- 1Department of Toxicology, School of Public Health, Jilin University, Changchun, China
- 2Department of Pediatrics, First Hospital of Jilin University, Jilin University, Changchun, China
Pre-B-cell leukemia homeobox transcription factor 1 (PBX1) is a member of the TALE (three-amino acid loop extension) family and functions as a homeodomain transcription factor (TF). When dimerized with other TALE proteins, it can act as a pioneer factor and provide regulatory sequences via interaction with partners. In vertebrates, PBX1 is expressed during the blastula stage, and its germline variations in humans are interrelated with syndromic anomalies of the kidney, which plays an important role in hematopoiesis and immunity among vertebrates. Herein, we summarize the existing data on PBX1 functions and the impact of PBX1 on renal tumors, PBX1-deficient animal models, and blood vessels in mammalian kidneys. The data indicated that the interaction of PBX1 with different partners such as the HOX genes is responsible for abnormal proliferation and variation of the embryonic mesenchyme, while truncating variants were shown to cause milder phenotypes (mostly cryptorchidism and deafness). Although such interactions have been identified to be the cause of many defects in mammals, some phenotypic variations are yet to be understood. Thus, further research on the TALE family is required.
1 Introduction
PBX1 was originally identified as part of a fusion protein caused by chromosomal translocation t (1; 19) in pre-B cell acute lymphoblastic leukemia. It was later renamed to PBX1 to distinguish it from the prolactin gene PRL-1 (Kamps et al., 1991). PBX1 encodes the PBX1 protein, and along with PBX2–4 belongs to a family of highly conserved homeodomain proteins (Monica et al., 1991; Wagner et al., 2001). It includes a homeodomain that usually presents three α-helices and a motif that enables PBX proteins to interact with DNA (Qian et al., 1989; Kissinger et al., 1990; Gehring et al., 1994). As part of the TALE family, PBX1 plays critical roles in embryogenesis, organogenesis, development, and differentiation (Wright et al., 1989). Our previous studies have reported that PBX1 facilitates hair follicle-derived mesenchymal stem cell DNA damage repair and attenuates senescence and programmed cell death (Jiang et al., 2019; Liu et al., 2019; Wang et al., 2020; Wang et al., 2021; Liu et al., 2022a; Wang et al., 2022a). Loss-of-function research on mice indicated that PBX1 is necessary for embryo growth and survival, since PBX1-knockout embryos reached embryonic lethality at about half-a-month post-pregnancy (Kim et al., 2002; Schnabel et al., 2003a). The mouse embryos lacking PBX1, which is also a key regulator of renal morphogenesis, were also shown to have mesenchymal dysfunction, which further leads to kidney developmental damage (Schnabel et al., 2003a). Moreover, it has been reported that PBX1 promotes tumor progression as an oncogene (Zhou et al., 2020; Lee et al., 2021; Liu et al., 2022b).
A large number of studies have been reported on human diseases caused by mutant PBX1 pathogenic genes such as asplenia (Arts et al., 2020), pulmonary hypoplasia (Alankarage et al., 2020; Arts et al., 2020), microsplenia (Arts et al., 2020), heart malformation or congenital heart disease (Le Tanno et al., 2017; Alankarage et al., 2020; Arts et al., 2020), bilateral diaphragmatic eventration (Arts et al., 2020), dysmorphic features (Le Tanno et al., 2017; Riedhammer et al., 2017; Alankarage et al., 2020; Arts et al., 2020), bone malformation (Riedhammer et al., 2017; Alankarage et al., 2020), dysmorphic features (Le Tanno et al., 2017; Riedhammer et al., 2017; Alankarage et al., 2020; Arts et al., 2020), sex reversal (Arts et al., 2020), cryptorchidism (Le Tanno et al., 2017; Riedhammer et al., 2017), neurological malformation (Le Tanno et al., 2017), global developmental delay (Riedhammer et al., 2017), growth retardation (Riedhammer et al., 2017; Alankarage et al., 2020), inborn defects of the kidney and urinary tract (Le Tanno et al., 2017; Riedhammer et al., 2017; Arts et al., 2020), poor prognosis (Magnani et al., 2011), and intellectual disability (Riedhammer et al., 2017; Alankarage et al., 2020). These prior studies demonstrate the importance of PBX1 in the development and the function of major tissues and organs. In this meta-analysis, we provide a comprehensive overview of the role of PBX1 in mammalian kidneys.
