lesions occur as a result of dermal fibrosis. The location of AD lesions varies with the age of the patient. In adults, it predominantly occurs in the skin flexures, face and extremities, while patients under 1 year of age generally present widely distributed lesions. The cheeks are usually the first affected area in infantil period (Bieber, 2022).
The most important factors in the etiopathogenesis of AD are genetic background, imbalance of the skin microbiome, environmental factors, as well as structural and immunological epidermal barrier defect which leads to transepidermal water loss (TEWL) (Figure 1). The increased TEWL which is associated with increased permeability of the stratum corneum (SC), is a characteristic finding of both lesional and non lesional skin of AD patients (Dizon et al., 2018). The magnitude of increase in TEWL is also correlated with the disease severity (Dizon et al., 2018). Dry skin which is one of the hallmarks of AD, occurs due to increased water loss and it leads to pruritus that impairs AD patients’ quality of life (Goerdt et al., 1999).
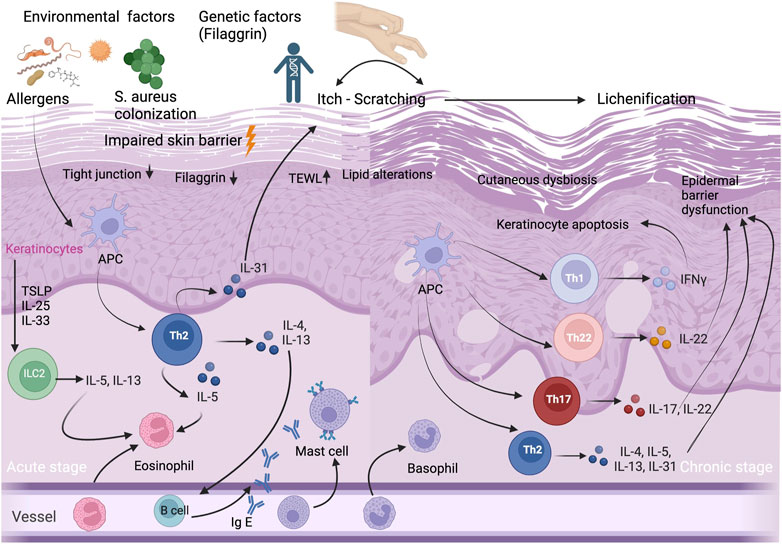
FIGURE 1. Immunopathogenesis of AD. Acute and chronic stages of AD and selected T-cell subpopulations and interleukins, other inflammatory cytokines and IgE antibodies, and selected cell populations ILC2, APCs, and eosinophils, mast cells, basophils, eosinophils and keratinocytes that play an important role in AD etiopathogenesis. Impaired skin barrier is associated with decrease in filaggrin protein, increase in TEWL, lipid alterations and cutaneous dysbiosis. Abbreviations: APC, antigen presenting cell; IFN, interferon; Ig E, immunoglobulin E; IL, interleukin; ILC2, innate lymphoid cell 2; S.aureus, Staphylococcus aureus; TEWL, transepidermal water loss; Th, T helper; TSLP, thymic stromal lymphopoietin cell.
This review will focus on the immunological and structural epidermal barrier dysfunction in AD and the role of keratinocytes, filaggrin (FLG) mutation, lipid alterations in SC, as well as skin microbiome in AD and the therapeutic options in the treatment of AD and their effects on AD skin.
The immune abnormalities in AD and their contribution to epidermal barrier dysfunction
AD is a complex, multifactorial disease in which both innate and adaptive immune system contribute to its etiopathogenesis (Figure 1). Previously, it has been thought that AD is simply a Th2-mediated inflammatory disease since the majority of patients have increased serum immunoglobulin E (IgE) levels and high numbers of circulating eosinophils (Tokura and Hayano, 2022). However, it has been demonstrated that there is a biphasic switch from Th2 to Th1 responses in both acute and chronic skin lesions of AD patients (Leung, 2000). Moreover, we know that the immunological pathway of AD is not simple and also characterized by a dysfunction in the immune system, with a dominant Th2/Th22 skewing, and variable activation of Th17/Th1 subtypes (Tokura and Hayano, 2022). In chronic AD lesions, it has been reported that there is a complex inflammation pathway between T helper cells (Th1/Th2/Th17/Th22) and hyperproliferative keratinocytes which are characterized by altered terminal differentiation (Renert-Yuval et al., 2021).
IL-4 and IL-13 are two major cytokines in the etiopathogenesis of AD and play a critical role in the differentiation of Th2 cells and the production of IgE. The increased skin expression of Th2 cytokines including IL-4, IL-5, and IL-13, has been shown in acute AD skin lesions (Oyoshi et al., 2009). These cytokines stimulate IgE antibodies and eosinophils in both skin and peripheral blood (Matsunaga and Yamauchi, 2016). IL-4 and IL-13 disrupt the epidermal barrier integrity by decrease of main terminal differentiation proteins including filaggrin, loricrin, and involucrin (Cork et al., 2006).
Additionally, IL-4 decreases the expression of genes in the epidermal differentiation complex in keratinocytes and it leads to epidermal barrier dysfunction and impaired innate skin immune system, and consequently to an increased risk of infections (Sehra et al., 2010). IL-13 overexpression has been found in both lesional and non-lesional skin of AD patients (Tsoi et al., 2019) and its levels correlate with AD disease severity (Szegedi et al., 2015; Guttman-Yassky et al., 2019; Tsoi et al., 2019). Moreover, it has been reported that IL-13 messenger RNA and protein levels are higher compared to IL-4, although both cytokines play a major role in the pathogenesis of AD (Tsoi et al., 2019). This highlights the importance of IL-13 inhibition in AD therapy.
The impairment of the epidermis due to various factors such as infectious agents, allergens or mechanical trauma may stimulate inflammation processes and leads to production of proinflammatory mediators such as TSLP (Thymic stromal lymphopoietin), which is an IL-7–like cytokine, IL-4, IL-13, IL-25, and IL-33 (Camelo et al., 2017; Klonowska et al., 2018). Thus, these increased inflammatory cytokines induce immune cell accumulation, which leads to formation of nitrogen oxide and reactive oxygen species (Mittal et al., 2014).
Various antimicrobial peptides play an important role in the innate immunity (Lehrer et al., 1993). The expression of human β-defensin 2 (hBD-2) and LL-37, is triggered by the inflammation of the skin (Frohm et al., 1997). In some AD patients, it has been reported that the decreased antimicrobial and immunomodulatory peptides such as LL-37, hBD-2, and hBD-3, are associated with skin infections (Niyonsaba et al., 2017; Nguyen et al., 2020). Host defense peptides (HDPs) induce cytokine and chemokine production and promote cell proliferation and migration (Reinholz et al., 2012; Pahar et al., 2020), and also contribute to normal epidermal barrier function against TEWL via organizing the distrubiton of tight junction protiens (Akiyama et al., 2014). The predominance of Th2-related cytokines, which is associated with an inhibitory effect against LL-37, hBD-2, and hBD-3 production, might be one of the reasons for reduced HDP levels in AD patients (Reinholz et al., 2012; Akiyama et al., 2014; Nguyen et al., 2020).
Keratinocytes play a major role in immunological and structural epidermal barrier impairment in AD
The human epidermis is a unique protective barrier against transcutaneous water loss, penetration of microbial pathogens and ingress of toxins and allergens (Marks, 2004; Elias, 2005). The epidermis consists of four layers: the stratum basale, the stratum spinosum, the stratum granulosum and the SC (Matsui and Amagai, 2015). The SC, which is the outermost layer of skin and the most important part of the epidermal barrier, comprises 20 layers of corneocytes, which are embedded in intercellular lipids (Oyoshi et al., 2009). The impairment of the SC, which protects against environmental factors, allergens and water loss, leads to epidermal barrier dysfunction in AD (Schleimer and Berdnikovs, 2017).
Keratinocytes have a critical role both in the pathophysiology of epidermal barrier defect and in the activation of the innate immune response. They receive both mechanical and inflammatory stimuli, and produce antimicrobial peptides (AMPs) and proinflammatory cytokines. In patients with AD, the epidermis is characterized by a block in terminal keratinocyte differentiation (Guttman-Yassky et al., 2009) which leads to allergen penetration through the epidermis and systemic IgE sensitization (Jensen et al., 2004), and reduced expression of skin barrier proteins including FLG, involucrin, loricrin, as well as AMPs (Ong et al., 2002; Howell et al., 2007). AMPs including LL-37, hBD-2, and hBD-3, play a major role in repairing the impaired epidermal barrier. They also show an autocrine function on keratinocytes, which produce pro-inflammatory cytokines including IL-25, IL-33, and TSLP. This inflammatory cytokines activate the innate lymphoid cell 2 (ILC2), dendritic cells and langerhans cells, and initiate the differentiation of type 2 immune response (Nakatsuji et al., 2017; Radi et al., 2022). ILC2s have an important role in homeostasis and produce a variety of cytokines, primarily IL-5 and IL13 (Mielke et al., 2013). ILC2s which present CD1a, have been found to be increased and activated in AD lesional skin (Hardman et al., 2017) (Figure 1).
TSLP which is secreted by epidermal keratinocytes, also induces other cells such as dendritic cells, T cells, as well as mast cells. Mast cells play an important role in IgE-mediated hypersensitivity and allergic diseases, as well as in AD. It has been shown that mast cells are significantly increased in AD skin (Kawakami et al., 2009). Not only keratinocytes and mast cells, but also T cells have a major role in skin barrier dysfunction via cytokine secretion (Humeau et al., 2022). Kallikreins (KLK) are proteases which have various functions including the induction of pro-inflammatory cytokine production by keratinocytes, the degradation of intercellular adhesion molecules, as well as the regulation of barrier integrity (Briot et al., 2009). In the SC of AD patients, the levels of KLK5 and KLK7 increase by the stimulation of IL-4 and IL-13 (Komatsu et al., 2007). IL-4, IL-13, IL-31, and IL-22 expressed by Th2 and Th22 cells, may disturb epidermal barrier function via scratching due to itch in AD patients (Furue et al., 2018). Although the predominance of type 2 response in AD is well-known, there is a complex pathway, including Th2, Th22, Th17 and Th1 subtypes, associated with regulatory T cell (Treg) dysfunction (Humeau et al., 2022).
In patients with AD, various abnormalities manifest not only in the SC, but also in other epidermal layers. Degradation in the cells of the stratum spinosum and stratum granulosum with a concomitant expansion of cells in the stratum basale has been described (Jensen et al., 2004). Totsuka et al. investigated the changes of structural proteins and adhesion molecules in the stratum spinosum of AD lesional skin, and the effect of Th2 cytokines including IL-4 and IL-13 on expression of these proteins (Totsuka et al., 2017). In AD lesional skin, they found decreased expression of keratin 1, keratin 10, desmoglein 1 and desmocollin 1, which is supressed by Th2 cytokines (Totsuka et al., 2017).
However, it is not certain that the epidermal skin barrier defect in lesional skin of AD is a primary factor or a process due to disease activity. The epidermal barrier abnormality has been reported not only in lesional skin, but also in non-lesional skin of AD patients (Proksch et al., 2006). Moreover, the epithelium of AD, which is not affected by skin lesions, is also characterized by bioelectric abnormalities in tight junctions (Pelc et al., 2018). Tight junctions are intercellular junction units and have an important role in the formation of the epidermal barrier against the transition of water, ions, and macromolecules (Basler et al., 2016). Dysfunction of tight junctions as a result of cutaneous inflammation in AD skin impairs epidermal barrier permeability by altering the pH of the SC which leads disruption of the mature lamellar structures, polar lipid formation and keratohyalin granules (Yuki et al., 2013). As a result, epidermal barrier permeability increases and it leads to increased ease of transition of the various bacterial agents and allergens, leading to a vicious circle of epidermal barrier dysfunction and cutaneous inflammation (Yokouchi et al., 2015; Katsarou et al., 2023).
Lipid alterations in AD
The epidermis contains basal keratinocytes, which are highly proliferative and have differentiation capability (van Smeden et al., 2014). They are characterized by the organized expression of specific proteins, intercellular junctions, enzymes such as proteases/antiproteases, as well as lipid components (van Smeden et al., 2014). The main SC lipids including ceramides (CERs), free fatty acids (FFAs) and cholesterol and its esters, are essential for a healthy skin barrier (Pappas, 2009; Jungersted and Agner, 2013; Loiseau et al., 2013; Li et al., 2016). SC lipids are secreted by keratinocytes into the extracellular space and move to the stratum corneum via lamellar bodies, which consist mostly of phospholipids, sphingolipids, and cholesterol (Wertz, 2018). In lamellar bodies, these lipids are metabolized by various enzymes such as sphingomyelinase, glucocerebrosidase and phospholipase (Wertz, 2018).
The intercellular lipid membrane shows a barrier role against infectious agents and maintains the integrity of the SC (Elias, 2005). An ideal ratio of the different SC lipids is one of the most important factors for healthy epidermal barrier function. AD is a common dermatological disease, characterized by impaired lipid barrier function. Mutations in lipid metabolizing enzymes or mutations that lead to increased protease activity or decreased protease inhibitor activity, could be associated with epidermal barrier dysfunction in AD. The lipid group most commonly reported to be deficient in AD is CERs (Levin et al., 2013). AD is characterized by abnormal skin lipids that are stimulated by hyperactivated type 2 immune response (Berdyshev et al., 2018). Inflammatory cytokines (IL-4, IL-13 and IL-31) reduce the expression of main CER synthesizing enzymes which are essential for lipid formation (Danso et al., 2017). This abnormality in SC lipids seems to occur independently of FLG mutations (Janssens et al., 2012; Joo et al., 2015). The metabolism of CERs in AD is affected by the immune/inflammatory response. Decreased total CER levels and variety in chain length such as increased short chain CERs, diminished long-chain CERs, increase in short chain FFAs, reduction in long chain FFAs and decrease in hydroxy-FFAs, have been shown in the etiopathogenesis of AD (van Smeden et al., 2014b; van Smeden and Bouwstra, 2016).
The enrichment of the skin microbiota with ceramidase-secreting bacteria, has been reported as a possible cause of reduced levels of CERs in AD skin (Ohnishi et al., 1999). Although S. aureus colonization is an important factor in AD patients, S. aureus does not show ceramidase activity (Ohnishi et al., 1999). Furthermore, so far no ceramidase-producing bacteria have been found in the skin of patients with AD (Pavel et al., 2022). One alternative hypothesis which seems more reasonable to explain the reduced levels of CERs, is the stimulation by Th2 cytokines such as IL-4 which leads to reduced CER synthesis in keratinocytes (Hatano et al., 2005).
These alterations in lipids lead to increased TEWL in AD skin (Janssens et al., 2012; Meckfessel and Brandt, 2014; Danso et al., 2017). Moreover, the decreased lipid levels are not only limited to lesional skin, but also have been demonstrated in non-lesional skin of patients with AD (van Smeden et al., 2014b; Joo et al., 2015; Toncic et al., 2020). This indicates that AD is not limited to visible skin lesions only.
