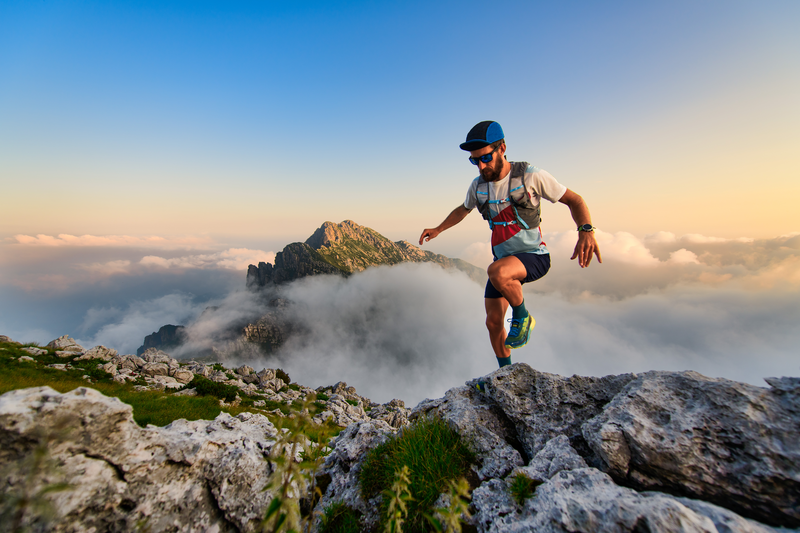
94% of researchers rate our articles as excellent or good
Learn more about the work of our research integrity team to safeguard the quality of each article we publish.
Find out more
REVIEW article
Front. Mol. Biosci. , 29 September 2022
Sec. Cellular Biochemistry
Volume 9 - 2022 | https://doi.org/10.3389/fmolb.2022.955193
This article is part of the Research Topic The Expanding Functional Network of Glutathione Transferases View all 6 articles
Oxidative stress is one of the main pathophysiological mechanisms for chronic respiratory disease. Glutathione S-transferase (GST) genes play important roles in antioxidant defences and may influence respiratory health. Although there is not consistent evidence that the three commonly studied genes of GSTM1, GSTT1 and GSTP1 are associated directly with respiratory outcomes, they seem to be related to disease susceptibility if exposure interactions are taken into account. Exposure to household air pollution may be particularly important in increasing lung oxidative stress. This review summarizes the relationships between GST genes, household air pollution and asthma and impaired lung function. Our findings support a role for GST polymorphisms in susceptibility to asthma and impaired lung function via oxidative stress pathways. Future research should additionally consider the role of gene-gene interactions, multiple environmental exposures, and gender in these complex associations, that are involved in maintaining antioxidant defences and lung health.
Over the past 20 years, the prevalence of asthma has been increasing significantly worldwide, particularly for countries transitioning to a developed economic status (Global Asthma Network, 2014). Asthma is not a single disease but rather an umbrella term for multiple phenotypes with similar clinical features including airway inflammation and reversible airway obstruction. In the longer-term asthma may lead to reduced lung function. Many people with chronic and/or severe asthma experience an accelerated and progressive loss of lung function over time and their lung function progresses from reversible to irreversible airway obstruction (Pascual and Peters, 2005).
Although the aetiology of asthma is unknown, many authors have found evidence that asthma is associated with increased oxidative stress in the airways; evidenced by the presence of increased oxidative biomarkers (Milos et al., 2017). Many air pollutants generate endogenous reactive oxygen species (ROS) that cause oxidant injury in the lungs. Detoxification is a primary method of defence from harmful environmental exposures. Glutathione S-Transferases (GSTs) play a major protective antioxidant role in the lungs and subsequently regulate inflammatory responses (Piacentini et al., 2013). GSTs catalyze the conjugation of antioxidants - glutathione (GSH) with electrophilic compounds that generate free radicals, thus leading to detoxifying effects. Polymorphisms of genes encoding GST enzymes are therefore crucial to regulating the detoxification of a wide range of endogenous compounds (Piacentini et al., 2013).
Findings on the relationship between household air pollution exposures and respiratory health outcomes have been inconsistent. A reason for the inconsistent findings between common oxidative household exposures and respiratory health could be the failure to account for potential gene-environment interactions. Over the last decade, there has been increasing evidence to suggest that gene-environment interactions are likely to be involved in the development, severity, and age-of-onset of many complex diseases including asthma (Minelli et al., 2011; Minelli and Gögele, 2011). It is possible that individuals with risk variants of GST genes may be more susceptible to environmental risk factors and more likely to develop asthma, compared to those without risk genes, even when exposed to the same environmental factors. However, there are few studies on GST gene-environment interactions and their associations with asthma and lung function and their findings are inconsistent. This review will discuss the three most researched classes of GST gene polymorphisms including pi 1 (GSTP1), mu 1 (GSTM1) and theta 1 (GSTT1), and summarize current knowledge on of complex mechanisms that may be involved in the interactions between household air pollution exposure and GST gene polymorphisms and potentially the aetiology of asthma in high risk populations who carry these risk alleles.