2 Results
2.1 PBX1 in renal carcinoma
The aberrant PBX1 expression is associated with poor prognoses (Magnani et al., 2015), tumorigeneses (Park et al., 2008), resistance to cancer therapy (Jung et al., 2016), and poor response to induction therapy (Veselska et al., 2019). Initial PBX1 is associated with cancers of the hematopoietic system (Shimabe et al., 2009), which are followed by solid tumors such as neuroblastoma (Veselska et al., 2019), renal carcinoma (Wei et al., 2018; Wang et al., 2022b), gastric cancers (He et al., 2017), and esophageal cancers (Zhu et al., 2017).
The “Oncomine” database shows that abnormal PBX1 overexpression exists in numerous cancer categories, including renal clear cell carcinomas (ccRCCs), accounting for 70% of renal tumors (Zhang et al., 2016; Hsieh et al., 2017; Wei et al., 2018). Furthermore, the expression of PBX1 was dynamically upregulated in four renal carcinoma cell lines (OS-RC-2, KAKi-2, 786-O, and 769-P) and the HK-2 proximal renal tubule cell line (Wei et al., 2018). There was significantly lower survival among patients with high PBX1 expression in the nucleus and cytoplasm of ccRCC cells than in those with low PBX1 expression. This may be related to the fact that PBX1 promotes the phosphorylation of STAT3Tyr705 to activate the JAK2/STAT3 signaling pathway in ccRCC. The decrease in STAT3Tyr705 phosphorylation after PBX1 knockout in ccRCC cells may also influence cancer cell growth and play an important role in renal tumor development (Wei et al., 2018).
It has been found that more than 80% of ccRCC tumors exhibit epigenetic changes or possess genetic changes in the von Hippel–Lindau (VHL) gene (Nickerson et al., 2008; Moore et al., 2011; Sato et al., 2013). Interestingly, in ccRCC patients with VHL mutations, PBX1 mRNA expression levels and PBX1 transcriptional scores were also associated with their clinical outcomes. Higher PBX1 transcription scores were associated with better total survival, whereas the decreased expression of PBX1 was associated with poor VHL mutation prognosis in ccRCC, which may be related to the molecular heterogeneity of the VHL-mutant ccRCC subgroup (Wang et al., 2022b). Another study by Margon et al. showed that HOX along with PBX could affect apoptosis of the renal cancer lines CaKi-2 and 769-P (Shears et al., 2008). The results of these studies indicate that PBX1 may be a novel prognostic factor for renal tumors and has potential applications in treating human renal tumors.
2.2 PBX1 in renal development
PBX1 encodes a TALE homeodomain transcription factor that regulates basic developmental processes in many different tissues, including the kidneys and spleen. It exists in structures derived from the intermediate mesoderm throughout all stages of urogenital system ontogeny and is expressed in the renal interstitium, nucleus medulla, and interstitial area and then into the posterior renal interstitium (Schnabel et al., 2001; Schnabel et al., 2003a; Schnabel et al., 2003b). Studies have found high expression of PBX1 in stromal cells and low expression of PBX1 in nephron progenitor cells (Schnabel et al., 2001; Schnabel et al., 2003a; Hurtado et al., 2015; Le Tanno et al., 2017), of which the latter is a direct target of Six2/Brg1 playing an important role in adjusting the fine balance between cell cycle progression and cell cycle maintenance (Li et al., 2021).
Since HOX genes are modified by PBX1 to perform certain functions and can play an important role in mammalian kidney development (Davis et al., 1995; Mann and Chan, 1996; Patterson et al., 2001; Wellik et al., 2002), it can be speculated that PBX1 and PBX regulatory protein families form nuclear complexes to improve the DNA-binding specificity of HOX proteins and regulate transcription during embryonic development (Slavotinek et al., 2017). In PBX1-deficient mice, the interference with this transcription factor is related to nephrogenesis (Schnabel et al., 2003a; Hurtado et al., 2015). However, that variation is restrained in PBX1 mutants with expanded areas of mesenchymal condensates, which contain a preponderance of cycling cells.