The key protein filaggrin in AD
FLG is major epidermal structural protein which is provided by upper-layer epidermal keratinocytes. FLG is produced as a polymer profilaggrin and it is located in the outer nucleated layers of the epidermis. FLG degradation products maintain the pH balance, skin hydration and antimicrobial function of the epidermal barrier (Brown and McLean, 2012; Harding et al., 2013; Drislane and Irvine, 2020; Kim and Lim, 2021). FLG mutations are the most well-known genetic risk factors in AD (Morar et al., 2007; Esparza-Gordillo et al., 2009). The Th2 pathway plays an important role in AD and other atopic comorbidities, such as allergic rhinitis, asthma, and food allergy. Th2-related (IL-4, IL-13, IL-25), and Th22-related (IL-22) cytokines, which lead to reduced FLG levels in keratinocytes, are involved in the etiopathogenesis of AD (Fenner and Silverberg, 2018).
FLG mutations which are the most common, affecting 30%–50% of white AD patients, are associated with an increased skin permeability (Patrick et al., 2021). FLG deficiency is a complex combination of dysregulation of molecules involved in inflammatory, proteolytic and cytoskeletal functions (Elias et al., 2017). FLG deficiency is associated with dry skin, in consequence of the impaired skin barrier function and increased TEWL. This altered epidermal barrier function also makes the skin vulnerable against irritants, haptens and allergens, which penetrate the skin and induce allergic sensitization (Ständer, 2021).
In murine models of AD, it has been shown that FLG deficiency changes the construction of keratinocytes and secretion of lipids (Man et al., 2008; Kawasaki et al., 2012; Thyssen and Kezic, 2014; Elias et al., 2017). The decreased skin lipids lead to the diminished production of epidermal AMPs and consequently an altered skin microbiome (Langan et al., 2020; Patrick et al., 2021). In support of this, in AD patients with FLG mutations, an elevated S. aureus colonization has been shown (Clausen et al., 2017). In addition to the imbalance of the skin microbiome, FLG mutation is also associated with higher risk of early onset of the disease, high serum levels of IgE and other manifestations of atopy, as well as the persistence of AD into adulthood (Zaniboni et al., 2016).
Haftek et al., examined biomechanical characteristics of corneocytes in children with AD with FLG mutations (Haftek et al., 2020). In these corneocytes, they showed a decreased elastic modulus which strongly correlated with FLG degradation products and TEWL, but not with SCORAD (SCORing Atopic Dermatitis) (Haftek et al., 2020). They suggested that AD patients have decreased corneocyte stiffness, which correlates with reduced levels of FLG degradation products and skin barrier function (Haftek et al., 2020). Furthermore, FLG metabolites, such as urocanic acid and pyrrolidone carboxylic acid, were shown to contribute to moisturization and maintenance of acidic pH of the SC. Both of these molecules may be crucial to epidermal barrier homoeostasis by regulating the activity of multiple enzymes which control desquamation, lipid synthesis and inflammation in AD skin (Meckfessel and Brandt, 2014; Moosbrugger-Martinz et al., 2022).
FLG deficiency may lead to impaired skin barrier function in AD through multiple pathways. It has been also shown in murine models, that the genetic modifications may affect microbial colonization (Kobayashi et al., 2015; Nakatsuji et al., 2016). However, FLG mutations have not been demonstrated in all of AD patients and also some patients with FLG mutations do not present dysbiosis of the microbiome (Kobayashi et al., 2015; Nakatsuji et al., 2016; Williams et al., 2020). Further studies seem to be necessary to clarify the relationship between the skin microbiome and FLG mutations. It is also clear that the FLG mutation is not the only and/or absolute factor in the etiopathogenesis of AD.
The environmental factors associated with AD
Two hypotheses have been suggested for the development of AD lesions. The first one is the “inside-outside hypothesis”. This hypothesis is explained with epidermal barrier impairment in AD as a secondary result of the inflammatory response to irritants and allergens (Leung, 2000). On the other hand, the second one is the “outside-inside hypothesis” which favors that the xerosis (Denda et al., 1998), and the abnormal permeability of the barrier (Elias et al., 1999) or both may lead to AD lesions (Elias et al., 1999; Chamlin et al., 2002).
Microbial pathogens including bacteria, viruses and fungi may trigger AD and initiate allergic sensitization. These factors are modifiable and recognized by cell receptors. Thus, the skin immune cells are activated and secrete inflammatory cytokines causing the development of AD (Cork et al., 2009). Aeroallergens are also one of the AD triggers and include indoor aeroallergens such as house dust mite (HDM), pet dander, fur, cockroach, and mold, and outdoor aeroallergens such as tree, grass, and weed pollen (Werfel et al., 2015; Chong et al., 2022). HDM is a common sensitizing factor in pediatric AD patients and it has been also reported that children with a strong skin prick test reaction to HDM have more severe disease (Kutlu et al., 2013).
Various chemicals and irritants have been shown to influence AD, including soaps and detergents, as well as washing with hard water (Danby et al., 2018). Epidermal barrier dysfunction makes the skin vulnerable against these environmental factors, resulting in epidermal barrier damage. Detergents which contain irritating ingredients such as surfactants, may cause skin dryness, tightness and roughness, resulting in erythema and swelling (Ananthapadmanabhan et al., 2004). In AD patients, Callahan et al. showed lower thresholds to irritancy by sodium lauryl sulphate, which is a chemical agent in hand cleansers or shampoos (Callahan et al., 2013). Mechanical epidermal trauma could also exacerbate the symptoms of AD (Lee, 2020).
Climate is one of the associated factors with AD. It has been suggested that warm temperatures, high sun exposure or UV index, and higher humidity are associated with decreased AD prevalence, while low UV exposure, low temperatures and indoor heating may increase the risk of disease onset (Silverberg et al., 2013). However, higher temperatures and increased sun exposure may exacerbate the disease in some AD patients (Sargen et al., 2014; Kantor and Silverberg, 2017). These factors could be associated with the alterations in keratinocyte metabolism and immune dysregulation, as well as the degradation of FLG, which is affected by climate changes (Kantor and Silverberg et al., 2017). Vocks et al. showed that the temperature change from very cold (−17°C) to moderate (+18°C) was associated with decreased pruritus severity in AD patients (Vocks et al., 2001). Moreover, it has been suggested that people living in warmer climates may spend more time outdoors, thus they have more UV exposure, which may protect against AD. Contraversely, people living in warmer climates use less indoor heating, which may aggravate AD (Silverberg et al., 2013).
The relationship between birth season and AD has been investigated. Calov et al. (2020) found a significant association between AD and fall birth and winter birth when compared to spring birth. They suggested that the higher prevalance of AD in specific seasons could be explained by reduced ultraviolet radiation exposure, as well as increased air pollution (Calov et al., 2020) Air pollution is one of several factors that people are exposed to in daily life, and a contributor to AD. Air pollutants may arise from indoor and/or outdoor environments and they could enter the systemic circulation through penetration of the skin (Ahn, 2014). Rutter et al. found that the exposure to heavy traffic during the 12 months before measurement was significantly associated with eczema symptoms in children (Rutter et al., 2019) In contrast, Huls et al. showed no association between the traffic-related air pollution and AD in the general population (Huls et al., 2019). However, they suggested that the prevalence of childhood AD is correlated with oxidative stress and inflammation (Huls et al., 2018). They also reported that the risk scores from glutathione S-transferase P1, tumor necrosis factor, Toll-like receptor (TLR)-2, and TLR-4 single-nucleotide polymorphisms are associated with AD up to the age of 2 years (Huls et al., 2019; Ahn et al., 2020).
Exposure to certain foods may induce an immunological response in the skin and exacerbate the symptoms of some AD patients through allergic and non-allergic hypersensitivity reactions. It has been shown that more severe disease activity is correlated with increased frequency of food allergy (Burks et al., 1998; Eigenmann and Calza, 2000). Children with food allergies have positive skin tests and/or presence of serum IgE antibodies against particularly eggs, milk, wheat, soy and peanuts (Wassmann-Otto et al., 2018; Wollenberg et al., 2020). In a large population-based study, it has been reported that infants with AD are 6 times more likely to have egg allergy and 11 times more likely to have peanut allergy by 12 months than infants without AD at 12 months of age (Martin et al., 2014). However, restricted diet is not recommended for most AD patients (Rustad et al., 2022) since the possible tolerance of food allergies is being developed until the age of three (Akdis et al., 2006; Pelc et al., 2018). Elimination diet should be recommended in case a food is clearly identified as an exacerbarating factor (Papapostolou et al., 2022).
The relationship between immune abnormalities, keratinocytes and cutaneous microbiome in AD
As the skin microbiome gains more and more interest as a key determinant of skin health, much effort is made to unravel the complex interplay of host cells and skin residents. In the last decade, it was shown in multiple studies that skin immunology and therefore also inflammatory processes are tightly and inevitably linked to the skin’s microbiota. Especially in the last few years a lot of evidence beginning to unravel the complex symbiosis between host and microorganisms was collected. The epidermis is colonized by bacteria, fungi, viruses and other microorganisms, and there is a complex interplay between host cells and the so-called commensals. From birth on, the skin of the new-born is colonized by microorganisms, with differences according to the mode of delivery (natural birth versus caesarean section). Based on environmental and individual factors, the microbiota stabilizes during the first years of life (Luna, 2020) Due to the importance of early microbial colonization, it can unsurprisingly be linked to the development of allergic diseases like food allergy or AD (Peroni et al., 2020). Therefore, human health is not only dependant on the actual microbial environment but also on the microbes that were encountered in the past, especially the first few years of life.
The cutaneous microbiome is important for both immune maturation and epidermal barrier function. There are many factors, including skin immune system, pH and water balance, the epidermal lipid composition and the expression of antimicrobial peptides, that contribute to the microbial balance in the epidermis (Zhang and Gallo, 2016; Wohlrab et al., 2018; Fölster-Holst, 2022). Because the skin’s microbiota (as well as microorganisms in other habitats) affects immunologic maturation, characteristic changes in skin colonization like the overrepresentation of pathogenic bacteria or the lack of contact to “good” ones in early life may have consequences for inflammatory skin diseases and might be even involved in their pathogenesis. For instance, this has been demonstrated for AD. Meylan and others found that S. aureus colonization in infancy was positively associated with the development of AD, and this colonization preceded disease onset (Meylan et al., 2017). In contrast, the presence of Staphylococcus hominis at age 3 months tended to be negatively associated with AD development, underscoring that different bacteria exert different effects to the skin and its immune system (Meylan et al., 2017). Moreover, during AD flares, the variety of skin bacteria colonization alters and S. aureus colonization increased and S. epidermidis decreased (Bjerre et al., 2017). On the contrary, S. epidermidis and species of Streptococcus, Corynebacterium and Propionibacterium increased during the remission phases (Kong et al., 2012). However, it has been reported that antibacterial agents against S. aureus are not superior to other non-antimicrobial treatments in AD (Bath-Hextall FJ et al., 2010). S. aureus may not be a major pathogenic factor in the etiopatogenesis of AD, nevertheless the presence of S. aureus could be associated with disease severity (Moosbrugger-Martinz et al., 2021). In adult patients with severe AD during disease flare, increased levels of S. aureus in lesional skin have been shown (Clausen et al., 2018; Smits et al., 2020). Moreover, S. aureus colonization in non-lesional skin and even twice more in lesional skin have been shown in patients with AD (Baker, 2006; Totté et al., 2016; Nowicka et al., 2022). However, it has not been found in all AD patients (Paller et al., 2019b) and it is not certain whether S. aureus promotes the inflammation in AD or whether the inflammation is the reason for the presence of S. aureus due to an impaired skin barrier. S. aureus secretes toxins and superantigens and stimulates the activation of T cells, thus contributes to skin inflammation in patients with AD (Schlievert et al., 2010). In support of this, Skop et al. suggested that the application of Staphylococcal enterotoxin B to non-lesional skin may induce eczematous lesions (Skov et al., 2000). On the other hand, the colonization with some “good” bacteria on the skin may have protective effects in AD (Cho et al., 2001). In patients with AD and Netherton syndrome, increased S. epidermidis and S. hominis in the post flare phase have been shown (Byrd et al., 2017; Moosbrugger-Martinz et al., 2021). Some strains of S. epidermidis may improve innate immunity and protect the skin against infection with pathogens by activating IL-17- expressing CD8+ T cells (Prescott et al., 2017).
The present microbes and their metabolites also prime the maturation of the host immune system. Innate as well as adaptive immune responses are different in neonates compared to adults. In order to ensure tolerance to self- and foreign antigens, neonates are less prone to inflammation, which is crucial for the rapidly developing tissues (Paller et al., 2019b). During this educational period for the host immune system, the foundation for health or disease in later life could be established, as demonstrated in animal models, e.g., for inflammatory bowel disease and asthma (Gensollen et al., 2016). For the skin, this was shown with the commensal Staphylococcus epidermidis, which is considered a beneficial bacterium in this tissue. Adult immunologic responses to this commensal were different in mice according to the time of introduction of S. epidermidis. Exposure in early life circumvented inflammation induced by contact in adult animals, and this effect was attributed to the development of regulatory T-cells in answer to early exposure (Scharschmidt et al., 2015).
Keratinocytes are directly involved in the regulation of commensal-specific T cells. Epidermal accumulation of lymphocytes is a phenomenon induced by the microbiota, with type 1 and type 17 cells present in these clusters. Commensal induced Th1 cells thereby are regulated by MHC class II expressing keratinocytes in an IL-22 dependent fashion (Tamoutounour et al., 2019). The epidermal microbiota seems to be more individual than the dermal one, which shows less inter-individual variability (Bay et al., 2020). Host cells like keratinocytes establish an environment in favour of beneficial microbes by secreting specific antimicrobial components including innate cytokines, pathogen associated molecular pattern (PAMP) molecules, the inflammasome, and AMPs, targeting unwanted microorganisms, while providing nutrition for those who can metabolize special lipids (Nakatsuji et al., 2010; Dahlhoff et al., 2016).
Next to specific antimicrobial substances, bacteria are influenced by other host signals whose primary function is the communication between host cells. For example, it was found that neuropeptides like substance P exert effects on the virulence of bacteria, thereby contributing to microbiota homeostasis (N’Diaye et al., 2017).