Eight classes of the most studied GST gene superfamily code for similarly named glutathione transferases: alpha, kappa, mu, pi, sigma, theta, omega and zeta have been described (Table 1). Each of these classes are found in many different tissues and have multiple isoforms (Buratti et al., 2021). (Alexander et al., 2013). Of these, the three most extensively studied are pi 1, mu 1 and theta 1. These are also the classes that have been found to be expressed in the lung. The GSTP1 gene is located on a ‘hot spot’ site for asthma-related genes - chromosome 11q13. The GSTP1 enzyme is the most common form of GST expressed in lung epithelium, responsible for over 90% of lung GST enzyme activity (Minelli et al., 2011). The GSTM1 gene spans a 97-kb region on chromosome 1p13 and is predominately expressed in the liver (Murdzoska et al., 2010). The GSTT1 gene is located on chromosome 22q11 and predominately expressed in erythrocytes (Murdzoska et al., 2010). However, both GSTM1 and GSTT1 are also expressed in lung tissue, specifically in the bronchial wall (Breton et al., 2009). Polymorphisms of all these GST genes may influence antioxidant function in the lungs and other organs, and this can confer genetic susceptibility to oxidative stress and disease like asthma. Given polymorphisms of GST genes are common in the general population, there is public health importance of studying GST susceptibility profiles (Table 1). A common polymorphism in the GSTP1 gene is Ile105Val, with the frequency of the Val105 allele lower in Asian and higher in European and African populations. The Val allele has been associated with higher risk of respiratory issues (Minelli et al., 2010). The frequency of absence polymorphisms for GSTM1/T1 also varies across ethnic groups. (From 15 to 62% in specific populations) The null genotype of GSTM1/T1 results in loss of enzyme activity and thus reduction of antioxidant capacity (Minelli et al., 2010). The variation of frequency of GST polymorphisms across ethnicities suggests a potential role of ethnicity in explaining the heterogeneity of associations found in the current literature.
Oxidative stress reflects the imbalance between the overproduction of oxidants in terms of ROS and reactive nitrogen species (RNS) and underproduction of antioxidant defence mechanisms in the body (Milos et al., 2017). There are many exogenous sources of ROS, such as cigarette smoke and air pollutants. These ROS play an important role in the pathogenesis of inflammatory conditions. After exposure to environmental triggers, normal physiological antioxidant defence mechanisms are usually able to eliminate ROS and repair damage. However, an imbalance between oxidants and antioxidants can have significant functional and structural consequences associated with excessive airway inflammation and without sufficient repair may lead to accumulated oxidative changes until this process reaches the “threshold” for lung damage and manifests as asthma (Milos et al., 2017). This mechanism is a long-term continuous and dynamic process and may explain the development and persistence of asthma (Milos et al., 2017).
The lungs are equipped with a range of antioxidant enzymes for managing the constant exposure to a wide range of oxidants. These enzymes include superoxide dismutase (SOD) and glutathione peroxidase Decreased activity or dysfunction of normal antioxidant enzyme defences can be identified in asthmatic airways. GSH is an important antioxidant substance in humans which can be found in the alveolar epithelial lining fluid and it protects against airway peroxidation (Fernando, 2013). People with mild asthma have been shown to have a high glutathione level, reflecting an adaptive response to increased ROS, compared to healthy people. However, in severe asthma, GSH levels have been shown to be significantly lower when compared to people with mild to moderate asthma or healthy controls. This may due to key enzymes involved in glutathione synthesis being highly dysfunctional (Fernando, 2013). The reduced GSH level may impair the ability to detoxify ROS.
GSTs can facilitate detoxification through both GSH dependant and GSH independent pathways. Firstly, GSTs can work in conjunction with GSH. During an antioxidative response, GSTs act as functional enzymes that catalyze the conjugation of GSH to several electrophilic compounds, these compounds are generally less reactive and more soluble. GSTs assist in the transport and elimination of conjugated oxidants by moving these compounds from cells via membrane-based GSH conjugate pumps (Martos-Maldonado et al., 2015). Secondly, GSTs also perform a large array of non-enzymatic functions, including inactivating compounds in the stress-response signalling pathways of the detoxification process (Hayes and Strange, 2000; Martos-Maldonado et al., 2015). GSTs can bind numerous ligands for cell surface receptors, thus they may block signalling to inflammatory compounds like prostaglandins (Hayes and Strange, 2000). Additionally, GST genes regulate a complex network of antioxidant defences involving many other antioxidant compounds such as arginine, citrulline, taurine, creatine, selenium, zinc and vitamins A, C, D and E, and antioxidant enzymes (Minelli and Gögele, 2011). Thus, genetic variations in GSTs are of interest in the study of disease susceptibility, as they may alter the influence of many household air exposures on respiratory health through oxidative stress and inflammatory pathways.
There is a strong biological rationale that GST polymorphisms may influence risk of asthma, and airway hyperresponsiveness via oxidative stress pathways (Lenney and Fryer, 2007), but the current evidence does not fully support this hypothesis (Table 2). No cohort study has demonstrated a main effect of GST genes on asthma outcomes, including two cohorts with large sample sizes (>2000 participants) and long-term follow up (Gilliland et al., 2002; Imboden et al., 2007). However, both these cohorts identified slower lung function growth/quicker lung function decline in populations with susceptible genotypes (Gilliland et al., 2002; Imboden et al., 2007). This discrepancy in findings may be partly due to the outcome of lung function, as a continuous variable, having greater power to detect associations compared to asthma outcomes (binary variable).
The most studied GST gene in relation to lung function and asthma outcomes is GSTP1, but the evidence for GSTP1 risk alleles is inconsistent. As GSTP1 with Ile/Ile genotypes has the highest enzyme activity, carriers of GSTP1 with any Val alleles would be expected to have a higher risk of asthma symptoms and poorer lung function. There is some support for this hypothesis. A case control study in Turkey found that subjects with GSTP1 homozygous Val/Val genotypes had a 3.55 fold increased risk of atopic asthma in adulthood (95%CI 1.10, 12.56) compared to those with homozygous Ile/Ile genotypes (Tamer et al., 2004). A United States cohort study over a 4-year period found that children with GSTP1 Val/Val genotypes had slower lung function growth for FEV1 (-0.34%, 95% CI -0.68, 0) and FVC (-0.35%, 95% CI -0.50, -0.04) compared to those with any Ile genotypes (Gilliland et al., 2002). However, some studies have found the opposite relationship or null associations. A United Kingdom study conducted on adults reported that having the GSTP1 homozygous Val variant was associated with a six-fold lower risk of asthma compared with homozygous Ile carriers (Anthony A et al., 2000). Moreover, there are several studies that have not found any association between the GSTP1 genotype and respiratory health (Chung, 2002; Safronova et al., 2003; Nickel et al., 2005; Oh et al., 2005; Renate et al., 2005; Ercan et al., 2006).