While PBX1-deficient kidneys lead to nephrogenesis, they have sustained proliferation of the mesenchyme without subsequent differentiation. In mild PBX1 mutant cases, ureteric buds have been shown to invade and branch, resulting in delayed renal vesicle formation, thicker cap condensate, and expanded c-ret expression (Schnabel et al., 2003a). Moreover, among most mutants, bilateral kidney formation is abnormal and manifests as delayed nephrogenesis and ureteral branch defects (Schnabel et al., 2003a).
The expression patterns described previously suggest that PBX1 may play an important role in fetal renal development. Using RT-qPCR, Le Tanno found that PBX1 was highly expressed in kidneys during the fetal period and was also relatively highly expressed in the brain during embryonic development. However, its expression was downregulated in adult kidneys (Le Tanno et al., 2017). The GUDMAP database displays that PBX1 is just highly expressed in not only renal tumor cells (for example, A-498, ACHN cell lines, and so on) but also in renal epithelial cells (for example, HK-2, RPTEC/TERT1 cell lines and so on).
PBX1 is crucial for interstitial–epithelial signal transduction, an important regulator of interstitial function in renal morphogenesis (Le Tanno et al., 2017), and critical for renal morphogenesis and development. For example, patients with pathogenic PBX1 mutations/microdeletions exhibited multifarious alloplasia (Slavotinek et al., 2017), and PBX1-deficient mice had delayed nephrogenesis and ureteral branching defects (Schnabel et al., 2003a). Congenital anomalies of the kidney and urinary tract (CAKUT) are diseases with various phenotypes presenting with congenital anatomical abnormalities of the urinary system. These include abnormal development of the kidney, ureter, bladder, and posterior urethra and constitute the main cause of chronic kidney disease in children caused by genovariation (Capone et al., 2017). PBX1 has been identified as a monogenic cause of CAKUT in mammals, with Le Tanno via microarray analysis previously reporting pathogenic variation in microdeletion-related genes as a cause of renal agenesis, with PBX1 as the smallest common region (Le Tanno et al., 2017). So far, more than 32 different pathogenic PBX1 variants have been reported. Renal agenesis, hyperechogenicity, pelvicalyceal dilation, bilateral nephroureters, ectopic kidneys, horseshoe-shaped kidneys, bilateral vesicoureteral reflux, and small urethral valves are common PBX1 mutant renal phenotypes, though renal agenesis is rarer. In the study by Petzold et al. (2022), chronic kidney disease stage 3 was observed at the time of the onset except in two adult patients with bilateral renal hypoplasia. Most patients were younger than 5 years of age at the time of their first diagnosis, and their renal functions declined variably. PBX1 mosaicism presents with a mild course and sporadicalness in CAKUT cases.
2.3 PBX1 in kidney blood vessels
PBX1 plays a pivotal role in promoting self-renewal and coordinating the extent of proliferation with the terminal differentiation of progenitor cells (Selleri et al., 2001; Ficara et al., 2008). Selleri et al. (2001) speculated that the loss of its function would lead to abnormal development of the cardiovascular system, and PBX1-null embryos in mice have displayed abnormal great artery morphogenesis due to failed establishment of initial complement of the branchial arch arteries (Chang et al., 2008; Stankunas et al., 2008). Hurtado et al. (2015) showed that PBX1 in renal vascular mural progenitor cells directly represses PDGFRB, which is a master initiator of vascular mural cell–blood vessel association in mice. Premature differentiation of vascular cells is associated with non-productive angiogenesis, abnormal renal artery branching, significant disturbance of the renal artery tree structure, and renal dysfunction. Moreover, ablation of PBX1 considerably remedies vascular patterning defects.
As one of the most unique transcription factors, PBX1 was found to have enriched expression or activity in glomerular capillaries, and its induction coincided with the ontology of the glomerulus (Barry et al., 2019). PBX1 may be recruited by renal epithelial cells to prune the gene expression that dominates vascular zonation from the embryonic period to the adult stage and appropriately adjust the specialized functions of the glomeruli (Barry et al., 2019).