The high diversity of the gut microbiome affects the immunity of the whole body, including skin. It improves the levels of regulatory T cells and short-chain fatty acids (Belkaid and Hand, 2014). On the other hand, an imbalance of the gut microbiome and increased levels of noxious microorganisms lead to secondary skin infections and immune-related diseases including AD (Belkaid and Hand, 2014). Infants with a diverse gut microbiome have a lower risk of development of AD (Ihekweazu and Versalovic, 2018; Park et al., 2021). In addition to this, infants with AD are more often colonized by Clostridium difficile and Escherichia coli than infants without AD (Penders et al., 2007). The priming of the cutaneous immune system enables the mature tissue to select for beneficial microorganisms, which at the same time prevent the outgrowth of pathogenic species. By competing for space and nutrition and by specific antagonistic mechanisms, commensal bacteria protect their respective niche. This delicately balanced homeostasis is disrupted in different disease settings. Dysbiosis, which means an adversely altered microbiota, can trigger inflammatory processes, e.g., by inducing IL-1α release by keratinocytes (Archer et al., 2019). Archer and others demonstrated in a filaggrin deficient mouse model, that skin injury and dysbiosis resulted in chronic inflammation in the animals, and that keratinocyte-derived IL-1α was the driver of this development. This suggests that skin microbiome modulation could be beneficial for AD patients.
Several regulators and signalling pathways were found to be involved in the skin-commensal crosstalk and the cutaneous microbiota homeostasis. In mice, dendritic epidermal T cells are regulated by the G protein coupled receptor 15 (GPR15) (Sezin et al., 2021). Gpr15−/− mice exhibit a severe deficiency in this cell type, with an overall reduced number of T cells in the epidermis, but not the dermis. The expression of GPR15 on T cells in turn is influenced by the skin as well as the gut microbiome (Kim et al., 2013; Jacob et al., 2020), and knock-out animals show alterations of their cutaneous microbiomes compared to wildtype littermates. Interestingly, GPR15 expression in human CD4+ cells is regulated by aryl hydrocarbon receptor (Ahr) (McAleer et al., 2018), and since dendritic epidermal T cells depend on Ahr signalling (Kadow et al., 2011), Sezin et al. speculated about GPR15 as a downstream target of Ahr (Sezin et al., 2021). Furthermore, keratinocyte Ahr is essential for barrier integrity in murine skin (Haas et al., 2016), demonstrating the importance of the Ahr pathway in different cell types in the epidermis. Just like Gpr15−/− mice, Ahr-deficient mice demonstrate an altered, more variable and probably more unstable microbiome (Haas et al., 2016). Ahr is activated by indole-3-aldehyde, a microbial tryptophan metabolite, and Ahr activation leads to production of anti-inflammatory IL-10 by Langerhans cells and subsequently inhibited CD4+ T cell proliferation (Liu et al., 2020). In addition, Ahr signalling in keratinocytes is influenced by the microbiota, with consequences for skin barrier function and repair (Uberoi et al., 2021).
A further player in the dialogue between skin immune cells and microbiota is the transcription factor JunB, which is expressed by keratinocytes. Mice deficient in epidermal JunB exhibit an AD-like phenotype, including proneness to spontaneous skin colonization by S. aureus. JunB negatively regulates MyD88 in keratinocytes, upon loss an inflammatory response cascade was reported. As inflammation was exacerbated in Rag1−/− mice, it was concluded that the adaptive immune system, presumably due to the production of IL-17A by T cells, is necessary to prevent S. aureus infection (Uluçkan et al., 2019).
Another example of the complexity of the regulation of epithelial cells, immune cells and commensals is the homeostatic control of sebaceous glands by innate lymphoid cells residing in hair follicles. Innate lymphoid cells express mediators that restrict sebocyte growth, thereby regulating the expression of sebaceous gland derived antimicrobial lipids. In this way, the commensal bacteria equilibrium is maintained (Kobayashi and Nagao, 2019). Hair follicles themselves rely on the endopeptidase ADAM10-Notch signalling axis, with a disruption in this pathway resulting in skin dysbiosis and destruction of hair follicles (Sakamoto et al., 2021).
While the interactions between host skin cells and commensals are already a complex and delicately balanced system, another layer of complexity is added when considering the skin as part of the whole human body. Not only keratinocytes and epidermal immune cells receive signals from within the body, so do the microorganisms which stand in contact with other microbial habitats from their host organism. Especially the gut as the most heavily colonized region is thought to act as a central signalling node for all peripheral microbial communities (Martínez et al., 2021). The cutaneous microbiome therefore is not only important for immune maturation and regulation but is directly involved in epidermal function. In conclusion, future work should be directed at further unravelling the network of interactions and molecular signalling mechanisms that include keratinocytes, skin immune cells and microorganisms.
Cutaneous inflammation, AD and cancer risk
The relationship between inflammatory skin disorders including AD, and cancer has been investigated (Zhu et al., 2022; Wan et al., 2023). AD has been found to be significantly correlated with an increased risk of non melanoma skin cancer (NMSC) (Zhu et al., 2022). In line with this, in another study, a greater risk of NMSC in children with mild AD was reported, however, a lower risk of melanoma in children with moderate AD was found (Vittrup, 2023). Further, an increased risk of lymphoma among children with severe AD has been found (Vittrup, 2023). In adults, a slightly increased risk of haematological malignancy, aslightly higher skin cancer risk and lower risk of solid organ malignancy has been reported (Vittrup, 2023). The authors suggested that although there is no overall association between AD and malignancy, AD may have heterogeneous effects by cancer subtype (Vittrup, 2023).
Although the mechanism of skin cancer in AD patients is not certain, skin cancer progression has been associated with the dysregulation of microbiome (Woo et al., 2022). Furthermore, it can be suggested that the increased risk of skin cancer in patients with AD may be a long-term side effect of phototherapy treatment. However, the reduced risk of melanoma contradicts this hypothesis (Vittrup, 2023). Moreover, in recents studies, no strong overall malignancy risk in AD but a possible increased lymphoma risk in patients with severe AD (Wan et al., 2023), particularly NHL, that increased with eczema severity (Mansfield et al., 2020), has been reported.
On the other hand, decreased risk of malignancy in the esophagus, stomach, colorectum, and liver, has been found in patients with allergic diseases including allergic rhinitis, asthma, and AD (Choi et al., 2023). This decreased risk in GI cancers in AD patients could be explained by the immunosurveillance hypothesis. According to this theory, excessive stimulation of T-helper cell type II immune response and other immune cells such as mast cells, natural killer cells and eosinophils can prevent the onset of cancer by detecting and destroying the damaged cells before the onset of carcinogenesis (Ji et al., 2009; Choi et al., 2023). On the contrary, it is known that a Th2-dominant environment downregulates tumor immunity (Morimura et al., 2021). However, Morimura et al., suggested that a high level of CCL17, known as thymus and activation-regulated chemokine, may work as a “safety-net” to reduce the risk of malignant tumors and positively contributes to tumor immunity via decreasing myeloid-derived suppressor cells in the Th2-dominant environment in AD patients (Morimura et al., 2021). This may be one of the explanations for the normal incidence of cancer among patients with AD, regardless of the Th2-dominant environment. However, it is clear that the incidence of cancer in patients with AD needs further investigation.
Therapeutic options for AD and their effects on the immunological and structural epidermal barrier dysfunction and skin microbiome
AD presents quite heterogeneously, both clinically and immunologically (Czarnowicki et al., 2019). There are various treatment options for AD according to age, the severity of pruritus, involved body-surface area and the clinical stage of the disease (mild, moderate, or severe) (Sidbury et al., 2014; Wollenberg et al., 2018). A multi-therapeutic approach is essential in the management of AD. Short-term management aims to control symptoms in the periods of exacerbation of AD, while long-term treatment aims to prevent from new lesions and prolong the time between flares (Boulos and Yan, 2018). The most important aim of AD treatment is disease prevention due to potential toxicity of immunosuppressive therapies (Diaz and Guttman-Yassky, 2019).
Although it is not possible to mention all of the drugs marketed or being tested, there are approved topical and systemic treatment options available in the treatment of AD. Basic therapy contributes to barrier function through improving skin hydration and regeneration of intercellular lipid lamellae and is always required in addition to other topical and systemic therapeutics (Staubach and Lunter, 2014). Moisturizers are used both for the therapy and prevention of AD, providing repair to the epidermal lipid matrix (Elias et al., 2019). Moisturizers also reduce the use of inflammatory products and improve epidermal barrier function (Loden, 2003; Szczepanowska et al., 2008; Czarnowicki et al., 2016; Giam et al., 2016). In premature newborns, moisturizers also decrease bacterial colonization, TEWL and disease severity (Nopper et al., 1996; Darmstadt et al., 2007). Additionally, petrolatum increases the expression of FLG and loricrin and also induces upregulation of major AMPs such as LL-37, lipocalin 2 and peptidase inhibitor 3 (Czarnowicki et al., 2016). Moreover, although there are opposing views, it has been reported that early moisturizing in high-risk newborns alters the skin microbiome and pH levels (Glatz et al., 2015).
In 1991, wet-wrap dressing was applied to pediatric AD patients for the first time (Goodyear et al., 1991). For such dressings, the patient takes a bath in warm water and dries off the water, after this, the lesions are covered with wet gauze. This wet material is then covered with a second layer of dry material for a certain time. Wet-wrap dressing may also be applied with moisturizer or weak topical corticosteroids according to the clinical findings of the disease (Twitchen and Lowe, 1998). Wet-wrap therapy is associated with improvement of epidermal barrier function in patients with AD. Lee et al. (2007) showed that wet-wrap dressing is associated with decreased SCORAD, increased epidermal water content, and decreased TEWL. However, they observed no change in keratinocyte differentiation and calcium ion gradient with wet-wrap dressing (Lee et al., 2007).
Application of topical corticosteroids (TCSs) remains to be the mainstay in the treatment of AD. TCSs have anti-inflammatory, anti-proliferative, and immunosuppressive effects which contribute to the treatment of AD (Del Rosso and Friedlander, 2005). Glucocorticoids show suppressive effects against neutrophils, monocytes, lymphocytes, Langerhans cells and cytokines such as IL-1α, IL-1β, IL-2, tumor necrosis factor, and granulocyte-monocyte colony stimulating factor (Del Rosso and Friedlander, 2005). In contrast to the beneficial effects of steroids, they also inhibit the synthesis of cholesterol, ceramides, and free fatty acids and disrupt the anti-bacterial function of the epidermis (Pelc et al., 2018). Long-term use of TCSs leads to various side effects including skin atrophy, striae distensae, teleangiectasia, and impaired skin barrier (Schoepe et al., 2006; Shlivko et al., 2014). Therefore, topical corticosteroids should be used only for a certain period of time and in appropriate amounts.
There are also other topical therapeutic options such as topical calcineurin inhibitors (TCIs) and topical phosphodiesterase 4 (PDE4) inhibitors with advantages of minimal side effects and possible long-term use (Kim et al., 2020; Freitas et al., 2022). TCIs inhibit the transcription of proinflammatory cytokine genes, including IL-2 (Bornhovd et al., 2001). TCIs are favorable particularly in skin folds and at the face, which are highly sensitive areas (Simpson, 2010). Jensen et al. reported improvement in all epidermal barrier parameters including TEWL in AD patients which were treated with both a topical steroid (betamethazone valerate) and a topical calcineurin inhibitor (pimecrolimus). Although both treatments normalized epidermal differentiation and reduced epidermal hyperproliferation, betamethazone valerate has been found to be more effective in reducing clinical symptoms and epidermal proliferation, however it induced epidermal thinning unlike pimecrolimus (Jensen et al., 2009). Their alternate use may be an option in AD lesions.
Crisaborole ointment 2% is a nonsteroidal PDE4 inhibitor for the treatment of mild-to-moderate AD. The inhibition of PDE4 may decrease the inflammatory processes associated with AD without significant serious adverse event incidences. PDE4 inhibitors reduce the occurrence of AD exacerbation, however they have a statistically significant risk of producing pain (Martín-Santiago et al., 2022). Moreover, roflumilast (Calverley et al., 2007) and apremilast (Papp et al., 2013) have several adverse effects after systemic administration, but topical application has been found to decrease exposure and minimize adverse effects including burning of skin, pruritus and skin infections (Ashcroft et al., 2005; Broeders et al., 2016; Abed and Pawliczak, 2019).
The gut-skin-axis is involved in several dermatological diseases including AD (De Pessemier et al., 2021). In inflammatory skin diseases like AD or psoriasis, increasing interest is directed at the microbial skin component as therapeutic target. Influencing skin commensals and/or pathogenic bacteria like S. aureus with probiotics could happen via the gut-skin axis with selected bacteria (Szántó et al., 2019). Topical or oral probiotics may have some beneficial effects on AD symptoms associated with gut microbiome dysbiosis, including the alteration of the abundance of skin commensals and/or pathogenic bacteria like S. aureus (Szántó et al., 2019; Fang et al., 2020). It has been reported that Lactobacillus johnsonii caused significant improvement in skin symptoms of AD patients (Szántó et al., 2019) and also Lactobacillus plantarum significantly decreased SCORAD index and influencds the gut microbiota composition (Fang et al., 2020). Other approachs are even more direct and are supposed to modulate the skin microbiome by immediate application of specific bacteraial strains to the skin. Isolation of Roseomonas mucosa strains from healthy volunteers and transplantation to adult and peadiatric AD patients was successfully performed and led to reduced S. aureus colonization (Myles et al., 2018; Myles et al., 2020). Furthermore, SCORAD and Eczema Area and Severity Index (EASI) decreased and patients needed less glucocorticoid treatment. The authors found that Roseomonas mucosa produced beneficial sphingolipids and induced TNFR2-mediated epithelial repair mechanisms (Myles et al., 2020).
In another study aiming at the decrease of S. aureus colonization, autologous transplantation of specific bacterial strains like S. epidermidis and S. hominis after screening for antimicrobial activity achieved good results in AD patients and demonstrated the importance of skin commensals for AD disease pathology (Nakatsuji et al., 2017). In a corresponding phase 1 trial, a S. hominis strain isolated from healthy human skin was used as bacteriotherapy for AD and decreased S. aureus (Nakatsuji et al., 2021). Although eczema severity was not significantly changed over all patients, a specific subgroup seemed to especially benefit from the S. hominis treatment. This finding highlights that when it comes to the microbiome and its role in diseases, there will be huge differences between patients and individualized therapy approaches are or will be warranted.
In recent years, new therapeutic options such as biologic drugs have gained more importance with better understanding of AD pathogenesis (Kim et al., 2020; Freitas et al., 2022). Dupilumab is a human monoclonal antibody against IL-4 receptor α. Berdyshev et al. investigated the role of dupilumab in the regulation of skin barrier structure and function. They reported that blocking IL-4/IL-13 signalling with dupilumab decreased the TEWL in AD lesions, normalized the lipid composition and increase the ceramide chain length in lesional as well as non-lesional SC of AD patients (Berdyshev et al., 2022). Recently, the effect of dupilumab on S. aureus colonization and microbial diversity of the skin has been investigated (Callewaert et al., 2020). During dupilumab therapy, Callewaert et al. showed in both, lesional and non-lesional skin, increased microbial diversity and decreased S. aureus colonization, which was correlated with clinical improvement of AD (Callewaert et al., 2020). Dupilumab seems to affect many factors such as immunological markers, lipid composition and microbial colonization in the etiopathogenesis of the AD.