People with GSTM1/GSTT1 null genotypes have complete loss of enzyme activity resulting in a reduction of antioxidant capacity (Minelli et al., 2011). Carriers of GSTM1 and GSTT1 null genotypes may be more susceptible to asthma. Several case control studies have found a higher prevalence of both GSTM1 null genotypes and GSTT1 null genotypes in asthma cases, compared with controls (Vavilin et al., 2002; Mostafa et al., 2004; Zhang et al., 2004; Hanene et al., 2007; Karam et al., 2012; Iorio et al., 2015). However, these were all small studies with sample sizes of less than 300 participants. In contrast, two large cohort studies, the Swiss Study on Air Pollution and Lung Diseases in Adults (SAPALDIA) (Imboden et al., 2008) (n = 4,422), and the Avon Longitudinal Study of Parents and Children (ALSPAC) (Minelli et al., 2010) in the United Kingdom (n = 7,262), did not find evidence of associations between GSTM1 or GSTT1 genotypes and asthma.
Two systematic reviews have been conducted on the associations between GSTM1/T1/P1 genotypes and asthma (Minelli et al., 2010; Piacentini et al., 2013). One found weak evidence for an association between GSTT1 null genotypes and increased risk of asthma (pooled OR 1.33, 95%CI 1.10,1.60), however, this study identified high heterogeneity in their analyses (Piacentini et al., 2013). There was little evidence, from these 2 reviews, to support a substantial independent role of GST genes in the development of asthma (Minelli et al., 2010; Piacentini et al., 2013). One hypothesis that may explain these findings is that GSTM1, GSTT1 and GSTP1 have overlapping substrate specificities, so that a deficiency in one isoform may be compensated for by another. One of the meta-analyses from these reviews included 14 additional studies to assess whether the combination of multiple GST risk genotypes may have an additive effect on respiratory health outcomes. This review found a higher risk of asthma for people with both GSTM1 null and GSTT1 null genotypes (OR 2.15, 95%CI 1.21, 3.71) compared to those with two non-null genotypes, but no evidence of an association in those with only one null GSTM1 or GSTT1 gene (Minelli et al., 2010). Other than GST genes, there are many antioxidants that may interact to impact the response to oxidative stress. One study investigated a dose-response relationship between GSTM1, GSTP1 genotypes, another respiratory inflammatory biomarker, Clara Cell secretory protein-16, (CC16) and asthma risk. Individuals with three putative high-risk genotypes had the highest risk of asthma (OR: 22.5, 95% CI 4.08, 123.48), followed by moderately increased risk for two putative high-risk genotypes (OR: 5.50, 95% CI 1.96, 15.41) and little evidence for one putative high-risk genotype (OR: 2.05, 95% CI 0.77, 5.41) (Mostafa et al., 2004).
Studies of GST genes greatly improve the understanding of the pathophysiological mechanisms that may lead to asthma and lung function deficits. Current studies have unexpectedly shown relatively small associations between GST genes alone and these medical conditions. Apart from this being because of capacity through several antioxidant pathways as indicated above, this may also be due to individual differences in exposure to environmental factors. Many household air exposures like Environmental Tobacco Smoke (ETS) have been shown to induce oxidative stress, however, individuals differ in their ability to deal with an oxidant burden, and these differences may be partially due to GST genetic variation. GST genes, regulating antioxidant defences, are likely to modulate the response to pollutants. Thus, the effects of environmental exposures on respiratory health may be found only for individuals with a high-risk genetic profile who are also exposed to a high-risk environment. This hypothesis has been investigated in several studies as outlined below.
Evidence for an interactive effect of GSTM1 on the association between household exposures and adverse respiratory outcomes is summarized in Table 3. The earliest studies that investigated GSTM1 interactions in United States in 2002, suggested in-utero exposure to maternal smoking was associated with an increased risk of childhood asthma and wheezing occurrence at school age, but only for children with GSTM1 null genotypes (Interaction p values <0.05). In this study, neither the GSTM1 genotype, nor in-utero exposure to smoking were individually associated with any asthma or wheezing outcomes (Frank D et al., 2002). Subsequently, similar studies have also identified significant interactions between household air pollution, GSTM1 null genotypes and asthma risk (Carr et al., 2004; Wu et al., 2014). In contrast, the evidence from some studies has not supported this GSTM1 interaction (Lee et al., 2007; Wang et al., 2011; Hersoug et al., 2012; Dai et al., 2019; Dai et al., 2021). The reasons are not clear, but the interaction may be exposure specific, with studies investigating tobacco smoke exposure more likely to find adverse associations when compared to other household air pollution exposures (Dai et al., 2018). Studies that investigated incense burning (Wang et al., 2011) and wood stove/candles during wintertime (Hersoug et al., 2012) found no evidence of interactions.
TABLE 3. Influence of GSTM1 polymorphisms on the relationship between household environmental exposures and asthma and lung function.