3 Conclusion
The role of PBX1 is complex, with diverse contributions. As transcription factors, PBX1 and its partners together influence the occurrence and development of various diseases. As mentioned previously, PBX1 functions in mammalian kidneys include promoting tumor progression, regulating basic developmental processes, and adjusting glomeruli vascularization. Most of the time, PBX1 as an oncogene promotes the proliferation of tumor cells, induces angiogenesis, and participates in the occurrence and development of tumors. Mutation and disorder of PBX1 will cause serious and pleiotropic consequences such as CAKUT (Figure 1). Therefore, further research should help us understand the specific involvement of PBX1 in each type of kidney disease and to consider the renal pathophysiological changes caused by an altered PBX1 expression or pathogenic mutations. The therapeutic effects of some small-molecular drugs targeting specific diseases have been proved effective, but the relevant research on PBX1 as a molecular drug still need to be carried out. Importantly, years of research on this protein have set the foundations for currently expanding biotech and industrial activity devoted to turning such knowledge into treatments for patients.
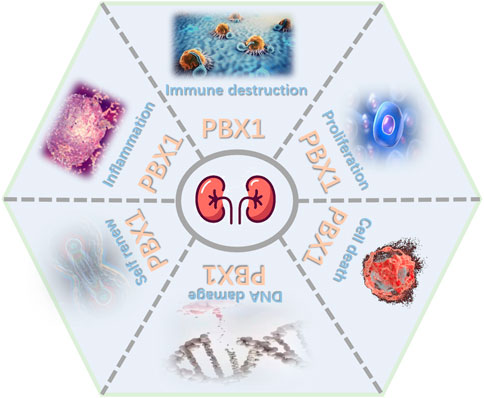
FIGURE 1. Major roles of PBX1. PBX1 contributes to kidney development, tumor progression, and blood vessels by interfering in proliferation, cell death, self-renewal, DNA damage, inflammation, and immune destruction (figure was modified from Servier Medical Art (http://smart.servier.com/).
Author contributions
FZ conceived and wrote the paper. ML, YS, and JL revised the manuscript. All authors have read and approved the final version of the manuscript.
Funding
This work was supported by the China Natural National Science Foundation (82273673).
Acknowledgments
The authors would like to thank Editage (www.editage.cn) for English language editing.
Conflict of interest
The authors declare that the research was conducted in the absence of any commercial or financial relationships that could be construed as a potential conflict of interest.
Publisher’s note
All claims expressed in this article are solely those of the authors and do not necessarily represent those of their affiliated organizations, or those of the publisher, the editors, and the reviewers. Any product that may be evaluated in this article, or claim that may be made by its manufacturer, is not guaranteed or endorsed by the publisher.
References
Alankarage, D., Szot, J. O., Pachter, N., Slavotinek, A., Selleri, L., Shieh, J. T., et al. (2020). Functional characterization of a novel PBX1 de novo missense variant identified in a patient with syndromic congenital heart disease. Hum. Mol. Genet. 29, 1068–1082. doi:10.1093/hmg/ddz231
Arts, P., Garland, J., Byrne, A. B., Hardy, T. S. E., Babic, M., Feng, J., et al. (2020). Paternal mosaicism for a novel PBX1 mutation associated with recurrent perinatal death: Phenotypic expansion of the PBX1-related syndrome. Am. J. Med. Genet. A 182, 1273–1277. doi:10.1002/ajmg.a.61541
Barry, D. M., McMillan, E. A., Kunar, B., Lis, R., Zhang, T., Lu, T., et al. (2019). Molecular determinants of nephron vascular specialization in the kidney. Nat. Commun. 10, 5705. doi:10.1038/s41467-019-12872-5