With immunological discoveries in the etiopathogenesis of AD, new biological therapies are gaining importance in the treatment of the disease. IL-13 is a potential therapeutic target for patients with AD (Zhang et al., 2022). In AD patients, increased expression of IL-13 in lesional tissues and elevated serum IL-13 levels have been found when compared to healthy controls (Tazawa et al., 2004). Moreover, it has been reported that an elevated IL-13 level is positively correlated with AD disease severity (Tazawa et al., 2004; Ungar et al., 2017). Tralokinumab is a human IgG4 monoclonal antibody, preventing IL-13 from binding to both IL-13Rα1 and IL-13Rα2 (Popovic et al., 2017). In a recent report from 2,285 patients, tralokinumab has been found to be a well-tolerated agent both in combination with TCS and as monotherapy, with long-term use up to 52 weeks for moderate-to-severe AD (Simpson et al., 2022). Lebrikizumab, which is also one of the new biological therapies, is a selective monoclonal antibody that targets IL-13. IL-13 plays an important role in multiple itch pathways and may contribute to the persistence of chronic itch in AD. The improvement of chronic itch in AD patients by lebrikizumab seems to be related to neuronal effects via IL-13 inhibition (Miron et al., 2022). A potential advantage of IL13 inhibitors is that the conjunctivitis, which is a common side effect of dupilumab (up to 22% in clinical trials), is less frequent under lebrikizumab (6.3%) and tralokinumab (6.2%) (Akinlade et al., 2019; Zhang et al., 2022).
Nemolizumab is a subcutaneously administered humanized monoclonal antibody against IL-31 receptor A. IL-31 blockage shows a direct effect against pruritus in AD patients (Nemoto et al., 2016; Oyama et al., 2018). Nemolizumab may also improve skin barrier function (Feld et al., 2016; Singh et al., 2016) and reduce the overall severity of AD (Liang et al., 2022). In a recent stıdy, it has been reported that nemolizumab achieves TIMEACLIR-Itch (MEAningful CLInical Response for itch reduction) more quickly than anti-IL-4 or -IL-4/13 agents (Lin et al., 2022). In 2022, nemolizumab has been approved in Japan for adults and children above the age of 13 years in the treatment of itch associated with AD, refractory to current treatments (Maruho, 2022). Additionally, numerous clinical trials are ongoing which are investigating the efficacy of nemolizumab in AD (Keam, 2022).
Topical and oral JAKi are potential treatment options which significantly improve the clinical symptoms of AD patients with unsatisfactory response to conventional therapeutics (Honstein and Werfel, 2020). IL-4, IL-13, and IL-31 are the major cytokines which influence AD pathogenesis via the JAK-STAT signalling pathway. Systemic JAKi including baricitinib (JAK1/2i), upadacitinib (JAK1i) and abrocitinib (JAK1i) are current treatment options in moderate to severe AD (Klein et al., 2022).
Ruxolitinib is also a selective JAK1/2 inhibitor available in both topical and oral administration options. Ruxolitinib 1.5% topical cream is the first topical JAKi, approved by US Food and Drug Administration (FDA) in patients (≥12 years) with mild-to-moderate AD (Owji et al., 2022). It has been reported that ruxolitinib and delgocitinib (pan JAKi) significantly improve pruritus and EASI in patients with moderate to severe AD (Kim et al., 2020; Nakagawa et al., 2020). In patients with mild to moderate AD with 2%–20% body surface area involvement, the efficacy of tofacitinib, which is a topical JAK 1/3 inhibitor, has also been reported (Bissonnette et al., 2016). In a recent meta-analysis, it has been found that particularly tofacitinib 2% has superior Investigator’s Global Assessment response over other included JAKi and PDE4 inhibitors, followed by ruxolitinib 1.5% and delgocitinib 3% (Zhang et al., 2021). However, it is clear that more studies comparing the effect of topical and/or biological agents in AD patients, are needed.
2 Conclusion
AD is a chronic inflammatory skin disease in which many factors such as immunological and structural epidermal barrier dysfunction, immune abnormalities, lipid alterations, FLG mutations and skin microbiome alterations are involved in its etiopathogenesis. Although much progress has been made regarding the pathophysiology of AD and its clinical manifestations in adults and children, there are still many points that need to be clarified including unclear, complex molecular and cellular mechanism and metabolics, and also the specific mechanism of the alterations of lipid compositions.
Although topical corticosteroids are the cornerstone of AD treatment, new biological therapies also achieve promising results. However, it is also necessary to monitor the long-term effects of biological treatments. With new discoveries in the etiopathogenesis of AD, the symptoms of the disease could be controlled more effectively with new targeted therapies.
Author contributions
TÇ: literature search, data acquisition, data analysis, manuscript preparation, manuscript editing, and manuscript review. LK: literature search, data acquisition, data analysis, manuscript preparation, manuscript editing, and manuscript review. RF-H: literature search, data acquisition, data analysis, manuscript preparation, manuscript editing, and manuscript review. All authors contributed to the article and approved the submitted version.
Acknowledgments
Image has been created with Biorender.com.
Conflict of interest
LK is working as a Medical Advisor Dermatology for the company of MEDICE. However she worked on the text while on parental leave without any financial support by MEDICE and without using MEDICE resources. General conflicts of interest of Regina Fölster-Holst: RFH has been an advisor or speaker for Biogen, Johnson & Johnson, La Roche Posay, LEO Pharma, Neubourg, Novartis, Pfizer, Pierre Fabre, Regeneron and Sanofi.
The remaining author declares that the research was conducted in the absence of any commercial or financial relationships that could be construed as a potential conflict of interest.
Publisher’s note
All claims expressed in this article are solely those of the authors and do not necessarily represent those of their affiliated organizations, or those of the publisher, the editors and the reviewers. Any product that may be evaluated in this article, or claim that may be made by its manufacturer, is not guaranteed or endorsed by the publisher.
References
Abedz´, N., and Pawliczak, R. (2019). Efficacy and safety of topical calcineurin inhibitors for the treatment of atopic dermatitis: meta-analysis of randomized clinical trials. Postepy. Dermatol. Alergol. 36, 752–759. doi:10.5114/ada.2019.91425
Ahn, K., Kim, B. E., Kim, J., and Leung, D. Y. (2020). Recent advances in atopic dermatitis. Curr. Opin. Immunol. 66, 14–21. doi:10.1016/j.coi.2020.02.007
Ahn, K. (2014). The role of air pollutants in atopic dermatitis. J. Allergy. Clin. Immunol. 134, 993–999. discussion 1000. doi:10.1016/j.jaci.2014.09.023
Akdis, C. A., Akdis, M., Bieber, T., Bindslev-Jensen, C., Boguniewicz, M., Eigenmann, P., et al. (2006). Diagnosis and treatment of atopic dermatitis in children and adults: european academy of allergology and clinical immunology/American academy of allergy, asthma and immunology/PRACTALL consensus report. Allergy 61, 969–987. doi:10.1111/j.1398-9995.2006.01153.x
Akinlade, B., Guttman-Yassky, E., de Bruin-Weller, M., Simpson, E., Blauvelt, A., Cork, M., et al. (2019). Conjunctivitis in dupilumab clinical trials. Br. J. Dermatol. 181 (3), 459–473. doi:10.1111/bjd.17869
Akiyama, T., Niyonsaba, F., Kiatsurayanon, C., Nguyen, T. T., Ushio, H., Fujimura, T., et al. (2014). The human cathelicidin LL-37 host defense peptide upregulates tight junction-related proteins and increases human epidermal keratinocyte barrier function. Innate. Immun. 6, 739–753. doi:10.1159/000362789
Ananthapadmanabhan, K. P., Moore, D. J., Subramanyan, K., Misra, M., and Meyer, F. (2004). Cleansing without compromise: the impact of cleansers on the skin barrier and the technology of mild cleansing. Dermatol. Ther. 17 (1), 16–25. doi:10.1111/j.1396-0296.2004.04s1002.x
Archer, N. K., Jo, J. H., Lee, S. K., Kim, D., Smith, B., Ortines, R. V., et al. (2019). Injury, dysbiosis, and filaggrin deficiency drive skin inflammation through keratinocyte IL-1α release. J. Allergy. Clin. Immunol. 143 (4), 1426–1443. doi:10.1016/j.jaci.2018.08.042
Ashcroft, D. M., Dimmock, P., Garside, R., Stein, K., and Williams, H. C. (2005). Efficacy and tolerability of topical pimecrolimus and tacrolimus in the treatment of atopic dermatitis: meta-analysis of randomised controlled trials. BMJ 330, 516. doi:10.1136/bmj.38376.439653.D3
Baker, B. S. (2006). The role of microorganisms in atopic dermatitis. Clin. Exp. Immunol. 144, 1–9. doi:10.1111/j.1365-2249.2005.02980.x
Basler, K., Bergmann, S., Heisig, M., Naegel, A., Zorn-Kruppa, M., and Brandner, J. M. (2016). The role of tight junctions in skin barrier function and dermal absorption. J. Control. Release. 242, 105–118. doi:10.1016/j.jconrel.2016.08.007
Bath-Hextall, F. J., Birnie, A. J., Ravenscroft, J. C., and Williams, H. C. (2010). Interventions to reduce Staphylococcus aureus in the management of atopic eczema: an updated cochrane review. Br. J. Dermatol. 163 (1), 12–26. doi:10.1111/j.1365-2133.2010.09743.x
Bay, L., Barnes, C. J., Fritz, B. G., Thorsen, J., Restrup, M. E. M., Rasmussen, L., et al. (2020). Universal dermal microbiome in human skin. mBio 11 (1), 029455–e3019. doi:10.1128/mBio.02945-19
Belkaid, Y., and Hand, T. W. (2014). Role of the microbiota in immunity and inflammation. Cell 157, 121–141. doi:10.1016/j.cell.2014.03.011
Berdyshev, E., Goleva, E., Bissonnette, R., Bronova, I., Bronoff, A. S., Richers, B. N., et al. (2022). Dupilumab significantly improves skin barrier function in patients with moderate-to-severe atopic dermatitis. Allergy 77 (11), 3388–3397. doi:10.1111/all.15432
Berdyshev, E., Goleva, E., Bronova, I., Dyjack, N., Rios, C., Jung, J., et al. (2018). Lipid abnormalities in atopic skin are driven by type 2 cytokines. JCI. Insight. 22 3(4), e98006. doi:10.1172/jci.insight.98006
Bieber, T. (2022). Atopic dermatitis: an expanding therapeutic pipeline for a complex disease. Nat. Rev. Drug. Discov. 21, 21–40. doi:10.1038/s41573-021-00266-6
Bissonnette, R., Papp, K. A., Poulin, Y., Gooderham, M., Raman, M., Mallbris, L., et al. (2016). Topical tofacitinib for atopic dermatitis: a phase IIa randomized trial. Br. J. Dermatol. 175, 902–911. doi:10.1111/bjd.14871
Bjerre, R. D., Bandier, J., Skov, L., Engstrand, L., and Johansen, J. D. (2017). The role of the skin microbiome in atopic dermatitis: a systematic review. Br. J. Dermatol 177, 1272–1278. doi:10.1111/bjd.15390
Bornhovd, E., Burgdorf, W. H., and Wollenberg, A. (2001). Macrolactam immunomodulators for topical treatment of inflammatory skin diseases. J. Am. Acad. Dermatol. 45 (5), 736–743. PubMed PMID: 11606925. doi:10.1067/mjd.2001.117525
Boulos, S., and Yan, A. C. (2018). Current concepts in the prevention of atopic dermatitis. Clin. Dermatol. 36 (5), 668–671. doi:10.1016/j.clindermatol.2017.03.004
Briot, A., Deraison, C., Lacroix, M., Bonnart, C., Robin, A., Besson, C., et al. (2009). Kallikrein 5 induces atopic dermatitis–like lesions through PAR2- mediated thymic stromal lymphopoietin expression in Netherton syndrome. J. Exp. Med. 206, 1135–1147. doi:10.1084/jem.20082242