Several studies have investigated interactions between GSTM1 polymorphisms and environmental exposures on the risk of lung function deficits. Overall, the evidence supports a relationship between GSTM1 null genotypes and increased risk of lung function deficits in individuals exposed to different kinds of household air pollution. Three studies found that carriers of GSTM1 null variants, who were exposed to smoking, had an increased risk of impaired lung function in terms of reduced FEV1 %predicted (Palmer et al., 2006a; Malling et al., 2012), FVC %predicted and FEV1/FVC ratio compared to those with GSTM1 present variants (Rogers et al., 2009). Recent studies have focused on lung function changes over a longer period, and have identified both reduced lung function growth (Owens et al., 2019) and increased lung function decline (Dai et al., 2021) for exposed participants with GSTM1 null genotypes. There appears to be more evidence for this interaction when measuring lung function as opposed to asthma even in the same participants. This may be partly explained by lung function being a continuous variable, with greater power to be detect associations when compared to categorized outcomes (asthma).
The evidence regarding an interactive effect of GSTT1 on the relationship between indoor exposures and adverse respiratory outcomes is presented in Table 4. Overall, there was less evidence for an interaction in relation to asthma outcomes (compared to GSTM1). There were only two cross-sectional studies that investigated asthma and both found significant interactions for GSTT1 in school aged children. One study found that the frequency of incense burning was associated with an increased risk of current asthma, asthma medication use, lifetime wheeze, nocturnal wheeze, and exercise wheeze in children with GSTT1 null genotypes. These associations were not seen in those with GSTT1 present genotypes (Wang et al., 2011). The second study also found evidence that GSTT1 null children had an increased risk of ever wheeze with ETS exposure, compared with GSTT1 present children not exposed to ETS. There were no associations found for GST present children with ETS exposure and GST null children without ETS exposure (Kabesch et al., 2004). A further study from Copenhagen considered an expanded number of common indoor air pollutants like the use of woodstoves and candles on wheeze outcomes, however, no evidence of an interaction was found (all interactions p > 0.2) (Hersoug et al., 2012).
TABLE 4. Influence of GSTT1 polymorphisms on the relationship between household environmental exposures and asthma and lung function.
There was more evidence for interaction on lung function compared with asthma. One study found that amongst infants exposed to tobacco smoke in utero, those with GSTT1 present had increased VFRCmax in the first year of life compared with GSTT1 null infants (interaction p = 0.037). No evidence of association was seen in the groups (GSTT1 present and absent) not exposed to in utero tobacco smoke (Murdzoska et al., 2010). Household smoking may also influence lung function changes over a longer term for those with GSTT1 null genotypes (Imboden et al., 2007; Dai et al., 2019; Dai et al., 2021). In contrast, another two cross-sectional studies conducted on adults that investigated current household air pollution exposure, found no evidence of an interaction (He et al., 2004; Imboden et al., 2007).
Evidence for a potential interactive effect of GSTP1 on the relationship between indoor exposures and adverse respiratory outcomes is presented in Table 5. Although GSTP1 is the most studied of the three mentioned GST polymorphisms, particularly in conjunction with more varied exposures (different forms of indoor air pollution), the findings with respect to risk alleles are more inconsistent. Some studies have provided evidence that GSTP1 with Val alleles is associated with increased risk of asthma/wheeze outcomes in childhood with exposure to in-utero smoking (Lee et al., 2007; Li et al., 2008; Panasevich et al., 2010; Wang et al., 2010). Another study found evidence for a three-way interaction in school-aged children with GSTP1 homozygous Ile genotypes and low vitamin A levels who had an increased risk of asthma diagnosis (OR 4.44, 95% CI 1.58, 12.52) compared to children who did not have these two risk factors (Lee et al., 2015). Other studies have found no evidence of an interaction between GSTP1 genotypes and indoor air exposures (Schroer et al., 2009; Gerbase et al., 2011; Munoz et al., 2012) and asthma. Recently, we performed a latent class analysis combining several household air pollution sources into exposure profiles. Our study found that profiles of household air pollution exposure were associated with increased risk of asthma in middle-aged adults with GSTP1 Ile/Ile genotypes; however, the associations were not found in participants with other GSTP1 genotypes (Dai et al., 2021). Though this study was again limited by small sample size, multiple exposure profiles were associated with higher respiratory risk than the individual household exposures (Dai et al., 2021). Not considering household air pollution exposures as complex mixtures or exposure phenotypes may be one reason for the lack of current evidence of an association between household air pollution (excluding smoking) and lung health outcomes.
TABLE 5. Influence of GSTP1 polymorphisms on the relationship between household environmental exposures and asthma and lung function.
Several studies have focussed on three-way interactions to take age into account. One childhood study provided evidence on the potential adverse role of GSTP1 val105 on lung function when exposed to second-hand tobacco smoke. This association was found only in older children (13–21 years vs 3–12 years) (Palmer et al., 2006b). The results were consistent with our study demonstrating that an interaction of GSTP1 with lung function was only found in participants at 18 years, not at 12 years (Dai et al., 2019). However, this study found that adolescents with GSTP1 Ile/Ile genotypes were more likely to have lower lung function (Dai et al., 2019). Gender is also a potential third factor to be considered in GSTP1 interactions. A Swiss study found that heterozygosity for GSTP1 (heterozygous Ile105Val) was associated with slower decline in FVC in female persistent smokers compared to those with homozygous Ile genotypes, while no association was observed in the female never-smokers’ group (Imboden et al., 2007). However, female smokers with GSTP1 with homozygous Val genotypes did not show an increased decline compared to those with a homozygous Ile genotype. These associations were not seen in males (Imboden et al., 2007).