Capone, V. P., Morello, W., Taroni, F., and Montini, G. (2017). Genetics of congenital anomalies of the kidney and urinary tract: The current state of play. Int. J. Mol. Sci. 18, 796. doi:10.3390/ijms18040796
Chang, C. P., Stankunas, K., Shang, C., Kao, S. C., Twu, K. Y., and Cleary, M. L. (2008). Pbx1 functions in distinct regulatory networks to pattern the great arteries and cardiac outflow tract. Development 135, 3577–3586. doi:10.1242/dev.022350
Davis, A. P., Witte, D. P., Hsieh-Li, H. M., Potter, S. S., and Capecchi, M. R. (1995). Absence of radius and ulna in mice lacking hoxa-11 and hoxd-11. Nature 375, 791–795. doi:10.1038/375791a0
Ficara, F., Murphy, M. J., Lin, M., and Cleary, M. L. (2008). Pbx1 regulates self-renewal of long-term hematopoietic stem cells by maintaining their quiescence. Cell Stem Cell 2, 484–496. doi:10.1016/j.stem.2008.03.004
Gehring, W. J., Qian, Y. Q., Billeter, M., Furukubo-Tokunaga, K., Schier, A. F., Resendez-Perez, D., et al. (1994). Homeodomain-DNA recognition. Cell 78, 211–223. doi:10.1016/0092-8674(94)90292-5
He, C., Wang, Z., Zhang, L., Yang, L., Li, J., Chen, X., et al. (2017). A hydrophobic residue in the TALE homeodomain of PBX1 promotes epithelial-to-mesenchymal transition of gastric carcinoma. Oncotarget 8, 46818–46833. doi:10.18632/oncotarget.17473
Hsieh, J. J., Purdue, M. P., Signoretti, S., Swanton, C., Albiges, L., Schmidinger, M., et al. (2017). Renal cell carcinoma. Nat. Rev. Dis. Prim. 3, 17009. doi:10.1038/nrdp.2017.9
Hurtado, R., Zewdu, R., Mtui, J., Liang, C., Aho, R., Kurylo, C., et al. (2015). Pbx1-dependent control of VMC differentiation kinetics underlies gross renal vascular patterning. Development 142, 2653–2664. doi:10.1242/dev.124776
Jiang, Y., Liu, F., Zou, F., Zhang, Y., Wang, B., Zhang, Y., et al. (2019). PBX homeobox 1 enhances hair follicle mesenchymal stem cell proliferation and reprogramming through activation of the AKT/glycogen synthase kinase signaling pathway and suppression of apoptosis. Stem Cell Res. Ther. 10, 268. doi:10.1186/s13287-019-1382-y
Jung, J. G., Shih, I. M., Park, J. T., Gerry, E., Kim, T. H., Ayhan, A., et al. (2016). Ovarian cancer chemoresistance relies on the stem cell reprogramming factor PBX1. Cancer Res. 76, 6351–6361. doi:10.1158/0008-5472.CAN-16-0980
Kamps, M. P., Look, A. T., and Baltimore, D. (1991). The human t(1;19) translocation in pre-B ALL produces multiple nuclear E2A-Pbx1 fusion proteins with differing transforming potentials. Genes Dev. 5, 358–368. doi:10.1101/gad.5.3.358
Kim, S. K., Selleri, L., Lee, J. S., Zhang, A. Y., Gu, X., Jacobs, Y., et al. (2002). Pbx1 inactivation disrupts pancreas development and in Ipf1-deficient mice promotes diabetes mellitus. Nat. Genet. 30, 430–435. doi:10.1038/ng860
Kissinger, C. R., Liu, B. S., Martin-Blanco, E., Kornberg, T. B., and Pabo, C. O. (1990). Crystal structure of an engrailed homeodomain-DNA complex at 2.8 A resolution: A framework for understanding homeodomain-DNA interactions. Cell 63, 579–590. doi:10.1016/0092-8674(90)90453-l
Le Tanno, P., Breton, J., Bidart, M., Satre, V., Harbuz, R., Ray, P. F., et al. (2017). PBX1 haploinsufficiency leads to syndromic congenital anomalies of the kidney and urinary tract (CAKUT) in humans. J. Med. Genet. 54, 502–510. doi:10.1136/jmedgenet-2016-104435