Broeders, J. A., Ahmed Ali, U., and Fischer, G. (2016). Systematic review and meta-analysis of randomized clinical trials (RCTs) comparing topical calcineurin inhibitors with topical corticosteroids for atopic dermatitis: a 15-year experience. J. Am. Acad. Dermatol. 75, 410–419. doi:10.1016/j.jaad.2016.02.1228
Brown, S. J., and McLean, W. H. (2012). One remarkable molecule: filaggrin. J. Investig. Dermatol. 132, 751–762. doi:10.1038/jid.2011.393
Burks, A., James, J. M., Hiegel, A., Wilson, G., Wheeler, J., Jones, S. M., et al. (1998). Atopic dermatitis and food hypersensitivity reactions. J. Pediatr. 132, 132–136. doi:10.1016/s0022-3476(98)70498-6
Bylund, S., von Kobyletzki, L. B., Svalstedt, M., and Svensson, A. (2020). Prevalence and incidence of atopic dermatitis: a systematic review. Acta. Derm. Venereol. 100 (12), adv00160. doi:10.2340/00015555-3510
Byrd, A. L., Deming, C., Cassidy, S. K. B., Harrison, O. J., Ng, W. I., Conlan, S., et al. (2017). Staphylococcus aureus and Staphylococcus epidermidis strain diversity underlying pediatric atopic dermatitis. Sci. Transl. Med. 9 (397), eaal4651. doi:10.1126/scitranslmed.aal4651
Callahan, A., Baron, E., Fekedulegn, D., Kashon, M., Yucesoy, B., Johnson, V. J., et al. (2013). Winter season, frequent hand washing, and irritant patch test reactions to detergents are associated with hand dermatitis in health care workers. Dermatitis 24 (4), 170–175. doi:10.1097/DER.0b013e318290c57f
Callewaert, C., Nakatsuji, T., Knight, R., Kosciolek, T., Vrbanac, A., Kotol, P., et al. (2020). IL-4Rα blockade by dupilumab decreases Staphylococcus aureus colonization and increases microbial diversity in atopic dermatitis. J. Invest. Dermatol. 140, 191–202. doi:10.1016/j.jid.2019.05.024
Calov, M., Alinaghi, F., Hamann, C. R., Silverberg, J., Egeberg, A., and Thyssen, J. P. (2020). The association between season of birth and atopic dermatitis in the northern hemisphere: a systematic review and metaanalysis. J. Allergy. Clin. Immunol. Pract. 8, 674–680. doi:10.1016/j.jaip.2019.10.007
Calverley, P. M., Sanchez-Toril, F., McIvor, A., Teichmann, P., Bredenbroeker, D., and Fabbri, L. M. (2007). Effect of 1-year treatment with roflumilast in severe chronic obstructive pulmonary disease. Am. J. Respir. Crit. Care. Med. 176, 154–161. doi:10.1164/rccm.200610-1563O
Camelo, A., Rosignoli, G., Ohne, Y., Stewart, R. A., Overed-Sayer, C., Sleeman, M. A., et al. (2017). IL-33, IL-25, and TSLP induce a distinct phenotypic and activation profile in human type 2 innate lymphoid cells. Blood. Adv. 1 (10), 577–589. doi:10.1182/bloodadvances.2016002352
Chamlin, S. L., Kao, J., Freiden, I. J., Sheu, M. Y., Fowler, A. J., Fluhr, J. W., et al. (2002). Ceramide-dominant barrier repair lipids alleviate childhood atopic dermatitis: changes in barrier function provide a sensitive indicator of disease activity. J. Am. Acad. Dermatol. 47, 198–208. doi:10.1067/mjd.2002.124617
Cho, S. H., Strickland, I., Boguniewicz, M., and Leung, D. Y. (2001). Fibronectin and fibrinogen contribute to the enhanced binding of Staphylococcus aureus to atopic skin. J. Allergy. Clin. Immunol. 108, 269–274. doi:10.1067/mai.2001.117455
Choi, Y. J., Han, K., Jin, E. H., Lim, J. H., Shin, C. M., and Lee, D. H. (2023). Allergic diseases and risk of malignancy of gastrointestinal cancers. Cancers. (Basel) 15(12), 3219. doi:10.3390/cancers15123219
Chong, A. C., Chwa, W. J., and Ong, P. Y. (2022). Aeroallergens in atopic dermatitis and chronic urticaria. Curr. Allergy. Asthma. Rep. 22, 67–75. doi:10.1007/s11882-022-01033-2
Clausen, M. L., Agner, T., Lilje, B., Edslev, S. M., Johannesen, T. B., and Andersen, P. S. (2018). Association of disease severity with skin microbiome and filaggrin gene mutations in adult atopic dermatitis. JAMA. Dermatol. 154 (3), 293–300. doi:10.1001/jamadermatol.2017.5440
Clausen, M. L., Edslev, S. M., Andersen, P. S., Clemmensen, K., Krogfelt, K. A., and Agner, T. (2017). Staphylococcus aureus colonization in atopic eczema and its association with filaggrin gene mutations. Br. J. Dermatol. 177, 1394–1400. doi:10.1111/bjd.15470
Cork, M. J., Danby, S. G., Vasilopoulos, Y., Hadgraft, J., Lane, M. E., Moustafa, M., et al. (2009). Epidermal barrier dysfunction in atopic dermatitis. J. Invest. Dermatol. 129 (8), 1892–1908. doi:10.1038/jid.2009.133
Cork, M. J., Robinson, D. A., Vasilopoulos, Y., Ferguson, A., Moustafa, M., MacGowan, A., et al. (2006). New perspectives on epidermal barrier dysfunction in atopic dermatitis: gene-environment interactions. J. Allergy. Clin. Immunol. 118, 3–21. doi:10.1016/j.jaci.2006.04.042
Czarnowicki, T., He, H., Krueger, J. G., and Guttman-Yassky, E. (2019). Atopic dermatitis endotypes and implications for targeted therapeutics. J. Allergy. Clin. Immunol. 143 (1), 1–11. doi:10.1016/j.jaci.2018.10.032
Czarnowicki, T., Malajian, D., Khattri, S., Correa da Rosa, J., Dutt, R., Finney, R., et al. (2016). Petrolatum: barrier repair and antimicrobial responses underlying this ‘‘inert’’ moisturizer. J. Allergy. Clin. Immunol. 137:1091–1102. doi:10.1016/j.jaci.2015.08.013
Dahlhoff, M., Zouboulis, C. C., and Schneider, M. R. (2016). Expression of dermcidin in sebocytes supports a role for sebum in the constitutive innate defense of human skin. J. Dermatol. Sci. 81, 124–126. doi:10.1016/j.jdermsci.2015.11.013
Danby, S. G., Brown, K., Wigley, A. M., Chittock, J., Pyae, P. K., Flohr, C., et al. (2018). The effect of water hardness on surfactant deposition after washing and subsequent skin irritation in atopic dermatitis patients and healthy control subjects. J. Invest. Dermatol. 138 (1), 68–77. doi:10.1016/j.jid.2017.08.037
Danso, M. O., Boiten, W., van Drongelen, V., Meijling, K. G., Gooris, G., El Ghalbzouri, A., et al. (2017). Altered expression of epidermal lipid bio-synthesis enzymes in atopic dermatitis skin is accompanied by changes in stratum corneum lipid composition. J. Dermatol. Sci. 88, 57–66. doi:10.1016/j.jdermsci.2017.05.005
Darmstadt, G. L., Saha, S. K., Ahmed, A. S. M. N. U., Choi, Y., Chowdhury, M. A. K. A., Islam, M., et al. (2007). Effect of topical emollient treatment of preterm neonates in Bangladesh on invasion of pathogens into the bloodstream. Pediatr. Res. 61, 588–593. doi:10.1203/pdr.0b013e3180459f75
De Pessemier, B., Grine, L., Debaere, M., Maes, A., Paetzold, B., and Callewaert, C. (2021). Gut-skin Axis: current knowledge of the interrelationship between microbial dysbiosis and skin conditions. Microorganisms 9 (2), 353. doi:10.3390/microorganisms9020353
Del Rosso, J., and Friedlander, S. F. (2005). Corticosteroids: options in the era of steroid-sparing therapy. J. Am. Acad. Dermatol. 53 (1), S50–S58. doi:10.1016/j.jaad.2005.04.030
Denda, M., Sato, J., Tsuchiya, T., Elias, P. M., and Feingold, K. R. (1998). Low humidity stimulates epidermal DNA synthesis and amplifies the hyperproliferative response to barrier disruption: implication for seasonal exacerbations of inflammatory dermatoses. J. Invest. Dermatol. 111, 873–878. doi:10.1046/j.1523-1747.1998.00364.x
Diaz, A., and Guttman-Yassky, E. (2019). Topical agents for the treatment of atopic dermatitis. Expert. Rev. Clin. Immunol. 15 (4), 369–382. doi:10.1080/1744666X.2019.1564038
Dizon, M. P., Yu, A. M., Singh, R. K., Wan, J., Chren, M. M., Flohr, C., et al. (2018). Systematic review of atopic dermatitis disease definition in studies using routinely collected health data. Br. J. Dermatol. 178, 1280–1287. doi:10.1111/bjd.16340
Drislane, C., and Irvine, A. D. (2020). The role of filaggrin in atopic dermatitis and allergic disease. Ann. Allergy. Asthma. Immunol. 124, 36–43. doi:10.1016/j.anai.2019.10.008
Eigenmann, P. A., and Calza, A. M. (2000). Diagnosis of IgE-mediated food allergy among Swiss children with atopic dermatitis. Pediatr. Allergy. Immunol. 11, 95–100. doi:10.1034/j.1399-3038.2000.00071.x
Elias, M. S., Long, H. A., Newman, C. F., Wilson, P. A., West, A., McGill, P. J., et al. (2017). Proteomic analysis of filaggrin deficiency identifies molecular signatures characteristic of atopic eczema. J. Allergy. Clin. Immunol. 140, 1299–1309. doi:10.1016/j.jaci.2017.01.039
Elias, P. M. (2005). “Physiologic lipids for barrier repair in dermatology,” in Cosmeceuticals. Editor Z. D. Draelos 1st ed (Philadelphia: Elsevier-Saunders), 63–70.
Elias, P. M. (2005). Stratum corneum defensive functions: an integrated view. J. Invest. Dermatol. 125, 183–200. doi:10.1111/j.0022-202X.2005.23668.x
Elias, P. M., Wakefield, J. S., and Man, M. Q. (2019). Moisturizers versus current and nextgeneration barrier repair therapy for the management of atopic dermatitis. Skin. Pharmacol. Physiol. 32, 1–7. doi:10.1159/000493641
Elias, P. M., Wood, L. C., and Feingold, K. R. (1999). Epidermal pathogenesis of inflammatory dermatoses. Am. J. Contact. Dermatol. 10, 119–126. doi:10.1097/01206501-199909000-00001
Esparza-Gordillo, J., Weidinger, S., Fölster-Holst, R., Bauerfeind, A., Ruschendorf, F., Patone, G., et al. (2009). A common variant on chromosome 11q13 is associated with atopic dermatitis. Nat. Genet. 41, 596–601. doi:10.1038/ng.347
Fang, Z., Lu, W., Zhao, J., Zhang, H., Qian, L., Wang, Q., et al. (2020). Probiotics modulate the gut microbiota composition and immune responses in patients with atopic dermatitis: a pilot study. Eur. J. Nutr. 59, 2119–2130. doi:10.1007/s00394-019-02061-x
Feld, M., Garcia, R., Buddenkotte, J., Katayama, S., Lewis, K., Muirhead, G., et al. (2016). The pruritus- and TH2-associated cytokine IL-31 promotes growth of sensory nerves. J. Allergy. Clin. Immunol. 138, 500–508. doi:10.1016/j.jaci.2016.02.020
Fenner, J., and Silverberg, N. B. (2018). Skin diseases associated with atopic dermatitis. Clin. Dermatol. 36, 631–640. doi:10.1016/j.clindermatol.2018.05.004
Fölster-Holst, R. (2022). The role of the skin microbiome in atopic dermatitis-correlations and consequences. J. Dtsch. Dermatol. Ges. 20 (5), 571–577. doi:10.1111/ddg.14709
Freitas, E., Gooderham, M., and Torres, T. (2022). New topical therapies in development for atopic dermatitis. Drugs 82 (8), 843–853. doi:10.1007/s40265-022-01722-2
Frohm, M., Agerberth, B., Ahangari, G., Stâhle-Bäckdahl, M., Lidén, S., Wigzell, H., et al. (1997). The expression of the gene coding for the antibacterial peptide LL-37 is induced in human keratinocytes during inflammatory disorders. J. Biol. Chem. 272, 15258–15263. doi:10.1074/jbc.272.24.15258
Furue, M., Yamamura, K., Kido-Nakahara, M., Nakahara, T., and Fukui, Y. (2018). Emerging role of interleukin-31 and interleukin-31 receptor in pruritus in atopic dermatitis. Allergy 73, 29–36. doi:10.1111/all.13239
Fuxench, Z. C., Block, J. K., Boguniewicz, M., Boyle, J., Fonacier, L., Gelfand, J. M., et al. (2019). Atopic dermatitis in America study: a cross-sectional study examining the prevalence and disease burden of atopic dermatitis in the US adult population. J. Invest. Dermatol. 139, 583–590. doi:10.1016/j.jid.2018.08.028
Gensollen, T., Iyer, S. S., Kasper, D. L., and Blumberg, R. S. (2016). How colonization by microbiota in early life shapes the immune system. Science 352 (6285), 539–544. doi:10.1126/science.aad9378
Giam, Y. C., Hebert, A. A., Dizon, M. V., Van Bever, H., Tiongco-Recto, M., Kim, K. H., et al. (2016). A review on the role of moisturizers for atopic dermatitis. Asia. Pac. Allergy. 6, 120–128. doi:10.5415/apallergy.2016.6.2.120
Glatz, M., Polley, E., Simpson, E., and Kong, H. (2015). Emollient therapy alters skin barrier and microbes in infants at risk for developing atopic dermatitis. J. Invest. Dermatol. 135, S31. doi:10.1371/journal.pone.0192443
Goerdt, S., Birk, R., Dippel, E., and Orfanos, C. E. (1999). Beyond inflammation: tolerance, immunotherapy and more. Eur. J. Dermatol. 9, 507.