This review has provided evidence that GST genes may modify the link between household air pollution exposures and asthma and lung function. However, the associations differ depending on exposure time, types, risk alleles, and study design. There was evidence that GST gene interactions were more consistent for studies conducted on children, suggesting that the effect of gene-pollution interactions on respiratory health may vary by age. There are many differences between children and adults regarding respiratory system pathophysiology and immune responses. Children are relatively naïve with respect to many body systems and may be more susceptible to noxious exposures. Consequently, oxidative capacity may be more important for children compared to adults. Furthermore, the exposures documented in childhood studies usually occur during early life when the lungs grow. Airways, alveoli and blood vessels grow rapidly up to 2 years of age and continue to expand until early adulthood, and exposures during this critical period have been found to detrimentally affect subsequent respiratory health (Dai et al., 2016). As such, my review also supports the notion that there are critical time windows for adverse exposures and the impact is higher during lung growth in early life. Asthma and lung function deficits are both conditions that develop over an extended period. Longitudinal cohorts provide better evidence than cross-sectional and case control studies for investigating these chronic conditions and, are more likely to detect associations, particularly for lung function outcomes.
Though GSTs play an important role in regulating oxidative stress, many other factors are also involved in supporting an antioxidative stress network to protect respiratory health. There are several antioxidant genes that have been identified as potentially important for lung health and have been studied including: NAD(P)H dehydrogenase [quinine] 1 (NQO1) and heme oxygenase 1 (HMOX-1) (Minelli et al., 2011). The effect of each antioxidant gene individually may be very small, hard to detect and dependent on the presence of specific environmental exposures. However, multiple interactions may alter individual susceptibility to the effects of air pollution on respiratory health (Minelli et al., 2011). One study suggested that supplemental nutrition intake, like vitamin A, could enhance antioxidant capacity and possibly prevent respiratory harm in susceptible children with risk GST genotypes exposed to ETS (Lee et al., 2015). In future, clinicians could consider antioxidant supplements as additions to personalised therapy for children found to have a high-risk genotype on genetic screening.
Findings from this review differed in terms of the specific risk alleles and exposures. This was particularly the case for GSTP1 risk alleles where the evidence was contradictory and inconsistent. It is difficult to draw a firm conclusion on GSTP1 risk alleles based on current evidence. One of the reasons for this lack of consistency could be that this polymorphism was investigated using Single Nucleotide Polymorphisms (SNPs), not haplotypes. GSTP1 has a more complex genetic variation than GSTM1 and GSTT1, and has recognized haplotypes, in terms of 4 alleles: GSTP1*A (Ile105-Ala114), GSTP1*B (Val105-Ala114), GSTP1*C (Val105-Val114) and GSTP1*D (Ile105-Val114). The included papers have generally focussed on the Ile105-Val105 substitution because the minor Ala114Val allele has a lower frequency and many of the studies had small numbers so were unable to address GSTP1 using these 4 distinct haplotypes. Most studies of GSTP1 interaction did not take into account the effect of the Ala114Val allele. However, Ala114Val may interact with Ile105Val alleles to produce heterogeneity in different scenarios [262]. Future studies investigating more comprehensive genetic variation and haplotypes of the GSTP1 genes would improve our understanding of the role of GSTP1 on respiratory health.
This review provides evidence for the presence of gene-environment interactions and highlights the potential biological mechanisms relating to the role of oxidative stress in the development and progression of respiratory disease. Many household air pollution exposures may have health effects for susceptible populations; for individuals with particular GST genotypes. Further studies should consider other exposures that may interact with GST genes to facilitate the oxidative stress pathway including nutrition, and physical activity. Personalised strategies may identify high risk people and help in targeted prevention of respiratory disease.
XD, SD and CL led the design, analysis and interpretation of data. XD wrote the initial draft of the manuscript which was critically revised for important content by all the authors. All authors approved the final version.
The authors thank the Allergy and Lung Health Unit team in the School of Population and Global Health, University of Melbourne.
SCD and CJL have received research funds from GSK’s for unrelated research.
The remaining authors declare that the research was conducted in the absence of any commercial or financial relationships that could be construed as a potential conflict of interest.
All claims expressed in this article are solely those of the authors and do not necessarily represent those of their affiliated organizations, or those of the publisher, the editors and the reviewers. Any product that may be evaluated in this article, or claim that may be made by its manufacturer, is not guaranteed or endorsed by the publisher.
Alexander, M., Karmaus, W., Holloway, J. W., Zhang, H., Roberts, G., Kurukulaaratchy, R. J., et al. (2013). “Effect of GSTM2-5 polymorphisms in relation to tobacco smoke exposures on lung function growth: A birth cohort study,” in BMC pulmonary medicine (England: BioMed Central).
Anthony A, F., Andrea, B., Michael, H., Peter W, J., Richard C, S., Monica A, S., et al. (2000). Polymorphism at the glutathione S-transferase GSTP1 locus: A new marker for bronchial hyperresponsiveness and asthma. Am. J. Respir. Crit. Care Med. 161 (5), 1437–1442. doi:10.1164/ajrccm.161.5.9903006
Breton, C. V., Vora, H., Salam, M. T., Islam, T., Wenten, M., Gauderman, W. J., et al. (2009). Variation in the GST mu locus and tobacco smoke exposure as determinants of childhood lung function. United States: American Thoracic Society.
Buratti, F. M., Darney, K., Vichi, S., Turco, L., Di Consiglio, E., Lautz, L. S., et al. (2021). Human variability in glutathione-S-transferase activities, tissue distribution and major polymorphic variants: Meta-analysis and implication for chemical risk assessment. Toxicol. Lett. 337, 78–90. doi:10.1016/j.toxlet.2020.11.007
Carr, D., Leupold, W., Weiland, S. K., and von Mutius, E. (2004). Glutathione S transferase deficiency and passive smoking increase childhood asthma. THORAX 59 (7), 569–573. doi:10.1136/thx.2003.016667
Chung, H. W. (2002). Polymorphisms of GSTM1, GSTT1, GSTP1, NA1II and CYP1A1 and the susceptibility to asthma. Genes & Genomics 24 (3), 259–266.