Lee, Y. L., Ito, K., Pi, W. C., Lin, I. H., Chu, C. S., Malik, S., et al. (2021). Mediator subunit MED1 is required for E2A-PBX1-mediated oncogenic transcription and leukemic cell growth. Proc. Natl. Acad. Sci. U. S. A. 118, e1922864118. doi:10.1073/pnas.1922864118
Li, J., Xu, J., Jiang, H., Zhang, T., Ramakrishnan, A., Shen, L., et al. (2021). Chromatin remodelers interact with Eya1 and Six2 to target enhancers to control nephron progenitor cell maintenance. J. Am. Soc. Nephrol. 32, 2815–2833. doi:10.1681/ASN.2021040525
Liu, F., Shi, J., Zhang, Y., Lian, A., Han, X., Zuo, K., et al. (2019). NANOG attenuates hair follicle-derived mesenchymal stem cell senescence by upregulating PBX1 and activating AKT signaling. Oxid. Med. Cell Longev. 2019, 4286213. doi:10.1155/2019/4286213
Liu, M., Liu, X., Wang, Y., Sui, Y., Liu, F., Liu, Z., et al. (2022a). Intrinsic ROS drive hair follicle cycle progression by modulating DNA damage and repair and subsequently hair follicle apoptosis and macrophage polarization. Oxid. Med. Cell Longev. 2022, 8279269. doi:10.1155/2022/8279269
Liu, M., Zhai, E., Chen, J., Qian, Y., Zhao, R., Ma, Y., et al. (2022b). m(6 A-mediated regulation of PBX1-GCH1 axis promotes gastric cancer proliferation and metastasis by elevating tetrahydrobiopterin levels. Cancer Commun. (Lond) 42, 327–344. doi:10.1002/cac2.12281
Magnani, L., Ballantyne, E. B., Zhang, X., and Lupien, M. (2011). PBX1 genomic pioneer function drives ERα signaling underlying progression in breast cancer. PLoS Genet. 7, e1002368. doi:10.1371/journal.pgen.1002368
Magnani, L., Patten, D. K., Nguyen, V. T., Hong, S. P., Steel, J. H., Patel, N., et al. (2015). The pioneer factor PBX1 is a novel driver of metastatic progression in ERα-positive breast cancer. Oncotarget 6, 21878–21891. doi:10.18632/oncotarget.4243
Mann, R. S., and Chan, S. K. (1996). Extra specificity from extradenticle: The partnership between HOX and PBX/EXD homeodomain proteins. Trends Genet. 12, 258–262. doi:10.1016/0168-9525(96)10026-3
Monica, K., Galili, N., Nourse, J., Saltman, D., and Cleary, M. L. (1991). PBX2 and PBX3, new homeobox genes with extensive homology to the human proto-oncogene PBX1. Mol. Cell Biol. 11, 6149–6157. doi:10.1128/mcb.11.12.6149
Moore, L. E., Nickerson, M. L., Brennan, P., Toro, J. R., Jaeger, E., Rinsky, J., et al. (2011). Von hippel-lindau (VHL) inactivation in sporadic clear cell renal cancer: Associations with germline VHL polymorphisms and etiologic risk factors. PLoS Genet. 7, e1002312. doi:10.1371/journal.pgen.1002312
Nickerson, M. L., Jaeger, E., Shi, Y., Durocher, J. A., Mahurkar, S., Zaridze, D., et al. (2008). Improved identification of von Hippel-Lindau gene alterations in clear cell renal tumors. Clin. Cancer Res. 14, 4726–4734. doi:10.1158/1078-0432.CCR-07-4921
Park, J. T., Shih Ie, M., and Wang, T. L. (2008). Identification of Pbx1, a potential oncogene, as a Notch3 target gene in ovarian cancer. Cancer Res. 68, 8852–8860. doi:10.1158/0008-5472.CAN-08-0517
Patterson, L. T., Pembaur, M., and Potter, S. S. (2001). Hoxa11 and Hoxd11 regulate branching morphogenesis of the ureteric bud in the developing kidney. Development 128, 2153–2161. doi:10.1242/dev.128.11.2153