Goodyear, H. M., Spowart, K., and Harper, J. I. (1991). ‘Wet-wrap’ dressings for the treatment of atopic eczema in children. Br. J. Dermatol. 125, 604. doi:10.1111/j.1365-2133.1991.tb14807.x
Guttman-Yassky, E., Diaz, A., Pavel, A. B., Fernandes, M., Lefferdink, R., Erickson, T., et al. (2019). Use of tape strips to detect immune and barrier abnormalities in the skin of children with early-onset atopic dermatitis. JAMA, Dermatol. 155 (12), 1358–1370. doi:10.1001/jamadermatol.2019.2983
Guttman-Yassky, E., Suarez-Fari∼nas, M., Chiricozzi, A., Nograles, K. E., Shemer, A., Fuentes-Duculan, J., et al. (2009). Broad defects in epidermal cornification in atopic dermatitis identified through genomic analysis. J. Allergy. Clin. Immunol. 124, 1235–1244. doi:10.1016/j.jaci.2009.09.031
Haas, K., Weighardt, H., Deenen, R., Köhrer, K., Clausen, B., Zahner, S., et al. (2016). Aryl hydrocarbon receptor in keratinocytes is essential for murine skin barrier integrity. J. Invest. Dermatol. 136 (11), 2260–2269. doi:10.1016/j.jid.2016.06.627
Haftek, M., McAleer, M. A., Jakasa, I., McLean, W. I., Kezic, S., and Irvine, A. D. (2020). Changes in nano-mechanical properties of human epidermal cornified cells in children with atopic dermatitis. Wellcome. Open. Res. 17 (5), 97. doi:10.12688/wellcomeopenres.15729.2
Harding, C. R., Aho, S., and Bosko, C. A. (2013). Filaggrin - revisited. Int. J. Cosmet. Sci. 35, 412–423. doi:10.1111/ics.12049
Hardman, C. S., Chen, Y. L., Salimi, M., Jarrett, R., Johnson, D., Järvinen, V. J., et al. (2017). CD1a presentation of endogenous antigens by group 2 innate lymphoid cells. Sci. Immunol. 22 (18), eaan5918. doi:10.1126/sciimmunol.aan5918
Hatano, Y., Terashi, H., Arakawa, S., and Katagiri, K. (2005). Interleukin-4 suppresses the enhancement of ceramide synthesis and cutaneous permeability barrier functions induced by tumor necrosis factor-alpha and interferon-gamma in human epidermis. J. Investig. Dermatol. 124, 786–792. doi:10.1111/j.0022-202X.2005.23651.x
Honstein, T., and Werfel, T. (2020). The show must go on: an update on clinical experiences and clinical studies on novel pharmaceutical developments for the treatment of atopic dermatitis. Curr. Opin. Allergy. Clin. Immunol. 20 (4), 386–394. doi:10.1097/ACI.0000000000000652
Howell, M. D., Kim, B. E., Gao, P., Grant, A. V., Boguniewicz, M., Debenedetto, A., et al. (2007). Cytokine modulation of atopic dermatitis filaggrin skin expression. J. Allergy. Clin. Immunol. 120, 150–155. doi:10.1016/j.jaci.2007.04.031
Huls, A., Abramson, M. J., Sugiri, D., Fuks, K., Kramer, U., Krutmann, J., et al. (2019). Nonatopic eczema in elderly women: effect of air pollution and genes. J. Allergy. Clin. Immunol. 143, 378–385. doi:10.1016/j.jaci.2018.09.031
Huls, A., Klumper, C., MacIntyre, E. A., Brauer, M., Melen, E., Bauer, M., et al. (2018). Atopic dermatitis: interaction between genetic variants of GSTP1, TNF, TLR2, and TLR4 and air pollution in early life. Pediatr. Allergy. Immunol. 29, 596–605. doi:10.1111/pai.12903
Humeau, M., Boniface, K., and Bodet, C. (2022). Cytokine-mediated crosstalk between keratinocytes and T cells in atopic dermatitis. Front. Immunol. 7 (13), 801579. doi:10.3389/fimmu.2022.801579
Ihekweazu, F. D., and Versalovic, J. (2018). Development of the pediatric gut microbiome: impact on health and disease. Am. J. Med. Sci. 356, 413–423. doi:10.1016/j.amjms.2018.08.005
Jacob, N., Jaiswal, S., Maheshwari, D., Nallabelli, N., Khatri, N., Bhatia, A., et al. (2020). Butyrate induced Tregs are capable of migration from the GALT to the pancreas to restore immunological tolerance during type-1 diabetes. Sci. Rep. 10, 19120. doi:10.1038/s41598-020-76109-y
Janssens, M., van Smeden, J., Gooris, G. S., Bras, W., Portale, G., Caspers, P. J., et al. (2012). Increase in short-chain ceramides correlates with an altered lipid organization and decreased barrier function in atopic eczema patients. J. Lipid. Res. 53, 2755–2766. doi:10.1194/jlr.P030338
Jensen, J. M., Fölster-Holst, R., Baranowsky, A., Schunck, M., Winoto-Morbach, S., Neumann, C., et al. (2004). Impaired sphingomyelinase activity and epidermal differentiation in atopic dermatitis. J. Invest. Dermatol. 122, 1423–1431. doi:10.1111/j.0022-202X.2004.22621.x
Jensen, J. M., Pfeiffer, S., Witt, M., Brautigam, M., Neumann, C., Weichenthal, M., et al. (2009). Different effects of pimecrolimus and betamethasone on the skin barrier in patients with atopic dermatitis. J. Allergy. Clin. Immunol. 124, R19–R28. doi:10.1016/j.jaci.2009.07.015
Ji, J., Shu, X., Li, X., Sundquist, K., Sundquist, J., and Hemminki, K. (2009). Cancer risk in hospitalised asthma patients. Br. J. Cancer. 100, 829–833. doi:10.1038/sj.bjc.6604890
Joo, K. M., Hwang, J. H., Bae, S., Nahm, D. H., Park, H. S., Ye, Y. M., et al. (2015). Relationship of ceramide-and free fatty acid-cholesterol ratios in the stratum corneum with skin barrier function of normal, atopic dermatitis lesional and non-lesional skins. J. Dermatol. Sci. 77, 71–74. doi:10.1016/j.jdermsci.2014.10.001
Jungersted, J. M., and Agner, T. (2013). Eczema and ceramides: an update. Contact. Dermat. 69, 65–71. doi:10.1111/cod.12073
Kadow, S., Jux, B., Zahner, S. P., Wingerath, B., Chmill, S., Clausen, B. E., et al. (2011). Aryl hydrocarbon receptor is critical for homeostasis of invariant gammadelta T cells in the murine epidermis. Immunol 187 (6), 3104–3110. doi:10.4049/jimmunol.1100912
Kantor, R., and Silverberg, J. I. (2017). Environmental risk factors and their role in the management of atopic dermatitis. Expert. Rev. Clin. Immunol. 13 (1), 15–26. doi:10.1080/1744666X.2016.1212660
Katsarou, S., Makris, M., Vakirlis, E., and Gregoriou, S. (2023). The role of tight junctions in atopic dermatitis: a systematic review. J. Clin. Med. 12 (4), 1538. doi:10.3390/jcm12041538
Kawakami, T., Ando, T., Kimura, M., Wilson, B. S., and Kawakami, Y. (2009). Mast cells in atopic dermatitis. Curr. Opin. Immunol. 21 (6), 666–678. doi:10.1016/j.coi.2009.09.006
Kawasaki, H., Nagao, K., Kubo, A., Hata, T., Shimizu, A., Mizuno, H., et al. (2012). Altered stratum corneum barrier and enhanced percutaneous immune responses in filaggrin-null mice. J. Allergy Clin. Immunol. 129, 1538–1546. doi:10.1016/j.jaci.2012.01.068
Keam, S. J. (2022). Nemolizumab: first approval. Drugs 82 (10), 1143–1150. doi:10.1007/s40265-022-01741-z
Kim, B. S., Howell, M. D., Sun, K., Papp, K., Nasir, A., Kuligowski, M. E., et al. (2020). Treatment of atopic dermatitis with ruxolitinib cream (JAK1/JAK2 inhibitor) or triamcinolone cream. J. Allergy. Clin. Immunol. 145 (2), 572–582. doi:10.1016/j.jaci.2019.08.042
Kim, J., Kim, B. E., and Leung, D. Y. M. (2019). Pathophysiology of atopic dermatitis: clinical implications. Allergy. Asthma. Proc. 40, 84–92. doi:10.2500/aap.2019.40.4202
Kim, S. V., Xiang, W. V., Kwak, C., Yang, Y., Lin, X. W., Ota, M., et al. (2013). GPR15-mediated homing controls immune homeostasis in the large intestine mucosa. Science 340, 1456–1459. doi:10.1126/science.1237013
Kim, Y., and Lim, K. M. (2021). Skin barrier dysfunction and filaggrin. Arch. Pharm. Res. 44, 36–48. doi:10.1007/s12272-021-01305-x
Klein, B., Treudler, R., and Simon, J. C. (2022). JAK-Inhibitors indermatology-small molecules, big impact? Overview of the mechanism of action, previous study results and potential adverse effects. J. Dtsch. Dermatol. Ges. 20 (1), 19–24. doi:10.1111/ddg.14668
Klonowska, J., Glen, J., Nowicki, R. J., and Trzeciak, M. (2018). New cytokines in the pathogenesis of atopic dermatitis-new therapeutic targets. Int. J. Mol. Sci. 19, 3086. doi:10.3390/ijms19103086
Kobayashi, T., Glatz, M., Horiuchi, K., Kawasaki, H., Akiyama, H., Kaplan, D. H., et al. (2015). Dysbiosis and Staphylococcus aureus colonization drives inflammation in atopic dermatitis. Immunity 42, 756–766. doi:10.1016/j.immuni.2015.03.014
Kobayashi, T., and Nagao, K. (2019). Host-microbial dialogues in atopic dermatitis. Int. Immunol. 31 (7), 449–456. doi:10.1093/intimm/dxz026
Komatsu, N., Saijoh, K., Kuk, C., Liu, A. C., Khan, S., Shirasaki, F., et al. (2007). Human tissue kallikrein expression in the stratum corneum and serum of atopic dermatitis patients. Exp. Dermatol. 16, 513–519. doi:10.1111/j.1600-0625.2007.00562.x
Kong, H. H., Oh, J., Deming, C., Conlan, S., Grice, E. A., Beatson, M. A., et al. (2012). Temporal shifts in the skin microbiome associated with disease flares and treatment in children with atopic dermatitis. Genome. Res. 22, 850–859. doi:10.1101/gr.131029.111
Kutlu, A., Karabacak, E., Aydin, E., Ozturk, S., Taskapan, O., Aydinoz, S., et al. (2013). Relationship between skin prick and atopic patch test reactivity to aeroallergens and disease severity in children with atopic dermatitis. Allergol. Immunopathol. 41 (6), 369–373. doi:10.1016/j.aller.2013.02.007
Langan, S. M., Irvine, A. D., and Weidinger, S. (2020). Atopic dermatitis. Lancet 396, 345–360. doi:10.1016/S0140-6736(20)31286-1
Lee, A. Y. (2020). Molecular mechanism of epidermal barrier dysfunction as primary abnormalities. Int. J. Mol. Sci. 21 (4), 1194. doi:10.3390/ijms21041194
Lee, H. H., Patel, K. R., Singam, V., Rastogi, S., and Silverberg, J. I. (2019). A systematic review and meta-analysis of the prevalence and phenotype of adult-onset atopic dermatitis. J. Am. Acad. Dermatol. 80, 1526–1532. doi:10.1016/j.jaad.2018.05.1241
Lee, J. H., Lee, S. J., Kim, D., and Bang, D. (2007). The effect of wet-wrap dressing on epidermal barrier in patients with atopic dermatitis. J. Eur. Acad. Dermatol. Venereol. 21 (10), 1360–1368. doi:10.1111/j.1468-3083.2007.02277.x
Lehrer, R. I., Lichtenstein, A. K., and Ganz, T. (1993). Defensins: antimicrobial and cytotoxic peptides of mammalian cells. Annu. Rev. Immunol. 11, 105–128. doi:10.1146/annurev.iy.11.040193.000541
Leung, D. Y. (2000). Atopic dermatitis: new insights and opportunities for therapeutic intervention. J. Allergy. Clin. Immunol. 105 (5), 860–876. doi:10.1067/mai.2000.106484
Levin, J., Friedlander, S. F., and Del Rosso, J. Q. (2013). Atopic dermatitis and the stratum corneum: part 2: other structural and functional characteristics of the stratum corneum barrier in atopic skin. J. Clin. Aesthet. Dermatol 6 (11), 49–54.
Li, S., Ganguli-Indra, G., and Indra, A. K. (2016). Lipidomic analysis of epidermal lipids: a tool to predict progression of inflammatory skin disease in humans. Expert. Rev. proteomic. 13, 451–456. doi:10.1080/14789450.2016.1177462
Liang, J., Hu, F., Dan, M., Sang, Y., Abulikemu, K., Wang, Q., et al. (2022). Safety and efficacy of nemolizumab for atopic dermatitis with pruritus: a systematic review and meta-regression analysis of randomized controlled trials. Front. Immunol. 26 (13), 825312. doi:10.3389/fimmu.2022.825312
Lin, D. H., Nguyen, C., and Fleischer, A. B. (2022). Time to meaningful clinical response in reduction of itch in atopic dermatitis. J. Dermatol. Treat. 33 (3), 1568–1571. doi:10.1080/09546634.2020.1856318
Liu, X., Zhang, X., Zhang, J., Luo, Y., Xu, B., Ling, S., et al. (2020). Activation of aryl hydrocarbon receptor in Langerhans cells by a microbial metabolite of tryptophan negatively regulates skin inflammation. J. Dermatol Sci. 100 (3), 192–200. doi:10.1016/j.jdermsci.2020.10.004
Loden, M. (2003). The skin barrier and use of moisturizers in atopic dermatitis. Clin. Dermatol. 21, 145–157. doi:10.1016/s0738-081x(02)00373-5
Loiseau, N., Obata, Y., Moradian, S., Sano, H., Yoshino, S., Aburai, K., et al. (2013). Altered sphingoid base profiles predict compromised membrane structure and permeability in atopic dermatitis. J. Dermatol. Sci. 72, 296–303. doi:10.1016/j.jdermsci.2013.08.003
Luna, P. C. (2020). Skin microbiome as years go by. Am. J. Clin. Dermatol. 21 (1), 12–17. doi:10.1007/s40257-020-00549-5
Man, M. Q., Hatano, Y., Lee, S. H., Man, M., Chang, S., Feingold, K. R., et al. (2008). Characterization of a hapten-induced, murine model with multiple features of atopic dermatitis: structural, immunologic, and biochemical changes following single versus multiple oxazolone challenges. J. Investig. Dermatol. 128, 79–86. doi:10.1038/sj.jid.5701011
Mansfield, K. E., Schmidt, S. A. J., Darvalics, B., Mulick, A., Abuabara, K., Wong, A. Y. S., et al. (2020). Association between atopic eczema and cancer in england and Denmark. JAMA Dermatol 156(10), 1086–1097. doi:10.1001/jamadermatol.2020.1948
Marks, R. (2004). The stratum corneum barrier: the final frontier. J. Nutr. 134, 2017S. –21S. doi:10.1093/jn/134.8.2017S
Martin, P. E., Eckert, J. K., Koplin, J., Lowe, A., Gurrin, L., Dharmage, S., et al. (2014). Which infants with eczema are at risk of food allergy? Results from a population-based cohort. Clin. Exp. Allergy. 45, 255–264. doi:10.1111/cea.12406
Martín-Santiago, A., Puig, S., Arumi, D., and Rebollo Laserna, F. J. (2022). Safety profile and tolerability of topical phosphodiesterase 4 inhibitors for the treatment of atopic dermatitis: a systematic review and meta-analysis. Curr. Ther. Res. Clin. Exp. 23, 100679. doi:10.1016/j.curtheres.2022.100679
Martínez, J. E., Vargas, A., Pérez-Sánchez, T., Encío, I. J., Cabello-Olmo, M., and Barajas, M. (2021). Human microbiota network: unveiling potential crosstalk between the different microbiota ecosystems and their role in health and disease, Nutrients 13(9), 2905. doi:10.3390/nu13092905
Maruho (2022). Maruho acquires manufacturing and marketing approval in Japan for "Mitchga® Subcutaneous Injection 60mg Syringes", a new treatment targeting itch associated with atopic dermatitis. Available at: https://www.maruho.co.jp/english/information/20220328.html.
Matsui, T., and Amagai, M. (2015). Dissecting the formation, structure and barrier function of the stratum corneum. Int. Immunol. 27 (6), 269–80. doi:10.1093/intimm/dxv013
Matsunaga, M. C., and Yamauchi, P. S. (2016). IL-4 and IL-13 inhibition in atopic dermatitis. J. Drugs. Dermatol. 15, 925–929.