Dai, X., Bowatte, G., Lowe, A. J., Matheson, M. C., Gurrin, L. C., Burgess, J. A., et al. (2018). Do glutathione S-transferase genes modify the link between indoor air pollution and asthma, allergies, and lung function? A systematic review. Curr. Allergy Asthma Rep. 1, 20. doi:10.1007/s11882-018-0771-0
Dai, X., Bui, D. S., Perret, J. L., Lowe, A. J., Frith, P. A., Bowatte, G., et al. (2021). Exposure to household air pollution over 10 years is related to asthma and lung function decline. Eur. Respir. J. 57 (1), 2000602. doi:10.1183/13993003.00602-2020
Dai, X., Dharmage, S. C., Bowatte, G., Waidyatillake, N. T., Perret, J. L., Hui, J., et al. (2019). Interaction of glutathione S-transferase M1, T1, and P1 genes with early life tobacco smoke exposure on lung function in adolescents. CHEST 155 (1), 94–102. doi:10.1016/j.chest.2018.08.1079
Dai, X., Dharmage, S. C., Lowe, A. J., Allen, K. J., Thomas, P. S., Perret, J., et al. (2016). Early smoke exposure is associated with asthma and lung function deficits in adolescents. J. Asthma 54 (6), 662–669. doi:10.1080/02770903.2016.1253730
Ercan, H., Birben, E., Dizdar, E. A., Keskin, O., Karaaslan, C., Soyer, O. U., et al. (2006). Oxidative stress and genetic and epidemiologic determinants of oxidant injury in childhood asthma. J. Allergy Clin. Immunol. 118 (5), 1097–1104. doi:10.1016/j.jaci.2006.08.012
Fernando, H. (2013). Oxidative stress in airway diseases. Ann. Am. Thorac. Soc. 10, S150–S157. doi:10.1513/AnnalsATS.201305-116AW
Frank D, G., Yu-Fen, L., Louis, D., Kiros, B., Edward, A., Rob, M., et al. (2002). Effects of glutathione S-transferase M1, maternal smoking during pregnancy, and environmental tobacco smoke on asthma and wheezing in children. Am. J. Respir. Crit. Care Med. 166 (4), 457–463. doi:10.1164/rccm.2112064
Gerbase, M. W., Keidel, D., Imboden, M., Gemperli, A., Bircher, A., Schmid-Grendelmeier, P., et al. (2011). Effect modification of immunoglobulin E-mediated atopy and rhinitis by glutathione S-transferase genotypes in passive smokers. Clin. Exp. Allergy 41 (11), 1579–1586. doi:10.1111/j.1365-2222.2011.03807.x
Gilliland, F. D., Gauderman, J. W., Vora, H., Rappaport, E., and Dubeau, L. (2002). Effects of glutathione-S-transferase M1, T1, and P1 on childhood lung function growth. Am. J. Respir. Crit. Care Med. 166 (5), 710–716. doi:10.1164/rccm.2112065
Global Asthma Network, (2014). Global Asthma Network Steering Group. Auckland, New Zealand: Global Asthma Network. Available at: http://www.globalasthmareport.org/resources/Global_Asthma_Report_2014.pdf.
Hanene, C., Jihene, L., Jamel, A., Kamel, H., and Agnés, H. (2007). Association of GST genes polymorphisms with asthma in Tunisian children. Mediat. Inflamm. 2007 (1), 19564. Special section p1-6. doi:10.1155/2007/19564
Hayes, J. D., and Strange, R. C. (2000). “Glutathione S-transferase polymorphisms and their biological consequences,” in Pharmacology (Switzerland: Karger).
He, J. Q., Connett, J. E., Anthonisen, N. R., Pare, P. D., and Sandford, A. J. (2004). Glutathione S-transferase variants and their interaction with smoking on lung function. Am. J. Respir. Crit. Care Med. 170 (4), 388–394. doi:10.1164/rccm.200312-1763OC
Hersoug, L.-G., Brasch-Andersen, C., Husemoen, L. L. N., Sigsgaard, T., and Linneberg, A. (2012). The relationship of glutathione-S-transferases copy number variation and indoor air pollution to symptoms and markers of respiratory disease. Clin. Respir. J. 6 (3), 175–185. doi:10.1111/j.1752-699X.2011.00258.x
Imboden, M., Downs, S. H., Senn, O., Matyas, G., Brandli, O., Russi, E. W., et al. (2007). Glutathione S-transferase genotypes modify lung function decline in the general population: SAPALDIA cohort study. Respir. Res. 8 (2), 2. doi:10.1186/1465-9921-8-2
Imboden, M., Rochat, T., Brutsche, M., Schindler, C., Downs, S. H., Gerbase, M. W., et al. (2008). Glutathione S-transferase genotype increases risk of progression from bronchial hyperresponsiveness to asthma in adults. Thorax 63 (4), 322–328. doi:10.1136/thx.2007.085555
Iorio, A., Polimanti, R., Piacentini, S., Liumbruno, G. M., Manfellotto, D., and Fuciarelli, M. (2015). Deletion polymorphism of GSTT1 gene as protective marker for allergic rhinitis. Clin. Respir. J. 9 (4), 481–486. doi:10.1111/crj.12170
Kabesch, M., Hoefler, C., Carr, D., Leupold, W., Weiland, S. K., and von Mutius, E. (2004). Glutathione S transferase deficiency and passive smoking increase childhood asthma. Thorax 59 (7), 569–573. doi:10.1136/thx.2003.016667
Karam, R. A., Pasha, H. F., El-Shal, A. S., Rahman, H. M. A., Gad, D. M., et al. (2012). Impact of glutathione-S-transferase gene polymorphisms on enzyme activity, lung function and bronchial asthma susceptibility in Egyptian children. Gene 497 (2), 314–319. doi:10.1016/j.gene.2012.01.059