Petzold, F., Jin, W., Hantmann, E., Korbach, K., Schonauer, R., and Halbritter, J. (2022). Novel somatic PBX1 mosaicism likely masking syndromic CAKUT in an adult with bilateral kidney hypoplasia. Clin. Kidney J. 15, 1333–1339. doi:10.1093/ckj/sfac092
Qian, Y. Q., Billeter, M., Otting, G., Muller, M., Gehring, W. J., and Wuthrich, K. (1989). The structure of the antennapedia homeodomain determined by NMR spectroscopy in solution: Comparison with prokaryotic repressors. Cell 59, 573–580. doi:10.1016/0092-8674(89)90040-8
Riedhammer, K. M., Siegel, C., Alhaddad, B., Montoya, C., Kovacs-Nagy, R., Wagner, M., et al. (2017). Identification of a novel heterozygous de novo 7-bp frameshift deletion in PBX1 by whole-exome sequencing causing a multi-organ syndrome including bilateral dysplastic kidneys and hypoplastic clavicles. Front. Pediatr. 5, 251. doi:10.3389/fped.2017.00251
Sato, Y., Yoshizato, T., Shiraishi, Y., Maekawa, S., Okuno, Y., Kamura, T., et al. (2013). Integrated molecular analysis of clear-cell renal cell carcinoma. Nat. Genet. 45, 860–867. doi:10.1038/ng.2699
Schnabel, C. A., Godin, R. E., and Cleary, M. L. (2003). Pbx1 regulates nephrogenesis and ureteric branching in the developing kidney. Dev. Biol. 254, 262–276. doi:10.1016/s0012-1606(02)00038-6
Schnabel, C. A., Selleri, L., and Cleary, M. L. (2003). Pbx1 is essential for adrenal development and urogenital differentiation. Genesis 37, 123–130. doi:10.1002/gene.10235
Schnabel, C. A., Selleri, L., Jacobs, Y., Warnke, R., and Cleary, M. L. (2001). Expression of Pbx1b during mammalian organogenesis. Mech. Dev. 100, 131–135. doi:10.1016/s0925-4773(00)00516-5
Selleri, L., Depew, M. J., Jacobs, Y., Chanda, S. K., Tsang, K. Y., Cheah, K. S., et al. (2001). Requirement for Pbx1 in skeletal patterning and programming chondrocyte proliferation and differentiation. Development 128, 3543–3557. doi:10.1242/dev.128.18.3543
Shears, L., Plowright, L., Harrington, K., Pandha, H. S., and Morgan, R. (2008). Disrupting the interaction between HOX and PBX causes necrotic and apoptotic cell death in the renal cancer lines CaKi-2 and 769-P. J. Urol. 180, 2196–2201. doi:10.1016/j.juro.2008.07.018
Shimabe, M., Goyama, S., Watanabe-Okochi, N., Yoshimi, A., Ichikawa, M., Imai, Y., et al. (2009). Pbx1 is a downstream target of Evi-1 in hematopoietic stem/progenitors and leukemic cells. Oncogene 28, 4364–4374. doi:10.1038/onc.2009.288
Slavotinek, A., Risolino, M., Losa, M., Cho, M. T., Monaghan, K. G., Schneidman-Duhovny, D., et al. (2017). De novo, deleterious sequence variants that alter the transcriptional activity of the homeoprotein PBX1 are associated with intellectual disability and pleiotropic developmental defects. Hum. Mol. Genet. 26, 4849–4860. doi:10.1093/hmg/ddx363
Stankunas, K., Shang, C., Twu, K. Y., Kao, S. C., Jenkins, N. A., Copeland, N. G., et al. (2008). Pbx/Meis deficiencies demonstrate multigenetic origins of congenital heart disease. Circ. Res. 103, 702–709. doi:10.1161/CIRCRESAHA.108.175489
Veselska, R., Jezova, M., Kyr, M., Mazanek, P., Chlapek, P., Dobrotkova, V., et al. (2019). Comparative analysis of putative prognostic and predictive markers in neuroblastomas: High expression of PBX1 is associated with a poor response to induction therapy. Front. Oncol. 9, 1221. doi:10.3389/fonc.2019.01221