McAleer, J. P., Fan, J., Roar, B., Primerano, D. A., and Denvir, J. (2018). Cytokine regulation in human CD4 T cells by the aryl hydrocarbon receptor and gq-coupled receptors. Sci. Rep. 8 (1), 10954. doi:10.1038/s41598-018-29262-4
Meckfessel, M., and Brandt, S. (2014). The structure, function, and importance of ceramides in skin and their use as therapeutic agents in skin-care products. J. Am. Acad. Dermatol. 71, 177–184. doi:10.1016/j.jaad.2014.01.891
Meylan, P., Lang, C., Mermoud, S., Johannsen, A., Norrenberg, S., Hohl, D., et al. (2017). Skin colonization by Staphylococcus aureus precedes the clinical diagnosis of atopic dermatitis in infancy. J. Invest. Dermatol. 137 (12), 2497–2504. doi:10.1016/j.jid.2017.07.834
Mielke, L. A., Groom, J. R., Rankin, L. C., Seillet, C., Masson, F., Putoczki, T., et al. (2013). TCF-1 controls ILC2 and NKp46+RORγt+ innate lymphocyte differentiation and protection in intestinal inflammation. J. Immunol. 191, 4383–4391. doi:10.4049/jimmunol.1301228
Miron, Y., Miller, P. E., Hughes, C., Indersmitten, T., Lerner, E. A., and Cevikbas, F. J. (2022). Mechanistic insights into the antipruritic effects of lebrikizumab, an anti-IL-13 mAb. Allergy. Clin. Immunol. 150 (3), 690–700. doi:10.1016/j.jaci.2022.01.028
Mittal, M., Siddiqui, M. R., Tran, K., Reddy, S. P., and Malik, A. B. (2014). Reactive oxygen species in inflammation and tissue injury. Antioxid. Redox. Signal. 20 (7), 1126–1167. doi:10.1089/ars.2012.5149
Moosbrugger-Martinz, V., Hackl, H., Gruber, R., Pilecky, M., Knabl, L., Orth-Höller, D., et al. (2021). Initial evidence of distinguishable bacterial and fungal dysbiosis in the skin of patients with atopic dermatitis or Netherton syndrome. J. Invest. Dermatol. 141 (1), 114–123. doi:10.1016/j.jid.2020.05.102
Moosbrugger-Martinz, V., Leprince, C., Méchin, M. C., Simon, M., Blunder, S., Gruber, R., et al. (2022). Revisiting the roles of filaggrin in atopic dermatitis. Int. J. Mol. Sci. 23 (10), 5318. doi:10.3390/ijms23105318
Morar, N., Cookson, W. O., Harper, J. I., and Moffatt, M. F. (2007). Filaggrin mutations in children with severe atopic dermatitis. J. Invest. Dermatol. 127, 1667–1672. doi:10.1038/sj.jid.5700739
Morimura, S., Sugaya, M., Oka, T., Suga, H., Miyagaki, T., Tsunemi, Y., et al. (2021). Increased regulatory T cells and decreased myeloid-derived suppressor cells induced by high CCL17 levels may account for normal incidence of cancers among patients with atopic dermatitis. Int. J. Mol. Sci. 22 (4), 2025. doi:10.3390/ijms22042025
Myles, I. A., Castillo, C. R., Barbian, K. D., Kanakabandi, K., Virtaneva, K., Fitzmeyer, E., et al. (2020). Therapeutic responses to Roseomonas mucosa in atopic dermatitis may involve lipid-mediated TNF-related epithelial repair. Sci. Transl. Med. 12 (560), eaaz8631. doi:10.1126/scitranslmed.aaz8631
Myles, I. A., Earland, N. J., Anderson, E. D., Moore, I. N., Kieh, M. D., Williams, K. W., et al. (2018). First-in-human topical microbiome transplantation with Roseomonas mucosa for atopic dermatitis. JCI Insight 3 (9), e120608. doi:10.1172/jci.insight.120608
N'Diaye, A., Gannesen, A., Borrel, V., Maillot, O., Enault, J., Racine, P. J., et al. (2017). Substance P and calcitonin gene-related peptide: key regulators of cutaneous microbiota homeostasis. Front. Endocrinol. (Lausanne) 30 (8), 15. doi:10.3389/fendo.2017.00015
Nakagawa, H., Nemoto, O., Igarashi, A., Saeki, H., Kaino, H., and Nagata, T. (2020). Delgocitinib ointment, a topical Janus kinase inhibitor, in adult patients with moderate to severe atopic dermatitis: a phase 3, randomized, double-blind, vehicle-controlled study and an open-label, long-term extension study. J. Am. Acad. Dermatol. 82, 823–831. doi:10.1016/j.jaad.2019.12.015
Nakatsuji, T., Chen, T. H., Narala, S., Chun, K. A., Two, A. M., Yun, T., et al. (2017). Antimicrobials from human skin commensal bacteria protect against Staphylococcus aureus and are deficient in atopic dermatitis. Sci. Transl. Med. 9, eaah4680. doi:10.1126/scitranslmed.aah4680
Nakatsuji, T., Chen, T. H., Two, A. M., Chun, K. A., Narala, S., Geha, R. S., et al. (2016). Staphylococcus aureus exploits epidermal barrier defects in atopic dermatitis to trigger cytokine expression. J. Invest. Dermatol. 136, 2192–2200. doi:10.1016/j.jid.2016.05.127
Nakatsuji, T., Hata, T. R., Tong, Y., Cheng, J. Y., Shafiq, F., Butcher, A. M., et al. (2021). Development of a human skin commensal microbe for bacteriotherapy of atopic dermatitis and use in a phase 1 randomized clinical trial. Nat. Med. 27 (4), 700–709. doi:10.1038/s41591-021-01256-2
Nakatsuji, T., Kao, M. C., Zhang, L., Zouboulis, C. C., Gallo, R. L., and Huang, C. M. (2010). Sebum free fatty acids enhance the innate immune defense of human sebocytes by upregulating β-defensin-2 expression. J. Investig. Dermatol. 130, 985–994. doi:10.1038/jid.2009.384
Nemoto, O., Furue, M., Nakagawa, H., Shiramoto, M., Hanada, R., Matsuki, S., et al. (2016). The first trial of CIM331, a humanized antihuman interleukin-31 receptor A antibody, in healthy volunteers and patients with atopic dermatitis to evaluate safety, tolerability and pharmacokinetics of a single dose in a randomized, double-blind, placebo-controlled study. Br. J. Dermatol. 174, 296–304. doi:10.1111/bjd.14207
Nguyen, H. L. T., Trujillo-Paez, J. V., Umehara, Y., Yue, H., Peng, G., Kiatsurayanon, C., et al. (2020). Role of antimicrobial peptides in skin barrier repair in individuals with atopic dermatitis. Int. J. Mol. Sci. 21, 7607. doi:10.3390/ijms21207607
Niyonsaba, F., Kiatsurayanon, C., Chieosilapatham, P., and Ogawa, H. (2017). Friends or foes? Host defense (antimicrobial) peptides and proteins in human skin diseases. Exp. Dermatol. 26, 989–998. doi:10.1111/exd.13314
Nopper, A. J., Horii, K. A., Sookdeo-Drost, S., Wang, T. H., Mancini, A. J., and Lane, A. T. (1996). Topical ointment therapy benefits premature infants. J. Pediatr. 128, 660–669. doi:10.1016/s0022-3476(96)80132-6
Nowicka, D., Chilicka, K., and Dzieńdziora-Urbińska, I. (2022). Host-Microbe interaction on the skin and its role in the pathogenesis and treatment of atopic dermatitis. Pathogens 11 (1), 71. doi:10.3390/pathogens11010071
Ohnishi, Y., Okino, N., Ito, M., and Imayama, S. (1999). Ceramidase activity in bacterial skin flora as a possible cause of ceramide deficiency in atopic dermatitis. Clin. Diagn. Lab. Immunol. 6, 101–104. doi:10.1128/CDLI.6.1.101-104.1999
Ong, P. Y., Ohtake, T., Brandt, C., Strickland, I., Boguniewicz, M., Ganz, T., et al. (2002). Endog-enous antimicrobial peptides and skin infections in atopic dermatitis. N. Engl. J. Med. 347, 1151–1160. doi:10.1056/NEJMoa021481
Owji, S., Caldas, S. A., and Ungar, B. (2022). Management of atopic dermatitis: clinical utility of ruxolitinib. J. Asthma. Allergy. 25 (15), 1527–1537. doi:10.2147/JAA.S342051
Oyama, S., Kitamura, H., Kuramochi, T., Higuchi, Y., Matsushita, H., Suzuki, T., et al. (2018). Cynomolgus monkey model of interleukin-31-induced scratching depicts blockade of human interleukin-31 receptor A by a humanized monoclonal antibody. Exp. Dermatol. 27, 14–21. doi:10.1111/exd.13236
Oyoshi, M. K., He, R., Kumar, L., Yoon, J., and Geha, R. S. (2009). Cellular and molecular mechanisms in atopic dermatitis. Adv. Immunol. 102, 135–226. doi:10.1016/S0065-2776(09)01203-6
Pahar, B., Madonna, S., Das, A., Albanesi, C., and Girolomoni, G. (2020). Immunomodulatory role of the antimicrobial LL-37 peptide in autoimmune diseases and viral infections. Vaccines 8, 517. doi:10.3390/vaccines8030517
Paller, A. S., Kong, H. H., Seed, P., Naik, S., Scharschmidt, T. C., Gallo, R. L., et al. (2019). The microbiome in patients with atopic dermatitis. J. Allergy. Clin. Immunol. 143, 26–35. doi:10.1016/j.jaci.2018.11.015
Paller, A. S., Spergel, J. M., Mina-Osorio, P., and Irvine, A. D. (2019). The atopic march and atopic multi-morbidity: many trajectories, many pathways. J. Allergy. Clin. Immunol. 143, 46–55. doi:10.1016/j.jaci.2018.11.006
Papapostolou, N., Xepapadaki, P., Gregoriou, S., and Makris, M. (2022). Atopic dermatitis and food allergy: a complex interplay what we know and what we would like to learn. J. Clin. Med. 11 (14), 4232. doi:10.3390/jcm11144232
Papp, K. A., Kaufmann, R., Thaçi, D., Hu, C., Sutherland, D., Rohane, P., et al. (2013). Efficacy and safety of apremilast in subjects with moderate to severe plaque psoriasis: results from a phase II, multicenter, randomized, double-blind, placebo-controlled, parallel-group, dose-comparison study. J. Eur. Acad. Dermatol. Venereol. 27, e376–e383. doi:10.1111/j.1468-3083.2012.04716.x
Park, D. H., Kim, J. W., Park, H. J., and Hahm, D. H. (2021). Comparative analysis of the microbiome across the gut-skin Axis in atopic dermatitis. Int. J. Mol. Sci. 22 (8), 4228. doi:10.3390/ijms22084228
Patrick, G. J., Archer, N. K., and Miller, L. S. (2021). Which way do we go? Complex interactions in atopic dermatitis pathogenesis. J. Invest. Dermatol. 141, 274–284. doi:10.1016/j.jid.2020.07.006
Pavel, P., Blunder, S., Moosbrugger-Martinz, V., Elias, P. M., and Dubrac, S. (2022). Atopic dermatitis: the fate of the fat. Int. J. Mol. Sci. 23 (4), 2121. doi:10.3390/ijms23042121
Pelc, J., Czarnecka-Operacz, M., and Adamski, Z. (2018). Structure and function of the epidermal barrier in patients with atopic dermatitis-treatment options. Part one. Postepy. Dermatol. Alergol. 35 (1), 1–5. doi:10.5114/ada.2018.73159
Penders, J., Thijs, C., van den Brandt, P. A., Kummeling, I., Snijders, B., Stelma, F., et al. (2007). Gut microbiota composition and development of atopic manifestations in infancy: the KOALA birth cohort study. Gut 56, 661–667. doi:10.1136/gut.2006.100164
Peroni, D. G., Nuzzi, G., Trambusti, I., Di Cicco, M. E., and Comberiati, P. (2020). Microbiome composition and its impact on the development of allergic diseases. Front. Immunol. 23 (11). doi:10.3389/fimmu.2020.00700
Popovic, B., Breed, J., Rees, D. G., Gardener, M. J., Vinall, L. M. K., Kemp, B., et al. (2017). Structural characterisation reveals mechanism of IL-13-neutralising monoclonal antibody tralokinumab as inhibition of binding to IL-13rα1 and IL-13rα2. J. Mol. Biol. 429 (2), 208–219. doi:10.1016/j.jmb.2016.12.005
Prescott, S. L., Larcombe, D. L., Logan, A. C., West, C., Burks, W., Caraballo, L., et al. (2017). The skin microbiome: impact of modern environments on skin ecology, barrier integrity, and systemic immune programming. World. Allergy. Organ. J. 10, 29. doi:10.1186/s40413-017-0160-5
Proksch, E., Fölster-Holst, R., and Jensen, J. M. (2006). Skin barrier function, epidermal proliferation and differentiation in eczema. J. Dermatol. Sci. 43, 159–169. doi:10.1016/j.jdermsci.2006.06.003
Radi, G., Campanti, A., Diotallevi, F., Martina, E., Marani, A., and Offidani, A. (2022). A systematic review of atopic dermatitis: the intriguing journey starting from physiopathology to treatment, from laboratory bench to bedside. Biomedicines 10 (11), 2700. Published online 2022 Oct 25. doi:10.3390/biomedicines10112700
Reinholz, M., Ruzicka, T., and Schauber, J. (2012). Cathelicidin LL37: an anti microbial peptide with a role in inflammatory skin disease. Ann. Dermatol 24, 126–135. doi:10.5021/ad.2012.24.2.126
Renert-Yuval, Y., Del Duca, E., Pavel, A. B., Fang, M., Lefferdink, R., Wu, J., et al. (2021). The molecular features of normal and atopic dermatitis skin in infants, children, adolescents, and adults. J. Allergy Clin. Immunol. 148, 148–163. doi:10.1016/j.jaci.2021.01.001
Rustad, A. M., Nickles, M. A., Bilimoria, S. N., and Lio, P. A. (2022). The role of diet modification in atopic dermatitis: navigating the complexity. Am. J. Clin. Dermatol. 23 (1), 27–36. doi:10.1007/s40257-021-00647-y
Rutter, C. E., Silverwood, R. J., Williams, H. C., Ellwood, P., Asher, I., Garcia-Marcos, L., et al. (2019). Are environmental factors for atopic eczema in ISAAC Phase Three due to reverse causation? J. Invest. Dermatol. 139, 1023–1036. doi:10.1016/j.jid.2018.08.035
Sakamoto, K., Jin, S. P., Goel, S., Jo, J. H., Voisin, B., Kim, D., et al. (2021). Disruption of the endopeptidase ADAM10-Notch signaling axis leads to skin dysbiosis and innate lymphoid cell-mediated hair follicle destruction. Immunity 54(10), 2321–2337.e10. doi:10.1016/j.immuni.2021.09.001
Sargen, M. R., Hoffstad, O., and Margolis, D. J. (2014). Warm, humid, and high sun exposure climates are associated with poorly controlled eczema: peer (pediatric eczema elective registry) cohort 2004–2012. J. Invest. Dermatol. 134, 51–57. doi:10.1038/jid.2013.274
Scharschmidt, T. C., Vasquez, K. S., Truong, H. A., Gearty, S. V., Pauli, M. L., Nosbaum, A., et al. (2015). A wave of regulatory T cells into neonatal skin MediatesToleranceto commensal microbes. Immunity 43 (5), 1011–1021. doi:10.1016/j.immuni.2015.10.016
Schleimer, R. P., and Berdnikovs, S. (2017). Etiology of epithelial barrier dysfunction in patients with type 2 inflammatory diseases. J. Allergy. Clin. Immunol. 139 (6), 1752–1761. doi:10.1016/j.jaci.2017.04.010