Lee, S.-Y., Kim, B.-S., Kwon, S.-O., Oh, S.-Y., Shin, H. L., Jung, Y.-H., et al. (2015). Modification of additive effect between vitamins and ETS on childhood asthma risk according to GSTP1 polymorphism: A cross -sectional study. BMC Pulm. Med. 15, 125. doi:10.1186/s12890-015-0093-0
Lee, Y. L., Lee, Y. C., and Guo, Y. L. (2007). Associations of glutathione S-transferase P1, M1, and environmental tobacco smoke with wheezing illness in school children. Allergy 62 (6), 641–647. doi:10.1111/j.1398-9995.2007.01380.x
Lenney, W., and Fryer, A. A. (2007). The genetics of asthma: Are the glutathione S-transferases serious players? Clin. Exp. Allergy 37 (8), 1124–1126. doi:10.1111/j.1365-2222.2007.02772.x
Li, Y. F., Gauderman, W. J., Conti, D. V., Lin, P. C., Avol, E., and Gilliland, F. D. (2008). Glutathione S-transferase P1, maternal smoking, and asthma in children: A haplotype-based analysis. Environ. Health Perspect. 116 (3), 409–415. doi:10.1289/ehp.10655
Malling, T. H., Sigsgaard, T., Brasch-Andersen, C., Frischknecht, L., Andersen, H. R., Kruse, T. A., et al. (2012). Genetic polymorphisms in antioxidative enzymes are associated to forced expiratory volume in 1 s (FEV1) in smokers independently of asthma. Clin. Respir. J. 6 (1), 46–55. doi:10.1111/j.1752-699X.2011.00245.x
Martos-Maldonado, M. C., Casas-Solvas, J. M., Vargas-Berenguel, A., and García-Fuentes, L. (2015). “Electrochemical detection of glutathione S-transferase: An important enzyme in the cell protective mechanism against oxidative stress,” in Advanced protocols in oxidative stress III. Editor D. Armstrong (New York, NY: Springer New York). doi:10.1007/978-1-4939-1441-8_10
Milos, J., Maria, Z., and Eva, B. (2017). Oxidative stress and bronchial asthma in children—causes or consequences? Front. Pediatr. 5, 162. doi:10.3389/fped.2017.00162
Minelli, C., and Gögele, M. (2011). The role of antioxidant gene polymorphisms in modifying the health effects of environmental exposures causing oxidative stress: A public health perspective. Free Radic. Biol. Med. 51, 925–930. doi:10.1016/j.freeradbiomed.2011.02.012
Minelli, C., Granell, R., Newson, R., Rose-Zerilli, M. J., Torrent, M., Ring, S. M., et al. (2010). Glutathione-S-transferase genes and asthma phenotypes: A human genome epidemiology (HuGE) systematic review and meta-analysis including unpublished data. Int. J. Epidemiol. 39 (2), 539–562. doi:10.1093/ije/dyp337
Minelli, C., Wei, I., Sagoo, G., Jarvis, D., Shaheen, S., and Burney, P. (2011). Interactive effects of antioxidant genes and air pollution on respiratory function and airway disease: A HuGE review. Am. J. Epidemiol. 173 (6), 603–620. doi:10.1093/aje/kwq403
Mostafa, S., Iraj, S., Zahra, S., and Ali, E. (2004). Combination of CC16, GSTM1, and GSTT1 genetic polymorphisms is associated with asthma. J. Allergy Clin. Immunol. 113 (5), 996–998. doi:10.1016/j.jaci.2004.02.007
Munoz, B., Magana, J. J., Romero-Toledo, I., Juarez-Perez, E., Lopez-Moya, A., Leyva-Garcia, N., et al. (2012). The relationship among IL-13, GSTP1, and CYP1A1 polymorphisms and environmental tobacco smoke in a population of children with asthma in Northern Mexico. Environ. Toxicol. Pharmacol. 33 (2), 226–232. doi:10.1016/j.etap.2011.12.007
Murdzoska, J., Devadason, S. G., Khoo, S. K., Landau, L. I., Young, S., Goldblatt, J., et al. (2010). In utero smoke exposure and role of maternal and infant glutathione s-transferase genes on airway responsiveness and lung function in infancy. Am. J. Respir. Crit. Care Med. 181 (1), 64–71. doi:10.1164/rccm.200812-1887OC
Nickel, R., Haider, A., Sengler, C., Lau, S., Niggemann, B., Deichmann, K. A., et al. (2005). Association study of Glutathione S-transferase P1 (GSTP1) with asthma and bronchial hyper-responsiveness in two German pediatric populations. Pediatr. Allergy Immunol. 16 (6), 539–541. doi:10.1111/j.1399-3038.2005.00307.x
Oh, J. M., Kim, S. H., Suh, C. H., Nahm, D. H., Park, H. S., Lee, Y. M., et al. (2005). Lack of association of glutathione S-transferase P1 Ile 105Val polymorphism with aspirin-intolerant asthma. Korean J. Intern. Med. 20 (3), 232–236. doi:10.3904/kjim.2005.20.3.232
Owens, L., Laing, I. A., Murdzoska, J., Zhang, G., Turner, S. W., and Le Souëf, P. N. (2019). Glutathione S -transferase genotype protects against in utero tobacco-linked lung function deficits. Am. J. Respir. Crit. Care Med. 200 (4), 462–470. doi:10.1164/rccm.201807-1332OC
Palmer, C. N. A., Doney, A. S. F., Lee, S. P., Murrie, I., Ismail, T., Macgregor, D. F., et al. (2006). Glutathione S-transferase M1 and P1 genotype, passive smoking, and peak expiratory flow in asthma. Pediatrics 118 (2), 710–716. doi:10.1542/peds.2005-3030
Palmer, C. N., Doney, A. S., Lee, S. P., Murrie, I., Ismail, T., Macgregor, D. F., et al. (2006). Glutathione S-transferase M1 and P1 genotype, passive smoking, and peak expiratory flow in asthma. Pediatrics 118 (2), 710–716. doi:10.1542/peds.2005-3030