Wagner, K., Mincheva, A., Korn, B., Lichter, P., and Popperl, H. (2001). Pbx4, a new Pbx family member on mouse chromosome 8, is expressed during spermatogenesis. Mech. Dev. 103, 127–131. doi:10.1016/s0925-4773(01)00349-5
Wang, B., Liu, F., Liu, Z., Han, X., Lian, A., Zhang, Y., et al. (2020). Internalization of the TAT-PBX1 fusion protein significantly enhances the proliferation of human hair follicle-derived mesenchymal stem cells and delays their senescence. Biotechnol. Lett. 42, 1877–1885. doi:10.1007/s10529-020-02909-x
Wang, H., Wang, X., Xu, L., and Zhang, J. (2022). PBX1, EMCN and ERG are associated with the sub-clusters and the prognosis of VHL mutant clear cell renal cell carcinoma. Sci. Rep. 12, 8955. doi:10.1038/s41598-022-13148-7
Wang, Y., Sui, Y., Lian, A., Han, X., Liu, F., Zuo, K., et al. (2021). PBX1 attenuates hair follicle-derived mesenchymal stem cell senescence and apoptosis by alleviating reactive oxygen species-mediated DNA damage instead of enhancing DNA damage repair. Front. Cell Dev. Biol. 9, 739868. doi:10.3389/fcell.2021.739868
Wang, Y., Sui, Y., Niu, Y., Liu, D., Xu, Q., Liu, F., et al. (2022). PBX1-SIRT1 positive feedback loop attenuates ROS-mediated HF-msc senescence and apoptosis. Stem Cell Rev. Rep. 19, 443–454. doi:10.1007/s12015-022-10425-w
Wei, X., Yu, L., and Li, Y. (2018). PBX1 promotes the cell proliferation via JAK2/STAT3 signaling in clear cell renal carcinoma. Biochem. Biophys. Res. Commun. 500, 650–657. doi:10.1016/j.bbrc.2018.04.127
Wellik, D. M., Hawkes, P. J., and Capecchi, M. R. (2002). Hox11 paralogous genes are essential for metanephric kidney induction. Genes Dev. 16, 1423–1432. doi:10.1101/gad.993302
Wright, C. V., Cho, K. W., Oliver, G., and De Robertis, E. M. (1989). Vertebrate homeodomain proteins: Families of region-specific transcription factors. Trends Biochem. Sci. 14, 52–56. doi:10.1016/0968-0004(89)90043-1
Zhou, Y., Fu, B., Xu, X., Zhang, J., Tong, X., Wang, Y., et al. (2020). PBX1 expression in uterine natural killer cells drives fetal growth. Sci. Transl. Med. 12, eaax1798. doi:10.1126/scitranslmed.aax1798
Zhang, Z., Duan, Q., Zhao, H., Liu, T., Wu, H., Shen, Q., et al. (2016). Gemcitabine treatment promotes pancreatic cancer stemness through the Nox/ROS/NF-κB/STAT3 signaling cascade. Cancer Lett. 382, 53–63. doi:10.1016/j.canlet.2016.08.023
Keywords: PBX1, kidney, renal carcinoma, development, blood vessels
Citation: Zou F, Liu M, Sui Y and Liu J (2023) Comprehensive overview of the role of PBX1 in mammalian kidneys. Front. Mol. Biosci. 10:1106370. doi: 10.3389/fmolb.2023.1106370
Received: 23 November 2022; Accepted: 01 March 2023;
Published: 17 March 2023.
Edited by:
Yifan Zhang, Shanghai Jiao Tong University, ChinaReviewed by:
Rebecca Ann Wingert, University of Notre Dame, United StatesCopyright © 2023 Zou, Liu, Sui and Liu. This is an open-access article distributed under the terms of the Creative Commons Attribution License (CC BY). The use, distribution or reproduction in other forums is permitted, provided the original author(s) and the copyright owner(s) are credited and that the original publication in this journal is cited, in accordance with accepted academic practice. No use, distribution or reproduction is permitted which does not comply with these terms.
*Correspondence: Jinyu Liu, anlfbGl1QGpsdS5lZHUuY24=