Schlievert, P. M., Strandberg, K. L., Lin, Y. C., Peterson, M. L., and Leung, D. Y. (2010). Secreted virulence factor comparison between methicillin-resistant and methicillin-sensitive Staphylococcus aureus, and its relevance to atopic dermatitis. J. Allergy. Clin. Immunol. 125, 39–49. doi:10.1016/j.jaci.2009.10.039
Schoepe, S., Schäcke, H., May, E., and Asadullah, K. (2006). Glucocorticoid therapy-induced skin atrophy. Exp. Dermatol. 15 (6), 406–420. doi:10.1111/j.0906-6705.2006.00435.x
Sehra, S., Yao, Y., Howell, M. D., Nguyen, E. T., Kansas, G. S., Leung, D. Y., et al. (2010). IL-4 regulates skin homeostasis and the predisposition toward allergic skin inflammation. J. Immunol. 184, 3186–3190. doi:10.4049/jimmunol.0901860
Sezin, T., Jegodzinski, L., Meyne, L. M., Gupta, Y., Mousavi, S., Ludwig, R. J., et al. (2021). The G protein-coupled receptor 15 (GPR15) regulates cutaneous immunology by maintaining dendritic epidermal T cells and regulating the skin microbiome. Eur. J. Immunol. 51 (6), 1390–1398. doi:10.1002/eji.202048887
Shlivko, I. L., Kamensky, V. A., Donchenko, E. V., and Agrba, P. (2014). Morphological changes in skin of different phototypes under the action of topical corticosteroid therapy and tacrolimus. Skin. Res. Technol. 20 (2), 136–140. doi:10.1111/srt.12095
Sidbury, R., Davis, D. M., Cohen, D. E., Cordoro, K. M., Berger, T. G., Bergman, J. N., et al. (2014). Guidelines of care for the management of atopic dermatitis: section 3. Management and treatment with phototherapy and systemic agents. J. Am. Acad. Dermatol. 71, 327–349. doi:10.1016/j.jaad.2014.03.030
Silverberg, J. I., Hanifin, J., and Simpson, E. L. (2013). Climatic factors are associated with childhood eczema prevalence in the United States. J. Invest. Dermatol. 133 (7), 1752–1759. doi:10.1038/jid.2013.19
Simpson, E. L. (2010). Atopic dermatitis: a review of topical treatment options. Curr. Med. Res. Opin. 26 (3), 633–640. doi:10.1185/03007990903512156
Simpson, E. L., Merola, J. F., Silverberg, J. I., Reich, K., Warren, R. B., Staumont-Sallé, D., et al. (2022). Safety of tralokinumab in adult patients with moderate-to-severe atopic dermatitis: pooled analysis of five randomized, double-blind, placebo-controlled phase II and phase III trials. Br. J. Dermatol. 9, 888–899. doi:10.1111/bjd.21867
Singh, B., Jegga, A. G., Shanmukhappa, K. S., Edukulla, R., Khurana Hershey, G. H., Medvedovic, M., et al. (2016). IL-31-Driven skin remodeling involves epidermal cell proliferation and thickening that lead to impaired skin-barrier function. PloS. One. 11, e0161877. doi:10.1371/journal.pone.0161877
Skov, L., Olsen, J. V., Giorno, R., Schlievert, P. M., Baadsgaard, O., and Leung, D. Y. (2000). Application of Staphylococcal enterotoxin B on normal and atopic skin induces up-regulation of T cells by a superantigen-mediated mechanism. J. Allergy. Clin. Immunol. 105, 820–826. doi:10.1067/mai.2000.105524
Smits, J. P. H., Ederveen, T. H. A., Rikken, G., van den Brink, N. J. M., van Vlijmen-Willems, I. M. J. J., Boekhorst, J., et al. (2020). Targeting the cutaneous microbiota in atopic dermatitis by coal tar via AHR-dependent induction of antimicrobial peptides. J. Invest. Dermatol. 140 (2), 415–424. doi:10.1016/j.jid.2019.06.142
Ständer, S. (2021). Atopic dermatitis. N. Engl. J. Med. 384 (12), 1136–1143. doi:10.1056/NEJMra2023911
Staubach, P., and Lunter, D. J. (2014). Basic or maintenance therapy in dermatology. Appropriate vehicles, possibilities and limitations. Hautarzt 65 (1), 63–72. doi:10.1007/s00105-013-2726-7
Szántó, M., Dózsa, A., Antal, D., Szabó, K., Kemény, L., and Bai, P. (2019). Targeting the gut-skin axis-Probiotics as new tools for skin disorder management? Exp. Dermatol. 28 (11), 1210–1218. doi:10.1111/exd.14016
Szczepanowska, J., Reich, A., and Szepietowski, J. C. (2008). Emollients improve treatment results with topical corticosteroids in childhood atopic dermatitis: a randomized comparative study. Pediatr. Allergy. Immunol. 19, 614–618. doi:10.1111/j.1399-3038.2007.00706.x
Szegedi, K., Lutter, R., Res, P. C., Bos, J. D., Luiten, R. M., Kezic, S., et al. (2015). Cytokine profiles in interstitial fluid from chronic atopic dermatitis skin. J. Eur. Acad. Dermatol. Venereol. 29 (11), 2136–2144. doi:10.1111/jdv.13160
Tamoutounour, S., Han, S. J., Deckers, J., Constantinides, M. G., Hurabielle, C., Harrison, O. J., et al. (2019). Keratinocyte-intrinsic MHCII expression controls microbiota-induced Th1 cell responses. Proc. Natl. Acad. Sci. U. S. A. 116 (47), 23643–23652. doi:10.1073/pnas.1912432116
Tazawa, T., Sugiura, H., Sugiura, Y., and Uehara, M. (2004). Relative importance of IL-4 and IL-13 in lesional skin of atopic dermatitis. Arch. Dermatol. Res. 295 (11), 459–464. doi:10.1007/s00403-004-0455-6
Thyssen, J. P., and Kezic, S. (2014). Causes of epidermal filaggrin reduction and their role in the pathogenesis of atopic dermatitis. J. Allergy. Clin. Immunol. 134, 792–799. doi:10.1016/j.jaci.2014.06.014
Tokura, Y., and Hayano, S. (2022). Subtypes of atopic dermatitis: from phenotype to endotype. Allergol. Int. 71 (1), 14–24. doi:10.1016/j.alit.2021.07.003
Toncic, R. J., Jakasa, I., Hadzavdic, S. L., Goorden, S. M., Vlugt, K. J. G., Stet, F. S., et al. (2020). Altered levels of sphingosine, sphinganine and their ceramides in atopic dermatitis are related to skin barrier function, disease severity and local cytokine milieu. Int. J. Mol. Sci. 13 (6), 1958. doi:10.3390/ijms21061958
Totsuka, A., Omori-Miyake, M., Kawashima, M., Yagi, J., and Tsunemi, Y. (2017). Expression of keratin 1, keratin 10, desmoglein 1 and desmocollin 1 in the epidermis: possible downregulation by interleukin-4 and interleukin-13 in atopic dermatitis. Eur. J. Dermatol. 27 (3), 247–253. doi:10.1684/ejd.2017.2985
Totté, J. E., van der Feltz, W. T., Hennekam, M., Van Belkum, A., Van Zuuren, E. J., and Pasmans, S. G. (2016). Prevalence and odds of Staphylococcus aureus carriage in atopic dermatitis: a systematic review and meta-analysis. Br. J. Dermatol. 175, 687–695. doi:10.1111/bjd.14566
Tsoi, L. C., Rodriguez, E., Degenhardt, F., Baurecht, H., Wehkamp, U., Volks, N., et al. (2019). Atopic dermatitis is an IL-13–dominant disease with greater molecular heterogeneity compared to psoriasis. J. Invest. Dermatol. 139 (7), 1480–1489. doi:10.1016/j.jid.2018.12.018
Twitchen, L. J., and Lowe, A. J. (1998). Atopic eczema and wet-wrap dressings. Prof. Nurse. 14, 113–116.
Uberoi, A., Bartow-McKenney, C., Zheng, Q., Flowers, L., Campbell, A., Knight, S. A. B., et al. (2021). Commensal microbiota regulates skin barrier function and repair via signaling through the aryl hydrocarbon receptor. Cell Host Microbe 29 (8), 1235–1248.e8. doi:10.1016/j.chom.2021.05.011
Uluçkan, Ö., Jiménez, M., Roediger, B., Schnabl, J., Díez-Córdova, L. T., Troulé, K., et al. (2019). Cutaneous immune cell-microbiota interactions are controlled by epidermal JunB/AP-1. Cell Rep. 29 (4), 844–859. doi:10.1016/j.celrep.2019.09.042
Ungar, B., Garcet, S., Gonzalez, J., Dhingra, N., Correa da Rosa, J., Shemer, A., et al. (2017). An integrated model of atopic dermatitis biomarkers highlights the systemic nature of the disease. J. Invest. Dermatol. 137 (3), 603–613. doi:10.1016/j.jid.2016.09.037
van Smeden, J., and Bouwstra, J. A. (2016). Stratum corneum lipids: their role for the skin barrier function in healthy subjects and atopic dermatitis patients. Curr. Probl. Dermatol 49, 8–26. doi:10.1159/000441540
van Smeden, J., Janssens, M., Gooris, G. S., and Bouwstra, J. A. (2014). The important role of stratum corneum lipids for the cutaneous barrier function. Biochim. Biophys. Acta. 1841, 295–313. doi:10.1016/j.bbalip.2013.11.006
van Smeden, J., Janssens, M., Kaye, E. C., Caspers, P. J., Lavrijsen, A. P., Vreeken, R. J., et al. (2014). The importance of free fatty acid chain length for the skin barrier function in atopic eczema patients. Exp. Dermatol. 23, 45–52. doi:10.1111/exd.12293
Vittrup, I. (2023). Malignancy risk in atopic dermatitis: heterogenous effects and perspectives. Br. J. Dermatol 189 (1), 4–5. doi:10.1093/bjd/ljad119
Vocks, E., Busch, R., Frohlich, C., Borelli, S., Mayer, H., and Ring, J. (2001). Influence of weather and climate on subjective symptom intensity in atopic eczema. Int. J. Biometeorol. 45, 27–33. doi:10.1007/s004840000077
Wan, J., Shin, D. B., Syed, M. N., Abuabara, K., Lemeshow, A. R., Fuxench, Z. C. C., et al. (2023). Malignancy risk in patients with atopic dermatitis: a population-based cohort study. Br. J. Dermatol. 189 (1), 53–61. doi:10.1093/bjd/ljad072
Wassmann-Otto, A., Heratizadeh, A., Wichmann, K., and Werfel, T. (2018). Birch pollen-related foods can cause late eczematous reactions in patients with atopic dermatitis. Allergy 73 (10), 2046–2054. doi:10.1111/all.13454
Werfel, T., Heratizadeh, A., Niebuhr, M., Kapp, A., Roesner, L. M., Karch, A., et al. (2015). Exacerbation of atopic dermatitis on grass pollen exposure in an environmental challenge chamber. J. Allergy. Clin. Immunol. 136 (1), 96–103. doi:10.1016/j.jaci.2015.04.015
Wertz, P. (2018). Epidermal lamellar granules. Skin. Pharmacol. Physiol. 31, 262–268. doi:10.1159/000491757
Williams, M. R., Cau, L., Wang, Y., Kaul, D., Sanford, J. A., Zaramela, L. S., et al. (2020). Interplay of staphylococcal and host proteases promotes skin barrier disruption in Netherton syndrome. Cell. Rep. 30 (9), 2923–2933. doi:10.1016/j.celrep.2020.02.021
Wohlrab, J., Gebert, A., and Neubert, R. H. H. (2018). Lipids in the skin and pH. Curr. Probl. Dermatol. 54, 64–70. doi:10.1159/000489519
Wollenberg, A., Barbarot, S., Bieber, T., Christen-Zaech, S., Deleuran, M., Fink-Wagner, A., et al. (2018). Consensus-based European guidelines for treatment of atopic eczema (atopic dermatitis) in adults and children: part I. J. Eur. Acad. Dermatol. Venereol. 32, 657–682. doi:10.1111/jdv.14891
Wollenberg, A., Christen-Zach, S., Taieb, A., Paul, C., Thyssen, J. P., de Bruin-Weller, M., et al. (2020). ETFAD/EADV Eczema task force 2020 position paper on diagnosis and treatment of atopic dermatitis in adults and children. J. Eur. Acad. Dermatol. Venereol. 34 (12), 2717–2744. doi:10.1111/jdv.16892
Woo, Y. R., Cho, S. H., Lee, J. D., and Kim, H. S. (2022). The human microbiota and skin cancer. Int. J. Mol. Sci. 23 (3), 1813. doi:10.3390/ijms23031813
Yokouchi, M., Kubo, A., Kawasaki, H., Yoshida, K., Ishii, K., Furuse, M., et al. (2015). Epidermal tight junction barrier function is altered by skin inflammation, but not by filaggrin-deficient stratum corneum. J. Dermatol. Sci. 77, 28–36. doi:10.1016/j.jdermsci.2014.11.007
Yuki, T., Komiya, A., Kusaka, A., Kuze, T., Sugiyama, Y., and Inoue, S. (2013). Impaired tight junctions obstruct stratum corneum formation by altering polar lipid and profilaggrin processing. J. Dermatol. Sci. 69, 148–158. doi:10.1016/j.jdermsci.2012.11.595
Zaniboni, M. C., Samorano, L. P., Orfali, R. L., and Aoki, V. (2016). Skin barrier in atopic dermatitis: beyond filaggrin. An. Bras. Dermatol. 91 (4), 472–478. doi:10.1590/abd1806-4841.20164412
Zhang, L., Du, D., Wang, L., Guo, L., and Jiang, X. J. (2021). Efficacy and safety of topical Janus kinase and phosphodiesterase inhibitor-4 inhibitors for the treatment of atopic dermatitis: a network meta-analysis. Dermatol 48 (12), 1877–1883. doi:10.1111/1346-8138.16126
Zhang, L. J., and Gallo, R. L. (2016). Antimicrobial peptides. Curr. Biol. 26 (1), R14–R19. doi:10.1016/j.cub.2015.11.017
Zhang, Y., Jing, D., Cheng, J., Chen, X., Shen, M., and Liu, H. (2022). The efficacy and safety of IL-13 inhibitors in atopic dermatitis: a systematic review and meta-analysis. Front. Immunol. 27 (13), 923362. doi:10.3389/fimmu.2022.923362
Keywords: skin microbiome, atopic dermatitis, keratinocytes, epidermal barrier, filaggrin
Citation: Çetinarslan T, Kümper L and Fölster-Holst R (2023) The immunological and structural epidermal barrier dysfunction and skin microbiome in atopic dermatitis-an update. Front. Mol. Biosci. 10:1159404. doi: 10.3389/fmolb.2023.1159404
Received: 05 February 2023; Accepted: 04 August 2023;
Published: 16 August 2023.
Edited by:
Ryan O’Shaughnessy, Queen Mary University of London, United KingdomReviewed by:
Pradeep Bist, Duke-NUS Medical School, SingaporeZhiqiang Song, Army Medical University, China
Miguel Bernabe-Rubio, King’s College London, United Kingdom
Copyright © 2023 Çetinarslan, Kümper and Fölster-Holst. This is an open-access article distributed under the terms of the Creative Commons Attribution License (CC BY). The use, distribution or reproduction in other forums is permitted, provided the original author(s) and the copyright owner(s) are credited and that the original publication in this journal is cited, in accordance with accepted academic practice. No use, distribution or reproduction is permitted which does not comply with these terms.
*Correspondence: Tubanur Çetinarslan, t_sarmis@windowslive.com