Panasevich, S., Lindgren, C., Kere, J., Wickman, M., Pershagen, G., Nyberg, F., et al. (2010). Interaction between early maternal smoking and variants in TNF and GSTP1 in childhood wheezing. Clin. Exp. Allergy 40 (3), 458–467. doi:10.1111/j.1365-2222.2010.03452.x
Pascual, R. M., and Peters, S. P. (2005). Airway remodeling contributes to the progressive loss of lung function in asthma: An overview. J. Allergy Clin. Immunol. 116, 477–486. doi:10.1016/j.jaci.2005.07.011
Piacentini, S., Polimanti, R., Simonelli, I., Donno, S., Pasqualetti, P., Manfellotto, D., et al. (2013). Glutathione S-transferase polymorphisms, asthma susceptibility and confounding variables: A meta-analysis. Mol. Biol. Rep. 40 (4), 3299–3313. doi:10.1007/s11033-012-2405-2
Renate, N., Assja, H., Claudia, S., Susanne, L., Bodo, N., Klaus A, D., et al. (2005). Association study of Glutathione S-transferase P1 (GSTP1) with asthma and bronchial hyper-responsiveness in two German pediatric populations. Pediatr. Allergy Immunol. 16 (6), 539–541. doi:10.1111/j.1399-3038.2005.00307.x
Rogers, A. J., Brasch-Andersen, C., Ionita-Laza, I., Murphy, A., Sharma, S., Klanderman, B. J., et al. (2009). The interaction of glutathione S-transferase M1-null variants with tobacco smoke exposure and the development of childhood asthma. Clin. Exp. Allergy 39 (11), 1721–1729. doi:10.1111/j.1365-2222.2009.03372.x
Safronova, O. G., Vavilin, V. A., Lyapunova, A. A., Makarova, S. I., Lyakhovich, V. V., Kaznacheeva, L. F., et al. (2003). Relationship between glutathione S-transferase P1 polymorphism and bronchial asthma and atopic dermatitis. Bull. Exp. Biol. Med. 136 (1), 73–75. doi:10.1023/a:1026001216033
Schroer, K. T., Biagini Myers, J. M., Ryan, P. H., LeMasters, G. K., Bernstein, D. I., Villareal, M., et al. (2009). Associations between multiple environmental exposures and Glutathione S-Transferase P1 on persistent wheezing in a birth cohort. J. Pediatr. 154 (3), 401–408. doi:10.1016/j.jpeds.2008.08.040
Tamer, L., Calikoglu, M., Ates, N. A., Yildirim, H., Ercan, B., Saritas, E., et al. (2004). Glutathione-S-transferase gene polymorphisms (GSTT1, GSTM1, GSTP1) as increased risk factors for asthma. Respirology 9 (4), 493–498. doi:10.1111/j.1440-1843.2004.00657.x
Vavilin, V. A., Makarova, S. I., Lyakhovich, V. V., and Gavalov, S. M. (2002). Polymorphic genes of xenobiotic-metabolizing enzymes associated with predisposition to bronchial asthma in hereditarily burdened and nonburdened children. Russ. J. Genet. 38 (4), 439–445. doi:10.1023/a:1015214606118
Wang, I. J., Guo, Y. L., Lin, T. J., Chen, P. C., and Wu, Y. N. (2010). GSTM1, GSTP1, prenatal smoke exposure, and atopic dermatitis. Ann. Allergy Asthma Immunol. 105 (2), 124–129. doi:10.1016/j.anai.2010.04.017
Wang, I. J., Tsai, C. H., Chen, C. H., Tung, K. Y., and Lee, Y. L. (2011). Glutathione S-transferase, incense burning and asthma in children. Eur. Respir. J. 37 (6), 1371–1377. doi:10.1183/09031936.00137210
Wu, C. C., Ou, C. Y., Chang, J. C., Hsu, T. Y., Kuo, H. C., Liu, C. A., et al. (2014). Gender-dependent effect of GSTM1 genotype on childhood asthma associated with prenatal tobacco smoke exposure. Biomed. Res. Int. 2014, 769452. (Article number: 769452). doi:10.1155/2014/769452
Keywords: review, lung function, asthma, household air pollution, glutathione S-transferase
Citation: Dai X, Dharmage SC and Lodge CJ (2022) Interactions between glutathione S-transferase genes and household air pollution on asthma and lung function. Front. Mol. Biosci. 9:955193. doi: 10.3389/fmolb.2022.955193
Received: 28 May 2022; Accepted: 06 September 2022;
Published: 29 September 2022.
Edited by:
Simona Piaggi, University of Pisa, ItalyReviewed by:
Eisuke Dohi, National Center of Neurology and Psychiatry, JapanCopyright © 2022 Dai, Dharmage and Lodge. This is an open-access article distributed under the terms of the Creative Commons Attribution License (CC BY). The use, distribution or reproduction in other forums is permitted, provided the original author(s) and the copyright owner(s) are credited and that the original publication in this journal is cited, in accordance with accepted academic practice. No use, distribution or reproduction is permitted which does not comply with these terms.
*Correspondence: Caroline J. Lodge, Y2xvZGdlQHVuaW1lbGIuZWR1LmF1
Disclaimer: All claims expressed in this article are solely those of the authors and do not necessarily represent those of their affiliated organizations, or those of the publisher, the editors and the reviewers. Any product that may be evaluated in this article or claim that may be made by its manufacturer is not guaranteed or endorsed by the publisher.
Research integrity at Frontiers
Learn more about the work of our research integrity team to safeguard the quality of each article we publish.